- 1Department of Biotherapy, State Key Laboratory of Biotherapy and Cancer Center, West China Hospital, Sichuan University, Chengdu, China
- 2West China School of Pharmacy, Sichuan University, Chengdu, China
- 3Department of Immunology, West China School of Basic Medical Sciences and Forensic Medicine, Sichuan University, Chengdu, China
- 4State Key Laboratory of Biotherapy and Cancer Center, West China Hospital, Sichuan University, Chengdu, China
CD4+CD25+Foxp3+ Regulatory (Treg) T cells are mainly generated within the thymus. However, the mechanism of thymic Treg cell (tTreg cell) generation remains to be fully revealed. Although the functions of TCR/CD28 co-stimulation have been widely accepted, the functions of cytokines in the generation of tTreg cells remain highly controversial. In this review, we summarize the existing studies on cytokine regulation of tTreg cell generation. By integrating the key findings of cytokines in tTreg cell generation, we have concluded that four members of γc family cytokines (IL-2, IL-4, IL-7 and IL-15), transforming growth factor β (TGF-β), and three members of TNF superfamily cytokines (GITRL, OX40L and TNF-α) play vitally important roles in regulating tTreg cell generation. We also point out all disputed points and highlight critical scientific questions that need to be addressed in the future.
Introduction
CD4+ Regulatory T (Treg) cells that express IL-2 receptor α-chain (CD25) and the transcription factor forkhead box P3 (Foxp3) are the major cell population that maintains immune tolerance (1–6). Since these cells were identified in 1995 (2), Treg cells have been demonstrated to play extremely important roles in maintaining tolerance to auto-antigens (7, 8) and commensal microbiota (9, 10), controlling maternal-fetal immune interactions (11, 12), and suppressing overactive immune responses during infection (13, 14). On the other hand, Treg cell-mediated immune suppression can also promote tumor immune escape (15, 16). Therefore, targeting Treg cells could be a promising strategy to treat autoimmune disorders, maternal-fetal conflict, infections, and malignant tumors.
A majority of Treg cells are generated in the thymus (thymic Treg cells, tTreg cells), however some Treg cells can also be generated in periphery (pTreg cells) (17). Although it has been well documented that tTreg cells are generated during CD4+ thymocyte development, the clear mechanisms of tTreg cell development is still not completely understood. Since T-cell receptor (TCR) stimulation from self-antigens and CD28 co-stimulation during thymocyte development are indispensable for tTreg cell generation (18–20), the mainstream view once believed that high-affinity TCR signal is the main driving force for inducing Treg cell differentiation (21–23). However, later studies demonstrated that tTreg cells could be generated from developing CD4+ thymocytes expressing TCRs with a broad range of self-reactivity (24, 25), showing that the self-reactivity of the TCR signal is not the deciding factor for tTreg cell generation.
In contrast, a two-step model of tTreg cell generation is gaining acceptance (26–29). The first step is driven by self-antigen induced TCR stimulation and CD28 co-stimulation, which leads to differentiation of CD4+ CD8- Foxp3- CD25+ tTreg cell precursors (CD25+ Foxp3- tTreg precursors) and CD4+ CD8- Foxp3+ CD25- Treg cell precursors (Foxp3+ CD25- tTreg precursors) from developing CD4+ CD8- thymocytes. The second step relies on IL-2, which leads to the generation of CD25+Foxp3+ mature tTreg cells from CD25+ Foxp3- tTreg precursors and Foxp3+ CD25- tTreg precursors. This model proposes that both precursor populations are induced by TCR/CD28 co-stimulation, and both precursor populations rely on IL-2 to differentiate into mature tTreg cells. However, one recent study indicated that CD25+ Foxp3- tTreg precursors and Foxp3+ CD25- tTreg precursors are generated through two distinct developmental programs (30), suggesting that besides TCR/CD28 co-stimulation, some other key factors must be involved during development of these two tTreg precursor populations. All this evidence shows that this model still needs further refinements.
Besides TCR/CD28 co-stimulation, the most probable factors that mediate the distinct developmental programs of tTreg cell are different cytokines. Other than IL-2 and IL-15, three members of the tumor necrosis factor (TNF) superfamily cytokines (GITRL, OX40L and TNF-α) were demonstrated to promote tTreg generation (31). Moreover, TGF-β has also been shown to be important for tTreg cell generation (25, 32). In this review, we summarize the existing studies showing the important functions of cytokines in tTreg cell generation. We conclude that IL-2, IL-7, IL-15, IL-4, TGF-β, GITRL, OX40L, and TNF-α all play important roles in regulating tTreg cell generation, although regulation mechanisms of these cytokines have yet to be confirmed.
Four γc Family Cytokines (IL-2, IL-7, IL-15 and IL-4)
Function of IL-2, IL-7, IL-15 and IL-4 in tTreg Cell Generation
Before Treg cells were well identified, it was determined that mice deficient in IL-2 (33–35), IL-2 receptor α chain (IL-2Rα, also called CD25) (36) or IL-2 receptor β chain (IL-2Rβ, also called CD122) (37) would develop severe autoimmunity. It was a surprising finding since IL-2 was found to be a critical T cell growth factor (38–40). Since Treg cells have been identified, CD25 was proven to be a surface marker of Treg cells (2), and then it was determined that Treg cell-deficient scurfy mice develop severe autoimmunity as well (3–5, 41). These findings suggested that IL-2 might play a vital role in Treg cell generation.
However, the function of IL-2 in tTreg cell generation is still contentious. Some studies are against the idea that IL-2 is key for tTreg cell generation, because a significant number of CD4+ CD8- CD25- FOXP3+ thymocytes were still present in IL-2 knockout (Il2-/-) mice, and these cells could still suppress inflammation in adaptive transfer mice model (42–44), although CD25- FOXP3+ thymocytes were defined as tTreg precursors in the two-step model (29). Moreover, a recent study found that IL-2 could modulate the tTreg cell epigenetic landscape by targeting genome wide chromatin accessibility (45). These studies showed that IL-2 is dispensable for tTreg cell development, but important for mature tTreg cell survival, tTreg cell stabilization, and tTreg cell suppression function. Consistent with this idea, it was determined that Foxp3 is a proapoptotic protein and these Foxp3+ CD25- tTreg precursors completed for the limited IL-2 to support their survival (28). In contrast, some studies found that although mice deficient in IL-2 or IL-2Rα had a certain number of Foxp3+ cells, their tTreg cells were not mature, and mice deficient in IL-2Rβ were shown to have a significant decrease in Treg numbers (44, 46), suggesting IL-2 should be important for tTreg cell development. Consistent with this idea, in the two-step model of tTreg cell development, it was found that CD25+ FOXP3- tTreg precursors needed IL-2 to convert to mature tTreg cells (26, 27).
