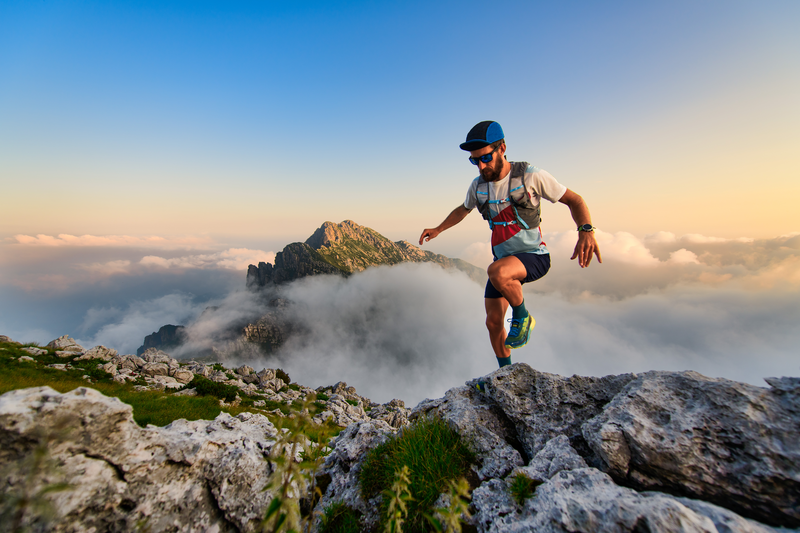
95% of researchers rate our articles as excellent or good
Learn more about the work of our research integrity team to safeguard the quality of each article we publish.
Find out more
ORIGINAL RESEARCH article
Front. Immunol. , 26 April 2022
Sec. Cancer Immunity and Immunotherapy
Volume 13 - 2022 | https://doi.org/10.3389/fimmu.2022.879030
This article is part of the Research Topic The Immune Escape Mechanism and Novel Immunotherapeutic Strategies of Leukemia View all 14 articles
Background: While chimeric antigen receptor (CAR)-T cell therapy is becoming widely used in hematological malignancies with remarkable remission rate, their high recurrence remains an obstacle to overcome. The role of consolidative transplantation following CAR-T cell-mediated remission remains controversial. We conducted a retrospective study to explore whether bridging to unrelated cord blood transplantation (UCBT) could improve the prognosis of patients entering remission after CAR-T therapy with different characteristics through subgroup analyses.
Methods: We reviewed 53 patients with relapsed/refractory (R/R) B-cell acute lymphoblastic leukemia (B-ALL) successfully infused with CD19 CAR-T cells and achieved complete remission (CR). In this study, 25 patients received consolidative UCBT (UCBT group) and 28 patients did not accept any intervention until relapse (non-UCBT group). Subgroup analysis on prognosis was then performed according to gender, age, number of previous relapses, tumor burden, presence of poor prognostic markers, and structure of CAR.
Results: Compared with the non-UCBT group, patients who underwent consolidative UCBT had better median event-free survival (EFS; 12.3 months vs. 6.2 months; P = 0.035) and relapse-free survival (RFS; 22.3 months vs. 7.2 months; P = 0.046), while no significant difference was found in overall survival (OS; 30.8 months vs. 15.3 months; P = 0.118). Subsequent multivariate analysis revealed that bridging to UCBT was a protective factor for RFS (P = 0.048) but had no significant effect on EFS (P = 0.205) or OS (P = 0.541). In the subgroup analysis, UCBT has an added benefit in patients with specific characteristics. Patients who experienced ≥2 relapses or with sustained non-remission (NR) showed better RFS (P = 0.025) after UCBT. Better EFS was seen in patients with poor prognostic markers (P = 0.027). In the subgroup with pre-infusion minimal residual disease (MRD) ≥5% or with extramedullary disease (EMD), UCBT significantly prolonged EFS (P = 0.009), RFS (P = 0.017), and OS (P = 0.026). Patients with occurrence of acute graft-versus-host disease (aGVHD) appeared to have a longer duration of remission (P = 0.007).
Conclusion: Consolidative UCBT can, to some extent, improve clinical outcomes of patients with R/R B-ALL entering remission following CD19 CAR-T therapy, especially in patients with more recurrences before treatment, patients with poor prognostic markers, and patients with a higher tumor burden. The occurrence of aGVHD after UCBT was associated with better RFS.
Chimeric antigen receptor (CAR)-T cell therapy has been proven to have remarkable efficacy in hematological malignancies in recent years and is considered one of the most promising targeted therapies for tumors. CD19-targeted CAR-T cell therapy has led to a paradigm shift in the treatment of relapsed and refractory (R/R) B-cell acute lymphoblastic leukemia (B-ALL). Numerous clinical trials revealed that patients treated with CD19 CAR-T cells can achieve a complete remission (CR) rate of 75%–93%. Despite the impressive results, however, approximately 38%–61% of patients eventually relapsed during long-term follow-up (1–8). Researchers have proposed a series of solutions to reduce the recurrence rate after CAR-T cell therapy such as optimizing CAR structure (7, 8), designing artificial antigen-presenting cells (AAPCs) (9), binding immunological checkpoint inhibitors (10), sequentially administering two groups of CAR-T cells (11), and bridging to transplantation. Some studies speculated that the combination of CAR-T therapy and transplantation prolonged survival and provided patients with more opportunities (12–14).
However, the role of consolidative transplantation following CAR-T therapy remains controversial while the effect can be influenced by pretreatment patient characteristics, lymphodepletion regimen, structure of CAR, and post-CAR-T therapy parameters (12). In some groups, it increases the economic burden and risk of death related to transplantation. It is necessary to weigh the advantages and disadvantages.
