- 1Division of Rheumatology, Department of Medicine, Solna, Karolinska Institutet, Stockholm, Sweden
- 2Center for Molecular Medicine, Karolinska Institutet, and Karolinska University Hospital Solna, Stockholm, Sweden
Anti-synthetase syndrome (ASSD) is an autoimmune disease characterized by the presence of autoantibodies targeting one of several aminoacyl t-RNA synthetases (aaRSs) along with clinical features including interstitial lung disease, myositis, Raynaud’s phenomenon, arthritis, mechanic’s hands, and fever. The family of aaRSs consists of highly conserved cytoplasmic and mitochondrial enzymes, one for each amino acid, which are essential for the RNA translation machinery and protein synthesis. Along with their main functions, aaRSs are involved in the development of immune responses, regulation of transcription, and gene-specific silencing of translation. During the last decade, these proteins have been associated with cancer, neurological disorders, infectious responses, and autoimmune diseases including ASSD. To date, several aaRSs have been described to be possible autoantigens in different diseases. The most commonly described are histidyl (HisRS), threonyl (ThrRS), alanyl (AlaRS), glycyl (GlyRS), isoleucyl (IleRS), asparaginyl (AsnRS), phenylalanyl (PheRS), tyrosyl (TyrRS), lysyl (LysRS), glutaminyl (GlnRS), tryptophanyl (TrpRS), and seryl (SerRS) tRNA synthetases. Autoantibodies against the first eight autoantigens listed above have been associated with ASSD while the rest have been associated with other diseases. This review will address what is known about the function of the aaRSs with a focus on their autoantigenic properties. We will also describe the anti-aaRSs autoantibodies and their association to specific clinical manifestations, and discuss their potential contribution to the pathogenesis of ASSD.
Introduction
Anti-synthetase syndrome (ASSD) is an autoimmune condition characterized by the presence of autoantibodies directed against an aminoacyl transfer RNA synthetase (aaRS) along with clinical features that include interstitial lung disease (ILD), myositis, Raynaud’s phenomenon, fever, mechanic’s hands, and arthritis (1, 2). ILD is the primary cause of morbidity and mortality in patients with ASSD (3–5).
Aminoacyl-tRNA synthetases (aaRSs) are a family of enzymes that catalyze the charging of amino acids onto their cognate tRNAs for protein synthesis (6). Twenty members are included in the aaRS family in most species with some exceptions (7). In humans, there are two sets of aaRSs for their actions in cytosol or mitochondria, respectively. In total, 37 aaRSs genes are encoded, which include 18 for cytoplasmic subunits (2 genes coding for separate subunits of the same aaRSs, and one gene for two fused aaRSs), 17 for mitochondrial subunits, and 2 for both sites (8). The discovery of autoantibodies against eight of these aaRS represented the first connection between aaRSs and human diseases (9).
The discovery of anti-aminoacyl-tRNA synthetase autoantibodies has allowed for the characterization of ASSD. The first detected autoantibody against an aaRS was reported in 1980 in patients with idiopathic inflammatory myopathies (IIM) (10). In 1983, Mathews et al. identified the target of Jo-1 autoantibody to be tRNAHis by immunoprecipitation (9). Afterwards, autoantibodies associated with similar clinical manifestations were identified against seven other aaRSs, including ThrRS, AlaRS, GlyRS, IleRS, AsnRS, PheRS, and TyrRS and were named anti-PL-7, anti-PL-12, anti-EJ, anti-OJ, anti-KS, anti-Zo, and anti-HA, respectively (11–17). At the time of discovery, it was thought that these autoantibodies identified subtypes of myositis. In 1991, Love et al. were the first that grouped patients representing distinctive clinical features with aaRSs antibodies as a unique syndrome, and in 1992, Targoff proposed to name this syndrome as ASSD (18, 19).
This review will address what is known about the function of the aaRSs and their potential autoantigenic properties. We will also describe the anti-aaRSs autoantibodies together with the associations to specific clinical manifestations and discuss their possible contribution to the pathogenesis of ASSD.
Aminoacyl-tRNA Synthetases
The history of aaRSs dates back to 1950s, when it was found that ATP was needed for the incorporation of amino acids to a polypeptide in vitro (20). Later in the mid-50s, Francis Crick introduced the adaptor hypothesis in which he proposed that each aaRS is synthesized by a unique amino acid specific enzyme (21). According to Crick, the minimum number of adaptors should be 20, one for each amino acid (22). Subsequently these adaptors were identified and are now known as tRNA molecules. The first complete tRNA sequence was published in 1965 and the structure of tRNAPhe was determined in 1974 (23) (Figure 1).
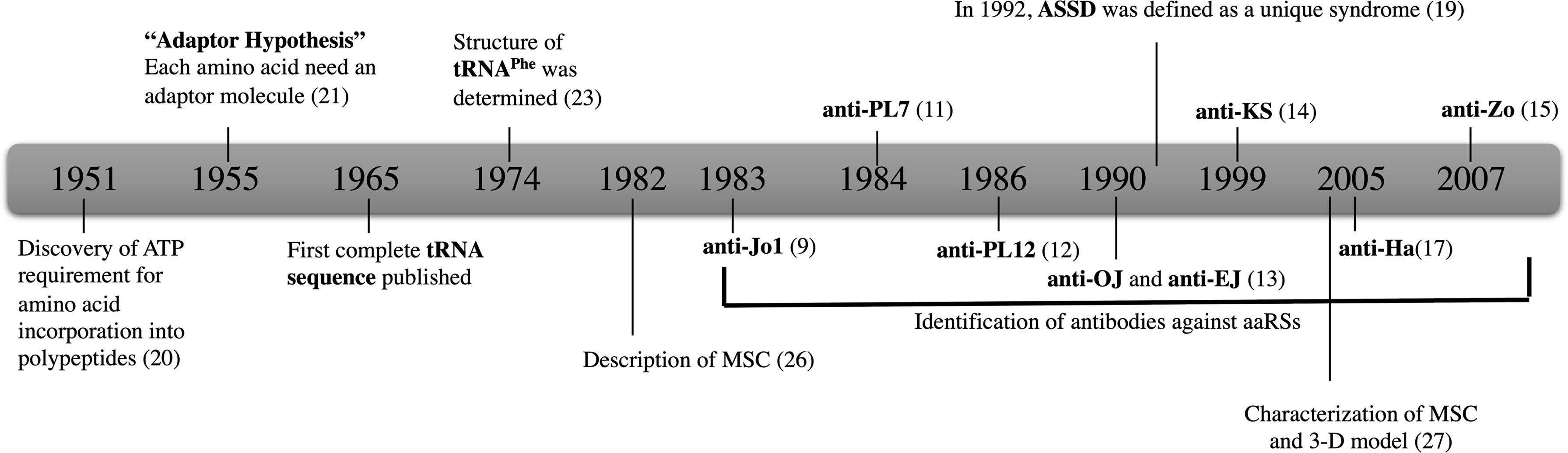
Figure 1 The history of aaRSs and discovery of antibodies against eight aaRSs. Anti-Jo-1; HisRS, anti-PL-7; ThrRS, anti-PL-12; AlaRS, anti-OJ; IleRS, anti-EJ; GlyRS, anti-KS; AsnRS anti-Ha; TyrRS, and anti-Zo; PheRS.
The aaRSs are grouped into two classes: class I and class II based on distinct features of the reactions they catalyze. Class I aaRSs approach the 3’-end of their cognate tRNA with their Rossmann nucleotide binding fold-based catalytic domain (CD), whereas class II aaRSs approach their cognate tRNAs from the major groove side with anti-parallel β-sheet and flanking α-helices (24, 25). In mammals, aaRSs can also be classified as free and complex-bound forms. In fact, eight of the aaRSs (LeuRS, IleuRS, EPRS, MetRS, GluRS, ArgRS, LysRS, and AspRS) do not function as single proteins as they are part of a multi-tRNA synthetase complex (MSC) together with 3 scaffold proteins called aaRS-interacting multi-functional proteins (AIMPs) (26, 27). The MSC is thought to contribute to the cellular homeostasis maintenance in higher eukaryotes (28–30). The AIMPs also exert diverse functions other than protein synthesis, encompassing induction of synthesis of various pro-inflammatory cytokines and chemokines, angiogenesis suppression, and prevention of hyperproliferation of lung cells (29). The canonical functions of aaRSs, which include charging of tRNA synthesis, aminoacylation, and editing, are highly conserved between species. However, during the evolution from prokaryotes to vertebrates, some aaRSs acquired additional domains with characteristic structures that were not required for the canonical functions. These evolved domains, mostly found on the amino (N-) or carboxy (C-) terminus, were indicated for non-canonical activities, including translation control, transcriptional regulation, signal transduction, cell migration, angiogenesis, inflammation, and tumorigenesis (31). Evidence from recent studies suggest that either canonical or non-canonical functions of aaRSs are associated with human diseases (32).
This review will focus on the contribution of the 20 cytoplasmic aaRSs to ASSD and other diseases. To date, autoantibodies against eight of these aaRSs have been reported to be associated with ASSD. They can be listed based on the prevalence of autoantibodies against them: HisRS, ThrRS, AlaRS, GlyRS, IleRS, AsnRS, PheRS, and TyrRS (Table 1). Additional aaRSs that have been linked to other diseases are LysRS, GlnRS, TrpRS, and SerRS. Autoantibodies against TrpRS and SerRS have also been found in patients with other autoimmune diseases; however, the clinical features were more associated with rheumatoid arthritis (RA) or systemic lupus erythematosus (SLE) and not ASSD or myositis (33–36). We will also discuss what is known about the remaining eight aaRSs, since even though they have not yet been indicated to play a role in the pathogenesis of diseases, mutations in their encoding genes have been associated with different pathological conditions.
Overview of Clinical Manifestations in ASSD
According to Connors criteria, diagnosis of ASSD is made when an individual is positive for one of the eight described anti-aaRS autoantibodies (anti-Jo-1, PL-12, PL-7, EJ, OJ, KS, Ha, or Zo) and presents with at least one clinical manifestation among myositis, ILD, arthritis, Raynaud’s phenomenon, mechanic’s hands, or fever (Table 2) (2). The clinical spectrum of ASSD associated with the different anti-aaRSs autoantibodies is not identical but rather heterogeneous, whereby isolated or combined features are possible (46, 47). Anti-Jo-1 antibodies are found in 20%–30% of patients with IIM while those targeting other aaRS are less common, each with a prevalence below 5% (47).
ILD often dominates the clinical picture of patients with ASSD without anti-Jo-1 autoantibodies, being the initial manifestation especially in patients with anti-PL-7, PL-12, and EJ autoantibodies (48). Severity range of ILD in patients with ASSD is broad, going from asymptomatic cases to acute distress respiratory syndrome. Within ILD patterns in patients with ASSD, non-specific interstitial pneumonia (NSIP) is the most frequent, followed by organizing pneumonia (OP) and usual interstitial pneumonia (UIP). Diffuse alveolar damage (DAD) has been reported in anti-EJ patients (49). Cumulative survival seems to be higher in anti-aaRS-positive patients with ILD compared to those with idiopathic pulmonary fibrosis (IPF) (50). Within patients with ASSD, rapid progressive ILD has been observed in individuals with anti-PL-7 or anti-EJ antibodies (51). Several longitudinal cohort studies have shown that anti-PL-12 and anti-PL-7 autoantibodies are associated with more prevalent and severe ILD compared to anti-Jo-1 patients (4, 37, 38, 48, 52–54), with a lower frequency or absence of myositis (40, 55). The ILD could lead to a secondary increase in the intrathoracic pressure or lower esophageal involvement manifested by increased gastrointestinal manifestations (37, 52). The overall outcome of ILD in the group of anti-PL-7/PL-12 is worse when compared to anti-Jo-1 patients, with a higher death rate associated with lung complications (37). This lower survival rate has been associated with a delay in diagnosis, since up to 50% of the non-Jo-1 anti-synthetase patients were initially diagnosed with an overlap disorder with minimal or no evidence of myositis (38, 56). However, this mortality rate might vary as suggested by another longitudinal cohort study where, despite finding more severe lung involvement in anti-PL-7 and anti-PL-12 autoantibody-positive patients than in those with anti-Jo-1, there were no significant mortality differences between the autoantibody groups. Possible explanations for differences among the studies might be due to characteristics of the populations and disease duration before diagnosis (52).
Myositis occurs more frequently in anti-Jo-1-positive patients than non anti-Jo-1 (49). Clinically, muscle involvement may be consistent with both polymyositis and dermatomyositis, while histologically, perifascicular atrophy, a characteristic feature of dermatomyositis, although with perifascicular necrosis, seems to be characteristic for the ASSD group. In addition, electron microscopy-based nuclear actin aggregation has been seen in ASSD muscle biopsies but not in other IIM subgroups (58–60). Anti-PL-12 antibodies have also been reported in patients with immune-mediated necrotizing myopathy (61). Esophageal muscles with subsequent dysphagia are affected in one-third of patients with ASSD (62). Arthritis usually occurs at the onset of ASSD, more frequently in anti-Jo-1 compared to other ASSD autoantibody groups. The arthritis spectrum in ASSD is non-erosive rheumatoid arthritis-like with smaller joints more often involved than the larger joints, especially in case of co-occurrence of anti-aaRS and ACPA antibodies (5-14% of ASSD cases) (48, 49). Mechanic’s hands, described as erythematous and fissured hyperkeratosis of the palmar or lateral edges of the fingers, highly correlate with ASSD diagnosis, although they have been reported in other overlap myositis, especially in the presence of anti Pm-Scl and anti-MDA5 autoantibodies. Mechanic’s hands have not been reported as an isolated initial manifestation (63, 64). Other skin lesions such as Gottron’s papules/sign, heliotrope rash, shawl, holster, or V sign, typically seen in DM, have also been described in patients with ASSD (65, 66). Raynaud’s phenomenon has more frequently been observed in patients with anti-PL-12 and anti-PL-7 than in patients with other anti-aaRS autoantibodies (49, 67). Relapsing-remitting fever is one of the symptoms in 20%–60% of ASSD patients (49).