IL-2 receptor γ chain (IL-2Rγ), also known as the common cytokine receptor γ chain (γc) or CD132, is a common component of the receptors for IL-2, IL-4, IL-7, IL-9, IL-15, and IL-21 (γc family cytokines) (47, 48). Therefore, besides IL-2, functions of other γc family cytokines in tTreg cell generation have also attracted a lot of attention. Importantly, mice deficient in IL-2Rβ resulted in a large reduction in the number of tTreg cells, whereas mice deficient in IL-2 or IL-2Rα still have high Foxp3 expression (42, 44, 46). IL-2Rβ is the receptor for both IL-2 and IL-15, so the function of IL-15 in tTreg cell generation was determined. Indeed, IL-2 and IL-15 double knockout (Il2-/-xIl15-/-) mice have a significant decrease in Treg numbers compared with Il2-/- mice (44), showing that IL-2 and IL-15 are important for tTreg cell generation. Moreover, mice deficient in IL-2Rγ were shown to be devoid of tTreg cells and have no expression of Foxp3 (42, 49), suggesting other γc family cytokines might also be important for tTreg cell generation. After in-depth research and verification, IL-7 was proven to be important for tTreg cell generation (50, 51). Moreover, IL-2Rβ and IL-7 receptor subunit α (IL-7Rα, also known as CD127) double knockout (Il2rb-/-xIl7ra-/-) mice were also devoid of tTreg cells, just like mice deficient in IL-2Rγ (50). Further studies proved that IL-2, IL-7, and IL-15 induces STAT5 phosphorylation and this process is indispensable for tTreg cell generation (49, 50), as STAT5 phosphorylation is critical for tTreg cell development by regulating Foxp3 expression (52–55). Taken together, three γc family cytokines, IL-2, IL-7, and IL-15 are essential for Treg cell generation (Figure 1). However, it remains to be confirmed whether these cytokines mainly induce tTreg cell development, promote tTreg cell survival, and/or maintain tTreg cell stabilization.
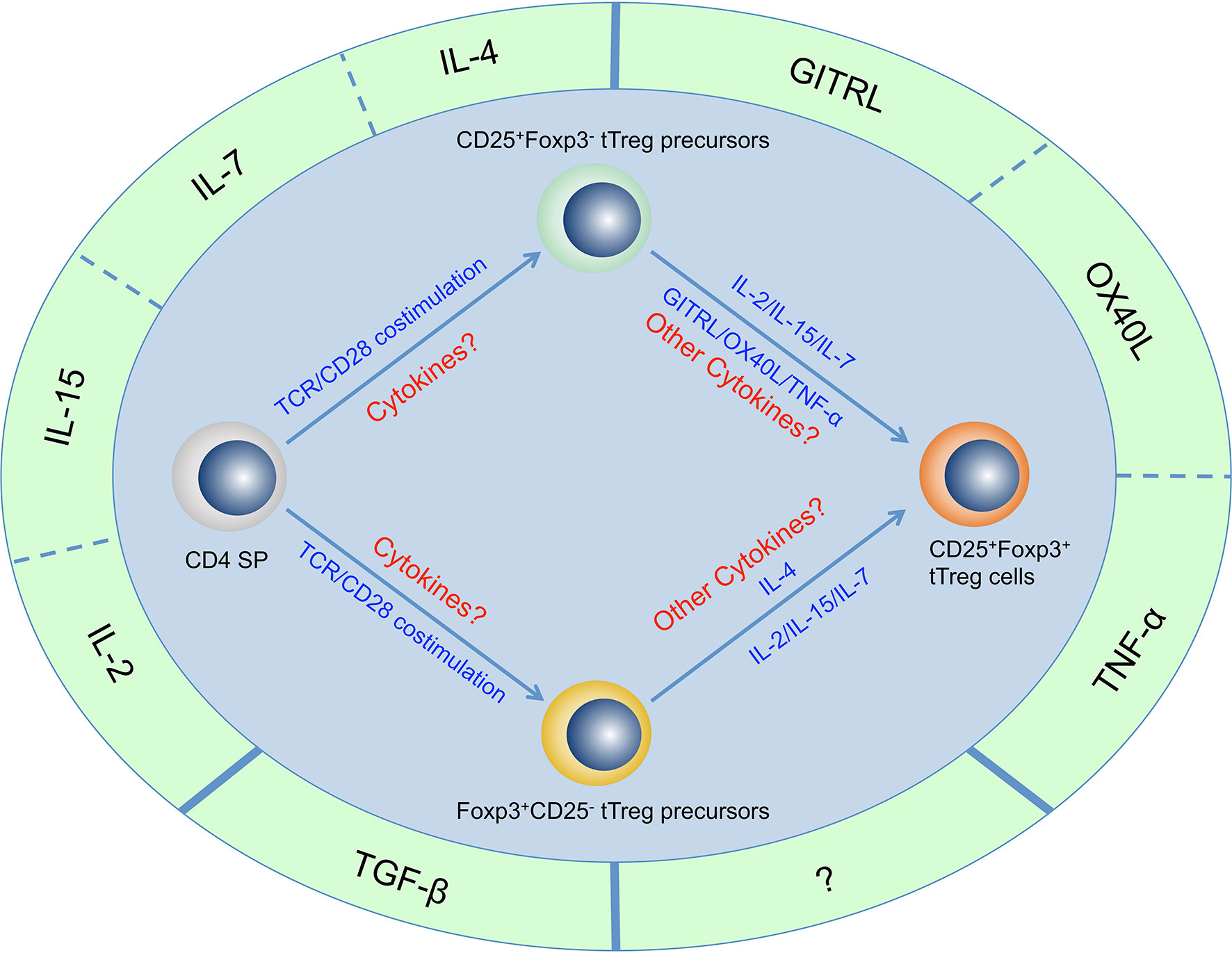
Figure 1 Cytokines that are important for tTreg cell generation. Four γc family cytokines (IL-2, IL-4, IL-7, and IL-15), Three TNF superfamily cytokines (GITRL, OX40L, and TNF-α) and TGF-β have been determined to be important for tTreg cell generation. There may be other cytokines that are important for tTreg cell generation but have not yet been identified. It has been proven that CD4+CD8-Foxp3-CD25+ thymocytes and CD4+CD8-Foxp3+CD25- thymocytes are two populations of tTreg cell precursors that generated through two distinct developmental programs, but the regulatory network of these cytokines in the development of these two precursor populations and mature tTreg cell has not been fully revealed.
In the beginning, another γc family cytokine IL-4 was thought to be not important for tTreg cell generation as mice deficient in IL-4 receptor α (IL-4Rα) had absolutely normal tTreg cell generation (50). Moreover, IL-4 was actually shown to suppress Treg cell generation and induce T helper-9 cells (Th9 cells) in periphery and in vitro (56–58). However, The same research team corrected the views (59), as they found that IL-4 could promote tTreg cell generation from Foxp3+ CD25- tTreg precursors, although IL-4 could not support tTreg cell generation from CD25+Foxp3- tTreg precursors (30). This evidence shows that IL-4 plays a role in tTreg cell development from Foxp3+CD25- tTreg precursors.
