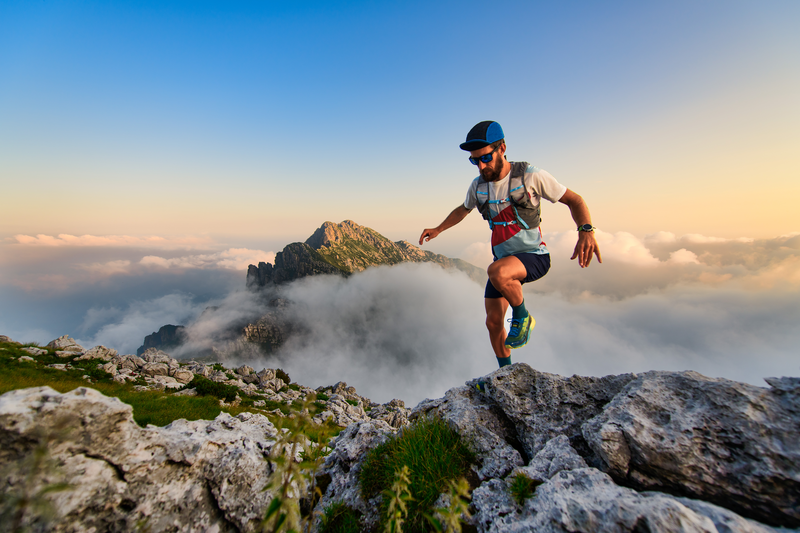
94% of researchers rate our articles as excellent or good
Learn more about the work of our research integrity team to safeguard the quality of each article we publish.
Find out more
ORIGINAL RESEARCH article
Front. Immunol. , 25 May 2022
Sec. Microbial Immunology
Volume 13 - 2022 | https://doi.org/10.3389/fimmu.2022.893611
This article is part of the Research Topic Global Excellence in Infection and Immunity: Asia and Australasia 2021 View all 3 articles
The NOD-like receptors (NLRs) have been shown to be involved in infection and autoinflammatory disease. Previously, we identified a zebrafish NLR, nlrc3-like, required for macrophage homeostasis in the brain under physiological conditions. Here, we found that a deficiency of nlrc3-like leads to decreased bacterial burden at a very early stage of Mycobacterium marinum infection, along with increased production of pro-inflammatory cytokines, such as il-1β and tnf-α. Interestingly, myeloid-lineage specific overexpression of nlrc3-like achieved the opposite effects, suggesting that the impact of nlrc3-like on the host anti-mycobacterial response is mainly due to its expression in the innate immune system. Fluorescence-activated cell sorting (FACS) and subsequent gene expression analysis demonstrated that inflammasome activation-related genes were upregulated in the infected macrophages of nlrc3-like deficient embryos. By disrupting asc, encoding apoptosis-associated speck-like protein containing a CARD, a key component for inflammasome activation, the bacterial burden increased in asc and nlrc3-like double deficient embryos compared with nlrc3-like single deficient embryos, implying the involvement of inflammasome activation in infection control. We also found extensive neutrophil infiltration in the nlrc3-like deficient larvae during infection, which was associated with comparable bacterial burden but increased tissue damage and death at a later stage that could be alleviated by administration of dexamethasone. Our findings uncovered an important role of nlrc3-like in the negative regulation of macrophage inflammasome activation and neutrophil infiltration during mycobacterial infection. This highlights the importance of a balanced innate immune response during mycobacterial infection and provides a potential molecular basis to explain how anti-inflammatory drugs can improve treatment outcomes in TB patients whose infection is accompanied by a hyperinflammatory response.
Tuberculosis (TB) remains a major threat for public health, with about 9.9 million new TB patients and 1.3 million deaths per year, according to the 2021 WHO annual TB report (1). Patients who are immunodeficient or undergoing long term immunosuppressive therapy have an increased risk of Mycobacterium tuberculosis (M.tb) infections (2, 3), but a hyperinflammatory response to infection can induce extensive tissue damage (4–7). Thus, a balanced immune response is critical for successful host control of M.tb infections.
Innate immune cells are the first line of defense against M.tb infection, and macrophages are considered to be the first and the primary cell-type infected. Macrophages not only provide shelter for the immune escape of M.tb, but also mediate immune killing (8). ASC mediated macrophage IL-1β maturation has been shown to be a vital mechanism for macrophage restriction of M.tb (9, 10), and macrophages also mediate bactericidal activity via TNF-α and ROS signaling (11–16). However, excessive production of pro-inflammatory cytokines such as TNF-α will cause accelerated mycobacterial extracellular growth and subsequent tissue damage (7, 17). Therefore, the proper regulation of macrophage functions is critical for M.tb control.
Neutrophils are the most abundant infected innate immune cell type in the airways of pulmonary TB patients (18) but whether they are beneficial or detrimental during M.tb infection remains controversial. The impact of neutrophils on mycobacterial infection seems to depend upon the specific experimental conditions in the immune system microenvironment, the stage of the infection and the animal model used for the study (19, 20). It is generally thought that the involvement of neutrophils at a very early stage of infection has a positive effect that contributes to host control of a mycobacterial infection (19–22). However, neutrophils may also play a detrimental role during M.tb infection, serving as a “Trojan horse” for bacterial dissemination and also causing tissue damage as the disease progresses (6, 23–25). Similar to macrophages, neutrophils are versatile innate immune cells that can help or hinder host control of M.tb infection and therefore require balanced regulation.
NLRs are a class of intracellular receptors that typically include a C terminal leucine-rich repeats (LRRs) receptor domain, a central nucleotide-binding domain (NBD) and an N terminal effector domain (26, 27). Several NLRs, such as CIITA, NOD2, NLRC3 and NLRP10, are important sensors or regulators of the host anti-M.tb immune response (28–31). Most NLRs, especially inflammasome NLRs, are positive regulators of the immune response (26, 27). Upon binding to their ligands, NLRs can form oligomers via central NBD self-oligomerization and then recruit downstream effector proteins ASC and caspase-1 to form inflammasomes. The activated caspase-1 then cleaves pro-IL-1β to make mature IL-1β and promotes its subsequent secretion (26).
In contrast, some mammalian NLRs, such as NLRP10 and NLRC3, function as negative regulators rather than sensors of distinct stimuli (30–37). The zebrafish nlrc3-like is also a negative regulator of the Asc mediated inflammasome pathway and required for proper microglial colonization and maintenance (38, 39). Although the negative regulator NLRC3 has been shown to be involved in the adaptive immune response against M.tb infection (30), the impact of negative regulators such as NLRC3 on the innate immune response during mycobacterial infection has not been explored.
Zebrafish are an emerging model for host-mycobacterial interactions, especially for studying the intact innate immune system present in their embryonic stage, before their adaptive immunity has developed (40, 41). Using this model, we investigated the impact of the negative regulator nlrc3-like on the innate immune response to mycobacterial infection. The bacterial burden decreased significantly during the very early stages of M. marinum infection in nlrc3-like deficient embryos, accompanied by an increased production of cytokines with bactericidal effects. Using tissue specific overexpression of nlrc3-like, disruption of Asc signaling and FACS analysis, we demonstrate that the effects of nlrc3-like deficiency on the anti-mycobacterium response are principally mediated through the activation of the inflammasome in infected macrophages. In the nlrc3-like deficient larvae there was extensive neutrophilic infiltration, accelerated late-stage tissue damage and increased death rates, but this phenotype could be alleviated by the anti-inflammatory steroid dexamethasone (DEX).
