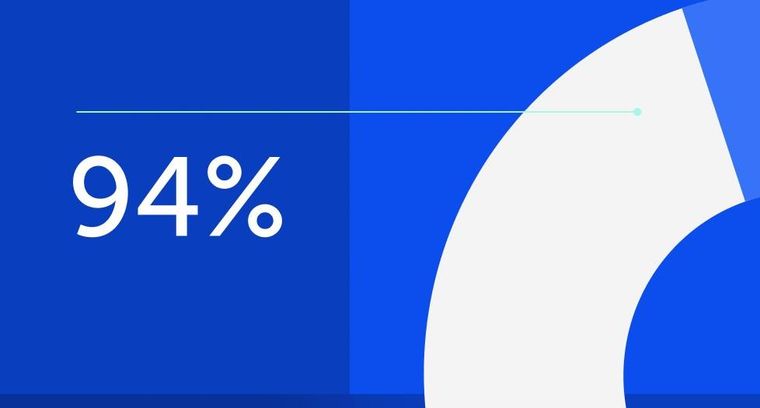
94% of researchers rate our articles as excellent or good
Learn more about the work of our research integrity team to safeguard the quality of each article we publish.
Find out more
ORIGINAL RESEARCH article
Front. Immunol., 07 July 2022
Sec. Parasite Immunology
Volume 13 - 2022 | https://doi.org/10.3389/fimmu.2022.891643
Vaccination is an effective method to prevent Cryptocaryon irritans infection. Although some vaccines have been developed, large-scale production of these vaccines is costly. Development of a heterogenous vaccine generated by low-cost antigens is an alternative method. In the present study, grouper immunized with Tetrahymena thermophila, a free-living ciliate that easily grows in inexpensive culture media at high density, showed protective immunity against C. irritans infection. Higher immobilization against C. irritans theronts was detected in T. thermophila–immunized grouper serum, which suggested the existence of a cross-reactive antibody in the serum. By immunoprecipitation and mass spectrometry analyses, tubulin was identified as a potential cross-reactive antigen between C. irritans and T. thermophila. Recombinant T. thermophila tubulin protein (rTt-tubulin) and its antibody were prepared, and immunofluorescence showed that both C. irritans and T. thermophila cilia were stained by the anti–rTt-tubulin antibody. Grouper immunized with rTt-tubulin showed a reduced infective rate after the C. irritans challenge. An enhanced level of C. irritans–binding immunoglobulin M (IgM) antibody was detected in serum from rTt-tubulin–immunized grouper. Moreover, specific antibodies were also found in the mucus and tissue culture medium from rTt-tubulin–immunized grouper. Overall, these findings suggested that vaccination with T. thermophila elicits cross-reactive protective immunity in grouper against C. irritans, and T. thermophila may be a potential heterologous antigen for vaccine development.
Cryptocaryon irritans (Brown, 1951), an obligate parasitic ciliate, can infect numerous species of marine fish, indicating a potential threat to marine aquaculture (1). Previous studies have found that sublethal exposure to C. irritans elicits immune protection (2, 3). Moreover, injection of inactivated C. irritans also provides protective resistance (4, 5). In addition, DNA and recombinant protein vaccines encoded by immobilization antigen (I-antigen) genes have also been developed to control this pathogen (6–8). These findings suggest that vaccination may be an effective method to prevent C. irritans infection. Although it is possible to grow C. irritans outside the host, the yields are limited (9, 10), and large-scale production of C. irritans is difficult (8). In addition, the generation of DNA and recombinant protein–based vaccines is often costly.
Previous research has shown that channel catfish (Ictalurus punctatus) immunized with different isolates of Tetrahymena pyriformis (Lwoff) cilia provide immune protection against an Ichthyophthirius multifiliis challenge, which is a freshwater counterpart of C. irritans (11, 12). In addition, Goldfish (Carassius auratus) using I. multifiliis or T. pyriformis for vaccination develop protective immunity against I. multifiliis and other parasitic ciliates (13). These findings suggest that cross-reactive protection against parasitic ciliates is induced by these free-living ciliates. Thus, the development of a heterogenous vaccine may be a promising method for controlling diseases caused by these parasitic ciliates.
Tetrahymena thermophila is a freshwater free-living ciliate, indicating that the risk of pathogenic contamination can be ruled out. More importantly, T. thermophila easily grows in inexpensive culture media at a high density, which is suitable for the large-scale production of vaccines. Our previous study found that a large number of homologous genes (~76%) are shared by T. thermophila, I. multifiliis, and C. irritans (14). Thus, we hypothesized that immunization with T. thermophila may provide immune protection for fish against C. irritans infection.