Source of IL-2, IL-7, IL-15 and IL-4 in the Thymus
Determining the cellular sources of IL-2, IL-7, and IL-15 within the thymus are important in revealing the generation of tTreg cells, and it is also important for autoimmunity treatment through the manipulation of tTreg cells. It has been shown that tTreg cells could not produce IL-2 to support tTreg cell development and survival because Foxp3 represses expression of IL-2 (3, 60). More than that, in IL-2 wild type (Il2+/+) and Il2-/- bone marrow chimera mice, tTreg cell generation was totally rectified in Il2-/- thymocytes and these bone marrow chimera mice did not develop autoimmunity (20). Therefore, tTreg cell generation mainly relied on IL-2 produced by non-Treg cells.
Although dendritic cells (DCs) and B cells were shown to be able to produce IL-2, mice that have selectively deleted IL-2 in DCs and B cells had been shown to have normal tTreg cell development and homeostasis (61, 62), showing DCs and B cells are not the major cellular sources of IL-2 in the thymus. In contrast, tTreg cell development was largely impaired in Il2f/f CD4-Cre mice, suggesting T cells are the key cellular source of IL-2 in the thymus (62). Moreover, a recent study determined that cells that secrete IL-2 are predominantly mature CD4+ CD8- (CD4SP) thymocytes in the thymus; it has further been identified that IL-2 is mainly produced by self-reactive CD4SP thymocytes through single-cell RNA sequencing analysis (63). This evidence shows that self-reactive CD4SP thymocytes are the major cellular sources of IL-2 in the thymus.
Unlike IL-2, the major cellular sources of IL-7 and IL-15 are not T cells. It was determined that both cortical thymic epithelial cells (TECs) and medullary TECs express high levels of IL-7, and IL-7 expression in cortical TECs is even higher than in medullary TECs (64). However, medullary TECs that highly expressed MHC class II were the major cellular source of IL-15 (65). Interestingly, it is well documented that tTreg cells are mainly generated in the medulla (66–69), suggesting it might be why IL-7 is not as important as IL-2 and IL-15 during tTreg cell generation in thymus. So far, the major cellular source of IL-4 in the thymus has not been determined (30).
TGF-β
Function of TGF-β in tTreg Cell Generation
Although it has been determined that TGF-β is the key inducer of Foxp3 in periphery and in vitro (70, 71), the function of TGF-β in tTreg cell generation is still in dispute. During early research, TGF-β was thought to be dispensable for tTreg cell development, because TGF-β1 deficient (8-10 days old) mice (Tgfb1-/-) had normal frequency of tTreg cell in thymus (72), and T cell-specific TGF-β receptor II-deficient mice (Tgfbr2f/f x CD4-Cre) did not change the frequency of tTreg cell in thymus (12-14 days old mice) either (73, 74). In contrast, it was shown that TGF-β is critical for tTreg cell stabilization and regulatory function (72–74). Although the same research team repudiated their earlier study and thought TGF-β was not important for tTreg cell function and stabilization (75, 76), a recent study determined that TGF-β is critical for tTreg cell function in specific tissue environments, but not important for tTreg cell stabilization (77).
Surprisingly, TGF-β was identified to be important for tTreg cell development by studying tTreg cell generation in 3-5 days old neonatal mice (32, 78). It was shown that deletion of TGF-β receptor I (Tgfbr1f/f x Lck-Cre) in T cells blocks tTreg cell development largely in 3-5 days old neonatal mice, then tTreg cell frequency was recovered and became even higher in thymus of 3-4 weeks old Tgfbr1f/f x Lck-Cre mice than that in WT mice (32). It was then shown that tTreg cell frequency was increased in thymus due to increased tTreg cell proliferation in Tgfbr1f/f x Lck-Cre mice, as thymocytes lacking TGF-β receptor I produced more IL-2 and tTreg cells lacking TGF-β receptor I proliferated much faster in response to IL-2 (32). More importantly, further deletion of IL-2 in Tgfbr1f/f x Lck-Cre mice (Tgfbr1f/f x Lck-Cre x Il2-/-) blocked tTreg cell development and expansion totally, as 3-4 weeks old Tgfbr1f/f x Lck-Cre x Il2-/- mice were devoid of tTreg cells as well (32).
The other group also reported a lack of tTreg cells in the thymus of 3-5 days old neonatal Tgfbr2f/f x CD4-Cre mice, but they proposed that this was due to increased tTreg cell apoptosis caused by the deletion of TGF-β signaling (78). Since TGF-β promotes thymocyte cell survival (79), a Treg cell-specific TGF-β receptor I-deficient mice (Tgfbr1f/f x Foxp3-Cre) was generated to determine whether the main function of TGF-β is to promote tTreg cell survival in the thymus (25). Surprisingly, it was found that tTreg cell frequency and number in Tgfbr1f/f x Foxp3-Cre mice did not decrease at all (25), and the aged Tgfbr1f/f x Foxp3-Cre mice had even more tTreg cells (77), showing the main function of TGF-β in tTreg cell generation is not to support tTreg cell survival. Existing mechanism studies have found that Smad3 could bind at the conserved noncoding sequence 1 (CNS1) of Foxp3 enhancer and induce Foxp3 expression (80, 81), but it was argued that Smad3 binding to the foxp3 enhancer was dispensable for tTreg cell development (82). Taken together, these findings show that TGF-β is critical to tTreg cell development, although the exact mechanisms need to be further identified (Figure 1).
Source of TGF-β in the Thymus
Thymocyte apoptosis has been identified to increase by day 2 after birth (83), TGF-β level was found to increase significantly in the thymus by day 3 after birth (25), and tTreg cells were shown to appear in large numbers in the thymus by day 3 after birth (84). This evidence suggests that tTreg cell generation, thymocyte apoptosis, and TGF-β production are highly correlated. Indeed, one study showed that the intrathymic concentration of TGF-β is highly dependent on thymocyte apoptosis (25). However, the major cellular source of TGF-β in the thymus has not been uncovered. Based on the existing studies, TGF-β is likely to be released from two possible cellular sources. The first possible source is apoptotic T cells that release TGF-β directly (85), and the second possible source is phagocytes that release TGF-β after these cells phagocytize apoptotic cells (86, 87).
It is worth mentioning that TGF-β is secreted into the extracellular matrix in an inactive latent form (latent TGF-β) and needs to be activated to produce bioactive TGF-β (88, 89). By now, it has not been determined how TGF-β is activated in the thymus. One possible mechanism for the activation of TGF-β in the thymus is through apoptotic cell-released ROS, as apoptotic thymocytes could release a high level of ROS (85), and ROS has been shown to induce TGF-β activation and promote Treg cell generation in periphery (90–92).
