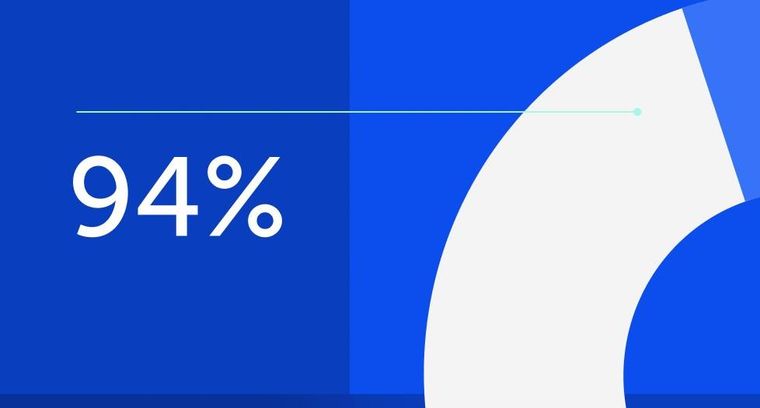
94% of researchers rate our articles as excellent or good
Learn more about the work of our research integrity team to safeguard the quality of each article we publish.
Find out more
ORIGINAL RESEARCH article
Front. Immunol., 20 May 2022
Sec. Vaccines and Molecular Therapeutics
Volume 13 - 2022 | https://doi.org/10.3389/fimmu.2022.892277
This article is part of the Research TopicInnate Immunity and Severe Asthma: From Microbiome to Target TherapyView all 8 articles
Coronavirus disease 2019 (COVID-19) vaccines effectively elicit humoral and cellular immunity against severe acute respiratory syndrome coronavirus 2 (SARS-CoV-2) in healthy populations. This immunity decreases several months after vaccination. However, the efficacy of vaccine-induced immunity and its durability in patients with severe asthma on biological therapy are unknown. In this study, we evaluated the effectiveness and durability of mRNA vaccine-induced SARS-CoV-2-specific humoral and cellular immunity in severe asthma patients on biological therapy. The study included 34 patients with severe asthma treated with anti-IgE (omalizumab, n=17), anti-IL5 (mepolizumab, n=13; reslizumab, n=3), or anti-IL5R (benralizumab, n=1) biological therapy. All patients were vaccinated with two doses of the BNT162b2 mRNA vaccine with a 6-week interval between the doses. We found that this COVID-19 vaccination regimen elicited SARS-CoV-2-specific humoral and cellular immunity, which had significantly declined 6 months after receipt of the second dose of the vaccine. The type of biological treatment did not affect vaccine-elicited immunity. However, patient age negatively impacted the vaccine-induced humoral response. On the other hand, no such age-related impact on vaccine-elicited cellular immunity was observed. Our findings show that treatment of patients with severe asthma with biological therapy does not compromise the effectiveness or durability of COVID-19 vaccine-induced immunity.
Severe acute respiratory syndrome coronavirus 2 (SARS-CoV-2) is the cause of the COVID-19 pandemic, and the efforts to fight this disease largely rely on prophylactic vaccination (1). The currently used COVID-19 vaccines provide a high level of protection against SARS-CoV-2 infection and severe forms of COVID-19 (2–6). These COVID-19 vaccines induce strong humoral and cellular responses in healthy populations (7). The humoral response leads to the production of anti-SARS-CoV-2 antibodies, which prevent the virus from infecting host cells and spreading in the body (8, 9). The cellular response, which largely relies on cytotoxic and memory T cells, prevents the virus from replicating in infected cells and protects patients from severe forms of COVID-19 (10–13). Despite the high performance of the current COVID-19 vaccines in eliciting robust protective immunity, the durability of this immunity, both humoral and cellular, has been found to be much shorter than previously expected (14–16). This finding led to a change in the vaccination strategy and administration of booster doses to reinvigorate antiviral immunity (17).
Unlike healthy populations, patients with many diseases have shown decreased efficacy for COVID-19 vaccines in eliciting protective immunity. This has been shown, for instance, in recipients of solid-organ transplants (18, 19), cancer patients (20), patients on dialysis (21), and patients receiving certain drugs (22). In addition to the compromised immunogenicity of current vaccines in these patients, the durability of the elicited immunity is still largely unknown.
Patients with severe asthma on biological treatment were initially expected to be at higher risk of SARS-CoV-2 infection and severe COVID-19 (23, 24). More recent studies, however, have not supported this expectation (25–27). Currently, there is no definite consensus on whether patients on these treatment plans have higher risks of SARS-CoV-2 infection and severe disease than the healthy population, and therefore, an individualized approach is recommended for these patients (28).
A recent study showed that vaccination of patients with severe asthma on biological therapy with the BNT162b2 mRNA vaccine was safe and tolerated, with a minimal impact on the control of their primary disease (29). However, the immunogenicity of this vaccine in these patients has not been evaluated.
In the present study, we evaluated the immunogenicity of the BNT162b2 mRNA vaccine and the durability of vaccine-elicited immunity in a cohort of 34 severe asthma patients on biological therapy, which is already a centralized standard of care in the Czech Republic. This cohort included patients on anti-IgE (omalizumab), anti-IL5 (mepolizumab, reslizumab), or anti-IL5R (benralizumab) therapy (30, 31). The immunogenicity of the vaccine was evaluated by assessing specific humoral and cellular responses after the administration of the first and second doses of the vaccine. The persistence of the elicited immunity was assessed six months after the administration of the second dose of the vaccine.
The inclusion criteria for patients for this study were no reported history of COVID-19 and no previously reported positivity for SARS-CoV-2. The study enrolled 37 patients with severe asthma who were on biological therapy and previously planned to undergo voluntary vaccination against COVID-19 with the BNT162b2 mRNA vaccine (Comirnaty, Pfizer-BioNTech, Mainz, Germany). Severe asthma patients on biological therapy underwent clinical and laboratory monitoring at regular three-month intervals at specialized allergy and pneumology centers of the Motol University Hospital in Prague. Blood samples were obtained between April 2021 and November 2021. The planned COVID-19 vaccination regimen included 2 doses of the BNT162b2 mRNA vaccine administered at a 6-week interval. According to the ongoing treatment regimen with monoclonal antibodies for severe asthma, a 48-h interval between COVID-19 vaccination and biologic administration was used. The patient samples for analysis included peripheral blood serum and unclotted peripheral blood and were collected during the planned health check-ups. A complete blood count with differential was performed. The serum and peripheral blood mononuclear cells (PBMCs) were isolated and cryopreserved as previously described (32, 33). Adverse reactions to mRNA vaccination were monitored. Each patient provided signed written informed consent for the use of their blood-derived products for future research. The study was approved according to the ethical standards of the institutional research committee – the Ethics Committee of the Motol University Hospital in Prague (EK-346/21) – and performed in compliance with the 1964 Helsinki Declaration and its later amendments or comparable ethical standards.
