- 1School of Life Sciences, Yunnan University, Kunming, China
- 2Key Laboratory of the University in Yunnan Province for International Cooperation in Intercellular Communications and Regulations, Yunnan University, Kunming, China
- 3Institute of Biochemistry and Molecular Biology II, Medical Faculty and University Hospital Düsseldorf, Heinrich Heine University Düsseldorf, Düsseldorf, Germany
- 4Department of Microbiology, Immunology and Molecular Genetics, University of Texas Health Science Center, San Antonio, TX, United States
- 5Department of Biochemistry and Structural Biology, University of Texas Health Science Center, San Antonio, TX, United States
Hemichannels (HCs)/gap junctions (GJs) and immunoglobulin (Ig)-like domain-containing proteins (IGLDCPs) are involved in the innate–adaptive immune response independently. Despite of available evidence demonstrating the importance of HCs/GJs and IGLDCPs in initiating, implementing, and terminating the entire immune response, our understanding of their mutual interactions in immunological function remains rudimentary. IGLDCPs include immune checkpoint molecules of the immunoglobulin family expressed in T and B lymphocytes, most of which are cluster of differentiation (CD) antigens. They also constitute the principal components of the immunological synapse (IS), which is formed on the cell surface, including the phagocytic synapse, T cell synapse, B cell synapse, and astrocytes–neuronal synapse. During the three stages of the immune response, namely innate immunity, innate–adaptive immunity, and adaptive immunity, HCs/GJs and IGLDCPs are cross-activated during the entire process. The present review summarizes the current understanding of HC-released immune signaling factors that influence IGLDCPs in regulating innate–adaptive immunity. ATP-induced “eat me” signals released by HCs, as well as CD31, CD47, and CD46 “don’t eat me” signaling molecules, trigger initiation of innate immunity, which serves to regulate phagocytosis. Additionally, HC-mediated trogocytosis promotes antigen presentation and amplification. Importantly, HC-mediated CD4+ T lymphocyte activation is critical in the transition of the innate immune response to adaptive immunity. HCs also mediate non-specific transcytosis of antibodies produced by mature B lymphocytes, for instance, IgA transcytosis in ovarian cancer cells, which triggers innate immunity. Further understanding of the interplay between HCs/GJs and IGLDCPs would aid in identifying therapeutic targets that regulate the HC–Ig-like domain immune response, thereby providing a viable treatment strategy for immunological diseases. The present review delineates the clinical immunology-related applications of HC–Ig-like domain cross-activation, which would greatly benefit medical professionals and immunological researchers alike. HCs/GJs and IGLDCPs mediate phagocytosis via ATP; “eat me and don’t eat me” signals trigger innate immunity; HC-mediated trogocytosis promotes antigen presentation and amplification in innate–adaptive immunity; HCs also mediate non-specific transcytosis of antibodies produced by mature B lymphocytes in adaptive immunity.
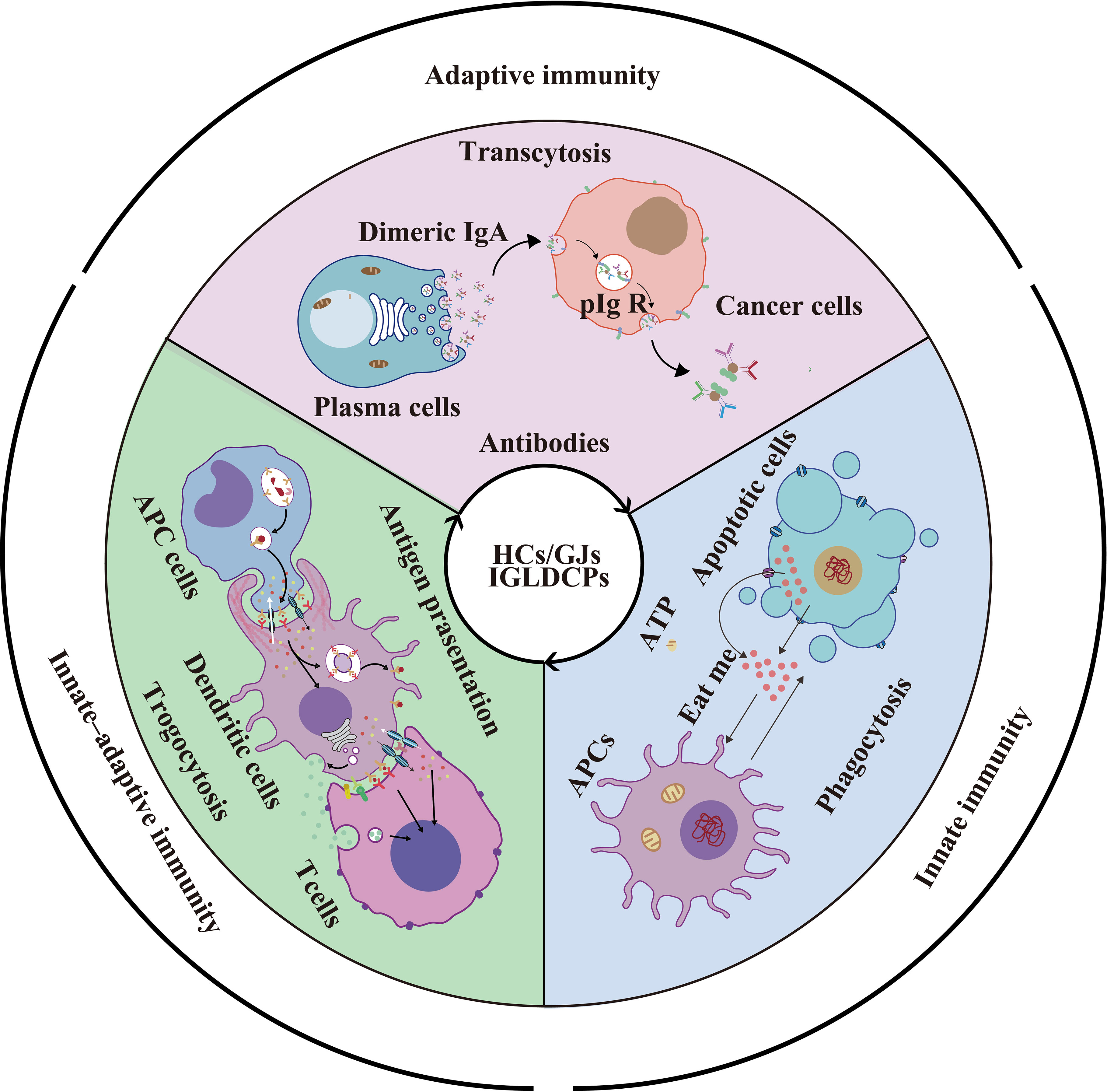
Graphical Abstract HCs/GJs and IGLDCPs mediate phagocytosis via ATP; “eat me and don’t eat me” signals trigger innate immunity; HC-mediated trogocytosis promotes antigen presentation and amplification in innate–adaptive immunity; HCs also mediate non-specific transcytosis of antibodies produced by mature B lymphocytes in adaptive immunity.
Highlights
● Cx43 directly or indirectly interact with at least 20 IGLDCPs
● HCs/Panx release ATP to regulate APCs for initiating innate immunity
● Cx43-GJs between T cells and B cells activate adaptive immunity
● IgA induces APCs to transit adaptive immunity to innate immunity
Introduction
Both types of immune responses, namely innate and adaptive, are linked to immune signal transduction. Hemichannel (HC)/gap junction (GJ)-mediated immune signal transduction in cells triggers an immune response. A similar immunological response is triggered by Ig-like domain-containing proteins (IGLDCPs). Both HCs/GJs and IGLDCPs localize on the immune cell surface to manipulate innate and adaptive immune responses. Previous studies have shown that the cross-activation of HCs/GJs and IGLDCPs is essential in mediating phagocyte migration, inflammation, and fever, among other successive stages of the innate immune response (1–6). In particular, in antigen-presenting cells (APCs), as well as T and B lymphocytes, HCs/GJs and IGLDCPs regulate the adaptive immune response (3, 7–11). However, numerous contentious issues persist, highlighting a potentially important goal: to elucidate the link between HCs/GJs and IGLDCPs in innate–adaptive immunity and provide available evidence on this potentially important topic.
Cell–cell communication during the immune responses confirms that HCs/GJs are closely involved in numerous cellular physiological processes. It is likely that antigen presentation, encompassing the T and B lymphocyte responses, involves in the regulation of cell migration and phagocytosis by pannexins (Panx) and GJ proteins, namely, connexins (Cxs) (12). Cxs form HCs on the cell surface; they can form both independent HCs and two HCs from two neighboring cells dock with each other to form intercellular gap junction channel. Conversely, Panxs form a structure, which is a single plasma membrane channel mediating extracellular communication. Cxs and Panxs are topologically similar with four transmembrane domains, two extracellular loops, one intracellular loop, and one N-terminal and one C-terminal. However, their potential interaction with IGLDCPs remains unclear.
The Ig-like domains are among the most widespread domains. Both sequence and structure of these domains can be found in diverse protein families. Proteins containing an Ig-like domain vary in their tissue distribution, amino acid composition, and biological function. IGLDCPs include immune checkpoint molecules of the immunoglobulin family expressed in T and B lymphocytes, most of which are cluster of differentiation (CD) antigens. The function of immune checkpoint modulators is to regulate immunological responses to infectious agents, foreign tissues, and cancerous cells; furthermore, they act to balance the immune response through either enhancement or inhibition (13–16). However, there are relatively few studies on the regulation of HCs/GJs by IGLDCPs
Although there is limited understanding of the interactive mechanisms between HCs/GJs and IGLDCPs, we have attempted to present a rational and balanced evaluation to bridge this gap. In the present review, several important questions have been raised on the seminal findings. HCs/GJs, which mediate intracellular and extracellular communication, are involved in immune response regulation. The following pertinent questions arise. Do HCs/GJs directly interact with IGLDCPs, including immune checkpoint molecules such as CD antigens, to regulate innate–adaptive immunity? Do HCs/GJs regulate IGLDCPs in T and B lymphocytes? Although both HCs/GJs and IGLDCPs regulate phagocytosis, what is the physical and function relationship between them? How do HCs/GJs and IGLDCPs trigger trogocytosis and transcytosis?
