- 1Princess Máxima Center for Pediatric Oncology, Utrecht, Netherlands
- 2Center for Translational Immunology, University Medical Center Utrecht, Utrecht, Netherlands
The outcome of allogeneic hematopoietic cell transplantation (allo-HCT) largely depends on the development and management of graft-versus-host disease (GvHD), infections, and the occurrence of relapse of malignancies. Recent studies showed a lower incidence of chronic GvHD and severe acute GvHD in patients receiving naive T cell depleted grafts compared to patients receiving complete T cell depleted grafts. On the other hand, the incidence of acute GvHD in patients receiving cord blood grafts containing only naive T cells is rather low, while potent graft-versus-leukemia (GvL) responses have been observed. These data suggest the significance of naive T cells as both drivers and regulators of allogeneic reactions. The naive T cell pool was previously thought to be a quiescent, homogenous pool of antigen-inexperienced cells. However, recent studies showed important differences in phenotype, differentiation status, location, and function within the naive T cell population. Therefore, the adequate recovery of these seemingly innocent T cells might be relevant in the imminent allogeneic reactions after allo-HCT. Here, an extensive review on naive T cells and their contribution to the development of GvHD and GvL responses after allo-HCT is provided. In addition, strategies specifically directed to stimulate adequate reconstitution of naive T cells while reducing the risk of GvHD are discussed. A better understanding of the relation between naive T cells and alloreactivity after allo-HCT could provide opportunities to improve GvHD prevention, while maintaining GvL effects to lower relapse risk.
Introduction
Allogeneic hematopoietic cell transplantation (allo-HTC) is an intensive, but potentially curative treatment for refractory hematologic malignant disorders, and life-threatening, non-malignant diseases, such as primary immune deficiencies and inborn metabolic disease. Complications associated with this therapy, such as graft rejection, acute or chronic graft-versus-host-disease (aGvHD and cGvHD respectively), infections, and disease relapse are life-threatening and limit allo-HCT success. T cell reconstitution after allo-HCT plays an important role, as T cell numbers associate with the occurrence of relapse in malignant disease and the outcome of GvHD responses (1–7). The effect of T cell reconstitution on outcome after allo-HCT seems dependent on a delicate balance between overly active, unwanted responses to ‘self’ and building productive immunity against relapsed disease.
Transplantation with T cell depleted (TCD) grafts results in lower GvHD incidence and graft rejection, but also leads to delayed immune reconstitution (IR) and thereby increases risk of disease relapse and viral infections (2, 3, 8–11). Recently, new evidence has highlighted the importance of specifically naive T cells in GvHD and graft-versus-leukemia (GvL) responses after allo-HCT; transplantation with naive TCD grafts significantly reduced the chance of developing cGvHD and severe aGvHD (12, 13), while allowing faster IR and lower disease relapse compared to patients receiving complete TCD grafts (12–14). The knowledge about naive T cell reconstitution after allo-HCT is, however, remarkably scarce and the precise mechanisms and role for naive T cells in alloreactivity needs to be elucidated in more detail.
In this review, we will provide an overview from recent literature on the heterogeneity and composition of the naive T cell population in different graft types and discuss their role in allogeneic reactions associated with GvHD and GvL after allo-HCT. Furthermore, possible naive T cell-targeted strategies to enhance allo-HCT outcome will be suggested. More knowledge about the underlying mechanisms of naive T cells in alloreactivity after allo-HCT will allow us to gather insights in how to prevent adverse reactions such as GvHD, while maintaining GvL responses to prevent leukemia relapse.
Naive T Cell Reconstitution After allo-HCT
Naive T cells differentiate from thymocytes in the thymus and are a heterogenous pool of T cells that have not yet encountered their cognate antigen (14–17). Thymocytes arise from hematopoietic stem cells (HSCs) in the bone marrow (BM), after which they migrate to the thymus where positive and negative selection takes place. Following transformation into a mature T cell, naive T cells migrate to the periphery and are called recent thymic emigrants (RTEs). RTEs are characterized by the surface expression of CD31, CR1 and CR2 (18), the production of IL-8, and high levels of T cell receptor excision circles (TRECs) (19). TRECs are generated during T cell receptor (TCR) gene rearrangements in the thymus, have a low intracellular degradation and do not replicate, thus can serve as a reliable marker for thymic output (20). In addition, RTEs have upregulated expression of TLR1 and appear to increase IL-8 production and upregulate CD45RO and CCR4 upon ligand stimulation (16), indicating that they are susceptible to innate stimuli. More mature naive T cells lower the production of IL-8 and express the lymphoid homing receptors CCR7 and CD62L, which are responsible for their continuous circulation between secondary lymphoid organs and the blood via the lymphatic system. Although the exact compartmentalization is tissue- and age-dependent, naive T cells are also widely distributed in non-lymphoid tissues, and some cells are suggested to hardly even recirculate through the blood (16, 21, 22). It is thought that tissue residency supports naive T cell maturation and long-term maintenance (22). However, the role of the tissue niche needs further investigation. Upon encounter and interaction with their cognate antigen, naive T cells will expand and differentiate into different types of effector and memory T cells in a matter of days. Recent studies on single-cell transcriptomics show that the differentiation of naïve T cells to central memory (CM), then effector memory (EM), and finally CD45RA+ EM (EMRA) T cells is accompanied by upregulation of chemokine and cytokine genes (23–26). A phenomenon also shown in innate T cells (27), and tissue resident T cells (28). Especially after HCT, single-cell sequencing of naïve T cells would provide important insight in the role of naïve T cells in GvHD and GvL.
A large and diverse TCR repertoire is of great importance for the formation of an adequate immune response against newly encountered pathogens. Normally, both thymic production and homeostatic peripheral expansion (HPE) of naive T cells are responsible for the TCR repertoire diversity and size of the naive T cell pool after allo-HCT (29, 30). During the first few months after allo-HCT, the lymphopenic state of the patient results in cytokine- and antigen-driven proliferation of graft-derived (naive) T cells for compensation, which can lead to the expansion of certain T cell clones at the expense of others (31–33), significantly limiting TCR diversity. Thymic function is needed to restore TCR repertoire diversity, however, the thymus is drastically damaged due to cancer treatment and HCT-conditioning. It generally takes 6-12 months for the thymus to regenerate and restore thymopoiesis, and reconstitution to reference levels and restoration of the TCR repertoire may require years (30, 34). A limited TCR repertoire after allo-HCT has been linked to worse survival chances (35, 36), and adequate naive T cell reconstitution might, therefore, be of clinical importance after transplantation (Figure 1).
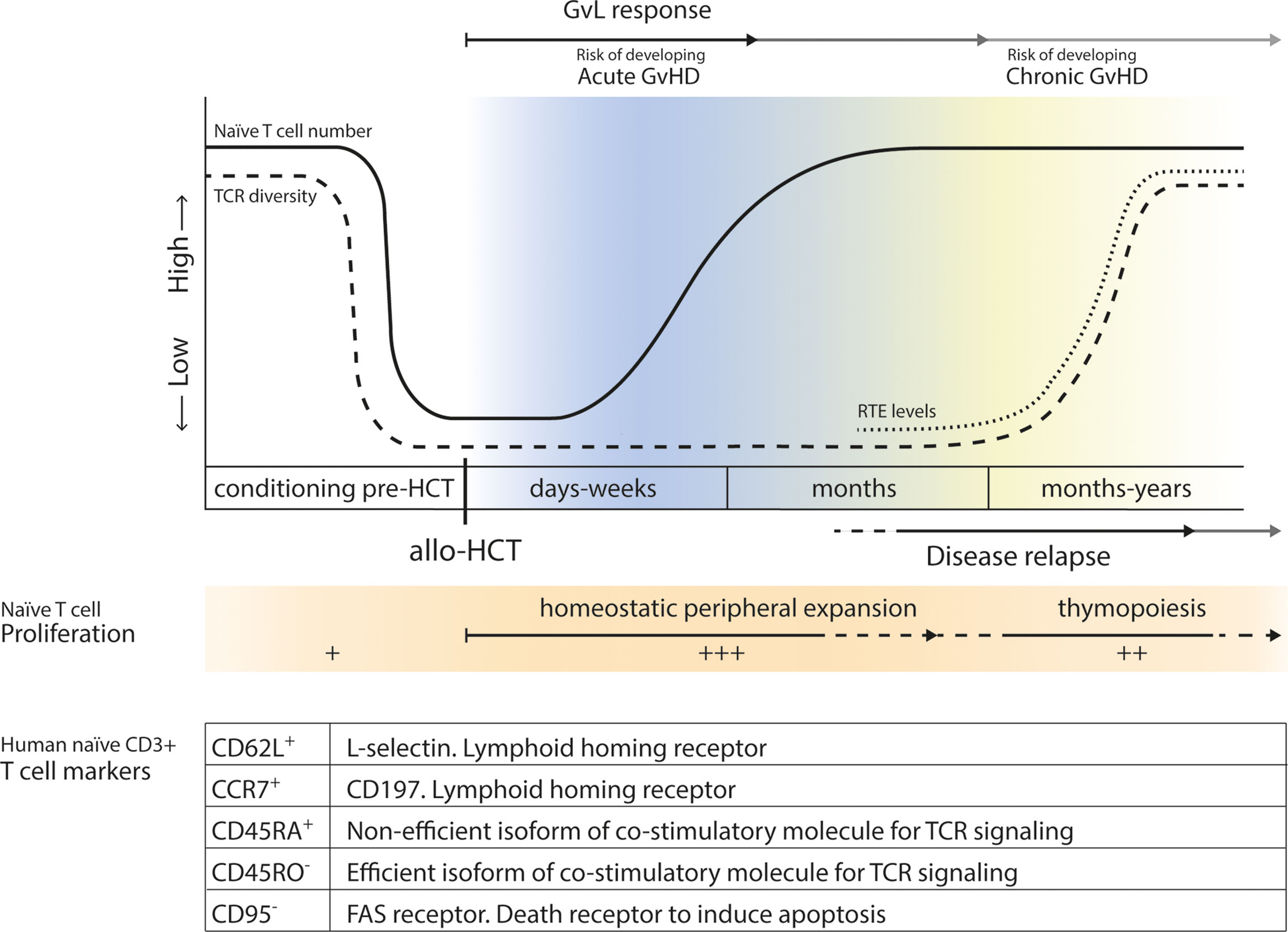
Figure 1 Levels of naive T cells before and after allo-HCT. Before transplantation, levels of recipient naive T cells drastically drop due to conditioning regimens, radiation and serotherapy. In the first few months after allo-HCT, after an ultimate low, lymphopenia induced HPE of donor-derived naive T cells will increase levels of naive T cells. After 6-12 months, thymopoiesis will start to be restored and production and release of RTEs leads to an increase in TCR diversity. Acute GvHD may develop early after allo-HCT, while chronic GvHD may occur later after transplantation. GvL responses contribute to the prevention of disease relapse later after allo-HCT. In the table markers associated with a naive CD3+ T cell phenotype are summarized, and their function is shortly described.