Patient sera were analyzed for the presence of anti-SARS-CoV-2 antibodies. Anti-SARS-CoV-2-spike glycoprotein receptor-binding domain (RBD) IgA and IgG antibodies were evaluated using IVD EIA COVID-19 RBD IgA or IgG (TestLine Clinical Diagnostics, Brno, Czech Republic). Anti-SARS-CoV-2 nucleocapsid protein (NCP) IgG antibodies were assessed using CLIA COVID-19 NP IgG (TestLine Clinical Diagnostics). The analyses were performed according to the manufacturer’s instructions. The levels of the specific antibodies are expressed according to the manufacturer’s instructions (in U/ml). The samples with U/ml values below 18 were considered negative, and levels above 18 U/ml were considered positive. Samples with antibody levels above the dynamic range of the method were reanalyzed using adequately diluted serum.
Cryopreserved PBMCs were processed as previously described with minor changes (33). Briefly, cells were reconstituted (2 × 106 cells/ml) overnight in human plasma serum-containing medium and then stimulated with overlapping peptides (0.5 µg/ml) of the SARS-CoV-2 spike glycoprotein (PepMix™ SARS-CoV-2 Spike Glycoprotein, cat.# PM-WCPV-S-1, JPT Peptide Technologies, Berlin, Germany). The stimulated cells were then cultured (37°C, 5% CO2) for 8 days in the presence of IL-2 (PeproTech, Cranbury, NJ) to enrich the peptide-reactive T cells.
Eight-day-enriched PBMCs were restimulated with SARS-CoV-2 spike glycoprotein-derived peptides (0.5 μg/ml) and cultured for 5 h in the presence of brefeldin A (BioLegend, San Diego, CA) added 1 h after stimulation was initiated. Samples stimulated with vehicle alone (the peptide solvent) were used as a control. After the stimulation, the cells were stained with a fixable live/dead stain, fixed, permeabilized, and stained with fluorophore-labelled CD3-, CD4-, CD8-, IFN-γ-, and TNF-α-specific antibodies as described previously (33). The stained cells were analyzed by flow cytometry (FACSAria II, Becton Dickinson, Heidelberg, Germany). FlowJo software (Tree Star, Ashland, OR) was used to analyze the acquired flow cytometry data. The percentages of the peptide-reactive cytokine-producing T-cell populations were calculated as the difference in the percentage of cytokine-producing T cells between the vehicle- and peptide-stimulated samples.
Values were calculated from the indicated sample size (n) using GraphPad Prism 6 (GraphPad Software, La Jolla, CA). The Wilcoxon matched-pair signed-rank test was used to calculate the statistical significance (NSp>0.05, *p<0.05, **p<0.01, ***p<0.001, ****p<0.0001) of differences between two groups. Matched-pair one-way ANOVA (Friedman test) with Dunn’s posttest or one-way ANOVA (Kruskal–Wallis test) with Dunn’s posttest was used to determine the statistical significance (NSp>0.05, *p<0.05, **p<0.01, ***p<0.001, ****p<0.0001) of differences among three or more groups. Spearman’s rank-order correlation coefficient (r) and the correlation’s statistical significance (p) were used to determine the associations between two variables. A p value below 0.05 was considered significant. Biorender.com was used to produce graphical images (accessed in January 2022, license number XM23WFJ35Q).
Thirty-seven patients with severe asthma were enrolled in the study. The inclusion criteria were no previous history of COVID-19 or positive tests for SARS-CoV-2 and ongoing management of the patient’s primary disease by biological therapy indicated according to GINA recommendations (34, 35). The cohort of 37 enrolled patients comprised 18 patients on omalizumab (anti-IgE therapy), 14 patients on mepolizumab (anti-IL5), 4 patients on reslizumab (anti-IL5), and 1 patient on benralizumab (anti-IL5R) therapy. The patients’ median age was 57 years (range 21–73 years), and the cohort included 22 women and 15 men. Patient baseline characteristics collected before the first COVID-19 vaccine dose are shown in Table 1 and further detailed in Table S1. All patients were administered two doses of the SARS-CoV-2 spike glycoprotein-based mRNA vaccine BNT162b2 with a 6-week interval between the two doses. We maintained a minimum interval of 48 h between COVID-19 vaccination and the administration of biologics. Samples were obtained within 1 week before the administration of the first and second doses of the vaccine and then 4 weeks and 6 months after the administration of the second dose of the vaccine (Figure 1A). Eighteen (49%) patients were completely free of any reactions. Nineteen (51%) patients reported commonly described side effects, most of which were classified as very common/common side effects and occurred after the second dose was administered. No differences were reported according to the ongoing biological therapy (data not shown).
Figure 1 The serum levels of anti-RBD IgA and IgG antibodies during and after vaccination. (A) The sample collection strategy. (B) Serum levels (U/ml) of anti-RBD IgA (left panel) and IgG (right panel) antibodies before the first (Pre) and second (1st) vaccine doses were administered and 4 weeks (2nd) and 6 months (6 mths) after the second vaccine dose was administered. (C) Correlations between the serum levels (U/ml) of anti-RBD IgA and IgG antibodies determined in (B) In B, box and whisker plots (2.5–97.5 percentile) are shown, and the significance of differences among the groups (Pre, 1st, 2nd, and 6 mths) is indicated (NSp>0.05, *p<0.05, **p<0.01, ****p<0.0001; n = 34; matched-pair one-way ANOVA with Dunn’s posttest). In C, Spearman’s rank-order correlation coefficient (r) and the significance (p value; n = 34) are indicated.
