- 1Division of Transplant Surgery and Transplant Surgery Research Laboratory, Brigham and Women’s Hospital, Harvard Medical School, Boston, MA, United States
- 2Institute of Medical Immunology, Charité Universitaetsmedizin Berlin, Berlin, Germany
- 3Division of Pulmonary and Critical Care Medicine, Brigham and Women’s Hospital, Boston, MA, United States
- 4Department of Medicine, Harvard Medical School, Boston, MA, United States
- 5Regenerative Medicine and Experimental Surgery, Department of General, Visceral and Transplant Surgery, Hannover Medical School, Hannover, Germany
- 6Department of Urology, Osaka Medical and Pharmaceutical University, Osaka, Japan
Transplant centers around the world have been using extended criteria donors to remedy the ongoing demand for lung transplantation. With a rapidly aging population, older donors are increasingly considered. Donor age, at the same time has been linked to higher rates of lung ischemia reperfusion injury (IRI). This process of acute, sterile inflammation occurring upon reperfusion is a key driver of primary graft dysfunction (PGD) leading to inferior short- and long-term survival. Understanding and improving the condition of older lungs is thus critical to optimize outcomes. Notably, ex vivo lung perfusion (EVLP) seems to have the potential of reconditioning ischemic lungs through ex-vivo perfusing and ventilation. Here, we aim to delineate mechanisms driving lung IRI and review both experimental and clinical data on the effects of aging in augmenting the consequences of IRI and PGD in lung transplantation.
Introduction
The world population is aging rapidly: predications suggest that more than 1.5 billion people above the age of 65 will inhabit our planet in 2050, accounting for 30 percent of the population (1). Similar trends are also observed in organ transplantation with increasing proportions of both, elderly donors and recipients (2, 3). Most donors are currently > than 50 years old and the proportion of donors > than 65 years has increased from 1 to 8% during the last decade (4, 5). United Network for Organ Sharing (UNOS) modeling suggests a potential of 22,000 available older donors/year (50-75 years) that are currently not considered (6). At least in theory, organs of those donors have the potential to narrow the gap between the ever-growing demand while measurements improving quality may therefore address prolonged waiting times and mortality on the waitlist. Not considering those organs or discarding them has been based on concerns of inferior outcomes (7, 8). Notably, it has been shown that older donor age decreases graft survival in hepatic, renal and heart transplantation (9–11). It is therefore likely that similar effects may be relevant in lung transplantation (12).
While lung donor selection criteria have historically been strict, thus limiting the donor pool, more recently, several transplant centers have used extended criteria donors including those from older donors with non-inferior outcomes (13–20). The clinical utilization of organs from donors > 65 years has nevertheless remained infrequent. A retrospective study examining outcomes of > than 10,000 lung transplant recipients reported an increase in 1- and 3-year mortality when transplanting lungs from donors >65 year. Using lungs from donors aged 55-64, however, has not been a risk factor for mortality and survival differences based on donor age have not been observed 30 days after transplantation (21). Primary graft dysfunction (PGD) represents one of the main risk factors for inferior short- and long-term survival (22–24). Interestingly, it remains unclear if donor age represents an independent risk factor for PGD (25–28).
Clinical Impact of Primary Graft Dysfunction on Lung Transplant Outcomes
Primary graft dysfunction is characterized by hypoxemia and alveolar infiltrates in the allograft within the first 72 hours after lung transplantation (29–31). Transplant-mediated immune signals originating from endothelial, epithelial cells and alveolar macrophages lead to excessive infiltration of monocytes, neutrophils, and T-cells. The subsequent release of pro-inflammatory cytokines, reactive oxygen intermediates, and proteolytic enzymes lead to graft dysfunction (32). Based on the International Society for Heart and Lung Transplantation (ISHLT) standardized definition, the severity of the injury is graded by a PGD score between 0-3 score with PGD-3 being the most severe stage (Table 1). By using only two clinical parameters (radiographic infiltrates, PaO2/FiO2 ratio) the classification contains both a time- and severity component, facilitating mechanistic and clinical trials (24, 29, 33). An analysis of the UNOS database including 7,322 first-time lung transplant recipients reported a 72hr post-transplant PGD-2 and 3 rates of 8.2% and 20.8%, respectively, findings that are in line with previous reports by others (34, 35). The incidence of PGD rates may differ depending on the assessment after transplantation with PGD-3 incidences of 19.8% and 15.4% reported 48 and 72hrs after transplantation (26). Although the risk of advanced PGD (stage 3) seems to be declining by day 3 after transplantation, the occurrence and its severity has a significant negative impact on both, short- and long-term survival (36). An analysis of > than 5,000 lung transplant recipients of the UNOS database reported a 30 day post-lung transplantation mortality of 9.7%, among which 43.6% had PGD indicating its role in defining early post-transplant outcomes after lung transplantation (37). In an additional single center cohort study of 1,000 adult lung recipients, medium- and long-term survival rates were significantly compromised in those that experienced PGD (graft survival by 1-, 5-, and 10-years in recipients with and without PGD: 72.8 vs. 87.1% vs., 43.9 vs. 59.8%, and 18.7% vs. 35.7%, p<0.001) (36). PGD was also identified as a risk factor for the development of bronchiolitis obliterans syndrome and other forms of chronic lung allograft rejection (36, 38).
Based on the clinical significance, several studies have been conducted aiming to define risk factors (39–43). A systemic review and meta-analysis of 13 studies published between 2000 to 2013 identified female gender, African American race, idiopathic pulmonary fibrosis, sarcoidosis, primary pulmonary hypertension, elevated BMI, and the use of cardiopulmonary bypass as significant risk factors for development of PGD. However, donor age as a risk for PGD was not assessed in the meta-analysis (25). Additional risk factors include a history of cigarette smoking and single lung transplants; cut-off times for ischemia have been discussed controversially (44–47). In 2016, the ISHLT working group reviewed donor, recipient, and surgical risk factors and found an association between donor age and reduced long-term survival, but its impact on PGD remain was unclear (48). While an earlier study reported a 7-fold increase in risk of severe PGD with donors beyond the age of 45 years, more recent studies have failed to confirm this association (28, 42, 49). At the same time, ischemia reperfusion injury (IRI) has been recognized as a key driver of PGD supported by a very recent integrated bioinformatics analysis that identified various IRI-PGD common pathways (25, 32, 50). Thus, it is crucial to understand the mechanisms which drive IRI to improve outcomes after lung transplantation.
Lung Ischemia Reperfusion Injury
Ischemia reperfusion injury represents an exacerbation of cellular dysfunction and cell death. Although restoration of blood flow is essential for recovery, reperfusion itself causes further damage, leading to a process of acute, sterile inflammation (51). Not being exclusive to transplantation medicine, damage after ischemia occurs in any tissue including the heart muscle following myocardial infarction or the brain after a stroke (52). In lung transplantation, this multi-factorial process leads to a complex pathology involving complex and broad molecular and cellular mechanisms (53). IRI thus distinguishes two phases of organ damage initiated with the discontinuation of blood supply (clamping of the organ during procurement) and a second phase at the time when blood flow is restored (reperfusion phase).
Reactive Oxygen Species (ROS) including superoxide, hydroxyl radicals and hydrogen peroxide play a key role in the development of IRI (54–57). Although low levels of ROS are a critical component of physiologic signaling pathways, the overload through both ischemia itself and reperfusion disturbs cellular function (58–60). This impairment is mainly driven by protein- and deoxyribonucleic acid-damage, alteration of signaling pathways and an augmentation of innate immune responses (61, 62). ROS are largely produced by alveolar type-II cells, vascular smooth muscle cells, endothelial cells and macrophages deriving from different sources including xanthine and NADPH oxidase, in addition to mitochondria (53, 63). Especially during reperfusion, restored oxygen facilitates the production of significant quantities of ROS (64, 65).
