- Tumor Vaccines and Biotechnology Branch, Division of Cellular and Gene Therapies, Office of Tissues and Advanced Therapies, Center for Biologics Evaluation and Research, Food and Drug Administration, Silver Spring, MD, United States
Interleukin-13 receptor subunit alpha-2 (IL-13Rα2, CD213A), a high-affinity membrane receptor of the anti-inflammatory Th2 cytokine IL-13, is overexpressed in a variety of solid tumors and is correlated with poor prognosis in glioblastoma, colorectal cancer, adrenocortical carcinoma, pancreatic cancer, and breast cancer. While initially hypothesized as a decoy receptor for IL-13-mediated signaling, recent evidence demonstrates IL-13 can signal through IL-13Rα2 in human cells. In addition, expression of IL-13Rα2 and IL-13Rα2-mediated signaling has been shown to promote tumor proliferation, cell survival, tumor progression, invasion, and metastasis. Given its differential expression in tumor versus normal tissue, IL-13Rα2 is an attractive immunotherapy target, as both a targetable receptor and an immunogenic antigen. Multiple promising strategies, including immunotoxins, cancer vaccines, and chimeric antigen receptor (CAR) T cells, have been developed to target IL-13Rα2. In this mini-review, we discuss recent developments surrounding IL-13Rα2-targeted therapies in pre-clinical and clinical study, including potential strategies to improve IL-13Rα2-directed cancer treatment efficacy.
Introduction
Cytokine receptor expression is tightly regulated; however, cancer cells can overexpress cytokine receptors to promote tumor development, progression, and immune evasion. Cytokine receptors are attractive cancer therapy targets given the differential expression on normal vs. tumor tissue, the capacity to alter tumor cell fitness and function via receptor modulation/signaling, and variety of strategies available for selective targeting.
Interleukin-13 receptor subunit alpha-2 (IL-13Rα2) is a high-affinity membrane receptor for the anti-inflammatory cytokine interleukin 13 (IL-13). IL-13Rα2 was originally considered a decoy receptor that sequestered IL-13 and inhibited signaling since: i) IL-13Rα2 has a short cytoplasmic tail and cannot signal through canonical JAK/STAT signaling pathway (1); ii) IL-13 has higher affinity for IL-13Rα2 than its other receptor, the interleukin 13 receptor subunit alpha 1/interleukin 4 receptor subunit alpha (IL-4Rα/IL-13Rα1) heterodimer (2); and iii) overexpression of IL-13Rα2 can inhibit IL-13 signaling (3). However, recent studies demonstrated that IL-13-mediated IL-13Rα2 signaling occurs via STAT6-independent pathways, involving activation of activator protein 1 (AP-1) and extracellular signal-related kinase (ERK), promoting tumor invasion, metastasis, and production of transforming growth factor beta (TGFβ) (4–9). Differential binding of IL-13 by IL-13Rα1 and IL-13Rα2 has been discussed elsewhere (10, 11). More recently, Chitinase 3-like-1 (CHI3L1) was also identified as an IL-13Rα2 ligand, and a membrane protein TMEM219 was shown to be involved in IL13-Rα2 signaling (12, 13). CHI3L1 binding induces activation of mitogen-activated protein kinase (MAPK), protein kinase B (PKB)/Akt, and/or Wnt/β-catenin signaling to promote TGFβ production and tumor metastasis (12–14).
IL-13Rα2 is overexpressed in melanoma (8, 15), renal cell carcinoma (RCC) (16), adrenocortical carcinoma (ACC) (17, 18), and a variety of brain tumors (19–21). Additionally, IL-13Rα2 overexpression correlates with advanced disease and poor prognosis in colorectal carcinoma (CRC) (22), gastric cancer (23), breast cancer (24, 25), clear cell ovarian cancer (26), lung cancer (27), ACC (28), papillary thyroid cancer (29), pancreatic ductal adenocarcinoma (30), and glioblastoma (GBM) (31–33). While IL-13Rα2 is a biomarker of prognosis for many solid tumors after therapeutic intervention, research has focused on GBM. GBM is the most common and aggressive malignant primary brain tumor in humans, and the existing treatments (i.e., tumor resection, radiotherapy, temozolomide) have limited impact on patient survival (34). IL-13Rα2 is overexpressed in ~76% of GBM but is not detected in normal brain tissue, making it a highly selective immunotherapy target (32, 33, 35).
In this mini-review, we focus on recent research advances for IL-13Rα2-targeted therapies. An overview of the therapies discussed and advantages/disadvantages of each are summarized in Figure 1. In addition, we discuss strategies to improve therapy efficacy and remaining questions in the field.
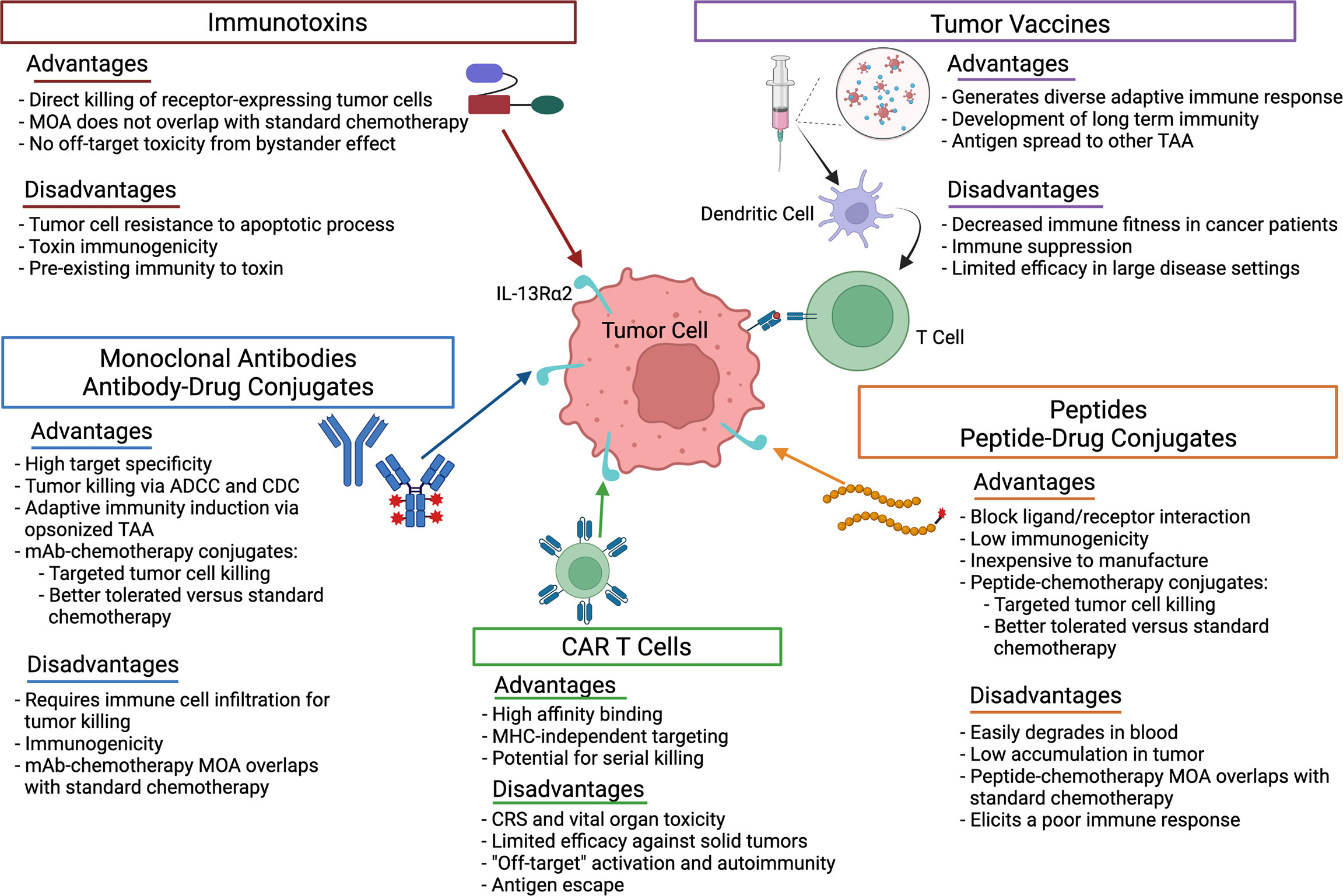
Figure 1 Advantages and disadvantages of the therapeutic strategies used to target IL-13Rα2 in cancer. ADCC, antibody-dependent cellular cytotoxicity; CDC, complement-dependent cytotoxicity; CRS, cytokine release syndrome; mAb, monoclonal antibody; MHC, major histocompatability complex; MOA, mechanism of action; TAA, tumor associated antigen.
IL-13Rα2-Targeted Immunotoxins
Recombinant immunotoxins were the first strategy to target IL-13Rα2 in cancer. These chimeric fusion proteins kill cancer cells via receptor binding, receptor internalization, and cleavage of the toxin moiety in the cell cytosol, which inhibits protein synthesis and induces apoptosis (36). To induce IL-13Rα2-directed killing of tumor cells, IL-13 was fused to truncated Pseudomonas aeruginosa exotoxin A (PE) (37) or diphtheria toxin (DT) (38).