Mutual Cross-Activation of HCs and IGLDCPs
Cxs and Panx1 on the Immunological Cell Surface
Cxs are localized on the cell membrane of at least nine subtypes of APCs, namely, monocytes, macrophages, dendritic cells (DCs), including follicular dendritic cells (FDCs), Kupffer cells, B cells, astrocytes, microglia, neutrophils, and natural killer (NK) cells. Panx1 is also found on the eight subtypes of APCs (Figure 1A). As shown in Figure 1A, Cx43 is expressed in the aforementioned nine APC subtypes. Moreover, Cx37 is expressed on macrophages and neutrophils; Cx45 is present on the surface of DCs and microglial cells; Cx40 on the membrane of B cells and neutrophil cells; and Cx26 on astrocytes. In addition to those listed above, Cx36 and Cx32 are expressed on the microglial cell surface. Seven Cxs, namely, Cx30.3, Cx31.1, Cx32, Cx40, Cx43, Cx45, and Cx46, are present in T cells (4, 17, 18, 27–31). Unexpectedly, 8 of the 21 Cxs in the human gap junction protein family serve as components of synapses or participate in them. There are probably more Cx subtypes that remain to be identified in future research, as most previous studies focused on immune checkpoint molecules without conclusively evaluating Cxs in innate–adaptive immunity. Therefore, elucidating the interaction between HCs and IGLDCPs will offer mechanistic insights into the innate–adaptive immune response. In the following section, we have further detailed the mutual interaction between Cxs and IGLDCPs.
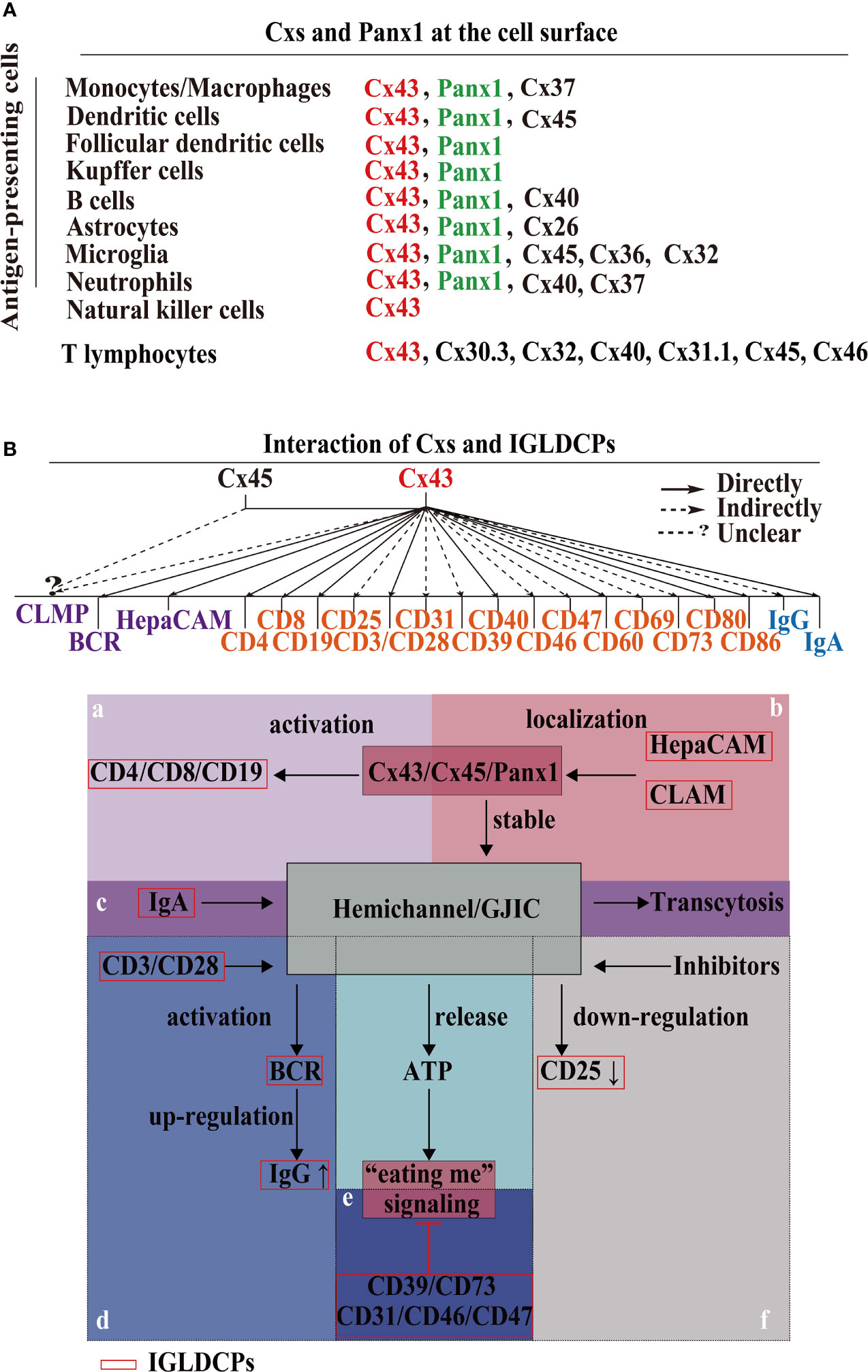
Figure 1 Cxs and Panx1 on the immunological cell surface and their mutual interaction with IGLDCPs. (A) Cxs and Panx1 localize at the cell surface. Cxs and Panx1 HCs have been identified in nine types of APCs and T lymphocytes, primarily for the signaling role of HCs with IGLDCPs in the innate–adaptive immune response (17, 18). (B) The mutual interaction of HCs and IGLDCPs. Cx43, which interacts with at least 20 IGLDCPs: a The activation of CD4/CD8/CD19 requires Cx43/HC (19–21). b HepaCAM and CLAM facilitates Cx43 membrane localization and GJIC establishment (22–24). c GJIC mediates the transcytosis of IgA in CD19+ B cells (21). d CD3/CD28/CD40 activate BCR signaling and upregulate IgG expression by Cx43/HC opening (25). e The “eating me” signaling pathway is inhibited by CD39/CD73/CD31/CD46/CD47 (14, 26). f CD25/69 are downregulated by the inhibition of Cx43/HC (3). Cxs, connexins; Panx, pannexin; IGLDCP, Ig-like domain-containing protein; HC, hemichannel; APC, antigen-presenting cell.
Mutual Interaction of Cxs and IGLDCPs
Cx43 interacts extensively with at least 20 IGLDCPS, either directly or indirectly, namely, CLMP, BCR, HepaCAM, CD4, CD8, CD19, CD25, CD3/CD28, CD31, CD39, CD40, CD46, CD47, CD60, CD69, CD73, CD80, CD86, IgG, and IgA (Figure 1B). Cx43 regulates T lymphocytes and DCs via IGLDCPs. Cx43/Cx40 maintains lymphocyte homeostasis and cytokine production, such as in the case of Cx43 HC inhibition, which suppresses IL-2 and IL-6 mRNA expression (32). Furthermore, the use of mimic peptides as an inhibitor of Cx43 HCs downregulated CD69 and CD25 activation in T cells, and led to IFN-γ by release by DC-stimulated NK cells (3). Similarly, CD3/CD28 induced ATP release by γδT cells, aided by HCs, resulting in cell activation (33, 34). Furthermore, Cx43 HCs in the plasma membrane of CD4+ T lymphocytes establish gap junction intracellular communication (GJIC) with macrophages to synthesize and secrete Igs and cytokines in immune regulation (19). Similarly, in DC–DC interaction, CD80, CD 86, and MHC class II are expressed (33, 35). Directly, Cx43 activates spleen cells and facilitates IgG production. Targeting Cx43 is a potential strategy to treat diseases associated with the antibody response (14). Cx43 regulates B lymphocyte activation directly, through BCR signaling, which involves migration and motility (7, 36).
The IGLDCPs regulate Cx43 HCs/GJs. The Ig-like domain in hepatocyte cell adhesion molecule (hepaCAM) stabilizes the Cx43 HCs on the cell surface. The hepaCAM gene was first described in hepatocellular carcinoma and was also discovered in the central nervous system (CNS); it is named GlialCAM, based on the site of its identification (37). HepaCAM is reportedly a member of the immunoglobulin superfamily (IgSF); it consists of an extracellular domain with two Ig loops, a transmembrane region, and a cytoplasmic tail, and functions in conjunction with Cx43 (38). In previous studies in U373 MG glioblastoma cells studies, it was found that hepaCAM expression redistributes Cx43, especially to the site of cell–cell contact, where co-localization of the two molecules is detected (38). Furthermore, altering the Ig-like domain of hepaCAM, especially the first extracellular IGLDCP reduces the co-localization of intercellular Cx43. Cx43 is shuttled back to the cytoplasm from the cell membrane, consequently decreasing its membrane-bound expression. In summary, the presence of IGLDCPs stabilizes Cx43 expression and promotes transport of a protein localized in the cytoplasm to the cell surface (22, 23). Additionally, CAR-like membrane protein (CLMP) regulates Cx43 and Cx45, and the absence of CLMP causes functional obstruction due to a lack of GJIC (24). Cxs and IGLDCPs are co-localized and interact at the immunological synapses (ISs). An increased intracellular Ca2+ level, which induces T cell activation and signal amplification, is facilitated by IS Cx43 HCs. Therefore, the formation of ISs is an important function structure, which allows us to understand how HCs/GJs and IGLDCP collaboratively modulate the precise roles in innate–adaptive immune responses.
HCs/GJs and IGLDCPs Interact to Form the Synapse
Importantly, HCs/GJs are complex signaling components of the ISs (39, 40)—phagocytic synapse, T cell synapse, B cell synapse and astrocyte–neuronal synapses. First, HCs are involved in phagocytic synapse formation between APCs and pathogens (41) (Figure 2A). Cx HC-linked “pathologic pores” are involved in spreading injury and perpetuating chronic disease. Opening HCs are involved in spinal cord injury progression and the spread of cellular edema. They also control important aspects of the innate–adaptive immune response, particularly under chronic disease conditions, as well as the initiation and perpetuation of the inflammasome pathway in astrocytes (45). It has been reported that Cx43 also regulates FDC development (46), implying Cx43 may form a phagocytic synapse and perform important functions, which warrant further research. Second, the T cell synapse contains GJs. GJIC established by Cx43 is an important functional component of the T cell synapse (41) (Figure 2B); it also activates T cells by sustaining the communication between T cells and APCs (11, 19, 47–49). Furthermore, in melanoma cancer cells, Cx43 GJIC plaques localized at the IS are required for augmenting granzyme B activity, to enable cytotoxic T lymphocytes (CTLs) to kill B16F19 melanoma cells (50). It has been reported that Cx43 GJIC between DCs and also activated T cells (51). These findings confirmed that Cx43 plays a vital role in the T cell synapse. Additionally, Cx43-forming HCs/GJs activate the T cell IS (25). Third, the B cell synapse is formed between B cells and APCs (42) (Figure 2C). However, compared with phagocytosis and T cell synapses, data on B cell synapse are rather limited. These findings demonstrated that the HCs/GJs are an intrinsic part of the ISs and are essential to mediate IS intracellular communication in regulating the delivery of immune factors.