In the present study, orange-spotted grouper (Epinephelus coioides) immunized with T. thermophila showed a lower infective rate when challenged with C. irritans, and a higher immobilization was found in immunized fish. Moreover, tubulin was identified as one of the potential cross-reactive antigens between T. thermophila and C. irritans by immunoprecipitation and mass spectrometry analyses. Immune protection occurred, and a specific antibody against C. irritans was present in grouper vaccinated with the recombinant T. thermophila tubulin protein. These findings suggested that T. thermophila may be an alternative candidate for the development of a heterogenous vaccine against C. irritans infection.
Healthy orange-spotted groupers (28 ± 3.1 g) and golden pompanos (Trachinotus ovatus, 235 ± 13.5 g) were obtained from a local farm in Guangdong, China, and they were maintained at 28°C in a flow-through water system (300 L) as previously described (15). Both fish were acclimated for at least 2 weeks and fed with commercial feed twice a day.
C. irritans used in this study was originally isolated from an infected golden pompano in a local farm of Daya Bay, Guangdong Province, China. The propagation of C. irritans was performed as previously described by using golden pompano as the host (16). Briefly, tomonts were collected from the bottom of a harvest unit and incubated in flow-through seawater. Three days after incubation, post-excystment theronts were collected and used to reinfect golden pompanos (10,000 theronts per fish) for 2 h. The golden pompanos were then transferred into the harvest unit for the next round of propagation.
T. thermophila strain SCAU07 used in this study was generated in our previous study (8). Briefly, T. thermophila strains CU427 (mating type 6) and CU428 (mating type 7) (a gift from Theodore G. Clark, Cornell University) were mated and transformed with pD5H8-GDCI3, which contains a paromomycin-resistant gene. The positive transformed cells were selected by paromomycin. The SCAU07 strain was cultured in NEFF medium (0.25% protease peptone, 0.25% yeast extract, 0.55% dextrose, and 33 µM FeCl3) at 30°C for 24 h with shaking (80 rpm) (8).
Both C. irritans theronts and T. thermophila were collected by centrifugation and diluted to 200,000 ciliates/ml with phosphate buffered saline (PBS). Both ciliates were individually emulsified with Freund’s Complete Adjuvant (FCA, for primary vaccination) or Freund’s Incomplete Adjuvant (FIA, for boost vaccination) at a final concentration of 100,000 ciliates/ml. Because C. irritans is an ectoparasite and its infection can induce mucosal immune response, herein for vaccination, groupers were subcutaneously injected (IC) with FCA-emulsified C. irritans theronts or T. thermophila at a dose of 10,000 ciliates per fish. Two weeks after the primary inoculation, groupers were boosted via IC injection of FIA-emulsified C. irritans theronts or T. thermophila at a dose of 10,000 ciliates per fish. Moreover, the control groupers were injected with FCA- or FIA-emulsified PBS.
For the C. irritans challenge, 4 weeks after the boost vaccination, groupers were randomly selected from each group and placed into a separate tank with 100 L of seawater. Post-excystment theronts collected within 2 h were added into each tank at a dose of 4,000 theronts per fish. Two hours after the infection, each grouper was transferred into a 15-L clean tank. The numbers of tomonts were recorded from each tank, and the received tomonts relative to the infective dose (4,000 theronts) were considered as the infective rate at day 5 after challenge. Serum samples for the following tests were collected at day 3 after challenge.
The immobilization assay was perfromed as presiously described (8). Briefly, 50 μl of grouper serum was serially diluted (two-fold dilutions) with seawater and placed into a 96-well plate. For C. irritans immobilization, 50 μl of seawater (containing 500 theronts) was added into each well and incubated for 30 min at room temperature. The last well, in which 50% of theronts was immobilized, was considered as the endpoint titer.
Protein samples were isolated from C. irritans theronts or T. thermophila using RIPA lysis buffer, and the cross-reactive antigens were detected by Western blot analysis. C. irritans theront protein samples (2 mg/ml) were isolated using RIPA lysis buffer and then incubated with diluted serum (1:5) collected from T. thermophila–injected groupers at 4°C for 12 h. The antigen-antibody complex was immunoprecipitated by a mouse anti-grouper IgM monoclonal antibody (mAb) affinity column (17) and washed with PBS. The complex was analyzed using liquid chromatography–tandem mass spectrometry and mapped to the ciliate proteome database (see schematic diagram in Figure 1).
Figure 1 Schematic diagram of heterogenous vaccine development in this study. C. irritans and T. thermophila were cultured and collected for antigen preparation. After emulsifying with adjuvants, vaccines were individually SQ injected into groupers. Four weeks after the boost vaccination, groupers were challenged with C. irritans theronts to measure the infective rate. Serum samples were collected from groupers before the challenge and used for identification of potential cross-reactive antigens by immunoprecipitation and mass spectrometry analyses. The recombinant potential cross-reactive antigen was prepared by prokaryotic expression and injected into grouper for infective rate detection. The schematic was created using BioRender.