Equally important, which source of stem cells for transplantation to choose is inconclusive. Current studies emphasize the interface between allogeneic hematopoietic stem cell transplantation (allo-HSCT) and CAR-T cell therapy, as allo-HSCT is considered the only recognized curative cellular therapy for patients with B-ALL. Few studies have focused on unrelated cord blood transplantation (UCBT) following CAR-T therapy. Umbilical cord blood (UCB) is gradually being considered as an alternative source of peripheral blood progenitor cells (PBPCs) or bone marrow (BM) transplantation, particularly when a human leukocyte antigen (HLA)-matched donor is not available. A retrospective study determined the optimal role of UCBT in adults with acute leukemia, and showed that leukemia-free survival (LFS) in patients after UCBT was equivalent to that after PBPC or BM transplantation (15). Thus, UCBT may also be considered as an option to improve the prognosis of patients who have undergone CAR-T therapy. Another study conducted at our center showed that patients who received consolidative UCBT after CAR-T-induced remission had a 26.7% 2-year cumulative incidence of relapse (CIR) (16), so it is worth exploring if UCBT can benefit patients who have obtained remission after CAR-T therapy. Our research aims to explore the role of consolidative UCBT in patients receiving CD19 CAR-T cells and which characteristics of patients can influence the effect of UCBT more obviously.
From January 2016 to April 2021, we reviewed patients with R/R B-ALL who were successfully infused with CD19 CAR-T cells at the First Affiliated Hospital of USCT (Anhui Provincial Hospital) and the Second Hospital of Anhui Medical University (SHAMU). The medical ethics committee of the two hospitals reviewed and approved the study protocols. Fifty-three patients were consecutively enrolled in this non-randomized clinical study according to exclusion criteria including the following: 1) unable to evaluate the effectiveness of CAR-T cell therapy, (2) failure to achieve CR after CAR-T cell therapy, (3) contraindications in terms of critical organ insufficiency and uncontrollable infections, and (4) a history of transplantation before CAR-T therapy. Twenty-five patients received consolidative UCBT at our center selectively, depending on pre-UCBT assessment including disease features, treatment history, comorbidities, and personal reasons including patient preference and economic considerations. In conformity with the Declaration of Helsinki, informed consent was provided from each participant.
All included patients were treated with conditioning chemotherapy including fludarabine (FLU, 30 mg/m2 × 3 days) in combination with cyclophosphamide (CY, 300 mg/m2 × 3 days) before the intravenous infusion of cryopreserved CD19 CAR-T cells at a dose of 1 × 106 cells/kg body weight. The CAR in this study consists of an CD19-specific single-chain antibody fragment (scFv) derived from FMC63 fused to a modified IgG4-hinge spacer, a costimulatory molecule including CD28 alone or both CD28 and CD137 (4-1BB), and a CD3ζ signaling domain. Enrichment of CAR-T cells from patient’s PBMCs was done using CD4-magnetic beads (Miltenyi Biotec GmbH) and CD8-magnetic beads (Miltenyi Biotec GmbH).
The selection of cord blood and HLA typing have been previously described (17). Concisely, molecular techniques with minimum antigenic segmentation level resolution for HLA-A and HLA-B and allele-level resolution for DRB1 were used when performing HLA typing. All recipients obtained single-unit cord blood from the Chinese Cord Blood Bank. Each unit of cord blood was high-resolution matched to the recipient’s HLA-A and HLA-B antigens and HLA-DRB1 and had a minimum of 3.0 × 107 total nucleated cells (TNCs)/kg of body weight and 1.2 × 105 CD34+ cells/kg of body weight until frozen. All recipients were given myeloablative regimen including FLU/BU/CY (FLU, 30 mg/m2 per day for 4 days; busulfan, total 12.8 mg/kg, 0.8 mg/kg every 6 h for 4 days; CY, 60 mg/kg daily for 2 days), FLU/BU/CY plus carmustine (BCNU) (250 mg/m2), FLU/CY plus total body irradiation (TBI; total 12 Gy, 4 fractions) or FLU/BU/CY plus semustine (320 mg/m2). A co-application of mycophenolate mofetil and cyclosporin A was used as prophylaxis for graft-versus-host disease (GVHD).
Bone marrow morphology with ≤5% blasts, no evidence of circulating blasts, and no extramedullary infiltration were considered as CR. Minimal residual disease (MRD)-negative CR was characterized as no immunophenotypically abnormal blasts detected in peripheral blood (PB)/BM by multiparametric flow cytometry (FCM). MRD status was assessed by 10-color FCM with a sensitivity of 104 nucleated cells. Disease relapse was defined as ≥5% of blasts in BM, reappearance of blasts in the PB, or extramedullary infiltration after CR. The endpoints were event-free survival (EFS), relapse-free survival (RFS), and overall survival (OS). We calculated EFS as the time interval from CAR-T cell infusion to disease progression (including MRD-positive and gene recurrence), relapse, or death, whichever came first, or last visit. RFS was calculated from the date of CAR-T cell infusion to the date of relapse, death, or last visit. For OS, death as the final endpoint was caused by any factor or the final follow-up date could be used. Acute GVHD (aGVHD) was assessed according to the Mount Sinai Acute GvHD International Consortium (MAGIC) criterion (18), and chronic GVHD (cGVHD) was assessed according to the consensus criteria of the National Institutes of Health (19).
Descriptive statistics were used to present the characteristics of the patients. The difference in non-relapse mortality (NRM) rate between two groups was determined by Fisher’s exact test. Kaplan–Meier analysis and Cox regression model were applied to perform univariate and multivariate analysis on factors affecting the survival of overall patients, respectively. The median EFS, RFS, and OS were demonstrated by Kaplan–Meier curves and were compared by log-rank test. The hazard ratio (HR) and 95% CI for EFS, PFS, and OS for subgroup analysis were estimated using a stratified Cox regression model and visualized by a forest plot. A two-tailed P value <0.05 was considered statistically significant. All statistical analyses were conducted using Statistical Product and Service Solutions (SPSS) 26.0, and GraphPad Prism 8.00 software (GraphPad Software, La Jolla, CA, USA) was used to create figures.
A total of 53 patients with B-ALL treated with CD19 CAR-T cells were consecutively enrolled. Characteristics of patients with a median age of 28 (range 3–66 years) years are presented in Table 1. Twenty-two (41.5%) patients experienced less than 2 relapses, and 31 (58.5%) patients experienced ≥2 relapses or remain in non-remission (NR). Thirty-six (67.9%) patients had poor prognostic markers including TP53, BCR-ABL1, E2A-PBX1, and MLL-AF4 and complex karyotype before CAR-T therapy. Furthermore, 43 (81.1%) patients had MRD >5% or with extramedullary disease (EMD) before CAR-T treatment, and 10 (18.9%) patients had MRD <5%. Thirty-eight (71.7%) patients were infused with CAR-T cells incorporating a CD28 co-stimulation domain, and 15 (28.3%) patients with CAR-T cells contained both CD28 and 4-1BB. All patients achieved CR before transplant, while 3 patients remained MRD-positive.