Increased risk of cancer in myositis especially in dermatomyositis has been extensively studied and reported. However, there are some discrepancies in the literature concerning prevalence of cancer in ASSD due to the varying definitions of cancer-associated myositis, the timing of malignancies, insufficient number of patients, and referral bias. Some studies show that the presence of ASSD autoantibodies, in particular anti-Jo1 and -EJ, have been associated with a lower risk of cancer (68–72), whereas some other studies and case reports describe an increased risk of cancer in anti-Jo-1-positive patients (37, 73, 74). On the other hand, a retrospective study including a small cohort showed that being male, over the age of 60, and the coexistence of anti-SSA/Ro autoantibodies along with ASSD were risk factors for the development of neoplasm (51). Hence, larger studies with proper age-matched controls are required to determine if there is an association between cancer and ASSD. Moreover, systematic reviews and meta-analysis recommend careful cancer screening in PM/DM patients with ILD, especially those with multiple risk factors for malignancy (75).
Autoantigenic aaRSs in Anti-Synthetase Syndrome and Clinical Associations
There are eight yet identified aaRSs that have become targets of the immune system with the development of autoantibodies and the clinical ASSD. The pathogenic mechanisms for this syndrome are not clear. The individual aaRSs have somewhat different properties and functions within the cells and extracellular functions. Here, we give an overview of these eight aaRSs and detailed clinical associations to the corresponding autoantibodies (Table 3).
Histidyl-tRNA Synthetase
Histidyl tRNA synthetase (HisRS) is a homodimeric enzyme, whose location is mainly cytoplasmic. HisRS is responsible for the incorporation of histidine into a growing peptide (95–97). The N-terminal fragment or CD includes the residues 1–320 and is responsible for the specific aminoacylation of tRNA (98). The C-terminal fragment is essential for the dimeric structure of the enzyme. Additionally, at least two HisRS-splice variants (SV) have been identified lacking the CD (Figure 2). Besides the intracellular cytoplasmic location of HisRS, this enzyme can also be found in the extracellular compartment (98), although its extracellular functions have not been fully clarified. Both in vitro and animal studies have suggested that HisRS is involved in several regulatory mechanisms of cell metabolism and the regulation of immune responses. Howard et al. have demonstrated that the N-terminal domain serves as a chemoattractant for naïve lymphocytes and immature dendritic cells (DCs) through interaction with CCR5 (77). The N-terminal has also been considered to be the immunodominant epitope, specifically the 1–60 amino acid fragment (100). In particular, in vitro studies performed in myositis samples showed that T-cell stimulation assays using a 13-mer peptide from the HisRS N-terminal domain elicited an inflammatory response in blood and bronchoalveolar lavage fluid (BALF) T cells (78). Interestingly, in vivo the levels of extracellular HisRS were higher in sera of anti-Jo-1-negative patients compared to healthy individuals and were almost undetectable in patients with Jo-1 antibodies. This observation suggested that another possible immune mechanism could be involved, such as the formation of immune complexes of autoantibodies with the protein, but this still needs to be confirmed.
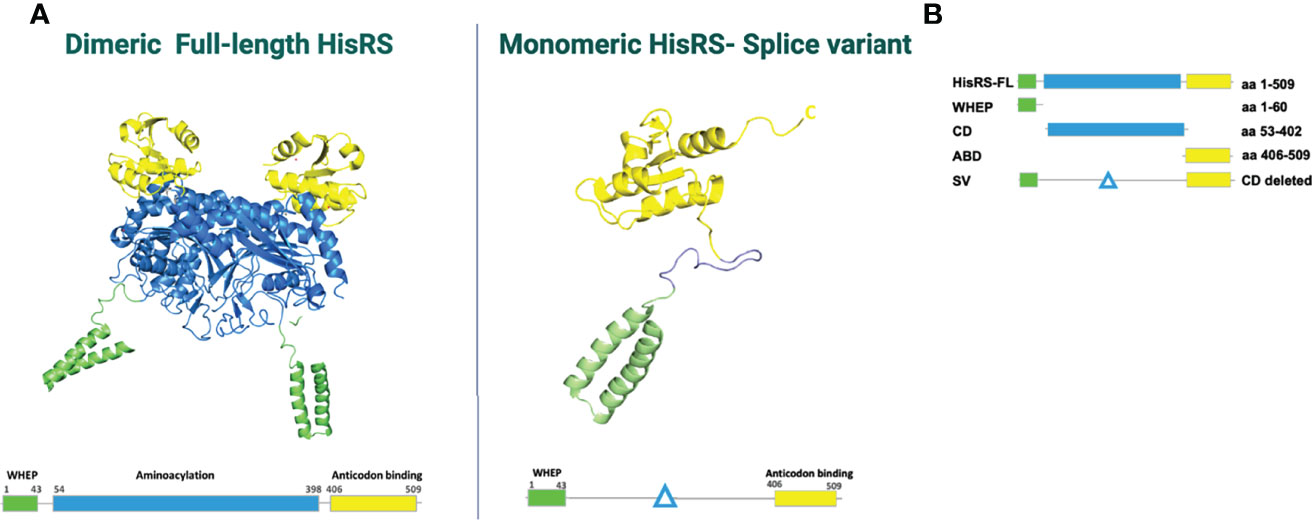
Figure 2 (A) HisRS structure visualized using PDB ID: 4G84 and 2LW7 (98). (B) Schematic figure of HisRS constructs, adapted from Notarnicola et al. (99). HisRS-FL, HisRS-full length; WHEP, WHEP domain; CD, catalytic domain; ABD, anti-codon binding domain; SV, splice variant.
Anti-HisRS autoantibodies (anti-Jo-1) were initially identified in 1983 by Mathews M. and Bernstein R (9). Anti-Jo-1 autoantibodies are the most common among the myositis-specific autoantibodies (MSAs) with frequencies between 20% and 30% in patients with IIM (101–103).
Worldwide epidemiological studies have shown that patients presenting with anti-Jo-1 autoantibodies can develop ILD in up to 90% of the cases (38, 41, 104). In the American and European Network of Antisynthetase Syndrome (AENEAS) cohort study of anti-Jo-1-positive patients, ILD was present in 50% at disease onset and in 84% after 80-month follow-up (67).
Threonyl-tRNA Synthetase
Threonyl-tRNA synthetase, ThrRS, also referred to as TRS and the target of anti-PL-7 autoantibodies, is an aminoacyl-tRNA synthetase that catalyzes the aminoacylation of tRNA by transferring threonine. Besides its essential function of catalyzing aminoacylation, ThrRS can be secreted into the extracellular compartment where it can have other non-canonical functions (105). In particular, the extracellular secretion of ThrRS has been associated with the exposure of vascular endothelial cells with proinflammatory cytokines such as tumor necrosis factor-a (TNF-a) or vascular endothelial growth factor (VEGF). Additionally, in vitro and in vivo assays have shown that ThrRS stimulates endothelial cell migration and angiogenesis, indicating that ThrRS can acts as an angiogenic pro-migratory extracellular signalling molecule (79).
A recent study showed that ThrRS can induce maturation and activation of DCs with a Th1 response in vitro and in vivo, upregulation of CD4+ and CD8+ T cells, increased IFN-gamma secretion associated with viral clearance in influenza virus (H1N1)-infected mice, and increased IL-12 production by the MAPK/NF-kB pathways. Interestingly, CD4+IFNg+ and CD8+IFNg+ T cells and IFNg levels of ThrRS-DC immunized mice were significantly upregulated in BALF compared with the control group (80).
In the muscle, ThrRS may play a role as negative regulator in myogenic differentiation, by inhibiting Axin1, through the kinase c-Jun N-terminal (JNK), a downstream target of ThrRS. In particular, the presence of UNE-T or TGS domains was necessary for ThrRS myogenic function (106). Further studies analyzing the role of this protein in the innate and immune response as well as the ThrRS expression in muscular diseases are still needed to further understand the role of this aaRS in the disease.
There are few reports on clinical presentations of patients specifically with anti-PL-7 autoantibodies. In a European multicenter study, the frequency of anti-PL7 was 1.87% (18/964) (107), whereas a single-center retrospective cohort from China reported that anti-PL-7 autoantibodies had the same frequency (27% 30/108) as anti-Jo-1 autoantibodies (30.5% 33/108) (108). In Asian studies, the most common ILD pattern observed in anti-PL-7-positive patients was NSIP (15%), which was in line with an earlier study that reported mainly NSIP patterns (50%) followed by OP (41.7%) (109). In this study, UIP was only observed in the anti-PL7-positive group (4%) and was associated with a low response to therapy (108). In addition, the presence of this autoantibody predicted the long-term deterioration in ILD (110).
Alanyl-tRNA Synthetase
In contrast to most aaRS, alanyl-tRNA synthetase (AlaRS), the target of anti-PL-12 autoantibodies, recognizes its substrate in an anticodon-dependent manner with recognition of a single G3:U70 wobble base pair, which is the dominant identity determinant for tRNA aminoacylation (82). This simplified mechanism increases the likelihood of mischarging by AlaRS and has been associated with neurodegenerative disorders such as Charcot-Marie-Tooth disease (CMT) type 2, which is characterized by axonal peripheral neuropathy with muscle weakness, wasting, and impaired sensation in the extremities (82).
Autoantibodies against alanyl-tRNA synthetase, also known as anti-PL-12, was the third myositis-specific autoantibody identified in 1986 by Bunn et al. (12). Unlike anti-Jo-1 and anti-PL-7 autoantibodies, anti-PL-12 IgG recognizes independently two antigens: alanyl-tRNA synthetase and its cognate tRNAAla, suggesting that this recognition occurs by separate autoantibodies. The antigenic epitope is located in the anticodon region (83), and an epitope mapping of this protein identified the immunoreactive region outside the CD, within amino acids 730–951 (111) (Table 2). In the case of anti-PL-12 autoantibodies, the severity of the clinical manifestations is mainly driven by the ILD, which was notable in cases associated with pulmonary hypertension (55). Both in American and European reports, a series of anti-PL-12-positive patients showed a low prevalence of muscular involvement, mechanic’s hands, and Raynaud’s phenomena (55, 112, 113). Regarding the histopathologic and radiographic features, UIP is the most common pattern of lung involvement (114).
Glycl-tRNA Synthetase
Glycyl-tRNA synthetase (GlyRS), the target of anti-EJ autoantibodies, is a dual-localized homodimeric aaRS, found in both the cytoplasm and the mitochondria. It catalyzes the attachment of glycine to its cognate tRNA. Mutations in the gene coding for human GlyRS are associated to neurodegenerative diseases including the distal spinal muscle atrophy type V and CMT disease (115). The impairment in the mitochondrial metabolism in neurons is one of the mechanisms through which mutations in GlyRS lead to neurological diseases (116). GlyRS has been also shown to circulate in serum of human subjects but, in contrast to HisRS, extracellular levels of GlyRS were not found to be significantly different between healthy individuals and patients with myositis (97). In vitro experiments have demonstrated that it can be secreted from macrophages in response to Fas ligand that is released from tumor cells (85). Therefore, it has been suggested that GlyRS is involved in immune surveillance against cancer (85).
Autoantibodies against GlyRS (anti-EJ) were described for the first time in 1990 by Targoff in patients with myositis and ILD (13). In anti-EJ-positive patients from the AENEAS cohort and a large Chinese cohort, ILD was the most frequent clinical manifestation, being isolated in almost 40% of the patient group (48, 117). ILD has been reported as an early manifestation of the anti-EJ-ASSD disease course (117, 118). Regarding ILD patterns, NSIP, UIP, OP, and DAD have been described in patients with anti-EJ antibodies (117–119). OP was also found to be an independent risk factor for developing rapidly progressive ILD (117). Among other ASSD features, fever, mechanic’s hands, and Raynaud´s phenomenon have been reported as accompanying findings (48, 117, 119).
Isoleucyl-tRNA Synthetase
Isoleucyl-tRNA synthetase, IleRS (IARS), the target of anti-OJ autoantibodies, is a component of the multi-enzyme complex (MSC) described above that is important for the stabilization of the interactions between the components (28–30, 120). In addition, IleRS is important for protein synthesis and signaling pathways.