Of additional relevance, intracellular calcium overload has been proposed to be an initial step in the pathogenesis of the injury (66, 67). With a lack of oxygen, anaerobic glycolysis prevails, resulting in a decrease of intracellular pH caused by lactate and acid accumulation (65). Hydrogen ions accumulate leading to intracellular hypernatremia as a consequence of an accelerated Na+H+ exchange (68). Additionally, depletion of adenosine triphosphate (ATP) limits the activity of the Na+K+ ATPase and ATP-dependent calcium re-uptake. Hypernatremia leads to an additional calcium influx as accumulating Na+ is exchanged with Ca2+ (69). These mechanisms gain importance upon reperfusion, since the prompt normalization of the extracellular pH by pericellular washout results in a massive H+ gradient across the plasma membrane resulting in an accelerated calcium influx (65).
Both the excessive calcium accumulation and the overproduction of ROS represent key drivers for the formation of the mitochondrial permeability transition pore (mPTP) located at the inner mitochondrial membrane (70). Activation and opening of the mPTP has been proposed as one of the main driving forces of IRI (71–73). In addition to the structural damage caused by mitochondrial swelling, open mPTP facilitate the influx of hydrogen ions that uncouple the electron transport chain (ETC), further compromising ATP production (73, 74). Even under physiological conditions, ETC mediate a minor electron leakage. However, during reperfusion, electron leakage increases massively as a consequence of mitochondrial dysfunction with the production of large amounts of ROS once oxygen is reintroduced (75). Augmented ROS levels, damaged mitochondria with open mPTP accelerate ROS release furthermore, a process referred to as the ROS-induced ROS release (76, 77).
As part of the events during reperfusion, calcium overload and the excessive ROS generation trigger both, apoptosis and necrosis causing a further release of ROS, proinflammatory cytokines and damage-associated molecular patterns (DAMPs) consisting of peptides, proteins and nucleotide fragments (78). DAMPs represent endogenous danger signals that are normally carefully prevented from release to the extracellular space and differ from microorganism derived pathogen associated molecular patterns (PAMPs) (79, 80). Both PAMPs and DAMPs are mainly recognized by their pattern recognition receptors including Tolllike receptors that play an important role in the induction of innate immune responses (81–83).
Subsequently, the inflammatory cascade is initiated through, ROS- and DAMP-triggered activated resident lung macrophages as a key early source of multiple proinflammatory mediators that orchestrate lung IRI (84). This inflammatory milieu leads to pulmonary neutrophil infiltration, further exacerbating and maintaining lung inflammation and injury (85, 86). Released ROS and inflammatory cytokines upregulate and activate adhesion molecules including ICAM-1, CD18 and P-selectin on leukocytes and endothelial cells (79, 87). The activation of adhesion molecules facilitates the migration of neutrophils from their intravascular location to the lung interstitium where they release more ROS and proteolytic enzymes resulting into the destruction of cellular and extracellular matrix (88). This process is reinforced by an augmented expression of vascular endothelial growth factor during the hypoxic phase that increases vascular permeability during acute lung injury (89, 90). Complement activation seems to play an additional important role during IRI by mediating leukocyte chemotaxis and initiating cellular damage (91, 92). Moreover, widely injured endothelial cells decrease the production of nitric oxide facilitating, under physiological conditions, vasorelaxation, bronchodilation, immunomodulation, and maintenance of microvascular function (88, 93, 94). Additionally, neutrophil extracellular traps (NETs) have been found to accumulate in both, IRI and PGD after lung transplantation (95–97). Those data have also been confirmed clinically with higher concentrations of NETs in the bronchoalveolar lavage fluid of lung transplant recipients with PGD (96).
All these inflammatory pathways and mechanism contribute to an increased pulmonary vascular resistance and microvascular permeability (53, 98) leading to pulmonary edema and compromised gas exchanges as the clinical hallmarks of primary graft dysfunction of the lung (Figure 1) (29, 53).
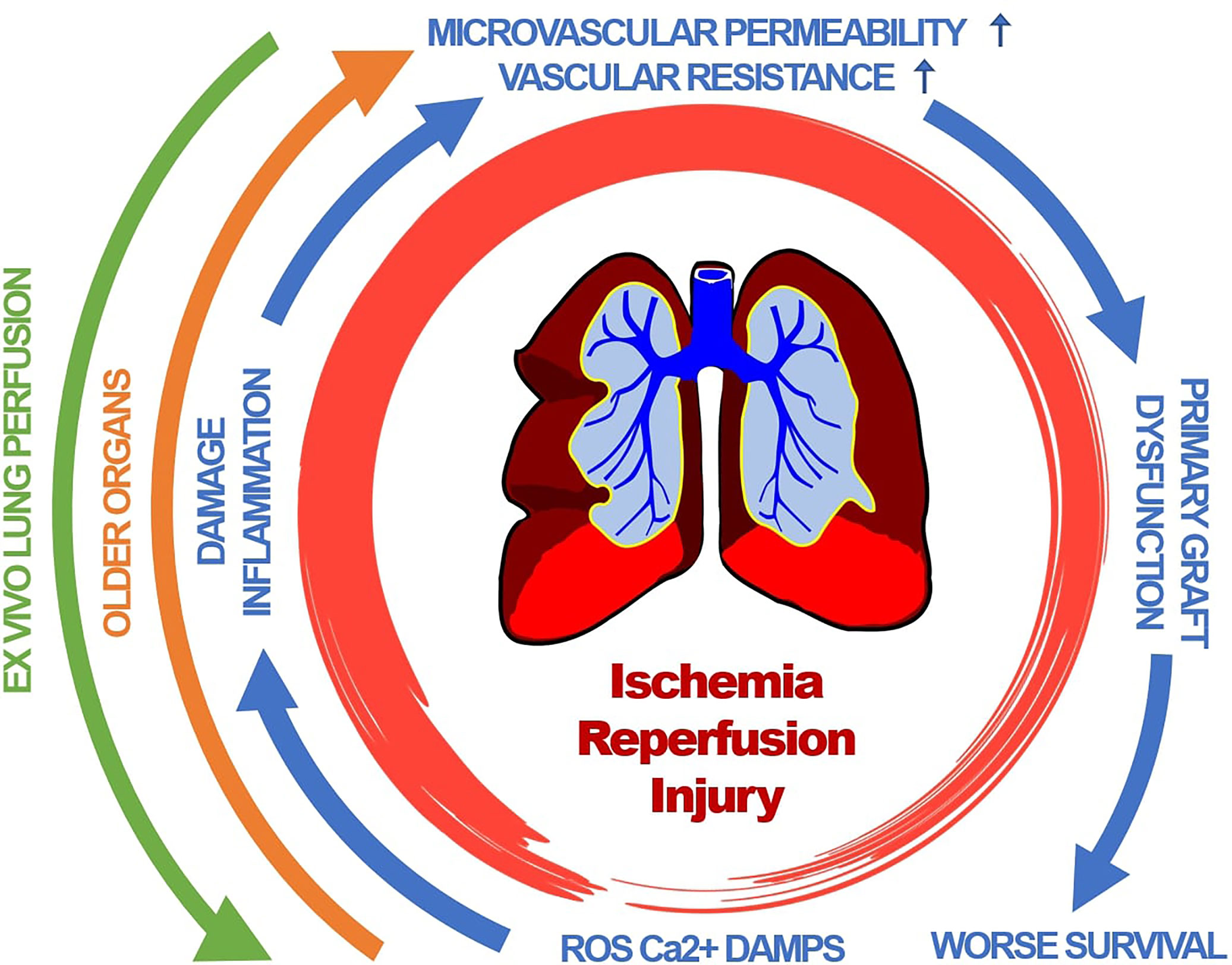
Figure 1 Primary graft dysfunction of lung transplants is driven by a complex cascade of pathophysiological events. Clinical hallmarks include pulmonary edema and compromised gas exchanges. Organ age accelerates those events. Therapeutic interventions include ex-vivo lung perfusion. ROS, reactive oxygen species; Ca2+, Calcium; DAMP, damage associated molecular pattern.
Impact of Aging on Ischemia Reperfusion Injury
Allogeneic lung transplantation represents the only curative approach for selected patients with end-stage lung disease. Advances in surgical techniques and immunosuppression therapy have improved graft survival rates to a median of 6.7 years compared to 4.7 year two decades ago (99). Of relevance, adult recipients who survived the first year after transplantation had a median survival of 8.9 years, emphasizing on the clinical relevance of PGD (100). Notably, with an increased experience and an augmented demand, donor age has increased steadily (101). Although early studies suggested that prolonged ischemia and increased donor age act synergistically towards worse survival rates, a recent report has failed to confirm this association (102–104).