Collectively, our findings uncover an important role for nlrc3-like in the negative regulation of both neutrophil infiltration and macrophage inflammasome activation during mycobacterial infection. This study demonstrates that the successful control of mycobacterial infection requires a balanced immune response. The results also suggest a molecular explanation for the benefit of anti-inflammatory drugs in patients with forms of tuberculosis associated with a hyperinflammatory state, such as tuberculosis meningitis or the immune reconstitution inflammatory syndrome (IRIS) in HIV/tuberculosis co-infections (42, 43).
All zebrafish were raised under standard conditions in compliance with laboratory animal guidelines for the ethical review of animal welfare (GB/T 35823-2018). All zebrafish experiments were approved by the Animal Care and Use Committee of the Shanghai Public Health and Clinical Center, Fudan University (2019-A016-01 & 2021-A043-01).
Wild Type AB, nlrc3-likehkz6 (39), ascΔ31 (aschkz7) (39), Tg(mfap4:GFP)hkz020t (44) and Tg(lyz:GFP)nz117 (45) were generated and maintained as previously described. All offspring from nlrc3-likehkz6 were maintained at 32°C.
Because only nlrc3-like -/- mutants show a phenotype (39), we could separate nlrc3-like -/- embryos from their siblings (nlrc3-like +/+ and nlrc3-like +/-). Nlrc3-like -/- embryos were identified by differential interference contrast (DIC) imaging of apoptotic-body accumulation in the midbrain region at 3 days post fertilization (dpf) as previously described (39).
Wasabi and Tdtomato fluorescently labeled M strain M. marinum (ATCC BAA-535) were generated by Dr. Ramakrishnan’s group (40). Zebrafish embryo infections were performed by microinjection of M. marinum via the duct of Cuvier unless otherwise indicated (46). For live imaging and Sudan Black (SB) staining, infections were by subcutaneous injection (47). The infecting dose was 250 CFU, if not otherwise specified, but 400 CFU were used for experiments designed for analysis by FACS.
Raw264.7 (ATCC TIB-71) cells were cultured in DMEM (Biological Industries) containing 10% fetal bovine serum at 37°C and 5% CO2 with a density of 2 x 106 cells per well in 6 well plates. For infections, the plated cells were infected with single-cell suspensions of H37Rv on the day after seeding, at a multiplicity of infection (MOI) of 10:1. After allowing 4 hours for phagocytosis, infected Raw264.7 cells were washed 3 times with 1 x PBS to remove extracellular bacteria and then replenished with fresh DMEM containing 10% FBS.
The full-length coding sequence of nlrc3-like was obtained from the pTol2-coronin1a-nlrc3-like plasmid and subcloned into the pTol2-β-actin vector to generate pTol2-β-actin-nlrc3-like (39). The control plasmids pTol2-β-actin-6xmyc and pTol2-coronin1a-6xmyc were generated similarly (39). Plasmid expression in zebrafish embryos was achieved by microinjection of 2 nL per embryo of each plasmid (10 ng/μL) together with transposase mRNA (100 ng/μL) at the single-cell stage.
Fluorescently labeled bacteria were quantified by measuring the integrated fluorescent intensity with Fiji ImageJ software.
Images were processed by Fiji ImageJ software using the “Analyze Particles” function.
Time-Lapse imaging for the neutrophil recruitment to the infection site was conducted and processed as previous described (48).
FACS was performed as previously described (49). For each experimental condition, 25 embryos were collected in a 1.5 mL microtube with 0.5 mL PBS. Embryos were homogenized by 5 passages through a 22-guage needle attached to a 5 ml syringe. The resultant homogenate was centrifuged at 300g for 5 minutes at 4°C, and the pellet was dissociated with 2 mL pre-warmed TrypLE (ThermoFisher Scientific) for 20 minutes at 32°C. Cells were washed by adding 2 mL pre-cooled 2% BSA/PBS solution and collected with a 100-μm strainer (Miltenyi). The passed-through cells were collected by centrifugation at 400 g for 5 minutes at 4°C and resuspended in 200 μL 1% BSA/PBS. The samples were pre-cooled on the collecting platform of a Bectin Dickinson FCSAria instrument before sorting. Sorted samples were collected into 4 μL lysis buffer of the QIAseq FX Single Cell RNA Library Kit (QIAGEN) in a 96-well plate.
Whole zebrafish embryos were homogenized by five passages through a 27-G needle in 350 μL lysis buffer, and RNA was extracted using the RNeasy Mini Kit (QIAGEN). cDNA was synthesized from 0.5 ~ 1 μg RNA using the PrimeScript RT reagent Kit (Takara). For sorted cells, RNA extraction and cDNA preparation were performed with the QIAseq FX Single Cell RNA Library Kit (QIAGEN), according to the manufacturer’s instructions. For infected Raw264.7 cells, samples were collected at 4, 10, 24, 72 hours post infection (hpi). Cells were washed with 1x PBS and lysed in 1mL TRizol (ThermoFisher Scientific) for future RNA isolation. Quantitative RT-PCR was performed with SYBR Green Supermix (Promega) on a CFX96 Real-Time System instrument (Bio-Rad). The primers used for quantitative RT-PCR are listed in Supplementary Table 1.
Il-1β WISH was performed as previous described (48).
Two color RNA and protein staining was performed as previously reported (39). WISH staining was first developed with a TSA plus cyanine 3 kit (Akoya, NEL744001KT). Anti-GFP staining was performed with goat polyclonal anti-GFP primary antibodies (Abcam, ab6658, 1:500) and AF488 donkey anti-goat (Invitrogen, A11055, 1:500) secondary antibodies.
SB staining was performed as previously described (39).
100 μM DEX treatment was started at 1 day post infection (dpi) and continued until all larvae had died. The larvae were incubated in egg water containing 100 μM DEX for 12 hours and changed to drug free egg water for 12 hours daily.
The statistical analysis and infection methods were previously described in detail (50). Briefly, embryos were infected with M. marinum: wasabi (~ 250 CFU), and the daily number of embryos deaths was recorded.
Zebrafish embryos at 6 dpi were fixed for at least 24 hours in 4% paraformaldehyde. Paraffin embedding, serial sectioning (4~5 μm), and subsequent H&E staining or acid-fast staining were performed as previously reported (50).
Statistical analyses were performed using GraphPad Prism software. All data are shown as the mean ± SEM. Differences were analyzed with unpaired two-tailed t test for comparisons between two groups, and One-Way ANOVA for multiple groups. Statistical significance was indicated as follows: *p ≤ 0.05; **p ≤ 0.01; ***p ≤ 0.001; ns, not statistically significant (p>0.05).
