- 1Department of Microbiology, Aggeu Magalhães Institute, Fiocruz Pernambuco, Recife, Brazil
- 2Research Support Center, Federal Rural University of Pernambuco, Recife, Brazil
- 3Institute of Biological Sciences, University of Pernambuco, Recife, Brazil
- 4Central Public Health Laboratory of Pernambuco, Endemics Laboratory, Recife, Brazil
- 5Department of Immunology, Aggeu Magalhães Institute, Fiocruz Pernambuco, Recife, Brazil
New therapeutic strategies for visceral leishmaniasis (VL) have been studied, and the development of an immunotherapeutic agent that modulates the host’s immune response is necessary. The aim of this study was to evaluate in vitro the bioactive extracts of photosynthetic microorganisms (PMs) for their leishmanicidal/leishmanistatic and immunomodulatory potentials. Bioactive extracts from PMs (Arthrospira platensis and Dunaliella tertiolecta) were obtained by sonication. Reference drugs, miltefosine (MTF) and N-methylglucamine antimoniate (SbV), were also evaluated. The selectivity index (SI) of treatments was determined by assays of inhibitory concentration (IC50) in Leishmania infantum cells and cytotoxic concentrations (CC50) in human peripheral blood mononuclear cells by the MTT method. The immune response was evaluated in healthy human cells by the production of cytokines and nitric oxide (NO) and the gene expression of Tbx21, GATA3, RORc, and FOXP3, using four concentrations (CC50, ½ CC50, ¼ CC50, and IC50) for in-vitro stimulation. Based on the data obtained, we observed that the extracts of D. tertiolecta (SI = 4.7) and A. platensis (SI = 3.8) presented better results when compared to SbV (SI = 2.1). When analyzing the immune response results, we identified that the extracts of PMs stimulated the production of cytokines of the Th1 profile more than the reference drugs. The extracts also demonstrated the ability to stimulate NO synthesis. Regarding gene expression, in all concentrations of A. platensis extracts, we found a balance between the Th1/Th2 profile, with the average expression of the Tbx21 gene more than the GATA3 in the highest concentration (CC50). Regarding the extract of D. tertiolecta, we can observe that, in the lowest concentrations, a balance between all the genes was present, with the average expression of the GATA3 gene being lower than the others. The best result was found in the ½ CC50 concentration, stimulating a balanced positive expression between the Th1×Th17×Treg profiles, with a negative expression of GATA3. Thus, PM extracts showed promising results, presenting low toxicity, leishmanicidal/leishmanistatic activity, and induction of the immune response, which could be potential therapeutic candidates for VL.
Introduction
The lack of therapeutic alternatives is one of the greatest obstacles to control visceral leishmaniasis (VL). In the treatment of VL, the drug of first choice for humans is N-methylglucamine antimoniate (Glucantime®, Sanofi-Aventis, France), and for dogs, it is miltefosine (Milteforan™, Virbac, France). However, these drugs are highly toxic to the host and have been presenting treatment failures and reports of parasite resistance (1).
The host’s immune response against the parasite must change, at least in part, to achieve therapeutic success against VL; in this context, the use of immunomodulatory drugs that can reverse the host’s immune profile, stimulating a profile of resistance against the parasite, is important to complement conventional treatment, helping in the patient’s clinical improvement (2). To investigate the protective immune response stimulated by a therapeutic candidate, understanding the immunological mechanisms in leishmaniasis is essential, as these parasites have developed, throughout evolution, several evasion mechanisms to survive the host’s immune system (3).
The host’s cellular immune response is mediated by CD4+ T lymphocytes which, in a simplified way, can present two types of profiles: resistance (Th1) and susceptibility (Th2). The Th1 profile produces cytokines such as interferon-gamma (IFN-γ), tumor necrosis factor (TNF), and interleukin 2 (IL-2), which are associated with the control of infection by macrophages, the production of nitric oxide (NO), and the consequent destruction of intracellular parasites. On the other hand, the Th2 profile is characterized by the synthesis of cytokines such as interleukin 4 (IL-4), interleukin 10 (IL-10), and tumor growth factor-beta (TGF-β), favoring the onset of the disease and its symptoms, with inhibition of synthesized NO by activated macrophages, providing the survival of the parasites (4–7).
Other cell subtypes such as regulatory T cells (Treg) and Th17 may also play a significant role in susceptibility and resistance against leishmaniasis (8, 9). However, despite the Th1/Th2 (resistance/susceptibility) paradigm being a simplified model, several studies have demonstrated its foundation (10–12).
Based on this knowledge about the immunological profile of VL, studies that aim to investigate new molecules with potential against visceral leishmaniasis can be performed. Microorganisms, such as photosynthetic microorganisms, are promising candidates for therapeutic investigations since they have a variety of metabolites with different biological activities that have not been fully studied yet (13–15). Studies with Chlorophyta extracts have demonstrated anti-inflammatory and antiparasitic activities (16, 17), in addition to an immunomodulatory potential (18). Thus, with a broader vision for the control of leishmaniasis, this study aimed to evaluate the in-vitro therapeutic potential for VL of the bioactive extracts of two species of photosynthetic microorganisms, Arthrospira platensis and Dunaliella tertiolecta, in human cells.
Material and Methods
Obtaining Bioactive Extracts of Photosynthetic Microorganisms
Two photosynthetic microorganisms (PMs), the cyanobacterium A. platensis (Utex, 1926) and the microalgae D. tertiolecta (Utex, 1644), were obtained from the University of Texas culture collection. Arthrospira platensis was cultivated in a 250-ml Erlenmeyer flask containing 100 ml of Schlösser medium (19) in an orbital shaker at 75 ± 5 rpm, while D. tertiolecta was cultivated in a 1-L Erlenmeyer flask containing 400 ml of f/2 medium (20), under constant aeration. Both cultivations used an initial biomass concentration of 50 mg/L, temperature of 27°C ± 1°C, and continuous light intensity of 52 ± 4 μmol photons m−2 s−1 (21).
After reaching the stationary growth phase, the cultures were centrifuged at 430×g for 10 min (Hermle Labortechnik 326 HK, Wehingen, Germany), lyophilized (SP Scientific BenchTop Pro, Warminster, USA), resuspended (100 mg ml−1) in 0.1 M of Tris–HCl buffer pH 7.0, and sonicated at 20 pulses in an ice bath with intervals of 1 min between each pulse (22). The homogenate was centrifuged at 10,000×g for 5 min at 4°C, and the supernatant filtered at 0.22 µm was denominated bioactive extract and used for further analysis. Protein concentration was determined by the method of Smith et al. (23) using the Micro BCA Protein Assay Kit (Pierce).
Cultivation of Leishmania infantum
The promastigotes of L. infantum (strain MHOM/BR/1972/BH46) were culture-expanded in Schneider medium (Sigma-Aldrich) pH 7.2, containing 10% of fetal bovine serum (FBS—Vitrocell®, Brazil) and 1% of antibiotic (penicillin 200 U/ml). The peaks were performed between the third and fourth day of cultivation and incubated in a biochemical oxygen demand greenhouse (BOD) at a temperature of ±26°C, to be used in the leishmanicidal effect tests.
To obtain the antigenic fractions, the parasitic mass was subjected to rapid freezing (−197°C) in liquid nitrogen, followed by thawing (40°C). Soon after, the cell suspension was subjected to the ultrasonication process (60 s/40 W), three times, and centrifuged at 10,000×g for 20 min at 4°C. The resulting supernatant was a soluble antigen fraction (L. infantum soluble antigen: LSA). Proteins were quantified by the Bradford method (24), and the fraction was stored at −80°C. LSA was used in the cell culture assays.