The anti-isoleucyl-tRNA synthetase autoantibody (also known as anti-OJ) was identified in 1990 and was initially described in a patient with a history of severe, progressive pulmonary fibrosis and pulmonary hypertension (13). This autoantibody has a low prevalence (<5%) among patients with IIM (57). Anti-OJ autoantibodies react with lysyl-tRNA synthetase (KARS) and the epitope of anti-OJ autoantibodies could be based on quaternary interactions between MSC components (57), making the detection in the clinical practice problematic. The low frequency of this autoantibody might be associated to the elusiveness of the primary target(s) of anti-OJ. In the clinical setting, multiplex assays based on immunoblotting such as line immunoassay (LIA) and dot blot assays (DBA) have poor performance of anti-OJ (121, 122). Anti-OJ autoantibodies have also been difficult to detect in ELISA, even with the use of recombinant proteins from Escherichia coli and Hi-5 (insect cell line), suggesting that either anti-OJ autoantibodies might recognize other components of the MSC or that the structural conformation of the complex is important for the recognition by this autoantibody (123). To date, immunoprecipitation (IP) remains the preferred method for anti-OJ autoantibody detection.
A review of 52 published cases identified ILD as the main clinical manifestation in 90% of the anti-OJ positive cases, with the patterns of UIP, OP, and NSIP being the most frequent. Prevalence of myositis seems to vary according to the criteria applied for ASSD classification and the ethnicity of the population included in the studies. In an Asian cohort, the frequency of severe myositis was reported to be as high as 57% (62), while in the AENEAS cohort study, 40% of the patients with anti-OJ autoantibodies had hypomyopathic forms of ASSD or never developed myositis (124). Arthritis, fever, Raynaud’s phenomenon, and mechanic’s hands are also present, but in a lower frequency, being an incomplete presentation of the ASSD frequent (57).
Asparaginyl-tRNA Synthetase
The asparaginyl-tRNA synthetase (AsnRS), the target of anti-KS autoantibodies, catalyzes the attachment of asparagine to its cognate tRNA during translation. As for non-canonical functions, AsnRS has been shown to be involved in growth regulation mediated by the Hippo signaling pathway (a pathway involved in growth regulation, dysregulation observed in many cancers) and, therefore, possibly implicated in tumorigenesis (125). Studies also reported pro-inflammatory functions of AsnRS. In particular, AsnRS was shown to induce CCR3-expressing cells to migrate and, like HisRS, chemoattract DCs (77). The non-translational chemokine activity of AsnRS is thought to be exerted by an additional domain at the N-terminal of the protein sequence, not present in the prokaryotic system. The modulating activity of AsnRS on CCR3 signaling has been suggested to be implicated in the pathophysiology of ASSD and ILD (126). Similar to GlyRS, AsnRS has been detected in extracellular compartments and serum levels were not significantly different between myositis patients and healthy individuals (97).
Autoantibodies against AsnRS (anti-KS) occur in less than 5% of patients with IIM and were first described in 1999 in two patients with ILD and no evidence of myositis (14). A review of the published literature about the clinical features associated with anti-KS autoantibodies has shown that ILD with NSIP or UIP patterns is the dominant feature, being the only manifestation in 50% of patients (63). Myositis seems to rarely affect this group of patients, while arthritis, Raynaud’s phenomenon, and mechanic’s hands may occur in one quarter of them (127)
Phenylalanyl-tRNA Synthetase
Phenylalanyl-tRNA synthetase, PheRS, the target of anti-Zo autoantibodies, has been linked to cancer by reports of increased expression in some cancers (128–130), and suggested to be a prognostic indicator for some cancers (131). Thus, expression of PheRS was increased in gastric cancer tissue and the levels of expression correlated with distant metastasis and poor survival (132). Mechanistically, PheRS regulates anti-apoptotic signaling and cell proliferation through its upstream interaction proteins (133); however, the regulation of oncogenesis and development of gastric cancer by PheRS need further investigation.
The presence of autoantibodies against PheRS, anti-Zo, was first described in 2007 in a patient with ASSD (15). Anti-Zo is a rare anti-synthetase autoantibody. The largest cohort positive for anti-Zo autoantibodies was a case series of nine patients in UK (134). Seven (78%) of the patients had ILD, and two patients had evidence of muscle involvement, suggesting that anti-Zo autoantibodies are associated with features of ASSD. Moreover, two-thirds of the patients had autoantibodies against anti-Ro52, which has been previously reported to co-exist with other anti-synthetase autoantibodies and more severe ILD (135, 136). A more recent study reported the prevalence of anti-Zo autoantibodies as 1.4% in patients with ILD and novel associations of anti-Zo with connective tissue-disease related ILD (CTD-ILD) and idiopathic pneumonias (45).
Tyrosyl-tRNA Synthetase
Tyrosyl-tRNA synthetase or TyrRS, the target of anti-YRS/anti-HA autoantibodies, can split into two separate fragments, and these fragments have distinct cytokine activities whereas the full-length TyrRS is inactive for cytokine activities (42, 137). Secretion of both fragments are induced by leukocyte digestion and active forms of TyrRS can be naturally generated by alternative splicing or proteolytic cleavage (42, 137). The fragment that includes the C-terminal domain induced migration of mononuclear phagocytes and stimulated production of TNFa. On the other hand, the N-terminal domain induced migration of polymorphonuclear leukocytes (PMNs) in a dose-dependent manner very similar to the CXC chemokine interleukin -8 (IL-8) (42). Similarities in the effects of IL-8 and mini TyRS (N-terminal) on PMNs indicate a possible functional correlation between mini TyrRS and IL-8 activity. In addition, human mini TyrRS induced angiogenesis in vivo in different organisms similar to IL-8 (138). The N-terminal domain of TyrRS was also reported to be present in platelets, from which they are released by unknown mechanisms and to regulate monocytes/macrophage differentiation during bacterial infections (139).
The first report of autoantibodies against TyrRS referred to as anti-YRS or anti-HA was published in 2005 in a patient with ASSD features (17). The prevalence of anti-HA autoantibodies in a large cohort of patients with ILD (n = 1,194) was 2% (45).
Possible Autoantigens in Other Diseases
Lysyl-tRNA Synthetase
As mentioned previously, aaRSs gained additional domains and functions throughout evolution. One example is Lysyl-tRNA synthetase (LysRS), also referred to as KRS or SC. LysRS binds to macrophages and monocytes leading to macrophage migration and TNF production when present in the extracellular media (140). Moreover, there are many reports in the literature indicating a role for LysRS in human immunodeficiency virus (HIV) replication, signal transduction, and neurodegenerative diseases (141). The mechanism of how LysRS contributes to HIV replication has been well studied, and it has been established that HIV recruits LysRS to serve as the reverse transcriptase through the interaction of LysRS and Gag protein (142–149). In addition, the contribution of LysRS in cancer has been established by many studies. LysRS has been shown to promote cancer metastasis by inducing cancer cell migration through the interaction with 67LR protein (150). LysRS can be secreted by cancer cells to induce inflammatory responses (151), whereas a more recent study showed a novel mechanism for the secretion of LysRS via exosomes or exosome-like extracellular vesicles from cancer cells, which is controlled by a caspase-8-dependent pathway (94).
There is also a possible connection of LysRS with amyotrophic lateral sclerosis (ALS). In some patients with ALS, a mutation in SOD1 is observed. The mutation in SOD1 induces apoptosis of motor neurons, thus leading to the onset of neurodegeneration and interestingly LysRS associates with mutant but not wild-type SOD1 (152). The abnormal interaction between SOD1 and LysRS contributes to mitochondrial dysfunction in ALS (153). In addition, a loss-of-function mutation in the CD of LysRS was implicated in CMT disease. It was reported that the mutation in LysRS severely affects the enzyme activity (154).
Glutaminyl-tRNA Synthetase
Glutaminyl-tRNA synthetase (GlnRS) is one of the two enzymes in this family that is not found in all organisms, such as Gram-positive eubacteria, archaebacteria, and organelles, suggesting that this aaRS has evolved along independent evolutionary pathways (155). Additionally, GlnRS appears to be the largest polypeptide in the human multienzyme synthetase complex and shares three significant regions of sequence similarity with the translation elongation factor EF-1 (156).
In humans, it is bifunctional, being specific for two amino acids (glutamine and proline) (28), acquiring the term glutamyl-prolyl-tRNA synthetase [Glu-ProRS or EPRS (157)]. Among the non-canonical functions of GlnRS is the ability to block apoptosis trough a negative regulation of the apoptosis signal-regulating kinase 1 (ASK1) (158). Additionally, in vivo studies in a rat model to evaluate changes in sensory neurons after nerve injury showed that the expression of GlnRS was decreased in the dorsal root ganglia (DRG). Thus, this aaRS may play a potential role as a neurotransmitter; however, further research is required (159). In addition, GlnRS expression may affect macrophage recruitment to injured DRG neurons (159–161).
Mutations in the gene encoding GlnRS have been associated to early-onset epileptic encephalopathy (162, 163). GlnRS deficiency has been associated with neurodegenerative disorders associated with severe developmental delay, microcephaly, delayed myelinization, and intractable epilepsy, which seems to be more severe than other disorders associated with mutations in tRNA synthetases (164).
Tryptophanyl-tRNA Synthetase
Mammalian tryptophanyl t-RNA synthetase, TrpRS or WRS, has gained functions such as alternative splicing and proteolytic cleavage through evolution. This aaRSs is found not only in the cytosol but also in the nucleus. In the cytosol, it plays a role in innate immune responses, angiogenesis, and type II IFN signaling.
TrpRS is secreted into the extracellular milieu by monocytes upon infection with certain pathogens and it interacts with TLR2 and TLR4 leading to the secretion of TNFα, neutrophil infiltration, and increased phagocytotic abilities (90–92). These responses help to clear out infections at the early phase, indicating the importance of TrpRS as a ligand for immune regulation through TLR signaling. In support of this hypothesis, high levels of TrpRS were found in sera of patients with sepsis, a potentially fatal complication due to infection, when compared to healthy controls (92). Additionally, increased TrpRS expression from CD4+ T cells resisted indoleamine 2,3-dioxygensase (IDO)-mediated immunosuppression from DC in Graves’ disease (89). Autoantibodies against TrpRS have been found in patients with autoimmune diseases, where the clinical features were associated with rheumatoid arthritis (33–35).
Seryl-tRNA Synthetase
There are few studies investigating the non-canonical functions of seryl-tRNA synthetase, SerRS. It was reported that SerRS interacts with a transcription factor called Yin Yang 1, to form a complex that negatively regulates vascular endothelial cell growth factor A during angiogenesis (165)
Autoantibodies against SerRS have been detected in a few cases of systemic lupus erythematosus or rheumatoid arthritis but not in myositis (36, 77, 166).
Others
So far, there are no reports in the literature about the remaining eight aaRSs being recognized as autoantigens. However, recently, several mutations in encoding genes in mitochondrial or cytosolic compartments have been identified. These mutations resulted in dysfunctional aaRSs that lead to a variety of multi-organ, neuronal, and metabolic disorders (Table 4) (8). Fifty-five percent of mutations in genes encoding for mitochondrial aaRSs were associated with disease, whereas in cytoplasmic aaRSs, the percentage is 20% (177). Notably, mutations in genes encoding for mitochondrial aaRS are often associated with some form of myopathy (Table 4).
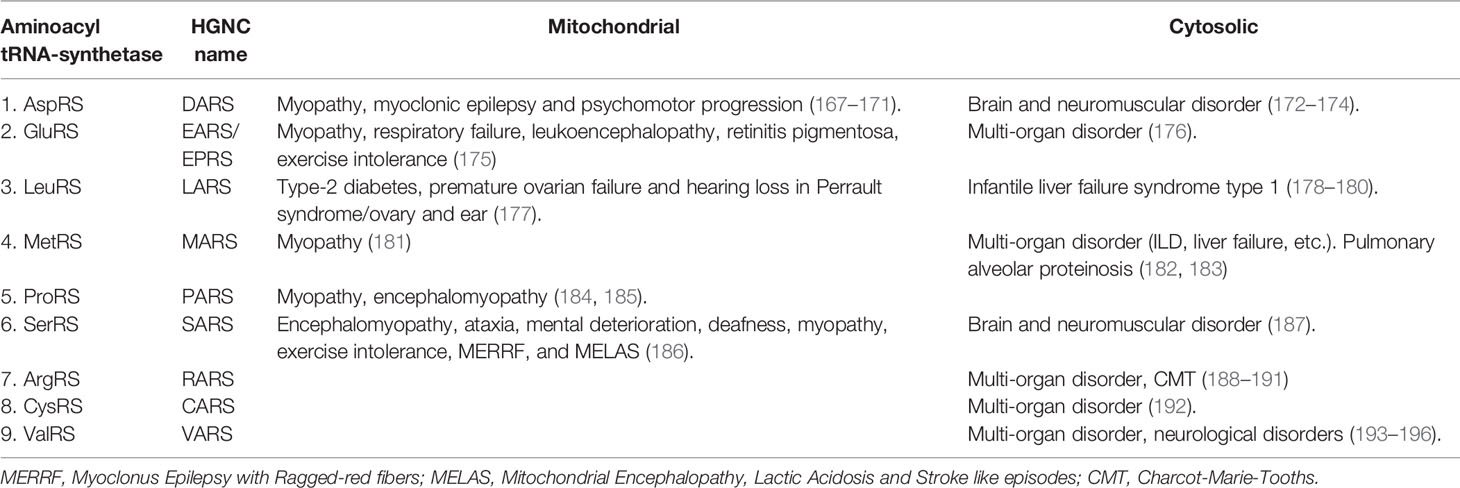
Table 4 Mutations in genes encoding mitochondrial or cytosolic aaRSs and associated disorders (8).