While conclusive data in lung transplantation are currently lacking, data from other organ transplants support the concept that organ age augments damages subsequent to IRI: in hearts, this process has been driven, at least in part, through alterations in gene expression, signal transduction cascades, and mitochondrial dysfunction, resulting in an impaired intrinsic tolerance against damaging stress (105, 106). Notably, a decline of silent information regulator of transcription 3 (SIRT3) protein with age appears to be associated with an augmented damage in older hearts (107). There is also other strong experimental evidence that aging enhances the susceptibility of IRI in liver transplantation (108). A multivariate analysis of potential risk factors in orthotopic liver transplantation revealed that both donor age and prolonged cold ischemia times were independently associated with a higher incidence of primary dysfunction (109, 110). Experimentally, older livers have been more susceptible to IRI, linked to a depletion of both sirtuin-1 and mitofusin-2, resulting in mPTP onset/mitochondrial dysfunction, and cell death (111). Of additional interest, pretreating old rats with pooled young plasma appeared to reduce age-dependent liver IRI (112). It was further demonstrated that old rats experience more severe consequences of kidney IRI linked to an augmented immune response and increased oxidative stress, mechanisms that may also apply for IRI in lung transplantation (113, 114). A synergistic relationship between donor age and prolonged ischemia was also shown in an experimental renal allograft model leading to both functional and morphological deterioration after transplantation (115, 116).
Aging also impacts graft immunogenicity. In a broad clinical analysis of renal transplant recipients listed in the UNOS data base, we have been able to show that older grafts had higher rates of acute rejection within the first post-transplant year. Conversely, acute rejections were significantly lower in older recipients although they were more likely to receive an older organ (117). That grafts from older organs are more immunogenic has also been confirmed experimentally with an increase in T-cell alloreactivity, cytokine production observed early after transplantation (118). Moreover, it has also been shown that older donor age and prolonged warm ischemia time are both associated with an increased risk for rejection, contributing synergistically towards an augmented innate immune activation (119).
Clinically, elevated perioperative levels of cell-free circulating plasma mitochondrial DNA (cf-mt-DNA) have been observed in lung transplant recipients with moderate or severe PGD (120). We have recently shown that organ age and IRI act synergistically, leading to an increased DAMP release. Old mice that underwent renal IRI showed a 15x increase in cf-mt-DNA levels that act as a DAMP, inducing sterile inflammation. Remarkably, the pretreatment with senolytics that selectively kill senescent cells prior to IRI had the capacity to reduce both cf-mt-DNA and pro-inflammatory T-cells. These senescent cells accumulate with age and contribute through the secretion of a myriad of pro-inflammatory factors termed the senescence-associated secretory phenotype (SASP) to a proinflammatory environment (121–124). Of additional clinical relevance, senolytics applied to organ donors prior to procurement, prolonged the survival of old cardiac allografts beyond that of young donors (125). Most recently, experimental models have indicated that IRI itself can induce senescence and that senolytics have the potential to ameliorate this injury (126–128). Notably, senolytics have been tested before in patients with idiopathic pulmonary fibrosis. This first in-human study supported the feasibility of senolytics to interfere with lung injury (129, 130). Thus, senolytics may represent, at least in theory, a therapeutic opportunity to improve the equality of older lungs for transplantation.
Ex vivo Lung Perfusion
Due to peri transplant complications such as PGD and its impact on long-term survival, careful selection of donor lungs is crucial, however, these can lead to lower donor utilization rates of 15% to 25% from multiorgan donors (131–133). Mortality remains high for patients waiting for lung transplantation. In 2017, 326 patients died or became too sick to undergo lung transplantation in the US accounting for > than 10% of the removals (134). In the past decade, modern technologies including Ex vivo lung perfusion (EVLP) have facilitated the evaluation and reconditioning of marginal donor lungs including those from older lungs, thus augmenting the pool of prospective donor organs (135, 136). Notably, lungs from older donors are more likely to undergo EVLP (137). This novel approach allows explanted donor lungs to be preserved in perfused, ventilated and normothermic condition, decreasing tissue ischemia and lung damage (138). Three different systems and protocols are currently used clinically: the Vivoline® LS1 system (Vivoline Medical, Lund, Sweden), the Organ Care System™ Lung (OCS, Transmedics, Andover, MA), and the XPS™ XVIVO Perfusion AB system (XVIVO Perfusion, Goetheborg, Sweden) (139).
In many ways, lung preservation has ploughed the way for novel preservation methods for other organs. In a prospective, non-randomized clinical trial, the Toronto group transplanted 20 high risk lungs which were evaluated for 4 hours while being perfused ex-vivo by the XVIVO Perfusion AB system. During this two-year period, 116 non-high-risk lungs were transplanted without EVLP and used as controls. Lungs in the EVLP group showed an improved PaO2/FiO2 ratio after EVLP resulting into a reduced PGD incidence (140). Recently, a randomized, open-label, phase 3 trial used the OCS system in 151 patients and compared them to 169 standard protocol recipients (cold static storage). This study also demonstrated reduced PGD rates (with and without EVLP: vs 29.7% vs. 17.7%, p=0.015), however not resulting into improved short-term survival (141).
Conclusion
Understanding the link between organ age, IRI and graft immunogenicity will be critical in optimally utilizing available lungs for transplantation. Although, the bulk of available data both, clinically and experimentally is currently provided through evidence outside of lung transplantation it can be assumed that data from other organ systems do also apply for lungs. The elimination of senescent cells and the assessment of older organs on machine perfusion devices may help safely increase the number of available lungs for transplant.
Author Contributions
All authors listed have made substantial contributions to the concept of this work, helped writing and editing the manuscript and approved the final version for publication.
Funding
This work was supported by the Biomedical Education Program (BMEP) of the German Academic Exchange Service (MJR and AS), the Osaka Medical College Foundation (to TM), NIH grant R01AG064165 (to SGT) and a grant by the Pepper Foundation (to HZ).
Conflict of Interest
The authors declare that the research was conducted in the absence of any commercial or financial relationships that could be construed as a potential conflict of interest.
Publisher’s Note
All claims expressed in this article are solely those of the authors and do not necessarily represent those of their affiliated organizations, or those of the publisher, the editors and the reviewers. Any product that may be evaluated in this article, or claim that may be made by its manufacturer, is not guaranteed or endorsed by the publisher.
References
1. United Nations, Department of Economic and Social Affairs, Population Division. World Population Ageing 2019 (ST/ESA/SER.A/444). Secretariat for the Convention on the Rights of Persons with Disabilities United Nations Department of Economic and Social Affairs (UN-DESA) Division for Social Policy and Development Two United Nations Plaza New York, NY United States of America (2020).
2. Valapour M, Lehr CJ, Skeans MA, Smith JM, Miller E, Goff R, et al. OPTN/SRTR 2019 Annual Data Report: Lung. Am J Transplant (2021) 21 Suppl 2:441–520. doi: 10.1111/ajt.16495
3. Yusen RD, Christie JD, Edwards LB, Kucheryavaya AY, Benden C, Dipchand AI, et al. The Registry of the International Society for Heart and Lung Transplantation: Thirtieth Adult Lung and Heart-Lung Transplant Report–2013; Focus Theme: Age. J Heart Lung Transplant (2013) 32(10):965–78. doi: 10.1016/j.healun.2013.08.007
4. Department of Health and Human Services HRaSA, Healthcare Systems Bureau, Division of Transplantation, Rockville, MD. United Network for Organ Sharing, Richmond. VA; Univ Renal Res Educ Association Ann Arbor MI. Deceased Donors Recovered U.S. by Donor Age OPTN/SRTR Annu Data Rep. Hoboken NJ, USA: Wiley-Blackwell (2020).