Sample Collection and Peripheral Blood Mononuclear Cell Isolation
Whole blood was collected from healthy human individuals by venipuncture using heparin tubes (18 ml) to perform the separation of peripheral blood mononuclear cells (PBMCs). Phosphate-buffered saline (PBS) (pH 7.2) was added to heparinized blood in a proportion of 1:2. This mixture was added to Ficoll-Hypaque at a ratio of 1:3 (Ficoll-Hypaque:blood) to obtain the ring of PBMCs after centrifugation at 900×g for 30 min without brake. Cells were washed twice in PBS and then resuspended in 1 ml of RPMI 1640 medium supplemented with 10% of FBS and 1% of penicillin/streptomycin (200 U/ml). Cells were counted in a Neubauer chamber using Trypan Blue dye. The cell value obtained was adjusted to a concentration of 1 × 106 cells/ml by adding RPMI 1640 medium supplemented with 10% of FBS, to be used in the assays.
Determination of the Selectivity Index
The selectivity index (SI) was calculated as the ratio between the 50% cytotoxic concentration (CC50) in human cells and the 50% inhibitory concentration (IC50) in parasite cultures. All cells were treated with bioactive extracts of photosynthetic microorganisms and reference drugs (N-methylglucamine antimoniate and miltefosine).
PBMCs from healthy humans were cultivated in 96-well plates and treated with different stimuli to evaluate the CC50. Cell treatment (containing 1 × 106 cells/ml) was performed in triplicate for each stimulus, incubated for 24 h, using concentrations of 62.5 to 1,000 µg/ml of bioactive extracts of PMs (25, 26). After the culture time, the supernatant was discarded and MTT [3-(4,5-dimethylthiazol-2-yl)-2,5-diphenyltetrazolium bromide] was added at a concentration of 1 mg/ml, solubilized in RPMI 1640 medium without phenol red, and incubated in an oven at 37°C for 3 h. Subsequently, the formazan crystals formed were solubilized with dimethylsulfoxide (DMSO) and then read in a spectrophotometer at 540 nm (27).
Cells of L. infantum in the promastigote form were cultivated for the evaluation of the IC50 of the PM bioactive extracts. Cell treatment was performed in triplicate for each stimulus, incubated for 48 h in a 96-well plate containing 1 × 106 cells/ml (28). After the culture time, the cells were counted and evaluated for their morphology under a light microscope. For natural extracts, protein concentrations of 15.63 to 500 µg/ml were used (29). For N-methylglucamine antimoniate and miltefosine, the concentration curves were 15.63 to 250 µg/ml and 0.1 to 4 µg/ml, respectively (30, 31). Three independent experiments were carried out in triplicates.
Immune Response Analysis
Whole blood was collected from healthy humans to obtain the PBMCs. PBMC cultures were performed in 48-well polystyrene plates, containing 5 × 105 cells/well, and stimulated with the mitogen phytohemagglutinin (PHA) (10 µg/ml) (positive control of the culture), the soluble antigen of L. infantum (LSA) (25 µg/ml) (32), N-methylglucamine antimoniate, miltefosine, and natural extracts. The concentration values—CC50, ½ CC50, ¼ CC50, and IC50—of the reference drugs and natural extracts were determined. Unstimulated wells served as a negative control.
Evaluation of Cytokine Production
From the supernatants of human PBMC cultures, cytokine secretion (IFN-γ, TNF, IL-2, IL-4, IL-6, IL-10) was measured using the BD™ CBA Human Th1/Th2 Cytokine kit (Becton Dickinson, United States). The reading was performed in the FACSCalibur flow cytometry equipment (Becton Dickinson, United States), according to the manufacturer’s guidelines. The results were analyzed using the FCAP Array 3.0 software (Becton Dickinson, United States), being normalized with the results obtained by non-stimulated cells. So, if the value is presented as negative, it means that there is an inhibition of cytokine production since the detected value is lower than that presented by the non-stimulated PBMCs.
Evaluation of Nitric Oxide Production
Quantification of NO levels was indirectly performed by measuring nitrite in culture supernatants, by the Griess reaction, according to Resende et al. (7). The stimuli (LSA, N-methylglucamine antimoniate, miltefosine, and natural extracts) were compared with the negative control (PBMCs without stimulus).
Evaluation of mRNA Expression
The evaluation of the relative expression of messenger RNA (mRNA) of specific transcription factors (Tbx21, GATA3, RORc, and FOXP3) from the culture of healthy human PBMCs was performed. After the cultivation time, RNA extraction was performed with the TRIzol® (Invitrogen, Thermo Fisher Scientific, United States) method, measuring the mRNA concentration in a spectrophotometer and storing it at −80°C.
After obtaining the mRNA, reverse transcription to complementary DNA (cDNA) was performed using the commercial TaqMan Reverse Transcription reagent kit (Applied Biosystems, Life Technology, United States). The cycling conditions followed the manufacturer’s guidelines (25°C for 10 min, 37°C for 30 min, 95°C for 5 min, 4°C until removal).
A real-time qPCR was performed from the cDNA (TaqMan® System, Applied Biosystems, Life Technology, United States) using the QuantStudio 5 sequence detection system and the TaqMan® Gene Expression PCR Master Mix kit (Applied Biosystems, Life Technology, United States) for the main regulatory genes T-bet/Tbx21 (ID: Hs00894392_m1), GATA3 (ID: Hs00231122_m1), RORc (ID: Hs01076112_m1), and FOXP3 (ID: Hs01085834_m1). As an endogenous control of the reaction, GAPDH was used (ID: Hs02786624_g1). All samples were analyzed in duplicate and expressed as the mean ± standard error of the mean, using the PHA mitogen as a calibrator to calculate the relative quantification value.
Data Analysis
Probit regression analyses were performed using the IBM SPSS Statistic 25 software program in order to determine the values of CC50 and IC50. Also, comparative analyses were performed using descriptive statistics techniques with absolute and percentage distributions. The Kolmogorov–Smirnov normality test was used, and according to the analysis, Student’s t-test (parametric) or the Mann–Whitney test (non-parametric) was performed for comparisons between two categories, or one-way analysis of variance (ANOVA) with Tukey post-test (parametric) or Kruskal–Wallis with Dunn’s post-test (non-parametric) was performed for comparisons between more than two categories. Correlation analyses were also performed using Pearson’s test (parametric) and Spearman’s test (non-parametric). All tests were performed using the GraphPad Prism 5 program (GraphPad Software Inc. 2007, San Diego, CA, USA), and a p-value <0.05 was considered significant.
Ethical Considerations
Before the collection, human individuals included in the study were invited to participate and sign an informed consent form, authorizing the use of the collected material for scientific purposes. This study is part of the project “Immunology applied to the development of new control strategies for leishmaniasis” which has the approval of the Research Ethics Committee: CAAE 89972718.8.0000.5190 No. 4,077,060 (Fiocruz/PE).
Results
Determination of the Selectivity Index
Assays for the determination of CC50 and IC50 were performed, and it was observed that the extract of A. platensis had the lowest toxicity for human PBMCs, presenting a CC50 of approximately 1,000 µg/ml. In turn, D. tertiolecta presented higher toxicity to L. infantum, with a lower IC50.
By analyzing the reference drugs SbV and MTF, we observed that MTF was more toxic to L. infantum cells as well as to the host cells. Despite the high toxicity, the MTF was over 100 times more selective than other treatments (SI = 129.6). On the other hand, SbV had the lowest SI (2.11), demonstrating that the extracts of A. platensis and D. tertiolecta were more selective for the parasite (SI = 3.8 and 4.7, respectively) than this reference drug, which is currently used for the treatment of VL in Brazil. Table 1 shows the CC50 and IC50 values as well as the SI.

Table 1 Leishmanicidal activity (IC50) in promastigotes and cytotoxic effect (CC50) in human cells treated with bioactive extracts of photosynthetic microorganisms and reference drugs.
Cytokine Quantification
For the extracts, we observed that both A. platensis and D. tertiolecta induced the production of cytokines of the Th1 profile by PBMCs, at all concentrations evaluated, demonstrating a positive stimulus against VL (Figure 1). We emphasized IL-10, which gradually decreased its production as the concentration of the extracts increased, with statistically significant differences between the concentrations of the A. platensis (p-value = 0.031) and D. tertiolecta (p-value = 0.007) extracts.