It has been shown that NLRC3, a negative regulator NLR, is involved in the adaptive immune response against M.tb infection (30), but its effect on the innate immune regulation during mycobacterial infection has not been explored. To investigate whether zebrafish negative regulatory nlrc3-like is also involved in the anti-mycobacterium innate immunity, we studied a nlrc3-like mutant (40, 41) in the embryo stage, when innate immunity is intact but adaptive immunity has not yet developed. Zebrafish embryos were infected with fluorescence-labeled M. marinum, and the relative fluorescence intensity was monitored to quantify the bacterial load of each embryo (Figure 1A). Compared with the sibling controls (nlrc3-like +/+ and nlrc3-like +/-), the bacterial burden in nlrc3-like -/- embryos was significantly decreased at 1 dpi, 2 dpi and 3 dpi (Figures 1B, C). We also found that there were fewer large granuloma-like structures in the nlrc3-like mutants (Figures 1D, E).
Figure 1 nlrc3-like deficiency promotes host control of the mycobacterial proliferation during early infection. (A) Zebrafish embryo infections and bacterial fluorescence intensity. The red arrow indicates the duct of Cuvier, the site where bacteria were injected. (B) Representative images for M. marinum:Wasabi infected embryos at 3 dpi. (C) Statistics of relative green fluorescence intensity at the indicated time points taken from two independent biological replicates. (D) Process for calculating granuloma-like structure in zebrafish embryos: i original image; ii. the image after fluorescence-pixelation processing – the area of the red pixels represents the relative size of each granuloma; iii. Calculation of the area of the red pixels. (E) Graph showing the number of large, small and all granuloma-like structures in nlrc3-like -/- and control embryos. (B, D) Scale bar = 200 μm *p ≤ 0.05; **p ≤ 0.01; ns, not statistically significant (p>0.05)..
Pro-inflammatory factors are required for the host anti-mycobacterial response and we wondered whether they are upregulated in the nlrc3-like deficient mutant. nlrc3-like mRNA expression increased during the first 3 dpi and then gradually returned to the basal level at 5 dpi (Figure 2A). We found that il-1β, tnf-α and other pro-inflammatory genes were up-regulated in the nlrc3-like -/- mutant (Figure 2B). We then confirmed the upregulation of il-1β mRNA by whole mount in situ hybridization (WISH) in the nlrc3-like deficient embryos at 1 dpi (Figure 2C) and also found that a large portion of il-1β mRNA co-localized with mfap4-GFP staining (Figure 2D), suggesting that macrophages are an important source for il-1β during mycobacterial infection.
Figure 2 nlrc3-like deficiency upregulates the expression of inflammatory genes during mycobacterial infection. (A) Quantitative RT-PCR showing expression of nlrc3-like in 3 dpf zebrafish embryos at 0, 1, 3, 5 and 7 days post infection with M. marinum:Wasabi. (B) Quantitative RT-PCR showing expression of multiple inflammatory genes at 1 dpi. The values shown represent an average of three independent biological experiments, 25 embryos per group in each experiment. (C) il-1β WISH shows significantly increased il-1β expression in nlrc3-like deficient mutants compared with their siblings at 1 dpi. Scale bar = 200 μm. (D) Co-staining of il-1β WISH (red) and anti-GFP antibody (green). White arrows point to co-staining signals of mfap4-GFP and il-1β, and white arrow heads point to the il-1β only signals. Scale bar = 50 μm **p ≤ 0.01; ***p ≤ 0.001; ns, not statistically significant (p>0.05).
While nlrc3-like -/- embryos had a reduced bacterial burden at early time points post infection, overexpression of nlrc3-like produced a significantly increased bacterial burden, as measured by bacterial fluorescence intensity (Figures 3A, B; Supplementary Figure 1A). To investigate whether the action of nlrc3-like during mycobacterial infection was mediated by innate immune cells or non-immune cells, we generated zebrafish that overexpressed nlrc3-like only in myeloid cells. The increased bacterial fluorescence showed that the myeloid cell specific overexpression of nlrc3-like resulted in an elevated bacteria burden, an increased number of large granuloma-like structures and reduced survival, similar to the effects seen in embryos with whole-body overexpression of nlrc3-like (Figures 3C-E; Supplementary 1B). These results suggest that the impact of nlrc3-like on mycobacterial proliferation in vivo is principally mediated via its expression in innate immune cells.
Figure 3 The impact of nlrc3-like on host defense against mycobacterial infection was mainly mediated by innate immune cells. (A) Schematic view of the construction of nlrc3-like overexpressing embryos and the M. marinum infection assay. pTol2 plasmids were injected at the single-cell stage and subsequently the grown embryos were infected via the duct of Cuvier at 3 dpf. Injections of pTol2-β-actin-nlrc3-like (β-actin-nlrc3l) and pTol2-coronin1a-nlrc3-like (coro1a-nlrc3l) generated embryos overexpressing nlrc3-like in all cells or only in myeloid cells, respectively. Black arrows indicate injection sites. (B) Graph of the relative green fluorescence intensity of the β-actin-nlrc3l group and the β-actin-6xmyc group at the indicated time points. (C) Graph of the relative green fluorescence intensity of the coro1a-nlrc3l and coro1a-6xmyc embryos at the indicated time points. (D) Graph showing the number of granuloma-like structures in the coro1a-nlrc3l and the coro1a-6xmyc embryos. (E) Survival curve of coro1a-nlrc3l and coro1a-6xmyc embryos. The data shown represents the averages from two independent biological replicates *p ≤ 0.05; **p ≤ 0.01; ***p ≤ 0.001; ns, not statistically significant (p>0.05).
Next, we explored how nlrc3-like acts on innate immune cells during mycobacterial infection. Because macrophages are the primary immune cell phagocytizing mycobacteria in vivo, we used FACS to collect macrophages containing phagocytosed fluorescent mycobacteria for gene expression analysis (Figure 4A). Through continuous observation we found that the number of infected macrophages peaked at approximately 10 hpi and therefore chose this time point for FACS analysis of infected macrophages (Figures 4B, C). Similar to the results with whole embryos, at 10 hpi the macrophages of nlrc3-like -/- mutants showed significantly increased expression of several inflammasome priming related genes such as il-1β and il-6 (Figure 4D). We then evaluated the expression of these pro-inflammatory factors in embryos with myeloid specific overexpression of nlrc3-like, and as expected, found dramatically decreased expression of pro-inflammatory genes (Figure 4E).