We first evaluated whether the 37 enrolled patients had been infected with SARS-CoV-2 before or during the study to eliminate interference with vaccine performance. The marker of a previous SARS-CoV-2 infection is the presence of anti-SARS-CoV-2 nucleocapsid protein (NCP) IgG antibodies in the serum (36). We found that before vaccination and 6 months after vaccination, 34 patients were negative (33 patients) or only borderline (1 patient) for anti-NCP IgG antibodies (Figure S1A). Among these 34 patients, only two patients had elevated prevaccination levels of anti-SARS-CoV-2 spike glycoprotein receptor-binding domain (RBD) IgA antibodies, and one patient had elevated prevaccination levels of anti-RBD IgG antibodies (Figure S1B). However, since these patients were negative for anti-NCP IgG antibodies and prepandemic antibodies raised against human seasonal coronaviruses were reported to cross-react with SARS-CoV-2 antigens (37), these patients were still included in the analyses. The patient with a borderline anti-NCP IgG antibody titer was also included, and this patient was, in contrast, found to be negative for prevaccination anti-RBD IgA and IgG antibodies (Figure S1C). The remaining 3 patients of the 37 enrolled patients had prevaccination levels of anti-RBD IgA and IgG antibodies that were also considered negative (Figure S1C). However, these 3 patients had elevated prevaccination levels of anti-NCP IgG antibodies, which were then decreased 6 months after vaccination, indicating a distant prevaccination SARS-CoV-2 infection in the past (36) (Figure S1A). Therefore, to minimize the impact of possible SARS-CoV-2 infection on the evaluation of COVID-19 vaccine performance in these patients during the study, we excluded these 3 patients from further analyses.
To determine COVID-19 vaccine performance in eliciting a humoral response in the tested patients, we analyzed the serum levels of anti-RBD IgA and IgG antibodies during and after vaccination. As shown in Figure 1B, the serum levels of anti-RBD IgA and IgG antibodies were significantly increased after the administration of the first dose of the vaccine. These serum levels were further and significantly increased after the administration of the second dose of the vaccine (Figure 1B). However, 6 months after the second dose of the vaccine was administered, these levels had returned to the levels observed after the administration of the first dose (Figure 1B). As shown in Figure 1C, the serum levels of anti-RBD IgA and IgG antibodies were found to correlate after the first dose of the vaccine was administered but not 4 weeks or 6 months after the second dose of the vaccine was administered. This finding indicated that the second dose of the vaccine promoted IgG antibody production more than IgA antibody production (Figure 1C). Collectively, the data show that the immunogenicity of the BNT162b2 mRNA vaccine is sufficient to induce strong humoral immunity in severe asthma patients on biological therapy but that this immunity then significantly declines within 6 months of the administration of the second dose of the vaccine.
Cellular immunity against SARS-CoV-2 is important for efficient protection against the virus (38). Unlike humoral immunity, which is mediated by antibodies that immediately neutralize the virus, cellular immunity can only mobilize against a virus after antigen challenge (39–41). This mobilization relies on both CD4+ T cells and CD8+ T cells recognizing appropriate target antigens and effectively proliferating after antigen recognition. To exclude the possibility that the biological therapies used to treat the patients compromised both of these abilities of T cells, we tested the cellular immunogenicity of the vaccine using peptides derived from the SARS-CoV-2 spike glycoprotein (33, 42). These peptides were used to enrich patient PBMCs in peptide-reactive TNFα-, IFNγ- or TNFα/IFNγ-producing CD4+ or CD8+ T-cell populations in vitro (Figure 2A).
Figure 2 Reactivity of enriched PBMCs to SARS-CoV-2 spike glycoprotein peptides. (A) The gating strategy for flow cytometry data (top panels). The frequencies of TNFα-, IFNγ-, or TNFα/IFNγ-producing CD4+ and CD8+ T cells in enriched PBMCs stimulated with vehicle (Vehicle-stim) or SARS-CoV-2 spike glycoprotein-derived peptides (CoV-2-stim) (bottom panels) determined by intracellular cytokine staining. The frequency of each peptide-reactive cytokine-producing T-cell population was calculated as the difference in the frequency of the cytokine-producing T cells between the vehicle- and peptide-stimulated samples. (B, C) The frequencies of peptide-reactive TNFα-, IFNγ-, or TNFα/IFNγ-producing CD4+ (B) and CD8+ (C) T cells before the first (Pre) and second (1st) vaccine doses were administered and 4 weeks (2nd) and 6 months (6 mths) after the second vaccine dose was administered. In B–C, box and whisker plots (2.5–97.5 percentile) are shown, and the significance of differences among the groups (Pre, 1st, 2nd, and 6 mths) is indicated (NSp > 0.05, *p < 0.05, **p < 0.01, ***p < 0.001, ****p < 0.0001; n = 34; matched-pair one-way ANOVA with Dunn’s posttest).
We found that COVID-19 vaccination enhanced the mobilization of SARS-CoV-2-reactive TNFα-producing CD4+ and CD8+ T cells, and this enhancement was significant after the second dose of the vaccine was administered (Figures 2B, C, top panels). However, this enhanced mobilization had significantly declined to prevaccination levels 6 months later (Figures 2B, C, top panels).
The mobilization of SARS-CoV-2-reactive IFNγ- or TNFα/IFNγ-producing CD4+ or CD8+ T-cell populations showed a tendency similar to that of TNFα-producing T cells (Figures 2B, C, middle and bottom panels), but the cohort data reached significance for only IFNγ-producing CD4+ T cells after the administration of the second dose of the vaccine (Figure 2B, middle panel). However, the data did show that the cellular mobilization of reactive cytokine-producing CD4+ and CD8+ T cells was significantly correlated (Figure 3), indicating polyfunctional responses by patient SARS-CoV-2-reactive T cells after antigen challenge. These data show that the immunogenicity of the BNT162b2 mRNA vaccine is sufficient to significantly promote cellular immunity in severe asthma patients on biological therapy but that this immunity, similar to humoral immunity, significantly declines within 6 months of the administration of the second dose of the vaccine.
Figure 3 The associations between SARS-CoV-2 spike glycoprotein peptide-specific CD4+ and CD8+ T-cell reactivity during and after vaccination. The correlations between the frequencies of peptide-reactive TNFα-, IFNγ-, or TNFα/IFNγ-producing CD4+ and CD8+ T cells before (1st) and 4 weeks (2nd) and 6 months (6 mths) after the second vaccine dose was administered. Spearman’s rank-order correlation coefficient (r) and the significance (p value; n = 34) are indicated.
The data showed that the COVID-19 vaccine induced both humoral and cellular immunity in severe asthma patients on biological therapy. Next, we analyzed whether there was a correlation between the extents of vaccine-elicited humoral and cellular immunity. We found that the serum levels of anti-RBD IgA and IgG antibodies minimally correlated with SARS-CoV-2-reactive CD4+ T cells (Figure S2). On the other hand, the correlations between antibody serum levels and SARS-CoV-2-reactive CD8+ T cells were more apparent (Figure 4). These findings showed that COVID-19-induced SARS-CoV-2-specific humoral immunity is more proportional to the CD8+ T-cell compartment.