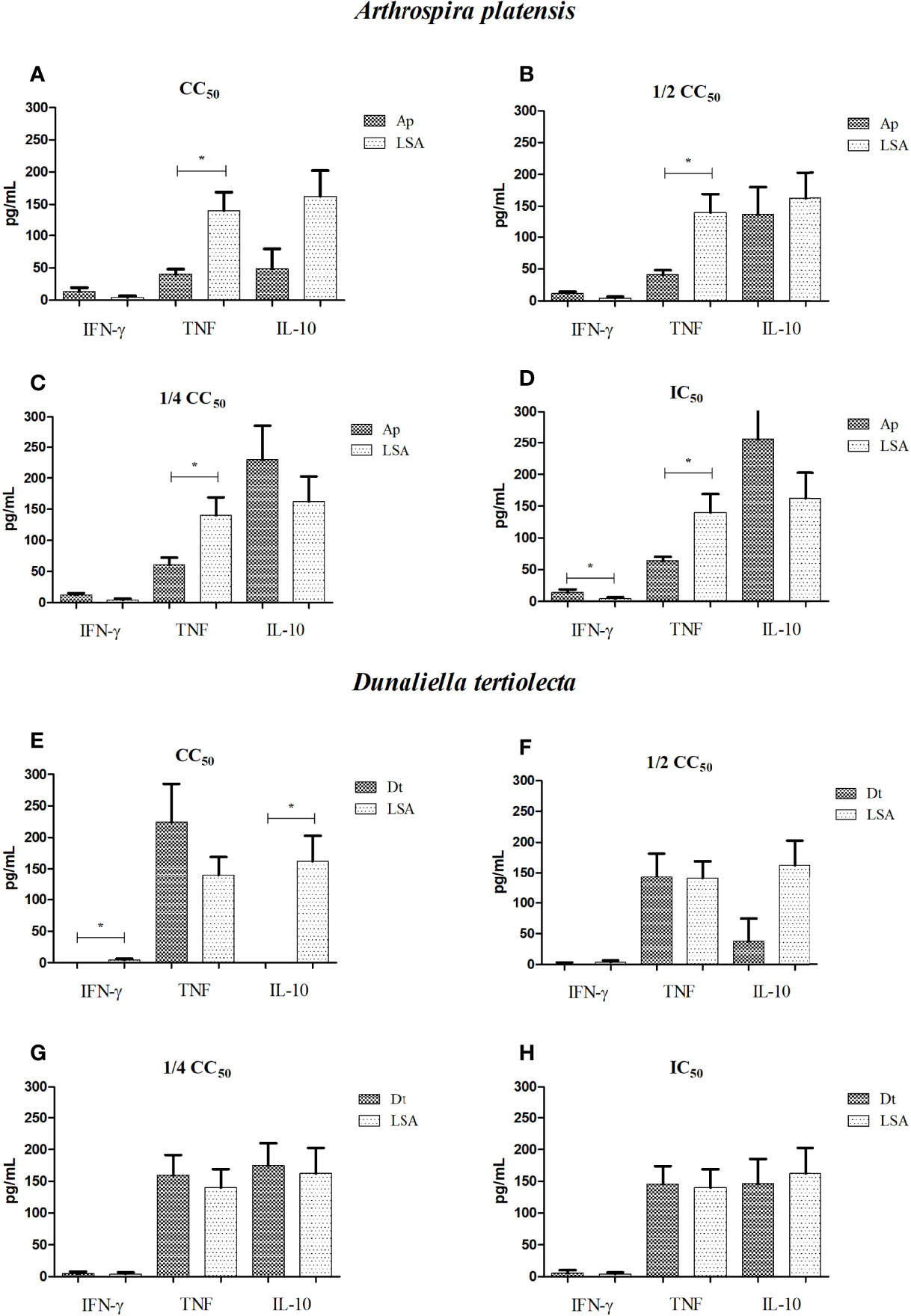
Figure 1 Dosage of cytokines produced by cells stimulated with bioactive extracts of photosynthetic microorganisms. (A, E) CC50 value; (B, F) half of the CC50 value; (C, G) one quarter of the CC50 value; (D, H) IC50 value. LSA, Leishmania infantum soluble antigen; Ap, Arthrospira platensis; Dt, Dunaliella tertiolecta. *Statistical difference = p-value < 0.05. Limit of detection (IFN-γ: 7.1; TNF: 2.8; IL-10: 2.8). The cytokine produced for each treatment was subtracted by the cytokine produced from non-stimulated cells.
Comparing the results obtained from the extract of A. platensis with LSA, we observed the presence of a Th1-protective response for all concentrations, with IFN-γ stimulation higher than that observed with the natural infection mimicked by LSA, with a statistically significant difference for the IC50 concentration (p-value = 0.032) (Figure 1D). For all concentrations (Figures 1A–D), the A. platensis extract did not stimulate TNF production that was superior to LSA, with statistically significant differences, but it stimulated the production of this cytokine more than the cells without stimulation. Regarding the extract of D. tertiolecta, compared to LSA, we detected that, for all concentrations, a Th1-protective response was found, with the mean of TNF stimulation higher than that observed with LSA (Figures 1E–H). Furthermore, at the concentration of CC50, a lower stimulus for IL-10 production (p-value = 0.002) and for IFN-γ (p-value = 0.008) was found than LSA (Figure 1E). Table 2 shows the mean values of cytokine production (in pg/ml) after cell stimulation with the four concentrations of each extract.
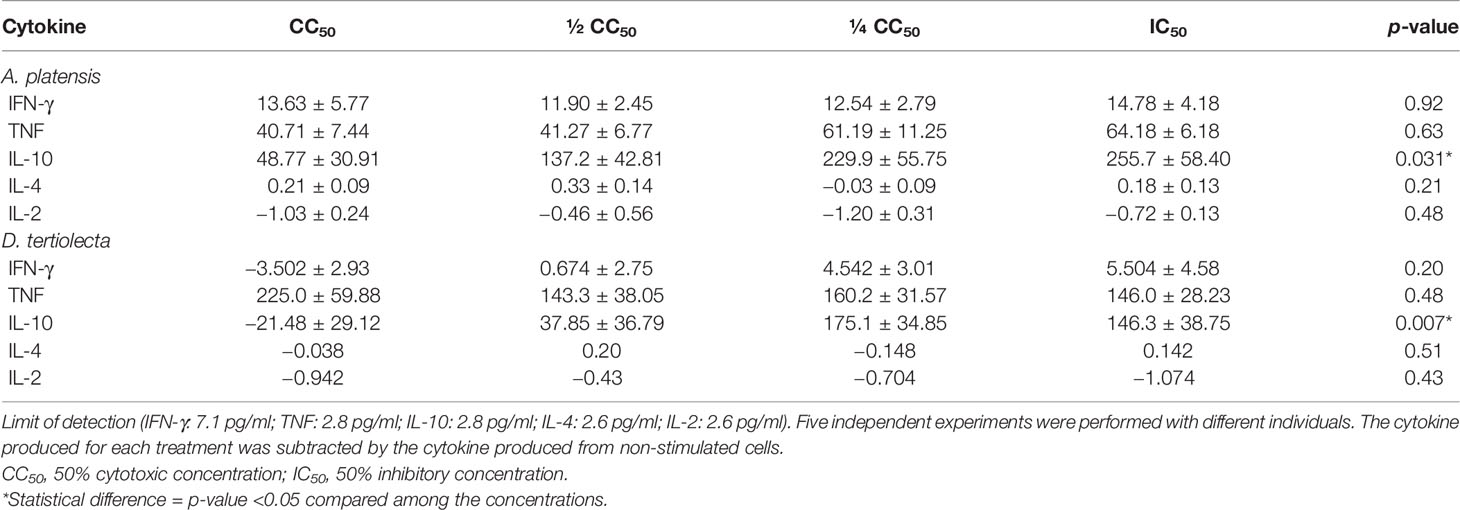
Table 2 Mean (± SEM) concentrations (in pg/ml) of cytokines produced by cells stimulated with the extracts of Arthrospira platensis and Dunaliella tertiolecta.