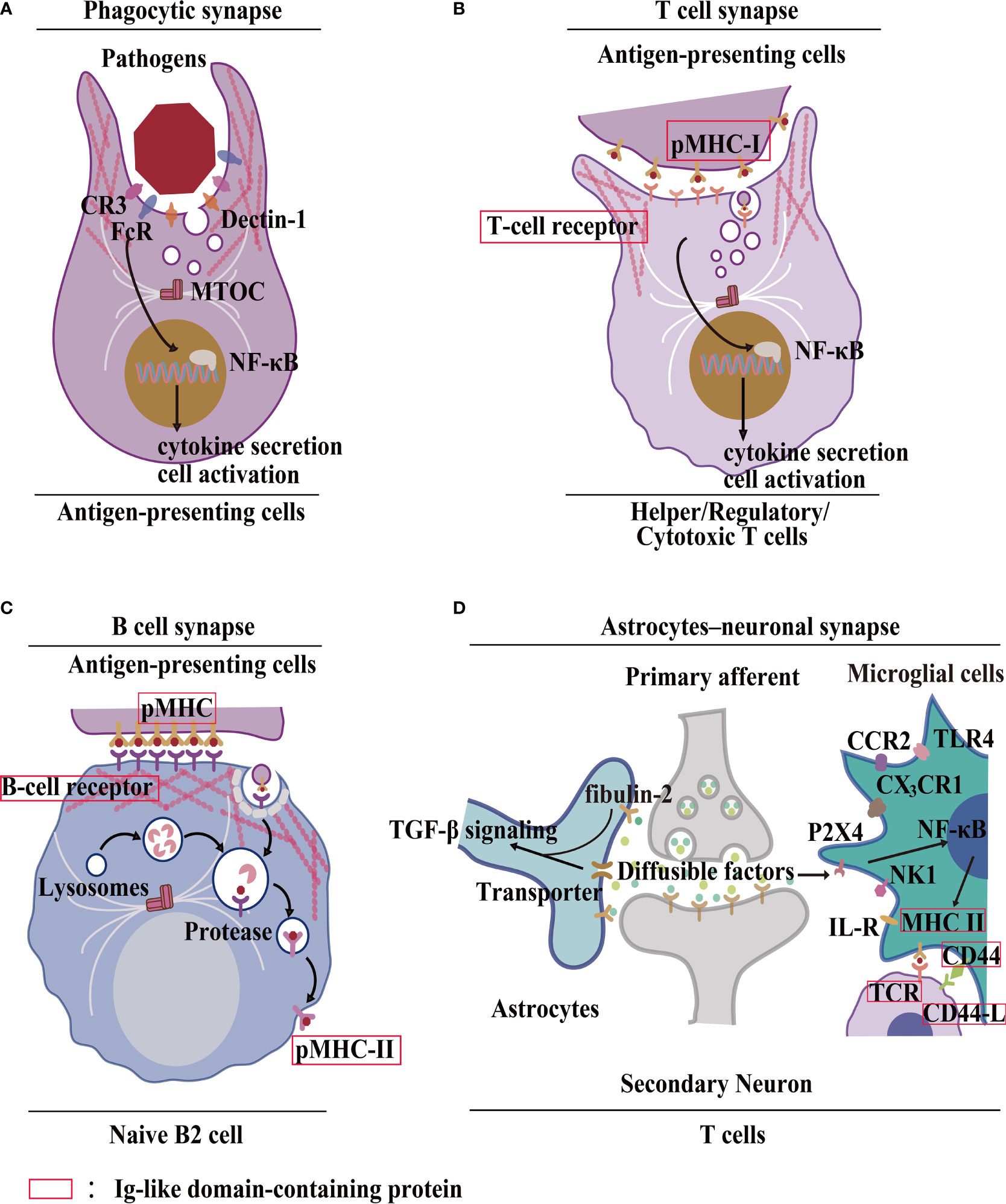
Figure 2 Formation of the IS by HC–IGLDCP interaction. (A) A phagocytic synapse formed by APCs. Phagocytes possess specific molecules on the synaptic surface that trigger phagocytosis. For example, recognition of Fcy receptor (FcR) sites, CR3 envelope site, Dectin-1 receptor trigger site (41). MTOC: Center for Microtubule Organization. (B) T-cell immunological synapse. A specific cellular contact between T cells and APCs. Major histocompatibility complex (pMHC-I) molecules on the surface of APCs bind to the T-cell receptor (TCR) and deliver the antigen, leading to the polarization of APCs by T cells and the coordinated recombination of various components of T cells, including signaling molecules and adhesion molecules, actin and microtubule cytoskeleton (41). (C) B-cell immunological synapse. A specific cellular contact between B cells and APCs. The pMHC-I molecules are phagocytosed in a clathrin-dependent manner. The antigens are transported to lysosomal vesicles for digestion, and the resulting peptides are loaded onto MHC-II molecules and transported back to the cell surface for presentation to T cells (42). (D) Neuronal Synapse. It consists of neurons, astrocytes, microglia, and T cells. Astrocyte-derived extracellular vesicles promote synapse formation through fibrin 2-mediated TGF-β signaling. Microglia MHC-II protein, CD40, and other stimulating molecules recruit T cells to deliver antigens. Different receptors bind to different ligands (43, 44). HC, hemichannel; IGLDCP, Ig-like domain-containing protein; Cx, connexin; Panx, pannexin; APC, antigen-presenting cell.
The astrocyte–neuronal synapse is established between neurons and astrocytes, which release diffusible factors to activate microglia via NF-κB signaling (41, 52–54). Astrocytes interact with neuronal synapses to establish astrocyte–neuronal communication (55). Research has shown that astrocyte-derived extracellular vesicles promote synaptic formation through fibrin 2-mediated TGF-β signaling. Consequently, microglia MHC-II protein, CD44, and other molecules recruit T cells for effective antigen delivery (43, 44) (Figure 2D). Investigating the interaction between HCs/GJs and IGLDCPs in the astrocyte–neuronal synapse presents a worthwhile research opportunity. Interactions of HCs/GJs and IGLDCPs with IS provide direct evidence suggesting that both may play an important role in immune responses.
Cx/Panx and IGLDCPs Display Dual Functions in Innate Immunity
ATP “Eat Me” Signaling, as Well as CD31, CD46, CD47 “Don’t Eat Me” Signal Molecules, Triggers Phagocytosis
Panx1 releases ATP from apoptotic cells to trigger an “eat me” signal (56) (Figures 3A–C). Key phagocytic inducers, ATP and UTP, have been confirmed to recruit apoptotic cells in vitro and in vivo. In contrast, “don’t eat-me” signals comprise CD31, CD46, and CD47 expression. These signals on healthy viable cells, which are capable of phosphatidylyserine (PtdSer) exposure under physiological conditions, may positively inhibit phagocytic uptake (26). These findings elucidate the mechanism governing HC–IGLDCP interaction in phagocytosis.
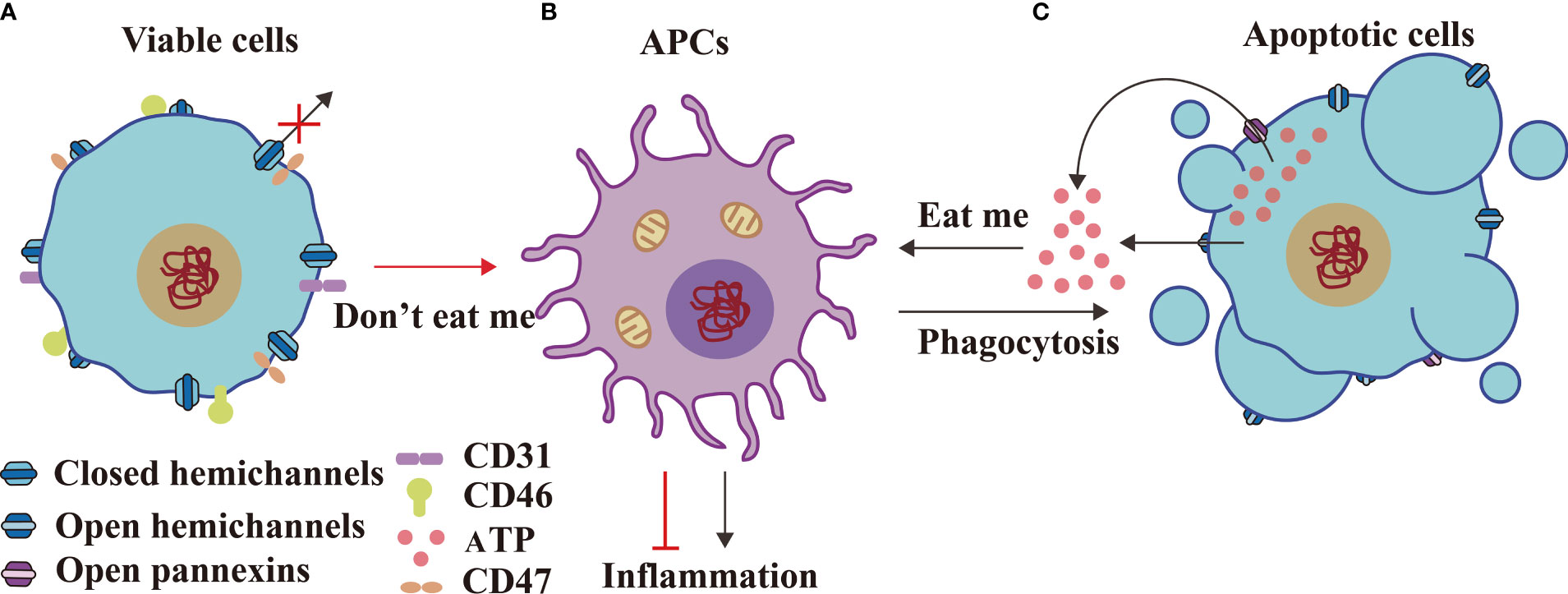
Figure 3 HCs and IGLDCPs display dual functions in innate immunity. Viable cells form closed HCs (A) with APCs (B) transmitting “don’t eat me” signals, including CD31, CD47, and CD46, to suppress inflammation. In contrast, apoptotic cells (C) send an “eat me” signal to APCs by opening the HCs and releasing ATP, which engulfs apoptotic cells and causes inflammation (56). HC, hemichannel; IGLDCP, Ig-like domain-containing protein; APC, antigen-presenting cell.
In the macrophage cell line J774, Cx43 RNAi showed impaired phagocytosis of the polystyrene-covered beads, and sheep erythrocytes opsonized by IgG (47); in contrast, in sheep erythrocytes with heterozygously or homozygously deleted Cx43, no changes were observed in phagocytosis (12, 57). Recently, Dosch et al. assessed Cx43 function in phagocytosis using Cx43 deletion and inhibition. It was determined that the inhibition of autocrine communication of Cx43-dependent ATP in macrophages improved sepsis outcome (33, 48, 49). Therefore, full expression of intact Cx43 is essential in regulating of the immune response through the directionality and rate of DC migration (58). Different cytokines regulate intercellular communication, facilitated by HCs/GJs in APCs, to execute purinergic signaling (3). This presents an interesting research opportunity for further investigation of Cx43-macrophage-phagocytosis. Apoptotic cells attract phagocytes by releasing chemotactic factors known as “find-me” signals (26).