Figure 4 The associations between SARS-CoV-2 spike glycoprotein peptide-reactive CD8+ T cells and the serum levels of anti-RBD antibodies during and after vaccination. (A, B) The correlations between the frequencies of peptide-reactive TNFα-, IFNγ-, or TNFα/IFNγ-producing CD8+ T cells and serum levels (U/ml) of anti-RBD IgA (A) or IgG (B) antibodies before (1st) and 4 weeks (2nd) and 6 months (6 mths) after the second vaccine dose was administered. Spearman’s rank-order correlation coefficient (r) and the significance (p value; n = 34) are indicated.
The COVID-19 vaccine effectively induced SARS-CoV-2-specific immunity in the studied cohort of 34 patients on biological therapy. We next analyzed whether the type of biological therapy impacted vaccine performance. We stratified the patients into 3 groups based on therapy type. The first group included 17 patients on anti-IgE therapy (omalizumab), the second group included 13 patients on anti-IL5 therapy (mepolizumab), and the third group included 3 patients on anti-IL5 therapy (reslizumab). One patient on a different drug, an anti-IL5R therapy (benralizumab), was excluded from these comparisons. Among the 3 groups, there were no significant differences in patient age or total IgE or eosinophil cationic protein (ECP) serum levels (Figure S3). The only difference in the tested parameters among the groups was the eosinophil count in the peripheral blood, which differed between the group treated with anti-IgE therapy (omalizumab) and the group treated with anti-IL5 therapy (mepolizumab) (Figure S3).
We found that the type of biological therapy had no significant impact on COVID-19 vaccine-elicited humoral immunity (Figure 5A). There was also no impact on vaccine-elicited cellular immunity (Figure 5B). These findings revealed that vaccine performance was similar in all patients regardless of the type of biologic received.
Figure 5 The impact of biological therapy on SARS-CoV-2 spike glycoprotein-specific humoral and cellular immunity during and after vaccination. (A) Patients were stratified into 3 groups based on the type of biological therapy they received. The groups were 17 patients on anti-IgE therapy (O; omalizumab), 13 patients on anti-IL5 therapy (M, mepolizumab), and 3 patients on anti-IL5 (R, reslizumab) therapy. The serum levels (U/ml) of anti-RBD IgA (top panels) and IgG (bottom panels) antibodies before (1st) and 4 weeks (2nd) and 6 months (6 mths) after the second vaccine dose was administered. (B) The frequencies of peptide-reactive TNFα-producing CD4+ (top panels) and CD8+ (bottom panels) T cells in the stratified groups in A before (1st) and 4 weeks (2nd) and 6 months (6 mths) after the second vaccine dose was administered. In (A, B), box and whisker plots (2.5–97.5 percentile) are shown, and the significance of differences among the groups (O, M, and R) is indicated (NSp > 0.05; n = 17 (O), 13 (M), or 3 (R); one-way ANOVA with Dunn’s posttest).
Thus far, we had not identified a clinical parameter that negatively affected COVID-19 vaccine performance in the studied cohort of severe asthma patients. We next analyzed whether any of the other clinical parameters evaluated affected vaccine-elicited humoral or cellular immunity. We found that the total IgE and ECP serum levels did not correlate with vaccine-elicited humoral (Figure S4) or cellular immunity (Figures S5, S6). Additionally, the peripheral blood eosinophil count did not correlate with vaccine-elicited CD8+ T-cell immunity (Figure S7). However, the eosinophil count correlated with the serum levels of vaccine-elicited anti-RBD IgG antibodies after the administration of the first dose and 6 months after the administration of the second dose of the vaccine (Figure S8A). After the administration of the first vaccine dose, the eosinophil count also correlated with vaccine-elicited TNFα- or IFNγ-producing CD4+ T cells (Figure S8B, top and middle panels).
We next analyzed whether patient age could be a clinical parameter impacting vaccine performance. Indeed, analyses revealed that patient age negatively affected the serum levels of vaccine-elicited anti-RBD IgG antibodies after the administration of the first dose and 6 months after the administration of the second dose of the vaccine (Figure 6A). In contrast, patient age did not negatively impact vaccine-elicited cellular immunity (Figure 6B and Figure S9). These data showed that vaccine-elicited humoral immunity was more sensitive to patient age than was cellular immunity.
Figure 6 The impact of patient age on the serum levels of anti-RBD antibodies and SARS-CoV-2 spike glycoprotein peptide-reactive CD4+ and CD8+ T cells during and after vaccination. (A) The correlations between patient age and the serum levels (U/ml) of anti-RBD IgA (top panels) and IgG (bottom panels) antibodies before (1st) and 4 weeks (2nd) and 6 months (6 mths) after the second vaccine dose was administered. (B) The correlations between patient age and the frequencies of peptide-reactive TNFα-producing CD4+ (top panels) and CD8+ (bottom panels) T cells before (1st) and 4 weeks (2nd) and 6 months (6 mths) after the second vaccine dose was administered. In (A, B), Spearman’s rank-order correlation coefficient (r) and the significance (p value; n = 34) are indicated.
This study showed that the COVID-19 vaccine used could elicit both humoral and cellular immunity against SARS-CoV-2 in patients with severe asthma on biological therapy. The type of biological therapy had no impact on either type of vaccine-elicited immunity. However, similar to the observed trend in healthy populations, vaccine-elicited immunity was significantly decreased 6 months after vaccination.