When analyzing the correlation of cytokines and the extracts, we observed that between TNF and IL-4, in the CC50 and ½ CC50 concentrations of the A. platensis extract, there were a very strong and significant negative correlation (r = −0.92; p-value = 0.02) and a significant positive correlation (r = −0.97; p-value = 0.01), respectively. For the extract of D. tertiolecta, we observed very strong but not significant correlations. Supplementary Table 1 shows the correlation data of cytokines induced by the extracts.
Regarding the reference drugs, all concentrations of SbV, except IC50, showed an immunological suppression, with only IL-10 being stimulated. As for MTF, all concentrations, except IC50, showed immunological suppression, with stimulation of only TNF at the ¼ CC50 concentration. Table 3 shows the mean values of cytokine production stimulated by the concentrations of each drug. Regarding the correlation between cytokines, for SbV, there were very strong but not significant correlations. For MTF, we observed very strong and significant negative correlations between IL-4 and IL-2 in the CC50 concentration (r = 0.90; p-value = 0.03) and between TNF and IL-10 in the IC50 concentration (r = 0.91; p-value = 0.01). Supplementary Table 2 shows the correlation data of cytokines induced by the reference drugs.
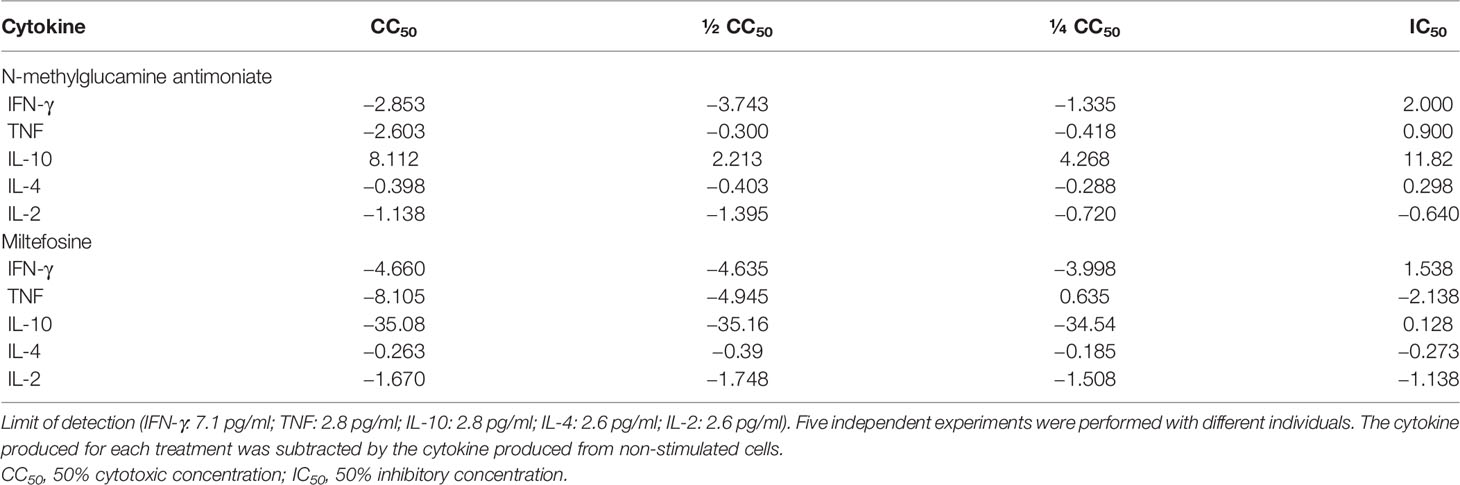
Table 3 Means of concentrations (in pg/ml) of cytokines produced by cells stimulated with the reference drugs.
Quantification of Nitric Oxide
For the extracts, we observed a slightly higher NO production when compared to cells without stimulus and stimulated with LSA (which demonstrated a reduction in NO production, when compared to cells without stimulus). We also identified that the nitrite dosage was directly proportional to the stimulus concentration (Figure 2). Statistically significant differences were observed between the highest concentration (CC50) of each extract compared with the LSA (A. platensis: p-value = 0.017; D. tertiolecta: p-value = 0.005).
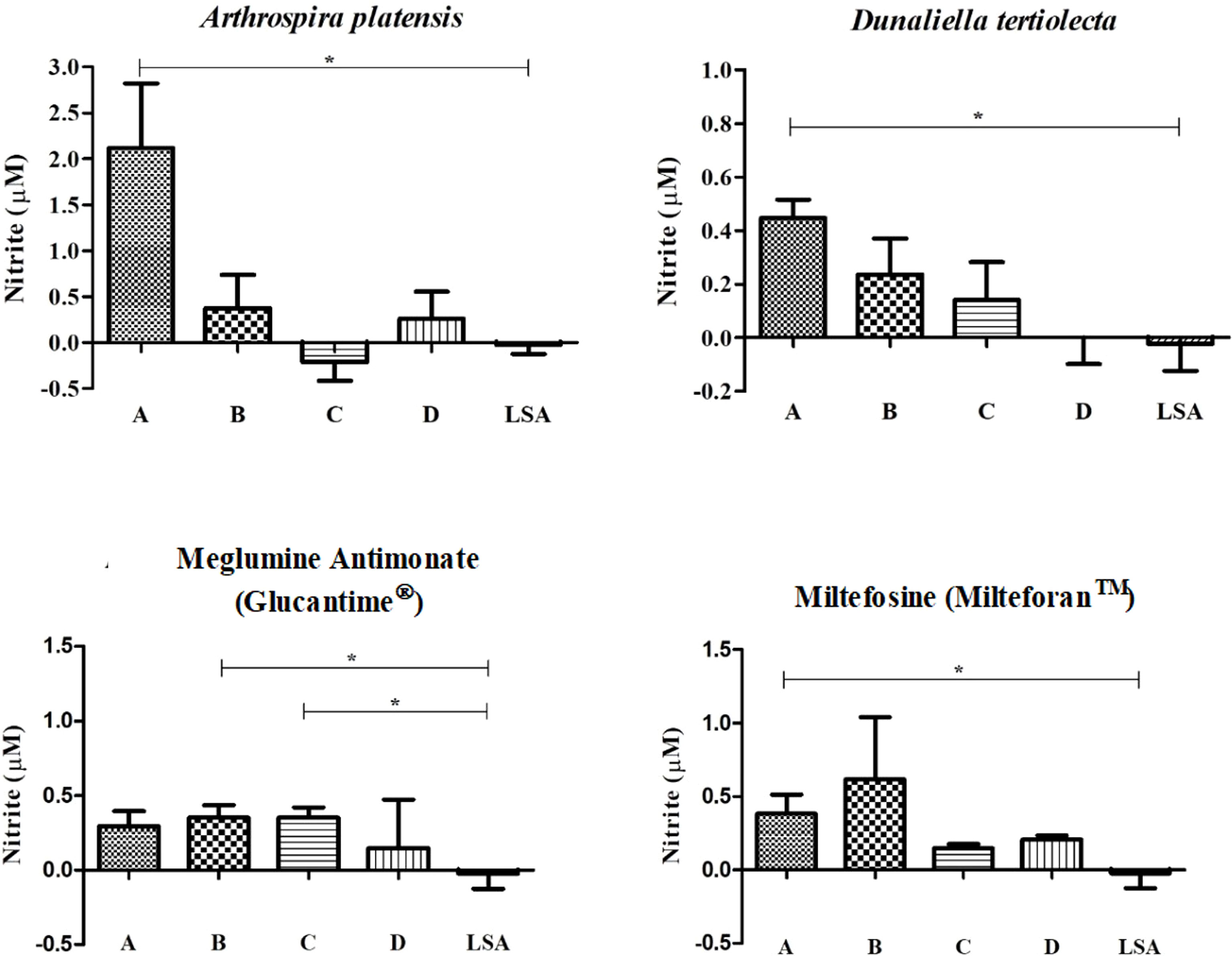
Figure 2 Measurement of nitrite after cell stimulation with bioactive extracts of photosynthetic microorganisms. LSA, soluble antigen of Leishmania infantum (25 µg/ml). *Statistical difference = p-value < 0.05. Limit of detection (0.055). The nitrite measured for each treatment was subtracted by the nitrite measured from non-stimulated cells.