Cx/Panx and IGLDCPs Regulate the Inflammatory Response
Cumulative evidence shows that ATP triggers the inflammatory response. Cx HCs serve as a major pathway for the release of cytoplasmic ATP into the extracellular space. In granulocytes, Cxs enhance the inflammatory responses and promote cellular activation (33) (Figure 4B). For example, ATP released by Panx1 promotes the opening of Cx43 HCs (62) and is also involved in the innate immune response and inflammation (26, 63–70). In contrast, the blockage of Cx43 isoform HCs alleviates inflammation and enhances healing (2). In other inflammatory pathologies, Cx43 expression regulates monocyte–endothelial adhesion, with criteria for baseline adhesion set by Cx43-expressing monocytes (71). Similarly, elevated macrophage Cx43 HC activation and Panx1 expression inhibit pathogenesis (1, 49). Cx43 GJs transfer hypoxia-induced miR-192-5p, allowing cancer cells to acquire immune-resistant phenotypes (25). During inflammation in response to spinal cord injury, a decrease in the expression of Cx43 proteins shortens animal recovery time (33). ATP release has been inhibited using several Cx43 mimic peptides, thereby influencing the inflammatory process (72). Therefore, ATP integrated with the HC function promotes inflammation.
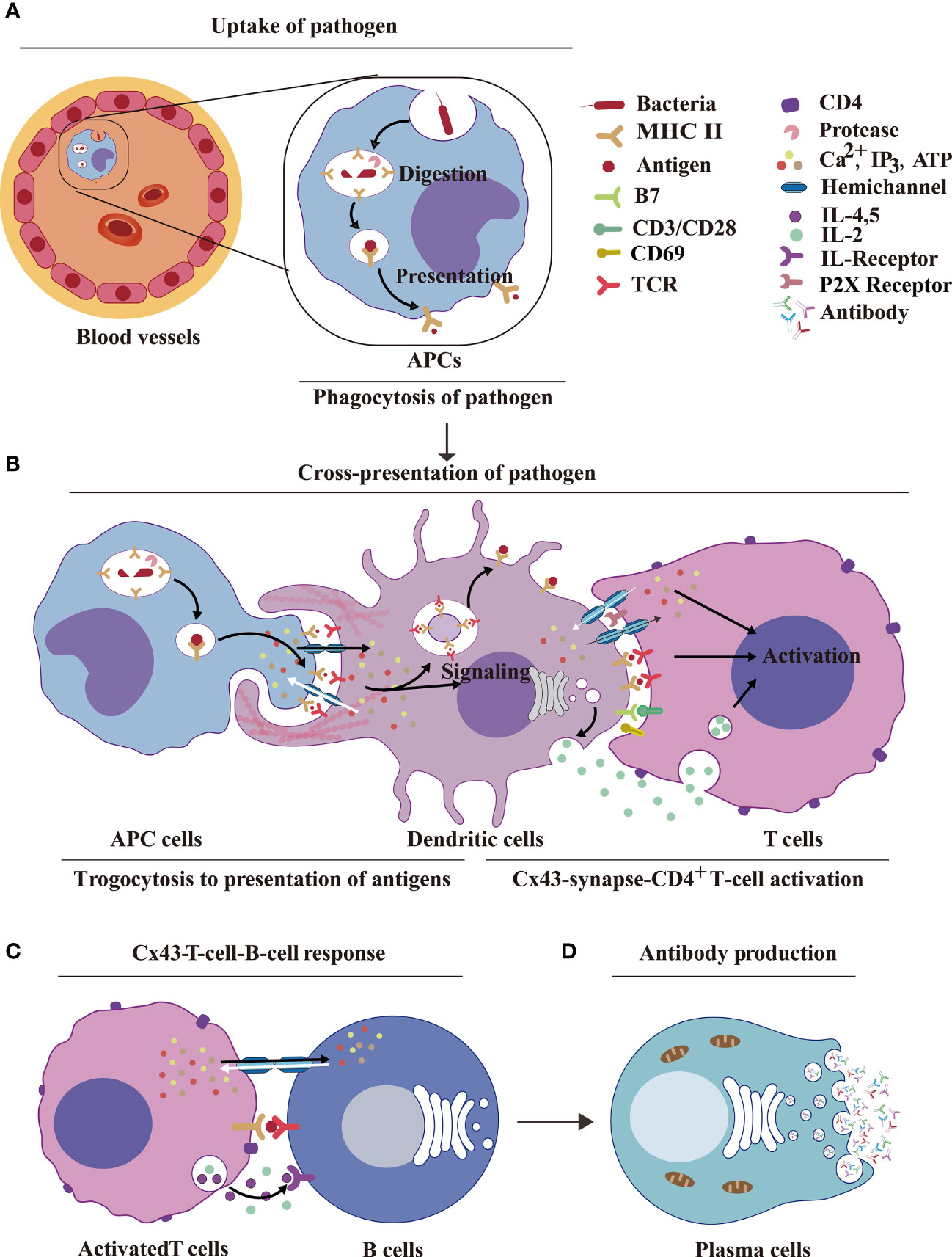
Figure 4 HCs and IGLDCPs activate innate–adaptive immunity. (A) Uptake of pathogens by APCs. The pathogenic antigens are phagocytosed by APCs and digested by proteases to form peptides, which are then transmitted by MHC molecules to the cell surface by phagocytosis. (B) Dendritic cell Cx43s are dependent on trogocytosis for antigen delivery to T cells. The antigen is processed by APCs and binds to MHC receptors on the APC membrane. The HCs and IGLDCPs in innate–adaptive immunity recognize and remember specific pathogens to trigger immunity. The former facilitates ATP release and autocrine feedback mechanisms that control Ca2+ entry. GJs between monocytes and CD8+ DCs transfer antigens via Cx43-synapse-CD4+ T cells (59, 60). (C) Cx43-T-cell–B-cell response. The activated T cells facilitate the opening of HCs, which liberally release ATP into B cells. This results in the simultaneous release of IL-2, IL-4, and IL-5, which act on the IL-R receptor and further stimulate B cells to produce antibodies. (D) Antibody production. Activated B cells form plasma cells, which produce antibodies (61). HC, hemichannel; IGLDCP, Ig-like domain-containing protein; APC, antigen-presenting cell; MHC, major histocompatibility complex; DC, dendritic cell.
Open Cxs HCs in macrophages facilitate an effective immune response. GJs and HCs help spread toxicity into neighboring areas to augment viral/bacterial replication, and promote the spread of the inflammatory response by infectious agents, such as HIV (33),. Ig-like domains presenting T cell immunoglobulin molecules regulate inflammation and immune responses (16, 73). Single immunoglobulin IL-1R-related molecule (SIGIRR) is a specific inhibitor of IL-1R and toll-like receptor signals (74, 75).
In summary, Cxs HCs and Panx1 release ATP, which serves as an “eat me” signal; conversely, CD31, CD47, and CD46 function as “don’t eat-me” signals, which regulate phagocytosis in innate immunity.
Cx43 and IGLDCPs Activate Innate–Adaptive Immunity
Cx43-Dependent Trogocytosis of Macrophages and Dendritic Cells in Antigen Presentation
The interaction between innate and adaptive immune response is defined as innate–adaptive immunity, which is important for antigen presentation. Cx43 contributes to trogocytosis (Figures 4A, B). The mechanism of innate control of adaptive immune responses involves multiple signaling pathways (16). We focused on how macrophages detect pathogens or injured cells. Trogocytosis is a process whereby lymphocytes extract surface molecules of APCs and express them on their own membranes (59, 60) (Figure 4B). However, the role of HCs and IGLDCPs in trogocytosis has not been examined adequately. Cx43-deleted macrophages are more proficient in T cell priming, implying an increased accumulation of antigens as these macrophages cannot transfer them to neighboring DCs, resulting in efficient presentation (57, 76–80). These findings delineate a potential mechanism by which HCs and IGLDCPs regulate antigen presentation.
GJs also have a pivotal function in DC activation and the amplification of antigen presentation, such as antigen transport, dendritic activation, and antigen cross-presentation (10, 33, 81–83). GJs-mediated antigen transfer between monocytes and CD8+DCs may serve as a simple and efficacious immunotherapy strategy for cancers, such as in the case of undifferentiated monocytes loaded with tumor antigen (20). Molecules containing Ig-like domains, such as pMHC-I and II, are involved in Cx43-dependent trogocytosis on the surface of acceptor cells (78). This is a valuable research direction to explore the underlying mechanism by which Cxs and IGLDCPs regulate antigen presentation via trogocytosis.
Cx43/Panx-Mediated Activation of CD4+ T Lymphocytes
In addition to the roles of Cx43 in regulating macrophages and dendritic cells. HCs also mediate CD4+ T lymphocyte activation is critical in the transition of the innate immune response to adaptive immunity (Figure 4C). In a previous study, it was found that Cx43 in the IS delivers microRNAs from hypoxic melanoma cells to CTLs (25). Therefore, Cx43 stimulates T lymphocytes by the delivery of immune factors. Cx43 is involved in the formation of GJs in CD4+ T lymphocytes, Th0, Th1, and Th2, and macrophages; this pathway was found to be especially prominent in Cx43-Th1–macrophage interaction (19). This, in turn, suggests the potential capability of HCs in controlling IGLDCP activation. Cx43-GJs at the IS between DCs and CD4+ T cells promote T cell activation during antigen presentation (11), whereas the inhibition of GJs hindered DC-mediated T cell activation, reflected by lower T cell proliferation, CD69 expression, and IL-2 secretion.
Interestingly, in the absence of DCs, Cx43 GJ blockers did not affect the activation of CD4+ T cells triggered by anti-CD3/anti-CD28. Therefore, it was inferred that suppression of Cx43 inhibits Cx43 GJ assembly between DCs and T cells, resulting in T cell inactivation (84). In the DC–T cell IS, the blocking of Cx43 HCs/GJs (on either DCs or T cells) inhibited IFN-γ secretion and decreased the intracellular Ca2+ concentration, upon interaction of T cells with antigen-loaded DCs. These results strongly suggested that Cx43 HCs act in signaling amplification and T cell activation, by either releasing ATP or taking up of inositol triphosphate (IP3) from DCs (25).
Cx43-GJs amplify antigens to activate T lymphocytes via the antigen cross-presentation pathway. In the immune synapse, Panx1, which releases ATP, controls Ca2+ entry to activate T cells; this happens by stimulating autocrine/paracrine receptors, such as P2X1 and P2X4 (85). Cx43-GJs between monocytes and CD8+ DCs transfer antigens (20), whereas Cx43 HCs activate CD4+ T cells (86). Together, Cx43-dependent trogocytosis of macrophages and dendritic cells promote antigen uptake, transfer, and presentation to activate innate–adaptive immunity. The GJ protein Cx43 induces B lymphocytes (8) to produce antibodies in plasma cells (61) (Figure 4D).