Biological therapy is increasingly used in clinical practice to treat severe asthma patients (43). Apart from treating the disease, it impacts the immune system. Patients with severe asthma are often treated with biologics, which are strong immunomodulators. Omalizumab binds to free IgE antibodies, which lowers free IgE levels and causes downregulation of FcϵRI receptors on basophils and mast cells (30). IgE antibodies are also important for cross-talk between innate and adaptive immunity and play roles in the susceptibility to respiratory infections (44) and in antiviral responses (45). As such, anti-IgE monoclonal antibodies were suggested as a potential treatment for COVID-19 that could enhance antiviral responses to the virus (46). Mepolizumab and reslizumab (anti-IL5 therapy) bind to IL-5 and thereby stop IL-5 from binding to its receptor on the surface of eosinophils. Inhibiting IL-5 binding to eosinophils reduces blood, tissue, and sputum eosinophil levels. Benralizumab specifically binds to IL-5Rα, thereby preventing the interaction between IL-5 and its receptor. Simultaneously, benralizumab, through its Fc region, binds to the receptor FcγIIIRa expressed by natural killer cells, thus inducing eosinophil apoptosis through the release of proapoptotic proteins such as granzymes and perforins (31). However, even though anti-IL5 therapy decreases the eosinophil count, this decrease does not prevent activation of any remaining eosinophils and subsequent humoral and cellular responses to viral challenge (47). Similar results were observed with anti-IL5R therapy, where the risk of respiratory infections due to a decreased eosinophil count was not largely increased (48, 49). Regardless of the different and potent mechanisms through which these biological drugs with an anti-type 2 inflammation effect modulate the immune system, these drugs were shown to not increase the risk of SARS-CoV-2 infection or worsen the clinical course and outcome of COVID-19 (50). Similarly, in our patients, these drugs did not prevent post-vaccination humoral and cellular immune responses. In addition, the duration of detectable immune responses was comparable to that in a healthy population (14–16). Therefore, the biological drugs used to treat the patients in this study are different from other immunomodulatory drugs, which have been shown to compromise the immunogenicity of the COVID-19 vaccine (51–53).
The COVID-19 vaccine has been shown to elicit SARS-CoV-2-specific immunity in multiple studies, and this capability relates to both humoral and cellular immunity (2–6). The dynamics of the humoral response and its durability in patients in our study were comparable to data obtained from healthy volunteers (54), indicating that the ongoing biological therapy used to treat the study patients had no apparent impact on the dynamics or durability of vaccine-elicited immunity. In agreement with other studies (54), our data also showed that the vaccine promoted stronger CD4+ T-cell SARS-CoV-2-specific immunity than CD8+ T-cell SARS-CoV-2-specific immunity (33, 55). Additionally, comparable to other reports, the extents of SARS-CoV-2-specific CD4+ and CD8+ T-cell immunity were correlated (7). In addition, the extent of humoral immunity in our study often correlated with T-cell immunity, namely, with CD8+ T-cell immunity. These data, therefore, show that the vaccine proportionally induces different arms of the immune system in severe asthma patients on biological therapy. However, whether this proportionality is skewed by the biological therapy needs to be further investigated.
The immunogenicity of mRNA vaccines decreases with the age of the vaccinated subjects (56, 57). This decrease can be observed in both vaccine-elicited humoral and cellular immunity (56). We also found a correlation between vaccine-elicited humoral immunity and the age of the vaccinated patients after the administration of the first dose of the vaccine and six months after the second dose was administered. These findings indicate that the age of severe asthma patients on biological therapy is a negative predictor of the onset and durability of vaccine-elicited humoral immunity. Surprisingly, patient age did not greatly affect SARS-CoV-2-specific cellular immunity. However, it should be noted that the study did not include patients over the age of 80 years, in whom this decline was found to be most pronounced (56). Regardless, the age of the severe asthma patients in this study played a significant role in the elicited humoral immunity, and similar to considerations for healthy populations, patient age needs to be considered as an indicator for a third booster vaccine dose, as the addition of such a dose was found to significantly restore sufficient SARS-CoV-2-specific immunity (58).
This study showed that patients with severe asthma on biological therapy could achieve activation of SARS-CoV-2-specific immune responses mediated by both arms of the adaptive immune system after a two-dose vaccination regimen with the BNT162b2 mRNA vaccine. However, comparable to those in healthy populations, these immune responses declined and presumably became not protective by 6 months after vaccination, and a booster dose should be considered.
The raw data supporting the conclusions of this article will be made available by the authors, without undue reservation.
The studies involving human participants were reviewed and approved by The Ethics Committee of the Motol University Hospital in Prague (protocol no. EK-346/21). The patients/participants provided their written informed consent to participate in this study.
MP, PT, DmS, and DaS designed the experiments. PT, DmS, MR, JL, and DaS conducted the experiments and/or analyzed the data. MP, AV, PP, and JB supervised the sample collection and clinical aspects of the study. MP and DaS wrote the manuscript. PT, DmS, MR, JL, AV, PP, and JB contributed to the writing of the manuscript. MP and DaS supervised the research. All authors contributed to the article and approved the submitted version.
Research in the authors’ laboratories was supported by the institutional IPE2 funding of Charles University, Second Faculty of Medicine in Prague and by funding from the Ministry of Health of the Czech Republic – project NU22-03-00300.
JB is a part-time employee and a minority shareholder of Sotio, a.s. PP is a member of the advisory board of GSK and received consultancy fees from AstraZeneca, Novartis, and Chiesi and speaker fees from GSK and Novartis.
The remaining authors declare that the research was conducted in the absence of any commercial or financial relationships that could be construed as a potential conflict of interest.
All claims expressed in this article are solely those of the authors and do not necessarily represent those of their affiliated organizations, or those of the publisher, the editors and the reviewers. Any product that may be evaluated in this article, or claim that may be made by its manufacturer, is not guaranteed or endorsed by the publisher.
We thank the clinical and laboratory research staff for their assistance, and John Wilson for his review of the manuscript.