In turn, the reference drugs also obtained a measurement of nitrite that was a little higher than the cells without stimulation and stimulated with LSA, but no proportionality between dosage and concentration was detected. For SbV, a statistically significant difference was found between the concentrations of ½ CC50 (p-value = 0.028) and ¼ CC50 (p-value = 0.023) with the LSA, pointing out a higher NO induction of these concentrations compared to the antigen. Moreover, for MTF, a statistically significant difference was also found between the CC50 and LSA (p-value = 0.041).
Gene Expression
From the stimulated cells, messenger RNA was extracted and reverse-transcribed to cDNA for quantification, targeting the genes Tbx21, GATA3, RORc, and FOXP3, which are related to the induction of T-cell differentiation for the Th1, Th2, Th17, and Treg profiles, respectively.
When analyzing the results of gene expression stimulated with natural extracts (Figure 3), we observed that in all concentrations of A. platensis extract, a balance between the Th1/Th2 profile was present, with the average expression of the Tbx21 gene being higher than that of GATA3 at the highest stimulus concentration, with no statistical difference observed, similar to the results of gene expression stimulated with LSA. Notably, the FOXP3 gene at the highest concentration showed a negative expression (Figure 3A), and the RORc gene varied from concentration to concentration, showing a greater expression at the ¼ CC50 concentration (Figure 3C; Table 4).
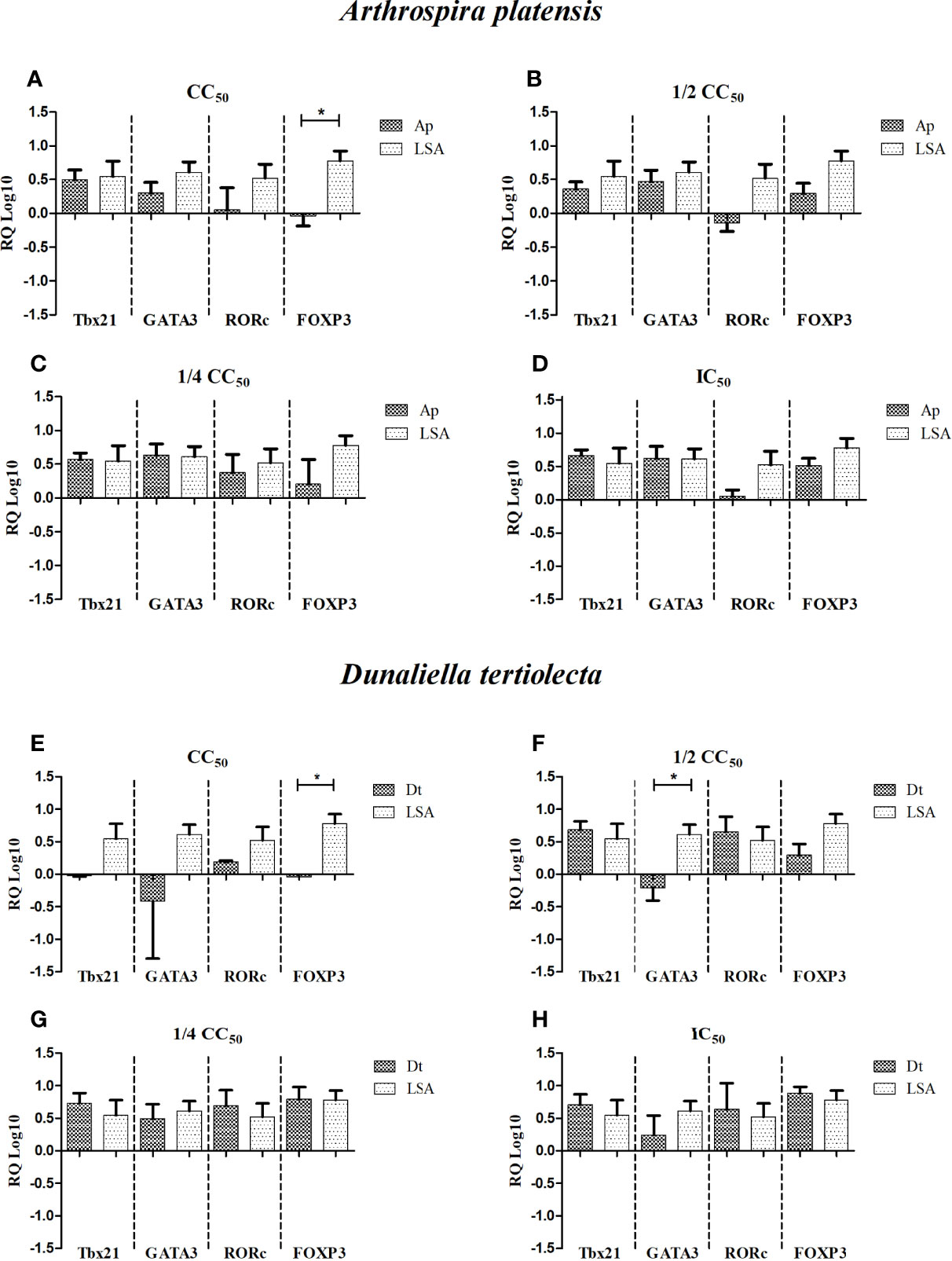
Figure 3 Relative quantification (RQ Log10) of gene expression of specific transcriptional factors after cell stimulation with bioactive extracts of photosynthetic microorganisms. (A, E) CC50 value; (B, F) half of the CC50 value; (C, G) one quarter of the CC50 value; (D, H) IC50 value. LSA, Leishmania infantum soluble antigen (25 µg/ml); Ap, Arthrospira platensis; Dt, Dunaliella tertiolecta. The calibrator used was phytohemagglutinin (PHA). *Statistical difference = p-value < 0.05.
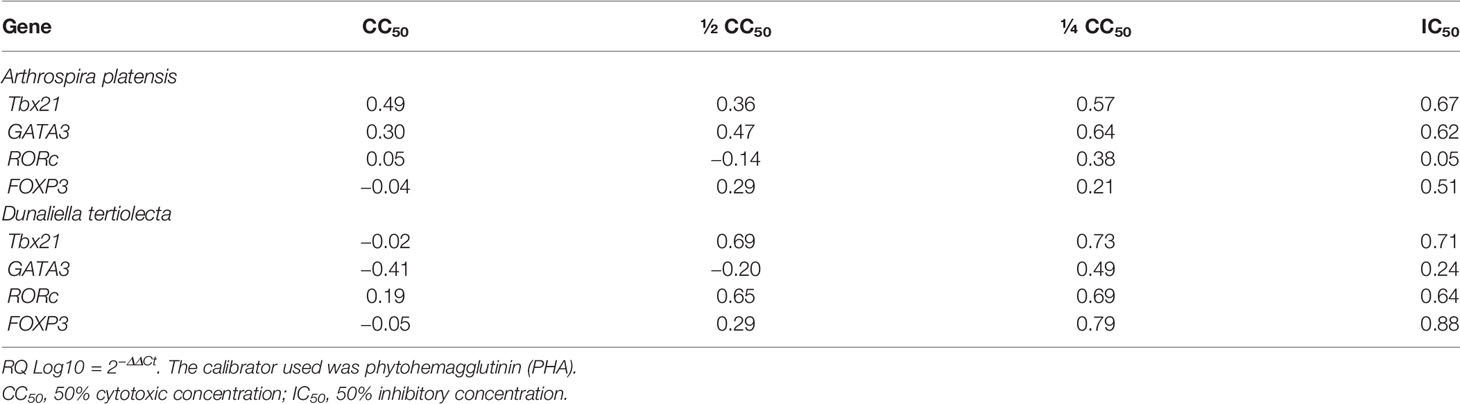
Table 4 Relative quantification (RQ Log10) of gene expression of specific transcriptional factors after cell stimulation with bioactive extracts of photosynthetic microorganisms.