The amino acid identity of T. thermophila tubulin (Tt-tubulin) with ciliate or grouper tubulin was analyzed by the BLAST program. The amino acid sequence of Tt-tubulin was aligned with other animals using the CLUSTALW server (http://www.ebi.ac.uk/clustalw). The three-dimensional (3D) model of T. thermophila and C. irritans tubulin was built using the SWISS-MODEL server (https://swissmodel.expasy.org/interactive). Phylogenic analysis between ciliates and teleosts was performed using the MEGA 5.04 program. All accession numbers of the sequences used in this part are listed in Supplementary Table 1.
A codon optimized Tt-tubulin sequence (XP_001023006) for Escherichia coli expression was synthesized by Generay Biotech Co., Ltd. (Shanghai) and then cloned into the pET32a-ΔTRX expression vector (prepared by this lab) to generate pET32a-ΔTRX-Tt-tubulin. The pET32a-ΔTRX-Tt-tubulin plasmid was isolated and transformed into E. coli BL21(DE3) cells. The positive bacterial cells were induced with 1 mM isopropyl b-d-1-thiogalactopyranoside (IPTG). Recombinant Tt-tubulin protein (rTt-tubulin) was purified using a nickel nitrilotriacetic acid column (Ni-NTA; Qiagen, Germany).
The purified rTt-tubulin was emulsified with FCA, and 1 mg of protein was injected into New Zealand white rabbits (weighing approximately 1.3 kg). The animals were then boosted with 0.5 mg of rTt-tubulin in FIA on two separate occasions. Serum was then prepared, and the polyclonal antibody (pAb) titers were determined by enzyme-linked immunosorbent assays (ELISAs) using rTt-tubulin as the antigen. Rabbit immunoglobulin G (IgG) was purified from rabbit antiserum using protein A agarose (Beyotime, Haimen, Jiangsu, China) according to the manufacturer’s instructions.
To study the immune response of rTt-tubulin vaccination, all samples for the subsequent analysis were collected at day 3 after challenge. For serum collection, blood was obtained using a 1-ml sterilized syringe via the caudal vein from MS-222–anesthetized groupers, and the blood was stored at room temperature for 2 h. Sera were collected by centrifugation at 800 × g for 10 min at 4°C. For skin mucus collection, skin mucus was gently scraped from anesthetized groupers using a clean slide in a 1.5-ml Eppendorf tube. After vigorously vortexing, the supernatant (skin mucus) was harvested by centrifugation at 10,000 × g for 10 min at 4°C and stored at −80°C. Grouper tissue explant culture was performed as previously described (5) with some modifications. Groupers were sacrificed by an overdose of MS-222, and skin, gill, spleen, and head kidney tissues were collected and washed with cold PBS to exclude the blood content. Each tissue was cut into approximately 30-mg portions, placed in a 24-well plate with AIM-V serum-free medium (supplemented with penicillin of 100 U/ml and streptomycin of 100 mg/ml) and then cultured at 28°C for 8 h. The culture medium was collected and centrifuged at 10,000 × g for 10 min at 4°C. A final dose of 1 mM phenylmethylsulfonyl fluoride was added into all collected samples, including serum, mucus, and cultured medium, and the samples were then stored at −80°C.
The purified rTt-tubulin was diluted with coating buffer (pH 9.6) at a dose of 1 μg/ml and then used to coat the wells in a 96-well plate followed by incubation at 37°C for 4 h. Each well was washed with PBST (PBS containing 0.1% Tween) and incubated with blocking buffer (PBST containing 3% BSA) at 4°C for 12 h. After washing with PBST, serum or purified IgG was diluted with dilution buffer (PBST containing 1% BSA) and added into each well at 37°C for 1 h. For the rabbit IgG antibody titer analysis, the wells were detected with horseradish peroxidase (HRP)-conjugated anti-rabbit IgG [1 μg/ml, Cell Signaling Technology (CST)]. For the grouper IgM antibody titer analysis, samples of diluted serum (doubling dilution), mucus (1:5), and tissue culture medium (1:1) were added into each well and incubated at 28°C for 1 h. The wells were then detected with anti-IgM mAb (1 μg/ml) followed by incubation with a HRP-conjugated anti-mouse antibody (1 μg/ml, CST). All wells were incubated with substrate solution, and the optical density at 450 nm (OD450) value was measured using a microplate reader. The last well, in which the value was greater than twice the blank value, was considered as the endpoint titer.
The parasite-binding antibody was measured as previously described (8). Briefly, theronts were collected and divided into 10,000 theronts per sample. Theronts were then blocked with incubation buffer (PBS containing 1% BSA) at 28°C for 1 h followed by incubation with serum (1:100 dilution) at 4°C for 12 h with agitation. Theronts were then washed with PBS, lysed with RIPA buffer, and boiled in SDS sample buffer for the subsequent Western blot detection with anti-IgM mAb.