Twenty-five patients received UCBT after CAR-T cell therapy (the UCBT group); 28 patients did not accept any treatment until relapse (the non-UCBT group). The median interval from CAR-T therapy to UCBT was 66 (range 30–268 days) days; 17 patients received UCBT within 3 months following CAR-T therapy. The median infused TNC count and CD34+ cells were 2.79 (range, 1.24–9.80) × 107/kg and 1.97 (range, 0.69–7.15) × 105/kg, respectively. Among the patients who underwent UCBT after CAR-T therapy, 11 (44.0%) patients had aGVHD and one patient had cGVHD in the follow-up. Pre-engraftment syndrome (PES) was observed in 19 (76.0%) patients (Table 1).
Disease status of 53 patients from the day of CAR-T cell infusion to the end of follow-up was shown in Figure 1A. The NRM rates in the UCBT and non-UCBT groups were 33.3% and 5%, respectively; no significant difference was found by Fisher’s exact test (P = 0.128) between the two groups. In the UCBT group, 10 (40.0%) patients relapsed at a median time of 6.2 months (range 1.4–22.3 months) including one CD19-negative relapse and one central nervous system leukemia (CNSL), and one patient underwent transformation from ALL to myelodysplastic syndrome (MDS) and eventually progressed to acute myeloid leukemia (AML). Ten (40.0%) patients remained disease-free until the end of follow-up; one patient was lost to follow-up at 5.6 months of remission. Four patients died because of transplant-related complications including GVHD, thrombotic microangiopathy (TMA), and severe infection. Among the patients who relapsed after UCBT, eight patients received reinfusions of CAR-T cells after relapse, five patients achieved CR again, and two of them remained in MRD-negative remission until the end of follow-up. The remaining two patients who received only chemotherapy or supportive therapy showed poor prognosis and died of primary disease in the short term. In the non-UCBT group, 18 (64.3%) patients experienced relapses at a median time of 6.5 months (range 1.4–58.9 months). Six patients achieved sustained MRD-negative remission without other interventions after CAR-T cell infusion until the end of follow up, one patient remained in MRD-negative remission and was lost to follow-up at 34.3 months, one patient showed a positive MRD at 36.5 months, and one patient showed a positive P210 at 17.7 months.
Figure 1 Treatment responses and long-term survival of each patient, survival analysis between the UCBT group and the non-UCBT group, and the association between aGVHD and RFS in the UCBT group. (A) Disease status from CAR-T cell infusion to the end of follow-up of each patient. (B–D) Differences of EFS (B), RFS (C), and OS (D) between the UCBT group and the non-UCBT group in overall patients. (E) The association between the occurrence of aGVHD and RFS of patients underwent consolidative UCBT after entering remission by CAR-T therapy. UCBT, unrelated cord blood transplantation; aGVHD, acute graft-versus-host disease; EFS, event-free survival; RFS, relapse-free survival; OS, overall survival; CAR, chimeric antigen receptor.
Initially, we performed an analysis of the factors associated with overall prognosis (Table 2). Univariate analysis indicated a significant difference in survival between the two groups, the estimated median EFS (12.3 months vs. 6.2 months; P = 0.035) and RFS (22.3 months vs. 7.2 months; P = 0.046) were better in the UCBT group than those in the non-UCBT group, while the OS showed no difference (30.8 months vs. 15.3 months; P = 0.118) (Figures 1B–D). Subsequent multivariate analysis for factors with P < 0.2 in the univariate analysis showed that consolidative UCBT was an independent protective factor for RFS (P = 0.048), but with no significant effect on overall EFS (P = 0.205) or OS (P = 0.541). Multivariate analysis also showed a worse EFS (P = 0.069) and OS (P = 0.045) in patients with poor prognostic markers.
Comparison of baseline characteristics of the two groups revealed no significant differences in gender, number of relapses, poor prognostic markers, pre-infusion tumor burden, and CAR structure; however, there were differences in the age distribution (Table 3). Considering if those factors may potentially associate with the influence of consolidative UCBT on the prognosis of patients, we subsequently subgrouped the patients to determine which group of patients were more likely to benefit from consolidative UCBT (Figure 2). In patients who experienced ≥2 relapses or sustained NR, UCBT prolonged RFS (P = 0.025) compared to non-UCBT. Consolidative UCBT had significant influences in EFS (P = 0.027) of patients with poor prognostic markers including MLL-AF4, BCR-ABL1, TP53, E2A-PBX1, and complex karyotype, while no significant effect was shown in patients without those markers. In the subgroup with MRD ≥5% or with EMD before infusion, UCBT prolonged EFS (P = 0.009), RFS (P = 0.017), and OS (P = 0.026) independent of poor prognostic markers; the improvement was not observed in patients with pre-infusion MRD <5%. No significant effect of UCBT was seen in either group subdivided according to gender (men and women), CAR structure (CD28 and CD28/4-1BB), and age (<25 years and ≥25 years).
Figure 2 Forest plot showing the hazard ratios for EFS, RFS, and OS of various subgroups. UCBT, unrelated cord blood transplantation; EFS, event-free survival; RFS, relapse-free survival; OS, overall survival; CAR, chimeric antigen receptor; NR, non-remission; MRD, minimal residual disease; EMD, extramedullary disease; HR, hazard ratio.
In our study, time between CAR-T cell infusion and UCBT did not influence EFS (P = 0.360), RFS (P = 0.413), or OS (P = 0.204) of the patients. Four patients bridging to UCBT at 3 months after CAR-T therapy still showed sustained remission. Our further exploration suggested a correlation between aGVHD after UCBT and prognosis, which showed that patients who had aGVHD tend to have a better RFS (P = 0.007) (Figure 1E), although EFS (P = 0.113) and OS (P = 0.593) did not significantly prolong, and the results need to be further confirmed by a large sample.