5. Israni AK, Zaun D, Rosendale JD, Schaffhausen C, McKinney W, Snyder JJ. OPTN/SRTR 2019 Annual Data Report: Deceased Organ Donors. Am J Transplant (2021) 21 Suppl 2:521–58. doi: 10.1111/ajt.16491
6. Klassen DK, Edwards LB, Stewart DE, Glazier AK, Orlowski JP, Berg CL. The OPTN Deceased Donor Potential Study: Implications for Policy and Practice. Am J Transplant (2016) 16(6):1707–14. doi: 10.1111/ajt.13731
7. Klein AS, Messersmith EE, Ratner LE, Kochik R, Baliga PK, Ojo AO. Organ Donation and Utilization in The United States, 1999-2008. Am J Transplant. (2010) 10(4 Pt 2):973–86. doi: 10.1111/j.1600-6143.2009.03008.x
8. Oberhuber R, Heinbokel T, Cetina Biefer HR, Boenisch O, Hock K, Bronson RT, et al. CD11c+ Dendritic Cells Accelerate the Rejection of Older Cardiac Transplants via Interleukin-17A. Circulation (2015) 132(2):122–31. doi: 10.1161/CIRCULATIONAHA.114.014917
9. Cuende N, Miranda B, Canon JF, Garrido G, Matesanz R. Donor Characteristics Associated With Liver Graft Survival. Transplantation (2005) 79(10):1445–52. doi: 10.1097/01.TP.0000158877.74629.AA
10. Oppenheimer F, Aljama P, Asensio Peinado C, Bustamante Bustamante J, Crespo Albiach JF, Guirado Perich L. The Impact of Donor Age on the Results of Renal Transplantation. Nephrol Dial Transplant (2004) 19 (Suppl 3):iii11–5. doi: 10.1093/ndt/gfh1008
11. Russo MJ, Chen JM, Sorabella RA, Martens TP, Garrido M, Davies RR, et al. The Effect of Ischemic Time on Survival After Heart Transplantation Varies by Donor Age: An Analysis of the United Network for Organ Sharing Database. J Thorac Cardiovasc Surg (2007) 133(2):554–9. doi: 10.1016/j.jtcvs.2006.09.019
12. Botha P, Rostron AJ, Fisher AJ, Dark JH. Current Strategies in Donor Selection and Management. Semin Thorac Cardiovasc Surg (2008) 20(2):143–51. doi: 10.1053/j.semtcvs.2008.04.006
13. Bhorade SM, Vigneswaran W, McCabe MA, Garrity ER. Liberalization of Donor Criteria May Expand The Donor Pool Without Adverse Consequence in Lung Transplantation. J Heart Lung Transplant (2000) 19(12):1199–204. doi: 10.1016/S1053-2498(00)00215-1
14. Christie IG, Chan EG, Ryan JP, Harano T, Morrell M, Luketich JD, et al. National Trends in Extended Criteria Donor Utilization and Outcomes for Lung Transplantation. Ann Thorac Surg (2021) 111(2):421–6. doi: 10.1016/j.athoracsur.2020.05.087
15. Dahlman S, Jeppsson A, Schersten H, Nilsson F. Expanding the Donor Pool: Lung Transplantation With Donors 55 Years and Older. Transplant Proc (2006) 38(8):2691–3. doi: 10.1016/j.transproceed.2006.07.037
16. Fischer S, Gohrbandt B, Struckmeier P, Niedermeyer J, Simon A, Hagl C, et al. Lung Transplantation With Lungs From Donors Fifty Years of Age And Older. J Thorac Cardiovasc Surg (2005) 129(4):919–25. doi: 10.1016/j.jtcvs.2004.07.053
17. Kotecha S, Hobson J, Fuller J, Paul E, Levvey BJ, Whitford H, et al. Continued Successful Evolution of Extended Criteria Donor Lungs for Transplantation. Ann Thorac Surg (2017) 104(5):1702–9. doi: 10.1016/j.athoracsur.2017.05.042
18. Meers C, Van Raemdonck D, Verleden GM, Coosemans W, Decaluwe H, De Leyn P, et al. The Number of Lung Transplants Can Be Safely Doubled Using Extended Criteria Donors; A Single-Center Review. Transpl Int (2010) 23(6):628–35. doi: 10.1111/j.1432-2277.2009.01033.x
19. Neizer H, Singh GB, Gupta S, Singh SK. Addressing Donor-Organ Shortages Using Extended Criteria in Lung Transplantation. Ann Cardiothorac Surg (2020) 9(1):49–50. doi: 10.21037/acs.2019.10.01
20. Somers J, Ruttens D, Verleden SE, Cox B, Stanzi A, Vandermeulen E, et al. A Decade of Extended-Criteria Lung Donors in A Single Center: Was It Justified? Transpl Int (2015) 28(2):170–9. doi: 10.1111/tri.12470
21. Bittle GJ, Sanchez PG, Kon ZN, Claire Watkins A, Rajagopal K, Pierson RN 3rd, et al. The Use of Lung Donors Older Than 55 Years: A Review of the United Network of Organ Sharing Database. J Heart Lung Transplant (2013) 32(8):760–8. doi: 10.1016/j.healun.2013.04.012
22. Arcasoy SM, Kotloff RM. Lung Transplantation. N Engl J Med (1999) 340(14):1081–91. doi: 10.1056/NEJM199904083401406
23. Christie JD, Sager JS, Kimmel SE, Ahya VN, Gaughan C, Blumenthal NP, et al. Impact of Primary Graft Failure on Outcomes Following Lung Transplantation. Chest (2005) 127(1):161–5. doi: 10.1378/chest.127.1.161
24. Snell GI, Yusen RD, Weill D, Strueber M, Garrity E, Reed A, et al. Report of the ISHLT Working Group on Primary Lung Graft Dysfunction, part I: Definition and Grading-A 2016 Consensus Group statement of the International Society for Heart and Lung Transplantation. J Heart Lung Transplant (2017) 36(10):1097–103. doi: 10.1016/j.healun.2017.07.021
25. Liu Y, Liu Y, Su L, Jiang SJ. Recipient-Related Clinical Risk Factors for Primary Graft Dysfunction After Lung Transplantation: A Systematic Review and Meta-Analysis. PloS One (2014) 9(3):e92773. doi: 10.1371/journal.pone.0092773
26. Samano MN, Fernandes LM, Baranauskas JC, Correia AT, Afonso JE Jr, Teixeira RH, et al. Risk Factors and Survival Impact of Primary Graft Dysfunction After Lung Transplantation in a Single Institution. Transplant Proc (2012) 44(8):2462–8. doi: 10.1016/j.transproceed.2012.07.134
27. Whitson BA, Nath DS, Johnson AC, Walker AR, Prekker ME, Radosevich DM, et al. Risk Factors for Primary Graft Dysfunction After Lung Transplantation. J Thorac Cardiovasc Surg (2006) 131(1):73–80. doi: 10.1016/j.jtcvs.2005.08.039
28. Christie JD, Kotloff RM, Pochettino A, Arcasoy SM, Rosengard BR, Landis JR, et al. Clinical Risk Factors for Primary Graft Failure Following Lung Transplantation. Chest (2003) 124(4):1232–41. doi: 10.1378/chest.124.4.1232