Mechanisms of Pathogenicity
There are different ways on how aaRSs contribute to the disease pathogenesis as discussed in this review. One mechanism is due to the aaRSs being recognized as autoantigens, thus inducing an abnormal immune response. Other possible mechanisms in disease pathogenesis are impairments in their canonical or non-canonical functions due to mutations as described above.
In many systemic autoimmune diseases, it has been established that substrates of granzyme B cleavage, which is a serine protease involved in apoptosis, are more often autoantigenic rather than the native form of the protein (39, 197). Autoantigens are usually secreted or located extracellularly, in membranes, or in apoptotic blebs and contain specific structures or sequences such as coiled-coil motifs, granzyme B cleavage sites, or ELR (Glu-Leu-Arg) motifs (198). Importantly IleRS, HisRS, and AlaRS all go through granzyme B cleavage and release fragments, which contain epitopes recognized by autoantibodies (128). For example, the WHEP domain, released from HisRS upon granzyme B cleavage, which is 50 aa long, has a helix-turn-helix conformation that is also referred as a “coiled-coil”. This has been recognized as a major epitope for anti-Jo-1 autoantibodies (98). Similarly, granzyme B cleavage sites are found in GlyRS, AlaRS, and IleRS, which are autoantigens for anti-EJ, anti-PL12, and anti-OJ, respectively (8). Although it is still a mystery how aaRSs are released to the extracellular environment to accomplish all the non-canonical functions, it has been proposed that it might be through passive release from cells that are undergoing necrosis. At least five aaRSs, HisRS, ThrRS, GlyRs, TyrRs, and AsnR, have been reported to be secreted out of cells (8, 42–44, 98, 199) during tissue damage, angiogenesis, and in cancer. For the remaining four aaRSs that have been reported as autoantigens in ASSD, (i) AsnRS has also been detected in serum of healthy individuals and myositis patients possibly due to tissue damage, (ii) the levels of PheRS were reported to be associated with various types of cancer whereas there are no reports yet on the extracellular location of (iii) IleRS and (iv) AlaRS. Interestingly, some of the aaRSs are secreted or directly act on monocytes such as TrpRS, GlyRS, TyrRS, and LysRS during infections, which could be a link between infection and ASSD (85, 90–92, 139, 140).
In addition to autoantigenic motifs, mutations in genes encoding aaRSs that lead to impaired functions were reported in multi-organ disorders, neurological diseases, or cancer (Table 3). The most common aaRS-associated monogenic disorder is CMT, a genetic peripheral nerve disorder (8). Currently, seven known members of aaRSs (GlyrRS, TyrRS, AlaRS, HisRS, TrpRS, MetRS, and LysRS), have been implicated in CMT disease, representing the largest protein family in CMT etiology, although the role of aaRSs in this disorder is still unclear (115, 200).
Conclusions
ASSD is an autoimmune condition characterized by the presence of autoantibodies targeting one of several aminoacyl t-RNA synthetases (aaRS) along with distinct clinical features. Although in the literature, reference to autoantibodies against 11 aaRSs can be found, autoantibodies against only eight aaRSs have been identified so far in ASSD patients as described in this review. We summarized clinical features of ASSD, what is known about ASSD-associated aaRSs, and also reviewed the known properties of the remaining aaRSs. It seems that even though the remaining aaRSs have not been confirmed to be autoantigens in diseases, their non-canonical functions inside and outside of the cell and impairment in their functions contribute to the pathogenesis of diseases such as cancer, multi-organ disorders, or neurological disorders. This strongly implicates that aaRSs have an essential role in the regulation of immune responses and more attention is needed to understand the underlying mechanisms of their pathogenic functions.
Author Contributions
AG-F, AN, and BH: writing. AG-F and BH: figure and tables. BH: concept. AG-F, AN, BH, and IL: revision and proofreading. All authors contributed to the article and approved the submitted version.
Funding
This work was supported by The Swedish Research Council (2020-01378), the Swedish Rheumatism Association, King Gustaf V 80 Year Foundation, King Gustaf V:s and Queen Victoria´s Freemason foundation, Funds at the Karolinska Institutet (KID), Stockholm Regional Council (ALF), Heart and Lung Foundation (2020-00380), Professor Nanna Svartz Foundation, and Karolinska Institutet Research Foundations (2020-02515). Nanna svartz: 2020-00380 KI Research Foundation : 2020-02515 The Swedish Research Council: 2020-01378
Conflict of Interest
IL has received consulting fees from Corbus Pharmaceuticals, Inc. and research grants from Astra Zeneca and has been serving on the advisory board for Astra Zeneca, Bristol Myers Squibb, Corbus Pharmaceutical, EMD Serono Research & Development Institute, Argenx, Octapharma, Kezaar, Orphazyme, and Janssen and has stock shares in Roche and Novartis.
The remaining authors declare that the research was conducted in the absence of any commercial or financial relationships that could be construed as a potential conflict of interest.
Publisher’s Note
All claims expressed in this article are solely those of the authors and do not necessarily represent those of their affiliated organizations, or those of the publisher, the editors and the reviewers. Any product that may be evaluated in this article, or claim that may be made by its manufacturer, is not guaranteed or endorsed by the publisher.
References
1. Witt LJ, Curran JJ, Strek ME. The Diagnosis and Treatment of Antisynthetase Syndrome. Clin Pulm Med (2016) 23(5):218–26. doi: 10.1097/CPM.0000000000000171
2. Connors GR, Christopher-Stine L, Oddis CV, Danoff SK. Interstitial Lung Disease Associated With the Idiopathic Inflammatory Myopathies: What Progress has Been Made in the Past 35 Years? Chest (2010) 138(6):1464–74. doi: 10.1378/chest.10-0180
3. Cojocaru M, Cojocaru IM, Chicos B. New Insights Into Antisynthetase Syndrome. Maedica (Bucur) (2016) 11(2):130–5.
4. Hervier B, Devilliers H, Stanciu R, Meyer A, Uzunhan Y, Masseau A, et al. Hierarchical Cluster and Survival Analyses of Antisynthetase Syndrome: Phenotype and Outcome Are Correlated With Anti-tRNA Synthetase Antibody Specificity. Autoimmun Rev (2012) 12(2):210–7. doi: 10.1016/j.autrev.2012.06.006
5. Marie I, Josse S, Hatron PY, Dominique S, Hachulla E, Janvresse A, et al. Interstitial Lung Disease in Anti-Jo-1 Patients With Antisynthetase Syndrome. Arthritis Care Res (Hoboken) (2013) 65(5):800–8. doi: 10.1002/acr.21895
6. Rajendran V, Kalita P, Shukla H, Kumar A, Tripathi T. Aminoacyl-tRNA Synthetases: Structure, Function, and Drug Discovery. Int J Biol Macromol (2018) 111:400–14. doi: 10.1016/j.ijbiomac.2017.12.157
7. Schimmel P. Aminoacyl tRNA Synthetases: General Scheme of Structure-Function Relationships in the Polypeptides and Recognition of Transfer RNAs. Annu Rev Biochem (1987) 56:125–58. doi: 10.1146/annurev.bi.56.070187.001013
8. Jiang L, Jones J, Yang XL. Human Diseases Linked to Cytoplasmic aminoacyl-tRNA Synthetases. Enzymes (2020) 48:277–319. doi: 10.1016/bs.enz.2020.06.009
9. Mathews MB, Bernstein RM. Myositis Autoantibody Inhibits histidyl-tRNA Synthetase: A Model for Autoimmunity. Nature (1983) 304(5922):177–9. doi: 10.1038/304177a0
10. Nishikai M, Reichlin M. Heterogeneity of Precipitating Antibodies in Polymyositis and Dermatomyositis. Characterization of the Jo-1 Antibody System. Arthritis Rheumatol (1980) 23(8):881–8. doi: 10.1002/art.1780230802
11. Mathews MB, Reichlin M, Hughes GR, Bernstein RM. Anti-threonyl-tRNA Synthetase, A Second Myositis-Related Autoantibody. J Exp Med (1984) 160(2):420–34. doi: 10.1084/jem.160.2.420
12. Bunn CC, Bernstein RM, Mathews MB. Autoantibodies Against alanyl-tRNA Synthetase and Trnaala Coexist and Are Associated With Myositis. J Exp Med (1986) 163(5):1281–91. doi: 10.1084/jem.163.5.1281
13. Targoff IN. Autoantibodies to Aminoacyl-Transfer RNA Synthetases for Isoleucine and Glycine. Two Additional Synthetases Are Antigenic in Myositis. J Immunol (1990) 144(5):1737–43.
14. Hirakata M, Suwa A, Nagai S, Kron MA, Trieu EP, Mimori T, et al. Anti-KS: Identification of Autoantibodies to Asparaginyl-Transfer RNA Synthetase Associated With Interstitial Lung Disease. J Immunol (1999) 162(4):2315–20.
15. Betteridge Z, Gunawardena H, North J, Slinn J, McHugh N. Anti-Synthetase Syndrome: A New Autoantibody to Phenylalanyl Transfer RNA Synthetase (Anti-Zo) Associated With Polymyositis and Interstitial Pneumonia. Rheumatol (Oxf) (2007) 46(6):1005–8. doi: 10.1093/rheumatology/kem045
16. Mimori T, Imura Y, Nakashima R, Yoshifuji H. Autoantibodies in Idiopathic Inflammatory Myopathy: An Update on Clinical and Pathophysiological Significance. Curr Opin Rheumatol (2007) 19(6):523–9. doi: 10.1097/BOR.0b013e3282f01a8c
17. Hashish LTE, Trieu E, Sadanandan O, Targoff IN. Identification of Autoantibodies to tyrosyl-tRNA Synthetase in Dermatomyositis With Features Consistent With Antisynthetase Syndrome. Arthritis Rheumatol (2005) 52.
18. Love LA, Leff RL, Fraser DD, Targoff IN, Dalakas M, Plotz PH, et al. A New Approach to the Classification of Idiopathic Inflammatory Myopathy: Myositis-Specific Autoantibodies Define Useful Homogeneous Patient Groups. Med (Baltimore) (1991) 70(6):360–74. doi: 10.1097/00005792-199111000-00002
19. Targoff IN, Trieu EP, Plotz PH, Miller FW. Antibodies to Glycyl-Transfer RNA Synthetase in Patients With Myositis and Interstitial Lung Disease. Arthritis Rheumatol (1992) 35(7):821–30. doi: 10.1002/art.1780350718
20. Crick F. On Degenerate Templates and the Adaptor Hypothesis (A Note for the RNA Tie Club). Cambridge, England: Francis Crick archives. (1955).
21. Ibba M, Becker HD, Stathopoulos C, Tumbula DL, Söll D. The Adaptor Hypothesis Revisited. Trends Biochem Sci (2000) 25(7):311–6. doi: 10.1016/S0968-0004(00)01600-5
22. Siekevitz P ZP. In Vitro Incorporation of 1-14C-DL-Alanine Into Proteins of Rat-Liver Granular Fractions. Fed Proc (1951) 10:246–7. doi: 10.1016/S0021-9258(18)57260-4
23. Ramakrishnan V. Unraveling the Structure of the Ribosome (Nobel Lecture). Angew Chem Int Ed Engl (2010) 49(26):4355–80. doi: 10.1002/anie.201001436
24. Ribas de Pouplana L, Schimmel P. Two Classes of tRNA Synthetases Suggested by Sterically Compatible Dockings on tRNA Acceptor Stem. Cell (2001) 104(2):191–3. doi: 10.1016/S0092-8674(01)00204-5
25. Eriani G, Delarue M, Poch O, Gangloff J, Moras D. Partition of tRNA Synthetases Into Two Classes Based on Mutually Exclusive Sets of Sequence Motifs. Nature (1990) 347(6289):203–6. doi: 10.1038/347203a0
26. Mirande M, Gache Y, Le Corre D, Waller JP. Seven Mammalian aminoacyl-tRNA Synthetases Co-Purified as High Molecular Weight Entities Are Associated Within the Same Complex. EMBO J (1982) 1(6):733–6. doi: 10.1002/j.1460-2075.1982.tb01238.x
27. Wolfe CL, Warrington JA, Treadwell L, Norcum MT. A Three-Dimensional Working Model of the Multienzyme Complex of aminoacyl-tRNA Synthetases Based on Electron Microscopic Placements of tRNA and Proteins. J Biol Chem (2005) 280(46):38870–8. doi: 10.1074/jbc.M502759200
28. Kwon NH, Fox PL, Kim S. Aminoacyl-tRNA Synthetases as Therapeutic Targets. Nat Rev Drug Discov (2019) 18(8):629–50. doi: 10.1038/s41573-019-0026-3
29. Havrylenko S, Mirande M. Aminoacyl-tRNA Synthetase Complexes in Evolution. Int J Mol Sci (2015) 16(3):6571–94. doi: 10.3390/ijms16036571
30. Lee SW, Cho BH, Park SG, Kim S. Aminoacyl-tRNA Synthetase Complexes: Beyond Translation. J Cell Sci (2004) 117(Pt 17):3725–34. doi: 10.1242/jcs.01342
31. Guo M, Yang XL, Schimmel P. New Functions of aminoacyl-tRNA Synthetases Beyond Translation. Nat Rev Mol Cell Biol (2010) 11(9):668–74. doi: 10.1038/nrm2956
32. Yao P, Fox PL. Aminoacyl-tRNA Synthetases in Medicine and Disease. EMBO Mol Med (2013) 5(3):332–43. doi: 10.1002/emmm.201100626
33. Paley EL, Alexandrova N, Smelansky L. Tryptophanyl-tRNA Synthetase as a Human Autoantigen. Immunol Lett (1995) 48(3):201–7. doi: 10.1016/0165-2478(95)02469-7
34. Vartanian OA. Detection of Autoantibodies Against Phenylalanyl-, Tyrosyl-, and tryptophanyl-tRNA-Synthetase and Anti-Idiotypic Antibodies to it in Serum From Patients With Autoimmune Diseases. Mol Biol (Mosk) (1991) 25(4):1033–9.