Cxs and IGLDCPs Mediate Adaptive Immunity and IgA Transits Adaptive Immunity to Innate Immunity
Cx43-CD39/CD73-Treg-Mediated Immunosuppression
In cellular suppression mechanisms, naturally occurring Treg cells and helper T cells communicate via GJs to deliver cAMP to responder T cells, thereby inhibiting T cell proliferation and IL-2 synthesis (87–89) (Figures 5A–C). In a recent review, it has been reported how the cross-talk between Cxs and cAMP regulates cell-cycle progression, particularly in cancer cell populations (90). Furthermore, Cx43 expressed by thymic Treg cell progenitors supports Treg cell development. Conversely, Cx43 deletion decreased the number of functional Tregs and increased non-functional CD4+CD25+GITR+FOXp3- T cells, which are incapable of producing inflammatory cytokines and inhibiting cancer cell progression (91). In human Treg cells, it has been shown that CD4-mediated activation involves elevation in the intracellular cAMP concentration. In contrast, the decrease in the cAMP level, caused by the application of adenylate cyclase (AC) inhibitor MDL12, resulted in the proliferation of Treg cells, in vitro and in vivo (87, 89) (Figure 5B). Consequently, it is inferred that Cx43 HCs may release cAMP; however, this needs to be studied further.
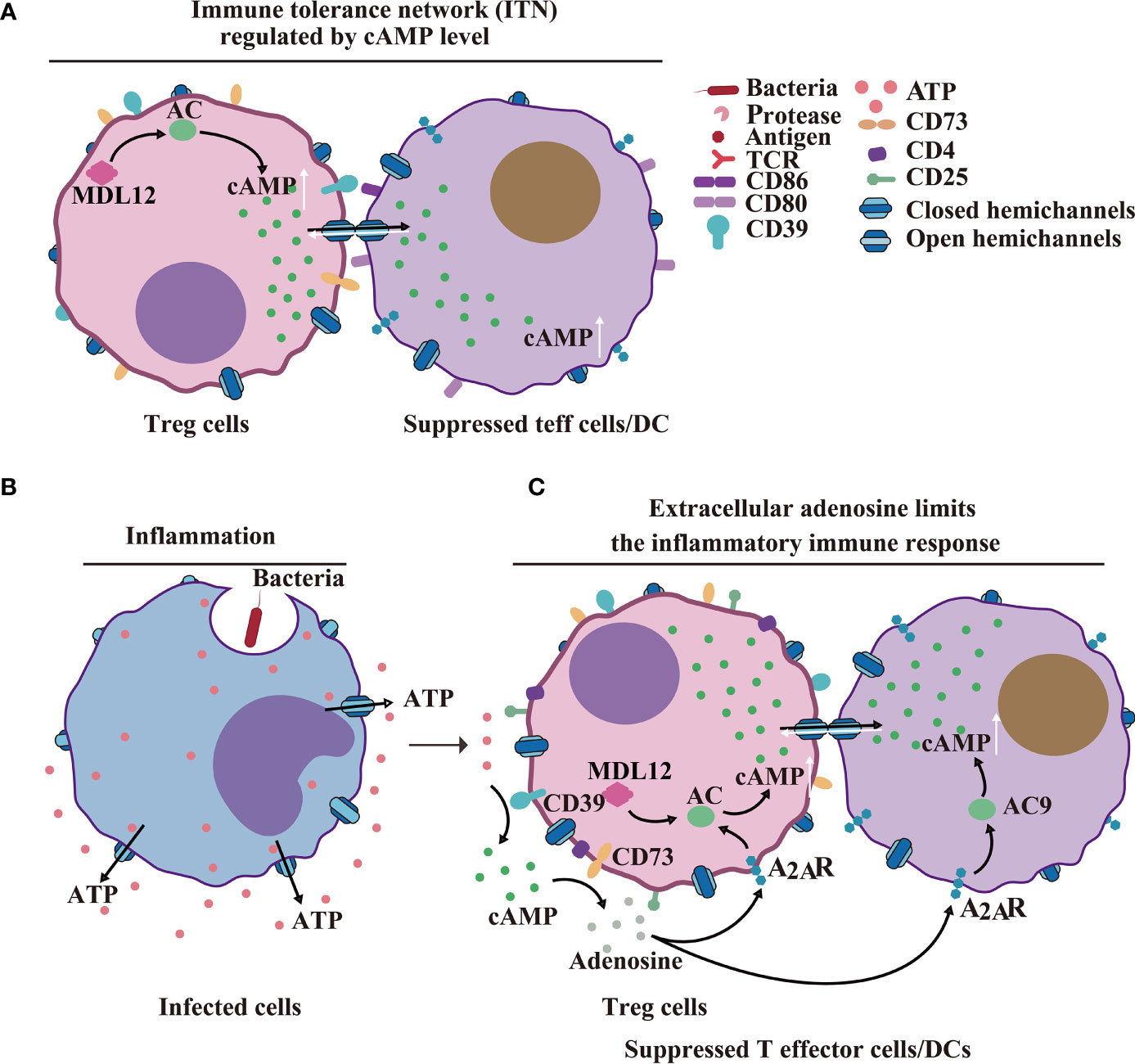
Figure 5 HCs/GJs and IGLDCPs mediate adaptive immunity. (A) Cx43-cAMP cell-mediated immune response. Regulatory T cell-mediated inhibition of naturally occurring Treg cells and conventional T cells delivers cAMP to responder T cells via GJs to inhibit T-cell proliferation and IL-22 synthesis (89). (B) HCs in infected inflammatory cells release ATP. (C) Extracellular adenosine limits the extent of the inflammatory immune response. Activated CD4 + T cells. The activated CD39 + cells release cAMP via paracrine signaling, to suppress T effector (Teff) cell and dendritic cell (DC) functions (89). HC, hemichannel; IGLDCP, Ig-like domain-containing protein.
Cx43-GJs accumulate at the cytotoxic IS, enabling CTL-mediated melanoma cell killing (50). Additionally, Cx43 regulates the proliferation of CD4+CD25+ T lymphocytes and production of cytokines (92). Cx43-GJs regulate CD4+CD25+ Treg lymphocyte activation and inflammatory cytokine (IL-2 and IL-6) production in hypertensive inflammation in the spleen of rats (32, 92).
CD19+ B Cell IgA Transcytosis Transits Adaptive Immunity to Innate Immunity
Recently, it was determined that tumor antigen-specific and tumor antigen-independent IgA transcytosis and antigen regulate ovarian cancer immunity. Tumor B cell-derived IgA binds to the polymeric immunoglobulin IgA receptors (pIgR) on ovarian cancer cells and reprograms myeloid cells against extracellular oncogenic drivers, such as EGFR and KRAS, which causes cell death. In particular, innate immunity triggered by antigen-independent IgA transcytosis is a novel strategy. IgA transcytosis through malignant epithelial cells causes tumor cells to encounter cytotoxic T cells, thereby hampering malignant progression; furthermore, the associated transcription changes result in suppression of the RAS pathway (21). In the ovarian cancer immunological response, IgA, B cells, and atypical B cells are observed (93). Transcytosis is a process in which molecules cross cellular barriers, which includes pinocytosis, endocytosis, and trafficking of vesicles to the opposite membrane (94).
In summary, Cx43-CD39/CD73-Treg-immunosuppression mediates adaptive immunity, specifically, IgA transcytosis, with tumor antigen-dependent and -independent mechanisms. It also regulates the establishment of immunity in ovarian cancer.
Concluding Remarks and Future Perspectives
In conclusion, GJs between two APCs interact with pMHC-1 of phagocytic APCs and TCR of trogocytic APCs to execute antigen delivery (14, 50) during innate immunity (Figure 6A). GJs interact with pMHC-I, B7 from APCs and TCR, CD28 from T cells to facilitate Ca2+-mediated T cell activation (Figure 6B); GJs interact with CD40, pMHC-II from activated T cells and with BCR, CD40-L from activated B cells to stimulate B cell response during innate–adaptive immunity (95) (Figure 6C). The adaptive immunological response involves the generation of antibodies by plasma cells; innate immunity is regulated by IgA transcytosis in ovarian cancer (96) (Figure 6D). The transition from the innate immune response to adaptive immune response involves antigen presentation, followed by T cell activation, and, finally, B cell activation. The immune system is an unitary entity, and its regulation is dependent on a range of complex and diverse factors. HCs and IGLDCPs play an essential role in the three stages of the immune response, namely, innate immunity, innate–adaptive immunity, and adaptive immunity.
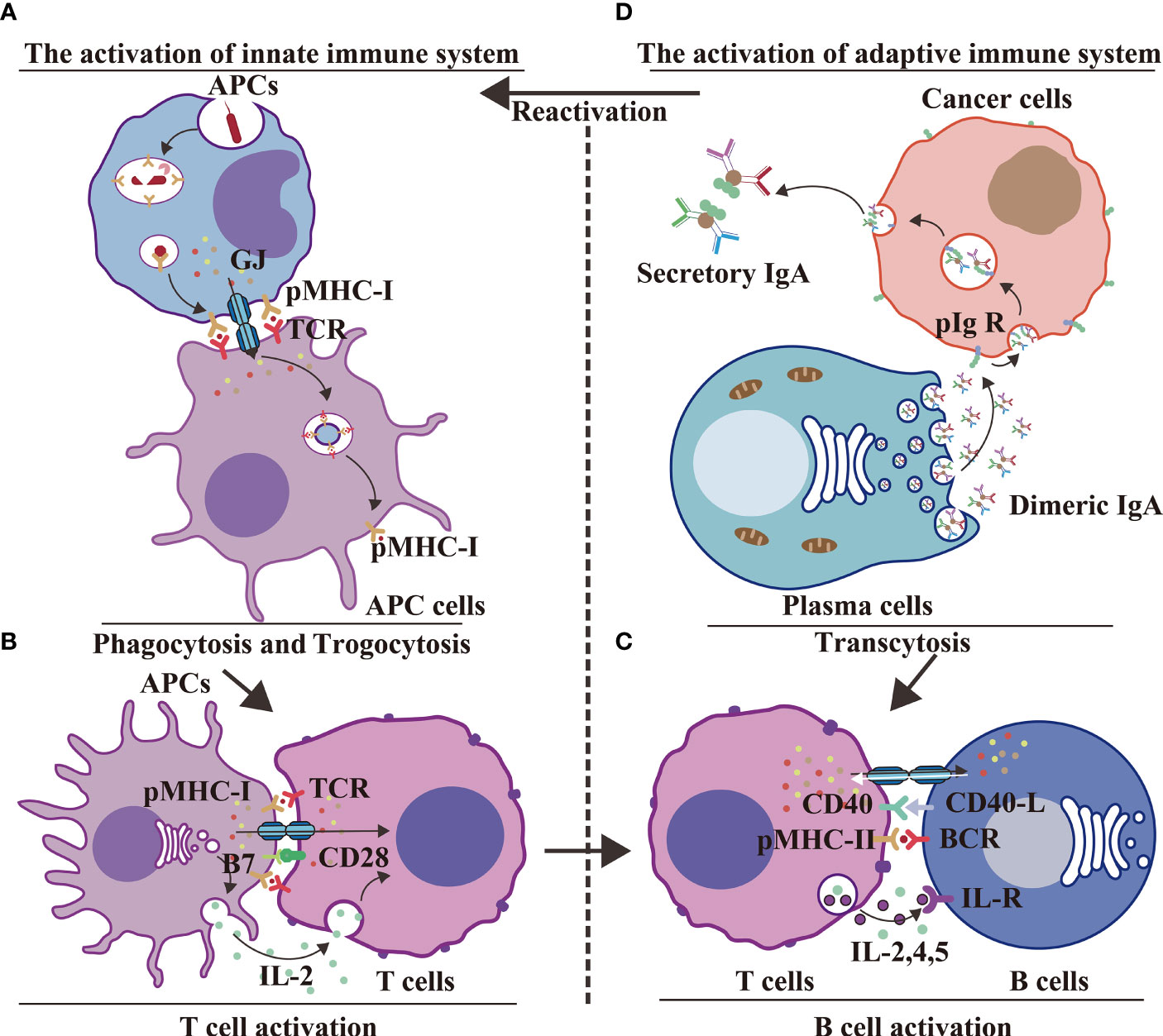
Figure 6 GJs and IGLDCPs regulate phagocytosis, trogocytosis, and transcytosis during innate–adaptive immunity. (A) Innate immunity – antigen production. APCs receive pathogens and form epitopes on the membrane surface through digestion and processing of antigens. Interaction of GJs with IGLDCPs triggers phagocytosis and trogocytosis, thereby resulting in T-cell activation (14, 50). (B) Innate immunity – T-cell activation. Interaction of GJs with IGLDCPs: the former delivers Ca2+ and ATP to T cells, whereas the latter, in contact with CD28 and facilitated by paracrine IL-2 signal transmission, activates T cells. (C) Adaptive immunity – B-cell activation. After CD4+ T-cell activation, pMHC-II establishes contact with the B-cell receptor (BCR). The resultant release of IL-2, IL-4, and IL-5 leads to B-cell activation. The adaptive immune response is jointly mediated by GJs and IGLDCPs (95). (D) Adaptive immunity – Antibodies affect pathogens. GJs activate the adaptive immune response to generate antibodies (D). Conversely, IgA can induce APCs to activate the innate immune response via transcytosis (A) IgA can also promote B-cell activation (C) (96). HC, hemichannel; IGLDCP, Ig-like domain-containing protein; APC, antigen-presenting cell; GJ, gap junction.