29. Christie JD, Carby M, Bag R, Corris P, Hertz M, Weill D. et al. Report of the ISHLT Working Group on Primary Lung Graft Dysfunction Part II: Definition. A Consensus Statement of the International Society for Heart and Lung Transplantation. J Heart Lung Transplant (2005) 24(10):1454–9. doi: 10.1016/j.healun.2004.11.049
30. Lee JC, Christie JD, Keshavjee S. Primary Graft Dysfunction: Definition, Risk Factors, Short- and Long-Term Outcomes. Semin Respir Crit Care Med (2010) 31(2):161–71. doi: 10.1055/s-0030-1249111
31. Porteous MK, Lee JC. Primary Graft Dysfunction After Lung Transplantation. Clin Chest Med (2017) 38(4):641–54. doi: 10.1016/j.ccm.2017.07.005
32. Gelman AE, Fisher AJ, Huang HJ, Baz MA, Shaver CM, Egan TM, et al. Report of the ISHLT Working Group on Primary Lung Graft Dysfunction Part III: Mechanisms: A 2016 Consensus Group Statement of the International Society for Heart and Lung Transplantation. J Heart Lung Transplant (2017) 36(10):1114–20. doi: 10.1016/j.healun.2017.07.014
33. Thabut G, Vinatier I, Stern JB, Leseche G, Loirat P, Fournier M, et al. Primary Graft Failure Following Lung Transplantation: Predictive Factors of Mortality. Chest (2002) 121(6):1876–82. doi: 10.1378/chest.121.6.1876
34. Jawitz OK, Raman V, Bryner BS, Klapper J, Hartwig MG. Center Volume and Primary Graft Dysfunction in Patients Undergoing Lung Transplantation in the United States - a Cohort Study. Transpl Int (2021) 34(1):194–203. doi: 10.1111/tri.13784
35. Porteous MK, Diamond JM, Christie JD. Primary Graft Dysfunction: Lessons Learned About the First 72 H After Lung Transplantation. Curr Opin Organ Transplant (2015) 20(5):506–14. doi: 10.1097/MOT.0000000000000232
36. Kreisel D, Krupnick AS, Puri V, Guthrie TJ, Trulock EP, Meyers BF, et al. Short- and Long-Term Outcomes of 1000 Adult Lung Transplant Recipients at a Single Center. J Thorac Cardiovasc Surg (2011) 141(1):215–22. doi: 10.1016/j.jtcvs.2010.09.009
37. Christie JD, Kotloff RM, Ahya VN, Tino G, Pochettino A, Gaughan C, et al. The Effect of Primary Graft Dysfunction on Survival After Lung Transplantation. Am J Respir Crit Care Med (2005) 171(11):1312–6. doi: 10.1164/rccm.200409-1243OC
38. Whitson BA, Prekker ME, Herrington CS, Whelan TP, Radosevich DM, Hertz MI, et al. Primary Graft Dysfunction and Long-Term Pulmonary Function After Lung Transplantation. J Heart Lung Transplant (2007) 26(10):1004–11. doi: 10.1016/j.healun.2007.07.018
39. Barr ML, Kawut SM, Whelan TP, Girgis R, Bottcher H, Sonett J, et al. Report of the ISHLT Working Group on Primary Lung Graft Dysfunction Part IV: Recipient-Related Risk Factors and Markers. J Heart Lung Transplant (2005) 24(10):1468–82. doi: 10.1016/j.healun.2005.02.019
40. King RC, Binns OA, Rodriguez F, Kanithanon RC, Daniel TM, Spotnitz WD, et al. Reperfusion Injury Significantly Impacts Clinical Outcome After Pulmonary Transplantation. Ann Thorac Surg (2000) 69(6):1681–5. doi: 10.1016/S0003-4975(00)01425-9
41. Kuntz CL, Hadjiliadis D, Ahya VN, Kotloff RM, Pochettino A, Lewis J, et al. Risk Factors for Early Primary Graft Dysfunction After Lung Transplantation: A Registry Study. Clin Transplant (2009) 23(6):819–30. doi: 10.1111/j.1399-0012.2008.00951.x
42. Shigemura N, Horai T, Bhama JK, D'Cunha J, Zaldonis D, Toyoda Y, et al. Lung Transplantation With Lungs From Older Donors: Recipient and Surgical Factors Affect Outcomes. Transplantation (2014) 98(8):903–8. doi: 10.1097/TP.0000000000000134
43. Shigemura N, Toyoda Y, Bhama JK, Gries CJ, Crespo M, Johnson B, et al. Donor Smoking History and Age in Lung Transplantation: A Revisit. Transplantation (2013) 95(3):513–8. doi: 10.1097/TP.0b013e3182751f1f
44. Diamond JM, Lee JC, Kawut SM, Shah RJ, Localio AR, Bellamy SL, et al. Clinical Risk Factors for Primary Graft Dysfunction After Lung Transplantation. Am J Respir Crit Care Med (2013) 187(5):527–34. doi: 10.1164/rccm.201210-1865OC
45. Fiser SM, Kron IL, Long SM, Kaza AK, Kern JA, Cassada DC, et al. Influence of Graft Ischemic Time on Outcomes Following Lung Transplantation. J Heart Lung Transplant (2001) 20(12):1291–6. doi: 10.1016/S1053-2498(01)00355-2
46. Gammie JS, Stukus DR, Pham SM, Hattler BG, McGrath MF, McCurry KR, et al. Effect of Ischemic Time on Survival in Clinical Lung Transplantation. Ann Thorac Surg (1999) 68(6):2015–9. doi: 10.1016/S0003-4975(99)00903-0
47. Grimm JC, Valero V 3rd, Kilic A, Magruder JT, Merlo CA, Shah PD, et al. Association Between Prolonged Graft Ischemia and Primary Graft Failure or Survival Following Lung Transplantation. JAMA Surg (2015) 150(6):547–53. doi: 10.1001/jamasurg.2015.12
48. Diamond JM, Arcasoy S, Kennedy CC, Eberlein M, Singer JP, Patterson GM, et al. Report of the International Society for Heart and Lung Transplantation Working Group on Primary Lung Graft Dysfunction, Part II: Epidemiology, Risk Factors, and Outcomes-a 2016 Consensus Group Statement of the International Society for Heart and Lung Transplantation. J Heart Lung Transplant (2017) 36(10):1104–13. doi: 10.1016/j.healun.2017.07.020
49. Lopez I, Zapata R, Sole J, Jauregui A, Deu M, Romero L, et al. Early and Mid-Term Results of Lung Transplantation With Donors 60 Years and Older. Interact Cardiovasc Thorac Surg (2015) 20(1):47–53. doi: 10.1093/icvts/ivu320
50. Zheng XY, Huang H, Wei ZT, Yan HJ, Wang XW, Xu L, et al. Genetic Effect of Ischemia-Reperfusion Injury Upon Primary Graft Dysfunction and Chronic Lung Allograft Dysfunction in Lung Transplantation: Evidence Based on Transcriptome Data. Transpl Immunol (2022) 71:101556. doi: 10.1016/j.trim.2022.101556