Early naive T cell reconstitution after allo-HCT highly depends on the number of naive T cells transferred with the graft (35, 37–39). Different types of allo-HCT grafts contain different frequencies of naive T cells; cord blood (CB) contains almost no memory T cells and CB-derived grafts, therefore, consist of a significantly larger fraction of naive CD4+ and CD8+ T cells than peripheral blood (PB-) and BM-derived grafts (40). Besides higher frequencies of naive T cells, the fast reconstitution of naive T cells after CBT might be a result of the highly proliferating fetal naive CD4+ T cells present in CB grafts (41, 42). This might explain why early naïve T cell counts are generally higher after cord blood transplantation (CBT) compared to bone marrow transplantation (BMT) and peripheral blood stem cell transplantation (PBT) (35, 37–39). In turn, PB and BM grafts contain higher frequencies of effector, central and terminally differentiated memory T cells due to the interaction with many antigens encountered during the life time of the donor (43). Notably, the neonatal naive T cell compartment differs in composition compared to the adult naive T cell compartment in BM and PB, which is extensively reviewed by van den Broek et al. (16). While naïve CB T cells recapitulate fetal ontogeny, naïve T cells after BMT resemble naïve T cells from peripheral blood (42). These fetal naive T cells in CB grafts seem to mediate a stronger anti-leukemic effect compared to adult naive T cells, and are poised to become regulatory T cells (41). The enhanced TCR signaling in CB naïve T cells is an important mechanism for the rapid CD4+ T cell restoration after CBT, as well as their strong GvL effect (41). Naïve T cells in G-CSF-mobilized PB and -BM grafts might be less functional, and more tolerogenic, since G-CSF-treatment was shown to induce immunologic tolerance and negatively affect T-cell functionality (44).
Next to early naïve T cell recovery, late recovery via thymopoiesis might also be different when starting with fetal- compared to adult stem cell sources. It is known that fetal and adult HSCs give rise to thymocytes with distinct gene signatures (41), indicating that thymopoiesis might be different after CBT and BMT/PBT. Indeed, the differentiation of donor-derived lymphoid progenitors in the thymus is faster after CBT compared with PBT and BMT, and a broader TCR repertoire diversity is present up to 3 years after CBT (35, 45). Thymic output increases similarly following PBT and BMT (34, 46), although higher naive T cell numbers have been reported early and later after transplantation in recipients of G-CSF-mobilized PB grafts compared with recipients of BM grafts (47). Importantly, it should be noted that thymopoiesis is significantly influenced by age, viral reactivation, immunosuppressive drugs and alloreactive donor T cells against host thymic epithelium (34, 45, 46, 48, 49).
The different naive T cell pool between graft sources, together with differences in patient characteristics, may lead to a differential naive T cell composition after allo-HCT. This possibly affects the type of immune response generated upon various types of triggers and may play a crucial role in both GvHD and GvL. Most studies show that graft-derived naive T cells are strongly related to GvHD risk (50, 51), although host tissue-resident T cells are also described to play a role (52). Naive T cells derived from thymopoiesis later after transplantation are less likely to induce alloreactivity, as they undergo selection in the patient’s thymus. Together, it would be valuable to better understand the function of naive T cells and to monitor naive T cell heterogeneity after allo-HCT.
Naive T Cells in GvHD
GvHD is one of the major complications after allo-HCT, associated with high morbidity and mortality risks, accounting for approximately 15% of deaths (53). Acute GvHD and chronic GvHD differ in clinical presentation, time of onset after transplantation and pathogenesis (54). Incidences vary between 20-60%, depending on specific patient characteristics and treatment protocols (55–57). It is thought that naive T cells play a major role in the development of both acute and chronic GvHD (12, 58), although the exact mechanisms remain elusive.
Acute GvHD has long thought to be mediated by donor T cells, which are activated by host antigen presenting cells (APCs) in the setting of an inflammatory environment and typically occurs in the first few months after transplantation (54, 59). However, recent evidence points towards a previously overlooked role of host tissue-resident T cells. These cells are able to survive HCT-conditioning and are not only present in the skin and the gut during aGvHD but are also activated, suggested to be a result of donor APCs (52). Since transplantation with naive TCD grafts does not reduce aGvHD incidence but does reduce severity of the disease (12), suggests that both donor and host T cells may be required for severe aGvHD. Upon activation, alloreactive effector memory T cells cause tissue damage to target tissues, such as the gastrointestinal tract, liver, skin, or thymus, by direct cytotoxicity or cytokine-mediated injury (4, 60). The question remains if the effector memory T cells measured in blood of patients are directly derived from the graft and proliferate upon encounter of an alloantigen, or that these cells are differentiated from graft-derived naive T cells that recognized allo-antigens and initiated an allogeneic reaction. This difference is important and should be considered when studying the possible role of naive T cells and effector memory T cells in alloreactivity after allo-HCT.
Some studies suggest that naive T cells are even more potent in the induction of aGvHD and cause a more severe type compared to memory T cells (56, 61–63), especially because naive T cells are the most potent inducers of alloreactive responses in vitro (50, 51, 64). An explanation for this could be that naive T cells have a high TCR repertoire diversity and a high proliferative capacity, resulting in an increased potential of recognizing allogeneic antigens presented by host APCs and initiating alloreactive immune responses. In line with this, high quantities of CD4+ naive T cells in allografts correlated with a higher incidence of aGvHD after transplantation (58). Additionally, aGvHD being associated with lower counts of CD4+ naive T cells in the peripheral blood suggests that graft-derived naive T cells became activated in the process of aGvHD (58). Fujii et al. demonstrated that successful treatment of aGvHD by infusion of human BM mesenchymal stem cell-derived extracellular vesicles in mice was mediated by suppression of the functional differentiation of naive T cells to effector T cells (65). These results suggest that graft-derived naive T cells can recognize allogeneic antigens and subsequently induce a potent alloreactive immune response by expansion and differentiation to effector memory T cells (62–65). Notably, homing to secondary lymphoid organs might be essential for the induction of aGvHD by naive T cells, as blocking of lymphoid homing receptors or the entry of lymphoid organs almost fully abolishes aGvHD in mice (66).
In chronic GvHD, persistent tissue injury and early post-transplant inflammation due to aGvHD and conditioning can result in enhanced antigen presentation by APCs and thereby activation of allo-reactive naive T cells (67). In addition, thymic damage due to alloreactive responses in aGvHD and as a result of the conditioning are thought to be responsible for a diminished naive regulatory T cell output and a defective negative selection of auto-reactive naive T cells after allo-HCT, which may contribute to the formation of allo-reactive responses as seen in cGvHD (49, 68–71). This underlines the importance to further clarify the role of naive T cell reconstitution in GvHD. Currently, clinical trials are being conducted to further evaluate naive TCD peripheral blood stem cell grafts. Recently reported data on three phase-II clinical trials showed very low rates of cGvHD and a decreased incidence of serious aGvHD in the HLA-matched HCT setting, similar to complete TCD grafts (12, 13), with no apparent increase in relapse rates and an improved immune reconstitution compared to complete T cell depletion (12, 13, 72–74). However, it should be noted that the observed outcome in recipients of naive TCD PB stem cell grafts cannot directly be translated to other graft sources. The outcomes of PBT and BMT are rather similar, however, recipient transplanted with CB grafts already showed reduced GvHD rates compared to PBT/BMT (75, 76). Since CB grafts almost completely consist of naive T cells, this suggests the presence of phenotypic differences in the naive T cell pool between graft sources. Specifically, fetal CD4+ naive T cells contain the propensity to adopt a regulatory T cell phenotype upon stimulation, thereby supporting tolerance to self, and potentially foreign, antigens (41).