Three TNF Superfamily Cytokines (GITRL, OX40L and TNF-α)
Function of GITRL, OX40L, and TNF-α in tTreg Cell Generation
The tumor necrosis factor (TNF) superfamily is a protein superfamily originally produced as type-II transmembrane proteins, but these proteins can function as cytokines once they are cleaved off the cell membrane by metalloproteinases (93). The receptors of the TNF superfamily are tumor necrosis factor receptor superfamily (TNFRSF) (94). It has been determined that CD25+ Foxp3- tTreg precursors and mature tTreg cells express high levels of TNFRSF members called Glucocorticoid-induced tumor necrosis factor receptor-related protein (GITR, also known as CD357 or TNFRSF18), OX40 (also known as CD134 or TNFRSF4) and TNFR2 (also known as CD120b or TNFRSF1B) (26, 31, 95). Moreover, it was found that a TNF superfamily member, TNF-α, a ligand of TNFR2, could promote Treg cell expansion in vivo (96–98). These findings suggest that the TNF superfamily might be important for tTreg cell generation.
Three TNF superfamily members, GITRL, OX40L, and TNF-α have been identified to promote tTreg cell generation (31, 99). One study reported that deficiency in TNFR2 reduced tTreg cell generation significantly (99). Another study showed that deficiency in all three of the TNFRSF members GITR, OX40, and TNFR2, or neutralization of TNF superfamily members GITRL, OX40L, and TNF-α together, markedly inhibited the generation of tTreg cells (31) (Figure 1). It was shown that GITRL, OX40L, and TNF-α could convert CD25+ Foxp3- tTreg precursors into mature Foxp3+ Treg cells at very low dose of IL-2 (31), showing these three TNF superfamily members promote tTreg cell mature from CD25+ Foxp3- tTreg precursors. However, it is still not clear whether TNF superfamily members and IL-2 complement each other, or TNF superfamily members just function as compensatory signals of IL-2 signal.
Source of GITRL, OX40L and TNF-α in the Thymus
Although the major cellular sources of GITRL, OX40L, and TNF-α have not been well defined, it was identified that medullary TECs expressed GITRL, OX40L, and TNF-α, while conventional dendritic cells (cDCs) and plasmacytoid dendritic cells (pDCs) expressed only GITRL and TNF-α (31). Further studies are needed to determine which kind of APCs are the major cellular source of GITRL, OX40L, and TNF-α. Moreover, whether membrane-bound or soluble GITRL, OX40L, and TNF-α play a more important role in tTreg cell generation has not yet been determined either.
Conclusions and Future Perspective
By summarizing the existing studies of cytokines in tTreg cell generation, we conclude that four members of γc family cytokines (IL-2, IL-4, IL-7 and IL-15), transforming growth factor β (TGF-β), and three members of TNF superfamily cytokines (GITRL, OX40L, and TNF-α) play vitally important roles in regulating tTreg cell generation, although regulation mechanisms of these cytokines have yet to be confirmed. Functions of these cytokines in tTreg cell generation are still divisive. For example, opinions are still divided on the functions of TGF-β and IL-2, whether they are important for tTreg cell development, survival, and/or proliferation are still controversial.
On the other hand, when and how cytokines interact with each other and mediate tTreg cell generation in the thymus remains to be fully revealed. Also, when and how these cytokines take effect during tTreg cell development is still unclear. Therefore, future studies should focus on why developing tTreg cells are divided into two populations of tTreg precursors. Since CD25+ Foxp3- tTreg precursors and Foxp3+ CD25- tTreg precursors are generated through two distinct developmental programs (30), it is very likely that cytokines play key roles in inducing these two precursor populations besides TCR/CD28 co-stimulation. So far, it has been proven that IL-4 can support tTreg cell generation from Foxp3+ CD25- tTreg precursors (30), and TNF superfamily cytokines (GITRL, OX40L and TNF-α) can support tTreg cell generation from CD25+ Foxp3- tTreg precursors (31). These findings can partially explain the differences of CD25+ Foxp3- tTreg precursors and Foxp3+ CD25- tTreg precursors. However, the regulatory network of these cytokines during the development of tTreg precursors and mature tTreg cell has not yet been fully revealed. It is beyond all doubt that answering these basic questions is extremely important for fully disclosing the generation of tTreg cells.
Author Contributions
MT drafted the manuscript. FJ, FN, FZ, and ZY reviewed and edited the manuscript. DZ supervised the work and wrote the manuscript. All authors contributed to the article and approved it for publication.
Funding
This work was supported by the National Natural Science Foundation of China (NO. 82171829, 81600876), the Key Project of the Science and Technology Department of Sichuan Province (NO. 2022YFH0100, 2020YFS0210), the 1·3·5 Project for Disciplines of Excellence, West China Hospital, Sichuan University (NO. ZYYC21012), and the Fundamental Research Funds for the Central Universities (NO. 20822041E4084).
Conflict of Interest
The authors declare that the research was conducted in the absence of any commercial or financial relationships that could be construed as a potential conflict of interest.
Publisher’s Note
All claims expressed in this article are solely those of the authors and do not necessarily represent those of their affiliated organizations, or those of the publisher, the editors and the reviewers. Any product that may be evaluated in this article, or claim that may be made by its manufacturer, is not guaranteed or endorsed by the publisher.
Acknowledgments
DZ sincerely wants to commemorate Dr. Sang-A Park, who passed away suddenly on January 22, 2018.
References
1. Sakaguchi S. Regulatory T Cells: Key Controllers of Immunologic Self-Tolerance. Cell (2000) 101(5):455–8. doi: 10.1016/s0092-8674(00)80856-9
2. Sakaguchi S, Sakaguchi N, Asano M, Itoh M, Toda M. Immunologic Self-Tolerance Maintained by Activated T Cells Expressing IL-2 Receptor Alpha-Chains (CD25). Breakdown of a Single Mechanism of Self-Tolerance Causes Various Autoimmune Diseases. J Immunol (1995) 155(3):1151–64.