IL13-PE (IL13-PE38QQR or cintredekin besudotox) has been used extensively in preclinical and several Phase 1-3 clinical studies. The PE38QQR exotoxin is mutated to prevent ubiquitous eukaryotic cell targeting via α2-macroglobulin and to enhance endoplasmic reticulum localization for production (36, 39). IL13-PE is highly cytotoxic to human solid tumor cell lines, including those derived from RCC, GBM, and head and neck squamous cell carcinoma (HNSCC) (32, 37, 40–42). IL13-PE cytotoxicity is correlated with expression of IL-13Rα2 (40, 43), and lack of expression on normal cells confers significant if not complete resistance to IL13-PE (43–45). IL13-PE treatment of orthotopic GBM and pancreatic xenografts significantly reduced tumor burden and increased overall survival (20, 45, 46). IL13-PE also decreased subcutaneous pheochromocytoma, pancreatic, and ACC xenograft tumor burden (18, 47, 48).
After intracerebral convection-enhanced delivery (CED) of IL13-PE was determined to be feasible and safe in Phase 1/2 clinical trials (49, 50), a randomized Phase 3 trial (PRECISE) with intraparenchymal IL-13PE administration was initiated in GBM patients. IL13-PE was well-tolerated but showed similar overall survival to carmustine-releasing Gliadel wafers, the only FDA-approved local treatment for recurrent GBM (51). During retrospective analysis, it was determined that only ~50% of patients had fully conforming catheters in respect to overall placement, and optimally positioned catheters had larger coverage volumes with regard to drug delivery (52, 53). Thus, efficacy of IL13-PE may have been severely constrained by delivery and diffusion issues in the PRECISE trial. Similarly, in a Phase 1 clinical trial in children with diffuse intrinsic pontine glioma, CED-delivered IL13-PE did not reach the entire MRI-defined tumor volume in any patient. Even with these constraints with drug delivery/diffusion, IL13-PE temporarily arrested disease progression in 2 of 5 patients and was well-tolerated (54). Additional clinical studies are needed to optimize distribution of CED-delivered IL13-PE.
Outside of brain malignancies, Phase 1 clinical trials using intravenous IL13-PE were initiated in RCC (55) and metastatic ACC with confirmed expression of IL-13Rα2 (56). In the ACC trial, dose limiting toxicities, including Grade 3 anemia, were observed at the higher dose (2 μg/kg) of IL13-PE. While the lower dose (1 μg/kg) of IL13-PE was well-tolerated, neutralizing antibodies were observed in all patients (56). It was not reported whether neutralizing antibodies were generated against both PE and IL-13, which will be important to identify potential toxicities. Additional clinical trials could not be performed due to lack of availability of clinical grade IL13-PE. However, to address immunogenicity issues (57), future studies should consider pre-treatment lymphodepletion, immunosuppression, or utilize a less immunogenic immunotoxin.
Two DT-conjugated IL-13 immunotoxins, DTIL13 and DT-IL13QM, also demonstrated cytotoxicity to GBM cell lines (38, 58, 59). In mice with orthotopic GBM xenografts, DTIL13 treatment significantly improved survival in a dose-dependent manner (38, 59). To improve tumor targeting and address antigen heterogeneity, bispecific immunotoxins were developed. Todhunter et al. synthesized DTAT13, which simultaneously targets overexpressed IL-13R and urokinase-type plasminogen activator receptor (60). Small subcutaneous GBM xenografts underwent regression for 40-50 days with DTAT13. Intracranial DTAT13 also had less toxicity versus DTIL13 (60). Stish et al. generated a bispecific DTEGF13 immunotoxin that targets IL-13R and epidermal growth factor (EGF) (61). Compared to monospecific immunotoxins, DTEGF13 increased cytotoxicity and reduced the growth of established prostate cancer xenografts (61). CED DTEGF13 promoted similar results in rats bearing orthotopic GBM xenografts (62). Since DT is derived from a bacterial toxin and most adults are vaccinated against diphtheria, patients may have pre-existing immunity to DT and/or quickly develop neutralizing antibodies to IL13-DT (63). Thus, similar to PE, lymphodepletion, immunosuppression, or de-immunization strategies may be needed to increase the therapeutic window of DT-conjugated immunotoxins.
IL-13Rα2-Specific CAR-T Cells
Chimeric antigen receptor (CAR) T cell therapy is a promising treatment approach for many malignancies. First generation CARs contain a synthetic receptor typically consisting of a tumor associated antigen (TAA)-targeted extracellular single chain variable fragment (scFv), a transmembrane domain, and only the TCR CD3ζ signaling domain. Second and third generation CARs carry one or multiple costimulatory domains, respectively (64, 65). CAR T cells have produced remarkably effective and durable clinical results. Five second-generation CAR T therapies are approved by the FDA for the treatment of B-cell hematological malignancies (66). The application of CAR T cells in solid malignancies has presented many challenges, and efficacy has been limited. Regardless, IL-13Rα2-targeted CAR T cells are under investigation in six clinical trials in primary CNS malignancies and one in melanoma (clinicaltrials.gov, Table 1). Two clinical trials in primary CNS malignancies have been completed (clinicaltrials.gov, Table 2).
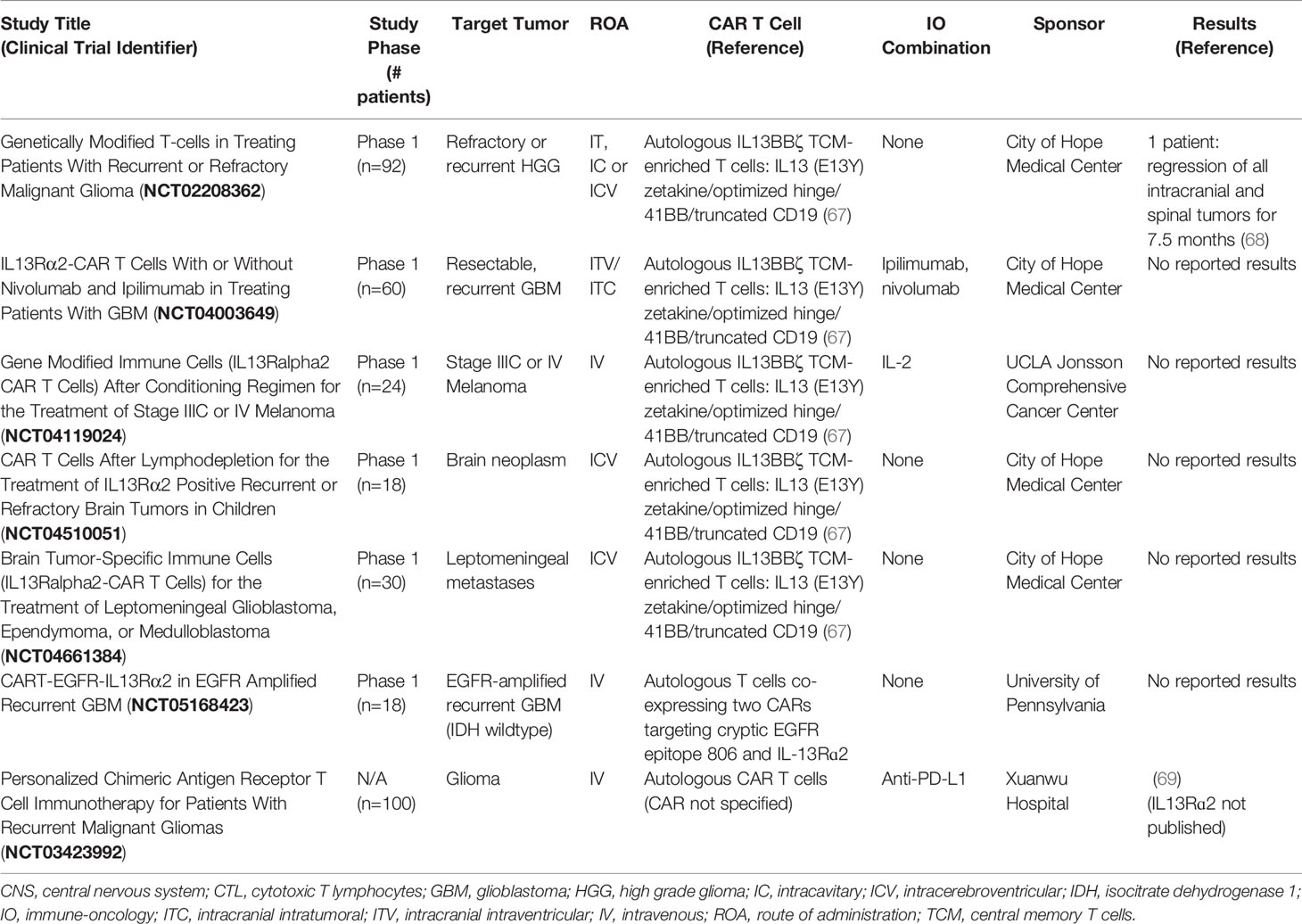
Table 1 Ongoing IL-13Rα2-targeted CAR T cell therapy clinical trials (from clinicaltrials.gov).