Naive T Cells in GvL
The curative potential of allo-HCT therapy in leukemia patients is predominantly regulated through alloreactivity, mediated by donor T cells directed at residual malignant cells. The first study on the cure of leukemia after total body irradiation and allo-HCT was published in 1956, and important insights into underlying processes were reported in a landmark study from the International Bone Marrow Transplant Registry in 1990 (77, 78). Interestingly, they showed that usage of TCD grafts, and grafts derived from identical twins, both resulted in a reduced GvL response (78). Accordingly, it was concluded that GvL is mediated by donor T cells and is dependent on the existence of histocompatibility differences between donor and recipient (68, 78). Moreover, it was shown that both CD8+ and CD4+ T cell subsets are involved in GvL reactions by direct target killing of tumor cells due to the recognition of antigens presented by MHCI and MHCII respectively (69, 79). Outcomes of patients transplanted with naive TCD grafts confirm an important role for donor T cells in GvL; usage of complete TCD grafts resulted in increased relapse rates (13, 68, 69, 78), while the recipients of naive TCD grafts show encouraged relapse-free survival rates (12, 72–74). Moreover, acute leukemia patients with minimal residual disease receiving a haplo-HCT with ATG and G-CSF, had a significantly delayed recovery of naïve T cells compared to that of HLA-matched sibling donor recipients (80–82). However, the cumulative incidence of relapse was lower after haplo-HCT. This suggests that other lymphocyte subsets may play a role in GvL in recipients of naive TCD and haploidentical grafts.
Effector memory T cells might mediate GvL effects without causing GvHD (62, 76, 83–85). Effector memory T cells are short-lived, possess reduced cytokine production capabilities after allo-HCT, and seem to have a lower threshold for activation of GvL effects than for induction of GvH responses in murine models (83, 84). This might be due to the requirement of a sustained and high-magnitude T cell response for GvHD, and not for GvL, that effector memory T cells can not generate. Another explanation may be linked to their localization; effector memory T cells have excellent access to leukemia cells in blood, bone marrow, and spleen without expression of additional inflammatory signals (79), while GvH responses are thought to be initiated in secondary lymphoid tissue, i.e. sites that are easily accessible for naive T cells but not for effector memory T cells due to their lack of lymphoid homing receptors CD62L and CCR7 (86).
Interestingly, CBT, in which the graft almost exclusively contains naive T cells, show high GvL potential in combination with reduced relapse risk, without increased GvHD incidence compared to BMT/PBT (75, 87). CB-derived T cells exhibit exceptional learning capacity, high anti-virus properties, and powerful anti-leukemic activity (88–90). Furthermore, within two years after CBT, a higher TCR repertoire diversity can be observed compared to BMT/PBT (35, 91). The intrinsic differences of CB-derived naive T cells compared to other graft types is largely unknown and of high interest for future investigation. One possible explanation is that fetal naive CB T cells are skewed to give rise to other T cell lineages compared to adult naive T cells (41). This emphasizes the importance of in-depth phenotyping of naive T cells derived from different graft types to study their role in IR and the development of GvHD and GvL after allo-HCT. This might provide implications for the depletion of naive T cells from BM or PB grafts, leading to a reduced GvHD risk without hampering GvL responses. CB-derived naive T cells are more tolerogenic and unmanipulated CB grafts might thus protect against alloreactive responses and contribute to an improved immune reconstitution.
Naive T Cell-Based Markers to Predict Allo-HCT Outcome
Several studies report a correlation of increased levels of naive T cells with the onset of cGvHD (58, 92, 93). Bohmann et al. described that both the proportion of naive cells among CD4+ T cells and absolute CD4+ naive T cell counts predict the onset of clinical symptoms of cGvHD (93). In addition, the absolute level of activated CD8+ effector memory T cells was shown to predict onset of aGvHD (4), showing the possible predictive value of naive T cells. However, given their lymphoid homing properties, the naive T cells present in the circulation might not be representative for the total naive T cell compartment. Future research into peripheral tissues may reveal specific niches for naive T cell maintenance and functional differentiation, which might improve understanding of the heterogenic naive T cell pool and their contribution to alloreactivity in the reconstituting immune system after allo-HCT. To achieve this, there is a need for harmonization and standardization of both the assessment methods and the markers used for naive T cell characterization. When naive T cell subsets and pool composition measurements can be compared between different transplantation centers, naive T cells can further be studied and validated as a predictive marker for GvHD.
In a study by Thus et al., a tool was developed to predict minor histocompatibility antigen mismatches and their correlation with patient survival and adverse events in different transplantation settings. Predicted Indirectly ReCognizable HLA Epitopes (PIRCHE) were used to predict donor T cell mediated recognition of mismatched-HLA derived peptides following allo-HCT, with peptides presented on HLA-I as PIRCHE-I score, and peptides presented on HLA-II as PIRCHE-II score. Those scores were correlated with patient survival, disease relapse and complication development (94). In BMT, low PIRCHE I and PIRCHE II scores in donor-recipient mismatch resembled similar allo-HCT outcomes as 10/10 matched grafts. However, in CBT, a high PIRCHE I and low PIRCHE II score in donor-recipient mismatch increased GvL responses without changing GvHD risk (94). This might lead to a new donor-recipient matching strategy in CBT to further enhance its GvL effects. Nevertheless, the mechanisms behind the different behavior of naive T cells in CBT compared to BMT and PBT remain elusive and more research into the heterogeneity and function of naive T cells is necessary to unravel this controversy.
Naive T Cell-Based Strategies to Improve Allo-HCT Outcome
Some centers are successfully using T cell depleted grafts to reduce GvHD risk. Despite initial success, complications, such as increased disease relapse and viral reactivation have emerged, caused by delayed or absent early T cell reconstitution after allo-HCT (2). Recent efforts are focused on improving T cell reconstitution by personalized dosing, timing and duration of immunosuppressive drugs used in allo-HCT. Commonly used drugs shown to affect (naive) T cell reconstitution are serotherapy (anti-thymocyte globulin; ATG) (1, 10), fludarabine (95) and steroids (96). Importantly, significantly smaller naive T cell numbers post-HCT are present when ATG is administered prior to transplantation (97), while naive T cell transferred with the graft survive posttransplant cyclophosphamide (98). A randomized phase 2 clinical trial (Trial no. NL4836) studied the effect of ATG dosing based on weight and absolute lymphocyte counts pre-HCT. CD4+ T cell reconstitution and event free survival were improved in the individualized dosing group without affecting the incidence of graft failure and GvHD (99). Fludarabine exposure is also highly variable between patients in current dosing regimens. Optimizing fludarabine exposure in individual patients, using weight and renal function, might thus be of clinical relevance in allo-HCT (95). The pharmacokinetic/pharmacodynamic relationship of steroids in allo-HCT is currently investigated in our center (NL8703). If such a relationship exists, a clinical trial to study personalized dosing might show improved immune reconstitution and outcome after allo-HCT.
Since naive T cells show higher alloreactive capacity and are more potent inducers of GvHD compared to effector memory T cells (51, 61, 62, 83, 100–102), depleting naive T cells from BM or PB grafts (and not CB grafts) might be a strategy to prevent GvHD without delaying T cell reconstitution (103). Bleakley et al. proved the feasibility of creating naive TCD PB stem cell grafts and its safety in allo-HCT (12). Although the incidence of aGvHD was not reduced, a remarkably low rate of cGvHD (9%) was observed in patients receiving naive TCD grafts, compared to cGvHD rates after complete TCD HCT (19%) or T cell-replete HCT (40-63%) (12, 104, 105). Additionally, memory CD4+ and CD8+ T cell numbers recovered much earlier, and no significant increase in disease relapse was observed after naive TCD grafts relative to patients that received complete TCD grafts (12, 14). Furthermore, viral reactivations in patients receiving naive TCD grafts were comparable with T cell-replete grafts, and other serious infections were rare (12, 106). These results are comparable to recent data on three prospective phase-II clinical trials that showed low rates of severe aGvHD and an exceptionally low rate of cGvHD (7%), with no associations with increased relapse rates or serious infections (13). Two other phase I clinical trials also show the feasibility of memory only- or naive TCD donor lymphocyte infusions and its association with a low incidence of GvHD (72, 73). However, these studies were single-arm first in-human clinical trials, so no formal comparisons of survival were conducted. A prospective randomized controlled clinical trial is currently being performed to directly compare naive TCD PB grafts with standard unmanipulated grafts (NCT03779854). In addition, another multicenter trial focusses on the comparison of naive TCD PB grafts with complete TCD PB grafts, PBT with post-HCT cyclophosphamide and PBT with tacrolimus and methotrexate to study whether naive T cell depletion directly reduces cGvHD (NCT03970096). Nevertheless, in combination with pre-clinical data, naive TCD grafts show great potential in prevention of GvHD without delaying IR and hampering GvL responses in allo-HCT.