Regarding the extract of D. tertiolecta, we observed that at the lowest concentrations (Figures 3G, H), there was a balance between all the inducing genes, with the average expression of the GATA3 gene being lower than the others. As shown by the results, the ½ CC50 concentration (Figure 3F) stimulated a balanced and positive expression between the Th1×Th17×Treg profiles, with a negative expression of GATA3, inducing the Th2 profile, and a statistically significant difference was observed when compared to LSA (p-value = 0.009). At the highest concentration, there was only a positive expression of the RORc gene (Figure 3E). Analyzing the correlation between genes, for A. platensis, a very strong positive correlation was observed between Tbx21 and RORc at the ½ CC50 concentration (r = 0.95; p-value = 0.047). Regarding the correlation analysis of the extract of D. tertiolecta, at the concentrations tested, no significant correlations were detected. Supplementary Table 3 shows the correlation data of gene expression induced by the extracts.
Discussion
When searching for new therapeutic strategies, low toxicity to host cells is one of the important factors to be considered in identifying a potent therapeutic candidate (33). In this study, A. platensis extract demonstrated a higher CC50 value than the active principles of the drugs currently used for the treatment of VL and CVL (Glucantime® and Milteforan™, respectively).
As alternative candidates for the development of therapeutics, photosynthetic marine organisms have been studied for their anti-Leishmania capacity; however, studies evaluating phytoplankton for their effect against Leishmania sp. are very scarce, being only reported by Pereira et al. (17), which evaluated the leishmanicidal activity of methanol extracts of four species of Chlorophyta, from three different genera (Nannochloris, Picochlorum, and Desmochloris). Therefore, further studies on the leishmanicidal effects of photosynthetic marine microorganisms are necessary, demonstrating the significance of this study. Thus, when comparing our results with those found by Pereira et al. (17), assessing the extracts of Chlorophyta species, we noted that the extracts of D. tertiolecta presented a lower IC50 value, that is, a greater leishmanicidal/leishmanistatic activity, since the concentration of Nannochloris sp. (250 µg/ml) that was used reduced the viability of L. infantum by 38%.
When observing the leishmanicidal/leishmanistatic activity of the natural extracts in this study, we noted that the IC50 values were lower when compared to the values of some organic plant extracts evaluated by Bouyahya et al. (34), with IC50 values ranging between 82.39 and 500 µg/ml, which were more toxic for L. infantum. Making a new comparison, this time with meroditerpenoid isolates from a brown macroalgae (Cystoseira baccata) evaluated by Sousa et al. (35), we observed in our study that the SI of the extract of D. tertiolecta was higher, since the SI obtained by the Sousa et al. was less than 10, using murine peritoneal macrophages, demonstrating that the extracts studied here may have promising potential.
Although the extracts from both A. platensis and D. tertiolecta studied here do not present an SI greater than 10 in assays with human cells, we do not exclude the immunomodulatory potential of these extracts. As observed in the study by Costa-Silva et al. (36), isolates from natural products, despite presenting an SI less than 10, had immunomodulatory activity against L. infantum, reducing the production of Th2 profile cytokines, in addition to stimulating chemokines associated with a decrease in the parasite load.
When evaluating the immune response induced by bioactive extracts of photosynthetic microorganisms of the species A. platensis and D. tertiolecta, a positive immune stimulus of the cells was observed. Different concentrations were studied, but all maintained the same general pattern of the immune response, a balance between pro-inflammatory and anti-inflammatory cytokines, with some concentrations having a slightly greater emphasis on the Th1 profile cytokines.
Arthrospira platensis is a cyanobacterium with some biological activities that have been known for some years by the scientific community. The immunomodulatory potential of this microorganism has been indicated in the literature, stimulating the production of IFN-γ and TNF and reducing the production of IL-10, both in mice (37) and in human PBMCs (38). In addition to the extract, phycocyanin (photosynthetic pigment), a protein isolated from A. platensis, studied by Sheikh et al. (39) when they performed PBMC treatment, also demonstrated an increase in IFN-γ. These findings corroborate our results, since we observed a greater production of IFN-γ and TNF compared to cells without stimulus, with special emphasis on IFN-γ that showed a greater synthesis compared to LSA, with a statistical difference in the lowest concentration.
In addition to stimulating the production of Th1 cytokines, the A. platensis extract also induced the synthesis of the leishmanicidal factor, strengthening the hypothesis that this extract is a good candidate for treating VL. The expression of specific transcriptional factor genes showed a balance, but with no statistically significant differences, strengthening the hypothesis that this extract stimulates the modulation of the immune response by expressing the Tbx21 gene, responsible for activating the differentiation of Th1 lymphocytes.
Although D. tertiolecta is a halophilic microalgae widely used in food and feed supplementation, there are few studies reporting the immunological activity of this species. Based on these few studies about immune response, the immunological activity of this species was observed in vitro in PBMCs from sheep, making it possible to identify the ability of phytosterols from this species to stimulate an immune response. Among these phytosterols, 7-dehydroporiferasterol and ergosterol induced the production of the cytokine IL-10 and the reduction of IL-6 and TNF, demonstrating an anti-inflammatory role (40). Likewise, in the study by Caroprese et al. (41), an increase in IL-10 synthesis was observed, with a reduction in pro-inflammatory cytokines. In addition, a dose-dependent IL-10 was identified, which was also found in our study; however, in our results, we observed that with increasing concentration, IL-10 decreased.
In our study, the extract of D. tertiolecta presented a high stimulation of TNF, surpassing LSA, and a low synthesis of IL-10, lower than LSA, mainly at the highest concentrations tested. The diverging results presented in this study from those found in the literature may be due to the difference in the molecules: the extracts investigated in our study were protein-based, whereas those in the studies by Caroprese et al. (40) and Ciliberti et al. (41) were from phytosterol isolates.
The cytokines produced by the Th1 profile were directly related to the production of the leishmanicidal factor, which was found in our study, stimulating the synthesis of IFN-γ and TNF, in addition to the production of NO. These results also corroborated the data found in the analysis of gene expression of transcriptional factors, which showed the Tbx21 gene to be a little more expressed than GATA3 or FOXP3, depending on the concentration used.
The strong correlations (positive or negative) found by the stimulation with the extracts, both for cytokines and transcription factor genes, are interesting. Although not significant, a strong negative correlation found between TNF and IL-10 for the extract of D. tertiolecta stimulus was observed in the graphs and corroborated with the hypothesis that IL-10 production is dose-dependent. For A. platensis, we also observed very strong negative correlations, although not significant, between TNF and Th2 profile cytokines, suggesting an immunomodulatory potential. The very strong positive correlation between the TNF and IL-4 cytokines is also noteworthy, since the increase of a Th1 profile cytokine together with anti-inflammatory cytokines can combat the parasite, as the balance between both profiles (but predominantly Th1) is important to achieve disease control (42).
Regarding the correlation between genes stimulated by the A. platensis extract, the significant positive correlation found between Tbx21 and RORc, transcriptional factors responsible for inducing Th1 and Th17 profiles, respectively, demonstrated the potential to stimulate pro-inflammatory cytokines, a significant aspect of the immune response against the VL parasite.
Finally, when comparing the results of the extracts with those of the reference drugs, we observed that MTF had a selectivity of more than 100 times for the parasite than for the cells of human and canine hosts, showing greater selectivity among all stimuli. On the other hand, SbV had the lowest SI value, demonstrating that all therapeutic candidates were more selective than SbV. Furthermore, an immunological susceptibility profile was observed, unlike the extracts, demonstrating the induction of a protective profile.
In conclusion, these data suggest and demonstrate that the extracts of PMs are promising therapeutic candidates to treat visceral leishmaniasis, with a protective response elicited in the human cells. These extracts will be further evaluated in relation to their ability i) to stimulate parasitological cure in in-vivo assays (preclinical trials), ii) to induce an immune response, and iii) to reduce the parasite load, and their action on amastigotes will also be analyzed.
Data Availability Statement
The original contributions presented in the study are included in the article/Supplementary Material. Further inquiries can be directed to the corresponding author.
Ethics Statement
The studies involving human participants were reviewed and approved by Aggeu Magalhães Institute - Fiocruz Pernambuco. The patients/participants provided their written informed consent to participate in this study.