While CAR-T cell therapy increases the remission rate of patients diagnosed with R/R B-cell hematological malignancies, the limited duration of remission and unsatisfactory long-term survival present challenges for CAR-T cell therapy. The two relapse patterns after CAR-T therapy include CD19-positive relapse and CD19-negative relapse. CD19-positive relapses are commonly associated with decreased CAR-T cell persistence, low potency and poor response of CAR, and transient B-cell aplasia (20). The mechanism of this kind of relapse may be related to CD19 gene mutation leading to CD19 loss (21), selection and lineage switch by immune pressure (22, 23), and trogocytosis and cooperative killing (24). Prevention for relapse after CAR-T therapy includes improving the structure of CAR (7, 25), optimizing lymphodepletion regimen (26), dual/multi-targeted CAR-T cells (23, 27), sequential infusion of two groups of CAR-T cells (11, 28), CAR-T cell combined with immune checkpoint inhibitor (10), and consolidative transplantation after CAR-T therapy (12–14, 29–33). HSCT has been chosen in several centers to consolidate remission after CAR-T treatment. Clinical studies by Lee et al. (14) demonstrated a decline in relapse rate in pediatric patients who were consolidated with HSCT. Hay et al. (34) reported a phase I/II data investigating the role of 4-1BB CD19 CAR-T cells in adult ALL, demonstrating prolonged EFS in patients who received HSCT after CAR-T therapy. A clinical study by Shalabi et al. (29) showed that CAR-T cell therapy combined with HSCT could synergistically improve LFS. However, whether to consolidate with transplantation after CAR-T is still a critical question, since treatment-related morbidity and mortality need to be balanced against the risk of relapse. Functional CAR-T cells that persisted in patients may be destroyed by transplantation and losing their antitumor activity. And there are some studies showing that no difference in prognosis was seen in patients who did and did not receive consolidative transplantation after fusion of CD28-based CD19 CAR-T therapy (6, 35).
Another issue to be addressed is which source of transplantation should be chosen. Current studies have focused on consolidative HSCT after CAR-T therapy, with fewer studies on UCBT. Stem cells can be obtained from BM, PB, or UCB. UCB offers benefits such as rapid acquisition, less constricted HLA matching, and lower rates of GVHD (36–38). A comparative analysis of UCB and BM in children with acute leukemia demonstrated that 4-6/6 HLA-matched UCB provided a similar probability of LFS to matched BM (39). Thus, we assumed that UCBT may serve as an alternative for patients without a compatible donor. However, there is still a deficiency of data on the outcomes of CAR-T therapy bridging to UCBT. Another study in our center showed a relatively high 2-year CIR in patients who received consolidative UCBT following CAR-T-mediated remission (16), so it is worth exploring whether UCBT can benefit patients who achieved remission after CAR-T therapy or not.
In this retrospective study, 25 of the 53 patients received subsequent UCBT after CAR-T therapy, and the other 28 received CAR-T therapy alone. In overall patients, the NRM rate appeared to be higher in the UCBT group, but there was no statistical difference between the two groups, and UCBT contributed a significant improvement in RFS, while there was no significant effect on EFS and OS. In studies focusing on consolidative allo-HSCT after CAR-T, the effect of HSCT was influenced by many potential factors including complex karyotypes, certain genes associated with poor prognosis, leukemia burden, number of relapses, high lactate dehydrogenase (LDH) levels, lymphodepletion regimen, and constructure of CARs (12). Therefore, we conducted a subgroup analysis to explore factors influencing the effect of UCBT.
A high pre-infusion tumor burden had been reported to increase the relapse rate after CAR-T therapy (6, 13, 40). A clinical trial showed that consolidative HSCT significantly prolonged EFS and RFS in patients with a high leukemia burden (13). In our study, we observed a pronounced influence of consolidative UCBT on patients with MRD ≥5% or with EMD, suggesting that patients with a higher tumor burden would be more likely to benefit from consolidative UCBT. Some studies have found that surface CAR expression was inversely correlated with tumor burden due to receptor internalization (41–43); this may contribute to a higher probability of relapse in patients with a high tumor burden. Those findings demonstrate the necessity of consolidative transplantation for patients with a high tumor burden after CAR-T therapy. In addition, we found that the number of relapses can influence the effect of UCBT, patients who experienced fewer than 2 relapses did not benefit from consolidative UCBT, while in patients with recurrent relapses or sustainable NR, consolidative UCBT improved their RFS significantly. As for patients with poor prognosis markers, while worse overall EFS and OS were observed in multivariate analysis, those patients showed an improved EFS after bridging to UCBT. MLL/AF4, BCR/ABL1, TP53, and E2A/PBX1 complex karyotypes are reported to indicate an inferior prognosis in B-ALL patients (44–48). Consolidative UCBT after CAR-T therapy may achieve a longer molecular response.
According to criteria published by Gökbuget et al. (49) age is the most important prognostic factor for ALL, and the prognosis of patients deteriorates with increasing age. Apart from that, some studies showed that adult patients are more likely to benefit from consolidative allo-HSCT, while younger patients can achieve durable remission without a consolidating allo-HSCT (4, 8). As the younger patients took a larger proportion in the UCBT group in our study, we further grouped the patients according to age (<25 years vs. ≥25 years) to reduce the influence of the confounding variable; the results showed that consolidative UCBT did not affect survival of patients in either group.
Another factor that can affect prognosis is the co-stimulatory structural domain of CAR. Preclinical studies indicated a relatively short duration after infusion of CAR-T cells with CD28 co-stimulatory domain (50, 51); moreover, numerous investigations have revealed that 4-1BB-based CAR-T cells exhibited more durable persistence than CAR-T cells that contained CD28 co-stimulatory domain (20, 32, 33, 52, 53). However, the outcomes of CAR-T cell containing CD28 co-stimulatory domains varied significantly among different studies. A retrospective analysis showed no improvement in EFS or OS in patients using CD28-based CD19 CAR-T cells bridged with HSCT (6). A study in pediatric patients using CD28 co-stimulatory CD19 CAR-T cells (54) suggested that HSCT leads to better EFS. Several reports of treating patients with 4-1BB-based CD19 CAR-T cells (13, 34) exhibited better RFS with consolidative HSCT, whereas patients receiving 4-1BB-containing CD19 CAR-T cells in the global ELIANA trial show no benefit in OS (8). It is unscrupulous to conclude the influence of different CAR structures on the effect of post-CAR-T transplantation. In our study, we did not observe an improvement in prognosis by consolidative UCBT in patients receiving CD28-based CAR nor in those receiving CD28/4-1BB-based CAR. Considering the limited sample size and confounding factors, a comparative study is needed.