Thymic regeneration, thereby recovering TCR repertoire diversity (107), may take months to years, leaving patients susceptible for infections and viral reactivation with increased morbidity and mortality risk (26, 108). Strategies to enhance the restoration of thymic function after allo-HCT are therefore of high interest, as extensively reviewed by Velardi et al. (109). Exogenous administration of keratinocyte growth factor (KGF), IL-7 or IL-22 in (pre-)clinical studies has shown to be beneficial in the rejuvenation of thymic function and thereby contribute to improved thymopoiesis, enhanced naive T cell recovery, expansion of the RTE pool and increased TCR repertoire diversity (110–115). In addition, thymosin-α1 administration in allo-HCT recipients was shown to be safe in a phase I/II clinical trial and resulted in increased T cell numbers together with earlier appearance of pathogen-specific T cell responses (116). However, the success of those therapies will partly depend on the degree of damage to the thymus caused by intensity of allo-HCT conditioning and additional complications. Again, this emphasizes the importance of personalized conditioning and treatment in allo-HCT to reduce the risk of thymic damage. Moreover, when thymic function can be restored early after transplantation, de novo production of naive T cells will contribute to a high TCR repertoire diversity and thus high antigen specificity, thereby support a long-lasting functional immune recovery (35).
Conclusions and Future Directions
Current insights show that the naive T cell compartment consists of a heterogenic population of T cells highly involved in GvHD and GvL responses after allo-HCT. The naive T cell pool contains the most diverse collection of TCRs, which is of great importance for the formation of adequate immune responses against newly encountered pathogens. Together with the capacity of naive T cells to promptly switch to effector phenotype and proliferate upon antigen encounter, this high TCR repertoire diversity potentiates the naive T cell pool for GvL. On the other hand, TCR diversity might be responsible for the recognition of allo-antigens and contribute to GvHD risk. Naive T cells derived from distinct graft types, such as CB versus BM and PB, show a different behavior regarding GvHD risk and GvL effect, and might thus have an intrinsic difference in the balance between GvHD and GvL alloreactivity. The differences between CB-derived and BM/PB-derived naive T cells need to be further explored and their role in alloreactivity needs to be elucidated. For this, the recent advances in tracking naïve T cells for single cell sequencing using natural barcoding, would be of major interest to better understand their role in GvHD and GvL effects (117). Moreover, factors that suppress GvHD might also limit the GvL potential of naive T cells, such as corticosteroids, conditioning with ATG, and naive T cell depletion in BMT/PBT approaches. Importantly, GvL responses by other lymphocyte populations might also be suppressed by GvHD treatment and prophylaxis. Therefore, individualized conditioning, evaluating the effect of steroid exposure, next to application of PIRCHE for donor selection, would be of interest for future study to potentiate naive T cell GvL function without exacerbating GvHD. Furthermore, harmonization and standardization of naive T cell characterization and monitoring between transplantation centers would help to further identify the role of naive T cells in GvHD development and relapse risk. More knowledge about the full composition of the naive T cell pool after allo-HCT can possibly function to better predict and understand alloreactivity. This might provide opportunities to better prevent GvHD reactions, while remaining GvL effects to lower relapse risk after allo-HCT.
Author Contributions
LD and ES wrote the manuscript; ES designed the figure; CK and SN conceptualized and revised the manuscript; CL revised the manuscript. All authors have read and agreed to the published version of the manuscript.
Funding
This work was supported by the Princess Máxima Center for Pediatric Oncology, Utrecht, The Netherlands.
Conflict of Interest
The authors declare that the research was conducted in the absence of any commercial or financial relationships that could be construed as a potential conflict of interest.
Publisher’s Note
All claims expressed in this article are solely those of the authors and do not necessarily represent those of their affiliated organizations, or those of the publisher, the editors and the reviewers. Any product that may be evaluated in this article, or claim that may be made by its manufacturer, is not guaranteed or endorsed by the publisher.
References
1. Admiraal R, Lindemans CA, van Kesteren C, Bierings MB, Versluijs AB, Nierkens S, et al. Excellent T-Cell Reconstitution and Survival Depend on Low ATG Exposure After Pediatric Cord Blood Transplantation. Blood (2016) 128:2734–41. doi: 10.1182/blood-2016-06-721936
2. Admiraal R, van Kesteren C, Jol-van derZijde CM, Lankester AC, Bierings MB, Egberts TC, et al. Association Between Anti-Thymocyte Globulin Exposure and CD4+ Immune Reconstitution in Paediatric Haemopoietic Cell Transplantation: A Multicentre, Retrospective Pharmacodynamic Cohort Analysis. Lancet Haematol (2015) 2:e194–203. doi: 10.1016/S2352-3026(15)00045-9
3. Bartelink IH, Belitser SV, Knibbe CA, Danhof M, de Pagter AJ, Egberts TC, et al. Immune Reconstitution Kinetics as an Early Predictor for Mortality Using Various Hematopoietic Stem Cell Sources in Children. Biol Blood Marrow Transpl (2013) 19:305–13. doi: 10.1016/j.bbmt.2012.10.010
4. Khandelwal P, Lane A, Chaturvedi V, Owsley E, Davies SM, Marmer D, et al. Peripheral Blood CD38 Bright CD8+ Effector Memory T Cells Predict Acute Graft-Versus-Host Disease. Biol Blood Marrow Transpl (2015) 21:1215–22. doi: 10.1016/j.bbmt.2015.04.010
5. Podgorny PJ, Liu Y, Dharmani-Khan P, Pratt LM, Jamani K, Luider J, et al. Immune Cell Subset Counts Associated With Graft-Versus-Host Disease. Biol Blood Marrow Transpl (2014) 20:450–62. doi: 10.1016/j.bbmt.2014.01.002
6. Bühlmann L, Buser AS, Cantoni N, Gerull S, Tichelli A, Gratwohl A, et al. Lymphocyte Subset Recovery and Outcome After T-Cell Replete Allogeneic Hematopoietic SCT. Bone Marrow Transpl (2011) 46:1357–62. doi: 10.1038/bmt.2010.306
7. Ritter J, Seitz V, Balzer H, Gary R, Lenze D, Moi S, et al. Donor CD4 T Cell Diversity Determines Virus Reactivation in Patients After HLA-Matched Allogeneic Stem Cell Transplantation. Am J Transpl (2015) 15:2170–9. doi: 10.1111/ajt.13241
8. Bosch M, Dhadda M, Hoegh-Petersen M, Liu Y, Hagel LM, Podgorny P, et al. Immune Reconstitution After Anti-Thymocyte Globulin-Conditioned Hematopoietic Cell Transplantation. Cytotherapy (2012) 14:1258–75. doi: 10.3109/14653249.2012.715243
9. Mohty M, Labopin M, Balère ML, Socié G, Milpied N, Tabrizi R, et al. Antithymocyte Globulins and Chronic Graft-vs-Host Disease After Myeloablative Allogeneic Stem Cell Transplantation From HLA-Matched Unrelated Donors: A Report From the Sociéte Française De Greffe De Moelle Et De Thérapie Cellulaire. Leukemia (2010) 24:1867–74. doi: 10.1038/leu.2010.200