35. Bolgarin RN, Rodnin NV, Sidorik LL. Autoantibodies to tryptophanyl-tRNA-Synthetase in Systemic Autoimmune Diseases. Mol Biol (Mosk) (1998) 32(4):745–9.
36. Sidorik LL. Seryl-tRNA Synthetase – A Novel Autoantigen During Systemic Autoimmune Diseases. Biopolym Cell (1994) 10(3-4):68–74. doi: 10.7124/bc.0003B0
37. Marie I, Josse S, Decaux O, Dominique S, Diot E, Landron C, et al. Comparison of Long-Term Outcome Between Anti-Jo1- and Anti-PL7/PL12 Positive Patients With Antisynthetase Syndrome. Autoimmun Rev (2012) 11(10):739–45. doi: 10.1016/j.autrev.2012.01.006
38. Aggarwal R, Cassidy E, Fertig N, Koontz DC, Lucas M, Ascherman DP, et al. Patients With Non-Jo-1 Anti-tRNA-Synthetase Autoantibodies Have Worse Survival Than Jo-1 Positive Patients. Ann Rheum Dis (2014) 73(1):227–32. doi: 10.1136/annrheumdis-2012-201800
39. Darrah E, Rosen A. Granzyme B Cleavage of Autoantigens in Autoimmunity. Cell Death Differ (2010) 17(4):624–32. doi: 10.1038/cdd.2009.197
40. Elferjani B, Liaqat A, Zaman M, Sexton M. Anti-Synthetase Syndrome-Related Interstitial Lung Disease With Anti-PL-12 Antibodies. Cureus (2021) 13(1):e12936. doi: 10.7759/cureus.12936
41. Marie I. Morbidity and Mortality in Adult Polymyositis and Dermatomyositis. Curr Rheumatol Rep (2012) 14(3):275–85. doi: 10.1007/s11926-012-0249-3
42. Wakasugi K, Schimmel P. Two Distinct Cytokines Released From a Human aminoacyl-tRNA Synthetase. Science (1999) 284(5411):147–51. doi: 10.1126/science.284.5411.147
43. Wakasugi K, Slike BM, Hood J, Otani A, Ewalt KL, Friedlander M, et al. A Human aminoacyl-tRNA Synthetase as a Regulator of Angiogenesis. Proc Natl Acad Sci USA (2002) 99(1):173–7. doi: 10.1073/pnas.012602099
44. Yang XL, Skene RJ, McRee DE, Schimmel P. Crystal Structure of a Human aminoacyl-tRNA Synthetase Cytokine. Proc Natl Acad Sci USA (2002) 99(24):15369–74. doi: 10.1073/pnas.242611799
45. Moll SA, Platenburg M, Platteel ACM, Vorselaars ADM, Janssen Bonas M, Roodenburg-Benschop C, et al. Prevalence of Novel Myositis Autoantibodies in a Large Cohort of Patients With Interstitial Lung Disease. J Clin Med (2020) 9(9):2944. doi: 10.3390/jcm9092944
46. Betteridge Z, Tansley S, Shaddick G, Chinoy H, Cooper RG, New RP, et al. Frequency, Mutual Exclusivity and Clinical Associations of Myositis Autoantibodies in a Combined European Cohort of Idiopathic Inflammatory Myopathy Patients. J Autoimmun (2019) 101:48–55. doi: 10.1016/j.jaut.2019.04.001
47. Betteridge Z, McHugh N. Myositis-Specific Autoantibodies: An Important Tool to Support Diagnosis of Myositis. J Intern Med (2016) 280(1):8–23. doi: 10.1111/joim.12451
48. Cavagna L, Trallero-Araguas E, Meloni F, Cavazzana I, Rojas-Serrano J, Feist E, et al. Influence of Antisynthetase Antibodies Specificities on Antisynthetase Syndrome Clinical Spectrum Time Course. J Clin Med (2019) 8(11). doi: 10.3390/jcm8112013
49. Opinc AH, Makowska JS. Antisynthetase Syndrome - Much More Than Just a Myopathy. Semin Arthritis Rheumatol (2021) 51(1):72–83. doi: 10.1016/j.semarthrit.2020.09.020
50. Graham J, Bauer Ventura I, Newton CA, Lee C, Boctor N, Pugashetti JV, et al. Myositis-Specific Antibodies Identify A Distinct Interstitial Pneumonia With Autoimmune Features Phenotype. Eur Respir J (2020) 56:20015. doi: 10.1183/13993003.01205-2020
51. Shi J, Li S, Yang H, Zhang Y, Peng Q, Lu X, et al. Clinical Profiles and Prognosis of Patients With Distinct Antisynthetase Autoantibodies. J Rheumatol (2017) 44(7):1051–7. doi: 10.3899/jrheum.161480
52. Pinal-Fernandez I, Casal-Dominguez M, Huapaya JA, Albayda J, Paik JJ, Johnson C, et al. A Longitudinal Cohort Study of the Anti-Synthetase Syndrome: Increased Severity of Interstitial Lung Disease in Black Patients and Patients With Anti-PL7 and Anti-PL12 Autoantibodies. Rheumatol (Oxf) (2017) 56(6):999–1007. doi: 10.1093/rheumatology/kex021
53. Ramos-Martinez E, Falfan-Valencia R, Perez-Rubio G, Mejia M, Buendia-Roldan I, Gonzalez-Perez MI, et al. Anti-Aminoacyl Transfer-RNA-Synthetases (Anti-tRNA) Autoantibodies Associated With Interstitial Lung Disease: Pulmonary Disease Progression has a Persistent Elevation of the Th17 Cytokine Profile. J Clin Med (2020) 9(5):1356. doi: 10.3390/jcm9051356
54. Tomonaga M, Sakamoto N, Ishimatsu Y, Kakugawa T, Harada T, Nakashima S, et al. Comparison of Pulmonary Involvement Between Patients Expressing Anti-PL-7 and Anti-Jo-1 Antibodies. Lung (2015) 193(1):79–83. doi: 10.1007/s00408-014-9665-7
55. Hervier B, Wallaert B, Hachulla E, Adoue D, Lauque D, Audrain M, et al. Clinical Manifestations of Anti-Synthetase Syndrome Positive for anti-alanyl-tRNA Synthetase (Anti-PL12) Antibodies: A Retrospective Study of 17 Cases. Rheumatology (2010) 49(5):972–6. doi: 10.1093/rheumatology/kep455
56. Rojas-Serrano J, Herrera-Bringas D, Mejia M, Rivero H, Mateos-Toledo H, Figueroa JE. Prognostic Factors in a Cohort of Antisynthetase Syndrome (ASS): Serologic Profile Is Associated With Mortality in Patients With Interstitial Lung Disease (ILD). Clin Rheumatol (2015) 34(9):1563–9. doi: 10.1007/s10067-015-3023-x
57. Vulsteke JB, Satoh M, Malyavantham K, Bossuyt X, De Langhe E, Mahler M. Anti-OJ Autoantibodies: Rare or Underdetected? Autoimmun Rev (2019) 18(7):658–64. doi: 10.1016/j.autrev.2019.05.002
58. Cerbelli B, Pisano A, Colafrancesco S, Pignataro MG, Biffoni M, Berni S, et al. Anti-aminoacyl-tRNA Synthetase-Related Myositis and Dermatomyositis: Clues for Differential Diagnosis on Muscle Biopsy. Virchows Arch (2018) 472(3):477–87. doi: 10.1007/s00428-017-2269-x
59. Mescam-Mancini L, Allenbach Y, Hervier B, Devilliers H, Mariampillay K, Dubourg O, et al. Anti-Jo-1 Antibody-Positive Patients Show a Characteristic Necrotizing Perifascicular Myositis. Brain (2015) 138(Pt 9):2485–92. doi: 10.1093/brain/awv192
60. Uruha A, Suzuki S, Suzuki N, Nishino I. Perifascicular Necrosis in Anti-Synthetase Syndrome Beyond Anti-Jo-1. Brain (2016) 139(Pt 9):e50. doi: 10.1093/brain/aww125
61. Mehndiratta P, Mehta S, Manjila SV, Kammer GM, Cohen ML, Preston DC. Isolated Necrotizing Myopathy Associated With ANTI-PL12 Antibody. Muscle Nerve (2012) 46(2):282–6. doi: 10.1002/mus.23383
62. Noguchi E, Uruha A, Suzuki S, Hamanaka K, Ohnuki Y, Tsugawa J, et al. Skeletal Muscle Involvement in Antisynthetase Syndrome. JAMA Neurol (2017) 74(8):992–9. doi: 10.1001/jamaneurol.2017.0934
63. Lilleker JB, Vencovsky J, Wang G, Wedderburn LR, Diederichsen LP, Schmidt J, et al. The EuroMyositis Registry: An International Collaborative Tool to Facilitate Myositis Research. Ann Rheum Dis (2018) 77(1):30–9. doi: 10.1136/annrheumdis-2017-211868
64. Gusdorf L, Morruzzi C, Goetz J, Lipsker D, Sibilia J, Cribier B. Mechanics Hands in Patients With Antisynthetase Syndrome: 25 Cases. Ann Dermatol Venereol (2019) 146(1):19–25. doi: 10.1016/j.annder.2018.11.010
65. Mahler M, Miller FW, Fritzler MJ. Idiopathic Inflammatory Myopathies and the Anti-Synthetase Syndrome: A Comprehensive Review. Autoimmun Rev (2014) 13(4-5):367–71. doi: 10.1016/j.autrev.2014.01.022
66. Lundberg IE, de Visser M, Werth VP. Classification of Myositis. Nat Rev Rheumatol (2018) 14(5):269–78. doi: 10.1038/nrrheum.2018.41
67. Cavagna L, Nuno L, Scire CA, Govoni M, Longo FJL, Franceschini F, et al. Clinical Spectrum Time Course in Anti Jo-1 Positive Antisynthetase Syndrome: Results From an International Retrospective Multicenter Study. Med (Baltimore) (2015) 94(32):e1144. doi: 10.1097/MD.0000000000001144
68. Andras C, Ponyi A, Constantin T, Csiki Z, Szekanecz E, Szodoray P, et al. Dermatomyositis and Polymyositis Associated With Malignancy: A 21-Year Retrospective Study. J Rheumatol (2008) 35(3):438–44.
69. Boleto G, Perotin JM, Eschard JP, Salmon JH. Squamous Cell Carcinoma of the Lung Associated With Anti-Jo1 Antisynthetase Syndrome: A Case Report and Review of the Literature. Rheumatol Int (2017) 37(7):1203–6. doi: 10.1007/s00296-017-3728-z
70. Chinoy H, Fertig N, Oddis CV, Ollier WE, Cooper RG. The Diagnostic Utility of Myositis Autoantibody Testing for Predicting the Risk of Cancer-Associated Myositis. Ann Rheum Dis (2007) 66(10):1345–9. doi: 10.1136/ard.2006.068502
71. Tiniakou E, Mammen AL. Idiopathic Inflammatory Myopathies and Malignancy: A Comprehensive Review. Clin Rev Allergy Immunol (2017) 52(1):20–33. doi: 10.1007/s12016-015-8511-x
72. Yang H, Peng Q, Yin L, Li S, Shi J, Zhang Y, et al. Identification of Multiple Cancer-Associated Myositis-Specific Autoantibodies in Idiopathic Inflammatory Myopathies: A Large Longitudinal Cohort Study. Arthritis Res Ther (2017) 19(1):259. doi: 10.1186/s13075-017-1469-8
73. Watkins RF-F J, Tahir H, Wykes F, Studdy PR, Beynon HLC. Jo-1 Syndrome With Associated Poorly Differentiated Adenocarcinoma. Rheumatology (2004) 43(3):389–90. doi: 10.1093/rheumatology/keg473
74. Legault D, McDermott J, Crous-Tsanaclis AM, Boire G. Cancer-Associated Myositis in the Presence of Anti-Jo1 Autoantibodies and the Antisynthetase Syndrome. J Rheumatol (2008) 35(1):169–71.