Figure 4 nlrc3-like deficiency promotes the activation of macrophage inflammasome. (A) Schematic view of FACS of mfap4-GFP+ macrophages with phagocytosed Tdtomato+ M. marinum and subsequent detection of the expression of inflammation-related genes by RT-PCR. (B) A representative image of zebrafish embryos infected with M. marinum:Tdtomato at 10 hpi. i uninfected Tg (mfap4-GFP) embryo; ii. Tg (mfap4-GFP) embryo infected with M. marinum:Tdtomato; iii. Enlarged view of caudal hematopoietic tissue (CHT) region in ii. white arrows indicate macrophages with phagocytosed bacteria; white triangles indicate macrophages without phagocytosed bacteria; white asterisks, free M. marinum. (C) Gating strategy for FACS. i: Sample: Tg(mfap4: GFP) embryos infected with M. marinum:Tdtomato; ii. uninfected wild-type zebrafish; iii. Tdtomato single color control, wild-type zebrafish infected with M. marinum:Tdtomato; iv. GFP single color control, uninfected Tg(mfap4: GFP). P1, free M. marinum:Tdtomato; P2, mfap4-GFP+ macrophages; P3, mfap4-GFP+ macrophages with phagocytosed M. marinum:Tdtomato. (D) Expression of pro-inflammatory genes in macrophages at 10 hpi. The values represent the average of three independent biological repeats, 25 embryos per group in each repeat. (E) The expression of pro-inflammatory genes in embryos with overexpressing nlrc3-like in myeloid cells. (F) Representative images of M. marinum:wasabi proliferation in nlrc3-like -/-, asc -/- nlrc3-like -/-, and their nonmutant siblings. (G) Graph of green fluorescence intensity in nlrc3-like -/-, asc -/- nlrc3-like -/-, and their nonmutant siblings at the indicated time points. The values shown are the combined results of two independent experimental replicates. (B, F) scale bar = 200 μm *p ≤ 0.05; **p ≤ 0.01; ***p ≤ 0.001; ns, not statistically significant (p>0.05).
Full activation of the inflammasome in macrophages requires both priming (signal 1) and activation of the inflammasome complex assembly (signal 2) (51). Therefore, if nlrc3-like deficiency causes the inflammasome activation, there should be an increased in mature Il-1β protein. However, due to the limited antibody resources in zebrafish, it was not possible to detect and measure the cleaved form of Il-1β. Instead, we disrupted the inflammasome activation required adaptor gene asc and found that the decreased bacterial burden seen in the nlrc3-like deficiency embryos was reversed in nlrc3-like and asc double mutants (Figures 4F, G). This suggested that the absence of nlrc3-like promotes the early control of mycobacterial proliferation via the activation of the asc mediated macrophage inflammasome pathway.
Unexpectedly, despite the lower bacterial burden in nlrc3-like -/- embryos during early infection, their survival curve was slightly but significantly worse than that of the nonmutant embryos after infection, of which very few died in the uninfected group (Figure 5A and data not shown). Neutrophils that are recruited soon after infection have bactericidal activity (6, 19, 21). Recruitment of neutrophils to the site of infection peaked at 5 hpi (Figure 5B), and live imaging in vivo revealed a more intense neutrophil infiltration in nlrc3-like -/- embryos (Figure 5C; Supplementary Video 1), as confirmed by SB staining (Figures 5D, E). In order to clarify whether the increased neutrophilic infiltration was mediated by immune or non-immune cells, we compared the recruitment of neutrophils in embryos with whole body overexpression of nlrc3-like to embryos in which only the myeloid cells overexpressed nlrc3-like. We found that the neutrophil recruitment was significantly decreased in both groups of embryos (Figures 5F, G), suggesting that, as with the increased bacterial burden, this effect of overexpressed nlrc3-like is mediated principally through its expression in myeloid cells. Taken together, these results suggest that the early restriction of mycobacterial proliferation in the setting of nlrc3-like deficiency may be partly due to the increased neutrophil recruitment to the site of infection.
Figure 5 nlrc3-like deficiency promotes neutrophil recruitment. (A) Survival curves of nlrc3-like -/- and non-mutant embryos after M. marinum infection, based on results from two independent biological replicates. (B) Numbers of neutrophils recruited to infection sites at the indicated time points. (C) Live imaging of neutrophils after M. marinum infection. 3 dpf Tg (lyz-GFP) zebrafish embryos were infected with M. marinum:Tdtomato. Time interval, 1 min 30 s. The data is representative of three independent biological replicates. (D) SB staining showing neutrophil recruitment to infection sites. The left panel shows the whole embryo while the right panel shows magnification of the area in the red box used for counting neutrophils. (E) Graph of neutrophils counted in (D, F) Graph of the neutrophils counted after SB staining of β-actin-nlrc3l (whole body expression of nlrc3-like) and β-actin-6Xmyc embryos. (G) Graph of neutrophils counted after SB staining in coro1a-nlrc3l (myeloid expression of nlrc3-like) and coro1a-6Xmyc embryos (C, D). Scale bar = 50 μm *p ≤ 0.05; **p ≤ 0.01; ***p ≤ 0.001.
We then examined the effects of nlrc3-like deficiency on the later stages of infection. Compared to the sibling control, there was a significantly increased immune cell infiltration, especially of neutrophils, in the caudal vein plexus in nlrc3-like -/- embryos (Figures 6A-C). However, the bacterial burdens were comparable between nlrc3-like -/- embryos and their siblings, as measured by acid-fast staining and bacterial fluorescence (Figures 6D, E). The expression of the pro-inflammatory nos2a mRNA was greatly increased (Figure 6F). Interestingly, the increased NO signaling was coupled with decreased expression of pro-inflammatory cytokines il-1β and il-6 (Figure 6F). A similar expression pattern of inflammatory genes was also observed in H37Rv infected Raw264.7 macrophages (Supplementary Figure 2).
Figure 6 nlrc3-like deficiency leads to excessive neutrophils infiltration and tissue damage at later stages of infection. (A) SB staining of the tail region in different groups of zebrafish embryos. (B) H&E staining of paraffin sections of the caudal vein plexus. (C) Quantification of the number of infiltrated immune cells in (B, D) Acid fast staining of paraffin sections in the caudal vein plexus. (E) Statistics of normalized bacterial fluorescence intensity at late stage of infection. (F) Quantitative RT-PCR assessed the expression of multiple cytokines at a late stage of infection (6 dpi). The values are averages from three independent biological repeats, 25 embryos per group in each repeat. (G) Survival curves of infected zebrafish with DEX treatment. The data is from one of two similar independent biological replicates (H). SB staining showing neutrophil recruitment to infection sites at 5 hpi. The left panel shows the whole embryo while the right panel shows an enlargement of the region in the red box that was used for counting neutrophils. (I) Graph showing the number of neutrophils, counted as in (H). The data shown was combined from three independent biological repeats. (A, B, D, H) Scale bar = 50 μm *p ≤ 0.05; **p ≤ 0.01; ***p ≤ 0.001; ns, not statistically significant (p>0.05).
To separate the beneficial, antibacterial effects of nlrc3-like deficiency from the destructive effects of hyperinflammation, we administered the anti-inflammatory corticosteroid DEX beginning at 1 dpi. The nlrc3-like -/- embryos treated with DEX survived significantly longer than their non-mutant siblings given DEX (Figure 6G). Although the neutrophilic infiltration and tissue damage were decreased in both groups, the reduction was greater in the nlrc3-like -/- mutants, thereby eliminating any statistical difference between the two groups (Figures 6A, C). A similar phenotype was also noted in the neutrophil recruitment assay using subcutaneous infection (Figures 6H, I). Together, these results suggest the detrimental, tissue damaging effects of nlrc3-like deficiency on the later stages of mycobacterial infection are most likely due to the excessive immune cell infiltration rather than increased bacterial burden.