10. Lindemans CA, Chiesa R, Amrolia PJ, Rao K, Nikolajeva O, de Wildt A, et al. Impact of Thymoglobulin Prior to Pediatric Unrelated Umbilical Cord Blood Transplantation on Immune Reconstitution and Clinical Outcome. Blood (2014) 123:126–32. doi: 10.1182/blood-2013-05-502385
11. Hiwarkar P, Gaspar HB, Gilmour K, Jagani M, Chiesa R, Bennett-Rees N, et al. Impact of Viral Reactivations in the Era of Pre-Emptive Antiviral Drug Therapy Following Allogeneic Haematopoietic SCT in Paediatric Recipients. Bone Marrow Transpl (2013) 48:803–8. doi: 10.1038/bmt.2012.221
12. Bleakley M, Heimfeld S, Loeb KR, Jones LA, Chaney C, Seropian S, et al. Outcomes of Acute Leukemia Patients Transplanted With Naive T Cell-Depleted Stem Cell Grafts. J Clin Invest (2015) 125:2677–89. doi: 10.1172/JCI81229
13. Bleakley M, Sehgal A, Seropian S, Biernacki MA, Krakow EF, Dahlberg A, et al. Naive T-Cell Depletion to Prevent Chronic Graft-Versus-Host Disease. J Clin Oncol (2022) 40(11):1174–85. doi: 10.1200/JCO.21.01755
14. Devine SM, Carter S, Soiffer RJ, Pasquini MC, Hari PN, Stein A, et al. Low Risk of Chronic GVHD and Relapse Associated With T-Cell Depleted PBSC Transplantation for AML. Biol Blood Marrow Transpl (2011) 17:1343–51. doi: 10.1016/j.bbmt.2011.02.002
15. Das A, Rouault-Pierre K, Kamdar S, Gomez-Tourino I, Wood K, Donaldson I, et al. Adaptive From Innate: Human IFN-γ + CD4 + T Cells Can Arise Directly From CXCL8-Producing Recent Thymic Emigrants in Babies and Adults. J Immunol (2017) 199:1696–705. doi: 10.4049/jimmunol.1700551
16. Van Den Broek T, Borghans JAM, Van Wijk F. The Full Spectrum of Human Naive T Cells. Nat Rev Immunol (2018) 18:363–73. doi: 10.1038/s41577-018-0001-y
17. Van Den Broek T, Delemarre EM, Janssen WJ, Nievelstein RA, Broen JC, Tesselaar K, et al. Neonatal Thymectomy Reveals Differentiation and Plasticity Within Human Naive T Cells. J Clin Invest (2016) 126:1126–36. doi: 10.1172/JCI84997
18. Pekalski ML, García AR, Ferreira RC, Rainbow DB, Smyth DJ, Mashar M, et al. Neonatal and Adult Recent Thymic Emigrants Produce IL-8 and Express Complement Receptors CR1 and CR2. JCI Insight (2017) 2:1–16. doi: 10.1172/jci.insight.93739
19. Kimmig S, Przybylski GK, Schmidt CA, Laurisch K, Möwes B, Radbruch A, et al. Two Subsets of Naive T Helper Cells With Distinct T Cell Receptor Excision Circle Content in Human Adult Peripheral Blood. J Exp Med (2002) 195:789–94. doi: 10.1084/jem.20011756
20. Mikhael NL, Elsorady M. Clinical Significance of T Cell Receptor Excision Circle (TREC) Quantitation After Allogenic HSCT. Blood Res (2019) 54:274–81. doi: 10.5045/br.2019.54.4.274
21. Wong MT, Ong DE, Lim FS, Teng KW, McGovern N, Narayanan S. A High-Dimensional Atlas of Human T Cell Diversity Reveals Tissue-Specific Trafficking and Cytokine Signatures Resource A High-Dimensional Atlas of Human T Cell Diversity Reveals Tissue-Specific Trafficking and Cytokine Signatures. Immunity (2016) 45:442–56. doi: 10.1016/j.immuni.2016.07.007
22. Thome JJC, Grinshpun B, Kumar BV, Kubota M, Ohmura Y, Lerner H, et al. Long-Term Maintenance of Human Naïve T Cells Through in Situ Homeostasis in Lymphoid Tissue Sites. Sci Immunol (2016) 1:eaah6506. doi: 10.1126/sciimmunol.aah6506
23. Berkley AM, Hendricks DW, Simmons KB, Fink PJ. Recent Thymic Emigrants and Mature Naive T Cells Exhibit Differential DNA Methylation at Key Cytokine Loci. J Immunol (2013) 190:6180–6. doi: 10.4049/jimmunol.1300181
24. Cunningham CA, Bergsbaken T, Fink PJ. Cutting Edge: Defective Aerobic Glycolysis Defines the Distinct Effector Function in Antigen-Activated CD8 + Recent Thymic Emigrants. J Immunol (2017) 198:4575–80. doi: 10.4049/jimmunol.1700465
25. Cano-Gamex E, Soskic B, Roumeliotis TI, So E, Smyth DJ. Single-Cell Transcriptomics Identifies Aneffectorness Gradient Shaping the Responseof CD4+T Cells to Cytokines. Nat Commun (2020) 11:1801. doi: 10.1038/s41467-020-15543-y
26. Wang X, Shen X, Chen S, Liu H, Hong N, Zhong H, et al. Reinvestigation of Classic T Cell Subsets and Identification of Novel Cell Subpopulations by Single-Cell RNA Sequencing. J Immunol (2021) 208(2):396–406. doi: 10.1101/2021.02.11.430754
27. Gutierrez-Arcelus M, Teslovich N, Mola AR, Polidoro RB, Nathan A, Kim H, et al. Lymphocyte Innateness Defined by Transcriptionalstates Reflects a Balance Between Proliferation and Effector Functions. Nat Commun (2019) 10:687. doi: 10.1038/s41467-019-08604-4
28. Li N, van Unen V, Abdelaal T, Guo N, Kasatskaya SA, Ladell K, et al. Memory Cd4+T Cells are Generated in the Human Fetal Intestine. Nat Immunol (2019) 20:301–12. doi: 10.1038/s41590-018-0294-9
29. Onoe T, Chittenden M, Zhao G, Yang Y-G, Sykes M. Homeostatic Expansion and Phenotypic Conversion of Human T Cells Depend on Peripheral Interactions With APC. J Immunol (2010) 184:6756–65. doi: 10.4049/jimmunol.0901711
30. Azevedo RI, Soares MV, Albuquerque AS, Tendeiro R, Soares RS, Martins M, et al. Long-Term Immune Reconstitution of Naive and Memory T Cell Pools After Haploidentical Hematopoietic Stem Cell Transplantation. Biol Blood Marrow Transpl (2013) 19:703–12. doi: 10.1016/j.bbmt.2013.01.017
31. Oehen S, Brduscha-Riem K. Naive Cytotoxic T Lymphocytes Spontaneously Acquire Effector Function in Lymphocytopenic Recipients: A Pitfall for T Cell Memory Studies? Eur J Immunol (1999) 29:608–14. doi: 10.1002/(SICI)1521-4141(199902)29:02<608::AID-IMMU608>3.0.CO;2-A
32. Ernst B, Lee DS, Chang JM, Sprent J, Surh CD. The Peptide Ligands Mediating Positive Selection in the Thymus Control T Cell Survival and Homeostatic Proliferation in the Periphery. Immunity (1999) 11:173–81. doi: 10.1016/S1074-7613(00)80092-8
33. Ge Q, Rao VP, Cho BK, Eisen HN, Chen J. Dependence of Lymphopenia-Induced T Cell Proliferation on the Abundance of Peptide/MHC Epitopes and Strength of Their Interaction With T Cell Receptors. Proc Natl Acad Sci USA (2001) 98:1728–33. doi: 10.1073/pnas.98.4.1728
34. Castermans E, Hannon M, Dutrieux J, Humblet-Baron S, Seidel L, Cheynier R, et al. Thymic Recovery After Allogeneic Hematopoietic Cell Transplantation With Non-Myeloablative Conditioning Is Limited to Patients Younger Than 60 Years of Age. Haematologica (2011) 96:298–306. doi: 10.3324/haematol.2010.029702
35. Talvensaari K, Clave E, Douay C, Rabian C, Garderet L, Busson M, et al. A Broad T-Cell Repertoire Diversity and an Efficient Thymic Function Indicate a Favorable Long-Term Immune Reconstitution After Cord Blood Stem Cell Transplantation. Blood (2002) 99:1458–64. doi: 10.1182/blood.V99.4.1458
36. Emerson R, Nikiforow S, Milano F. TCR Repertoire Diversity Assessed With Immunosequencing Is Associated With Patient Mortality Following Cord Blood Transplant. Blood (2014) 124:583349. doi: 10.1182/blood.V124.21.1262.1262
37. Törlén J, Gaballa A, Remberger M, Mörk LM, Sundberg B, Mattsson J, et al. Effect of Graft-Versus-Host Disease Prophylaxis Regimens on T and B Cell Reconstitution After Allogeneic Hematopoietic Stem Cell Transplantation. Biol Blood Marrow Transpl (2019) 25:1260–8. doi: 10.1016/j.bbmt.2019.01.029
38. Komanduri KV, St John LS, de Lima M, McMannis J, Rosinski S, McNiece I, et al. Delayed Immune Reconstitution After Cord Blood Transplantation is Characterized by Impaired Thymopoiesis and Late Memory T-Cell Skewing. Blood (2007) 110:4543–51. doi: 10.1182/blood-2007-05-092130