Our study demonstrated that consolidative UCBT after CAR-T therapy can improve the clinical outcomes in specific groups of patients; however, the recurrence rate after transplantation was relatively high (40.0%, 10/25). Patients without occurrence of aGVHD appeared to have a shorter duration of remission. The occurrence of aGVHD after UCBT may indicate better RFS. A study showed that time from CAR-T cell application to transplantation associated with the risk of death (55). Some researchers recommended early consolidative UCBT to maximize the benefit, considering that approximately 10% of patients relapsed after CAR-T therapy within 3 months (12). However, our study did not show a correlation between the interval and long-term survival; among the 10 patients in the UCBT group who achieved sustained remission, four patients had an interval ≥3 months from CAR-T infusion to transplantation. Thus, the optimal time for consolidative transplantation needs to be determined by multicenter and large-sample studies. It is imperative to seek a treatment option for patients who relapse after transplantation because these patients generally have a poor prognosis. Eight patients accepted re-infusion of CAR-T cells in this study, five of them achieved CR and the median OS after relapse was 21.9 months (range 9.4–39.5 months), the remaining two patients who did not receive a second CAR-T therapy died rapidly after relapse. Comparative studies with larger samples are necessary to explore the role of re-infusion of CAR-T cells.
Our study has its shortcomings due to its retrospective nature and the uniformity in baseline characteristics of patients; election bias on transplantation may also exist. However, the results from real-world data by subgroup analysis are still informative. Large-sample studies with consistent patient baseline characteristics are still needed.
To conclude, our study showed that consolidative UCBT provides an option for post-CAR-T consolidation. In patients with a history of ≥2 relapses or sustained NR, with poor prognostic markers, or with MRD ≥5% or EMD, long-term survival was significantly improved by consolidative UCBT. In contrast, UCBT does not necessarily result in a better prognosis for patients with a lower number of recurrences, no prognostic markers, and a lower tumor burden. For posttransplantation relapse, re-infusion of CAR-T cells may be an option to consider.
The original contributions presented in the study are included in the article/supplementary material. Further inquiries can be directed to the corresponding author.
This study was approved by the medical ethics committee of the First Affiliated Hospital of USTC (Anhui Provincial Hospital), Hefei, China (2016-101), and the Second Hospital of Anhui Medical University (SHAMU). All patients gave their written informed consent in accordance with the Declaration of Helsinki.
QX, LX, and XBW analyzed the data and wrote the paper. FA, HX, LW, LG, XHZ, KS, WY, XW, JT, HL, XL, XYZ, ZZ, ZS, and XBW provided the study patients. XBW designed the research and edited the article. All authors contributed to the article and approved the submitted version.
This study received funding from the National Natural Science Foundation of China #1 under Grant 82170221. The funder was not involved in the study design, collection, analysis, interpretation of data, the writing of this article or the decision to submit it for publication. All authors declare no other competing interests.
The authors declare that the research was conducted in the absence of any commercial or financial relationships that could be construed as a potential conflict of interest.
All claims expressed in this article are solely those of the authors and do not necessarily represent those of their affiliated organizations, or those of the publisher, the editors and the reviewers. Any product that may be evaluated in this article, or claim that may be made by its manufacturer, is not guaranteed or endorsed by the publisher.
The authors would like to thank all members of the study team and the patients and their families.
1. Neelapu SS, Locke FL, Bartlett NL, Lekakis LJ, Miklos DB, Jacobson CA, et al. Axicabtagene Ciloleucel CAR T-Cell Therapy in Refractory Large B-Cell Lymphoma. N Engl J Med (2017) 377(26):2531–44. doi: 10.1056/NEJMoa1707447
2. June CH, Sadelain M. Chimeric Antigen Receptor Therapy. N Engl J Med (2018) 379(1):64–73. doi: 10.1056/NEJMra1706169
3. Pan J, Yang JF, Deng BP, Zhao XJ, Zhang X, Lin YH, et al. High Efficacy and Safety of Low-Dose CD19-Directed CAR-T Cell Therapy in 51 Refractory or Relapsed B Acute Lymphoblastic Leukemia Patients. Leukemia (2017) 31(12):2587–93. doi: 10.1038/leu.2017.145
4. Gardner RA, Finney O, Annesley C, Brakke H, Summers C, Leger K, et al. Intent-To-Treat Leukemia Remission by CD19 CAR T Cells of Defined Formulation and Dose in Children and Young Adults. Blood (2017) 129(25):3322–31. doi: 10.1182/blood-2017-02-769208
5. Wang M, Munoz J, Goy A, Locke FL, Jacobson CA, Hill BT, et al. KTE-X19 CAR T-Cell Therapy in Relapsed or Refractory Mantle-Cell Lymphoma. N Engl J Med (2020) 382(14):1331–42. doi: 10.1056/NEJMoa1914347
6. Park JH, Riviere I, Gonen M, Wang X, Senechal B, Curran KJ, et al. Long-Term Follow-Up of CD19 CAR Therapy in Acute Lymphoblastic Leukemia. N Engl J Med (2018) 378(5):449–59. doi: 10.1056/NEJMoa1709919