A balanced immune response is critical for successful host control of mycobacterial infections. An insufficient immune response will be unable to limit the infection while an excessive immune response will cause tissue damage and bacterial dissemination (7, 52, 53). NLRs are a class of intracellular receptors, the majority of which function by detecting external stimuli and activating downstream inflammasome pathways (26). Several NLRs, however, appear to play a regulatory function in autoimmune and infectious diseases by mediating immune homeostasis (26). Compared with inflammasome NLRs, the role of regulatory NLRs in mycobacterial infections has not been well studied, and their possible involvement in adaptive immune regulation has been merely implied (30, 31). To learn about how regulatory NLRs affect innate immune cells involved in the host anti-mycobacterial response, we used M. marinum infections of zebrafish embryos as a model. Zebrafish embryos are useful because they have intact innate immunity but have not yet developed an adaptive immune system. By studying the embryos of zebrafish lacking nlrc3-like, a negative regulatory NLR, we could show that it is required for the regulation of innate immunity during mycobacterial infection. Negative regulatory NLRs could also play a key role in the innate immune response to tuberculosis infections in humans, although the specific details may be different.
While cytokines with bactericidal effects are essential for host control of mycobacterial infections, and defects in cytokines such as IL-1β or TNF-α can lead to the death of infected individuals (9, 52, 53), excessive production of these same cytokines is detrimental to the host (7, 17, 52). In this study, the nlrc3-like defect was associated with increased expression of pro-inflammatory cytokines and reduced bacterial proliferation during the early stages of infection (Figure 7). At later stages of infection, however, the nlrc3-like -/- embryos showed uncontrolled immune cell infiltration and increased tissue damage (Figure 7).
Figure 7 Model for the impact of negative regulator NLR nlrc3-like on innate immune responses during mycobacterial infection. During the early stage of infection, the defect of nlrc3-like can boost the expression of mycobacterial elicited pro-inflammatory genes via Asc mediated inflammasome activation in infected macrophages, which results in a bacterial killing effect accompanied by an increased neutrophil recruitment. However, during the late stage of infection the host shifts into a hyperinflammatory status with uncontrolled excessive neutrophil infiltration and increased tissue damage and death. The administration of DEX can block the excessive inflammatory response and improve disease outcome.
The tissue damage may have been the result of the increased expression of nos2a mRNA (54). High levels of Nos2a could result in increased nitric oxide (NO) production, leading to enhanced bactericidal activity and increased tissue damage in nlrc3-like -/- mutant embryos (54–56). This would explain how a lower bacterial burden in nlrc3-like -/- embryos at early stage is associated with reduced long-term survival. Interestingly, the increased nos2a mRNA was coupled with a decreased expression of pro-inflammatory cytokines il-1β and il-6 (Figure 6D), perhaps because increased NO in the mutant damped the activation of inflammasome as has been previously described (5, 56). Similar expression patterns of inflammatory genes were also observed in the H37Rv infected Raw264.7 macrophages (Supplementary Figure 2). Thus, it appears that the negative regulatory protein Nlrc3-like is indispensable for controlling the inflammatory response and thereby limiting tissue damage that can occur at late stages of mycobacterial infections.
Mycobacterial infections activate macrophage inflammasome components such as NLRP3 and AIM2 (10, 57), which require ASC as a key adaptor molecule for the successful initiation of the host anti-pathogen response. Without tight regulation however, this can cause excessive inflammation and collateral tissue damage (5). In this study, we demonstrated that nlrc3-like deficiency leads to uncontrolled Asc dependent inflammasome activation. Interestingly, we also noted that several inflammasome-independent genes were also elevated, suggesting the negative regulating role of nlrc3-like seem to be non-specific. In mammals, several NLRs, such as NLRP10 and NLRC3, function as negative regulators to attenuate inflammatory signaling (26, 30, 31, 34, 35), and NLRC3 has recently been reported to function as a negative regulator for CD4+ T cell activation during M.tb infection (30). In addition to its regulation of the adaptive immune response, NLRC3 has also been implicated in damping innate immune signaling after challenges with LPS and herpes simplex virus 1 (36, 37). While both the zebrafish Nlrc3-like and the mammalian NLRC3 contain a NACHT domain, the zebrafish protein lacks the leucine rich repeats. In spite of this difference, it would be interesting to see if mammalian NLRC3 functions in a similar manner to negatively regulate the innate immune response during M.tb infection.
Neutrophil infiltration during mycobacterial infection is a double edged sword (19). At early stages of infection we observed significantly increased neutrophil infiltration and significantly decreased bacterial burden in nlrc3-like deficient embryos. This might be the result of the increased il-1β production during the early infection, as il-1β has been shown to be a potent stimulus for neutrophil recruitment (48, 58). Another possibility is that the elevated tnf-α expression leads to macrophage lysis, and the intracellular material released by lytic cell death functions as a neutrophil attractant (39, 47). This is consistent with a recent study showing that macrophage pyroptosis is beneficial for host control of mycobacterial infection (59).
However, while the excessive immune cell infiltration seen in nlrc3-like deficient embryos may help control the infection at early stages, the bacterial burdens do not show significant difference at late stages. The excessive immune cell infiltration also leads to tissue damage at later stages that was ameliorated by the application of DEX. These data suggest the initial control of infection is accomplished by increased inflammation but too much inflammation eventually leads to a relatively attenuated control that allows the lower early bacterial burden to catch up at later stages. Although the relative lower survival of nlrc3-like -/- mutant embryos after M. marinum infection was reverted by the application of DEX, both treated groups had worse survival curves than untreated WT embryos. This suggests that DEX can ameliorate the hyperinflammation induced tissue damage during mycobacterial infection but also has detrimental side effects, although these that might be reduced by optimizing the dose and timing of the drug. One reason that the role of neutrophils in mycobacterial infections remains controversial is because contradictory data has been generated from studies in different models systems, such as human, mouse and zebrafish (19–21, 23). In our system, we observed both protective and detrimental effects, which suggest that the role of neutrophils during mycobacterial infection may depend upon the specific experimental conditions, such as the stage of infection and the host immune status.
Collectively, our results show that NLR Nlrc3-like regulates the host anti-mycobacterial response by limiting macrophage activation and neutrophil infiltration, thus highlighting that the successful control of mycobacterial infection requires a balanced immune response. Further experiments are needed to define all the components of the immune system that are regulated by Nlrc3-like during mycobacterial infection, but our findings suggest that the role of negative NLR regulators in innate immune regulation in human TB warrants investigation. The results also provide a molecular explanation for the beneficial effect of anti-inflammatory drugs in patients whose M. tb infection is associated with a hyperinflammatory state, such as seen in tuberculosis meningitis and the IRIS in HIV/tuberculosis co-infections.
The original contributions presented in the study are included in the article/Supplementary Material. Further inquiries can be directed to the corresponding authors.
All zebrafish were raised under standard conditions in compliance with laboratory animal guidelines for the ethical review of animal welfare (GB/T 35823-2018). All zebrafish experiments were reviewed and approved by the Animal Care and Use Committee of the Shanghai Public Health Clinical Center, Fudan University (2019-A016-01 & 2021-A043-01).