75. Lu X, Yang H, Shu X, Chen F, Zhang Y, Zhang S, et al. Factors Predicting Malignancy in Patients With Polymyositis and Dermatomyostis: A Systematic Review and Meta-Analysis. PloS One (2014) 9(4):e94128. doi: 10.1371/journal.pone.0094128
76. Ascherman DP, Oriss TB, Oddis CV, Wright TM. Critical Requirement for Professional APCs in Eliciting T Cell Responses to Novel Fragments of histidyl-tRNA Synthetase (Jo-1) in Jo-1 Antibody-Positive Polymyositis. J Immunol (2002) 169(12):7127–34. doi: 10.4049/jimmunol.169.12.7127
77. Howard OM, Dong HF, Yang D, Raben N, Nagaraju K, Rosen A, et al. Histidyl-tRNA Synthetase and asparaginyl-tRNA Synthetase, Autoantigens in Myositis, Activate Chemokine Receptors on T Lymphocytes and Immature Dendritic Cells. J Exp Med (2002) 196(6):781–91. doi: 10.1084/jem.20020186
78. Galindo-Feria AS, Albrecht I, Fernandes-Cerqueira C, Notarnicola A, James EA, Herrath J, et al. Proinflammatory Histidyl-Transfer RNA Synthetase-Specific CD4+ T Cells in the Blood and Lungs of Patients With Idiopathic Inflammatory Myopathies. Arthritis Rheumatol (2020) 72(1):179–91. doi: 10.1002/art.41075
79. Williams TF, Mirando AC, Wilkinson B, Francklyn CS, Lounsbury KM. Secreted Threonyl-tRNA Synthetase Stimulates Endothelial Cell Migration and Angiogenesis. Sci Rep (2013) 3:1317. doi: 10.1038/srep01317
80. Jung HJ, Park SH, Cho KM, Jung KI, Cho D, Kim TS. Threonyl-tRNA Synthetase Promotes T Helper Type 1 Cell Responses by Inducing Dendritic Cell Maturation and IL-12 Production via an NF-kappaB Pathway. Front Immunol (2020) 11:571959. doi: 10.3389/fimmu.2020.571959
81. Lo WS, Gardiner E, Xu Z, Lau CF, Wang F, Zhou JJ, et al. Human tRNA Synthetase Catalytic Nulls With Diverse Functions. Science (2014) 345(6194):328–32. doi: 10.1126/science.1252943
82. Zhang H, Yang XL, Sun L. The Uniqueness of AlaRS and its Human Disease Connections. RNA Biol (2021) 18(11):1501–11. doi: 10.1080/15476286.2020.1861803
83. Bunn CC, Mathews MB. Autoreactive Epitope Defined as the Anticodon Region of Alanine Transfer RNA. Science (1987) 238(4830):1116–9. doi: 10.1126/science.2446387
84. Shiba K, Ripmaster T, Suzuki N, Nichols R, Plotz P, Noda T, et al. Human alanyl-tRNA Synthetase: Conservation in Evolution of Catalytic Core and Microhelix Recognition. Biochemistry (1995) 34(33):10340–9. doi: 10.1021/bi00033a004
85. Park MC, Kang T, Jin D, Han JM, Kim SB, Park YJ, et al. Secreted Human glycyl-tRNA Synthetase Implicated in Defense Against ERK-Activated Tumorigenesis. Proc Natl Acad Sci USA (2012) 109(11):E640–7. doi: 10.1073/pnas.1200194109
86. Hirakata M, Suwa A, Takeda Y, Matsuoka Y, Irimajiri S, Targoff IN, et al. Autoantibodies to Glycyl-Transfer RNA Synthetase in Myositis. Association With Dermatomyositis and Immunologic Heterogeneity. Arthritis Rheumatol (1996) 39(1):146–51. doi: 10.1002/art.1780390119
87. Levine SM, Rosen A, Casciola-Rosen LA. Anti-Aminoacyl tRNA Synthetase Immune Responses: Insights Into the Pathogenesis of the Idiopathic Inflammatory Myopathies. Curr Opin Rheumatol (2003) 15(6):708–13. doi: 10.1097/00002281-200311000-00005
88. Ho MT, Lu J, Brunssen D, Suter B. A Translation-Independent Function of PheRS Activates Growth and Proliferation in Drosophila. Dis Model Mech (2021) 14(3). doi: 10.1242/dmm.048132
89. Wang S, Mao C, Zhao Z, Gu Q, Jin M, Xiao Y, et al. Increased TTS Abrogates IDO-Mediated CD4(+) T Cells Suppression in Patients With Graves’ Disease. Endocrine (2009) 36(1):119–25. doi: 10.1007/s12020-009-9184-0
90. Ahn YH, Park S, Choi JJ, Park BK, Rhee KH, Kang E, et al. Secreted tryptophanyl-tRNA Synthetase as a Primary Defence System Against Infection. Nat Microbiol (2016) 2:16191. doi: 10.1038/nmicrobiol.2016.191
91. Park BS, Song DH, Kim HM, Choi BS, Lee H, Lee JO. The Structural Basis of Lipopolysaccharide Recognition by the TLR4-MD-2 Complex. Nature (2009) 458(7242):1191–5. doi: 10.1038/nature07830
92. Jin M. Unique Roles of tryptophanyl-tRNA Synthetase in Immune Control and its Therapeutic Implications. Exp Mol Med (2019) 51(1):1–10. doi: 10.1038/s12276-018-0196-9
93. Ofir-Birin Y, Fang P, Bennett SP, Zhang HM, Wang J, Rachmin I, et al. Structural Switch of lysyl-tRNA Synthetase Between Translation and Transcription. Mol Cell (2013) 49(1):30–42. doi: 10.1016/j.molcel.2012.10.010
94. Kim SB, Kim HR, Park MC, Cho S, Goughnour PC, Han D, et al. Caspase-8 Controls the Secretion of Inflammatory lysyl-tRNA Synthetase in Exosomes From Cancer Cells. J Cell Biol (2017) 216(7):2201–16. doi: 10.1083/jcb.201605118
95. Mathews MB, Bernstein RM. Myositis Autoantibody Inhibits histidyl-tRNA Synthetase. Nature (1983), 177–9. doi: 10.1038/304177a0
96. Freist W, Verhey JF, Ruhlmann A, Gauss DH, Arnez JG. Histidyl-tRNA Synthetase. Biol Chem (1999) 380(6):623–46. doi: 10.1515/BC.1999.079
97. Adams RA, Fernandes-Cerqueira C, Notarnicola A, Mertsching E, Xu Z, Lo WS, et al. Serum-Circulating His-tRNA Synthetase Inhibits Organ-Targeted Immune Responses. Cell Mol Immunol (2021) 18(6):1463–75. doi: 10.1038/s41423-019-0331-0
98. Zhou JJ, Wang F, Xu Z, Lo WS, Lau CF, Chiang KP, et al. Secreted histidyl-tRNA Synthetase Splice Variants Elaborate Major Epitopes for Autoantibodies in Inflammatory Myositis. J Biol Chem (2014) 289(28):19269–75. doi: 10.1074/jbc.C114.571026
99. Notarnicola A, Preger C, Lundström SL, Renard N, Wigren E-, Gompel EV, et al. Longitudinal Assessment of Reactivity and Affinity Profile of Anti-Jo1 Autoantibodies to Distinct HisRS Domains and a Splice Variant in a Cohort of Patients With Myositis and Anti-Synthetase Syndrome. Arthritis Res Ther (2022) 24(1):62. doi: 10.1186/s13075-022-02745-6
100. Raben N, Nichols R, Dohlman J, McPhie P, Sridhar V, Hyde C, et al. A Motif in Human histidyl-tRNA Synthetase Which is Shared Among Several aminoacyl-tRNA Synthetases Is a Coiled-Coil That is Essential for Enzymatic Activity. Biol Chem (1994), 24277–83. doi: 10.1016/S0021-9258(19)51078-X
101. Brouwer R, Hengstman GJ, Vree Egberts W, Ehrfeld H, Bozic B, Ghirardello A, et al. Autoantibody Profiles in the Sera of European Patients With Myositis. Ann Rheum Dis (2001) 60(2):116–23. doi: 10.1136/ard.60.2.116
102. Targoff IN. Humoral Immunity in Polymyositis/Dermatomyositis. J Invest Dermatol (1993) 100(1):116S–23S. doi: 10.1111/1523-1747.ep12356607
103. Wasicek CA, Reichlin M, Montes M, Raghu G. Polymyositis and Interstitial Lung Disease in a Patient With Anti-Jo1 Prototype. Am J Med (1984) 76(3):538–44. doi: 10.1016/0002-9343(84)90677-6
104. Richards TJ, Eggebeen A, Gibson K, Yousem S, Fuhrman C, Gochuico BR, et al. Characterization and Peripheral Blood Biomarker Assessment of Anti-Jo-1 Antibody-Positive Interstitial Lung Disease. Arthritis Rheumatol (2009) 60(7):2183–92. doi: 10.1002/art.24631
105. Smirnova EV, Lakunina VA, Tarassov I, Krasheninnikov IA, Kamenski PA. Noncanonical Functions of aminoacyl-tRNA Synthetases. Biochem (Mosc) (2012) 77(1):15–25. doi: 10.1134/S0006297912010026
106. Dai C, Reyes-Ordonez A, You JS, Chen J. A Non-Translational Role of threonyl-tRNA Synthetase in Regulating JNK Signaling During Myogenic Differentiation. FASEB J (2021) 35(10):e21948. doi: 10.1096/fj.202101094R
107. Labirua-Iturburu A, Selva-O’Callaghan A, Vincze M, Danko K, Vencovsky J, Fisher B, et al. Anti-PL-7 (anti-threonyl-tRNA Synthetase) Antisynthetase Syndrome: Clinical Manifestations in a Series of Patients From a European Multicenter Study (EUMYONET) and Review of the Literature. Med (Baltimore) (2012) 91(4):206–11. doi: 10.1097/MD.0b013e318260977c
108. Zhan X, Yan W, Wang Y, Li Q, Shi X, Gao Y, et al. Clinical Features of Anti-Synthetase Syndrome Associated Interstitial Lung Disease: A Retrospective Cohort in China. BMC Pulm Med (2021) 21(1):57. doi: 10.1186/s12890-021-01399-5
109. Zuo Y, Ye L, Liu M, Li S, Liu W, Chen F, et al. Clinical Significance of Radiological Patterns of HRCT and Their Association With Macrophage Activation in Dermatomyositis. Rheumatol (Oxf) (2020) 59(10):2829–37. doi: 10.1093/rheumatology/keaa034
110. Fujisawa T, Hozumi H, Kono M, Enomoto N, Nakamura Y, Inui N, et al. Predictive Factors for Long-Term Outcome in Polymyositis/Dermatomyositis-Associated Interstitial Lung Diseases. Respir Investig (2017) 55(2):130–7. doi: 10.1016/j.resinv.2016.09.006
111. Garcia-Lozano JR, Gonzalez-Escribano MF, Rodriguez R, Rodriguez-Sanchez JL, Targoff IN, Wichmann I, et al. Detection of Anti-PL-12 Autoantibodies by ELISA Using a Recombinant Antigen; Study of the Immunoreactive Region. Clin Exp Immunol (1998) 114(2):161–5. doi: 10.1046/j.1365-2249.1998.00720.x
112. Kalluri M, Sahn SA, Oddis CV, Gharib SL, Christopher-Stine L, Danoff SK, et al. Clinical Profile of Anti-PL-12 Autoantibody. Cohort Study and Review of the Literature. Chest (2009) 135(6):1550–6. doi: 10.1378/chest.08-2233
113. Targoff IN, Arnett FC. Clinical Manifestations in Patients With Antibody to PL-12 Antigen (alanyl-tRNA Synthetase). Am J Med (1990) 88(3):241–51. doi: 10.1016/0002-9343(90)90149-8
114. Schneider F, Yousem SA, Oddis CV, Aggarwal R. Pulmonary Pathologic Manifestations of Anti-Alanyl-tRNA Synthetase (Anti-PL-12)-Related Inflammatory Myopathy. Arch Pathol Lab Med (2018) 142(2):191–7. doi: 10.5858/arpa.2017-0010-OA
115. Vinogradova ES, Nikonov OS, Nikonova EY. Associations Between Neurological Diseases and Mutations in the Human Glycyl-tRNA Synthetase. Biochem (Mosc) (2021) 86(Suppl 1):S12–23. doi: 10.1134/S0006297921140029
116. Nicoucar GR. Modifications of Blood Proteins in Otorhinolaryngologic Cancers. J Fr Otorhinolaryngol Chir Maxillofac (1966) 15(8):987–96.