Sodium Dodecyl Sulfate PolyAcrylamide Gel Electrophoresis (SDS-PAGE) and Western blot analysis were conducted as previously described (18). Briefly, equal amounts of the protein samples were electrophoresed using a 10% SDS-PAGE gel and transferred to a polyvinylidene fluoride (PVDF) membrane. The PVDF membranes were blocked with 5% skim milk for 1 h at 37°C. For parasite-binding antibody detection, the membranes were incubated with anti-grouper IgM mAb (1 μg/ml) followed by detection with HRP-conjugated anti-mouse IgG (1 μg/ml, CST). For detection of cross-reactive antigens, the membranes were firstly incubated with grouper anti-serum (1:100 dilution) before incubation with anti-grouper IgM mAb. For tubulin detection, the membranes were incubated with anti–rTt-tubulin pAb (1 μg/ml) followed by detection with HRP-conjugated anti-rabbit IgG (1 μg/ml, CST). The resulting bands were visualized using the SuperSignal West Pico Chemiluminescent Substrate.
C. irritans theronts or T. thermophila were added dropwise to a slide and fixed with 4% paraformaldehyde (PFA) at 28°C for 15 min. The slides were washed and blocked with 10% goat serum at 37°C for 1 h. For detection of cross-reactive antigens, the slides were firstly incubated with grouper anti-serum (1:100 dilution) followed by incubation with anti-grouper IgM mAb and Alexa 488–conjugated anti-mouse IgG (1 μg/ml, CST). For tubulin detection, the slides were incubated with anti–rTt-tubulin pAb (1 μg/ml) followed by detection with Alexa 488–conjugated anti-rabbit IgG (1 μg/ml, CST). All slides were stained with 1 μg/ml 4′,6-diamidino-2-phenylindole (DAPI, Invitrogen) and mounted in anti-fluorescence quenching agent (Beyotime). All slides were photographed using a NIH-Elements System (Nikon).
C. irritans theronts were collected and fixed with 4% PFA at 28°C for 30 min. Ciliates cells were blocked with 5% goat serum at 28°C for 30 min and stained with anti–Tt-tubulin pAb (rabbit, 1 μg/ml) or normal rabbit IgG (as control) at 28°C for 1 h. After washing with PBS, the Alexa 488–conjugated anti-rabbit IgG secondary antibody (1 μg/ml, CST) was added followed by incubation at 4°C for 1 h. The stained cells were counted by flow cytometry using a CytoFLEX (Beckman) and analyzed by FlowJo software (Tree Star).
The differences between groups were analyzed by an unpaired Student’s t-test, and multiple comparisons were analyzed by the Dunn’s test (Prism version 8.0; GraphPad). p < 0.05 was considered statistically significant.
Previous research has shown that grouper injected with C. irritans elicit protective immunity against this parasite’s infection (5). In the present study, we aimed to study whether another ciliate, T. thermophila, serves as a heterogenous vaccine and provides immune protection for grouper. After primary and secondary inoculations with C. irritans theronts or T. thermophila cells, the infection rate was significantly reduced in groupers injected with C. irritans theronts (CI group, 5.5%) or T. thermophila (TT group, 8.9%) compared to the control group (15.3%) after the challenge (Figure 2A), which confirmed that vaccination with T. thermophila elicited immune protection against the C. irritans challenge. Furthermore, an in vitro immobilization assay showed that higher titers were detected in the CI and TT group sera, which indicated that both the CI and TT groups generated a specific antibody in their sera (Figure 2B).
Figure 2 Grouper immunized with C. irritans or T. thermophila are resistant to C. irritans infection. (A) C. irritans infection rate of C. irritans– or T. thermophila–immunized groupers (n = 7). Significant differences are represented as *** (p < 0.001). (B) Increased immobilization titers were found in serum from C. irritans– or T. thermophila–immunized groupers (n = 5). Significant differences are represented as *** (p < 0.001).
To confirm whether any potential cross-reactive antigens exist between C. irritans and T. thermophila, immunofluorescence staining and Western blot analysis using sera from the CI and TT groups as the primary antibodies were performed. Immunofluorescence staining showed that C. irritans was stained with CI or TT group serum but not the control serum (Figure 3A). Western blot analysis using C. irritans theronts as antigens showed that various bands were detected using the CI or TT group serum, and no signal was detected with the control serum (Figure 3B). Further, to identify the cross-reactive antigens, total protein of C. irritans theronts was isolated, incubated with TT group serum, and then immunoprecipitated by an anti-IgM affinity column. After mass spectrometry analysis, some potential cross-reactive antigens were identified (Supplementary Table 1). The mapping profiles of the top three identified sequences of potential cross-reactive antigens are listed in Supplementary Figure 1. Of note, the tubulin molecule had the top score among these potential cross-reactive antigens, which agreed with a previous immune proteomic study (19). In addition, given that tubulin protein is a crucial composition for cilia assembly (20), it may be an effective cross-reactive antigen in ciliates. Thus, the tubulin protein was selected for additional study.