39. Mackall CL, Fleisher TA, Brown MR, Andrich MP, Chen CC Age, Thymopoiesis, and CD4+ T-Lymphocyte Regeneration After Intensive Chemotherapy. N Engl J Med (1995) 332:143–9. doi: 10.1056/NEJM199501193320303
40. Szabolcs P, Park KD, Reese M, Marti L, Broadwater G, Kurtzberg J., et al. Coexistent Naïve Phenotype and Higher Cycling Rate of Cord Blood T Cells as Compared to Adult Peripheral Blood. Exp Hematol (2003) 31:708–14. doi: 10.1016/S0301-472X(03)00160-7
41. Mold JE, Venkatasubrahmanyam S, Burt TD, Michaëlsson J, Rivera JM, Galkina SA, et al. Fetal and Adult Hematopoietic Stem Cells Give Rise to Distinct T Cell Lineages in Humans. Science (2010) 330:1695–9. doi: 10.1126/science.1196509
42. Hiwarkar P, Hubank M, Qasim W, Chiesa R, Gilmour KC, Saudemont A, et al. Cord Blood Transplantation Recapitulates Fetal Ontogeny With a Distinct Molecular Signature That Supports CD4+ T-Cell Reconstitution. Blood Adv (2017) 1:2206–16. doi: 10.1182/bloodadvances.2017010827
43. Stikvoort A, Gaballa A, Solders M, Nederlof I, Önfelt B, Sundberg B, et al. Risk Factors for Severe Acute Graft-Versus-Host Disease in Donor Graft Composition. Biol Blood Marrow Transpl (2018) 24:467–77. doi: 10.1016/j.bbmt.2017.11.026
44. Tischer S, Bunse C, Bak S, Oelke M, Figueiredo C, Thomas S, et al. Impact of G-CSF Mobilization on T-Cell Functionality: New Aspects for Adoptive Immunotherapy. Cytotherapy (2017) 19:S82. doi: 10.1016/j.jcyt.2017.02.137
45. Hauri-Hohl MM, Keller MP, Gill J, Hafen K, Pachlatko E, Boulay T, et al. Donor T-Cell Alloreactivity Against Host Thymic Epithelium Limits T-Cell Development After Bone Marrow Transplantation. Blood (2007) 109:4080–8. doi: 10.1182/blood-2006-07-034157
46. Wils EJ, van der Holt B, Broers AE, Posthumus-van Sluijs SJ, Gratama JW, Braakman E, et al. Insufficient Recovery of Thymopoiesis Predicts for Opportunistic Infections in Allogeneic Hematopoietic Stem Cell Transplant Recipients. Haematologica (2011) 96:1846–54. doi: 10.3324/haematol.2011.047696
47. Waller EK, Logan BR, Fei M, Lee SJ, Confer D, Howard A Kinetics of Immune Cell Reconstitution Predict Survival in Allogeneic Bone Marrow and G-CSF–mobilized Stem Cell Transplantation. Blood Adv (2019) 3:2250–63. doi: 10.1182/bloodadvances.2018029892
48. De Koning C, Admiraal R, Nierkens S, Boelens JJ. Human Herpesvirus 6 Viremia Affects T-Cell Reconstitution After Allogeneic Hematopoietic Stem Cell Transplantation. Blood Adv (2018) 2:428–32. doi: 10.1182/bloodadvances.2017012724
49. de Koning C, Plantinga M, Besseling P, Boelens JJ, Nierkens S. Immune Reconstitution After Allogeneic Hematopoietic Cell Transplantation in Children. Biol Blood Marrow Transpl (2016) 22:195–206. doi: 10.1016/j.bbmt.2015.08.028
50. Yakoub-Agha I, Saule P, Depil S, Grutzmacher C, Boulanger F, Magro L, et al. Comparative Analysis of Naïve and Memory CD4+ and CD8+ T-Cell Subsets in Bone Marrow and G-CSF-Mobilized Peripheral Blood Stem Cell Allografts: Impact of Donor Characteristics. Exp Hematol (2007) 35:861–71. doi: 10.1016/j.exphem.2007.03.006
51. Chérel M, Choufi B, Trauet J, Cracco P, Dessaint JP, Yakoub-Agha I, et al. Naïve Subset Develops the Most Important Alloreactive Response Among Human CD4+ T Lymphocytes in Human Leukocyte Antigen-Identical Related Setting. Eur J Haematol (2014) 92:491–6. doi: 10.1111/ejh.12283
52. Divito SJ, Aasebø AT, Matos TR, Hsieh PC, Collin M, Elco CP, et al. Peripheral Host T Cells Survive Hematopoietic Stem Cell Transplantation and Promote Graft-Versus-Host Disease. J Clin Invest (2020) 130:4624–36. doi: 10.1172/JCI129965
53. D’Souza A, Fretham C. (2019). Current Uses and Outcomes of Hematopoietic Cell Transplantation (HCT): CIBMTR Summary Slides.
54. Sarantopoulos S, Ritz J. T Cell– and B Cell–Mediated Pathology in Chronic Graft-Versus-Host Disease. Immune Biology of Allogeneic Hematopoietic Stem Cell Transplantation. Biol Blood Marrow Transpl (2019) 21(1):16–23. doi: 10.1016/b978-0-12-812630-1.00015-3
55. Jagasia M, Arora M, Flowers ME, Chao NJ, McCarthy PL, Cutler CS, et al. Risk Factors for Acute GVHD and Survival After Hematopoietic Cell Transplantation. Blood (2012) 119:296–307. doi: 10.1182/blood-2011-06-364265
56. Berger M, Lanino E, Cesaro S, Zecca M, Vassallo E, Faraci M, et al. Feasibility and Outcome of Haploidentical Hematopoietic Stem Cell Transplantation With Post-Transplant High-Dose Cyclophosphamide for Children and Adolescents With Hematologic Malignancies: An AIEOP-GITMO Retrospective Multicenter Study. Biol Blood Marrow Transpl (2016) 22:902–9. doi: 10.1016/j.bbmt.2016.02.002
57. Locatelli F, Merli P, Pagliara D, Li Pira G, Falco M, Pende D, et al. Outcome of Children With Acute Leukemia Given HLA-Haploidentical HSCT After Ab T-Cell and B-Cell Depletion. Blood (2017) 130:677–85. doi: 10.1182/blood-2017-04-779769
58. Chang YJ, Zhao XY, Huo MR, Huang XJ. Expression of CD62L on Donor CD4+ T Cells in Allografts: Correlation With Graft-Versus-Host Disease After Unmanipulated Allogeneic Blood and Marrow Transplantation. J Clin Immunol (2009) 29:696–704. doi: 10.1007/s10875-009-9293-9
59. Soares MV, Azevedo RI, Ferreira IA, Bucar S, Ribeiro AC, Vieira A, et al. Naive and Stem Cell Memory T Cell Subset Recovery Reveals Opposing Reconstitution Patterns in CD4 and CD8 T Cells in Chronic Graft vs. Host Disease. Front Immunol (2019) 10. doi: 10.3389/fimmu.2019.00334
60. Lindemans CA, Martelsmann A, O'Cannor M, Calafiore M, Dudakov JA, Jeng R, et al. IL-22 Directly Regulates Intestinal Stem Cells, Protecting Epithelium From GvHD and Reducing GvHD Mortality. Biol Blood Marrow Transpl (2015) 21:S58–9. doi: 10.1016/j.bbmt.2014.11.058
61. Dutt S, Tseng D, Ermann J, George TI, Liu YP, Davis CR, et al. Naive and Memory T Cells Induce Different Types of Graft-Versus-Host Disease. J Immunol (2007) 179:6547–54. doi: 10.4049/jimmunol.179.10.6547
62. Anderson BE, McNiff J, Yan J, Doyle H, Mamula M, Shlomchik MJ, et al. Memory CD4+ T Cells Do Not Induce Graft-Versus-Host Disease. J Clin Invest (2003) 112:101–8. doi: 10.1172/JCI17601
63. Foster AE, Marangolo M, Sartor MM, Alexander SI, Hu M, Bradstock KF, et al. Human CD62L- Memory T Cells Are Less Responsive to Alloantigen Stimulation Than CD62L+ Naive T Cells: Potential for Adoptive Immunotherapy and Allodepletion. Blood (2004) 104:2403–9. doi: 10.1182/blood-2003-12-4431
64. Loschi M, Porcher R, Peffault deLatour R, Vanneaux V, Robin M, Xhaard A, et al. High Number of Memory T Cells Is Associated With Higher Risk of Acute Graft-Versus-Host Disease After Allogeneic Stem Cell Transplantation. Biol Blood Marrow Transpl (2015) 21:569–74. doi: 10.1016/j.bbmt.2014.12.009
65. Fujii S, Miura Y, Fujishiro A, Shindo T, Shimazu Y, Hirai H, et al. Graft-Versus-Host Disease Amelioration by Human Bone Marrow Mesenchymal Stromal/Stem Cell-Derived Extracellular Vesicles Is Associated With Peripheral Preservation of Naive T Cell Populations. Stem Cells (2018) 36:434–45. doi: 10.1002/stem.2759
66. Beilhaek A, Schulz S, Baker J, Beilhack GF, Nishimura R, Baker EM, et al. Prevention of Acute Graft-Versus-Host Disease by Blocking T-Cell Entry to Secondary Lymphoid Organs. Blood (2008) 111:2919–28. doi: 10.1182/blood-2007-09-112789