7. Cao J, Wang G, Cheng H, Wei C, Qi K, Sang W, et al. Potent Anti-Leukemia Activities of Humanized CD19-Targeted Chimeric Antigen Receptor T (CAR-T) Cells in Patients With Relapsed/Refractory Acute Lymphoblastic Leukemia. Am J Hematol (2018) 93(7):851–8. doi: 10.1002/ajh.25108
8. Maude SL, Laetsch TW, Buechner J, Rives S, Boyer M, Bittencourt H, et al. Tisagenlecleucel in Children and Young Adults With B-Cell Lymphoblastic Leukemia. N Engl J Med (2018) 378(5):439–48. doi: 10.1056/NEJMoa1709866
9. Singh H, Figliola MJ, Dawson MJ, Olivares S, Zhang L, Yang G, et al. Manufacture of Clinical-Grade CD19-Specific T Cells Stably Expressing Chimeric Antigen Receptor Using Sleeping Beauty System and Artificial Antigen Presenting Cells. PloS One (2013) 8(5):e64138. doi: 10.1371/journal.pone.0064138
10. Li AM, Hucks GE, Dinofia AM, Seif AE, Teachey DT, Baniewicz D, et al. Checkpoint Inhibitors Augment CD19-Directed Chimeric Antigen Receptor (CAR) T Cell Therapy in Relapsed B-Cell Acute Lymphoblastic Leukemia. Blood (2018) 132(Supplement 1):556–6. doi: 10.1182/blood-2018-99-112572
11. Huang L, Wang N, Cao Y, Li C, Xiao Y, Xiao M, et al. CAR22/19 Cocktail Therapy for Patients With Refractory/Relapsed B-Cell Malignancies. Blood (2018) 132(Supplement 1):1408–8. doi: 10.1182/blood-2018-99-113714
12. Jiang H, Hu Y, Mei H. Consolidative Allogeneic Hematopoietic Stem Cell Transplantation After Chimeric Antigen Receptor T-Cell Therapy for Relapsed/Refractory B-Cell Acute Lymphoblastic Leukemia: Who? When? Why? biomark Res (2020) 8(1):66. doi: 10.1186/s40364-020-00247-8
13. Jiang H, Li C, Yin P, Guo T, Liu L, Xia L, et al. Anti-CD19 Chimeric Antigen Receptor-Modified T-Cell Therapy Bridging to Allogeneic Hematopoietic Stem Cell Transplantation for Relapsed/Refractory B-Cell Acute Lymphoblastic Leukemia: An Open-Label Pragmatic Clinical Trial. Am J Hematol (2019) 94(10):1113–22. doi: 10.1002/ajh.25582
14. Lee DW, Stetler-Stevenson M, Yuan CM, Shah NN, Delbrook C, Yates B, et al. Long-Term Outcomes Following CD19 CAR T Cell Therapy for B-ALL Are Superior in Patients Receiving a Fludarabine/Cyclophosphamide Preparative Regimen and Post-CAR Hematopoietic Stem Cell Transplantation. Blood (2016) 128(22):218–8. doi: 10.1182/blood.V128.22.218.218
15. Eapen M, Rocha V, Sanz G, Scaradavou A, Zhang M-J, Arcese W, et al. Effect of Graft Source on Unrelated Donor Haemopoietic Stem-Cell Transplantation in Adults With Acute Leukaemia: A Retrospective Analysis. Lancet Oncol (2010) 11(7):653–60. doi: 10.1016/s1470-2045(10)70127-3
16. Sun G, Tang B, Wan X, Yao W, Song K, Tu M, et al. CAR-T Cell Therapy Followed by Unrelated Cord Blood Transplantation for the Treatment of Relapsed/Refractory B-Cell ALL in Children and Young Adults: Superior Survival But Relatively High Posttransplant Relapse. Transplant Cell Ther (2021) 28(2):71.e1–8. doi: 10.1016/j.jtct.2021.11.011
17. Zhu X, Huang L, Zheng C, Tang B, Liu H, Geng L, et al. European Group for Blood and Marrow Transplantation Risk Score Predicts the Outcome of Patients With Acute Leukemia Receiving Single Umbilical Cord Blood Transplantation. Biol Blood Marrow Transplant (2017) 23(12):2118–26. doi: 10.1016/j.bbmt.2017.08.011
18. Schoemans HM, Lee SJ, Ferrara JL, Wolff D, Levine JE, Schultz KR, et al. EBMT-NIH-CIBMTR Task Force Position Statement on Standardized Terminology & Guidance for Graft-Versus-Host Disease Assessment. Bone Marrow Transplant (2018) 53(11):1401–15. doi: 10.1038/s41409-018-0204-7
19. Jagasia MH, Greinix HT, Arora M, Williams KM, Wolff D, Cowen EW, et al. National Institutes of Health Consensus Development Project on Criteria for Clinical Trials in Chronic Graft-Versus-Host Disease: I. The 2014 Diagnosis and Staging Working Group Report. Biol Blood Marrow Transplant (2015) 21(3):389–401.e381. doi: 10.1016/j.bbmt.2014.12.001
20. Maude SL, Frey N, Shaw PA, Aplenc R, Barrett DM, Bunin NJ, et al. Chimeric Antigen Receptor T Cells for Sustained Remissions in Leukemia. N Engl J Med (2014) 371(16):1507–17. doi: 10.1056/NEJMoa1407222
21. Sotillo E, Barrett DM, Black KL, Bagashev A, Oldridge D, Wu G, et al. Convergence of Acquired Mutations and Alternative Splicing of CD19 Enables Resistance to CART-19 Immunotherapy. Cancer Discov (2015) 5(12):1282–95. doi: 10.1158/2159-8290.CD-15-1020
22. Grupp SA, Kalos M, Barrett D, Aplenc R, Porter DL, Rheingold SR, et al. Chimeric Antigen Receptor-Modified T Cells for Acute Lymphoid Leukemia. N Engl J Med (2013) 368(16):1509–18. doi: 10.1056/NEJMoa1215134
23. Jacoby E, Nguyen SM, Fountaine TJ, Welp K, Gryder B, Qin H, et al. CD19 CAR Immune Pressure Induces B-Precursor Acute Lymphoblastic Leukaemia Lineage Switch Exposing Inherent Leukaemic Plasticity. Nat Commun (2016) 7:12320. doi: 10.1038/ncomms12320
24. Hamieh M, Dobrin A, Cabriolu A, van der Stegen SJC, Giavridis T, Mansilla-Soto J, et al. CAR T Cell Trogocytosis and Cooperative Killing Regulate Tumour Antigen Escape. Nature (2019) 568(7750):112–6. doi: 10.1038/s41586-019-1054-1
25. Guedan S, Posey AD Jr, Shaw C, Wing A, Da T, Patel PR, et al. Enhancing CAR T Cell Persistence Through ICOS and 4-1BB Costimulation. JCI Insight (2018) 3(1):e96976. doi: 10.1172/jci.insight.96976
26. Tengfei Z, Ling C, Jing X, Ni S, Zhen Z, Zhenzhen L, et al. Efficiency of CD19 Chimeric Antigen Receptor-Modified T Cells for Treatment of B Cell Malignancies in Phase I Clinical Trials a Meta-Analysis. Oncotarget (2015) 6(32):33961–71. doi: 10.18632/oncotarget.5582