51. Cowled P, Fitridge R, Fitridge R, Thompson M. Adelaide (AU). University of Adelaide Press (2011).
52. Collard CD, Gelman S. Pathophysiology, Clinical Manifestations, and Prevention of Ischemia-Reperfusion Injury. Anesthesiology (2001) 94(6):1133–8. doi: 10.1097/00000542-200106000-00030
53. den Hengst WA, Gielis JF, Lin JY, Van Schil PE, De Windt LJ, Moens AL. Lung Ischemia-Reperfusion Injury: A Molecular and Clinical View on a Complex Pathophysiological Process. Am J Physiol Heart Circ Physiol (2010) 299(5):H1283–99. doi: 10.1152/ajpheart.00251.2010
54. Laubach VE, Sharma AK. Mechanisms of Lung Ischemia-Reperfusion Injury. Curr Opin Organ Transplant (2016) 21(3):246–52. doi: 10.1097/MOT.0000000000000304
55. Pak O, Sydykov A, Kosanovic D, Schermuly RT, Dietrich A, Schroder K, et al. Lung Ischaemia-Reperfusion Injury: The Role of Reactive Oxygen Species. Adv Exp Med Biol (2017) 967:195–225. doi: 10.1007/978-3-319-63245-2_12
56. Talaie T, DiChiacchio L, Prasad NK, Pasrija C, Julliard W, Kaczorowski DJ, et al. Ischemia-Reperfusion Injury in the Transplanted Lung: A Literature Review. Transplant Direct (2021) 7(2):e652. doi: 10.1097/TXD.0000000000001104
57. White CW, Messer SJ, Large SR, Conway J, Kim DH, Kutsogiannis DJ, et al. Transplantation of Hearts Donated After Circulatory Death. Front Cardiovasc Med (2018) 5:8. doi: 10.3389/fcvm.2018.00008
58. Egemnazarov B, Sydykov A, Schermuly RT, Weissmann N, Stasch JP, Sarybaev AS, et al. Novel Soluble Guanylyl Cyclase Stimulator BAY 41-2272 Attenuates Ischemia-Reperfusion-Induced Lung Injury. Am J Physiol Lung Cell Mol Physiol (2009) 296(3):L462–9. doi: 10.1152/ajplung.90377.2008
59. Lima B, Forrester MT, Hess DT, Stamler JS. S-Nitrosylation in Cardiovascular Signaling. Circ Res (2010) 106(4):633–46. doi: 10.1161/CIRCRESAHA.109.207381
60. Zhu X, Zuo L. Characterization of Oxygen Radical Formation Mechanism at Early Cardiac Ischemia. Cell Death Dis (2013) 4:e787. doi: 10.1038/cddis.2013.313
61. Kalogeris T, Baines CP, Krenz M, Korthuis RJ. Cell Biology of Ischemia/Reperfusion Injury. Int Rev Cell Mol Biol (2012) 298:229–317. doi: 10.1016/B978-0-12-394309-5.00006-7
62. Schroder K. NADPH Oxidases in Redox Regulation of Cell Adhesion and Migration. Antioxid Redox Signal (2014) 20(13):2043–58. doi: 10.1089/ars.2013.5633
63. Granger DN, Kvietys PR. Reperfusion Injury and Reactive Oxygen Species: The Evolution of a Concept. Redox Biol (2015) 6:524–51. doi: 10.1016/j.redox.2015.08.020
64. Chen W, Li D. Reactive Oxygen Species (ROS)-Responsive Nanomedicine for Solving Ischemia-Reperfusion Injury. Front Chem (2020) 8:732. doi: 10.3389/fchem.2020.00732
65. Sanada S, Komuro I, Kitakaze M. Pathophysiology of Myocardial Reperfusion Injury: Preconditioning, Postconditioning, and Translational Aspects of Protective Measures. Am J Physiol Heart Circ Physiol (2011) 301(5):H1723–41. doi: 10.1152/ajpheart.00553.2011
66. Chen-Yoshikawa TF. Ischemia-Reperfusion Injury in Lung Transplantation. Cells (2021) 10(6). doi: 10.3390/cells10061333
67. Weissmann N, Sydykov A, Kalwa H, Storch U, Fuchs B, Mederos y Schnitzler M, et al. Activation of TRPC6 Channels Is Essential for Lung Ischaemia-Reperfusion Induced Oedema in Mice. Nat Commun (2012) 3:649. doi: 10.1038/ncomms1660
68. Pike MM, Luo CS, Clark MD, Kirk KA, Kitakaze M, Madden MC, et al. NMR Measurements of Na+ and Cellular Energy in Ischemic Rat Heart: Role of Na(+)-H+ Exchange. Am J Physiol (1993) 265Pt 2):H2017–26. doi: 10.1152/ajpheart.1993.265.6.H2017
69. Schafer C, Ladilov Y, Inserte J, Schafer M, Haffner S, Garcia-Dorado D, et al. Role of the Reverse Mode of the Na+/Ca2+ Exchanger in Reoxygenation-Induced Cardiomyocyte Injury. Cardiovasc Res (2001) 51(2):241–50. doi: 10.1016/S0008-6363(01)00282-6
70. Bauer TM, Murphy E. Role of Mitochondrial Calcium and the Permeability Transition Pore in Regulating Cell Death. Circ Res (2020) 126(2):280–93. doi: 10.1161/CIRCRESAHA.119.316306
71. Cosgun T, Iskender I, Yamada Y, Arni S, Lipiski M, van Tilburg K, et al. Ex vivo Administration of Trimetazidine Improves Post-Transplant Lung Function in Pig Mode. Eur J Cardiothorac Surg (2017) 52(1):171–7. doi: 10.1093/ejcts/ezx053
72. Di Lisa F, Bernardi P. A CaPful Of Mechanisms Regulating the Mitochondrial Permeability Transition. J Mol Cell Cardiol (2009) 46(6):775–80. doi: 10.1016/j.yjmcc.2009.03.006
73. Halestrap AP, Pasdois P. The Role of the Mitochondrial Permeability Transition Pore in Heart Disease. Biochim Biophys Acta (2009) 1787(11):1402–15. doi: 10.1016/j.bbabio.2008.12.017
74. Honda HM, Korge P, Weiss JN. Mitochondria and Ischemia/Reperfusion Injury. Ann N Y Acad Sci (2005) 1047:248–58. doi: 10.1196/annals.1341.022
75. Chen YR, Zweier JL. Cardiac Mitochondria and Reactive Oxygen Species Generation. Circ Res (2014) 114(3):524–37. doi: 10.1161/CIRCRESAHA.114.300559
76. Zorov DB, Filburn CR, Klotz LO, Zweier JL, Sollott SJ. Reactive Oxygen Species (ROS)-Induced ROS Release: A New Phenomenon Accompanying Induction of the Mitochondrial Permeability Transition in Cardiac Myocytes. J Exp Med (2000) 192(7):1001–14. doi: 10.1084/jem.192.7.1001
77. Zorov DB, Juhaszova M, Sollott SJ. Mitochondrial Reactive Oxygen Species (ROS) and ROS-Induced ROS Release. Physiol Rev (2014) 94(3):909–50. doi: 10.1152/physrev.00026.2013
78. Sanada S, Kitakaze M. Ischemic Preconditioning: Emerging Evidence, Controversy, and Translational Trials. Int J Cardiol (2004) 97(2):263–76. doi: 10.1016/j.ijcard.2003.12.002
79. Patel PM, Connolly MR, Coe TM, Calhoun A, Pollok F, Markmann JF, et al. Minimizing Ischemia Reperfusion Injury in Xenotransplantation. Front Immunol (2021) 12:681504. doi: 10.3389/fimmu.2021.681504
80. Roh JS, Sohn DH. Damage-Associated Molecular Patterns in Inflammatory Diseases. Immune Netw (2018) 18(4):e27. doi: 10.4110/in.2018.18.e27
81. Arslan F, de Kleijn DP, Pasterkamp G. Innate Immune Signaling in Cardiac Ischemia. Nat Rev Cardiol (2011) 8(5):292–300. doi: 10.1038/nrcardio.2011.38
82. Kawashima M, Juvet SC. The Role of Innate Immunity in the Long-Term Outcome of Lung Transplantation. Ann Transl Med (2020) 8(6):412. doi: 10.21037/atm.2020.03.20
83. Kumar H, Kawai T, Akira S. Pathogen Recognition by the Innate Immune System. Int Rev Immunol (2011) 30(1):16–34. doi: 10.3109/08830185.2010.529976
84. Naidu BV, Krishnadasan B, Farivar AS, Woolley SM, Thomas R, Van Rooijen N, et al. Early Activation of the Alveolar Macrophage Is Critical to the Development of Lung Ischemia-Reperfusion Injury. J Thorac Cardiovasc Surg (2003) 126(1):200–7. doi: 10.1016/S0022-5223(03)00390-8
85. Hu R, Chen ZF, Yan J, Li QF, Huang Y, Xu H, et al. Endoplasmic Reticulum Stress of Neutrophils Is Required for Ischemia/Reperfusion-Induced Acute Lung Injury. J Immunol (2015) 195(10):4802–9. doi: 10.4049/jimmunol.1500073