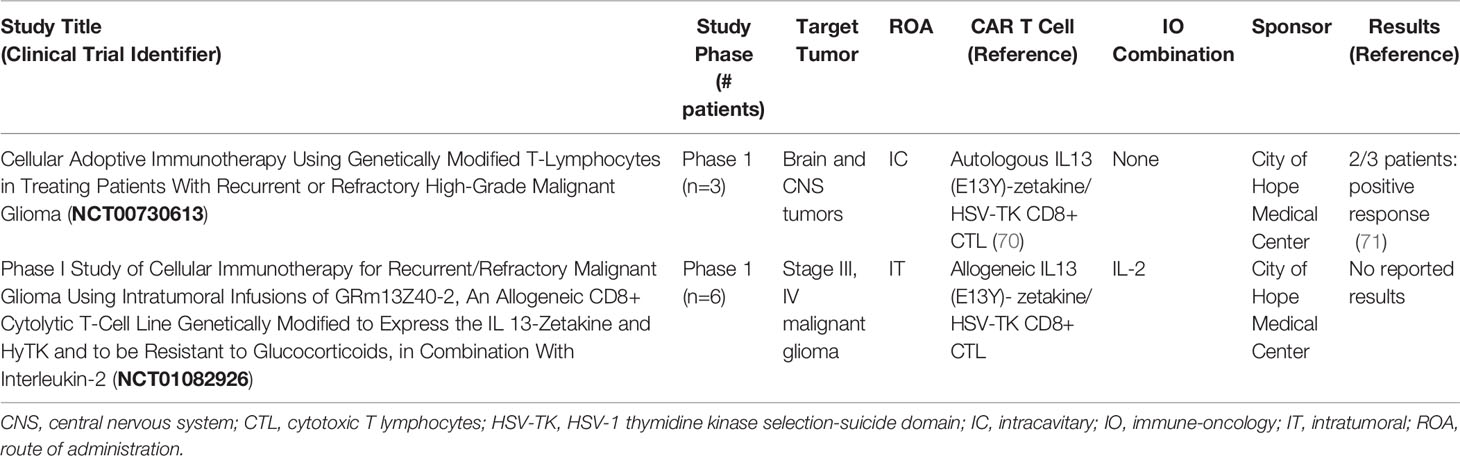
Table 2 Completed IL-13Rα2-targeted CAR T cell therapy clinical trials (from clinicaltrials.gov).
First generation IL-13(E13Y) zetakine CAR T cells (IL13-zetakine CTL (cytotoxic T lymphocytes)) utilized a membrane-tethered, mutated IL-13 (E13Y) instead of scFv to redirect T cells to IL-13Rα2 and reduce cross-reactivity with IL-13Rα1. Initial studies demonstrated remarkable efficacy of IL13-zetakine CTL against human GBM orthotopic xenografts and no-cross reactivity with IL-13Rα1 (70). However, additional studies suggested IL13-zetakine CTLs do recognize IL-13Rα1-positive targets, which could lead to off-target toxicities (71–73). The first-in-human trial using intracranial delivery of first generation IL13-zetakine CAR T cells demonstrated that repeated administration was well-tolerated, and two of three patients underwent transient anti-glioma responses. Durable responses were not observed, which correlated with short CAR T cell persistence (71).
Second-generation IL13BBζ CAR T cells were genetically engineered to incorporate IL-13 (E13Y-mutated), 4-1BB (CD137), and mutated IgG4-Fc linker, which resulted in the enrichment of central memory T cells (TCM). In orthotopic human GBM models, anti-tumor activity and T cell persistence were significantly improved in IL13BBζ (67). IL13BBζ CAR T cells are currently in clinical trials. In one patient, multiple intracranial infusions of IL13BBζ CAR T cells over 220 days were well-tolerated, increased cytokine levels and immune cell frequencies were observed in the cerebrospinal fluid, and the patient underwent regression for 7.5 months (68). While none of the initial tumors recurred, preliminary results suggest recurrence of new tumors with reduced expression of IL-13Rα2. Thus, antigen escape may be a significant issue against the generation of durable responses.
More recently, to further reduce cross-reactivity with IL-13Rα1, Kim et al. reported the generation of YYB-103, a mutated IL-13-based CAR with multiple amino acid substitutions (E13K, R66D, S69D, and R109K) (74). YYB-103 was more selective than IL-13(E13Y) zetakine CTL for IL-13Rα2. Intracranial and intravenous administration of YYB-103 reduced orthotopic GBM xenograft tumor burden and increased survival (74).
On the other hand, Krenciute et al. constructed a panel of IL-13Rα2-specific CARs containing an anti-IL-13Rα2-scFv instead of a mutated IL-13, short or long spacer regions, a transmembrane domain, endodomains derived from costimulatory molecules CD28, 4-1BB, or OX40, and CD3ζ (75). In a murine GBM study, IL-13Rα2-CAR T cells with short spacer regions and CD28ζ, 4-1BBζ, and CD28.OX40ζ displayed potent anti-glioma activity with high specificity for IL-13Rα2 and no cross-reactivity to IL-13Rα1 (75). CD28ζ CAR T cells persisted and proliferated in the brains of glioma-bearing mice after intracranial administration (76). Importantly, mice that underwent complete IL-13Rα2-positive tumor regression were also protected against rechallenge with IL-13Rα2-negative tumors, suggesting that the IL-13Rα2-specific CARs assist in the development of diversified long-term anti-glioma immunity (76).
Given the heterogeneity of antigens on GBM tumors, multi-antigen-targeted CAR molecules may enhance efficacy and mitigate antigen escape observed with unispecific IL13BBζ CAR T cells (68). Hegde et al. discovered that 96% of patient GBM tumors expressed human epidermal growth factor 2 (HER2), IL-13Rα2, or ephrin-A2 (EphA2) (77). Co-expression of HER2- and IL-13Rα2-specific CARs enhanced T cell functionality against autologous glioma cells. Compared with individual or pooled monospecific CAR T cells, HER2/IL-13Rα2 CARs improved tumor control and survival in a human orthotopic GBM model (77). TanCAR, a CAR T cell containing a bispecific HER2-binding scFv/mutated IL-13 heterodimer CAR, also improved survival in a GBM model verses monospecific CAR T cells (78). To further improve the efficacy of TanCAR T cells, Bielamowicz et al. generated trivalent CAR T cells using a single universal tricistronic transgene to co-express CARs specific for HER2, IL-13Rα2, and EphA2 on patient T cells (UCAR T cells) (79). All three CARs were successfully co-expressed and had antigen-specific functionality. In a tumor model using patient GBM xenografts and patient-matched T cells, UCAR T cells derived from 2/2 patients decreased tumor burden and increased survival versus univalent CAR T cells. Similar results were observed for UCAR T cells derived from 1/2 patients when compared to bivalent CAR T cells (79).
IL-13Rα2-Targeted Peptides and Inhibitors of IL-13Rα2 Signaling
Peptides targeting IL-13Rα2 can be used to block native ligand-receptor interaction/signaling and/or deliver conjugated therapeutic agents to the tumor microenvironment (TME). Compared to antibodies, peptides display lower immunogenicity, better tumoral diffusion due to low molecular weight, and are easier and inexpensive to synthesize (80). Various techniques are used to improve peptide stability in biological fluids and increase accumulation in the tumor, including the use of D-amino acids and PEGylation (80).
Using phage display technology, Pandaya et al. identified Pep-1L peptide that binds specifically to IL-13Rα2 via a non-competitive binding site for IL-13 (81). Intravenous Pep-1L accumulated in both subcutaneous and orthotopic GBM tumors (81–83), indicating that Pep-1L efficiently crosses the blood-brain-tumor barrier. Pep-1L binding also induced IL-13Rα2 internalization (81, 84), making it an attractive candidate to deliver cytotoxic agents to IL-13Rα2-expressing tumors. Indeed, intracranial CED of a Pep-1L-alpha particle emitter conjugate promoted GBM cytotoxicity and increased overall survival in an orthotopic murine GBM model (85). Similarly, Pep-1L-paclitaxel (chemotherapy) nanoparticle conjugates reduced intracranial glioma growth and increased overall survival (83).
Bartolome et al. used the recently elucidated structure of the IL-13/IL-13Rα2 complex to engineer a 12-mer peptide (D1) that specifically blocks the IL-13/IL-13Rα2 signaling axis (86). D1 peptide inhibited IL-13Rα2-mediated signaling to a greater extent than IL-13Rα1 signaling. D1 also significantly reduced IL-13-mediated binding, cell migration, and invasion of CRC and GBM cell lines, and increased survival in xenograft models (86).
Recent insights into the downstream signaling of IL-13Rα2 have also allowed for targeting of IL-13Rα2-mediated signaling by small molecule inhibitors. Bartolome et al. identified protein tyrosine phosphatase-1B (PTP1B) as a mediator of IL-13Rα2 signaling (87). PTP1B is overexpressed in many tumor types, and high expression correlated with reduced overall survival of GBM, CRC, and ovarian cancer patients. Use of the PTP1B inhibitor, Claramine significantly reduced tumor burden, metastasis and increased survival in mice with CRC and GBM xenografts (87).
IL-13Rα2-Directed Monoclonal Antibodies
Many agents targeting IL-13Rα2 lack the selectivity to bind IL-13Rα2 but not IL-13Rα1. Monoclonal antibodies (mAbs) bind targets with high affinity/specificity and mediate efficacy via: i) manipulation/inhibition of critical signaling pathways required for the malignant phenotype; ii) initiation of antibody-dependent cellular cytotoxicity (ADCC); iii) complement-dependent cytotoxicity (CDC) by complement activation; and/or iv) increased presentation of opsonized antigens by antigen presenting cells (APC) (88, 89).
In order to target IL-13Rα2 but not IL-13Rα1, Balyasnikova et al. characterized a novel antibody that blocks IL-13/IL-13Rα2 interaction (90). This mAb bound IL-13Rα2 in GBM tissue and improved survival of mice with orthotopic human glioma xenografts (90). Using a similar strategy, Jaen et al. generated a D1 peptide-specific mAb that blocked IL-13/IL-13Rα2 -mediated signaling (91). Correlating with inhibition of IL-13-mediated CRC cell migration and invasion in vitro, the D1-specific mAb also reduced liver metastasis of CRC tumors and improved survival (91).