27. Gardner R, Annesley C, Finney O, Summers C, Lamble AJ, Rivers J, et al. Early Clinical Experience of CD19 X CD22 Dual Specific CAR T Cells for Enhanced Anti-Leukemic Targeting of Acute Lymphoblastic Leukemia. Blood (2018) 132(Supplement 1):278–8. doi: 10.1182/blood-2018-99-113126
28. Huang L, Wang N, Li C, Cao Y, Xiao Y, Xiao M, et al. Sequential Infusion of Anti-CD22 and Anti-CD19 Chimeric Antigen Receptor T Cells for Adult Patients With Refractory/Relapsed B-Cell Acute Lymphoblastic Leukemia. Blood (2017) 130(Supplement 1):846–6. doi: 10.1182/blood.V130.Suppl_1.846.846
29. Shalabi H, Delbrook C, Stetler-Stevenson M, Yuan C, Steinberg SM, Yates B, et al. Chimeric Antigen Receptor T-Cell (CAR-T) Therapy can Render Patients With ALL Into PCR-Negative Remission and can be an Effective Bridge to Transplant (HCT). Biol Blood Marrow Transplantation (2018) 24(3):S25–6. doi: 10.1016/j.bbmt.2017.12.018
30. Yang J, Li J, Zhang X, LV F, Guo X, Wang Q, et al. A Feasibility and Safety Study of CD19 and CD22 Chimeric Antigen Receptors-Modified T Cell Cocktail for Therapy of B Cell Acute Lymphoblastic Leukemia. Blood (2018) 132(Supplement 1):277–7. doi: 10.1182/blood-2018-99-114415
31. Gardner R, Wu D, Cherian S, Fang M, Hanafi L-A, Finney O, et al. Acquisition of a CD19-Negative Myeloid Phenotype Allows Immune Escape of MLL-Rearranged B-ALL From CD19 CAR-T-Cell Therapy. Blood (2016) 127(20):2406–10. doi: 10.1182/blood-2015-08-665547
32. Brentjens RJ, Davila ML, Riviere I, Park J, Wang X, Cowell LG, et al. CD19-Targeted T Cells Rapidly Induce Molecular Remissions in Adults With Chemotherapy-Refractory Acute Lymphoblastic Leukemia. Sci Transl Med (2013) 5(177):177ra138. doi: 10.1126/scitranslmed.3005930
33. Lee DW, Kochenderfer JN, Stetler-Stevenson M, Cui YK, Delbrook C, Feldman SA, et al. T Cells Expressing CD19 Chimeric Antigen Receptors for Acute Lymphoblastic Leukaemia in Children and Young Adults: A Phase 1 Dose-Escalation Trial. Lancet (2015) 385(9967):517–28. doi: 10.1016/s0140-6736(14)61403-3
34. Hay KA, Gauthier J, Hirayama AV, Voutsinas JM, Wu Q, Li D, et al. Factors Associated With Durable EFS in Adult B-Cell ALL Patients Achieving MRD-Negative CR After CD19 CAR T-Cell Therapy. Blood (2019) 133(15):1652–63. doi: 10.1182/blood-2018-11-883710
35. Shah BD, Ghobadi A, Oluwole OO, Logan AC, Boissel N, Cassaday RD, et al. KTE-X19 for Relapsed or Refractory Adult B-Cell Acute Lymphoblastic Leukaemia: Phase 2 Results of the Single-Arm, Open-Label, Multicentre ZUMA-3 Study. Lancet (2021) 398(10299):491–502. doi: 10.1016/s0140-6736(21)01222-8
36. Ruggeri A, Volt F, Locatelli F, Michel G, Diaz de Heredia C, Abecasis M, et al. Unrelated Cord Blood Transplantation for Acute Leukemia Diagnosed in the First Year of Life: Outcomes and Risk Factor Analysis. Biol Blood Marrow Transplant (2017) 23(1):96–102. doi: 10.1016/j.bbmt.2016.10.014
37. Rocha V, Cornish J, Sievers EL, Filipovich A, Locatelli F, Peters C, et al. Comparison of Outcomes of Unrelated Bone Marrow and Umbilical Cord Blood Transplants in Children With Acute Leukemia. Blood (2001) 97(10):2962–71. doi: 10.1182/blood.V97.10.2962
38. Zhang H, Chen J, Que W. A Meta-Analysis of Unrelated Donor Umbilical Cord Blood Transplantation Versus Unrelated Donor Bone Marrow Transplantation in Acute Leukemia Patients. Biol Blood Marrow Transplant (2012) 18(8):1164–73. doi: 10.1016/j.bbmt.2012.01.015
39. Eapen M, Rubinstein P, Zhang MJ, Stevens C, Kurtzberg J, Scaradavou A, et al. Outcomes of Transplantation of Unrelated Donor Umbilical Cord Blood and Bone Marrow in Children With Acute Leukaemia: A Comparison Study. Lancet (2007) 369(9577):1947–54. doi: 10.1016/S0140-6736(07)60915-5
40. Kebriaei P, Singh H, Huls MH, Figliola MJ, Bassett R, Olivares S, et al. Phase I Trials Using Sleeping Beauty to Generate CD19-Specific CAR T Cells. J Clin Invest (2016) 126(9):3363–76. doi: 10.1172/JCI86721
41. Kalos M, Levine BL, Porter DL, Katz S, Grupp SA, Bagg A, et al. T Cells With Chimeric Antigen Receptors Have Potent Antitumor Effects and can Establish Memory in Patients With Advanced Leukemia. Sci Transl Med (2011) 3(95):95ra73. doi: 10.1126/scitranslmed.3002842
42. Ghosh A, Smith M, James SE, Davila ML, Velardi E, Argyropoulos KV, et al. Donor CD19 CAR T Cells Exert Potent Graft-Versus-Lymphoma Activity With Diminished Graft-Versus-Host Activity. Nat Med (2017) 23(2):242–9. doi: 10.1038/nm.4258
43. Eyquem J, Mansilla-Soto J, Giavridis T, van der Stegen SJ, Hamieh M, Cunanan KM, et al. Targeting a CAR to the TRAC Locus With CRISPR/Cas9 Enhances Tumour Rejection. Nature (2017) 543(7643):113–7. doi: 10.1038/nature21405
44. Gleißner B, Gökbuget N, Bartram CR, Janssen B, Rieder H, Janssen JWG, et al. Leading Prognostic Relevance of the BCR-ABL Translocation in Adult Acute B-Lineage Lymphoblastic Leukemia: A Prospective Study of the German Multicenter Trial Group and Confirmed Polymerase Chain Reaction Analysis. Blood (2002) 99(5):1536–43. doi: 10.1182/blood.V99.5.1536
45. Schlieben S, Borkhardt A, Reinisch I, Ritterbach J, Janssen JW, Ratei R, et al. Incidence and Clinical Outcome of Children With BCR/ABL-Positive Acute Lymphoblastic Leukemia (ALL). A Prospective RT-PCR Study Based on 673 Patients Enrolled in the German Pediatric Multicenter Therapy Trials ALL-BFM-90 and CoALL-05-92. Leukemia (Basingstoke) (1996) 10(6):957–63.