3. Hori S, Nomura T, Sakaguchi S. Control of Regulatory T Cell Development by the Transcription Factor Foxp3. Science (2003) 299(5609):1057–61. doi: 10.1126/science.1079490
4. Fontenot JD, Gavin MA, Rudensky AY. Foxp3 Programs the Development and Function of CD4+CD25+ Regulatory T Cells. Nat Immunol (2003) 4(4):330–6. doi: 10.1038/ni904
5. Khattri R, Cox T, Yasayko SA, Ramsdell F. An Essential Role for Scurfin in CD4+CD25+ T Regulatory Cells. Nat Immunol (2003) 4(4):337–42. doi: 10.1038/ni909
6. Malek TR, Yu A, Vincek V, Scibelli P, Kong L. CD4 Regulatory T Cells Prevent Lethal Autoimmunity in IL-2Rbeta-Deficient Mice. Implications for the Nonredundant Function of IL-2. Immunity (2002) 17(2):167–78. doi: 10.1016/s1074-7613(02)00367-9
7. Zhang D, Tu E, Kasagi S, Zanvit P, Chen Q, Chen W. Manipulating Regulatory T Cells: A Promising Strategy to Treat Autoimmunity. Immunotherapy (2015) 7(11):1201–11. doi: 10.2217/imt.15.79
8. Sakaguchi S. Naturally Arising CD4+ Regulatory T Cells for Immunologic Self-Tolerance and Negative Control of Immune Responses. Annu Rev Immunol (2004) 22:531–62. doi: 10.1146/annurev.immunol.21.120601.141122
9. Cebula A, Seweryn M, Rempala GA, Pabla SS, McIndoe RA, Denning TL, et al. Thymus-Derived Regulatory T Cells Contribute to Tolerance to Commensal Microbiota. Nature (2013) 497(7448):258–62. doi: 10.1038/nature12079
10. Round JL, Mazmanian SK. Inducible Foxp3+ Regulatory T-Cell Development by a Commensal Bacterium of the Intestinal Microbiota. Proc Natl Acad Sci USA (2010) 107(27):12204–9. doi: 10.1073/pnas.0909122107
11. Aluvihare VR, Kallikourdis M, Betz AG. Regulatory T Cells Mediate Maternal Tolerance to the Fetus. Nat Immunol (2004) 5(3):266–71. doi: 10.1038/ni1037
12. Kahn DA, Baltimore D. Pregnancy Induces a Fetal Antigen-Specific Maternal T Regulatory Cell Response That Contributes to Tolerance. Proc Natl Acad Sci USA (2010) 107(20):9299–304. doi: 10.1073/pnas.1003909107
13. Lanteri MC, O’Brien KM, Purtha WE, Cameron MJ, Lund JM, Owen RE, et al. Tregs Control the Development of Symptomatic West Nile Virus Infection in Humans and Mice. J Clin Invest (2009) 119(11):3266–77. doi: 10.1172/JCI39387
14. Oldenhove G, Bouladoux N, Wohlfert EA, Hall JA, Chou D, Dos Santos L, et al. Decrease of Foxp3+ Treg Cell Number and Acquisition of Effector Cell Phenotype During Lethal Infection. Immunity (2009) 31(5):772–86. doi: 10.1016/j.immuni.2009.10.001
15. Curiel TJ. Tregs and Rethinking Cancer Immunotherapy. J Clin Invest (2007) 117(5):1167–74. doi: 10.1172/JCI31202
16. Tanaka A, Sakaguchi S. Regulatory T Cells in Cancer Immunotherapy. Cell Res (2017) 27(1):109–18. doi: 10.1038/cr.2016.151
17. Shevach EM, Thornton AM. Ttregs, Ptregs, and Itregs: Similarities and Differences. Immunol Rev (2014) 259(1):88–102. doi: 10.1111/imr.12160
18. Jordan MS, Boesteanu A, Reed AJ, Petrone AL, Holenbeck AE, Lerman MA, et al. Thymic Selection of CD4+CD25+ Regulatory T Cells Induced by an Agonist Self-Peptide. Nat Immunol (2001) 2(4):301–6. doi: 10.1038/86302
19. Apostolou I, Sarukhan A, Klein L, von Boehmer H. Origin of Regulatory T Cells With Known Specificity for Antigen. Nat Immunol (2002) 3(8):756–63. doi: 10.1038/ni816
20. Tai X, Cowan M, Feigenbaum L, Singer A. CD28 Costimulation of Developing Thymocytes Induces Foxp3 Expression and Regulatory T Cell Differentiation Independently of Interleukin 2. Nat Immunol (2005) 6(2):152–62. doi: 10.1038/ni1160
21. Stritesky GL, Jameson SC, Hogquist KA. Selection of Self-Reactive T Cells in the Thymus. Annu Rev Immunol (2012) 30:95–114. doi: 10.1146/annurev-immunol-020711-075035
22. Caton AJ, Kropf E, Simons DM, Aitken M, Weissler KA, Jordan MS. Strength of TCR Signal From Self-Peptide Modulates Autoreactive Thymocyte Deletion and Foxp3(+) Treg-Cell Formation. Eur J Immunol (2014) 44(3):785–93. doi: 10.1002/eji.201343767
23. Hsieh CS, Lee HM, Lio CW. Selection of Regulatory T Cells in the Thymus. Nat Rev Immunol (2012) 12(3):157–67. doi: 10.1038/nri3155
24. Lee HM, Bautista JL, Scott-Browne J, Mohan JF, Hsieh CS. A Broad Range of Self-Reactivity Drives Thymic Regulatory T Cell Selection to Limit Responses to Self. Immunity (2012) 37(3):475–86. doi: 10.1016/j.immuni.2012.07.009
25. Konkel JE, Jin W, Abbatiello B, Grainger JR, Chen W. Thymocyte Apoptosis Drives the Intrathymic Generation of Regulatory T Cells. Proc Natl Acad Sci USA (2014) 111(4):E465–73. doi: 10.1073/pnas.1320319111
26. Lio CW, Hsieh CS. A Two-Step Process for Thymic Regulatory T Cell Development. Immunity (2008) 28(1):100–11. doi: 10.1016/j.immuni.2007.11.021
27. Burchill MA, Yang J, Vang KB, Moon JJ, Chu HH, Lio CW, et al. Linked T Cell Receptor and Cytokine Signaling Govern the Development of the Regulatory T Cell Repertoire. Immunity (2008) 28(1):112–21. doi: 10.1016/j.immuni.2007.11.022
28. Tai X, Erman B, Alag A, Mu J, Kimura M, Katz G, et al. Foxp3 Transcription Factor is Proapoptotic and Lethal to Developing Regulatory T Cells Unless Counterbalanced by Cytokine Survival Signals. Immunity (2013) 38(6):1116–28. doi: 10.1016/j.immuni.2013.02.022
29. Tai X, Singer A. Basis of Treg Development in the Thymus. Cell Cycle (2014) 13(4):501–2. doi: 10.4161/cc.27787
30. Owen DL, Mahmud SA, Sjaastad LE, Williams JB, Spanier JA, Simeonov DR, et al. Thymic Regulatory T Cells Arise via Two Distinct Developmental Programs. Nat Immunol (2019) 20(2):195–205. doi: 10.1038/s41590-018-0289-6
31. Mahmud SA, Manlove LS, Schmitz HM, Xing Y, Wang Y, Owen DL, et al. Costimulation via the Tumor-Necrosis Factor Receptor Superfamily Couples TCR Signal Strength to the Thymic Differentiation of Regulatory T Cells. Nat Immunol (2014) 15(5):473–81. doi: 10.1038/ni.2849
32. Liu Y, Zhang P, Li J, Kulkarni AB, Perruche S, Chen W. A Critical Function for TGF-Beta Signaling in the Development of Natural CD4+CD25+Foxp3+ Regulatory T Cells. Nat Immunol (2008) 9(6):632–40. doi: 10.1038/ni.1607
33. Schorle H, Holtschke T, Hunig T, Schimpl A, Horak I. Development and Function of T Cells in Mice Rendered Interleukin-2 Deficient by Gene Targeting. Nature (1991) 352(6336):621–4. doi: 10.1038/352621a0
34. Sadlack B, Merz H, Schorle H, Schimpl A, Feller AC, Horak I. Ulcerative Colitis-Like Disease in Mice With a Disrupted Interleukin-2 Gene. Cell (1993) 75(2):253–61. doi: 10.1016/0092-8674(93)80067-o
35. Kramer S, Schimpl A, Hunig T. Immunopathology of Interleukin (IL) 2-Deficient Mice: Thymus Dependence and Suppression by Thymus-Dependent Cells With an Intact IL-2 Gene. J Exp Med (1995) 182(6):1769–76. doi: 10.1084/jem.182.6.1769
36. Willerford DM, Chen J, Ferry JA, Davidson L, Ma A, Alt FW. Interleukin-2 Receptor Alpha Chain Regulates the Size and Content of the Peripheral Lymphoid Compartment. Immunity (1995) 3(4):521–30. doi: 10.1016/1074-7613(95)90180-9
37. Suzuki H, Kundig TM, Furlonger C, Wakeham A, Timms E, Matsuyama T, et al. Deregulated T Cell Activation and Autoimmunity in Mice Lacking Interleukin-2 Receptor Beta. Science (1995) 268(5216):1472–6. doi: 10.1126/science.7770771
38. Farrar JJ, Fuller-Farrar J, Simon PL, Hilfiker ML, Stadler BM, Farrar WL. Thymoma Production of T Cell Growth Factor (Interleukin 2). J Immunol (1980) 125(6):2555–8.