Figure 3 Identification of cross-reactive antigens between C. irritans and T. thermophila. (A) Immunofluorescence staining of C. irritans using serum from C. irritans– or T. thermophila–immunized grouper. (B) Western blot analysis of total protein from C. irritans and T. thermophila using serum from immunized grouper.
The predicted 3D model showed that C. irritans and T. thermophila tubulin share similar structure (Figure 4A). Homology and alignment analyses showed that T. thermophila tubulin shares higher amino acid identity with parasitic ciliates (97%–99%) than grouper (90.7%) (Figures 4B, C). Furthermore, the phylogenetic tree indicated the formation of two major clades, representing tubulin of ciliates and teleosts (Figure 4D). Taken together, we hypothesized that ciliate tubulin may serve as a heterologous antigen in fish against parasitic ciliates and that it is less likely to induce an autoimmune response in fish.
Figure 4 Characterization of ciliate tubulin protein. (A) The 3D homology model of C. irritans tubulin was performed using the T. thermophila tubulin model as a template. (B) Heatmap of the amino acid identity between grouper and ciliates. (C) Sequence alignment of tubulin between grouper and ciliates. (D) Phylogenetic tree between fish and ciliate tubulin. A phylogenetic tree illustrating the relationship between fish and ciliate tubulin using the neighbor-joining method within the MEGA program. Ec, E. coioides; Im, I multifiliis; Tt, T. thermophila; Ci, C. irritans.
As ciliates have a codon usage bias, a codon optimized sequence of T. thermophila tubulin adapted to E. coil expression was synthesized. After induction with IPTG, an additional band was found after E. coil lysis (Figure 5A). The protein was subsequently purified by a Ni affinity column, and an evident band migrating at approximately 55 kDa was detected by SDS-PAGE followed by Coomassie blue staining (Figure 5A). High-titer antiserum was prepared by injecting a rabbit with the purified rTt-tubulin. Anti–rTt-tubulin IgG was purified by Protein G affinity column. Flow cytometry analysis showed that more than 96.4% of C. irritans theronts were stained with the anti–rTt-tubulin antibody (Figure 5B). The specificity of the resulting antibody was confirmed by Western blot analysis with two bands of approximately 55 and 35 kDa when the total protein from C. irritans theronts or T. thermophila cells was screened (Figure 5C). Immunofluorescence staining of ciliate cells showed that tubulin was diffusely localized in cilia from both C. irritans and T. thermophila cells (Figure 5D).
Figure 5 Expression and antibody preparation of T. thermophila tubulin. (A) SDS-PAGE analysis of purified recombinant T. thermophila tubulin in E. coli. M, protein marker; Lane 1, non-induced BL21 cells transformed with pET32a-ΔTRX-Tt-tubulin; Lane 2, induced BL21 cells transformed with pET32a-ΔTRX-Tt-tubulin for 4 h; and Lane 3, purified recombinant T. thermophila tubulin (approximately 55 kDa) with Ni-NTA. (B) Flow cytometry analysis of C. irritans using the anti–Tt-tubulin antibody. (C) Western blot analysis of total protein from C. irritans and T. thermophila using the anti–Tt-tubulin antibody. (D) Immunofluorescence staining of C. irritans and T. thermophila using the anti–Tt-tubulin antibody. Scale bar = 10 μm.
To verify whether T. thermophila tubulin contributes to the immune protection against C. irritans infection, orange-spotted groupers were injected with rTt-tubulin and challenged with C. irritans for 4 weeks after the secondary immunization. The infection rate was significantly reduced in groupers injected with rTt-tubulin (11.4%) compared to the control group (14.8%) after the challenge (Figure 6A). To study parasite-specific IgM antibody responses in groupers, serum, mucus, and tissue culture medium samples were collected from the control groupers and rTt-tubulin–vaccinated groupers at day 3 after challenge. The parasite-binding antibody was pulled down by theronts, and a specific IgM antibody was detected by Western blot analysis. As expected, an increment of parasite-specific antibodies was detected in rTt-tubulin–vaccinated grouper serum compared to the control grouper serum (Figures 6B, C). To further analyze the generation of a specific antibody in vaccinated grouper, ELISAs using rTt-tubulin as the antigen were conducted. Similarly, a higher antibody titer was detected in rTt-tubulin–vaccinated grouper serum compared to control grouper serum (Figure 6D). As the level of antibody is relatively low in mucus and tissue culture medium, the specific antibody level in these samples is presented as an OD value. As expected, higher anti–rTt-tubulin specific antibody levels were found in the mucus and culture medium samples from the skin, gill, spleen, and head kidney from rTt-tubulin–vaccinated groupers compared to the control groupers (Figures 6E–I).
Figure 6 Tt-tubulin provides immune protection for grouper against C. irritans infection. (A) C. irritans infection rate of rTt-tubulin–immunized groupers (n = 10). (B) Western blot analysis and (C) densitometric analysis of parasite-binding IgM in sera from control and rTt-tubulin–immunized groupers at 72 h after C. irritans infection (n = 3). (D–I) ELISA detection of the anti–rTt-tubulin IgM antibody in serum (D), mucus (E), skin culture medium (F), gill culture medium (G), spleen culture medium (H), and head kidney culture medium (I) (n = 5). Significant differences are represented as * (p < 0.05) and *** (p < 0.001).