Like peptides, mAbs can be conjugated to other therapeutic agents. Biodistribution studies performed by Gupta et al. demonstrated that intravenous IL-13Rα2 mAb led to a time-dependent, selective accumulation of mAb in IL-13Rα2-expressing tumors (91). Accumulation was not affected by conjugation to auristatin, an antimitotic agent (92, 93). The mAb-auristatin conjugate significantly reduced melanoma xenograft growth in a dose-dependent manner, and 90% of mice underwent complete tumor rejection at the highest dose (93). Interestingly, conjugation of another IL-13Rα2 mAb to auristatin did not impact growth of certain IL-13Rα2-expressing diffuse intrinsic pontine glioma (DIPG) cell lines (94), suggesting selection of appropriate drug conjugates is critical for efficacy in different tumor settings.
IL-13Rα2-Targeted Therapeutic Cancer Vaccines
In contrast to passive therapeutic approaches like peptides or mAb, therapeutic cancer vaccines can promote the development of a diverse, long-term immune response against TAA. Therapeutic cancer vaccines can consist of whole tumor cells, tumor cell lysate or peptides/proteins mixed with an adjuvant, viruses genetically-modified to express a TAA, or TAA-pulsed APCs (95).
The most clinically advanced IL-13Rα2-directed vaccine studies utilize peptide-pulsed dendritic cells (DCs). IL-13Rα2 peptide-pulsed DCs induce T cell responses in recurrent glioma patients (96–98). However, most recent clinical trials evaluate efficacy of DCs pulsed with IL-13Rα2 antigen and other glioma-associated peptides. Okada et al. evaluated DCs loaded with four peptides, including IL-13Rα2, in combination with Poly-ICLC adjuvant (99). Nine of 22 patients achieved progression-free status lasting at least 12 months (99). After showing favorable results in a Phase 1 trial (98), ICT-107, an autologous DC vaccine with six synthetic GBM-associated peptides including IL-13Rα2, was evaluated in a double-blinded, randomized phase 2 clinical trial in newly diagnosed patients with GBM. Overall, ICT-107 was well-tolerated and increased progression-free survival by 2.2 months (100). A Phase 3 trial of ICT-107 has been established (NCT02546102).
Vaccination with IL-13Rα2 DNA significantly reduced murine syngeneic tumor growth through induction of T and B cell responses (101, 102). Anti-tumor efficacy was enhanced with IL-13Rα2 DNA priming plus a protein/adjuvant boost consisting of the extracellular domain of IL-13Rα2 protein, CpG, and incomplete Freund’s adjuvant (IFA) (103). Human studies have not been initiated. Instead of using DNA vaccination, Pollack et al. immunized children with newly diagnosed diffuse brainstem and high-grade gliomas using three glioma-associated antigens, including IL-13Rα2, in combination with Poly ICLC adjuvant (104). Anti-glioma antigen immune responses to IL-13Rα2 were observed in 10 of 13 evaluable patients. Two patients had prolonged disease-free status after surgery (104).
Conclusions and Future Perspectives
Over the past 20 years, IL-13Rα2 has been confirmed as an effective target for novel cancer therapies. We predict that investigation of IL-13Rα2-targeted therapies will continue in the clinic, with increased treatment of solid tumors outside of gliomas. While transient improvements in patient outcomes have been observed with IL-13Rα2-targeted monotherapies, even in hard-to-treat tumors like GBM, the overall clinical response has been underwhelming. Thus, combinatorial approaches are likely necessary for the development of robust IL-13Rα2-targeted anti-tumor responses.
Chemotherapy is considered standard of care in many indications and works with an entirely different mechanism of action compared to targeted therapies. We have combined chemotherapy with IL-13Rα2-directed immunotoxin therapy. When pancreatic tumor cell lines were treated with gemcitabine, IL-13Rα2 was upregulated, resulting into increased IL13-PE-mediated killing in vitro and improved survival of mice implanted with pancreatic tumor xenografts (105). Similar results were observed in oral squamous cell carcinoma preclinical models (106). Thus, chemotherapy may synergize or enhance antitumor effects through upregulation of IL-13Rα2 in low expressing tumors or through additional mechanisms yet to be elucidated. However, chemotherapy/IL-13Rα2-targeted combinations may not be effective in certain indications. For example, a correlation between temozolomide resistance and IL-13Rα2 expression has been observed in GBM (31). Other therapeutic approaches like use of histone deacetylase (HDAC) inhibitors, which can cause over-expression of some tumor-associated genes, may be a viable substitute for chemotherapy combination in resistant tumors. Fujisawa et al. reported that HDAC inhibition upregulated IL-13Rα2 and increased IL13-PE-mediated responses in pancreatic tumor models (48).
While therapeutics like IL13-PE or IL-13Rα2-mAb-chemotherapy conjugates are effective in reducing tumor burden through direct cytotoxicity, combination with other IL-13Rα2-targeted therapies such as cancer vaccines or CAR T cells may be necessary to ensure long-term responses. Supporting this, IL-13Rα2 DNA vaccine/IL13-PE combination synergized to reduce murine sarcoma and breast tumor growth via multiple immune mechanisms, including direct tumor killing and increased T cell tumor infiltration (102). As an alternative to using multiple IL-13Rα2-targeted therapies, to address tumor antigen heterogeneity, it may be better to combine agents that target distinct TAA. IL-13Rα2 expression declines during IL-13Rα2-targeted therapy (71), likely due to selective killing of IL-13Rα2-expressing cells and downregulation of IL-13Rα2 as a resistance mechanism to mediate tumor escape. Utilizing therapies to target multiple TAA may prevent antigen escape; however, targeting multiple TAA, especially when using bi- or multi-specific molecules, may increase on-target, off-tumor or off-target toxicities and/or lower the maximal tolerated dose. Finally, the combination of IL13Rα2-targeted therapies with checkpoint inhibitors may reduce immunosuppression within the tumor microenvironment (TME) and improve efficacy of IL-13Rα2-specific CAR T cells (107). One recent study showed a positive correlation of IL-13Rα2 and the immune regulatory protein VISTA in oral squamous cell carcinoma (108). Other checkpoint inhibitors may also be upregulated in tandem with IL-13Rα2.
In addition to identifying the combinations to improve IL-13Rα2-targeted therapies, toxicities associated with targeting IL-13Rα2 should be considered. While many IL-13Rα2-targeted therapies are engineered to reduce cross-reactivity with IL-13Rα1, some still display such cross-reactivity. IL-13Rα1, unlike IL-13Rα2, is ubiquitously expressed in humans. As such, there is a hypothetical risk of IL-13Rα1-related off-target toxicities when using IL-13Rα2-targeted therapies. To date, these toxicities have not been observed in the clinic, but close monitoring of patients is important. Most active clinical trials deliver IL-13Rα2-targeted therapies locally in the brain, so it is possible that novel toxicities may be observed with systemic or intracavital administration.
Importantly, the on-target, off-tumor effects of IL-13Rα2-targeted therapies have not been widely investigated. Certain immune cells express IL-13Rα2, including monocytes, myeloid-derived suppressor cells (MDSC), and macrophages (6, 109–111). After IL-13Rα2-targeted CAR T cell treatment, mice with orthotopic murine gliomas have a transient reduction in MDSC number in the tumor and spleen (76). IL13-PE treatment also reduced the frequency of MDSC in the tumor and/or spleen of mice bearing syngeneic HNSCC and breast tumors (102, 112). It has not been elucidated whether reduction in MDSC prevalence is due to direct killing of IL-13Rα2-expressing MDSC or cytokine-mediated reprogramming of these cells to a different phenotype. Both mechanisms are likely, as IL-13Rα2 signaling has been linked to M2 polarization of human macrophages (6, 111). While IL-13Rα2-mediated MDSC depletion would likely improve anti-tumor responses, reduction of other IL-13Rα2-expressing immune cell populations, especially those that mediate anti-tumor efficacy, may have negative effects. Thus, immune expression of IL-13Rα2 within the TME or in tumor-bearing subjects requires further elucidation.
Author Contributions
RKP conceived, reviewed, and supervised the mini-review. KMK, SH, and MSM collected literature, wrote the review, and revised the manuscript. MSM and KMK generated the tables and figure. BHJ and SRH reviewed and edited the manuscript. All authors reviewed and approved the manuscript.
Funding
The writing of this review was supported through the intramural funding of the Office of Tissues and Advanced Therapies, Center for Biologics Evaluation and Research, FDA.
Conflict of Interest
The authors declare that the research was conducted in the absence of any commercial or financial relationships that could be construed as a potential conflict of interest.
Publisher’s Note
All claims expressed in this article are solely those of the authors and do not necessarily represent those of their affiliated organizations, or those of the publisher, the editors and the reviewers. Any product that may be evaluated in this article, or claim that may be made by its manufacturer, is not guaranteed or endorsed by the publisher.
Acknowledgments
We thank Drs. Ronit Mazor, Graeme Price, Carolyn Laurencot, and Steven Oh of the Center for Biologics Evaluation and Research, FDA, Silver Spring, for their critical review of the manuscript and for providing helpful comments. The figure was created with BioRender.com.
References
1. Roy B, Bhattacharjee A, Xu B, Ford D, Maizel AL, Cathcart MK. IL-13 Signal Transduction in Human Monocytes: Phosphorylation of Receptor Components, Association With Jaks, and Phosphorylation/Activation of Stats. J Leukoc Biol (2002) 72(3):580–9. doi: 10.1189/jlb.72.3.580
2. Donaldson DD, Whitters MJ, Fitz LJ, Neben TY, Finnerty H, Henderson SL, et al. The Murine IL-13 Receptor Alpha 2: Molecular Cloning, Characterization, and Comparison With Murine IL-13 Receptor Alpha 1. J Immunol (1998) 161(5):2317–24.