39. Friedmann MC, Migone TS, Russell SM, Leonard WJ. Different Interleukin 2 Receptor Beta-Chain Tyrosines Couple to at Least Two Signaling Pathways and Synergistically Mediate Interleukin 2-Induced Proliferation. Proc Natl Acad Sci U.S.A. (1996) 93(5):2077–82. doi: 10.1073/pnas.93.5.2077
40. Morgan DA, Ruscetti FW, Gallo R. Selective In Vitro Growth of T Lymphocytes From Normal Human Bone Marrows. Science (1976) 193(4257):1007–8. doi: 10.1126/science.181845
41. Godfrey VL, Wilkinson JE, Russell LB. X-Linked Lymphoreticular Disease in the Scurfy (Sf) Mutant Mouse. Am J Pathol (1991) 138(6):1379–87.
42. Fontenot JD, Rasmussen JP, Gavin MA, Rudensky AY. A Function for Interleukin 2 in Foxp3-Expressing Regulatory T Cells. Nat Immunol (2005) 6(11):1142–51. doi: 10.1038/ni1263
43. D’Cruz LM, Klein L. Development and Function of Agonist-Induced CD25+Foxp3+ Regulatory T Cells in the Absence of Interleukin 2 Signaling. Nat Immunol (2005) 6(11):1152–9. doi: 10.1038/ni1264
44. Furtado GC, Curotto de Lafaille MA, Kutchukhidze N, Lafaille JJ. Interleukin 2 Signaling Is Required for CD4(+) Regulatory T Cell Function. J Exp Med (2002) 196(6):851–7. doi: 10.1084/jem.20020190
45. Chorro L, Suzuki M, Chin SS, Williams TM, Snapp EL, Odagiu L, et al. Interleukin 2 Modulates Thymic-Derived Regulatory T Cell Epigenetic Landscape. Nat Commun (2018) 9(1):5368. doi: 10.1038/s41467-018-07806-6
46. Soper DM, Kasprowicz DJ, Ziegler SF. Il-2rbeta Links Il-2r Signaling With Foxp3 Expression. Eur J Immunol (2007) 37(7):1817–26. doi: 10.1002/eji.200737101
47. Leonard WJ, Lin JX, O’Shea JJ. The Gammac Family of Cytokines: Basic Biology to Therapeutic Ramifications. Immunity (2019) 50(4):832–50. doi: 10.1016/j.immuni.2019.03.028
48. Lin JX, Migone TS, Tsang M, Friedmann M, Weatherbee JA, Zhou L, et al. The Role of Shared Receptor Motifs and Common Stat Proteins in the Generation of Cytokine Pleiotropy and Redundancy by IL-2, IL-4, IL-7, IL-13, and IL-15. Immunity (1995) 2(4):331–9. doi: 10.1016/1074-7613(95)90141-8
49. Burchill MA, Yang J, Vogtenhuber C, Blazar BR, Farrar MA. IL-2 Receptor Beta-Dependent STAT5 Activation Is Required for the Development of Foxp3+ Regulatory T Cells. J Immunol (2007) 178(1):280–90. doi: 10.4049/jimmunol.178.1.280
50. Vang KB, Yang J, Mahmud SA, Burchill MA, Vegoe AL, Farrar MA. IL-2, -7, and -15, But Not Thymic Stromal Lymphopoeitin, Redundantly Govern CD4+Foxp3+ Regulatory T Cell Development. J Immunol (2008) 181(5):3285–90. doi: 10.4049/jimmunol.181.5.3285
51. Bayer AL, Lee JY, de la Barrera A, Surh CD, Malek TR. A Function for IL-7R for CD4+CD25+Foxp3+ T Regulatory Cells. J Immunol (2008) 181(1):225–34. doi: 10.4049/jimmunol.181.1.225
52. Yao Z, Kanno Y, Kerenyi M, Stephens G, Durant L, Watford WT, et al. Nonredundant Roles for Stat5a/B in Directly Regulating Foxp3. Blood (2007) 109(10):4368–75. doi: 10.1182/blood-2006-11-055756
53. Burchill MA, Goetz CA, Prlic M, O’Neil JJ, Harmon IR, Bensinger SJ, et al. Distinct Effects of STAT5 Activation on CD4+ and CD8+ T Cell Homeostasis: Development of CD4+CD25+ Regulatory T Cells Versus CD8+ Memory T Cells. J Immunol (2003) 171(11):5853–64. doi: 10.4049/jimmunol.171.11.5853
54. Dikiy S, Li J, Bai L, Jiang M, Janke L, Zong X, et al. A Distal Foxp3 Enhancer Enables Interleukin-2 Dependent Thymic Treg Cell Lineage Commitment for Robust Immune Tolerance. Immunity (2021) 54(5):931–46.e11. doi: 10.1016/j.immuni.2021.03.020
55. Kawakami R, Kitagawa Y, Chen KY, Arai M, Ohara D, Nakamura Y, et al. Distinct Foxp3 Enhancer Elements Coordinate Development, Maintenance, and Function of Regulatory T Cells. Immunity (2021) 54(5):947–61.e8. doi: 10.1016/j.immuni.2021.04.005
56. Dardalhon V, Awasthi A, Kwon H, Galileos G, Gao W, Sobel RA, et al. IL-4 Inhibits TGF-Beta-Induced Foxp3+ T Cells and, Together With TGF-Beta, Generates IL-9+ IL-10+ Foxp3(-) Effector T Cells. Nat Immunol (2008) 9(12):1347–55. doi: 10.1038/ni.1677
57. Nakatsukasa H, Zhang D, Maruyama T, Chen H, Cui K, Ishikawa M, et al. The DNA-Binding Inhibitor Id3 Regulates IL-9 Production in CD4(+) T Cells. Nat Immunol (2015) 16(10):1077–84. doi: 10.1038/ni.3252
58. Tu L, Chen J, Zhang H, Duan L. Interleukin-4 Inhibits Regulatory T Cell Differentiation Through Regulating Cd103+ Dendritic Cells. Front Immunol (2017) 8:214. doi: 10.3389/fimmu.2017.00214
59. Owen DL, Sjaastad LE, Farrar MA. Regulatory T Cell Development in the Thymus. J Immunol (2019) 203(8):2031–41. doi: 10.4049/jimmunol.1900662
60. Wu Y, Borde M, Heissmeyer V, Feuerer M, Lapan AD, Stroud JC, et al. Foxp3 Controls Regulatory T Cell Function Through Cooperation With Nfat. Cell (2006) 126(2):375–87. doi: 10.1016/j.cell.2006.05.042