86. Ross SD, Tribble CG, Gaughen JR Jr, Shockey KS, Parrino PE, Kron IL. Reduced Neutrophil Infiltration Protects Against Lung Reperfusion Injury After Transplantation. Ann Thorac Surg (1999) 67(5):1428–33. doi: 10.1016/S0003-4975(99)00248-9
87. Moore TM, Khimenko P, Adkins WK, Miyasaka M, Taylor AE. Adhesion Molecules Contribute to Ischemia and Reperfusion-Induced Injury in the Isolated Rat Lung. J Appl Physiol (1985 1995) 78(6):2245–52. doi: 10.1152/jappl.1995.78.6.2245
88. Gourdin MJ, Bree B, De Kock M. The Impact of Ischaemia-Reperfusion on the Blood Vessel. Eur J Anaesthesiol (2009) 26(7):537–47. doi: 10.1097/EJA.0b013e328324b7c2
89. Abraham D, Taghavi S, Riml P, Paulus P, Hofmann M, Baumann C, et al. VEGF-A and -C but Not -B Mediate Increased Vascular Permeability in Preserved Lung Grafts. Transplantation (2002) 73(11):1703–6. doi: 10.1097/00007890-200206150-00003
90. Kaner RJ, Ladetto JV, Singh R, Fukuda N, Matthay MA, Crystal RG. Lung Overexpression of the Vascular Endothelial Growth Factor Gene Induces Pulmonary Edema. Am J Respir Cell Mol Biol (2000) 22(6):657–64. doi: 10.1165/ajrcmb.22.6.3779
91. Ali HA, Pavlisko EN, Snyder LD, Frank M, Palmer SM. Complement System in Lung Transplantation. Clin Transplant (2018) 32(4):e13208. doi: 10.1111/ctr.13208
92. Kulkarni HS, Ramphal K, Ma L, Brown M, Oyster M, Speckhart KN, et al. Local Complement Activation Is Associated With Primary Graft Dysfunction After Lung Transplantation. JCI Insight (2020) 5(17). doi: 10.1172/jci.insight.138358
93. Jungraithmayr W. Novel Strategies for Endothelial Preservation in Lung Transplant Ischemia-Reperfusion Injury. Front Physiol (2020) 11:581420. doi: 10.3389/fphys.2020.581420
94. Zheng ZK, Wang JJ, Hu H, Jiang K, Nie J, Zhang J, et al. Short-term Inhalation of Nitric Oxide Inhibits Activations of Toll-Like Receptor 2 and 4 in the Lung After Ischemia-Reperfusion Injury in Mice. J Huazhong Univ Sci Technolog Med Sci (2013) 33(2):219–23. doi: 10.1007/s11596-013-1100-4
95. Ge L, Zhou X, Ji WJ, Lu RY, Zhang Y, Zhang YD, et al. Neutrophil Extracellular Traps in Ischemia-Reperfusion Injury-Induced Myocardial No-Reflow: Therapeutic Potential of DNase-Based Reperfusion Strategy. Am J Physiol Heart Circ Physiol (2015) 308(5):H500–9. doi: 10.1152/ajpheart.00381.2014
96. Sayah DM, Mallavia B, Liu F, Ortiz-Munoz G, Caudrillier A, DerHovanessian A, et al. Neutrophil Extracellular Traps Are Pathogenic in Primary Graft Dysfunction After Lung Transplantation. Am J Respir Crit Care Med (2015) 191(4):455–63. doi: 10.1164/rccm.201406-1086OC
97. Scozzi D, Wang X, Liao F, Liu Z, Zhu J, Pugh K, et al. Neutrophil Extracellular Trap Fragments Stimulate Innate Immune Responses That Prevent Lung Transplant Tolerance. Am J Transplant (2019) 19(4):1011–23. doi: 10.1111/ajt.15163
98. Ng CS, Wan S, Arifi AA, Yim AP. Inflammatory Response To Pulmonary Ischemia-Reperfusion Injury. Surg Today (2006) 36(3):205–14. doi: 10.1007/s00595-005-3124-2
99. Bos S, Vos R, Van Raemdonck DE, Verleden GM. Survival in Adult Lung Transplantation: Where Are We in 2020? Curr Opin Organ Transplant (2020) 25(3):268–73. doi: 10.1097/MOT.0000000000000753
100. Chambers DC, Cherikh WS, Harhay MO, Hayes D Jr., Hsich E, Khush KK, et al. The International Thoracic Organ Transplant Registry of the International Society for Heart and Lung Transplantation: Thirty-Sixth Adult Lung and Heart-Lung Transplantation Report-2019; Focus Theme: Donor and Recipient Size Match. J Heart Lung Transplant (2019) 38(10):1042–55. doi: 10.1016/j.healun.2019.08.001
101. Chaney J, Suzuki Y, Cantu E. 3rd Lung Donor Selection Criteria. J Thorac Dis (2014) 6(8):1032–8. doi: 10.3978/j.issn.2072-1439.2014.03.24
102. Meyer DM, Bennett LE, Novick RJ, Hosenpud JD. Effect of Donor Age and Ischemic Time on Intermediate Survival and Morbidity After Lung Transplantation. Chest (2000) 118(5):1255–62. doi: 10.1378/chest.118.5.1255
103. Mulvihill MS, Gulack BC, Ganapathi AM, Speicher PJ, Englum BR, Hirji SA, et al. The Association of Donor Age and Survival Is Independent of Ischemic Time Following Deceased Donor Lung Transplantation. Clin Transplant (2017) 31(7). doi: 10.1111/ctr.12993
104. Novick RJ, Bennett LE, Meyer DM, Hosenpud JD. Influence of Graft Ischemic Time and Donor Age on Survival After Lung Transplantation. J Heart Lung Transplant (1999) 18(5):425–31. doi: 10.1016/S1053-2498(98)00057-6
105. Boengler K, Schulz R, Heusch G. Loss of Cardioprotection With Ageing. Cardiovasc Res (2009) 83(2):247–61. doi: 10.1093/cvr/cvp033
106. Juhaszova M, Rabuel C, Zorov DB, Lakatta EG, Sollott SJ. Protection in the Aged Heart: Preventing the Heart-Break of Old Age? Cardiovasc Res (2005) 66(2):233–44. doi: 10.1016/j.cardiores.2004.12.020
107. Porter GA, Urciuoli WR, Brookes PS, Nadtochiy SM. SIRT3 Deficiency Exacerbates Ischemia-Reperfusion Injury: Implication for Aged Hearts. Am J Physiol Heart Circ Physiol (2014) 306(12)::H1602–9. doi: 10.1152/ajpheart.00027.2014
108. Dickson KM, Martins PN. Implications of Liver Donor Age on Ischemia Reperfusion Injury and Clinical Outcomes. Transplant Rev (Orlando) (2020) 34(3):100549. doi: 10.1016/j.trre.2020.100549
109. Czigany Z, Lurje I, Schmelzle M, Schoning W, Ollinger R, Raschzok N, et al. Ischemia-Reperfusion Injury in Marginal Liver Grafts and the Role of Hypothermic Machine Perfusion: Molecular Mechanisms and Clinical Implications. J Clin Med (2020) 9(3). doi: 10.3390/jcm9030846
110. Ploeg RJ, D'Alessandro AM, Knechtle SJ, Stegall MD, Pirsch JD, Hoffmann RM, et al. Risk Factors for Primary Dysfunction After Liver Transplantation–A Multivariate Analysis. Transplantation (1993) 55(4):807–13. doi: 10.1097/00007890-199304000-00024
111. Chun SK, Lee S, Flores-Toro J, RY U, MJ Y, KL Go, et al. Loss of Sirtuin 1 and Mitofusin 2 Contributes to Enhanced Ischemia/Reperfusion Injury in Aged Livers. Aging Cell (2018) 17(4):e12761. doi: 10.1111/acel.12761
112. Liu A, Yang J, Hu Q, Dirsch O, Dahmen U, Zhang C, et al. Young Plasma Attenuates Age-Dependent Liver Ischemia Reperfusion Injury. FASEB J (2019) 33(2):3063–73. doi: 10.1096/fj.201801234R
113. Kusaka J, Koga H, Hagiwara S, Hasegawa A, Kudo K, Noguchi T. Age-Dependent Responses to Renal Ischemia-Reperfusion Injury. J Surg Res (2012) 172(1):153–8. doi: 10.1016/j.jss.2010.08.034
114. Xu X, Fan M, He X, Liu J, Qin J, Ye J. Aging Aggravates Long-Term Renal Ischemia-Reperfusion Injury in a Rat Model. J Surg Res (2014) 187(1):289–96. doi: 10.1016/j.jss.2013.10.008
115. Tullius SG, Reutzel-Selke A, Egermann F, Nieminen-Kelha M, Jonas S, Bechstein WO, et al. Contribution of Prolonged Ischemia and Donor Age to Chronic Renal Allograft Dysfunction. J Am Soc Nephrol (2000) 11(7):1317–24. doi: 10.1681/ASN.V1171317