3. Zhang JG, Hilton DJ, Willson TA, McFarlane C, Roberts BA, Moritz RL, et al. Identification, Purification, and Characterization of a Soluble Interleukin (IL)-13-Binding Protein. Evidence That it is Distinct From the Cloned Il-13 Receptor and Il-4 Receptor Alpha-Chains. J Biol Chem (1997) 272(14):9474–80. doi: 10.1074/jbc.272.14.9474
4. Fujisawa T, Joshi BH, Puri RK. IL-13 Regulates Cancer Invasion and Metastasis Through IL-13rα2 via ERK/AP-1 Pathway in Mouse Model of Human Ovarian Cancer. Int J Cancer (2012) 131(2):344–56. doi: 10.1002/ijc.26366
5. Brunner SM, Schiechl G, Kesselring R, Martin M, Balam S, Schlitt HJ, et al. IL-13 Signaling via IL-13rα2 Triggers TGF-β1-Dependent Allograft Fibrosis. Transplant Res (2013) 2(1):16. doi: 10.1186/2047-1440-2-16
6. Fichtner-Feigl S, Strober W, Kawakami K, Puri RK, Kitani A. IL-13 Signaling Through the IL-13alpha2 Receptor is Involved in Induction of TGF-Beta1 Production and Fibrosis. Nat Med (2006) 12(1):99–106. doi: 10.1038/nm1332
7. Márquez-Ortiz RA, Contreras-Zárate MJ, Tesic V, Alvarez-Eraso KLF, Kwak G, Littrell Z, et al. Il13rα2 Promotes Proliferation and Outgrowth of Breast Cancer Brain Metastases. Clin Cancer Res (2021) 27(22):6209. doi: 10.1158/1078-0432.CCR-21-0361
8. Okamoto H, Yoshimatsu Y, Tomizawa T, Kunita A, Takayama R, Morikawa T, et al. Interleukin-13 Receptor α2 is a Novel Marker and Potential Therapeutic Target for Human Melanoma. Sci Rep (2019) 9(1):1281. doi: 10.1038/s41598-019-39018-3
9. Bhardwaj R, Suzuki A, Leland P, Joshi BH, Puri RK. Identification of a Novel Role of IL-13rα2 in Human Glioblastoma Multiforme: Interleukin-13 Mediates Signal Transduction Through AP-1 Pathway. J Transl Med (2018) 16(1):369. doi: 10.1186/s12967-018-1746-6
10. Junttila IS. Tuning the Cytokine Responses: An Update on Interleukin (IL)-4 and IL-13 Receptor Complexes. Front Immunol (2018) 9:888. doi: 10.3389/fimmu.2018.00888
11. Lupardus PJ, Birnbaum ME, Garcia KC. Molecular Basis for Shared Cytokine Recognition Revealed in the Structure of an Unusually High Affinity Complex Between IL-13 and IL-13ralpha2. Structure (London Engl 1993) (2010) 18(3):332–42. doi: 10.1016/j.str.2010.01.003
12. He Chuan H, Lee Chun G, Dela Cruz Charles S, Lee C-M, Zhou Y, Ahangari F, et al. Chitinase 3-Like 1 Regulates Cellular and Tissue Responses via IL-13 Receptor α2. Cell Rep (2013) 4(4):830–41. doi: 10.1016/j.celrep.2013.07.032
13. Lee CM, He CH, Nour AM, Zhou Y, Ma B, Park JW, et al. IL-13rα2 Uses TMEM219 in Chitinase 3-Like-1-Induced Signalling and Effector Responses. Nat Commun (2016) 7:12752. doi: 10.1038/ncomms12752
14. He CH, Lee CG, Ma B, Kamle S, Choi AMK, Elias JA. N-Glycosylation Regulates Chitinase 3–Like-1 and IL-13 Ligand Binding to IL-13 Receptor α2. Am J Respir Cell Mol Biol (2020) 63(3):386–95. doi: 10.1165/rcmb.2019-0446OC
15. Beard RE, Abate-Daga D, Rosati SF, Zheng Z, Wunderlich JR, Rosenberg SA, et al. Gene Expression Profiling Using Nanostring Digital RNA Counting to Identify Potential Target Antigens for Melanoma Immunotherapy. Clin Cancer Res (2013) 19(18):4941. doi: 10.1158/1078-0432.CCR-13-1253
16. Shibasaki N, Yamasaki T, Kanno T, Arakaki R, Sakamoto H, Utsunomiya N, et al. Role of IL13RA2 in Sunitinib Resistance in Clear Cell Renal Cell Carcinoma. PloS One (2015) 10(6):e0130980. doi: 10.1371/journal.pone.0130980
17. Fernandez-Ranvier GG, Weng J, Yeh R-F, Khanafshar E, Suh I, Barker C, et al. Identification of Biomarkers of Adrenocortical Carcinoma Using Genomewide Gene Expression Profiling. Arch Surg (2008) 143(9):841–6. doi: 10.1001/archsurg.143.9.841
18. Jain M, Zhang L, He M, Patterson EE, Nilubol N, Fojo AT, et al. Interleukin-13 Receptor Alpha2 is a Novel Therapeutic Target for Human Adrenocortical Carcinoma. Cancer (2012) 118(22):5698–708. doi: 10.1002/cncr.27629
19. Kawakami M, Kawakami K, Takahashi S, Abe M, Puri RK. Analysis of Interleukin-13 Receptor α2 Expression in Human Pediatric Brain Tumors. Cancer (2004) 101(5):1036–42. doi: 10.1002/cncr.20470
20. Debinski W, Gibo DM, Hulet SW, Connor JR, Gillespie GY. Receptor for Interleukin 13 Is a Marker and Therapeutic Target for Human High-Grade Gliomas. Clin Cancer Res (1999) 5(5):985.
21. Joshi BH, Puri RA, Leland P, Varricchio F, Gupta G, Kocak M, et al. Identification of Interleukin-13 Receptor α2 Chain Overexpression in Situ in High-Grade Diffusely Infiltrative Pediatric Brainstem Glioma. Neuro-Oncology (2008) 10(3):265–74. doi: 10.1215/15228517-2007-066
22. Barderas R, Bartolomé RA, Fernandez-Aceñero MJ, Torres S, Casal JI. High Expression of IL-13 Receptor α2 in Colorectal Cancer Is Associated With Invasion, Liver Metastasis, and Poor Prognosis. Cancer Res (2012) 72(11):2780. doi: 10.1158/0008-5472.CAN-11-4090
23. Lin C, Liu H, Zhang H, He H, Li H, Shen Z, et al. Interleukin-13 Receptor α2 is Associated With Poor Prognosis in Patients With Gastric Cancer After Gastrectomy. Oncotarget (2016) 7(31):49281–8. doi: 10.18632/oncotarget.10297
24. Kwon HJ, Choi JE, Bae YK. Interleukin-13 Receptor Alpha 2 Expression in Tumor Cells is Associated With Reduced Disease-Free Survival in Patients With Luminal Subtype Invasive Breast Cancer. Tumor Biol (2018) 40(6):1010428318783657. doi: 10.1177/1010428318783657
25. Papageorgis P, Ozturk S, Lambert AW, Neophytou CM, Tzatsos A, Wong CK, et al. Targeting IL13Ralpha2 Activates STAT6-TP63 Pathway to Suppress Breast Cancer Lung Metastasis. Breast Cancer Res (2015) 17(1):98. doi: 10.1186/s13058-015-0607-y
26. Kioi M, Kawakami M, Shimamura T, Husain SR, Puri RK. Interleukin-13 Receptor α2 Chain: A Potential Biomarker and Molecular Target for Ovarian Cancer Therapy. Cancer (2006) 107(6):1407–18. doi: 10.1002/cncr.22134
27. Xie M, Wu X-j, Zhang J-j, He C-s. IL-13 Receptor α2 is a Negative Prognostic Factor in Human Lung Cancer and Stimulates Lung Cancer Growth in Mice. Oncotarget (2015) 6(32):32902–13. doi: 10.18632/oncotarget.5361
28. Kumar A, Bellayr IH, Singh HS, Puri RK. IL-13rα2 Gene Expression is a Biomarker of Adverse Outcome in Patients With Adrenocortical Carcinoma. PloS One (2021) 16(2):e0246632. doi: 10.1371/journal.pone.0246632
29. Chong ST, Tan KM, Kok CYL, Guan SP, Lai SH, Lim C, et al. IL13RA2 Is Differentially Regulated in Papillary Thyroid Carcinoma vs Follicular Thyroid Carcinoma. J Clin Endocrinol Metab (2019) 104(11):5573–84. doi: 10.1210/jc.2019-00040
30. Fujisawa T, Shimamura T, Goto K, Nakagawa R, Muroyama R, Ino Y, et al. A Novel Role of Interleukin 13 Receptor Alpha2 in Perineural Invasion and its Association With Poor Prognosis of Patients With Pancreatic Ductal Adenocarcinoma. Cancers (2020) 12(5):1294–308. doi: 10.3390/cancers12051294
31. Han J, Puri RK. Analysis of the Cancer Genome Atlas (TCGA) Database Identifies an Inverse Relationship Between Interleukin-13 Receptor α1 and α2 Gene Expression and Poor Prognosis and Drug Resistance in Subjects With Glioblastoma Multiforme. J Neuro-Oncology (2018) 136(3):463–74. doi: 10.1007/s11060-017-2680-9
32. Wykosky J, Gibo DM, Stanton C, Debinski W. Interleukin-13 Receptor α2, EphA2, and Fos-Related Antigen 1 as Molecular Denominators of High-Grade Astrocytomas and Specific Targets for Combinatorial Therapy. Clin Cancer Res (2008) 14(1):199. doi: 10.1158/1078-0432.CCR-07-1990
33. Zeng J, Zhang J, Yang Y-Z, Wang F, Jiang H, Chen H-D, et al. IL13RA2 is Overexpressed in Malignant Gliomas and Related to Clinical Outcome of Patients. Am J Transl Res (2020) 12(8):4702–14.