In the present review, we have discussed the interactive roles of HCs and IGLDCPs. Our goal is to provide novel insights based on existing concepts, and we believe that this will serve as a foundation for future research. The questions raised in the introductory section of the manuscript have been addressed and the knowledge gaps in the existing literature have been acknowledged. Along this line of investigation, potential clinical and research-related applications would greatly benefit immunological researchers and medical professionals.
Author Contributions
K-JL, J-HM, C-XC, and JXJ structured the manuscript. K-JL, J-HM, C-XC, JXJ, HZ, and MRA wrote the manuscript. C-XC constructed the figures. K-JL, J-HM, and C-XC collected and compiled the references. K-JL, MRA, JXJ, and HZ proofread the manuscript. All authors contributed to the article and approved the submitted version.
Funding
K-JL was supported by the National Natural Science Foundation of China (NSFC) (32160662, 31772225, 31471823, 31260448, 31060251) and the Science and Technology Planning Project in Key Areas of Yunnan Province (202001BB050002). K-JL was also supported by the Donglu Scholar Program of Yunnan University. JXJ was supported by the Welch Foundation (AQ-1507). MRA was supported by the German Federal Ministry of Education and Research (BMBF)—German Network of RASopathy Research (GeNeRARe; grant number: 01GM1902C) and the European Network on Noonan Syndrome and Related Disorders (NSEuroNet; grant number: 01GM1621B).
Conflict of Interest
The authors declare that the research was conducted in the absence of any commercial or financial relationships that could be construed as a potential conflict of interest.
Publisher’s Note
All claims expressed in this article are solely those of the authors and do not necessarily represent those of their affiliated organizations, or those of the publisher, the editors and the reviewers. Any product that may be evaluated in this article, or claim that may be made by its manufacturer, is not guaranteed or endorsed by the publisher.
References
1. Chen W, Zhu S, Wang Y, Li J, Qiang X, Zhao X, et al. Enhanced Macrophage Pannexin 1 Expression and Hemichannel Activation Exacerbates Lethal Experimental Sepsis. Sci Rep (2019) 9(1):160. doi: 10.1038/s41598-018-37232-z
2. Calder BW, Matthew Rhett J, Bainbridge H, Fann SA, Gourdie RG, Yost MJ. Inhibition of Connexin 43 Hemichannel-Mediated Atp Release Attenuates Early Inflammation During the Foreign Body Response. Tissue Eng Part A (2015) 21(11-12):1752–62. doi: 10.1089/ten.TEA.2014.0651
3. Saez PJ, Shoji KF, Aguirre A, Saez JC. Regulation of Hemichannels and Gap Junction Channels by Cytokines in Antigen-Presenting Cells. Mediators Inflammation (2014) 2014:742734. doi: 10.1155/2014/742734
4. Orellana JA, Velasquez S, Williams DW, Sáez JC, Berman JW, Eugenin EA. Pannexin1 Hemichannels Are Critical for Hiv Infection of Human Primary Cd4+ T Lymphocytes. J Leukoc Biol (2013) 94(3):399–407. doi: 10.1189/jlb.0512249
5. Burra S, Nicolella DP, Francis WL, Freitas CJ, Mueschke NJ, Poole K, et al. Dendritic Processes of Osteocytes Are Mechanotransducers That Induce the Opening of Hemichannels. Proc Natl Acad Sci USA (2010) 107(31):13648–53. doi: 10.1073/pnas.1009382107
6. Ni X, Wang A, Zhang L, Shan LY, Zhang HC, Li L, et al. Up-Regulation of Gap Junction in Peripheral Blood T Lymphocytes Contributes to the Inflammatory Response in Essential Hypertension. PloS One (2017) 12(9):e0184773. doi: 10.1371/journal.pone.0184773
7. Machtaler S, Choi K, Dang-Lawson M, Falk L, Pournia F, Naus CC, et al. The Role of the Gap Junction Protein Connexin43 in B Lymphocyte Motility and Migration. FEBS Lett (2014) 588(8):1249–58. doi: 10.1016/j.febslet.2014.01.027
8. Machtaler S, Dang-Lawson M, Choi K, Jang C, Naus CC, Matsuuchi L. The Gap Junction Protein Cx43 Regulates B-Lymphocyte Spreading and Adhesion. J Cell Sci (2011) 124(Pt 15):2611–21. doi: 10.1242/jcs.089532
9. Ring S, Karakhanova S, Johnson T, Enk AH, Mahnke K. Gap Junctions Between Regulatory T Cells and Dendritic Cells Prevent Sensitization of Cd8(+) T Cells. J Allergy Clin Immun (2010) 125(1):237–46. doi: 10.1016/j.jaci.2009.10.025
10. Pang B, Neijssen J, Qiao X, Janssen L, Janssen H, Lippuner C, et al. Direct Antigen Presentation and Gap Junction Mediated Cross-Presentation During Apoptosis. J Immunol (2009) 183(2):1083–90. doi: 10.4049/jimmunol.0900861
11. Elgueta R, Tobar JA, Shoji KF, De Calisto J, Kalergis AM, Bono MR, et al. Gap Junctions at the Dendritic Cell-T Cell Interface Are Key Elements for Antigen-Dependent T Cell Activation. J Immunol (2009) 183(1):277–84. doi: 10.4049/jimmunol.0801854
12. Glass AM, Snyder EG, Taffet SM. Connexins and Pannexins in the Immune System and Lymphatic Organs. Cell Mol Life Sci (2015) 72(15):2899–910. doi: 10.1007/s00018-015-1966-3
13. Flies AS, Blackburn NB, Lyons AB, Hayball JD, Woods GM. Comparative Analysis of Immune Checkpoint Molecules and Their Potential Role in the Transmissible Tasmanian Devil Facial Tumor Disease. Front Immunol (2017) 8:513(513). doi: 10.3389/fimmu.2017.00513
14. Huang Y, Mao Z, Zhang X, Yang X, Sawada N, Takeda M, et al. Connexin43 Is Required for the Effective Activation of Spleen Cells and Immunoglobulin Production. Int J Mol Sci (2019) 20(22):1–15. doi: 10.3390/ijms20225789
15. Köhler N, Ruess DA, Kesselring R, Zeiser R. The Role of Immune Checkpoint Molecules for Relapse After Allogeneic Hematopoietic Cell Transplantation. Front Immunol (2021) 12:634435(535). doi: 10.3389/fimmu.2021.634435
16. Sun L, Wang X, Saredy J, Yuan Z, Yang X, Wang H. Innate-Adaptive Immunity Interplay and Redox Regulation in Immune Response. Redox Biol (2020) 37:101759. doi: 10.1016/j.redox.2020.101759
17. Willebrords J, Crespo Yanguas S, Maes M, Decrock E, Wang N, Leybaert L, et al. Connexins and Their Channels in Inflammation. Crit Rev Biochem Mol Biol (2016) 51(6):413–39. doi: 10.1080/10409238.2016.1204980
18. Takeuchi H, Suzumura A. Gap Junctions and Hemichannels Composed of Connexins: Potential Therapeutic Targets for Neurodegenerative Diseases. Front Cell Neurosci (2014) 8:189. doi: 10.3389/fncel.2014.00189
19. Bermudez-Fajardo A, Ylihärsilä M, Evans WH, Newby AC, Oviedo-Orta E. Cd4+ T Lymphocyte Subsets Express Connexin 43 and Establish Gap Junction Channel Communication With Macrophages in Vitro. J Leukoc Biol (2007) 82(3):608–12. doi: 10.1189/jlb.0307134
20. Huang MN, Nicholson LT, Batich KA, Swartz AM, Kopin D, Wellford S, et al. Antigen-Loaded Monocyte Administration Induces Potent Therapeutic Antitumor T Cell Responses. J Clin Invest (2020) 130(2):774–88. doi: 10.1172/JCI128267
21. Biswas S, Mandal G, Payne KK, Anadon CM, Gatenbee CD, Chaurio RA, et al. Iga Transcytosis and Antigen Recognition Govern Ovarian Cancer Immunity. Nature (2021) 591(7850):464–70. doi: 10.1038/s41586-020-03144-0
22. Wu M, Moh MC, Schwarz H. Hepacam Associates With Connexin 43 and Enhances Its Localization in Cellular Junctions. Sci Rep (2016) 6(1):36218. doi: 10.1038/srep36218
23. Ai XL, Chi Q, Qiu Y, Li HY, Li DJ, Wang JX, et al. Gap Junction Protein Connexin43 Deregulation Contributes to Bladder Carcinogenesis Via Targeting Mapk Pathway. Mol Cell Biochem (2017) 428(1-2):109–18. doi: 10.1007/s11010-016-2921-9
24. Langhorst H, Juttner R, Groneberg D, Mohtashamdolatshahi A, Pelz L, Purfurst B, et al. The Igcam Clmp Regulates Expression of Connexin43 and Connexin45 in Intestinal and Ureteral Smooth Muscle Contraction in Mice. Dis Model Mech (2018) 11(2):1–14. doi: 10.1242/dmm.032128
25. Tittarelli A, Navarrete M, Gleisner MA, Gebicke-Haerter P, Salazar-Onfray F. Connexin-Mediated Signaling at the Immunological Synapse. Int J Mol Med (2020) 21(10):1–16. doi: 10.3390/ijms21103736
26. Poon IK, Lucas CD, Rossi AG, Ravichandran KS. Apoptotic Cell Clearance: Basic Biology and Therapeutic Potential. Nat Rev Immunol (2014) 14(3):166–80. doi: 10.1038/nri3607