The Supplementary Material for this article can be found online at: https://www.frontiersin.org/articles/10.3389/fimmu.2022.892277/full#supplementary-material
1. Khan WH, Hashmi Z, Goel A, Ahmad R, Gupta K, Khan N, et al. Covid-19 Pandemic and Vaccines Update on Challenges and Resolutions. Front Cell Infect Microbiol (2021) 11:690621. doi: 10.3389/fcimb.2021.690621
2. Polack FP, Thomas SJ, Kitchin N, Absalon J, Gurtman A, Lockhart S, et al. Safety and Efficacy of the BNT162b2 Mrna Covid-19 Vaccine. N Engl J Med (2020) 383(27):2603–15. doi: 10.1056/NEJMoa2034577
3. Baden LR, El Sahly HM, Essink B, Kotloff K, Frey S, Novak R, et al. Efficacy and Safety of the Mrna-1273 SARS-CoV-2 Vaccine. N Engl J Med (2021) 384(5):403–16. doi: 10.1056/NEJMoa2035389
4. Dunkle LM, Kotloff KL, Gay CL, Anez G, Adelglass JM, Barrat Hernandez AQ, et al. Efficacy and Safety of NVX-CoV2373 in Adults in the United States and Mexico. N Engl J Med (2021) 386:531–43. doi: 10.1056/NEJMoa2116185
5. Sadoff J, Gray G, Vandebosch A, Cardenas V, Shukarev G, Grinsztejn B, et al. Safety and Efficacy of Single-Dose Ad26.Cov2.s Vaccine Against Covid-19. N Engl J Med (2021) 384(23):2187–201. doi: 10.1056/NEJMoa2101544
6. Voysey M, Clemens SAC, Madhi SA, Weckx LY, Folegatti PM, Aley PK, et al. Safety and Efficacy of the ChAdOx1 nCoV-19 Vaccine (AZD1222) Against SARS-CoV-2: An Interim Analysis of Four Randomised Controlled Trials in Brazil, South Africa, and the UK. Lancet (2021) 397(10269):99–111. doi: 10.1016/S0140-6736(20)32661-1
7. Sahin U, Muik A, Derhovanessian E, Vogler I, Kranz LM, Vormehr M, et al. Covid-19 Vaccine BNT162b1 Elicits Human Antibody and TH1 T Cell Responses. Nature (2020) 586(7830):594–9. doi: 10.1038/s41586-020-2814-7
8. Kemp SA, Collier DA, Datir RP, Ferreira I, Gayed S, Jahun A, et al. Sars-CoV-2 Evolution During Treatment of Chronic Infection. Nature (2021) 592(7853):277–82. doi: 10.1038/s41586-021-03291-y
9. Khoury DS, Cromer D, Reynaldi A, Schlub TE, Wheatley AK, Juno JA, et al. Neutralizing Antibody Levels Are Highly Predictive of Immune Protection From Symptomatic SARS-CoV-2 Infection. Nat Med (2021) 27(7):1205–11. doi: 10.1038/s41591-021-01377-8
10. Deeks SG, Martin JN, Sinclair E, Harris J, Neilands TB, Maecker HT, et al. Strong Cell-Mediated Immune Responses Are Associated With the Maintenance of Low-Level Viremia in Antiretroviral-Treated Individuals With Drug-Resistant Human Immunodeficiency Virus Type 1. J Infect Dis (2004) 189(2):312–21. doi: 10.1086/380098
11. Mudd PA, Martins MA, Ericsen AJ, Tully DC, Power KA, Bean AT, et al. Vaccine-Induced CD8+ T Cells Control AIDS Virus Replication. Nature (2012) 491(7422):129–33. doi: 10.1038/nature11443
12. Israelow B, Mao T, Klein J, Song E, Menasche B, Omer SB, et al. Adaptive Immune Determinants of Viral Clearance and Protection in Mouse Models of SARS-Cov-2. Sci Immunol (2021) 6(64):eabl4509. doi: 10.1126/sciimmunol.abl4509
13. McMahan K, Yu J, Mercado NB, Loos C, Tostanoski LH, Chandrashekar A, et al. Correlates of Protection Against SARS-CoV-2 in Rhesus Macaques. Nature (2021) 590(7847):630–4. doi: 10.1038/s41586-020-03041-6
14. Dolgin E. COVID Vaccine Immunity is Waning - How Much Does That Matter? Nature (2021) 597(7878):606–7. doi: 10.1038/d41586-021-02532-4
15. Goldberg Y, Mandel M, Bar-On YM, Bodenheimer O, Freedman L, Haas EJ, et al. Waning Immunity After the BNT162b2 Vaccine in Israel. N Engl J Med (2021) 385(24):e85. doi: 10.1056/NEJMoa2114228
16. Levin EG, Lustig Y, Cohen C, Fluss R, Indenbaum V, Amit S, et al. Waning Immune Humoral Response to BNT162b2 Covid-19 Vaccine Over 6 Months. N Engl J Med (2021) 385:e84. doi: 10.1056/NEJMoa2114583
17. Munro APS, Janani L, Cornelius V, Aley PK, Babbage G, Baxter D, et al. Safety and Immunogenicity of Seven COVID-19 Vaccines as a Third Dose (Booster) Following Two Doses of ChAdOx1 nCov-19 or BNT162b2 in the UK (Cov-BOOST): A Blinded, Multicentre, Randomised, Controlled, Phase 2 Trial. Lancet (2021) 398(10318):2258–76. doi: 10.1016/S0140-6736(21)02717-3
18. Boyarsky BJ, Werbel WA, Avery RK, Tobian AAR, Massie AB, Segev DL, et al. Antibody Response to 2-Dose SARS-CoV-2 Mrna Vaccine Series in Solid Organ Transplant Recipients. JAMA (2021) 325(21):2204–6. doi: 10.1001/jama.2021.7489
19. Marion O, Del Bello A, Abravanel F, Couat C, Faguer S, Esposito L, et al. Safety and Immunogenicity of Anti-SARS-CoV-2 Messenger RNA Vaccines in Recipients of Solid Organ Transplants. Ann Intern Med (2021) 174(9):1336–8. doi: 10.7326/M21-1341
20. Becerril-Gaitan A, Vaca-Cartagena BF, Ferrigno AS, Mesa-Chavez F, Barrientos-Gutierrez T, Tagliamento M, et al. Immunogenicity and Risk of Severe Acute Respiratory Syndrome Coronavirus 2 (Sars-CoV-2) Infection After Coronavirus Disease 2019 (Covid-19) Vaccination in Patients With Cancer: A Systematic Review and Meta-Analysis. Eur J Cancer (2022) 160:243–60. doi: 10.1016/j.ejca.2021.10.014
21. Chen JJ, Lee TH, Tian YC, Lee CC, Fan PC, Chang CH. Immunogenicity Rates After SARS-CoV-2 Vaccination in People With End-stage Kidney Disease: A Systematic Review and Meta-Analysis. JAMA Netw Open (2021) 4(10):e2131749. doi: 10.1001/jamanetworkopen.2021.31749
22. Haberman RH, Herati R, Simon D, Samanovic M, Blank RB, Tuen M, et al. Methotrexate Hampers Immunogenicity to BNT162b2 Mrna COVID-19 Vaccine in Immune-Mediated Inflammatory Disease. Ann Rheum Dis (2021) 80(10):1339–44. doi: 10.1136/annrheumdis-2021-220597