34. Sengupta S, Thaci B, Crawford AC, Sampath P. Interleukin-13 Receptor Alpha 2-Targeted Glioblastoma Immunotherapy. BioMed Res Int (2014) 2014:952128–. doi: 10.1155/2014/952128
35. Saikali S, Avril T, Collet B, Hamlat A, Bansard J-Y, Drenou B, et al. Expression of Nine Tumour Antigens in a Series of Human Glioblastoma Multiforme: Interest of EGFRvIII, IL-13rα2, Gp100 and TRP-2 for Immunotherapy. J Neuro-Oncology (2007) 81(2):139–48. doi: 10.1007/s11060-006-9220-3
36. Pastan I, Hassan R, Fitzgerald DJ, Kreitman RJ. Immunotoxin Therapy of Cancer. Nat Rev Cancer (2006) 6(7):559–65. doi: 10.1038/nrc1891
37. Debinski W, Obiri NI, Pastan I, Puri RK. A Novel Chimeric Protein Composed of Interleukin 13 and Pseudomonas Exotoxin Is Highly Cytotoxic to Human Carcinoma Cells Expressing Receptors for Interleukin 13 and Interleukin 4 (∗). J Biol Chem (1995) 270(28):16775–80. doi: 10.1074/jbc.270.28.16775
38. Li C, Hall WA, Jin N, Todhunter DA, Panoskaltsis-Mortari A, Vallera DA. Targeting Glioblastoma Multiforme With an IL-13/Diphtheria Toxin Fusion Protein In Vitro and In Vivo in Nude Mice. Protein Engineering Design Selection (2002) 15(5):419–27. doi: 10.1093/protein/15.5.419
39. Havaei SM, Aucoin MG, Jahanian-Najafabadi A. Pseudomonas Exotoxin-Based Immunotoxins: Over Three Decades of Efforts on Targeting Cancer Cells With the Toxin. Front Oncol (2021) 11. doi: 10.3389/fonc.2021.781800
40. Puri RK, Leland P, Obiri NI, Husain SR, Kreitman RJ, Haas GP, et al. Targeting of Interleukin-13 Receptor on Human Renal Cell Carcinoma Cells by a Recombinant Chimeric Protein Composed of Interleukin-13 and a Truncated Form of Pseudomonas Exotoxin A (PE38QQR). Blood (1996) 87(10):4333–9. doi: 10.1182/blood.V87.10.4333.bloodjournal87104333
41. Debinski W, Obiri NI, Powers SK, Pastan I, Puri RK. Human Glioma Cells Overexpress Receptors for Interleukin 13 and are Extremely Sensitive to a Novel Chimeric Protein Composed of Interleukin 13 and Pseudomonas Exotoxin. Clin Cancer Res (1995) 1(11):1253.
42. Kawakami K, Kawakami M, Joshi BH, Puri RK. Interleukin-13 Receptor-Targeted Cancer Therapy in an Immunodeficient Animal Model of Human Head and Neck Cancer. Cancer Res (2001) 61(16):6194.
43. Kawakami K, Husain SR, Bright RK, Puri RK. Gene Transfer of Interleukin 13 Receptor α2 Chain Dramatically Enhances the Antitumor Effect of IL-13 Receptor–Targeted Cytotoxin in Human Prostate Cancer Xenografts. Cancer Gene Ther (2001) 8(11):861–8. doi: 10.1038/sj.cgt.7700373
44. Kawakami K, Joshi BH, Puri RK. Sensitization of Cancer Cells to Interleukin 13-Pseudomonas Exotoxin-Induced Cell Death by Gene Transfer of Interleukin 13 Receptor α Chain. Hum Gene Ther (2004) 11(13):1829–35. doi: 10.1089/10430340050129459
45. Kawakami K, Kioi M, Liu Q, Kawakami M, Puri RK. Evidence That IL-13rα2 Chain in Human Glioma Cells Is Responsible for the Antitumor Activity Mediated by Receptor-Directed Cytotoxin Therapy. J Immunotherapy (2005) 28(3):193–202. doi: 10.1097/01.cji.0000161393.04207.e1
46. Shimamura T, Fujisawa T, Husain SR, Joshi B, Puri RK. Interleukin 13 Mediates Signal Transduction Through Interleukin 13 Receptor α2 in Pancreatic Ductal Adenocarcinoma: Role of IL-13 Pseudomonas Exotoxin in Pancreatic Cancer Therapy. Clin Cancer Res (2010) 16(2):577. doi: 10.1158/1078-0432.CCR-09-2015
47. Lai EW, Joshi BH, Martiniova L, Dogra R, Fujisawa T, Leland P, et al. Overexpression of Interleukin-13 Receptor-α2 in Neuroendocrine Malignant Pheochromocytoma: A Novel Target for Receptor Directed Anti-Cancer Therapy. J Clin Endocrinol Metab (2009) 94(8):2952–7. doi: 10.1210/jc.2009-0309
48. Fujisawa T, Joshi BH, Puri RK. Histone Modification Enhances the Effectiveness of IL-13 Receptor Targeted Immunotoxin in Murine Models of Human Pancreatic Cancer. J Transl Med (2011) 9(1):37. doi: 10.1186/1479-5876-9-37
49. Kunwar S, Prados MD, Chang SM, Berger MS, Lang FF, Piepmeier JM, et al. Direct Intracerebral Delivery of Cintredekin Besudotox (IL13-PE38QQR) in Recurrent Malignant Glioma: A Report by the Cintredekin Besudotox Intraparenchymal Study Group. J Clin Oncol (2007) 25(7):837–44. doi: 10.1200/JCO.2006.08.1117
50. Vogelbaum MA, Sampson JH, Kunwar S, Chang SM, Shaffrey M, Asher AL, et al. Convection-Enhanced Delivery of Cintredekin Besudotox (Interleukin-13-PE38QQR) Followed by Radiation Therapy With and Without Temozolomide in Newly Diagnosed Malignant Gliomas: Phase 1 Study of Final Safety Results. Neurosurgery (2007) 61(5):1031–7; discussion 7-8. doi: 10.1227/01.neu.0000303199.77370.9e
51. Kunwar S, Chang S, Westphal M, Vogelbaum M, Sampson J, Barnett G, et al. Phase III Randomized Trial of CED of IL13-PE38QQR vs Gliadel Wafers for Recurrent Glioblastoma†. Neuro-Oncology (2010) 12(8):871–81. doi: 10.1093/neuonc/nop054
52. Mueller S, Polley M-Y, Lee B, Kunwar S, Pedain C, Wembacher-Schröder E, et al. Effect of Imaging and Catheter Characteristics on Clinical Outcome for Patients in the PRECISE Study. J Neuro-Oncology (2011) 101(2):267–77. doi: 10.1007/s11060-010-0255-0
53. Sampson JH, Archer G, Pedain C, Wembacher-Schröder E, Westphal M, Kunwar S, et al. Poor Drug Distribution as a Possible Explanation for the Results of the PRECISE Trial. J Neurosurg (2010) 113(2):301–9. doi: 10.3171/2009.11.JNS091052
54. Heiss JD, Jamshidi A, Shah S, Martin S, Wolters PL, Argersinger DP, et al. Phase I Trial of Convection-Enhanced Delivery of IL13-Pseudomonas Toxin in Children With Diffuse Intrinsic Pontine Glioma. J Neurosurg Pediatr (2018) 23(3):333–42. doi: 10.3171/2018.9.PEDS17225
55. Joshi BH, Husain SR, Leland P, Puri RK. Interleukin-13 Receptors and Development of IL-13-Pseudomonas Exotoxin for Human Cancer Therapy. In: Kawakami K, Aggawal BB, Puri RK, editors. Cytotoxins and Immunotoxins for Cancer Therapy: Clinical Applications. Boca Raton, FL: CRC Press (2004). p. 45–70.