Conceptualization: HT, QG, and BY. Data curation: LN, GL, and RL. Formal analysis: LN, GL, RL, CQ, DW, and SS. Funding acquisition: QG and BY. Investigation: LN, GL, RL, CQ, JY, LX, KZ, YT, and BY. Methodology: LN, GL, DW, SS, and BY. Project administration: QG and BY. Resources: K-WW, XF, QG, and BY. Supervision: QG and BY. Validation: LN and GL. Visualization: LN, GL, and BY. Writing - original draft: LN, GL, and BY. Writing - review and editing: all co-authors. All authors contributed to the article and approved the submitted version.
This work was supported by the National Natural Science Foundation of China (81801977 to B.Y.; 81661128043, 81871625 to Q.G.), Shanghai Key Clinical Specialty Construction Project (Tuberculosis) (shslczdzk03002), the Technology Service Platform for Detecting High level Biological Safety Pathogenic Microorganism Supported by Shanghai Science and Technology Commission (21DZ2291300), Shanghai Public Health Clinical Center Intramural Research Funding (KY-GW-2022-01), the Outstanding Youth Training Program of Shanghai Municipal Health Commission (2018YQ54 to B.Y.), and the Shanghai Sailing Program (18YF1420400 to B.Y.).
The authors declare that the research was conducted in the absence of any commercial or financial relationships that could be construed as a potential conflict of interest.
All claims expressed in this article are solely those of the authors and do not necessarily represent those of their affiliated organizations, or those of the publisher, the editors and the reviewers. Any product that may be evaluated in this article, or claim that may be made by its manufacturer, is not guaranteed or endorsed by the publisher.
We thank Dr. Z. Wen for sharing the zebrafish strains, Dr. L. Ramakrishnan for sharing M. marinum strains, Ding Zhang for helps and technical suggestions for histology.
The Supplementary Material for this article can be found online at: https://www.frontiersin.org/articles/10.3389/fimmu.2022.893611/full#supplementary-material
1. WHO. Global Tuberculosis Report 2021. (2021). Geneva, Switzerland: World Health Organization (WHO).
2. Boisson-Dupuis S, Bustamante J, El-Baghdadi J, Camcioglu Y, Parvaneh N, El Azbaoui S, et al. Inherited and Acquired Immunodeficiencies Underlying Tuberculosis in Childhood. Immunol Rev (2015) 264:103–20. doi: 10.1111/imr.12272
3. Brode SK, Jamieson FB, Ng R, Campitelli MA, Kwong JC, Paterson JM, et al. Increased Risk of Mycobacterial Infections Associated With Anti-Rheumatic Medications. Thorax (2015) 70:677–82. doi: 10.1136/thoraxjnl-2014-206470
4. Nunes-Alves C, Booty MG, Carpenter SM, Jayaraman P, Rothchild AC, Behar SM. In Search of a New Paradigm for Protective Immunity to TB. Nat Rev Microbiol (2014) 12:289–99. doi: 10.1038/nrmicro3230
5. Mishra BB, Rathinam VA, Martens GW, Martinot AJ, Kornfeld H, Fitzgerald KA, et al. Nitric Oxide Controls the Immunopathology of Tuberculosis by Inhibiting NLRP3 Inflammasome-Dependent Processing of IL-1beta. Nat Immunol (2013) 14:52–60. doi: 10.1038/ni.2474
6. Mishra BB, Lovewell RR, Olive AJ, Zhang G, Wang W, Eugenin E, et al. Nitric Oxide Prevents a Pathogen-Permissive Granulocytic Inflammation During Tuberculosis. Nat Microbiol (2017) 2:17072. doi: 10.1038/nmicrobiol.2017.72
7. Tobin DM, Vary JC Jr., Ray JP, Walsh GS, Dunstan SJ, Bang ND, et al. The Lta4h Locus Modulates Susceptibility to Mycobacterial Infection in Zebrafish and Humans. Cell (2010) 140:717–30. doi: 10.1016/j.cell.2010.02.013
8. Russell DG, Barry CE 3rd, Flynn JL. Tuberculosis: What We Don't Know can, and Does, Hurt Us. Science (2010) 328:852–6. doi: 10.1126/science.1184784
9. Mayer-Barber KD, Barber DL, Shenderov K, White SD, Wilson MS, Cheever A, et al. Caspase-1 Independent IL-1beta Production is Critical for Host Resistance to Mycobacterium Tuberculosis and Does Not Require TLR Signaling In Vivo. J Immunol (2010) 184:3326–30. doi: 10.4049/jimmunol.0904189
10. Dorhoi A, Nouailles G, Jorg S, Hagens K, Heinemann E, Pradl L, et al. Activation of the NLRP3 Inflammasome by Mycobacterium Tuberculosis is Uncoupled From Susceptibility to Active Tuberculosis. Eur J Immunol (2012) 42:374–84. doi: 10.1002/eji.201141548
11. Kaufmann SH. Protection Against Tuberculosis: Cytokines, T Cells, and Macrophages. Ann Rheum Dis (2002) 61 Suppl 2:ii54–8. doi: 10.1136/ard.61.suppl_2.ii54
12. Domingo-Gonzalez R, Prince O, Cooper A, Khader SA. Cytokines and Chemokines in Mycobacterium Tuberculosis Infection. Microbiol Spectr (2016) 4(5):10.1128 . doi: 10.1128/microbiolspec.TBTB2-0018-2016
13. Mayer-Barber KD, Yan B. Clash of the Cytokine Titans: Counter-Regulation of Interleukin-1 and Type I Interferon-Mediated Inflammatory Responses. Cell Mol Immunol (2017) 14:22–35. doi: 10.1038/cmi.2016.25
14. Cooper AM, Mayer-Barber KD, Sher A. Role of Innate Cytokines in Mycobacterial Infection. Mucosal Immunol (2011) 4:252–60. doi: 10.1038/mi.2011.13
15. Flesch IE, Kaufmann SH. Role of Cytokines in Tuberculosis. Immunobiology (1993) 189:316–39. doi: 10.1016/S0171-2985(11)80364-5
16. Guirado E, Schlesinger LS, Kaplan G. Macrophages in Tuberculosis: Friend or Foe. Semin Immunopathol (2013) 35:563–83. doi: 10.1007/s00281-013-0388-2
17. Tobin DM, Roca FJ, Oh SF, McFarland R, Vickery TW, Ray JP, et al. Host Genotype-Specific Therapies can Optimize the Inflammatory Response to Mycobacterial Infections. Cell (2012) 148:434–46. doi: 10.1016/j.cell.2011.12.023
18. Eum SY, Kong JH, Hong MS, Lee YJ, Kim JH, Hwang SH, et al. 3rd, Neutrophils are the Predominant Infected Phagocytic Cells in the Airways of Patients With Active Pulmonary TB. Chest (2010) 137:122–8. doi: 10.1378/chest.09-0903