46. Iqbal Z, Akhtar T, Awan T, Aleem A, Sabir N, Rasool M, et al. High Frequency and Poor Prognosis of Late Childhood BCR-ABL-Positive and MLL-AF4-Positive ALL Define the Need for Advanced Molecular Diagnostics and Improved Therapeutic Strategies in Pediatric B-ALL in Pakistan. Mol Diagn Ther (2015) 19(5):277–87. doi: 10.1007/s40291-015-0149-0
47. Forero-Castro M, Robledo C, Benito R, Bodega-Mayor I, Rapado I, Hernandez-Sanchez M, et al. Mutations in TP53 and JAK2 are Independent Prognostic Biomarkers in B-Cell Precursor Acute Lymphoblastic Leukaemia. Br J Cancer (2017) 117(2):256–65. doi: 10.1038/bjc.2017.152
48. Foa R, Vitale A, Mancini M, Cuneo A, Mecucci C, Elia L, et al. E2A-PBX1 Fusion in Adult Acute Lymphoblastic Leukaemia: Biological and Clinical Features. Br J Haematol (2003) 120(3):484–7. doi: 10.1046/j.1365-2141.2003.04113.x
49. Gökbuget N, Hoelzer D. Treatment of Adult Acute Lymphoblastic Leukemia. Semin Hematol (2009) 46(1):64–75. doi: 10.1053/j.seminhematol.2008.09.003
50. Long AH, Haso WM, Shern JF, Wanhainen KM, Murgai M, Ingaramo M, et al. 4-1BB Costimulation Ameliorates T Cell Exhaustion Induced by Tonic Signaling of Chimeric Antigen Receptors. Nat Med (2015) 21(6):581–90. doi: 10.1038/nm.3838
51. Zhao Z, Condomines M, van der Stegen SJC, Perna F, Kloss CC, Gunset G, et al. Structural Design of Engineered Costimulation Determines Tumor Rejection Kinetics and Persistence of CAR T Cells. Cancer Cell (2015) 28(4):415–28. doi: 10.1016/j.ccell.2015.09.004
52. Davila ML, Riviere I, Wang X, Bartido S, Park J, Curran K, et al. Efficacy and Toxicity Management of 19-28z CAR T Cell Therapy in B Cell Acute Lymphoblastic Leukemia. Sci Transl Med (2014) 6(224):224ra25. doi: 10.1126/scitranslmed.3008226
53. Jacoby E, Bielorai B, Avigdor A, Itzhaki O, Hutt D, Nussboim V, et al. Locally Produced CD19 CAR T Cells Leading to Clinical Remissions in Medullary and Extramedullary Relapsed Acute Lymphoblastic Leukemia. Am J Hematol (2018) 93(12):1485–92. doi: 10.1002/ajh.25274
54. Shah NN, Lee DW, Yates B, Yuan CM, Shalabi H, Martin S, et al. Long-Term Follow-Up of CD19-CAR T-Cell Therapy in Children and Young Adults With B-ALL. J Clin Oncol (2021) 39(15):1650–9. doi: 10.1200/JCO.20
Keywords: chimeric antigen receptor (CAR), unrelated cord blood transplantation, acute B lymphoblastic leukemia, CD19, prognosis
Citation: Xu Q, Xue L, An F, Xu H, Wang L, Geng L, Zhang X, Song K, Yao W, Wan X, Tong J, Liu H, Liu X, Zhu X, Zhai Z, Sun Z and Wang X (2022) Impact of Consolidative Unrelated Cord Blood Transplantation on Clinical Outcomes of Patients With Relapsed/Refractory Acute B Lymphoblastic Leukemia Entering Remission Following CD19 Chimeric Antigen Receptor T Cells. Front. Immunol. 13:879030. doi: 10.3389/fimmu.2022.879030
Received: 18 February 2022; Accepted: 28 March 2022;
Published: 26 April 2022.
Edited by:
Trent Spencer, Emory University, United StatesReviewed by:
Xiao-Dong Mo, Peking University People’s Hospital, ChinaCopyright © 2022 Xu, Xue, An, Xu, Wang, Geng, Zhang, Song, Yao, Wan, Tong, Liu, Liu, Zhu, Zhai, Sun and Wang. This is an open-access article distributed under the terms of the Creative Commons Attribution License (CC BY). The use, distribution or reproduction in other forums is permitted, provided the original author(s) and the copyright owner(s) are credited and that the original publication in this journal is cited, in accordance with accepted academic practice. No use, distribution or reproduction is permitted which does not comply with these terms.
*Correspondence: Xingbing Wang, d2FuZ3hpbmdiaW5nQHVzdGMuZWR1LmNu
†These authors have contributed equally to this work and share first authorship
Disclaimer: All claims expressed in this article are solely those of the authors and do not necessarily represent those of their affiliated organizations, or those of the publisher, the editors and the reviewers. Any product that may be evaluated in this article or claim that may be made by its manufacturer is not guaranteed or endorsed by the publisher.
Research integrity at Frontiers
Learn more about the work of our research integrity team to safeguard the quality of each article we publish.