Author Contributions
VV-A planned and performed the experiments, analyzed the data, and wrote the manuscript. JS, IR, and MM helped in carrying out the experiments. JS and AA were responsible for the cultivation of the photosynthetic microorganisms and obtaining the extracts. PA collaborated with referral drugs. RB, DM, and SS wrote sections of the manuscript. RS, VL, and MP contributed substantially to the data analysis and assisted in the writing of the manuscript. All authors contributed to the review of the manuscript and read and approved the submitted version.
Funding
This study was partially financed by the Coordination for the Improvement of Higher Education Personnel – Brazil (CAPES) (Finance Code 001), the Research Excellence Program (CNPq/PROEP/FIOCRUZ) (400711/2019-2), the National Council for Scientific and Technological Development (CNPq) (PQ 301223/2018.1), and the Foundation for Science and Technology Pernambuco (FACEPE) (Finance Code PBPG – 13666-2.11/18).
Conflict of Interest
The authors declare that the research was conducted in the absence of any commercial or financial relationships that could be construed as a potential conflict of interest.
Publisher’s Note
All claims expressed in this article are solely those of the authors and do not necessarily represent those of their affiliated organizations, or those of the publisher, the editors and the reviewers. Any product that may be evaluated in this article, or claim that may be made by its manufacturer, is not guaranteed or endorsed by the publisher.
Acknowledgments
We would like to thank Dr. Maria Edileusa Felinto de Brito, coordinator of the Leishmaniasis Reference Services at Fiocruz-PE, for supplying the Leishmania infantum strain.
Supplementary Material
The Supplementary Material for this article can be found online at: https://www.frontiersin.org/articles/10.3389/fimmu.2022.891495/full#supplementary-material
References
1. Ponte-Sucre A, Gamarro F, Dujardin JC, Barrett MP, López-Vélez R, García-Hernández R, et al. Drug Resistance and Treatment Failure in Leishmaniasis: A 21st Century Challenge. PLoS Negl Trop Dis (2017) 11(12):1–24. doi: 10.1371/journal.pntd.0006052
2. Proverbio D, Spada E, Bagnagatti-De-Giorgi G, Perego R. Failure of Miltefosine Treatment in Two Dogs With Natural Leishmania Infantum Infection. Case Rep Vet Med (2014) 2014:1–6. doi: 10.1155/2014/640151
3. Sukuraman B, Madhubala R. Leishmaniasis: Current Status of Vaccine Development. Curr Mol Med (2004) 4:667–79. doi: 10.2174/1566524043360203
4. Alexander J, Brombacher F. Thelper1/Thelper2 Cells and Resistance/ Susceptibility to Leishmania Infection: Is This Paradigm Still Relevant? Front Immunol (2012) 3:80. doi: 10.3389/fimmu.2012.00080
5. Bhowmick S, Ravindran R, Ali N. IL-4 Contributes to Failure, and Colludes With IL-10 to Exacerbate Leishmania Donovani Infection Following Administration of a Subcutaneous Leishmanial Antigen Vaccine. BMC Microbiol (2014) 14(1):8–20. doi: 10.1186/1471-2180-14-8
6. Moreno J, Vouldoukis L, Schreiber P, Martin V, McGahie D, Gueguen S, et al. Primary Vaccination With the LiESP/QA-21 Vaccine (CaniLeish®) Produces a Cell-Mediated Immune Response Which is Still Present 1 Year Later. Vet Immunol Immunopathol (2014) 158(3-4):199–207. doi: 10.1016/j.vetimm.2014.01.011
7. Resende LA, Roatt BM, Aguiar-Soares RDO, Viana KF, Mendonça LZ, Lanna MF, et al. Cytokine and Nitric Oxide Patterns in Dogs Immunized Withlbsap Vaccine, Before and After Experimental Challenge With Leishmania Chagasi Plus Saliva of Lutzomyia Longipalpis. Vet Parasitol (2013) 198(3-4):371–81. doi: 10.1016/j.vetpar.2013.09.011
8. Nylén S, Gautam S. Immunological Perspectives of Leishmaniasis. J Global Infect Dis (2010) 2(2):135–46. doi: 10.4103/0974-777X.62876
9. Paul WE, Zhu J, Yamane H. Differentiation of Effector CD4 T Cell Populations. Annu Rev Immunol (2010) 28(1):445–89. doi: 10.1146/annurev-immunol-030409-101212
10. Costa-Pereira C, Moreira ML, Soares RP, Marteleto BH, Ribeiro VM, França-Dias MH, et al. One-Year Timeline Kinetics of Cytokine-Mediated Cellular Immunity in Dogs Vaccinated Against Visceral Leishmaniasis. BioMed Cent Vet Res (2015) 11(1):92–102. doi: 10.1186/s12917-015-0397-6
11. Shahbazi M, Zahedifard F, Saljoughian N, Doroud D, Jamshidi S, Mahdavi N, et al. Immunological Comparison of DNA Vaccination Using Two Delivery Systems Against Canine Leishmaniasis. Vet Parasitol (2015) 212:130–9. doi: 10.1016/j.vetpar.2015.07.005
12. Solano-Gallego L, Montserrat-Sangra S, Ordeix L, Martínez-Orellana P. Leishmania Infantum-Specific Production of IFN-γ and IL-10 in Stimulated Blood From Dogs With Clinical Leishmaniosis. Parasites Vectors (2016) 9:317–47. doi: 10.1186/s13071-016-1598-y
13. Amaro HM, Guedes AC, Malcata FX. Antimicrobial Activities of Microalgae: An Invited Review. In: In Science Against Microbial Pathogens: Communicating Current Research and Technological Advances; Formatex Research Center vol. 3. Badajoz, Spain (2011). p. 1272–84.
14. Gamal AAEL. Biological Importance of Marine Algae. Saudi Pharm J (2010) 18(1):1–25. doi: 10.1016/j.jsps.2009.12.001
15. Yamthe LT, Appiah-Opong R, Fokou PT, Tsabang N, Boyom FF, Nyarko A, et al. Marine Algae as Source of Novel Antileishmanial Drugs: A Review. Mar Drugs (2017) 15(11):323–51. doi: 10.3390/md15110323
16. Lauritano C, Andersen JH, Hansen E, Albrigtsen M, Escalera L, Esposito F, et al. Bioactivity Screening of Microalgae for Antioxidant, Anti-Inflammatory, Anticancer, Anti-Diabetes, and Antibacterial Activities. Front Mar Sci (2016) 3:68. doi: 10.3389/fmars.2016.00068
17. Pereira H, Custódio L, Rodrigues M, De Sousa C, Oliveira M, Barreira L, et al. Biological Activities and Chemical Composition of Methanolic Extracts of Selected Autochthonous Microalgae Strains From the Red Sea. Mar Drugs (2015) 13(6):3531–49. doi: 10.3390/md13063531
18. Kwak JK, Baek SH, Woo Y, Han JK, Kim BG, Kim OY, et al. Beneficial Immunostimulatory Effect of Short-Term Chlorellasupplementation: Enhancement of Natural Killer Cell Activity and Early Inflammatory Response (Randomized, Double-Blinded, Placebo-Controlled Trial). Nutr J (2012) 11:53. doi: 10.1186/1475-2891-11-53
19. Schlosser UG. Sammlung Von Algenkulturen. Ber Dtsch Bot Ges (1982) 95:181–276. doi: 10.1111/j.1438-8677.1982.tb02862.x
20. Guillard RRL. Culture of Phytoplankton for Feeding Marine Invertebrates. New York: Plenum Press (1975) p. 26–60. doi: 10.1007/978-1-4615-8714-9_3
21. Barros PDS, Costa-e-Silva PE, Nascimento TP, Costa RMPB, Bezerra RP, Porto ALF. Fibrinolytic Enzyme From Arthrospira Platensis Cultivated in Medium Culture Supplemented With Corn Steep Liquor. Int J Biol Macromol (2020) 164:3446–53. doi: 10.1016/j.ijbiomac.2020.08.217
22. Silva-Júnior JN, Aguiar EM, Mota RA, Bezerra RP, Porto ALF, Herculano PN, et al. Antimicrobial Activity of Photosynthetic Microorganisms Biomass Extract Against Bacterial Isolates Causing Mastitis. Dayri Vet Sci (2019) 10(3):1–7. doi: 10.19080/JDVS.2019.10.555788
23. Smith PK, Krohn RI, Hermanson GT, Mallia AK, Gartner FH, Provenzano MD, et al. Measurement of Protein Using Bicinchoninic. Acid Anal Biochem (1985) 150:76–85. doi: 10.1016/0003-2697(85)90442-7
24. Bradford MM. A Rapid and Sensitive Method for the Quantitation of Microgram Quantities of Protein Utilizing the Principle of Protein-Dye Binding. Anal Biochem (1976) 72:248–54. doi: 10.1006/abio.1976.9999
25. Basak P, Paul S, Majumder R. In Vitro Cytotoxic Study of Aloe Vera Whole Leaf Extract on PBMC and Breast Cancer Cell Line. 2nd International Conference for Convergence in Technology (i2ct) (2017), 124–7. doi: 10.1109/I2CT.2017.8226106.