56. Liu-Chittenden Y, Jain M, Kumar P, Patel D, Aufforth R, Neychev V, et al. Phase I Trial of Systemic Intravenous Infusion of Interleukin-13-Pseudomonas Exotoxin in Patients With Metastatic Adrenocortical Carcinoma. Cancer Med (2015) 4(7):1060–8. doi: 10.1002/cam4.449
57. Mazor R, Onda M, Pastan I. Immunogenicity of Therapeutic Recombinant Immunotoxins. Immunol Rev (2016) 270(1):152–64. doi: 10.1111/imr.12390
58. Liu TF, Cai J, Gibo DM, Debinski W. Reoxygenation of Hypoxic Glioblastoma Multiforme Cells Potentiates the Killing Effect of an Interleukin-13-Based Cytotoxin. Clin Cancer Res (2009) 15(1):160–8. doi: 10.1158/1078-0432.CCR-08-2151
59. Rustamzadeh E, Hall WA, Todhunter DA, Low WC, Liu H, Panoskaltsis-Mortari A, et al. Intracranial Therapy of Glioblastoma With the Fusion Protein DTIL13 in Immunodeficient Mice. Int J Cancer (2006) 118(10):2594–601. doi: 10.1002/ijc.21647
60. Todhunter DA, Hall WA, Rustamzadeh E, Shu Y, Doumbia SO, Vallera DA. A Bispecific Immunotoxin (DTAT13) Targeting Human IL-13 Receptor (IL-13R) and Urokinase-Type Plasminogen Activator Receptor (uPAR) in a Mouse Xenograft Model. Protein Engineering Design Selection (2004) 17(2):157–64. doi: 10.1093/protein/gzh023
61. Stish BJ, Chen H, Shu Y, Panoskaltsis-Mortari A, Vallera DA. A Bispecific Recombinant Cytotoxin (DTEGF13) Targeting Human Interleukin-13 and Epidermal Growth Factor Receptors in a Mouse Xenograft Model of Prostate Cancer. Clin Cancer Res (2007) 13(21):6486. doi: 10.1158/1078-0432.CCR-07-0938
62. Oh S, Ohlfest JR, Todhunter DA, Vallera VD, Hall WA, Chen H, et al. Intracranial Elimination of Human Glioblastoma Brain Tumors in Nude Rats Using the Bispecific Ligand-Directed Toxin, DTEGF13 and Convection Enhanced Delivery. J Neurooncol (2009) 95(3):331–42. doi: 10.1007/s11060-009-9932-2
63. Shafiee F, Aucoin MG, Jahanian-Najafabadi A. Targeted Diphtheria Toxin-Based Therapy: A Review Article. Front Microbiol (2019) 10. doi: 10.3389/fmicb.2019.02340
64. Feins S, Kong W, Williams EF, Milone MC, Fraietta JA. An Introduction to Chimeric Antigen Receptor (CAR) T-Cell Immunotherapy for Human Cancer. Am J Hematol (2019) 94(S1):S3–s9. doi: 10.1002/ajh.25418
65. June Carl H, O’Connor Roddy S, Kawalekar Omkar U, Ghassemi S, Milone Michael C. CAR T Cell Immunotherapy for Human Cancer. Science (2018) 359(6382):1361–5. doi: 10.1126/science.aar6711
66. FDA. Approved Cellular and Gene Therapy Products. Available at: https://www.fda.gov/vaccines-blood-biologics/cellular-gene-therapy-products/approved-cellular-and-gene-therapy-products.
67. Brown CE, Aguilar B, Starr R, Yang X, Chang W-C, Weng L, et al. Optimization of IL13Rα2-Targeted Chimeric Antigen Receptor T Cells for Improved Anti-Tumor Efficacy Against Glioblastoma. Mol Ther (2018) 26(1):31–44. doi: 10.1016/j.ymthe.2017.10.002
68. Brown CE, Alizadeh D, Starr R, Weng L, Wagner JR, Naranjo A, et al. Regression of Glioblastoma After Chimeric Antigen Receptor T-Cell Therapy. New Engl J Med (2016) 375(26):2561–9. doi: 10.1056/NEJMoa1610497
69. Lin Q, Ba T, Ho J, Chen D, Cheng Y, Wang L, et al. First-In-Human Trial of EphA2-Redirected CAR T-Cells in Patients With Recurrent Glioblastoma: A Preliminary Report of Three Cases at the Starting Dose. Front Oncol (2021) 11:694941. doi: 10.3389/fonc.2021.694941
70. Kahlon KS, Brown C, Cooper LJN, Raubitschek A, Forman SJ, Jensen MC. Specific Recognition and Killing of Glioblastoma Multiforme by Interleukin 13-Zetakine Redirected Cytolytic T Cells. Cancer Res (2004) 64(24):9160. doi: 10.1158/0008-5472.CAN-04-0454
71. Brown CE, Badie B, Barish ME, Weng L, Ostberg JR, Chang W-C, et al. Bioactivity and Safety of IL13Rα2-Redirected Chimeric Antigen Receptor CD8+ T Cells in Patients With Recurrent Glioblastoma. Clin Cancer Res (2015) 21(18):4062. doi: 10.1158/1078-0432.CCR-15-0428
72. Yaghoubi SS, Jensen MC, Satyamurthy N, Budhiraja S, Paik D, Czernin J, et al. Noninvasive Detection of Therapeutic Cytolytic T Cells With 18F-FHBG PET in a Patient With Glioma. Nat Clin Pract Oncol (2009) 6(1):53–8. doi: 10.1038/ncponc1278
73. Krebs S, Chow KKH, Yi Z, Rodriguez-Cruz T, Hegde M, Gerken C, et al. T Cells Redirected to Interleukin-13Rα2 With Interleukin-13 Mutein–Chimeric Antigen Receptors Have Anti-Glioma Activity But Also Recognize Interleukin-13Rα1. Cytotherapy (2014) 16(8):1121–31. doi: 10.1016/j.jcyt.2014.02.012
74. Kim K, Gwak H-S, Han N, Hong EK, Choi BK, Lee S, et al. Chimeric Antigen Receptor T Cells With Modified Interleukin-13 Preferentially Recognize Il13rα2 and Suppress Malignant Glioma: A Preclinical Study. Front Immunol (2021) 12(4592). doi: 10.3389/fimmu.2021.715000
75. Krenciute G, Krebs S, Torres D, Wu M-F, Liu H, Dotti G, et al. Characterization and Functional Analysis of scFv-Based Chimeric Antigen Receptors to Redirect T Cells to IL13Rα2-Positive Glioma. Mol Ther (2016) 24(2):354–63. doi: 10.1038/mt.2015.199
76. Pituch KC, Miska J, Krenciute G, Panek WK, Li G, Rodriguez-Cruz T, et al. Adoptive Transfer of IL13Rα2-Specific Chimeric Antigen Receptor T Cells Creates a Pro-Inflammatory Environment in Glioblastoma. Mol Ther (2018) 26(4):986–95. doi: 10.1016/j.ymthe.2018.02.001
77. Hegde M, Corder A, Chow KKH, Mukherjee M, Ashoori A, Kew Y, et al. Combinational Targeting Offsets Antigen Escape and Enhances Effector Functions of Adoptively Transferred T Cells in Glioblastoma. Mol Ther (2013) 21(11):2087–101. doi: 10.1038/mt.2013.185
78. Hegde M, Mukherjee M, Grada Z, Pignata A, Landi D, Navai SA, et al. Tandem CAR T Cells Targeting HER2 and IL13Rα2 Mitigate Tumor Antigen Escape. J Clin Invest (2016) 126(8):3036–52. doi: 10.1172/JCI83416
79. Bielamowicz K, Fousek K, Byrd TT, Samaha H, Mukherjee M, Aware N, et al. Trivalent CAR T Cells Overcome Interpatient Antigenic Variability in Glioblastoma. Neuro-Oncology (2018) 20(4):506–18. doi: 10.1093/neuonc/nox182
80. Marqus S, Pirogova E, Piva TJ. Evaluation of the Use of Therapeutic Peptides for Cancer Treatment. J Biomed Sci (2017) 24(1):21. doi: 10.1186/s12929-017-0328-x
81. Pandya H, Gibo DM, Garg S, Kridel S, Debinski W. An Interleukin 13 Receptor α 2–Specific Peptide Homes to Human Glioblastoma Multiforme Xenografts. Neuro-Oncology (2012) 14(1):6–18. doi: 10.1093/neuonc/nor141
82. Sai KKS, Sattiraju A, Almaguel FG, Xuan A, Rideout A, Krishnaswamy RS, et al. Peptide-Based PET Imaging of the Tumor Restricted IL13RA2 Biomarker. Oncotarget (2017) 8(31):50997–1007. doi: 10.18632/oncotarget.16549
83. Wang B, Lv L, Wang Z, Jiang Y, Lv W, Liu X, et al. Improved Anti-Glioblastoma Efficacy by IL-13rα2 Mediated Copolymer Nanoparticles Loaded With Paclitaxel. Sci Rep (2015) 5:16589–. doi: 10.1038/srep16589
84. Wang B, Lv L, Wang Z, Zhao Y, Wu L, Fang X, et al. Nanoparticles Functionalized With Pep-1 as Potential Glioma Targeting Delivery System via Interleukin 13 Receptor α2-Mediated Endocytosis. Biomaterials (2014) 35(22):5897–907. doi: 10.1016/j.biomaterials.2014.03.068
85. Sattiraju A, Solingapuram Sai KK, Xuan A, Pandya DN, Almaguel FG, Wadas TJ, et al. IL13RA2 Targeted Alpha Particle Therapy Against Glioblastomas. Oncotarget (2017) 8(26):42997–3007. doi: 10.18632/oncotarget.17792
86. Bartolomé RA, Jaén M, Casal JI. An Il13rα2 Peptide Exhibits Therapeutic Activity Against Metastatic Colorectal Cancer. Br J Cancer (2018) 119(8):940–9. doi: 10.1038/s41416-018-0259-7
87. Bartolomé RA, Martín-Regalado Á, Jaén M, Zannikou M, Zhang P, de los Ríos V, et al. Protein Tyrosine Phosphatase-1b Inhibition Disrupts Il13rα2-Promoted Invasion and Metastasis in Cancer Cells. Cancers (2020) 12(2):500–16. doi: 10.3390/cancers12020500