Many studies have suggested that vaccination may be an effective way to control C. irritans infection, and some vaccines, such as inactivated C. irritans, DNA, or recombinant subunit vaccines, have been developed (4, 5, 11, 13, 21). However, large-scale production of these vaccines is costly. Thus, heterogenous vaccines may help solve this problem. It has been confirmed that cross-reactive protection is induced by vaccination with free-living ciliates, which are easy to grow in inexpensive culture media (11–13). In the present study, we found that immunization with T. thermophila provided grouper with protective immunity against C. irritans infection. The higher immobilization titer detected in immunized grouper serum suggested that an anti-parasite antibody was generated after inoculation and that cross-reactive antigens existed between C. irritans and T. thermophila. This hypothesis was investigated by screening C. irritans using T. thermophila–immunized grouper serum.
To identify the antigen in T. thermophila that contributes to the cross-reactive immunity, immunoprecipitation and mass spectrometry analyses were performed. We identified a C. irritans tubulin protein that was immunoprecipitated by the T. thermophila–immunized grouper IgM antibody. By immune proteomic screening, tubulin has been determined as one of the candidates for vaccine development against C. irritans (19). In addition, recombinant β-tubulin has been suggested as a target antigen to control scuticociliatosis, which is caused by parasitic ciliates (22). I-antigens are surface membrane proteins present on ciliates, and they are considered as the primary vaccine candidates against parasitic ciliates (23–25). However, as the protective immunity induced by I-antigens is serotype specific (26, 27), vaccination based on I-antigens may not cover different C. irritans serotypes existing in nature. Tubulin is a well-conserved protein in ciliates, and vaccines using this molecule may solve the problem raised by different serotypes (22). As expected, grouper immunized with rTt-tubulin showed induced protective immunity, but the protective rate was lower than that of T. thermophila–immunized grouper, which may due to other antigens existing in T. thermophila that may provide immunity stimulation.
Immunofluorescence staining showed that the surface cilia on C. irritans were stained by the antibody raised against rTt-tubulin. The large numbers of cilia present on the their surface is an identical structure characterized by ciliates (20), suggesting that a specific antibody targeting cilia may be an alternative method for eliminating parasitic ciliate infection. As tubulin is crucial for cilia assembly, the protection induced by rTt-tubulin was most likely due to the specific anti-tubulin antibody development in grouper. As expected, an increased level of parasite-binding IgMs was detected in rTt-tubulin–immunized grouper serum. Moreover, specific antibodies were also found in mucus and tissue culture medium from rTt-tubulin–immunized grouper. These data confirmed that the rTt-tubulin is a protective cross-reactive antigen, which may be a promising candidate for vaccine development.
In conclusion, vaccination with T. thermophila provided groupers immune protection against the C. irritans challenge. In addition, tubulin is one of the protective cross-reactive antigens contributing to this process, which stimulates the production of a specific antibody against C. irritans. Thus, using T. thermophila as a heterologous antigen is a potential method for vaccine development against C. irritans.
The raw data supporting the conclusions of this article will be made available by the authors, without undue reservation.
The animal study was reviewed and approved by the Animal Administration and Ethics Committee of College of Marine Sciences, South China Agricultural University.
Conceptualization: YL, XD, and ZM; Investigation: ZM, XL, HW, YH, WG, and YD; Methodology: ZM and HW; Project administration: XD and YL; Supervision: XD; Visualization: ZM; Writing—original draft: ZM and YL; Writing—review and editing: XD, YL, and ZM. All authors contributed to the article and approved the submitted version.
This work was supported by the National Natural Science Foundation of China (42006100, 41976081, and 41876162), the Guangdong Basic and Applied Basic Research Foundation (2019A1515110424), the China Postdoctoral Science Foundation (2019M662938), the Guangdong Provincial Special Fund for Modern Agriculture Industry Technology Innovation Teams (2019KJ141), and the China Modern Agricultural Industry Technology System (The Control of Parasites Infection on Marine Fish, CARS-47-18).
The authors declare that the research was conducted in the absence of any commercial or financial relationships that could be construed as a potential conflict of interest.
All claims expressed in this article are solely those of the authors and do not necessarily represent those of their affiliated organizations, or those of the publisher, the editors and the reviewers. Any product that may be evaluated in this article, or claim that may be made by its manufacturer, is not guaranteed or endorsed by the publisher.