67. Cooke KR, Luznik L, Sarantopoulos S, Hakim FT, Jagasia M, Fowler DH, et al. The Biology of Chronic Graft-Versus-Host Disease: A Task Force Report From the National Institutes of Health Consensus Development Project on Criteria for Clinical Trials in Chronic Graft-Versus-Host Disease HHS Public Access. Biol Blood Marrow Transpl (2017) 23:211–34. doi: 10.1016/j.bbmt.2016.09.023
68. Goldman JA, Gale RP, Horowitz MM, Biggs JC, Champlin RE, Gluckman E, et al. Bone Marrow Transplantation for Chronic Myelogenous Leukemia in Chronic Phase. Increased Risk for Relapse Associated With T-Cell Depletion. Ann Intern Med (1988) 108:806–14. doi: 10.7326/0003-4819-108-6-806
69. Matte-Martone C, Liu J, Jain D, McNiff J, Shlomchik WD. CD8 + But Not CD4 + T Cells Require Cognate Interactions With Target Tissues to Mediate GVHD Across Only Minor H Antigens, Whereas Both CD4 + and CD8 + T Cells Require Direct Leukemic Contact to Mediate GVL. Blood (2008) 111:3884–92. doi: 10.1182/blood-2007-11-125294
70. De Koning C, Nierkens S, Boelens JJ. Strategies Before, During, and After Hematopoietic Cell Transplantation to Improve T-Cell Immune Reconstitution. Blood (2016) 128:2607–15. doi: 10.1182/blood-2016-06-724005
71. Fukushi N, Arase H, Wang B, Ogasawara K, Gotohda T, Good RA, et al. Thymus: A Direct Target Tissue in Graft-Versus-Host Reaction After Allogeneic Bone Marrow Transplantation That Results in Abrogation of Induction of Self-Tolerance. Proc Natl Acad Sci USA (1990) 87:6301–5. doi: 10.1073/pnas.87.16.6301
72. Muffly L, Sheehan K, Armstrong R, Jensen K, Tate K, Rezvani AR, et al. Infusion of Donor-Derived CD8+ Memory T Cells for Relapse Following Allogeneic Hematopoietic Cell Transplantation. Blood Adv (2018) 2:681–90. doi: 10.1182/bloodadvances.2017012104
73. Maung KK, Chen BJ, Barak I, Li Z, Rizzieri DA, Gasparetto C, et al. Phase I Dose Escalation Study of Naive T-Cell Depleted Donor Lymphocyte Infusion Following Allogeneic Stem Cell Transplantation. Bone Marrow Transpl (2021) 56:137–43. doi: 10.1038/s41409-020-0991-5
74. Triplett BM, Muller B, Kang G, Li Y, Cross SJ, Moen J, et al. Selective T-Cell Depletion Targeting CD45RA Reduces Viremia and Enhances Early T-Cell Recovery Compared With CD3-Targeted T-Cell Depletion. Transpl Infect Dis (2018) 20:10.1111/tid.12823. doi: 10.1111/tid.12823
75. Milano F, Lee Nelson J, Delaney C. Fetal Maternal Immunity and Antileukemia Activity in Cord-Blood Transplant Recipients. Bone Marrow Transpl (2013) 48:321–2. doi: 10.1038/bmt.2012.157
76. Huang W, Chao NJ. Memory T Cells: A Helpful Guard for Allogeneic Hematopoietic Stem Cell Transplantation Without Causing Graft-Versus-Host Disease. Hematol Oncol Stem Cell Ther (2017) 10:211–9. doi: 10.1016/j.hemonc.2017.05.006
77. Barnes D, Corp M, Loutit J, Neal F. Treatment of Murine Leukaemia With X Rays and Homologous Bone Marrow; Preliminary Communication. Br Med J (1956) 2:626–7. doi: 10.1136/bmj.2.4993.626
78. Horowitz MM, Gale RP, Sondel PM, Goldman JM, Kersey J, Kolb HJ, et al. Graft-Versus-Leukemia Reactions After Bone Marrow Transplantation. Blood (1990) 75:555–62. doi: 10.1182/blood.V75.3.555.555
79. Matte-Martone C, Venkatesan S, Tan HS, Athanasiadis I, Chang J, Pavisic J, et al. GVL Against Mouse BC-CML and CP-CML: Shared Mechanisms of T Cell Killing, But PD-Ligands Render CP-CML and Not BC-CML GVL-Resistant. J Immunol (2011) 187:1653. doi: 10.4049/jimmunol.1100311
80. Guo H, Chang YJ, Hong Y, Xu LP, Wang Y, Zhang XH, et al. Dynamic Immune Profiling Identifies the Stronger Graft-Versus-Leukemia (GVL) Effects With Haploidentical Allografts Compared to HLA-Matched Stem Cell Transplantation. Cell Mol Immunol (2021) 18:1172–85. doi: 10.1038/s41423-020-00597-1
81. Chang Y, Wang Y, Xu LP, Zhang XH, Chen H, Chen YH, et al. Haploidentical Donor is Preferred Over Matched Sibling Donor for Pre-Transplantation MRD Positive ALL: A Phase 3 Genetically Randomized Study. J Hematol Oncol (2020) 13:27. doi: 10.1186/s13045-020-00860-y
82. Luo Y, Xiao H, Lai X, Shi J, Tan Y, He J, et al. T-Cell-Replete Haploidentical HSCT With Low-Dose Anti-T-Lymphocyte Globulin Compared With Matched Sibling HSCT and Unrelated HSCT. Blood (2014) 124:2735–43. doi: 10.1182/blood-2014-04-571570
83. Chen BJ, Cui X, Sempowski GD, Liu C, Chao NJ. Transfer of Allogeneic CD62L- Memory T Cells Without Graft-Versus-Host Disease. Blood (2004) 103:1534–41. doi: 10.1182/blood-2003-08-2987
84. Zheng H, Matte-Martone C, Li H, Anderson BE, Venketesan S Effector Memory CD4 T Cells Mediate Graft-Versus-Leukemia Without Inducing Graft-Versus-Host Disease. Blood (2008) 111(4):2476–84. doi: 10.1182/blood-2007-08-109678
85. Li N, Matte-Martone C, Zheng H, Cui W, Venkatesan S, Tan HS, et al. Memory T Cells From Minor Histocompatibility Antigen-Vaccinated and Virus-Immune Donors Improve GVL and Immune Reconstitution. Blood (2011) 118:5965–76. doi: 10.1182/blood-2011-07-367011
86. Anderson BE, Taylor PA, McNiff JM, Jain D, Demetris AJ, Panoskaltsis-Mortari A, et al. Effects of Donor T-Cell Trafficking and Priming Site on Graft-Versus-Host Disease Induction by Naive and Memory Phenotype CD4 T Cells. Blood (2008) 111:5242–51. doi: 10.1182/blood-2007-09-107953
87. Milano F, Appelbaum FR, Delaney C. Cord-Blood Transplantation in Patients With Minimal Residual Disease. N Engl J Med (2016) 375:944–53. doi: 10.1056/NEJMoa1602074
88. Eapen M, Rubinstein P, Zhang MJ, Stevens C, Kurtzberg J, Scaradavou A, et al. Outcomes of Transplantation of Unrelated Donor Umbilical Cord Blood and Bone Marrow in Children With Acute Leukaemia: A Comparison Study. Lancet (2007) 369:1947–54. doi: 10.1016/S0140-6736(07)60915-5
89. Brunstein CG, Gutman JA, Weisdorf DJ, Woolfrey AE, Defor TE, Gooley TA, et al. Allogeneic Hematopoietic Cell Transplantation for Hematologic Malignancy: Relative Risks and Benefits of Double Umbilical Cord Blood. Blood (2010) 116:4693–9. doi: 10.1182/blood-2010-05-285304
90. Hiwarkar P, Qasim W, Ricciardelli I, Gilmour K, Quezada S, Saudemont A, et al. Cord Blood T Cells Mediate Enhanced Antitumor Effects Compared With Adult Peripheral Blood T Cells. Blood (2015) 126:2882–91. doi: 10.1182/blood-2015-06-654780
91. van Heijst JWJ, Ceberio I, Lipuma LB, Samilo DW, Wasilewski GD, Gonzales AM, et al. Quantitative Assessment of T Cell Repertoire Recovery After Hematopoietic Stem Cell Transplantation. Nat Med (2013) 19:372–7. doi: 10.1038/nm.3100
92. Greinix HT, Kuzmina Z, Weigl R, Körmoczi U, Rottal A, Wolff D, et al. CD19+CD21low B Cells and CD4+CD45RA+CD31+ T Cells Correlate With First Diagnosis of Chronic Graft-Versus-Host Disease. Biol Blood Marrow Transpl (2015) 21:250–8. doi: 10.1016/j.bbmt.2014.11.010
93. Bohmann EM, Fehn U, Holler B, Weber D, Holler E, Herr W, et al. Altered Immune Reconstitution of B and T Cells Precedes the Onset of Clinical Symptoms of Chronic Graft-Versus-Host Disease and Is Influenced by the Type of Onset. Ann Hematol (2017) 96:299–310. doi: 10.1007/s00277-016-2881-x
94. Thus KA, de Hoop TA, de Weger RA, Bierings MB, Boelens JJ, Spierings E, et al. Predicted Indirectly ReCognizable HLA Epitopes Class I Promote Antileukemia Responses After Cord Blood Transplantation: Indications for a Potential Novel Donor Selection Tool. Biol Blood Marrow Transpl (2016) 22:170–3. doi: 10.1016/j.bbmt.2015.08.014