19. Lowe DM, Redford PS, Wilkinson RJ, O'Garra A, Martineau AR. Neutrophils in Tuberculosis: Friend or Foe? Trends Immunol (2012) 33:14–25. doi: 10.1016/j.it.2011.10.003
20. Mayer-Barber KD, Barber DL. Innate and Adaptive Cellular Immune Responses to Mycobacterium Tuberculosis Infection. Cold Spring Harb Perspect Med (2015) 5(12):a018424. doi: 10.1101/cshperspect.a018424
21. Yang CT, Cambier CJ, Davis JM, Hall CJ, Crosier PS, Ramakrishnan L. Neutrophils Exert Protection in the Early Tuberculous Granuloma by Oxidative Killing of Mycobacteria Phagocytosed From Infected Macrophages. Cell Host Microbe (2012) 12:301–12. doi: 10.1016/j.chom.2012.07.009
22. Seiler P, Aichele P, Raupach B, Odermatt B, Steinhoff U, Kaufmann SH. Rapid Neutrophil Response Controls Fast-Replicating Intracellular Bacteria But Not Slow-Replicating Mycobacterium Tuberculosis. J Infect Dis (2000) 181:671–80. doi: 10.1086/315278
23. Dallenga T, Repnik U, Corleis B, Eich J, Reimer R, Griffiths GW, et al. M. Tuberculosis-Induced Necrosis of Infected Neutrophils Promotes Bacterial Growth Following Phagocytosis by Macrophages. Cell Host Microbe (2017) 22:519–30.e3. doi: 10.1016/j.chom.2017.09.003
24. Lovewell RR, Baer CE, Mishra BB, Smith CM, Sassetti CM. Granulocytes Act as a Niche for Mycobacterium Tuberculosis Growth. Mucosal Immunol (2021) 14:229–41. doi: 10.1038/s41385-020-0300-z
25. Abadie V, Badell E, Douillard P, Ensergueix D, Leenen PJ, Tanguy M, et al. Neutrophils Rapidly Migrate via Lymphatics After Mycobacterium Bovis BCG Intradermal Vaccination and Shuttle Live Bacilli to the Draining Lymph Nodes. Blood (2005) 106:1843–50. doi: 10.1182/blood-2005-03-1281
26. Davis BK, Wen H, Ting JP. The Inflammasome NLRs in Immunity, Inflammation, and Associated Diseases. Annu Rev Immunol (2011) 29:707–35. doi: 10.1146/annurev-immunol-031210-101405
27. Chen G, Shaw MH, Kim YG, Nunez G. NOD-Like Receptors: Role in Innate Immunity and Inflammatory Disease. Annu Rev Pathol (2009) 4:365–98. doi: 10.1146/annurev.pathol.4.110807.092239
28. Repique CJ, Li A, Brickey WJ, Ting JP, Collins FM, Morris SL. Susceptibility of Mice Deficient in the MHC Class II Transactivator to Infection With Mycobacterium Tuberculosis. Scand J Immunol (2003) 58:15–22. doi: 10.1046/j.1365-3083.2003.01266.x
29. Divangahi M, Mostowy S, Coulombe F, Kozak R, Guillot L, Veyrier F, et al. NOD2-Deficient Mice Have Impaired Resistance to Mycobacterium Tuberculosis Infection Through Defective Innate and Adaptive Immunity. J Immunol (2008) 181:7157–65. doi: 10.4049/jimmunol.181.10.7157
30. Hu S, Du X, Huang Y, Fu Y, Yang Y, Zhan X, et al. NLRC3 Negatively Regulates CD4+ T Cells and Impacts Protective Immunity During Mycobacterium Tuberculosis Infection. PLoS Pathog (2018) 14:e1007266. doi: 10.1371/journal.ppat.1007266
31. Vacca M, Bohme J, Zambetti LP, Khameneh HJ, Paleja BS, Laudisi F, et al. NLRP10 Enhances CD4(+) T-Cell-Mediated IFNgamma Response via Regulation of Dendritic Cell-Derived IL-12 Release. Front Immunol (2017) 8:1462. doi: 10.3389/fimmu.2017.01462
32. Uchimura T, Oyama Y, Deng M, Guo H, Wilson JE, Rampanelli E, et al. The Innate Immune Sensor NLRC3 Acts as a Rheostat That Fine-Tunes T Cell Responses in Infection and Autoimmunity. Immunity (2018) 49:1049–61.e6. doi: 10.1016/j.immuni.2018.10.008
33. Li X, Deng M, Petrucelli AS, Zhu C, Mo J, Zhang L, et al. Viral DNA Binding to NLRC3, an Inhibitory Nucleic Acid Sensor, Unleashes STING, a Cyclic Dinucleotide Receptor That Activates Type I Interferon. Immunity (2019) 50:591–9.e6. doi: 10.1016/j.immuni.2019.02.009
34. Clay GM, Valadares DG, Graff JW, Ulland TK, Davis RE, Scorza BM, et al. An Anti-Inflammatory Role for NLRP10 in Murine Cutaneous Leishmaniasis. J Immunol (2017) 199:2823–33. doi: 10.4049/jimmunol.1500832
35. Imamura R, Wang Y, Kinoshita T, Suzuki M, Noda T, Sagara J, et al. Anti-Inflammatory Activity of PYNOD and its Mechanism in Humans and Mice. J Immunol (2010) 184:5874–84. doi: 10.4049/jimmunol.0900779
36. Schneider M, Zimmermann AG, Roberts RA, Zhang L, Swanson KV, Wen H, et al. The Innate Immune Sensor NLRC3 Attenuates Toll-Like Receptor Signaling via Modification of the Signaling Adaptor TRAF6 and Transcription Factor NF-Kappab. Nat Immunol (2012) 13:823–31. doi: 10.1038/ni.2378
37. Zhang L, Mo J, Swanson KV, Wen H, Petrucelli A, Gregory SM, et al. NLRC3, a Member of the NLR Family of Proteins, is a Negative Regulator of Innate Immune Signaling Induced by the DNA Sensor STING. Immunity (2014) 40:329–41. doi: 10.1016/j.immuni.2014.01.010
38. Shiau CE, Monk KR, Joo W, Talbot WS. An Anti-Inflammatory NOD-Like Receptor is Required for Microglia Development. Cell Rep (2013) 5:1342–52. doi: 10.1016/j.celrep.2013.11.004
39. Wang T, Yan B, Lou L, Lin X, Yu T, Wu S, et al. Nlrc3-Like is Required for Microglia Maintenance in Zebrafish. J Genet Genomics (2019) 46:291–9. doi: 10.1016/j.jgg.2019.06.002
40. Takaki K, Davis JM, Winglee K, Ramakrishnan L. Evaluation of the Pathogenesis and Treatment of Mycobacterium Marinum Infection in Zebrafish. Nat Protoc (2013) 8:1114–24. doi: 10.1038/nprot.2013.068
41. Meijer AH, Spaink HP. Host-Pathogen Interactions Made Transparent With the Zebrafish Model. Curr Drug Targets (2011) 12:1000–17. doi: 10.2174/138945011795677809
42. Thwaites GE, Nguyen DB, Nguyen HD, Hoang TQ, Do TT, Nguyen TC, et al. Dexamethasone for the Treatment of Tuberculous Meningitis in Adolescents and Adults. N Engl J Med (2004) 351:1741–51. doi: 10.1056/NEJMoa040573