The Supplementary Material for this article can be found online at: https://www.frontiersin.org/articles/10.3389/fimmu.2022.891643/full#supplementary-material
1. Li YW, Jiang BA, Mo ZQ, Li AX, Dan XM. Cryptocaryon Irritans (Brown 1951) is a Serious Threat to Aquaculture of Marine Fish. Rev Aquaculture (2021) 14:218–36. doi: 10.1111/raq.12594
2. Yambot AV, Song YL. Immunization of Grouper, Epinephelus Coioides, Confers Protection Against a Protozoan Parasite, Cryptocaryon Irritans. Aquaculture (2006) 260:1–9. doi: 10.1016/j.aquaculture.2006.05.055
3. Yoshinaga T, Nakazoe J-I. Acquired Protection and Production of Immunobilization Antibody Against Cryptocaryon Irritans (Ciliophora, Hymenostomatida) in Mummichog (Fundulus Heteroclitus). Fish Pathol (1997) 32:229–30. doi: 10.3147/jsfp.32.229
4. Bai JS, Xie MQ, Zhu XQ, Dan XM, Li AX. Comparative Studies on the Immunogenicity of Theronts, Tomonts and Trophonts of Cryptocaryon Irritans in Grouper. Parasitol Res (2008) 102:307–13. doi: 10.1007/s00436-007-0766-6
5. Luo XC, Xie MQ, Zhu XQ, Li AX. Protective Immunity in Grouper (Epinephelus Coioides) Following Exposure to or Injection With Cryptocaryon Irritans. Fish Shellfish Immunol (2007) 22:427–32. doi: 10.1016/j.fsi.2006.04.011
6. Jose Priya TA, Lin YH, Wang YC, Yang CS, Chang PS, Song YL. Codon Changed Immobilization Antigen (iAg), a Potent DNA Vaccine in Fish Against Cryptocaryon Irritans Infection. Vaccine (2012) 30:893–903. doi: 10.1016/j.vaccine.2011.11.102
7. Jose Priya TA, Chien KH, Lin HY, Huang HN, Wu CJ, Song YL. Immobilization Antigen Vaccine Adjuvanted by Parasitic Heat Shock Protein 70C Confers High Protection in Fish Against Cryptocaryonosis. Fish Shellfish Immunol (2015) 45:517–27. doi: 10.1016/j.fsi.2015.04.036
8. Mo ZQ, Xu S, Cassidy-Hanley DM, Li YW, Kolbin D, Fricke JM, et al. Characterization and Immune Regulation Role of an Immobilization Antigen From Cryptocaryon Irritans on Groupers. Sci Rep (2019) 9:1029. doi: 10.1038/s41598-018-25710-3
9. Yambot AV, Song YL. Short Term In Vitro Culture of Cryptocaryon Irritans, a Protozoan Parasite of Marine Fishes. Fish Pathol (2004) 39:175–81. doi: 10.3147/jsfp.39.175
10. Yoshinaga T, Akiyama K, Nishida S, Nakane M, Ogawa K, Hirose H. In Vitro Culture Technique for Cryptocaryon Irritans, a Parasitic Ciliate of Marine Teleosts. Dis Aquat Organisms (2007) 78:155–60. doi: 10.3354/dao01857
11. Dickerson HW, Brown J, Dawe DL, Gratzek JB. Tetrahymena Pyriformis as a Protective Antigen Against Ichthyophthirius Multifiliis Infection: Comparisons Between Isolates and Ciliary Preparations. J Fish Biol (1984) 24:523–8. doi: 10.1111/j.1095-8649.1984.tb04822.x
12. Goven BA, Dawe DL, Gratzek JB. Protection of Channel Catfish (Ictalurus Punctatus) Against Ichthyophthirius Multifiliis (Fouquet) by Immunization With Varying Doses of Tetrahymena Pyriformis (Lwoff) Cilia. Aquaculture (1981) 23:269–73. doi: 10.1016/0044-8486(81)90020-X
13. Ling KH, Sin YM, Lam TJ. Protection of Goldfish Against Some Common Ectoparasitic Protozoans Using Ichthyophthirius Multifiliis and Tetrahymena Pyriformis for Vaccination. Aquaculture (1993) 116:303–14. doi: 10.1016/0044-8486(93)90415-U
14. Mo ZQ, Li YW, Wang HQ, Wang JL, Ni LY, Yang M, et al. Comparative Transcriptional Profile of the Fish Parasite Cryptocaryon Irritans. Parasit Vectors (2016) 9:630. doi: 10.1186/s13071-016-1919-1
15. Mo ZQ, Wu HC, Hu YT, Lu ZJ, Lai XL, Chen HP, et al. Transcriptomic Analysis Reveals Innate Immune Mechanisms of an Underlying Parasite-Resistant Grouper Hybrid (Epinephelus Fuscogutatus X Epinephelus Lanceolatus). Fish Shellfish Immunol (2021) 119:67–75. doi: 10.1016/j.fsi.2021.09.041
16. Dan XM, Li AX, Lin XT, Teng N, Zhu XQ. A Standardized Method to Propagate Cryptocaryon Irritans on a Susceptible Host Pompano Trachinotus Ovatus. Aquaculture (2006) 258:127–33. doi: 10.1016/j.aquaculture.2006.04.026