95. Langenhorst JB, van Kesteren C, van Maarseveen EM, Dorlo TPC, Nierkens S, Lindemans CA, et al. Fludarabine Exposure in the Conditioning Prior to Allogeneic Hematopoietic Cell Transplantation Predicts Outcomes. Blood Adv (2019) 3:2179–87. doi: 10.1182/bloodadvances.2018029421
96. Kong F, Chen CH, Cooper MD. Reversible Disruption of Thymic Function by Steroid Treatment. J Immunol (2002) 168:6500–5. doi: 10.4049/jimmunol.168.12.6500
97. Ito A, Kitano S, Tajima K, Kim Y, Tanaka T, Inamoto Y, et al. Impact of Low-Dose Anti-Thymocyte Globulin on Immune Reconstitution After Allogeneic Hematopoietic Cell Transplantation. Int J Hematol (2020) 111:120–30. doi: 10.1007/s12185-019-02756-1
98. Roberto A, Castagna L, Zanon V, Bramanti S, Crocchiolo R, McLaren JE, et al. Role of Naive-Derived T Memory Stem Cells in T-Cell Reconstitution Following Allogeneic Transplantation. Blood (2015) 125:2855–64. doi: 10.1182/blood-2014-11-608406
99. Admiraal R, Nierkens S, Bierings MB, Bredius RGM, van Vliet I, Jiang Y, et al. Individualised Dosing of Anti-Thymocyte Globulin in Paediatric Unrelated Allogeneic Haematopoietic Stem-Cell Transplantation (PARACHUTE): A Single-Arm, Phase 2 Clinical Trial. Lancet Haematol (2022) 9:e111–20. doi: 10.1016/S2352-3026(21)00375-6
100. Li B, New JY, Tay YK, Goh E, Yap EH, Chan SH, et al. Delaying Acute Graft-Versus-Host Disease in Mouse Bone Marrow Transplantation by Treating Donor Cells With Antibodies Directed at L-Selectin and α4-Integrin Prior to Infusion. Scand J Immunol (2004) 59:464–8. doi: 10.1111/j.0300-9475.2004.01414.x
101. Xystrakis E, Bernard I, Dejean AS, Alsaati T, Druet P, Saoudi A, et al. Alloreactive CD4 T Lymphocytes Responsible for Acute and Chronic Graft-Versus-Host Disease are Contained Within the CD45RChigh But Not the CD456RClow Subset. Eur J Immunol (2004) 34:408–17. doi: 10.1002/eji.200324528
102. Huang W, Mo W, Jiang J, Chao NJ, Chen BJ. Donor Allospecific CD44high Central Memory T Cells Have Decreased Ability to Mediate Graft-vs.-Host Disease. Front Immunol (2019) 10:1–9. doi: 10.3389/fimmu.2019.00624
103. Perez-Martinez A, Gasior M, Bueno D, Badell I, Sissini L, Torrent M, et al. Naive T-Cell Depleted Allografts-Graft Engineered Hematopoietic Stem Cell Transplant in Pediatric Patients. Biol Blood Marrow Transpl (2018) 24:S203–4. doi: 10.1016/j.bbmt.2017.12.038
104. Anasetti C, Logan BR, Lee SJ, Waller EK, Weisdorf DJ, Wingard JR, et al. Peripheral-Blood Stem Cells Versus Bone Marrow From Unrelated Donors. N Engl J Med (2012) 367:1487–96. doi: 10.1056/NEJMoa1203517
105. Pasquini MC, Devine S, Mendizabal A, Baden LR, Wingard JR, Lazarus HM, et al. Comparative Outcomes of Donor Graft CD34+ Selection and Immune Suppressive Therapy as Graft-Versus-Host Disease Prophylaxis for Patients With Acute Myeloid Leukemia in Complete Remission Undergoing HLA-Matched Sibling Allogeneic Hematopoietic Cell Transpl. J Clin Oncol (2012) 30:3194–201. doi: 10.1200/JCO.2012.41.7071
106. Guerrero A, Riddell SR, Storek J, Stevens-Ayers T, Storer B, Zaia JA, et al. Cytomegalovirus Viral Load and Virus-Specific Immune Reconstitution After Peripheral Blood Stem Cell Versus Bone Marrow Transplantation. Biol Blood Marrow Transpl (2012) 18:66–75. doi: 10.1016/j.bbmt.2011.05.010
107. Roux E, Dumont-Girard F, Starobinski M, Siegrist CA, Helg C, Chapuis B, et al. Recovery of Immune Reactivity After T-Cell-Depleted Bone Marrow Transplantation Depends on Thymic Activity. Blood (2000) 96:2299–303. doi: 10.1182/blood.V96.6.2299
108. Bejanyan N, Brunstein CG, Cao Q, Lazaryan A, Luo X, Curtsinger J, et al. Delayed Immune Reconstitution After Allogeneic Transplantation Increases the Risks of Mortality and Chronic GVHD. Blood Adv (2018) 2:909–22. doi: 10.1182/bloodadvances.2017014464
109. Velardi E, Tsai JJ, van den Brink MRM. T Cell Regeneration After Immunological Injury. Nat Rev Immunol (2021) 21:1. doi: 10.1038/s41577-020-00457-z
110. Wils EJ, Aerts-Kaya FS, Rombouts EJ, van Mourik I, Rijken-Schelen A, Visser TP, et al. Keratinocyte Growth Factor and Stem Cell Factor to Improve Thymopoiesis After Autologous CD34 + Cell Transplantation in Rhesus Macaques. Biol Blood Marrow Transpl (2012) 18:55–65. doi: 10.1016/j.bbmt.2011.09.010
111. Dudakov JA, Hanash AM, van den Brink MRM. Interleukin-22: Immunobiology and Pathology. Annu Rev Immunol (2015) 33:747–85. doi: 10.1146/annurev-immunol-032414-112123
112. Perales MA, Goldberg JD, Yuan J, Koehne G, Lechner L, Papadopoulos EB, et al. Recombinant Human Interleukin-7 (CYT107) Promotes T-Cell Recovery After Allogeneic Stem Cell Transplantation. Blood (2012) 120:4882–91. doi: 10.1182/blood-2012-06-437236
113. van Lent AU, Dontje W, Nagasawa M, Siamari R, Bakker A, Pouw S, et al. IL-7 Enhances Thymic Human T Cell Development in “Human Immune System” Rag2 –/– IL-2rγ C –/– Mice Without Affecting Peripheral T Cell Homeostasis. J Immunol (2009) 183:7645–55. doi: 10.4049/jimmunol.0902019
114. Rossi S, Blazar BR, Farrell CL, Danilenko DM, Lacey DL, Weinberg KI, et al. Keratinocyte Growth Factor Preserves Normal Thymopoiesis and Thymic Microenvironment During Experimental Graft-Versus-Host Disease. Blood (2002) 100:682–91. doi: 10.1182/blood.V100.2.682
115. Min D, Taylor PA, Panoskaltsis-Mortari A, Chung B, Danilenko DM, Farrell C, et al. Protection From Thymic Epithelial Cell Injury by Keratinocyte Growth Factor: A New Approach to Improve Thymic and Peripheral T-Cell Reconstitution After Bone Marrow Transplantation. Blood (2002) 99:4592–600. doi: 10.1182/blood.V99.12.4592
116. Perruccio K, Bonifazi P, Topini F, Tosti A, Bozza S, Aloisi T, et al. Thymosin α1 to Harness Immunity to Pathogens After Haploidentical Hematopoietic Transplantation. Ann N Y Acad Sci (2010) 1194:153–61. doi: 10.1111/j.1749-6632.2010.05486.x
Keywords: allogeneic hematopoietic cell transplantation, naive T cells, graft versus host disease, graft versus leukemia, alloreactivity
Citation: Dekker L, Sanders E, Lindemans CA, de Koning C and Nierkens S (2022) Naive T Cells in Graft Versus Host Disease and Graft Versus Leukemia: Innocent or Guilty? Front. Immunol. 13:893545. doi: 10.3389/fimmu.2022.893545
Received: 10 March 2022; Accepted: 18 May 2022;
Published: 20 June 2022.
Edited by:
Niels Olsen Saraiva Camara, University of São Paulo, BrazilReviewed by:
Ying-Jun Chang, Peking University People’s Hospital, ChinaSuhag Parikh, Emory University, United States
Qingxiao Song, City of Hope National Medical Center, United States
Copyright © 2022 Dekker, Sanders, Lindemans, de Koning and Nierkens. This is an open-access article distributed under the terms of the Creative Commons Attribution License (CC BY). The use, distribution or reproduction in other forums is permitted, provided the original author(s) and the copyright owner(s) are credited and that the original publication in this journal is cited, in accordance with accepted academic practice. No use, distribution or reproduction is permitted which does not comply with these terms.
*Correspondence: Stefan Nierkens, Uy5OaWVya2Vucy0yQHByaW5zZXNtYXhpbWFjZW50cnVtLm5s
†These authors have contributed equally to this work
‡These authors have contributed equally to this work