116. Tullius SG, Reutzel-Selke A, Nieminen-Kelha M, Jonas S, Egermann F, Heinzelmann T, et al. Contribution of Donor Age and Ischemic Injury in Chronic Renal Allograft Dysfunction. Transplant Proc (1999) 31(1-2):1298–9. doi: 10.1016/S0041-1345(98)02004-1
117. Tullius SG, Tran H, Guleria I, Malek SK, Tilney NL, Milford E. The Combination of Donor and Recipient Age Is Critical in Determining Host Immunoresponsiveness and Renal Transplant Outcome. Ann Surg (2010) 252(4):662–74. doi: 10.1097/SLA.0b013e3181f65c7d
118. Reutzel-Selke A, Jurisch A, Denecke C, Pascher A, Martins PN, Kessler H, et al. Donor Age Intensifies the Early Immune Response After Transplantation. Kidney Int (2007) 71(7):629–36. doi: 10.1038/sj.ki.5002098
119. Denecke C, Yuan X, Ge X, Kim IK, Bedi D, Boenisch O, et al. Synergistic Effects of Prolonged Warm Ischemia and Donor Age on the Immune Response Following Donation After Cardiac Death Kidney Transplantation. Surgery (2013) 153(2):249–61. doi: 10.1016/j.surg.2012.07.035
120. Scozzi D, Ibrahim M, Liao F, Lin X, Hsiao HM, Hachem R, et al. Mitochondrial Damage-Associated Molecular Patterns Released by Lung Transplants Are Associated With Primary Graft Dysfunction. Am J Transplant (2019) 19(5):1464–77. doi: 10.1111/ajt.15232
121. Coppe JP, Desprez PY, Krtolica A, Campisi J. The Senescence-Associated Secretory Phenotype: The Dark Side of Tumor Suppression. Annu Rev Pathol (2010) 5:99–118. doi: 10.1146/annurev-pathol-121808-102144
122. Kirkland JL, Tchkonia T. Cellular Senescence: A Translational Perspective. EBioMedicine (2017) 21:21–8. doi: 10.1016/j.ebiom.2017.04.013
123. Roesel MJ, Matsunaga T, Tullius SG. Opportunities and Challenges of Targeting an Aging Immune System. Transplantation (2021) 105(12):2515–6. doi: 10.1097/TP.0000000000003930
124. Schneider JL, Rowe JH, Garcia-de-Alba C, Kim CF, Sharpe AH, Haigis MC. The Aging Lung: Physiology, Disease, and Immunity. Cell (2021) 184(8):1990–2019. doi: 10.1016/j.cell.2021.03.005
125. Iske J, Seyda M, Heinbokel T, Maenosono R, Minami K, Nian Y, et al. Senolytics Prevent Mt-DNA-Induced Inflammation and Promote the Survival of Aged Organs Following Transplantation. Nat Commun (2020) 11(1):4289. doi: 10.1038/s41467-020-18039-x
126. Lee JR, Park BW, Park JH, Lim S, Kwon SP, Hwang JW, et al. Local Delivery of a Senolytic Drug in Ischemia and Reperfusion-Injured Heart Attenuates Cardiac Remodeling and Restores Impaired Cardiac Function. Acta Biomater (2021) 135:520–33. doi: 10.1016/j.actbio.2021.08.028
127. Li C, Shen Y, Huang L, Liu C, Wang J. Senolytic Therapy Ameliorates Renal Fibrosis Postacute Kidney Injury by Alleviating Renal Senescence. FASEB J (2021) 35(1):e21229. doi: 10.1096/fj.202001855RR
128. Lim S, Kim TJ, Kim YJ, Kim C, Ko SB, Kim BS. Senolytic Therapy for Cerebral Ischemia-Reperfusion Injury. Int J Mol Sci (2021) 22(21). doi: 10.3390/ijms222111967
129. Justice JN, Nambiar AM, Tchkonia T, LeBrasseur NK, Pascual R, Hashmi SK, et al. Senolytics in Idiopathic Pulmonary Fibrosis: Results From a First-in-Human, Open-Label, Pilot Study. EBioMedicine (2019) 40:554–63. doi: 10.1016/j.ebiom.2018.12.052
130. Xu M, Pirtskhalava T, Farr JN, Weigand BM, Palmer AK, Weivoda MM, et al. Senolytics Improve Physical Function and Increase Lifespan in Old Age. Nat Med (2018) 24(8):1246–56. doi: 10.1038/s41591-018-0092-9
131. Hornby K, Ross H, Keshavjee S, Rao V, Shemie SD. Non-Utilization of Hearts and Lungs After Consent for Donation: A Canadian Multicentre Study. Can J Anaesth (2006) 53(8):831–7. doi: 10.1007/BF03022801
132. Van Raemdonck D, Neyrinck A, Verleden GM, Dupont L, Coosemans W, Decaluwe H, et al. Lung Donor Selection and Management. Proc Am Thorac Soc (2009) 6(1):28–38. doi: 10.1513/pats.200808-098GO
133. Ware LB, Wang Y, Fang X, Warnock M, Sakuma T, Hall TS, et al. Assessment of Lungs Rejected for Transplantation and Implications For Donor Selection. Lancet (2002) 360(9333):619–20. doi: 10.1016/S0140-6736(02)09774-X
134. Valapour M, Lehr CJ, Skeans MA, Smith JM, Uccellini K, Lehman R, et al. OPTN/SRTR 2017 Annual Data Report: Lung. Am J Transplant (2019) 19 Suppl 2:404–84. doi: 10.1111/ajt.15279
135. Cypel M, Yeung JC, Hirayama S, Rubacha M, Fischer S, Anraku M, et al. Technique For Prolonged Normothermic Ex Vivo Lung Perfusion. J Heart Lung Transplant (2008) 27(12):1319–25. doi: 10.1016/j.healun.2008.09.003
136. Chan PG, Kumar A, Subramaniam K, Sanchez PG. Ex Vivo Lung Perfusion: A Review of Research and Clinical Practices. Semin Cardiothorac Vasc Anesth (2020) 24(1):34–44. doi: 10.1177/1089253220905147
137. Chakos A, Ferret P, Muston B, Yan TD, Tian DH. Ex-vivo Lung Perfusion Versus Standard Protocol Lung Transplantation-Mid-Term Survival and Meta-Analysis. Ann Cardiothorac Surg (2020) 9(1):1–9. doi: 10.21037/acs.2020.01.02
138. Iske J, Hinze CA, Salman J, Haverich A, Tullius SG, Ius F. The Potential of Ex vivo Lung Perfusion on Improving Organ Quality and Ameliorating Ischemia Reperfusion Injury. Am J Transplant (2021) 21(12):3831–9. doi: 10.1111/ajt.16784
139. Nakajima D, Date H. Ex vivo Lung Perfusion in Lung Transplantation. Gen Thorac Cardiovasc Surg (2021) 69(4):625–30. doi: 10.1007/s11748-021-01609-1
140. Cypel M, Yeung JC, Liu M, Anraku M, Chen F, Karolak W, et al. Normothermic ex vivo Lung Perfusion In Clinical Lung Transplantation. N Engl J Med (2011) 364(15):1431–40. doi: 10.1056/NEJMoa1014597
141. Warnecke G, Van Raemdonck D, Smith MA, Massard G, Kukreja J, Rea F, et al. Normothermic Ex-Vivo Preservation With the Portable Organ Care System Lung Device for Bilateral Lung Transplantation (INSPIRE): A Randomised, Open-Label, Non-Inferiority, Phase 3 Study. Lancet Respir Med (2018) 6(5):357–67. doi: 10.1016/S2213-2600(18)30136-X
Keywords: aging, ischemia reperfusion injury, primary graft dysfunction, lung transplantation, senolytics
Citation: Roesel MJ, Sharma NS, Schroeter A, Matsunaga T, Xiao Y, Zhou H and Tullius SG (2022) Primary Graft Dysfunction: The Role of Aging in Lung Ischemia-Reperfusion Injury. Front. Immunol. 13:891564. doi: 10.3389/fimmu.2022.891564
Received: 07 March 2022; Accepted: 21 April 2022;
Published: 24 May 2022.
Edited by:
Jordi Ochando, Icahn School of Medicine at Mount Sinai, United StatesReviewed by:
Emilia Lecuona, Northwestern University, United StatesCopyright © 2022 Roesel, Sharma, Schroeter, Matsunaga, Xiao, Zhou and Tullius. This is an open-access article distributed under the terms of the Creative Commons Attribution License (CC BY). The use, distribution or reproduction in other forums is permitted, provided the original author(s) and the copyright owner(s) are credited and that the original publication in this journal is cited, in accordance with accepted academic practice. No use, distribution or reproduction is permitted which does not comply with these terms.
*Correspondence: Stefan G. Tullius, stullius@bwh.harvard.edu