17. Han Q, Hu Y, Lu Z, Wang J, Chen H, Mo ZQ, et al. Study on the Characterization of Grouper (Epinephelus Coioides) Immunoglobulin T and its Positive Cells. Fish Shellfish Immunol (2021) 118:102–10. doi: 10.1016/j.fsi.2021.08.031
18. Mo ZQ, Han Q, Zeng YL, Wang JL, Li XZ, Li YW, et al. Molecular Characterization and Function Analysis of Grouper (Epinephelus Coioides) Bruton's Tyrosine Kinase BTK. Fish Shellfish Immunol (2018) 77:91–9. doi: 10.1016/j.fsi.2018.03.039
19. Mai YZ, Li YW, Li RJ, Li W, Huang XZ, Mo ZQ, et al. Proteomic Analysis of Differentially Expressed Proteins in the Marine Fish Parasitic Ciliate Cryptocaryon Irritans. Veterinary Parasitol (2015) 211:1–11. doi: 10.1016/j.vetpar.2015.05.004
20. Bayless BA, Galati DF, Pearson CG. Tetrahymena Basal Bodies. Cilia (2015) 5:1. doi: 10.1186/s13630-016-0022-8
21. Xu Z, Takizawa F, Parra D, Gomez D, von Gersdorff Jorgensen L, LaPatra SE, et al. Mucosal Immunoglobulins at Respiratory Surfaces Mark an Ancient Association That Predates the Emergence of Tetrapods. Nat Commun (2016) 7:10728. doi: 10.1038/ncomms10728
22. Kim SM, Lee EH, Kwon SR, Lee SJ, Kim SK, Nam YK, et al. Preliminary Analysis of Recombinant β-Tubulin of Pseudocohnilembus Persalinus (Ciliophora: Scuticociliatida) as a Vaccine Antigen Candidate Against Scuticociliatosis. Aquaculture (2006) 260:21–6. doi: 10.1016/j.aquaculture.2006.06.016
23. Clark TG, Lin T-L, Dickerson HW. Surface Immobilization Antigens of Ichthyophthirius Multifiliis: Their Role in Protective Immunity. Annu Rev Fish Dis (1995) 5:113–31. doi: 10.1016/0959-8030(95)00005-4
24. Clark TG, Lin TL, Dickerson HW. Surface Antigen Cross-Linking Triggers Forced Exit of a Protozoan Parasite From its Host. Proc Natl Acad Sci United States America (1996) 93:6825–9. doi: 10.1073/pnas.93.13.6825
25. Hatanaka A, Umeda N, Yamashita S, Hirazawa N. Identification and Characterization of a Putative Agglutination/Immobilization Antigen on the Surface of Cryptocaryon Irritans. Parasitology (2007) 134:1163–74. doi: 10.1017/S003118200700265X
26. Hatanaka A, Umeda N, Hirazawa N. Molecular Cloning of a Putative Agglutination/Immobilization Antigen Located on the Surface of a Novel Agglutination/Immobilization Serotype of Cryptocaryon Irritans. Parasitology (2008) 135:1043–52. doi: 10.1017/S0031182008004617
Keywords: Cryptocaryon irritans, Tetrahymena thermophila, tubulin, heterologous vaccine, grouper
Citation: Mo Z, Wu H, Hu Y, Lai X, Guo W, Duan Y, Dan X and Li Y (2022) Protection of Grouper Against Cryptocaryon irritans by Immunization With Tetrahymena thermophila and Protective Cross-Reactive Antigen Identification. Front. Immunol. 13:891643. doi: 10.3389/fimmu.2022.891643
Received: 08 March 2022; Accepted: 03 May 2022;
Published: 07 July 2022.
Edited by:
Brian Dixon, University of Waterloo, CanadaReviewed by:
Jing Xing, Ocean University of China, ChinaCopyright © 2022 Mo, Wu, Hu, Lai, Guo, Duan, Dan and Li. This is an open-access article distributed under the terms of the Creative Commons Attribution License (CC BY). The use, distribution or reproduction in other forums is permitted, provided the original author(s) and the copyright owner(s) are credited and that the original publication in this journal is cited, in accordance with accepted academic practice. No use, distribution or reproduction is permitted which does not comply with these terms.
*Correspondence: Xueming Dan, ZHhtNzJAc2NhdS5lZHUuY24=; Yanwei Li, eWFud2VpbGlAc2NhdS5lZHUuY24=
†These authors have contributed equally to this work
Disclaimer: All claims expressed in this article are solely those of the authors and do not necessarily represent those of their affiliated organizations, or those of the publisher, the editors and the reviewers. Any product that may be evaluated in this article or claim that may be made by its manufacturer is not guaranteed or endorsed by the publisher.
Research integrity at Frontiers
Learn more about the work of our research integrity team to safeguard the quality of each article we publish.