61. Granucci F, Vizzardelli C, Pavelka N, Feau S, Persico M, Virzi E, et al. Inducible IL-2 Production by Dendritic Cells Revealed by Global Gene Expression Analysis. Nat Immunol (2001) 2(9):882–8. doi: 10.1038/ni0901-882
62. Owen DL, Mahmud SA, Vang KB, Kelly RM, Blazar BR, Smith KA, et al. Identification of Cellular Sources of IL-2 Needed for Regulatory T Cell Development and Homeostasis. J Immunol (2018) 200(12):3926–33. doi: 10.4049/jimmunol.1800097
63. Hemmers S, Schizas M, Azizi E, Dikiy S, Zhong Y, Feng Y, et al. IL-2 Production by Self-Reactive CD4 Thymocytes Scales Regulatory T Cell Generation in the Thymus. J Exp Med (2019) 216(11):2466–78. doi: 10.1084/jem.20190993
64. Hara T, Shitara S, Imai K, Miyachi H, Kitano S, Yao H, et al. Identification of IL-7-Producing Cells in Primary and Secondary Lymphoid Organs Using IL-7-GFP Knock-in Mice. J Immunol (2012) 189(4):1577–84. doi: 10.4049/jimmunol.1200586
65. Cui G, Hara T, Simmons S, Wagatsuma K, Abe A, Miyachi H, et al. Characterization of the IL-15 Niche in Primary and Secondary Lymphoid Organs In Vivo. Proc Natl Acad Sci USA (2014) 111(5):1915–20. doi: 10.1073/pnas.1318281111
66. Klein L, Kyewski B, Allen PM, Hogquist KA. Positive and Negative Selection of the T Cell Repertoire: What Thymocytes See (and Don’t See). Nat Rev Immunol (2014) 14(6):377–91. doi: 10.1038/nri3667
67. Ohkura N, Kitagawa Y, Sakaguchi S. Development and Maintenance of Regulatory T Cells. Immunity (2013) 38(3):414–23. doi: 10.1016/j.immuni.2013.03.002
68. Aschenbrenner K, D’Cruz LM, Vollmann EH, Hinterberger M, Emmerich J, Swee LK, et al. Selection of Foxp3+ Regulatory T Cells Specific for Self Antigen Expressed and Presented by Aire+ Medullary Thymic Epithelial Cells. Nat Immunol (2007) 8(4):351–8. doi: 10.1038/ni1444
69. Cowan JE, Parnell SM, Nakamura K, Caamano JH, Lane PJ, Jenkinson EJ, et al. The Thymic Medulla is Required for Foxp3+ Regulatory But Not Conventional CD4+ Thymocyte Development. J Exp Med (2013) 210(4):675–81. doi: 10.1084/jem.20122070
70. Chen W, Jin W, Hardegen N, Lei KJ, Li L, Marinos N, et al. Conversion of Peripheral CD4+CD25- Naive T Cells to CD4+CD25+ Regulatory T Cells by TGF-Beta Induction of Transcription Factor Foxp3. J Exp Med (2003) 198(12):1875–86. doi: 10.1084/jem.20030152
71. Chen W, Ten Dijke P. Immunoregulation by Members of the Tgfbeta Superfamily. Nat Rev Immunol (2016) 16(12):723–40. doi: 10.1038/nri.2016.112
72. Marie JC, Letterio JJ, Gavin M, Rudensky AY. TGF-Beta1 Maintains Suppressor Function and Foxp3 Expression in CD4+CD25+ Regulatory T Cells. J Exp Med (2005) 201(7):1061–7. doi: 10.1084/jem.20042276
73. Li MO, Sanjabi S, Flavell RA. Transforming Growth Factor-Beta Controls Development, Homeostasis, and Tolerance of T Cells by Regulatory T Cell-Dependent and -Independent Mechanisms. Immunity (2006) 25(3):455–71. doi: 10.1016/j.immuni.2006.07.011
74. Marie JC, Liggitt D, Rudensky AY. Cellular Mechanisms of Fatal Early-Onset Autoimmunity in Mice With the T Cell-Specific Targeting of Transforming Growth Factor-Beta Receptor. Immunity (2006) 25(3):441–54. doi: 10.1016/j.immuni.2006.07.012
75. Josefowicz SZ, Niec RE, Kim HY, Treuting P, Chinen T, Zheng Y, et al. Extrathymically Generated Regulatory T Cells Control Mucosal Th2 Inflammation. Nature (2012) 482(7385):395–9. doi: 10.1038/nature10772
76. Zheng Y, Josefowicz S, Chaudhry A, Peng XP, Forbush K, Rudensky AY. Role of Conserved non-Coding DNA Elements in the Foxp3 Gene in Regulatory T-Cell Fate. Nature (2010) 463(7282):808–12. doi: 10.1038/nature08750
77. Konkel JE, Zhang D, Zanvit P, Chia C, Zangarle-Murray T, Jin W, et al. Transforming Growth Factor-Beta Signaling in Regulatory T Cells Controls T Helper-17 Cells and Tissue-Specific Immune Responses. Immunity (2017) 46(4):660–74. doi: 10.1016/j.immuni.2017.03.015
78. Ouyang W, Beckett O, Ma Q, Li MO. Transforming Growth Factor-Beta Signaling Curbs Thymic Negative Selection Promoting Regulatory T Cell Development. Immunity (2010) 32(5):642–53. doi: 10.1016/j.immuni.2010.04.012
79. Chen W, Jin W, Tian H, Sicurello P, Frank M, Orenstein JM, et al. Requirement for Transforming Growth Factor Beta1 in Controlling T Cell Apoptosis. J Exp Med (2001) 194(4):439–53. doi: 10.1084/jem.194.4.439
80. Tone Y, Furuuchi K, Kojima Y, Tykocinski ML, Greene MI, Tone M. Smad3 and NFAT Cooperate to Induce Foxp3 Expression Through its Enhancer. Nat Immunol (2008) 9(2):194–202. doi: 10.1038/ni1549
81. Ruan Q, Kameswaran V, Tone Y, Li L, Liou HC, Greene MI, et al. Development of Foxp3(+) Regulatory T Cells Is Driven by the C-Rel Enhanceosome. Immunity (2009) 31(6):932–40. doi: 10.1016/j.immuni.2009.10.006