88. Kimiz-Gebologlu I, Gulce-Iz S, Biray-Avci C. Monoclonal Antibodies in Cancer Immunotherapy. Mol Biol Rep (2018) 45(6):2935–40. doi: 10.1007/s11033-018-4427-x
89. Weiner LM, Dhodapkar MV, Ferrone S. Monoclonal Antibodies for Cancer Immunotherapy. Lancet (2009) 373(9668):1033–40. doi: 10.1016/S0140-6736(09)60251-8
90. Balyasnikova IV, Wainwright DA, Solomaha E, Lee G, Han Y, Thaci B, et al. Characterization and Immunotherapeutic Implications for a Novel Antibody Targeting Interleukin (IL)-13 Receptor α2*. J Biol Chem (2012) 287(36):30215–27. doi: 10.1074/jbc.M112.370015
91. Jaén M, Bartolomé RA, Aizpurua C, Martin-Regalado Á, Imbaud JI, Casal JI. Inhibition of Liver Metastasis in Colorectal Cancer by Targeting IL-13/Il13rα2 Binding Site With Specific Monoclonal Antibodies. Cancers (2021) 13(7):1731–47. doi: 10.3390/cancers13071731
92. Gupta P, Wentland J-A, Leal M, Ma D, Roach R, Esparza A, et al. Assessment of Near-Infrared Fluorophores to Study the Biodistribution and Tumor Targeting of an IL13 Receptor α2 Antibody by Fluorescence Molecular Tomography. Oncotarget (2017) 8(34):57231–45. doi: 10.18632/oncotarget.19569
93. Gupta P, Jiang ZK, Yang B, Manzuk L, Rosfjord E, Yao J, et al. Targeting and Pharmacology of an Anti-IL13Rα2 Antibody and Antibody-Drug Conjugate in a Melanoma Xenograft Model. MAbs (2021) 13(1):1958662–. doi: 10.1080/19420862.2021.1958662
94. Lian X, Kats D, Rasmussen S, Martin LR, Karki A, Keller C, et al. Design Considerations of an IL13Rα2 Antibody–Drug Conjugate for Diffuse Intrinsic Pontine Glioma. Acta Neuropathologica Commun (2021) 9(1):88. doi: 10.1186/s40478-021-01184-9
95. Saxena M, van der Burg SH, Melief CJM, Bhardwaj N. Therapeutic Cancer Vaccines. Nat Rev Cancer (2021) 21(6):360–78. doi: 10.1038/s41568-021-00346-0
96. Shimato S, Natsume A, Wakabayashi T, Tsujimura K, Nakahara N, Ishii J, et al. Identification of a Human Leukocyte Antigen-A24–restricted T-Cell Epitope Derived From Interleukin-13 Receptor α2 Chain, a Glioma-Associated Antigen: Laboratory Investigation. J Neurosurgery JNS (2008) 109(1):117–22. doi: 10.3171/JNS/2008/109/7/0117
97. Iwami K, Shimato S, Ohno M, Okada H, Nakahara N, Sato Y, et al. Peptide-Pulsed Dendritic Cell Vaccination Targeting Interleukin-13 Receptor α2 Chain in Recurrent Malignant Glioma Patients With HLA-A*24/A*02 Allele. Cytotherapy (2012) 14(6):733–42. doi: 10.3109/14653249.2012.666633
98. Phuphanich S, Wheeler CJ, Rudnick JD, Mazer M, Wang H, Nuño MA, et al. Phase I Trial of a Multi-Epitope-Pulsed Dendritic Cell Vaccine for Patients With Newly Diagnosed Glioblastoma. Cancer Immunol Immunother (2013) 62(1):125–35. doi: 10.1007/s00262-012-1319-0
99. Okada H, Kalinski P, Ueda R, Hoji A, Kohanbash G, Donegan TE, et al. Induction of CD8+ T-Cell Responses Against Novel Glioma–Associated Antigen Peptides and Clinical Activity by Vaccinations With α-Type 1 Polarized Dendritic Cells and Polyinosinic-Polycytidylic Acid Stabilized by Lysine and Carboxymethylcellulose in Patients With Recurrent Malignant Glioma. J Clin Oncol (2010) 29(3):330–6. doi: 10.1200/JCO.2010.30.7744
100. Wen PY, Reardon DA, Armstrong TS, Phuphanich S, Aiken RD, Landolfi JC, et al. A Randomized Double-Blind Placebo-Controlled Phase II Trial of Dendritic Cell Vaccine ICT-107 in Newly Diagnosed Patients With Glioblastoma. Clin Cancer Res (2019) 25(19):5799. doi: 10.1158/1078-0432.CCR-19-0261
101. Kawakami K, Terabe M, Kawakami M, Berzofsky JA, Puri RK. Characterization of a Novel Human Tumor Antigen Interleukin-13 Receptor α2 Chain. Cancer Res (2006) 66(8):4434. doi: 10.1158/0008-5472.CAN-05-1265
102. Nakashima H, Terabe M, Berzofsky JA, Husain SR, Puri RK. A Novel Combination Immunotherapy for Cancer by IL-13rα2-Targeted DNA Vaccine and Immunotoxin in Murine Tumor Models. J Immunol (2011) 187(10):4935–46. doi: 10.4049/jimmunol.1102095
103. Nakashima H, Fujisawa T, Husain SR, Puri RK. Interleukin-13 Receptor α2 DNA Prime Boost Vaccine Induces Tumor Immunity in Murine Tumor Models. J Transl Med (2010) 8:116–. doi: 10.1186/1479-5876-8-116
104. Pollack IF, Jakacki RI, Butterfield LH, Hamilton RL, Panigrahy A, Potter DM, et al. Antigen-Specific Immune Responses and Clinical Outcome After Vaccination With Glioma-Associated Antigen Peptides and Polyinosinic-Polycytidylic Acid Stabilized by Lysine and Carboxymethylcellulose in Children With Newly Diagnosed Malignant Brainstem and Nonbrainstem Gliomas. J Clin Oncol (2014) 32(19):2050–8. doi: 10.1200/JCO.2013.54.0526
105. Fujisawa T, Nakashima H, Nakajima A, Joshi BH, Puri RK. Targeting IL-13rα2 in Human Pancreatic Ductal Adenocarcinoma With Combination Therapy of IL-13-PE and Gemcitabine. Int J Cancer (2011) 128(5):1221–31. doi: 10.1002/ijc.25437
106. Kioi M, Shimamura T, Nakashima H, Hirota M, Tohnai I, Husain SR, et al. IL-13 Cytotoxin has Potent Antitumor Activity and Synergizes With Paclitaxel in a Mouse Model of Oral Squamous Cell Carcinoma. Int J Cancer (2009) 124(6):1440–8. doi: 10.1002/ijc.24067
107. Yin Y, Boesteanu AC, Binder ZA, Xu C, Reid RA, Rodriguez JL, et al. Checkpoint Blockade Reverses Anergy in IL-13rα2 Humanized scFv-Based CAR T Cells to Treat Murine and Canine Gliomas. Mol Ther Oncolytics (2018) 11:20–38. doi: 10.1016/j.omto.2018.08.002
108. Wu L, Deng W-W, Huang C-F, Bu L-L, Yu G-T, Mao L, et al. Expression of VISTA Correlated With Immunosuppression and Synergized With CD8 to Predict Survival in Human Oral Squamous Cell Carcinoma. Cancer Immunology Immunotherapy (2017) 66(5):627–36. doi: 10.1007/s00262-017-1968-0
109. Fichtner-Feigl S, Terabe M, Kitani A, Young CA, Fuss I, Geissler EK, et al. Restoration of Tumor Immunosurveillance via Targeting of Interleukin-13 Receptor-α2. Cancer Res (2008) 68(9):3467. doi: 10.1158/0008-5472.CAN-07-5301
110. Daines MO, Hershey GKK. A Novel Mechanism by Which Interferon-γ Can Regulate Interleukin (IL)-13 Responses: Evidence For Intracellular Stores Of Il-13 Receptor A-2 And Their Rapid Mobilization By Interferon-γ*. J Biol Chem (2002) 277(12):10387–93. doi: 10.1074/jbc.M108109200
111. Xu N, Bo Q, Shao R, Liang J, Zhai Y, Yang S, et al. Chitinase-3-Like-1 Promotes M2 Macrophage Differentiation and Induces Choroidal Neovascularization in Neovascular Age-Related Macular Degeneration. Invest Ophthalmol Visual Sci (2019) 60(14):4596–605. doi: 10.1167/iovs.19-27493
Keywords: interleukin 13 receptor α2, IL-13Rα2, immunotoxin, CAR (chimeric antigen receptor) T cells, gliobastoma (GBM)
Citation: Knudson KM, Hwang S, McCann MS, Joshi BH, Husain SR and Puri RK (2022) Recent Advances in IL-13Rα2-Directed Cancer Immunotherapy. Front. Immunol. 13:878365. doi: 10.3389/fimmu.2022.878365
Received: 17 February 2022; Accepted: 17 March 2022;
Published: 08 April 2022.
Edited by:
Brent Johnston, Dalhousie University, CanadaReviewed by:
Warren Strober, National Institutes of Health (NIH), United StatesDaniel A Vallera, University of Minnesota Twin Cities, United States
Copyright © 2022 Knudson, Hwang, McCann, Joshi, Husain and Puri. This is an open-access article distributed under the terms of the Creative Commons Attribution License (CC BY). The use, distribution or reproduction in other forums is permitted, provided the original author(s) and the copyright owner(s) are credited and that the original publication in this journal is cited, in accordance with accepted academic practice. No use, distribution or reproduction is permitted which does not comply with these terms.
*Correspondence: Karin M. Knudson, karin.knudson@fda.hhs.gov