27. Araya R, Eckardt D, Maxeiner S, Kruger O, Theis M, Willecke K, et al. Expression of Connexins During Differentiation and Regeneration of Skeletal Muscle: Functional Relevance of Connexin43. J Cell Sci (2005) 118(1):27–37. doi: 10.1242/jcs.01553
28. Gonzalez HE, Eugenin EA, Garces G, Solis N, Pizarro M, Accatino L, et al. Regulation of Hepatic Connexins in Cholestasis: Possible Involvement of Kupffer Cells and Inflammatory Mediators. Am J Physiol-Gastroint Liver Physiol (2002) 282(6):G991–G1001. doi: 10.1152/ajpgi.00298.2001
29. Kielian T. Glial Connexins and Gap Junctions in Cns Inflammation and Disease. J Neurochem (2008) 106(3):1000–16. doi: 10.1111/j.1471-4159.2008.05405.x
30. Altevogt BM, Kleopa KA, Postma FR, Scherer SS, Paul DL. Connexin29 Is Uniquely Distributed Within Myelinating Glial Cells of the Central and Peripheral Nervous Systems. J Neurosci (2002) 22(15):6458–70. doi: 10.1523/jneurosci.22-15-06458.2002
31. Odermatt B, Wellershaus K, Wallraff A, Seifert G, Degen J, Euwens C, et al. Connexin 47 (Cx47)-Deficient Mice With Enhanced Green Fluorescent Protein Reporter Gene Reveal Predominant Oligodendrocytic Expression of Cx47 and Display Vacuolized Myelin in the Cns. J Neurosci (2003) 23(11):4549–59. doi: 10.1523/jneurosci.23-11-04549.2003
32. Ni X, Li XZ, Fan ZR, Wang A, Zhang HC, Zhang L, et al. Increased Expression and Functionality of the Gap Junction in Peripheral Blood Lymphocytes Is Associated With Hypertension-Mediated Inflammation in Spontaneously Hypertensive Rats. Cell Mol Biol Lett (2018) 23:1–18. doi: 10.1186/s11658-018-0106-0
33. Valdebenito S, Barreto A, Eugenin EA. The Role of Connexin and Pannexin Containing Channels in the Innate and Acquired Immune Response. Biochim Biophys Acta Biomembr (2018) 1860(1):154–65. doi: 10.1016/j.bbamem.2017.05.015
34. Cerny J, Striz I. Adaptive Innate Immunity or Innate Adaptive Immunity? Clin Sci (Lond) (2019) 133(14):1549–65. doi: 10.1042/CS20180548
35. Matsue H, Yao J, Matsue K, Nagasaka A, Sugiyama H, Aoki R, et al. Gap Junction-Mediated Intercellular Communication Between Dendritic Cells (Dcs) Is Required for Effective Activation of Dcs. J Immunol (2006) 176(1):181–90. doi: 10.4049/jimmunol.176.1.181
36. Falk L, Dang-Lawson M, Vega JL, Pournia F, Choi K, Jang C, et al. Mutations of Cx43 That Affect B Cell Spreading in Response to Bcr Signaling. Biol Open (2014) 3(3):185–91. doi: 10.1242/bio.20147328
37. Favre-Kontula L, Rolland A, Bernasconi L, Karmirantzou M, Power C, Antonsson B, et al. Glialcam, an Immunoglobulin-Like Cell Adhesion Molecule Is Expressed in Glial Cells of the Central Nervous System. Glia (2008) 56(6):633–45. doi: 10.1002/glia.20640
38. Tan B, Tan JX, Du HF, Quan Z, Xu XD, Jiang XL, et al. Hepacam Inhibits Clear Cell Renal Carcinoma 786-0 Cell Proliferation Via Blocking Pkc Epsilon Translocation From Cytoplasm to Plasma Membrane. Mol Cell Biochem (2014) 391(1-2):95–102. doi: 10.1007/s11010-014-1991-9
39. Tittarelli A, Navarrete M, Lizana M, Hofmann-Vega F, Salazar-Onfray F. Hypoxic Melanoma Cells Deliver Micrornas to Dendritic Cells and Cytotoxic T Lymphocytes Through Connexin-43 Channels. Int J Mol Sci (2020) 21(20):7567. doi: 10.3390/ijms21207567
40. Oviedo-Orta E, Evans WH. Gap Junctions and Connexins: Potential Contributors to the Immunological Synapse. J Leukoc Biol (2002) 72(4):636–42. doi: 10.1189/jlb.72.4.636
41. Niedergang F, Di Bartolo V, Alcover A. Comparative Anatomy of Phagocytic and Immunological Synapses. Front Immunol (2016) 7:18. doi: 10.3389/fimmu.2016.00018
42. Kuokkanen E, Sustar V, Mattila PK. Molecular Control of B Cell Activation and Immunological Synapse Formation. Traffic (2015) 16(4):311–26. doi: 10.1111/tra.12257
43. Lakatos A. State-Of-Art Modelling of Inflammatory Astrocyte-Synapse Interactions in Injury and Amyotrophic Lateral Sclerosis. Neural Regener Res (2017) 12(1):75–6. doi: 10.4103/1673-5374.198977
44. Sikandar S, Patel R, Patel S, Sikander S, Bennett DL, Dickenson AH. Genes, Molecules and Patients–Emerging Topics to Guide Clinical Pain Research. Eur J Pharmacol (2013) 716(1-3):188–202. doi: 10.1016/j.ejphar.2013.01.069
45. Leybaert L, Lampe PD, Dhein S, Kwak BR, Ferdinandy P, Beyer EC, et al. Connexins in Cardiovascular and Neurovascular Health and Disease: Pharmacological Implications. Pharmacol Rev (2017) 69(4):396–478. doi: 10.1124/pr.115.012062
46. Rajnai H, Teleki I, Kiszner G, Meggyeshazi N, Balla P, Vancsik T, et al. Connexin 43 Communication Channels in Follicular Dendritic Cell Development and in Follicular Lymphomas. J Immunol Res (2015) 2015:528098. doi: 10.1155/2015/528098
47. Anand RJ, Dai S, Gribar SC, Richardson W, Kohler JW, Hoffman RA, et al. A Role for Connexin43 in Macrophage Phagocytosis and Host Survival After Bacterial Peritoneal Infection. J Immunol (2008) 181(12):8534–43. doi: 10.4049/jimmunol.181.12.8534
48. Dosch M, Zindel J, Jebbawi F, Melin N, Sanchez-Taltavull D, Stroka D, et al. Connexin-43-Dependent Atp Release Mediates Macrophage Activation During Sepsis. Elife (2019) 8:1–24. doi: 10.7554/eLife.42670
49. Rodjakovic D, Salm L, Beldi G. Function of Connexin-43 in Macrophages. Int J Mol Sci (2021) 22(3):1–11. doi: 10.3390/ijms22031412
50. Hofmann F, Navarrete M, Alvarez J, Guerrero I, Gleisner MA, Tittarelli A, et al. Cx43-Gap Junctions Accumulate at the Cytotoxic Immunological Synapse Enabling Cytotoxic T Lymphocyte Melanoma Cell Killing. Int J Mol Sci (2019) 20(18):1–16. doi: 10.3390/ijms20184509
51. Mendoza-Naranjo A, Bouma G, Pereda C, Ramírez M, Webb KF, Tittarelli A, et al. Functional Gap Junctions Accumulate at the Immunological Synapse and Contribute to T Cell Activation. J Immunol (2011) 187(6):3121–32. doi: 10.4049/jimmunol.1100378
52. Moretto G, Xu RY, Kim SU. Cd44 Expression in Human Astrocytes and Oligodendrocytes in Culture. J Neuropathol Exp Neurol (1993) 52(4):419–23. doi: 10.1097/00005072-199307000-00009
53. Zhang Y, Sloan SA, Clarke LE, Caneda C, Plaza CA, Blumenthal PD, et al. Purification and Characterization of Progenitor and Mature Human Astrocytes Reveals Transcriptional and Functional Differences With Mouse. Neuron (2016) 89(1):37–53. doi: 10.1016/j.neuron.2015.11.013
54. Liu X, Bates R, Yin D-M, Shen C, Wang F, Su N, et al. Specific Regulation of Nrg1 Isoform Expression by Neuronal Activity. J Neurosci (2011) 31(23):8491–501. doi: 10.1523/jneurosci.5317-10.2011
55. Allen NJ, Eroglu C. Cell Biology of Astrocyte-Synapse Interactions. Neuron (2017) 96(3):697–708. doi: 10.1016/j.neuron.2017.09.056
56. Westman J, Grinstein S, Marques PE. Phagocytosis of Necrotic Debris at Sites of Injury and Inflammation. Front Immunol (2020) 10:3030. doi: 10.3389/fimmu.2019.03030
57. Glass AM, Wolf BJ, Schneider KM, Princiotta MF, Taffet SM. Connexin43 Is Dispensable for Phagocytosis. J Immunol (2013) 190(9):4830–5. doi: 10.4049/jimmunol.1202884
58. Ruez R, Dubrot J, Zoso A, Bacchetta M, Molica F, Hugues S, et al. Dendritic Cell Migration Toward Ccl21 Gradient Requires Functional Cx43. Front Physiol (2018) 9:288. doi: 10.3389/fphys.2018.00288
59. Martinez-Martin N, Fernandez-Arenas E, Cemerski S, Delgado P, Turner M, Heuser J, et al. T Cell Receptor Internalization From the Immunological Synapse Is Mediated by Tc21 and Rhog Gtpase-Dependent Phagocytosis. Immunity (2011) 35(2):208–22. doi: 10.1016/j.immuni.2011.06.003
60. Thaiss CA, Semmling V, Franken L, Wagner H, Kurts C. Chemokines: A New Dendritic Cell Signal for T Cell Activation. Front Immunol (2011) 2:31. doi: 10.3389/fimmu.2011.00031
61. Kwek SS, Cha E, Fong L. Unmasking the Immune Recognition of Prostate Cancer With Ctla4 Blockade. Nat Rev Cancer (2012) 12(4):289–97. doi: 10.1038/nrc3223
62. Garre JM, Yang G, Bukauskas FF, Bennett MVL. Fgf-1 Triggers Pannexin-1 Hemichannel Opening in Spinal Astrocytes of Rodents and Promotes Inflammatory Responses in Acute Spinal Cord Slices. J Neurosci (2016) 36(17):4785–801. doi: 10.1523/jneurosci.4195-15.2016
63. Alves LA, de Carvalho AC, Savino W. Gap Junctions: A Novel Route for Direct Cell-Cell Communication in the Immune System? Immunol Today (1998) 19(6):269–75. doi: 10.1016/s0167-5699(98)01256-0