82. Schlenner SM, Weigmann B, Ruan Q, Chen Y, von Boehmer H. Smad3 Binding to the Foxp3 Enhancer is Dispensable for the Development of Regulatory T Cells With the Exception of the Gut. J Exp Med (2012) 209(9):1529–35. doi: 10.1084/jem.20112646
83. Surh CD, Sprent J. T-Cell Apoptosis Detected in Situ During Positive and Negative Selection in the Thymus. Nature (1994) 372(6501):100–3. doi: 10.1038/372100a0
84. Fontenot JD, Dooley JL, Farr AG, Rudensky AY. Developmental Regulation of Foxp3 Expression During Ontogeny. J Exp Med (2005) 202(7):901–6. doi: 10.1084/jem.20050784
85. Chen W, Frank ME, Jin W, Wahl SM. TGF-Beta Released by Apoptotic T Cells Contributes to an Immunosuppressive Milieu. Immunity (2001) 14(6):715–25. doi: 10.1016/s1074-7613(01)00147-9
86. Perruche S, Zhang P, Liu Y, Saas P, Bluestone JA, Chen W. Cd3-Specific Antibody-Induced Immune Tolerance Involves Transforming Growth Factor-Beta From Phagocytes Digesting Apoptotic T Cells. Nat Med (2008) 14(5):528–35. doi: 10.1038/nm1749
87. Fadok VA, Bratton DL, Konowal A, Freed PW, Westcott JY, Henson PM. Macrophages That Have Ingested Apoptotic Cells In Vitro Inhibit Proinflammatory Cytokine Production Through Autocrine/Paracrine Mechanisms Involving TGF-Beta, PGE2, and PAF. J Clin Invest (1998) 101(4):890–8. doi: 10.1172/JCI1112
88. Travis MA, Sheppard D. TGF-Beta Activation and Function in Immunity. Annu Rev Immunol (2014) 32:51–82. doi: 10.1146/annurev-immunol-032713-120257
89. Shi M, Zhu J, Wang R, Chen X, Mi L, Walz T, et al. Latent TGF-Beta Structure and Activation. Nature (2011) 474(7351):343–9. doi: 10.1038/nature10152
90. Barcellos-Hoff MH, Dix TA. Redox-Mediated Activation of Latent Transforming Growth Factor-Beta 1. Mol Endocrinol (1996) 10(9):1077–83. doi: 10.1210/mend.10.9.8885242
91. Zhang D, Jin W, Wu R, Li J, Park SA, Tu E, et al. High Glucose Intake Exacerbates Autoimmunity Through Reactive-Oxygen-Species-Mediated Tgf-Beta Cytokine Activation. Immunity (2019) 51(4):671–81.e5. doi: 10.1016/j.immuni.2019.08.001
92. Zhang D, Chia C, Jiao X, Jin W, Kasagi S, Wu R, et al. D-Mannose Induces Regulatory T Cells and Suppresses Immunopathology. Nat Med (2017) 23(9):1036–45. doi: 10.1038/nm.4375
93. Meylan F, Siegel RM. TNF Superfamily Cytokines in the Promotion of Th9 Differentiation and Immunopathology. Semin Immunopathol (2017) 39(1):21–8. doi: 10.1007/s00281-016-0612-y
94. Croft M. The Role of TNF Superfamily Members in T-Cell Function and Diseases. Nat Rev Immunol (2009) 9(4):271–85. doi: 10.1038/nri2526
95. Klinger M, Kim JK, Chmura SA, Barczak A, Erle DJ, Killeen N. Thymic OX40 Expression Discriminates Cells Undergoing Strong Responses to Selection Ligands. J Immunol (2009) 182(8):4581–9. doi: 10.4049/jimmunol.0900010
96. Grinberg-Bleyer Y, Saadoun D, Baeyens A, Billiard F, Goldstein JD, Gregoire S, et al. Pathogenic T Cells Have a Paradoxical Protective Effect in Murine Autoimmune Diabetes by Boosting Tregs. J Clin Invest (2010) 120(12):4558–68. doi: 10.1172/JCI42945
97. Chopra M, Riedel SS, Biehl M, Krieger S, von Krosigk V, Bauerlein CA, et al. Tumor Necrosis Factor Receptor 2-Dependent Homeostasis of Regulatory T Cells as a Player in TNF-Induced Experimental Metastasis. Carcinogenesis (2013) 34(6):1296–303. doi: 10.1093/carcin/bgt038
98. Chen X, Baumel M, Mannel DN, Howard OM, Oppenheim JJ. Interaction of TNF With TNF Receptor Type 2 Promotes Expansion and Function of Mouse CD4+CD25+ T Regulatory Cells. J Immunol (2007) 179(1):154–61. doi: 10.4049/jimmunol.179.1.154
Keywords: tTreg cells, IL-2, IL-15, TGF-β, γc family cytokines, TNF superfamily, TNFRSF
Citation: Tang M, Jia F, Nan F, Zuo F, Yuan Z and Zhang D (2022) Role of Cytokines in Thymic Regulatory T Cell Generation: Overview and Updates. Front. Immunol. 13:883560. doi: 10.3389/fimmu.2022.883560
Received: 25 February 2022; Accepted: 11 March 2022;
Published: 31 March 2022.
Edited by:
Bin Zhao, Central South University, ChinaReviewed by:
Shunqun Luo, National Cancer Institute (NIH), United StatesYan Lu, Third Affiliated Hospital of Sun Yat-sen University, China
Copyright © 2022 Tang, Jia, Nan, Zuo, Yuan and Zhang. This is an open-access article distributed under the terms of the Creative Commons Attribution License (CC BY). The use, distribution or reproduction in other forums is permitted, provided the original author(s) and the copyright owner(s) are credited and that the original publication in this journal is cited, in accordance with accepted academic practice. No use, distribution or reproduction is permitted which does not comply with these terms.
*Correspondence: Dunfang Zhang, izdf@163.com