43. Meintjes G, Skolimowska KH, Wilkinson KA, Matthews K, Tadokera R, Conesa-Botella A, et al. Corticosteroid-Modulated Immune Activation in the Tuberculosis Immune Reconstitution Inflammatory Syndrome. Am J Respir Crit Care Med (2012) 186:369–77. doi: 10.1164/rccm.201201-0094OC
44. Wang Z, Lin L, Chen W, Zheng X, Zhang Y, Liu Q, et al. Neutrophil Plays Critical Role During Edwardsiella Piscicida Immersion Infection in Zebrafish Larvae. Fish Shellfish Immunol (2019) 87:565–72. doi: 10.1016/j.fsi.2019.02.008
45. Hall C, Flores MV, Storm T, Crosier K, Crosier P. The Zebrafish Lysozyme C Promoter Drives Myeloid-Specific Expression in Transgenic Fish. BMC Dev Biol (2007) 7:42. doi: 10.1186/1471-213X-7-42
46. Wu X, Wu Y, Zheng R, Tang F, Qin L, Lai D, et al. Sensing of Mycobacterial Arabinogalactan by Galectin-9 Exacerbates Mycobacterial Infection. EMBO Rep (2021) 22:e51678. doi: 10.15252/embr.202051678
47. Niu L, Wang C, Zhang K, Kang M, Liang R, Zhang X, et al. Visualization of Macrophage Lytic Cell Death During Mycobacterial Infection in Zebrafish Embryos via Intravital Microscopy. J Vis Exp (2019), 143. doi: 10.3791/60698
48. Yan B, Han P, Pan L, Lu W, Xiong J, Zhang M, et al. IL-1beta and Reactive Oxygen Species Differentially Regulate Neutrophil Directional Migration and Basal Random Motility in a Zebrafish Injury-Induced Inflammation Model. J Immunol (2014) 192:5998–6008. doi: 10.4049/jimmunol.1301645
49. Xu J, Wang T, Wu Y, Jin W, Wen Z. Microglia Colonization of Developing Zebrafish Midbrain Is Promoted by Apoptotic Neuron and Lysophosphatidylcholine. Dev Cell (2016) 38:214–22. doi: 10.1016/j.devcel.2016.06.018
50. Dong W, Nie X, Zhu H, Liu Q, Shi K, You L, et al. Mycobacterial Fatty Acid Catabolism is Repressed by FdmR to Sustain Lipogenesis and Virulence. Proc Natl Acad Sci U S A (2021) 118(16):e2019305118. doi: 10.1073/pnas.2019305118
51. Sheedy FJ, Grebe A, Rayner KJ, Kalantari P, Ramkhelawon B, Carpenter SB, et al. CD36 Coordinates NLRP3 Inflammasome Activation by Facilitating Intracellular Nucleation of Soluble Ligands Into Particulate Ligands in Sterile Inflammation. Nat Immunol (2013) 14:812–20. doi: 10.1038/ni.2639
52. Roca FJ, Ramakrishnan L. TNF Dually Mediates Resistance and Susceptibility to Mycobacteria via Mitochondrial Reactive Oxygen Species. Cell (2013) 153:521–34. doi: 10.1016/j.cell.2013.03.022
53. Flynn JL, Goldstein MM, Chan J, Triebold KJ, Pfeffer K, Lowenstein CJ, et al. Tumor Necrosis Factor-Alpha is Required in the Protective Immune Response Against Mycobacterium Tuberculosis in Mice. Immunity (1995) 2:561–72. doi: 10.1016/1074-7613(95)90001-2
54. Berisha HI, Pakbaz H, Absood A, Said SI. Nitric Oxide as a Mediator of Oxidant Lung Injury Due to Paraquat. Proc Natl Acad Sci U S A (1994) 91:7445–9. doi: 10.1073/pnas.91.16.7445
55. Darwin KH, Ehrt S, Gutierrez-Ramos JC, Weich N, Nathan CF. The Proteasome of Mycobacterium Tuberculosis is Required for Resistance to Nitric Oxide. Science (2003) 302:1963–6. doi: 10.1126/science.1091176
56. Braverman J, Stanley SA. Nitric Oxide Modulates Macrophage Responses to Mycobacterium Tuberculosis Infection Through Activation of HIF-1alpha and Repression of NF-Kappab. J Immunol (2017) 199:1805–16. doi: 10.4049/jimmunol.1700515
57. Yan S, Shen H, Lian Q, Jin W, Zhang R, Lin X, et al. Deficiency of the AIM2-ASC Signal Uncovers the STING-Driven Overreactive Response of Type I IFN and Reciprocal Depression of Protective IFN-Gamma Immunity in Mycobacterial Infection. J Immunol (2018) 200:1016–26. doi: 10.4049/jimmunol.1701177
58. Chen CJ, Kono H, Golenbock D, Reed G, Akira S, Rock KL. Identification of a Key Pathway Required for the Sterile Inflammatory Response Triggered by Dying Cells. Nat Med (2007) 13:851–6. doi: 10.1038/nm1603
Keywords: mycobacterial infection, NLR, innate immunity, macrophage, neutrophil, inflammasome
Citation: Niu L, Luo G, Liang R, Qiu C, Yang J, Xie L, Zhang K, Tian Y, Wang D, Song S, Takiff HE, Wong K-W, Fan X, Gao Q and Yan B (2022) Negative Regulator Nlrc3-like Maintain the Balanced Innate Immune Response During Mycobacterial Infection in Zebrafish. Front. Immunol. 13:893611. doi: 10.3389/fimmu.2022.893611
Received: 10 March 2022; Accepted: 25 April 2022;
Published: 25 May 2022.
Edited by:
Hridayesh Prakash, Amity University, IndiaReviewed by:
Paras K. Anand, Imperial College London, United KingdomCopyright © 2022 Niu, Luo, Liang, Qiu, Yang, Xie, Zhang, Tian, Wang, Song, Takiff, Wong, Fan, Gao and Yan. This is an open-access article distributed under the terms of the Creative Commons Attribution License (CC BY). The use, distribution or reproduction in other forums is permitted, provided the original author(s) and the copyright owner(s) are credited and that the original publication in this journal is cited, in accordance with accepted academic practice. No use, distribution or reproduction is permitted which does not comply with these terms.
*Correspondence: Bo Yan, Ym8ueWFuQHNocGhjLm9yZy5jbg==; Qian Gao, cWlhbmdhb0BmdWRhbi5lZHUuY24=
†These authors have contributed equally to this work
Disclaimer: All claims expressed in this article are solely those of the authors and do not necessarily represent those of their affiliated organizations, or those of the publisher, the editors and the reviewers. Any product that may be evaluated in this article or claim that may be made by its manufacturer is not guaranteed or endorsed by the publisher.
Research integrity at Frontiers
Learn more about the work of our research integrity team to safeguard the quality of each article we publish.