64. Chekeni FB, Elliott MR, Sandilos JK, Walk SF, Kinchen JM, Lazarowski ER, et al. Pannexin 1 Channels Mediate 'Find-Me' Signal Release and Membrane Permeability During Apoptosis. Nature (2010) 467(7317):863–7. doi: 10.1038/nature09413
65. Elliott MR, Chekeni FB, Trampont PC, Lazarowski ER, Kadl A, Walk SF, et al. Nucleotides Released by Apoptotic Cells Act as a Find-Me Signal for Phagocytic Clearance. Nature (2009) 461(7261):282–6. doi: 10.1038/nature08296
66. Hochreiter-Hufford A, Ravichandran KS. Clearing the Dead: Apoptotic Cell Sensing, Recognition, Engulfment, and Digestion. Cold Spring Harbor Perspect Biol (2013) 5(1):a008748. doi: 10.1101/cshperspect.a008748
67. Jankowski J, Perry HM, Medina CB, Huang L, Yao J, Bajwa A, et al. Epithelial and Endothelial Pannexin1 Channels Mediate Aki. J Am Soc Nephrol (2018) 29(7):1887–99. doi: 10.1681/ASN.2017121306
68. Oviedo-Orta E, Howard Evans W. Gap Junctions and Connexin-Mediated Communication in the Immune System. Biochim Biophys Acta (2004) 1662(1-2):102–12. doi: 10.1016/j.bbamem.2003.10.021
69. Poon IK, Hulett MD, Parish CR. Molecular Mechanisms of Late Apoptotic/Necrotic Cell Clearance. Cell Death Differ (2010) 17(3):381–97. doi: 10.1038/cdd.2009.195
70. Saez JC, Branes MC, Corvalan LA, Eugenin EA, Gonzalez H, Martinez AD, et al. Gap Junctions in Cells of the Immune System: Structure, Regulation and Possible Functional Roles. Braz J Med Biol Res (2000) 33(4):447–55. doi: 10.1590/s0100-879x2000000400011
71. Yuan D, Sun G, Zhang R, Luo C, Ge M, Luo G, et al. Connexin 43 Expressed in Endothelial Cells Modulates Monocyteendothelial Adhesion by Regulating Cell Adhesion Proteins. Mol Med Rep (2015) 12(5):7146–52. doi: 10.3892/mmr.2015.4273
72. Xu C-Y, Zhang W-S, Zhang H, Cao Y, Zhou H-Y. The Role of Connexin-43 in the Inflammatory Process: A New Potential Therapy to Influence Keratitis. J Ophthalmol (2019) 2019:9312827. doi: 10.1155/2019/9312827
73. Song L, Sun J, Soderholm M, Melander O, Orho-Melander M, Nilsson J, et al. Association of Tim-1 (T-Cell Immunoglobulin and Mucin Domain 1) With Incidence of Stroke. Arterioscler Thromb Vasc Biol (2020) 40(7):1777–86. doi: 10.1161/ATVBAHA.120.314269
74. Zhao R, Song C, Liu L, Liu Q, Zhou N, Zhou X, et al. Single Immunoglobulin and Tollinterleukin1 Receptor Domain Containing Molecule Protects Against Severe Acute Pancreatitis in Vitro by Negatively Regulating the Tolllike Receptor4 Signaling Pathway: A Clinical and Experimental Study. Mol Med Rep (2020) 22(4):2851–9. doi: 10.3892/mmr.2020.11379
75. Zhang J, Hou C, Dou S, Li G, Wang Z, Liu Y, et al. T Cell Immunoglobulin and Mucin Domain Protein 3 Inhibits Glycolysis in Raw 264.7 Macrophages Through Hexokinase 2. Scand J Immunol (2020) 93(2):e12981. doi: 10.1111/sji.12981
76. Hamieh M, Dobrin A, Cabriolu A, van der Stegen SJC, Giavridis T, Mansilla-Soto J, et al. Car T Cell Trogocytosis and Cooperative Killing Regulate Tumour Antigen Escape. Nature (2019) 568(7750):112–6. doi: 10.1038/s41586-019-1054-1
77. Joly E, Hudrisier D. What Is Trogocytosis and What Is Its Purpose? Nat Immunol (2003) 4(9):815–. doi: 10.1038/ni0903-815
78. Mazzini E, Massimiliano L, Penna G, Rescigno M. Oral Tolerance Can Be Established Via Gap Junction Transfer of Fed Antigens From Cx3cr1(+) Macrophages to Cd103(+) Dendritic Cells. Immunity (2014) 40(2):248–61. doi: 10.1016/j.immuni.2013.12.012
79. Smyth LA, Hervouet C, Hayday T, Becker PD, Ellis R, Lechler RI, et al. Acquisition of Mhc:Peptide Complexes by Dendritic Cells Contributes to the Generation of Antiviral Cd8+ T Cell Immunity in Vivo. J Immunol (2012) 189(5):2274–82. doi: 10.4049/jimmunol.1200664
80. Wakim LM, Bevan MJ. Cross-Dressed Dendritic Cells Drive Memory Cd8+ T-Cell Activation After Viral Infection. Nature (2011) 471(7340):629–32. doi: 10.1038/nature09863
81. Handel A, Yates A, Pilyugin SS, Antia R. Gap Junction-Mediated Antigen Transport in Immune Responses. Trends Immunol (2007) 28(11):463–6. doi: 10.1016/j.it.2007.08.006
82. Mendoza-Naranjo A, Saez PJ, Johansson CC, Ramirez M, Mandakovic D, Pereda C, et al. Functional Gap Junctions Facilitate Melanoma Antigen Transfer and Cross-Presentation Between Human Dendritic Cells. J Immunol (2007) 178(11):6949–57. doi: 10.4049/jimmunol.178.11.6949
83. Neijssen J, Herberts C, Drijfhout JW, Reits E, Janssen L, Neefjes J. Cross-Presentation by Intercellular Peptide Transfer Through Gap Junctions. Nature (2005) 434(7029):83–8. doi: 10.1038/nature03290
84. Eugenin EA. Role of Connexin/Pannexin Containing Channels in Infectious Diseases. FEBS Lett (2014) 588(8):1389–95. doi: 10.1016/j.febslet.2014.01.030
85. Woehrle T, Yip L, Elkhal A, Sumi Y, Chen Y, Yao Y, et al. Pannexin-1 Hemichannel-Mediated Atp Release Together With P2x1 and P2x4 Receptors Regulate T-Cell Activation at the Immune Synapse. Blood (2010) 116(18):3475–84. doi: 10.1182/blood-2010-04-277707
86. Oviedo-Orta E, Perreau M, Evans WH, Potolicchio I. Control of the Proliferation of Activated Cd4+ T Cells by Connexins. J Leukoc Biol (2010) 88(1):79–86. doi: 10.1189/jlb.0909613
87. Klein M, Bopp T. Cyclic Amp Represents a Crucial Component of Treg Cell-Mediated Immune Regulation. Front Immunol (2016) 7:315(315). doi: 10.3389/fimmu.2016.00315
88. Bopp T, Becker C, Klein M, Klein-Hessling S, Palmetshofer A, Serfling E, et al. Cyclic Adenosine Monophosphate Is a Key Component of Regulatory T Cell-Mediated Suppression. J Exp Med (2007) 204(6):1303–10. doi: 10.1084/jem.20062129
89. Zhao H, Liao X, Kang Y. Tregs: Where We Are and What Comes Next? Front Immunol (2017) 8:1578. doi: 10.3389/fimmu.2017.01578
90. Chen CX, He HJ, Cai QC, Zhang W, Kou TC, Zhang XW, et al. Bracovirus-Mediated Innexin Hemichannel Closure in Cell Disassembly. iScience (2021) 24(4):102281. doi: 10.1016/j.isci.2021.102281
91. Kuczma M, Lee JR, Kraj P. Connexin 43 Signaling Enhances the Generation of Foxp3+ Regulatory T Cells. J Immunol (2011) 187(1):248–57. doi: 10.4049/jimmunol.1003785
92. Zhang HC, Zhang ZS, Zhang L, Wang A, Zhu H, Li L, et al. Connexin 43 in Splenic Lymphocytes Is Involved in the Regulation of Cd4(+)Cd25(+) T Lymphocyte Proliferation and Cytokine Production in Hypertensive Inflammation. Int J Mol Med (2018) 41(1):13–24. doi: 10.3892/ijmm.2017.3201
93. Lichtman A. The Abcs of Ovarian Cancer Immunology: Iga, B Cells, and Ctls. Sci Immunol (2021) 6(57):1–5. doi: 10.1126/sciimmunol.abh3184
94. Tuma PL, Hubbard AL. Transcytosis: Crossing Cellular Barriers. Physiol Rev (2003) 83(3):871–932. doi: 10.1152/physrev.00001.2003
95. Kreer C, Rauen J, Zehner M, Burgdorf S. Cross-Presentation: How to Get There - or How to Get the Er. Front Immunol (2011) 2:87. doi: 10.3389/fimmu.2011.00087
Keywords: connexin, pannexin, immunological synapse, T and B lymphocytes, cluster of differentiation antigens, phagocytosis, trogocytosis, transcytosis
Citation: Meng J-H, Chen C-X, Ahmadian MR, Zan H, Luo K-J and Jiang JX (2022) Cross-Activation of Hemichannels/Gap Junctions and Immunoglobulin-Like Domains in Innate–Adaptive Immune Responses. Front. Immunol. 13:882706. doi: 10.3389/fimmu.2022.882706
Received: 24 February 2022; Accepted: 23 June 2022;
Published: 15 July 2022.
Edited by:
Hang Hubert Yin, Tsinghua University, ChinaReviewed by:
Carola J. Maturana, Princeton University, United StatesChenju Yi, Sun Yat-Sen University, China
Anaclet Ngezahayo, Leibniz University Hannover, Germany
Copyright © 2022 Meng, Chen, Ahmadian, Zan, Luo and Jiang. This is an open-access article distributed under the terms of the Creative Commons Attribution License (CC BY). The use, distribution or reproduction in other forums is permitted, provided the original author(s) and the copyright owner(s) are credited and that the original publication in this journal is cited, in accordance with accepted academic practice. No use, distribution or reproduction is permitted which does not comply with these terms.
*Correspondence: Kai-Jun Luo, kaijun_luo@ynu.edu.cn; Jean X. Jiang, jiangj@uthscsa.edu
†These authors have contributed equally to this work