23. Yang JM, Koh HY, Moon SY, Yoo IK, Ha EK, You S, et al. Allergic Disorders and Susceptibility to and Severity of COVID-19: A Nationwide Cohort Study. J Allergy Clin Immunol (2020) 146(4):790–8. doi: 10.1016/j.jaci.2020.08.008
24. Zhu Z, Hasegawa K, Ma B, Fujiogi M, Camargo CA Jr, Liang L. Association of Asthma and Its Genetic Predisposition With the Risk of Severe COVID-19. J Allergy Clin Immunol (2020) 146327-329(2):e324. doi: 10.1016/j.jaci.2020.06.001
25. Chhiba KD, Patel GB, Vu THT, Chen MM, Guo A, Kudlaty E, et al. Prevalence and Characterization of Asthma in Hospitalized and Nonhospitalized Patients With COVID-19. J Allergy Clin Immunol (2020) 146307-314(2):e304. doi: 10.1016/j.jaci.2020.06.010
26. Lovinsky-Desir S, Deshpande DR, De A, Murray L, Stingone JA, Chan A, et al. Asthma Among Hospitalized Patients With COVID-19 and Related Outcomes. J Allergy Clin Immunol (2020) 1461027-1034(5):e1024. doi: 10.1016/j.jaci.2020.07.026
27. Robinson LB, Fu X, Bassett IV, Triant VA, Foulkes AS, Zhang Y, et al. Covid-19 Severity in Hospitalized Patients With Asthma: A Matched Cohort Study. J Allergy Clin Immunol Pract (2021) 9(1):497–500. doi: 10.1016/j.jaip.2020.10.021
28. Lombardi C, Gani F, Berti A, Comberiati P, Peroni D, Cottini M. Asthma and COVID-19: A Dangerous Liaison? Asthma Res Pract (2021) 7(1):9. doi: 10.1186/s40733-021-00075-z
29. Caminati M, Guarnieri G, Batani V, Scarpieri E, Finocchiaro A, Chieco-Bianchi F, et al. Covid-19 Vaccination in Patients With Severe Asthma on Biologic Treatment: Safety, Tolerability, and Impact on Disease Control. Vaccines (Basel) (2021) 9(8):1–8. doi: 10.3390/vaccines9080853
30. Rodrigo GJ, Neffen H, Castro-Rodriguez JA. Efficacy and Safety of Subcutaneous Omalizumab vs Placebo as Add-on Therapy to Corticosteroids for Children and Adults With Asthma: A Systematic Review. Chest (2011) 139(1):28–35. doi: 10.1378/chest.10-1194
31. Edris A, De Feyter S, Maes T, Joos G, Lahousse L. Monoclonal Antibodies in Type 2 Asthma: A Systematic Review and Network Meta-Analysis. Respir Res (2019) 20(1):179. doi: 10.1186/s12931-019-1138-3
32. Taborska P, Bartunkova J, Smrz D. Simultaneous In Vitro Generation of Human CD34(+)-derived Dendritic Cells and Mast Cells From Non-Mobilized Peripheral Blood Mononuclear Cells. J Immunol Methods (2018) 458:63–73. doi: 10.1016/j.jim.2018.04.005
33. Taborska P, Lastovicka J, Stakheev D, Strizova Z, Bartunkova J, Smrz D. Sars-CoV-2 Spike Glycoprotein-Reactive T Cells can be Readily Expanded From COVID-19 Vaccinated Donors. Immun Inflammation Dis (2021) 9(4):1452–67. doi: 10.1002/iid3.496
34. Reddel HK, FitzGerald JM, Bateman ED, Bacharier LB, Becker A, Brusselle G, et al. GINA 2019: A Fundamental Change in Asthma Management: Treatment of Asthma With Short-Acting Bronchodilators Alone is No Longer Recommended for Adults and Adolescents. Eur Respir J (2019) 53(6):1–7. doi: 10.1183/13993003.01046-2019
35. Reddel HK, Bacharier LB, Bateman ED, Brightling CE, Brusselle GG, Buhl R, et al. Global Initiative for Asthma (Gina) Strategy 2021 - Executive Summary and Rationale for Key Changes. J Allergy Clin Immunol Pract (2021) 10(1):S1–S18. doi: 10.1016/j.jaip.2021.10.001
36. Smits VAJ, Hernandez-Carralero E, Paz-Cabrera MC, Cabrera E, Hernandez-Reyes Y, Hernandez-Fernaud JR, et al. The Nucleocapsid Protein Triggers the Main Humoral Immune Response in COVID-19 Patients. Biochem Biophys Res Commun (2021) 543:45–9. doi: 10.1016/j.bbrc.2021.01.073
37. Galipeau Y, Siragam V, Laroche G, Marion E, Greig M, McGuinty M, et al. Relative Ratios of Human Seasonal Coronavirus Antibodies Predict the Efficiency of Cross-Neutralization of SARS-CoV-2 Spike Binding to ACE2. EBioMedicine (2021) 74:103700. doi: 10.1016/j.ebiom.2021.103700
38. Altmann DM, Boyton RJ. SARS-Cov-2 T Cell Immunity: Specificity, Function, Durability, and Role in Protection. Sci Immunol (2020) 5(49), 1–5. doi: 10.1126/sciimmunol.abd6160
39. Rosenberg ES, Billingsley JM, Caliendo AM, Boswell SL, Sax PE, Kalams SA, et al. Vigorous HIV-1-specific Cd4+ T Cell Responses Associated With Control of Viremia. Science (1997) 278(5342):1447–50. doi: 10.1126/science.278.5342.1447
40. Combadiere B, Boissonnas A, Carcelain G, Lefranc E, Samri A, Bricaire F, et al. Distinct Time Effects of Vaccination on Long-Term Proliferative and IFN-Gamma-Producing T Cell Memory to Smallpox in Humans. J Exp Med (2004) 199(11):1585–93. doi: 10.1084/jem.20032083
41. Oberhardt V, Luxenburger H, Kemming J, Schulien I, Ciminski K, Giese S, et al. Rapid and Stable Mobilization of CD8(+) T Cells by SARS-CoV-2 mRNA Vaccine. Nature (2021) 597(7875):268–73. doi: 10.1038/s41586-021-03841-4
42. Taborska P, Strizova Z, Stakheev D, Sojka L, Bartunkova J, Smrz D. Cd4(+) T Cells of Prostate Cancer Patients Have Decreased Immune Responses to Antigens Derived From SARS-Cov-2 Spike Glycoprotein. Front Immunol (2021) 12:629102. doi: 10.3389/fimmu.2021.629102
43. Brusselle GG, Koppelman GH. Biologic Therapies for Severe Asthma. N Engl J Med (2022) 386(2):157–71. doi: 10.1056/NEJMra2032506
44. Esquivel A, Busse WW, Calatroni A, Togias AG, Grindle KG, Bochkov YA, et al. Effects of Omalizumab on Rhinovirus Infections, Illnesses, and Exacerbations of Asthma. Am J Respir Crit Care Med (2017) 196(8):985–92. doi: 10.1164/rccm.201701-0120OC