26. Correia VCS, Lima NO, Oliveira FAZ, Santos APA, Teles CBG, Oliveira J, et al. Evaluation of the Antiplasmodial and Leishmanicidal Potential of Myrciaria Dubia (Myrtaceae) Extract. Rev Soc Bras Med Trop (2016) 49(5):586–92. doi: 10.1590/0037-8682-0227-2016
27. Mosmann T. Rapid Colorimetric Assay for Cellular Growth and Survival: Application to Proliferation and Cytotoxicity Assays: Application to Proliferation and Cytotoxicity Assays. J Immunol Methods (1983) 65(1-2):55–63. doi: 10.1016/0022-1759(83)90303-4
28. Khademvatan S, Eskandari A, Saki J, Foroutan-Rad M. Cytotoxic Activity of Holothuria Leucospilota Extract Against Leishmania Infantum In Vitro. Adv Pharmacol Sci (2016) 2016:1–6. doi: 10.1155/2016/8195381
29. Comandolli-Wyrepkowski CD, Jensen BB, Grafova I, Santos PA, Barros AMC, Soares FV, et al. Antileishmanial Activity of Extracts From Libidibia Ferrea: Development of In Vitro and In Vivo Tests. Acta Amazonica (2017) 47(4):331–40. doi: 10.1590/1809-4392201700871
30. Morais-Teixeira E, Carvalho AS, Costa JCS, Duarte SL, Mendonça JS, Boechat N, et al. In Vitro and In Vivo Activity of Meglumine Antimoniate Produced at Farmanguinhos-Fiocruz, Brazil, Against Leishmania (Leishmania) Amazonensis, L (L.) Chagasi and L (Viannia) Braziliensis. Mem Inst Oswaldo Cruz (2008) 103(4):358–62. doi: 10.1590/s0074-02762008000400008
31. Morais-Teixeira E, Damasceno QS, Galuppo MK, Romanha AJ, Rabello A. The In Vitro Leishmanicidal Activity of Hexadecylphosphocholine (Miltefosine) Against Four Medically Relevant Leishmania Species of Brazil. Mem Inst Oswaldo Cruz (2011) 106(4):475–8. doi: 10.1590/S0074-02762011000400015
32. Pessoa-E-Silva R, Trajano-Silva LAM, Vaitkevicius-Antão V, Santos WJT, Magalhães FB, Moura DMN, et al. Immunoprophylactic Potential of a New Recombinant Leishmania Infantum Antigen for Canine Visceral Leishmaniasis: An In Vitro Finding. Front Immunol (2021) 11:605044. doi: 10.3389/fimmu.2020.605044
33. Bhargava P, Singh R. Developments in Diagnosis and Antileishmanial Drugs. Interdiscip Perspect Infect Dis (2012) 2012:1–13. doi: 10.1155/2012/626838
34. Bouyahya A, Et-Touys A, Bakka N, Fellah H, Abrini J, Bakri Y. Antileishmanial Potential of Medicinal Plant Extracts From the North-West of Morocco. Beni-Suef Univ J Basic And Appl Sci (2018) 7(1):50–4. doi: 10.1016/j.bjbas.2017.06.003
35. Sousa CB, Gangadhar KN, Morais TR, Conserva GAA, Vizetto-Duarte C, Pereira H, et al. Antileishmanial Activity of Meroditerpenoids From the Macroalgae Cystoseira Baccata. Exp Parasitol (2017) 174:1–9. doi: 10.1016/j.exppara.2017.01.002
36. Costa-Silva TA, Conserva GAA, Galisteo AJ Jr, Tempone AG, Lago JHG. Antileishmanial Activity and Immunomodulatory Effect of Secosubamolide, a Butanolide Isolated From Nectandra Oppositifolia (Lauraceae). J Venom Anim And Toxins Incl Trop Dis (2019) 25:1–8. doi: 10.1590/1678-9199-jvatitd-2019-0008
37. Shokri H, Khosravi A, Taghavi M. Efficacy of Spirulina Platensis on Immune Functions in Cancer Mice With Systemic Candidiasis. J Mycol Res (2014) 1(1):7–13.
38. Mao TK, Water JV, Gershwin ME. Effect of Spirulina on the Secretion of Cytokines From Peripheral Blood Mononuclear Cells. J Med Food (2000) 3(3):135–40. doi: 10.1089/jmf.2000.3.135
39. Sheikh SM, Shalaby MAM, Hafez RA, Metwally WSA, El-Ayoty YM. The Immunomodulatory Effects of Probiotic Bacteria on Peripheral Blood Mononuclear Cells (PBMCs) of Allergic Patients. Am J Immunol (2014) 10(3):116–30. doi: 10.3844/ajisp.2014.116.130
40. Ciliberti M, Francavilla M, Intini S, Albenzio M, Marino R, Santillo A, et al. Phytosterols From Dunaliella Tertiolecta Reduce Cell Proliferation in Sheep Fed Flaxseed During Post Partum. Mar Drugs (2017) 15(7):216–30. doi: 10.3390/md15070216
41. Caroprese M, Albenzio MGC, Matteo F, Agostino S. A Mixture of Phytosterols From Dunaliella Tertiolecta Affects Proliferation of Peripheral Blood Mononuclear Cells and Cytokine Production in Sheep. Vet Immunol And Immunopathol (2012) 150(1):27–35. doi: 10.1016/j.vetimm.2012.08.002
Keywords: cyanobacteria, Leishmania infantum, microalgae, immunomodulation, therapy
Citation: Vaitkevicius-Antão V, Moreira-Silva J, Reino IBdSM, Melo MGN, Silva-Júnior JN, Andrade AF, Araújo PSR, Bezerra RP, Marques DdAV, Ferreira S, Pessoa-e-Silva R, Lorena VMB and Paiva-Cavalcanti M (2022) Therapeutic Potential of Photosynthetic Microorganisms for Visceral Leishmaniasis: An Immunological Analysis. Front. Immunol. 13:891495. doi: 10.3389/fimmu.2022.891495
Received: 07 March 2022; Accepted: 27 May 2022;
Published: 30 June 2022.
Edited by:
Abhay Satoskar, The Ohio State University, United StatesReviewed by:
Fátima Ribeiro-Dias, Universidade Federal de Goiás, BrazilRanadhir Dey, United States Food and Drug Administration, United States
Copyright © 2022 Vaitkevicius-Antão, Moreira-Silva, Reino, Melo, Silva-Júnior, Andrade, Araújo, Bezerra, Marques, Ferreira, Pessoa-e-Silva, Lorena and Paiva-Cavalcanti. This is an open-access article distributed under the terms of the Creative Commons Attribution License (CC BY). The use, distribution or reproduction in other forums is permitted, provided the original author(s) and the copyright owner(s) are credited and that the original publication in this journal is cited, in accordance with accepted academic practice. No use, distribution or reproduction is permitted which does not comply with these terms.
*Correspondence: Milena de Paiva-Cavalcanti, bWlsZW5hLmNhdmFsY2FudGlAZmlvY3J1ei5icg==