117. Zhang Y, Ge Y, Yang H, Chen H, Tian X, Huang Z, et al. Clinical Features and Outcomes of the Patients With Anti-Glycyl tRNA Synthetase Syndrome. Clin Rheumatol (2020) 39(8):2417–24. doi: 10.1007/s10067-020-04979-8
118. Schneider F, Yousem SA, Bi D, Gibson KF, Oddis CV, Aggarwal R. Pulmonary Pathologic Manifestations of anti-glycyl-tRNA Synthetase (Anti-EJ)-Related Inflammatory Myopathy. J Clin Pathol (2014) 67(8):678–83. doi: 10.1136/jclinpath-2014-202367
119. Giannini M, Notarnicola A, Dastmalchi M, Lundberg IE, Lopalco G, Iannone F. Heterogeneous Clinical Spectrum of Interstitial Lung Disease in Patients With Anti-EJ Anti-Synthetase Syndrome: A Case Series. Clin Rheumatol (2016) 35(9):2363–7. doi: 10.1007/s10067-016-3258-1
120. Godar DE, Godar DE, Garcia V, Jacobo A, Aebi U, Yang DC. Structural Organization of the Multienzyme Complex of Mammalian aminoacyl-tRNA Synthetases. Biochemistry (1988) 27(18):6921–8. doi: 10.1021/bi00418a038
121. Cavazzana I, Fredi M, Ceribelli A, Mordenti C, Ferrari F, Carabellese N, et al. Testing for Myositis Specific Autoantibodies: Comparison Between Line Blot and Immunoprecipitation Assays in 57 Myositis Sera. J Immunol Methods (2016) 433:1–5. doi: 10.1016/j.jim.2016.02.017
122. Hamaguchi Y, Kuwana M, Takehara K. Comparison of Anti-OJ Antibody Detection Assays Between an Immunoprecipitation Assay and Line Blot Assay. Mod Rheumatol (2017) 27(3):551–2. doi: 10.1080/14397595.2016.1213947
123. Nakashima R, Imura Y, Hosono Y, Seto M, Murakami A, Watanabe K, et al. The Multicenter Study of a New Assay for Simultaneous Detection of Multiple anti-aminoacyl-tRNA Synthetases in Myositis and Interstitial Pneumonia. PloS One (2014) 9(1):e85062. doi: 10.1371/journal.pone.0085062
124. Castaneda S, Cavagna L, Gonzalez-Gay MA. New Criteria Needed for Antisynthetase Syndrome. JAMA Neurol (2018) 75(2):258–9. doi: 10.1001/jamaneurol.2017.3872
125. Yeom E, Kwon DW, Lee J, Kim SH, Lee JH, Min KJ, et al. Asparaginyl-tRNA Synthetase, A Novel Component of Hippo Signaling, Binds to Salvador and Enhances Yorkie-Mediated Tumorigenesis. Front Cell Dev Biol (2020) 8:32. doi: 10.3389/fcell.2020.00032
126. Park JS, Park MC, Lee KY, Goughnour PC, Jeong SJ, Kim HS, et al. Unique N-Terminal Extension Domain of Human asparaginyl-tRNA Synthetase Elicits CCR3-Mediated Chemokine Activity. Int J Biol Macromol (2018) 120(Pt A):835–45. doi: 10.1016/j.ijbiomac.2018.08.171
127. Ge Y, Li S, Li S, He L, Lu X, Wang G. Interstitial Lung Disease is a Major Characteristic of Anti-KS Associated Ant-Synthetase Syndrome. Ther Adv Chronic Dis (2020) 11:2040622320968417. doi: 10.1177/2040622320968417
128. Park SG, Schimmel P, Kim S. Aminoacyl tRNA Synthetases and Their Connections to Disease. Proc Natl Acad Sci USA (2008) 105(32):11043–9. doi: 10.1073/pnas.0802862105
129. Lieber M, Smith B, Szakal A, Nelson-Rees W, Todaro G. A Continuous Tumor-Cell Line From a Human Lung Carcinoma With Properties of Type II Alveolar Epithelial Cells. Int J Cancer (1976) 17(1):62–70. doi: 10.1002/ijc.2910170110
130. Rodova M, Ankilova V, Safro MG. Human phenylalanyl-tRNA Synthetase: Cloning, Characterization of the Deduced Amino Acid Sequences in Terms of the Structural Domains and Coordinately Regulated Expression of the Alpha and Beta Subunits in Chronic Myeloid Leukemia Cells. Biochem Biophys Res Commun (1999) 255(3):765–73. doi: 10.1006/bbrc.1999.0141
131. Thul PJ, Lindskog C. The Human Protein Atlas: A Spatial Map of the Human Proteome. Protein Sci (2018) 27(1):233–44. doi: 10.1002/pro.3307
132. Gao X, Guo R, Li Y, Kang G, Wu Y, Cheng J, et al. Contribution of Upregulated aminoacyl-tRNA Biosynthesis to Metabolic Dysregulation in Gastric Cancer. J Gastroenterol Hepatol (2021) 36(11):3113–26. doi: 10.1111/jgh.15592
133. Ruan W, Wang Y, Ma Y, Xing X, Lin J, Cui J, et al. HSP60, a Protein Downregulated by IGFBP7 in Colorectal Carcinoma. J Exp Clin Cancer Res (2010) 29:41. doi: 10.1186/1756-9966-29-41
134. Tansley SL, Betteridge Z, Lu H, Davies E, Rothwell S, New PP, et al. The Myositis Clinical Phenotype Associated With Anti-Zo Autoantibodies: A Case Series of Nine UK Patients. Rheumatol (Oxf) (2020) 59(7):1626–31. doi: 10.1093/rheumatology/kez504
135. La Corte R, Lo Mo Naco A, Locaputo A, Dolzani F, Trotta F. In Patients With Antisynthetase Syndrome the Occurrence of Anti-Ro/SSA Antibodies Causes a More Severe Interstitial Lung Disease. Autoimmunity (2006) 39(3):249–53. doi: 10.1080/08916930600623791
136. Vancsa A, Csipo I, Nemeth J, Devenyi K, Gergely L, Danko K. Characteristics of Interstitial Lung Disease in SS-A Positive/Jo-1 Positive Inflammatory Myopathy Patients. Rheumatol Int (2009) 29(9):989–94. doi: 10.1007/s00296-009-0884-9
137. Wakasugi K, Schimmel P. Highly Differentiated Motifs Responsible for Two Cytokine Activities of a Split Human tRNA Synthetase. J Biol Chem (1999) 274(33):23155–9. doi: 10.1074/jbc.274.33.23155
138. Wakasugi K, Slike BM, Hood J, Ewalt KL, Cheresh DA, Schimmel P. Induction of Angiogenesis by a Fragment of Human tyrosyl-tRNA Synthetase. J Biol Chem (2002) 277(23):20124–6. doi: 10.1074/jbc.C200126200
139. Won E, Morodomi Y, Kanaji S, Shapiro R, Vo MN, Orje JN, et al. Extracellular tyrosyl-tRNA Synthetase Cleaved by Plasma Proteinases and Stored in Platelet Alpha-Granules: Potential Role in Monocyte Activation. Res Pract Thromb Haemost (2020) 4(7):1167–77. doi: 10.1002/rth2.12429
140. Rabouille C. KRS: A Cut Away From Release in Exosomes. J Cell Biol (2017) 216(7):1891–3. doi: 10.1083/jcb.201706039
141. Motzik A, Nechushtan H, Foo SY, Razin E. Non-Canonical Roles of lysyl-tRNA Synthetase in Health and Disease. Trends Mol Med (2013) 19(12):726–31. doi: 10.1016/j.molmed.2013.07.011
142. Javanbakht H, Halwani R, Cen S, Saadatmand J, Musier-Forsyth K, Gottlinger H, et al. The Interaction Between HIV-1 Gag and Human lysyl-tRNA Synthetase During Viral Assembly. J Biol Chem (2003) 278(30):27644–51. doi: 10.1074/jbc.M301840200
143. Kovaleski BJ, Kennedy R, Khorchid A, Kleiman L, Matsuo H, Musier-Forsyth K. Critical Role of Helix 4 of HIV-1 Capsid C-Terminal Domain in Interactions With Human lysyl-tRNA Synthetase. J Biol Chem (2007) 282(44):32274–9. doi: 10.1074/jbc.M706256200
144. Khorchid A, Javanbakht H, Wise S, Halwani R, Parniak MA, Wainberg MA, et al. Sequences Within Pr160gag-Pol Affecting the Selective Packaging of Primer tRNA(Lys3) Into HIV-1. J Mol Biol (2000) 299(1):17–26. doi: 10.1006/jmbi.2000.3709
145. Kobbi L, Octobre G, Dias J, Comisso M, Mirande M. Association of Mitochondrial Lysyl-tRNA Synthetase With HIV-1 GagPol Involves Catalytic Domain of the Synthetase and Transframe and Integrase Domains of Pol. J Mol Biol (2011) 410(5):875–86. doi: 10.1016/j.jmb.2011.03.005
146. Saadatmand J, Guo F, Cen S, Niu M, Kleiman L. Interactions of Reverse Transcriptase Sequences in Pol With Gag and LysRS in the HIV-1 Trnalys3 Packaging/Annealing Complex. Virology (2008) 380(1):109–17. doi: 10.1016/j.virol.2008.07.015
147. Jones CP, Saadatmand J, Kleiman L, Musier-Forsyth K. Molecular Mimicry of Human Trnalys Anti-Codon Domain by HIV-1 RNA Genome Facilitates tRNA Primer Annealing. RNA (2013) 19(2):219–29. doi: 10.1261/rna.036681.112
148. Halwani R, Cen S, Javanbakht H, Saadatmand J, Kim S, Shiba K, et al. Cellular Distribution of Lysyl-tRNA Synthetase and Its Interaction With Gag During Human Immunodeficiency Virus Type 1 Assembly. J Virol (2004) 78(14):7553–64. doi: 10.1128/JVI.78.14.7553-7564.2004
149. Dewan V, Liu T, Chen KM, Qian Z, Xiao Y, Kleiman L, et al. Cyclic Peptide Inhibitors of HIV-1 Capsid-Human lysyl-tRNA Synthetase Interaction. ACS Chem Biol (2012) 7(4):761–9. doi: 10.1021/cb200450w
150. Kim DG, Choi JW, Lee JY, Kim H, Oh YS, Lee JW, et al. Interaction of Two Translational Components, lysyl-tRNA Synthetase and P40/37LRP, in Plasma Membrane Promotes Laminin-Dependent Cell Migration. FASEB J (2012) 26(10):4142–59. doi: 10.1096/fj.12-207639
151. Park SG, Kim HJ, Min YH, Choi EC, Shin YK, Park BJ, et al. Human lysyl-tRNA Synthetase Is Secreted to Trigger Proinflammatory Response. Proc Natl Acad Sci USA (2005) 102(18):6356–61. doi: 10.1073/pnas.0500226102
152. Kunst CB, Mezey E, Brownstein MJ, Patterson D. Mutations in SOD1 Associated With Amyotrophic Lateral Sclerosis Cause Novel Protein Interactions. Nat Genet (1997) 15(1):91–4. doi: 10.1038/ng0197-91
153. Kawamata H, Magrane J, Kunst C, King MP, Manfredi G. Lysyl-tRNA Synthetase is a Target for Mutant SOD1 Toxicity in Mitochondria. J Biol Chem (2008) 283(42):28321–8. doi: 10.1074/jbc.M805599200
154. McLaughlin HM, Sakaguchi R, Liu C, Igarashi T, Pehlivan D, Chu K, et al. Compound Heterozygosity for Loss-of-Function lysyl-tRNA Synthetase Mutations in a Patient With Peripheral Neuropathy. Am J Hum Genet (2010) 87(4):560–6. doi: 10.1016/j.ajhg.2010.09.008
155. Kaiser E, Hu B, Becher S, Eberhard D, Schray B, Baack M, et al. The Human EPRS Locus (Formerly the QARS Locus): A Gene Encoding a Class I and a Class II aminoacyl-tRNA Synthetase. Genomics (1994) 19(2):280–90. doi: 10.1006/geno.1994.1059
156. Fett R, Knippers R. The Primary Structure of Human glutaminyl-tRNA Synthetase. A Highly Conserved Core, Amino Acid Repeat Regions, and Homologies With Translation Elongation Factors. J Biol Chem (1991) 266(3):1448–55. doi: 10.1016/S0021-9258(18)52315-2
157. Cerini C, Kerjan P, Astier M, Gratecos D, Mirande M, Semeriva M. A Component of the Multisynthetase Complex is a Multifunctional aminoacyl-tRNA Synthetase. EMBO J (1991) 10(13):4267–77. doi: 10.1002/j.1460-2075.1991.tb05005.x
158. Ko YG, Kang YS, Park H, Seol W, Kim J, Kim T, et al. Apoptosis Signal-Regulating Kinase 1 Controls the Proapoptotic Function of Death-Associated Protein (Daxx) in the Cytoplasm. J Biol Chem (2001) 276(42):39103–6. doi: 10.1074/jbc.M105928200
159. Park BS, Jo HW, Jung J. Expression Profile of aminoacyl-tRNA Synthetases in Dorsal Root Ganglion Neurons After Peripheral Nerve Injury. J Mol Histol (2015) 46(1):115–22. doi: 10.1007/s10735-014-9601-4
160. Laast VA, Shim B, Johanek LM, Dorsey JL, Hauer PE, Tarwater PM, et al. Macrophage-Mediated Dorsal Root Ganglion Damage Precedes Altered Nerve Conduction in SIV-Infected Macaques. Am J Pathol (2011) 179(5):2337–45. doi: 10.1016/j.ajpath.2011.07.047
161. Ton BH, Chen Q, Gaina G, Tucureanu C, Georgescu A, Strungaru C, et al. Activation Profile of Dorsal Root Ganglia Iba-1 (+) Macrophages Varies With the Type of Lesion in Rats. Acta Histochem (2013) 115(8):840–50. doi: 10.1016/j.acthis.2013.04.007
162. Chan DL, Rudinger-Thirion J, Frugier M, Riley LG, Ho G, Kothur K, et al. A Case of QARS1 Associated Epileptic Encephalopathy and Review of Epilepsy in aminoacyl-tRNA Synthetase Disorders. Brain Dev (2022) 44(2):142–7. doi: 10.1016/j.braindev.2021.10.009