45. Rowe RK, Pyle DM, Tomlinson AR, Lv T, Hu Z, Gill MA. Ige Cross-Linking Impairs Monocyte Antiviral Responses and Inhibits Influenza-Driven TH1 Differentiation. J Allergy Clin Immunol (2017) 140(1):294–298 e298. doi: 10.1016/j.jaci.2016.11.035
46. Farmani AR, Mahdavinezhad F, Moslemi R, Mehrabi Z, Noori A, Kouhestani M, et al. Anti-IgE Monoclonal Antibodies as Potential Treatment in COVID-19. Immunopharmacol Immunotoxicol (2021) 43(3):259–64. doi: 10.1080/08923973.2021.1925906
47. Sabogal Pineros YS, Bal SM, van de Pol MA, Dierdorp BS, Dekker T, Dijkhuis A, et al. Anti-IL-5 in Mild Asthma Alters Rhinovirus-Induced Macrophage, B-Cell, and Neutrophil Responses (Material). A Placebo-Controlled, Double-Blind Study. Am J Respir Crit Care Med (2019) 199(4):508–17. doi: 10.1164/rccm.201803-0461OC
48. Castro M, Zangrilli J, Wechsler ME, Bateman ED, Brusselle GG, Bardin P, et al. Reslizumab for Inadequately Controlled Asthma With Elevated Blood Eosinophil Counts: Results From Two Multicentre, Parallel, Double-Blind, Randomised, Placebo-Controlled, Phase 3 Trials. Lancet Respir Med (2015) 3(5):355–66. doi: 10.1016/S2213-2600(15)00042-9
49. Jackson DJ, Korn S, Mathur SK, Barker P, Meka VG, Martin UJ, et al. Safety of Eosinophil-Depleting Therapy for Severe, Eosinophilic Asthma: Focus on Benralizumab. Drug Saf (2020) 43(5):409–25. doi: 10.1007/s40264-020-00926-3
50. Poddighe D, Kovzel E. Impact of Anti-Type 2 Inflammation Biologic Therapy on COVID-19 Clinical Course and Outcome. J Inflamm Res (2021) 14:6845–53. doi: 10.2147/JIR.S345665
51. Havlin J, Skotnicova A, Dvorackova E, Hubacek P, Svorcova M, Lastovicka J, et al. Impaired Humoral Response to Third Dose of BNT162b2 Mrna COVID-19 Vaccine Despite Detectable Spike Protein-Specific T Cells in Lung Transplant Recipients. Transplantation (2021) 106(3):e183–4. doi: 10.1097/TP.0000000000004021
52. Havlin J, Svorcova M, Dvorackova E, Lastovicka J, Lischke R, Kalina T, et al. Immunogenicity of BNT162b2 Mrna COVID-19 Vaccine and SARS-CoV-2 Infection in Lung Transplant Recipients. J Heart Lung Transplant (2021) 40(8):754–8. doi: 10.1016/j.healun.2021.05.004
53. Lee A, Wong SY, Chai LYA, Lee SC, Lee MX, Muthiah MD, et al. Efficacy of covid-19 Vaccines in Immunocompromised Patients: Systematic Review and Meta-Analysis. BMJ (2022) 376:e068632. doi: 10.1136/bmj-2021-068632
54. Naaber P, Tserel L, Kangro K, Sepp E, Jurjenson V, Adamson A, et al. Dynamics of Antibody Response to BNT162b2 Vaccine After Six Months: A Longitudinal Prospective Study. Lancet Reg Health Eur (2021) 10:100208. doi: 10.1016/j.lanepe.2021.100208
55. Guerrera G, Picozza M, D’Orso S, Placido R, Pirronello M, Verdiani A, et al. BNT162b2 Vaccination Induces Durable SARS-Cov-2-Specific T Cells With a Stem Cell Memory Phenotype. Sci Immunol (2021) 6(66):eabl5344. doi: 10.1126/sciimmunol.abl5344
56. Collier DA, Ferreira I, Kotagiri P, Datir RP, Lim EY, Touizer E, et al. Age-Related Immune Response Heterogeneity to SARS-CoV-2 Vaccine BNT162b2. Nature (2021) 596(7872):417–22. doi: 10.1038/s41586-021-03739-1
57. Muller L, Andree M, Moskorz W, Drexler I, Walotka L, Grothmann R, et al. Age-Dependent Immune Response to the Biontech/Pfizer Bnt162b2 Coronavirus Disease 2019 Vaccination. Clin Infect Dis (2021) 73(11):2065–72. doi: 10.1093/cid/ciab381
Keywords: severe asthma patients on biological therapy, SARS-CoV-2, COVID-19 vaccination, cellular immunity, humoral immunity
Citation: Podrazil M, Taborska P, Stakheev D, Rataj M, Lastovicka J, Vlachova A, Pohunek P, Bartunkova J and Smrz D (2022) Effectiveness and Durability of mRNA Vaccine-Induced SARS-CoV-2-Specific Humoral and Cellular Immunity in Severe Asthma Patients on Biological Therapy. Front. Immunol. 13:892277. doi: 10.3389/fimmu.2022.892277
Received: 08 March 2022; Accepted: 22 April 2022;
Published: 20 May 2022.
Edited by:
Matthew S Buckland, Great Ormond Street Hospital for Children NHS Foundation Trust, United KingdomReviewed by:
Teresa Cutino-Moguel, Queen Mary University of London, United KingdomCopyright © 2022 Podrazil, Taborska, Stakheev, Rataj, Lastovicka, Vlachova, Pohunek, Bartunkova and Smrz. This is an open-access article distributed under the terms of the Creative Commons Attribution License (CC BY). The use, distribution or reproduction in other forums is permitted, provided the original author(s) and the copyright owner(s) are credited and that the original publication in this journal is cited, in accordance with accepted academic practice. No use, distribution or reproduction is permitted which does not comply with these terms.
*Correspondence: Daniel Smrz, ZGFuaWVsLnNtcnpAbGZtb3RvbC5jdW5pLmN6
†These authors share first authorship
Disclaimer: All claims expressed in this article are solely those of the authors and do not necessarily represent those of their affiliated organizations, or those of the publisher, the editors and the reviewers. Any product that may be evaluated in this article or claim that may be made by its manufacturer is not guaranteed or endorsed by the publisher.
Research integrity at Frontiers
Learn more about the work of our research integrity team to safeguard the quality of each article we publish.