163. Kodera H, Osaka H, Iai M, Aida N, Yamashita A, Tsurusaki Y, et al. Mutations in the glutaminyl-tRNA Synthetase Gene Cause Early-Onset Epileptic Encephalopathy. J Hum Genet (2015) 60(2):97–101. doi: 10.1038/jhg.2014.103
164. Datta A, Ferguson A, Simonson C, Zannotto F, Michoulas A, Roland E, et al. Case Report. J Child Neurol (2017) 32(4):403–7. doi: 10.1177/0883073816685508
165. Fu CY, Wang PC, Tsai HJ. Competitive Binding Between Seryl-tRNA Synthetase/YY1 Complex and NFKB1 at the Distal Segment Results in Differential Regulation of Human Vegfa Promoter Activity During Angiogenesis. Nucleic Acids Res (2017) 45(5):2423–37. doi: 10.1093/nar/gkw1187
166. Gelpi C, Sontheimer EJ, Rodriguez-Sanchez JL. Autoantibodies Against a Serine tRNA-Protein Complex Implicated in Cotranslational Selenocysteine Insertion. Proc Natl Acad Sci USA (1992) 89(20):9739–43. doi: 10.1073/pnas.89.20.9739
167. Antonellis A, Green ED. The Role of aminoacyl-tRNA Synthetases in Genetic Diseases. Annu Rev Genomics Hum Genet (2008) 9:87–107. doi: 10.1146/annurev.genom.9.081307.164204
168. Lin J, Chiconelli Faria E, Da Rocha AJ, Rodrigues Masruha M, Pereira Vilanova LC, Scheper GC, et al. Leukoencephalopathy With Brainstem and Spinal Cord Involvement and Normal Lactate: A New Mutation in the DARS2 Gene. J Child Neurol (2010) 25(11):1425–8. doi: 10.1177/0883073810370897
169. Labauge P, Dorboz I, Eymard-Pierre E, Dereeper O, Boespflug-Tanguy O. Clinically Asymptomatic Adult Patient With Extensive LBSL MRI Pattern and DARS2 Mutations. J Neurol (2011) 258(2):335–7. doi: 10.1007/s00415-010-5755-5
170. Messmer M, Florentz C, Schwenzer H, Scheper GC, van der Knaap MS, Marechal-Drouard L, et al. A Human Pathology-Related Mutation Prevents Import of an aminoacyl-tRNA Synthetase Into Mitochondria. Biochem J (2011) 433(3):441–6. doi: 10.1042/BJ20101902
171. Yamashita S, Miyake N, Matsumoto N, Osaka H, Iai M, Aida N, et al. Neuropathology of Leukoencephalopathy With Brainstem and Spinal Cord Involvement and High Lactate Caused by a Homozygous Mutation of DARS2. Brain Dev (2013) 35(4):312–6. doi: 10.1016/j.braindev.2012.05.007
172. Taft RJ, Vanderver A, Leventer RJ, Damiani SA, Simons C, Grimmond SM, et al. Mutations in DARS Cause Hypomyelination With Brain Stem and Spinal Cord Involvement and Leg Spasticity. Am J Hum Genet (2013) 92(5):774–80. doi: 10.1016/j.ajhg.2013.04.006
173. Wolf NI, Toro C, Kister I, Latif KA, Leventer R, Pizzino A, et al. DARS-Associated Leukoencephalopathy Can Mimic a Steroid-Responsive Neuroinflammatory Disorder. Neurology (2015) 84(3):226–30. doi: 10.1212/WNL.0000000000001157
174. Zhang J, Liu M, Zhou L, Zhang ZB, Wang JM, Jiang YW, et al. DARS Mutations Responsible for Hypomyelination With Brain Stem and Spinal Cord Involvement and Leg Spasticity: Report of Two Cases and Review of Literature. Zhonghua Er Ke Za Zhi (2018) 56(3):211–5. doi: 10.3760/cma.j.issn.0578-1310.2018.03.011
175. Friederich MW, Timal S, Powell CA, Dallabona C, Kurolap A, Palacios-Zambrano S, et al. Pathogenic Variants in glutamyl-tRNA(Gln) Amidotransferase Subunits Cause a Lethal Mitochondrial Cardiomyopathy Disorder. Nat Commun (2018) 9(1):4065. doi: 10.1038/s41467-018-06250-w
176. Mendes MI, Gutierrez Salazar M, Guerrero K, Thiffault I, Salomons GS, Gauquelin L, et al. Bi-Allelic Mutations in EPRS, Encoding the Glutamyl-Prolyl-Aminoacyl-tRNA Synthetase, Cause a Hypomyelinating Leukodystrophy. Am J Hum Genet (2018) 102(4):676–84. doi: 10.1016/j.ajhg.2018.02.011
177. Datt M, Sharma A. Evolutionary and Structural Annotation of Disease-Associated Mutations in Human aminoacyl-tRNA Synthetases. BMC Genomics (2014) 15:1063. doi: 10.1186/1471-2164-15-1063
178. Casey JP, McGettigan P, Lynam-Lennon N, McDermott M, Regan R, Conroy J, et al. Identification of a Mutation in LARS as a Novel Cause of Infantile Hepatopathy. Mol Genet Metab (2012) 106(3):351–8. doi: 10.1016/j.ymgme.2012.04.017
179. Casey JP, Slattery S, Cotter M, Monavari AA, Knerr I, Hughes J, et al. Clinical and Genetic Characterisation of Infantile Liver Failure Syndrome Type 1, Due to Recessive Mutations in LARS. J Inherit Metab Dis (2015) 38(6):1085–92. doi: 10.1007/s10545-015-9849-1
180. Lin WX, Zheng QQ, Guo L, Cheng Y, Song YZ. Clinical Feature and Molecular Diagnostic Analysis of the First Non-Caucasian Child With Infantile Liver Failure Syndrome Type 1. Zhongguo Dang Dai Er Ke Za Zhi (2017) 19(8):913–20. doi: 10.7499/j.issn.1008-8830.2017.08.013
181. Pierce SB, Chisholm KM, Lynch ED, Lee MK, Walsh T, Opitz JM, et al. Mutations in Mitochondrial Histidyl tRNA Synthetase HARS2 Cause Ovarian Dysgenesis and Sensorineural Hearing Loss of Perrault Syndrome. Proc Natl Acad Sci USA (2011) 108(16):6543–8. doi: 10.1073/pnas.1103471108
182. van Meel E, Wegner DJ, Cliften P, Willing MC, White FV, Kornfeld S, et al. Rare Recessive Loss-of-Function methionyl-tRNA Synthetase Mutations Presenting as a Multi-Organ Phenotype. BMC Med Genet (2013) 14:106. doi: 10.1186/1471-2350-14-106
183. Hadchouel A, Wieland T, Griese M, Baruffini E, Lorenz-Depiereux B, Enaud L, et al. Biallelic Mutations of Methionyl-tRNA Synthetase Cause a Specific Type of Pulmonary Alveolar Proteinosis Prevalent on Reunion Island. Am J Hum Genet (2015) 96(5):826–31. doi: 10.1016/j.ajhg.2015.03.010
184. Edvardson S, Shaag A, Kolesnikova O, Gomori JM, Tarassov I, Einbinder T, et al. Deleterious Mutation in the Mitochondrial Arginyl-Transfer RNA Synthetase Gene is Associated With Pontocerebellar Hypoplasia. Am J Hum Genet (2007) 81(4):857–62. doi: 10.1086/521227
185. Cassandrini D, Cilio MR, Bianchi M, Doimo M, Balestri M, Tessa A, et al. Pontocerebellar Hypoplasia Type 6 Caused by Mutations in RARS2: Definition of the Clinical Spectrum and Molecular Findings in Five Patients. J Inherit Metab Dis (2013) 36(1):43–53. doi: 10.1007/s10545-012-9487-9
186. Belostotsky R, Ben-Shalom E, Rinat C, Becker-Cohen R, Feinstein S, Zeligson S, et al. Mutations in the Mitochondrial seryl-tRNA Synthetase Cause Hyperuricemia, Pulmonary Hypertension, Renal Failure in Infancy and Alkalosis, HUPRA Syndrome. Am J Hum Genet (2011) 88(2):193–200. doi: 10.1016/j.ajhg.2010.12.010
187. Musante L, Puttmann L, Kahrizi K, Garshasbi M, Hu H, Stehr H, et al. Mutations of the aminoacyl-tRNA-Synthetases SARS and WARS2 are Implicated in the Etiology of Autosomal Recessive Intellectual Disability. Hum Mutat (2017) 38(6):621–36. doi: 10.1002/humu.23205
188. Latour P, Thauvin-Robinet C, Baudelet-Mery C, Soichot P, Cusin V, Faivre L, et al. A Major Determinant for Binding and Aminoacylation of tRNA(Ala) in Cytoplasmic Alanyl-tRNA Synthetase is Mutated in Dominant Axonal Charcot-Marie-Tooth Disease. Am J Hum Genet (2010) 86(1):77–82. doi: 10.1016/j.ajhg.2009.12.005
189. McLaughlin HM, Sakaguchi R, Giblin W, Program NCS, Wilson TE, Biesecker L, et al. A Recurrent Loss-of-Function alanyl-tRNA Synthetase (AARS) Mutation in Patients With Charcot-Marie-Tooth Disease Type 2N (CMT2N). Hum Mutat (2012) 33(1):244–53. doi: 10.1002/humu.21635
190. Wolf NI, Salomons GS, Rodenburg RJ, Pouwels PJ, Schieving JH, Derks TG, et al. Mutations in RARS Cause Hypomyelination. Ann Neurol (2014) 76(1):134–9. doi: 10.1002/ana.24167
191. Mendes MI, Green LMC, Bertini E, Tonduti D, Aiello C, Smith D, et al. RARS1-Related Hypomyelinating Leukodystrophy: Expanding the Spectrum. Ann Clin Transl Neurol (2020) 7(1):83–93. doi: 10.1002/acn3.50960
192. Kuo ME, Theil AF, Kievit A, Malicdan MC, Introne WJ, Christian T, et al. Cysteinyl-tRNA Synthetase Mutations Cause a Multi-System, Recessive Disease That Includes Microcephaly, Developmental Delay, and Brittle Hair and Nails. Am J Hum Genet (2019) 104(3):520–9. doi: 10.1016/j.ajhg.2019.01.006
193. Stephen J, Nampoothiri S, Banerjee A, Tolman NJ, Penninger JM, Elling U, et al. Loss of Function Mutations in VARS Encoding Cytoplasmic valyl-tRNA Synthetase Cause Microcephaly, Seizures, and Progressive Cerebral Atrophy. Hum Genet (2018) 137(4):293–303. doi: 10.1007/s00439-018-1882-3
194. Okur V, Ganapathi M, Wilson A, Chung WK. Biallelic Variants in VARS in a Family With Two Siblings With Intellectual Disability and Microcephaly: Case Report and Review of the Literature. Cold Spring Harb Mol Case Stud (2018) 4(5). doi: 10.1101/mcs.a003301
195. Friedman J, Smith DE, Issa MY, Stanley V, Wang R, Mendes MI, et al. Biallelic Mutations in valyl-tRNA Synthetase Gene VARS are Associated With a Progressive Neurodevelopmental Epileptic Encephalopathy. Nat Commun (2019) 10(1):707. doi: 10.1038/s41467-018-07067-3
196. Siekierska A, Stamberger H, Deconinck T, Oprescu SN, Partoens M, Zhang Y, et al. Biallelic VARS Variants Cause Developmental Encephalopathy With Microcephaly That is Recapitulated in Vars Knockout Zebrafish. Nat Commun (2019) 10(1):708. doi: 10.1038/s41467-018-07953-w
197. Casciola-Rosen L, Andrade F, Ulanet D, Wong WB, Rosen A. Cleavage by Granzyme B is Strongly Predictive of Autoantigen Status: Implications for Initiation of Autoimmunity. J Exp Med (1999) 190(6):815–26. doi: 10.1084/jem.190.6.815
198. Backes C, Ludwig N, Leidinger P, Harz C, Hoffmann J, Keller A, et al. Immunogenicity of Autoantigens. BMC Genomics (2011) 12:340. doi: 10.1186/1471-2164-12-340
199. He W, Bai G, Zhou H, Wei N, White NM, Lauer J, et al. CMT2D Neuropathy is Linked to the Neomorphic Binding Activity of glycyl-tRNA Synthetase. Nature (2015) 526(7575):710–4. doi: 10.1038/nature15510
Keywords: Anti-synthetase syndrome (ASSD), aminoacyl-tRNA synthetase, interstitial lung disease, myositis, autoantibodies, autoantigens, autoimmunity
Citation: Galindo-Feria AS, Notarnicola A, Lundberg IE and Horuluoglu B (2022) Aminoacyl-tRNA Synthetases: On Anti-Synthetase Syndrome and Beyond. Front. Immunol. 13:866087. doi: 10.3389/fimmu.2022.866087
Received: 30 January 2022; Accepted: 28 March 2022;
Published: 13 May 2022.
Edited by:
Guochun Wang, China-Japan Friendship Hospital, ChinaReviewed by:
Shuang Ye, Shanghai Jiao Tong University, ChinaXiaoming Shu, China-Japan Friendship Hospital, China
Copyright © 2022 Galindo-Feria, Notarnicola, Lundberg and Horuluoglu. This is an open-access article distributed under the terms of the Creative Commons Attribution License (CC BY). The use, distribution or reproduction in other forums is permitted, provided the original author(s) and the copyright owner(s) are credited and that the original publication in this journal is cited, in accordance with accepted academic practice. No use, distribution or reproduction is permitted which does not comply with these terms.
*Correspondence: Begum Horuluoglu, begum.horuluoglu@ki.se