- 1Department of Medicine, Division of Pulmonary, Allergy, Critical Care and Sleep Medicine, Emory University, Atlanta, GA, United States
- 2Atlanta Veterans Affairs Health Care System, Decatur, GA, United States
Although the epidemiology of bacterial pneumonia and excessive alcohol use is well established, the mechanisms by which alcohol induces risk of pneumonia are less clear. Patterns of alcohol misuse, termed alcohol use disorders (AUD), affect about 15 million people in the United States. Compared to otherwise healthy individuals, AUD increase the risk of respiratory infections and acute respiratory distress syndrome (ARDS) by 2-4-fold. Levels and fragmentation of hyaluronic acid (HA), an extracellular glycosaminoglycan of variable molecular weight, are increased in chronic respiratory diseases, including ARDS. HA is largely involved in immune-assisted wound repair and cell migration. Levels of fragmented, low molecular weight HA are increased during inflammation and decrease concomitant with leukocyte levels following injury. In chronic respiratory diseases, levels of fragmented HA and leukocytes remain elevated, inflammation persists, and respiratory infections are not cleared efficiently, suggesting a possible pathological mechanism for prolonged bacterial pneumonia. However, the role of HA in alcohol-induced immune dysfunction is largely unknown. This mini literature review provides insights into understanding the role of HA signaling in host immune defense following excessive alcohol use. Potential therapeutic strategies to mitigate alcohol-induced immune suppression in bacterial pneumonia and HA dysregulation are also discussed.
Introduction
Excessive alcohol use associated with alcohol use disorders (AUD) (1) is linked to over 5 million annual deaths globally (2), in part due to an increased risk of respiratory infections (3) and acute respiratory distress syndrome (ARDS) (4). Pneumonia is a serious respiratory infection that is caused by at least one of several opportunistic bacteria, viruses, or fungi. Nearly 44,000 people die annually due to pneumonia in the United States, while another 1.5 million are hospitalized for pneumonia as a primary diagnosis (5). Ethanol (EtOH) impairs mucociliary clearance in the upper airway (6, 7) and diminishes innate immune defense in the lower airway by impairing the ability of alveolar macrophages (AM) to phagocytose pathogens (8–11), such as bacterial pneumonia (11, 12). Upon pneumonia-associated microbial evasion of host immune defense mechanisms in the upper airway, microbial culture in the lower airways causes pneumonia. This mini review focuses on molecular mechanisms, such as that of hyaluronic acid (HA), that may be implicated in increased susceptibility to bacterial pneumonia during acute and chronic EtOH use. Modulation of HA metabolism, signaling, and intracellular communication that impact cellular immune functions during bacterial pneumonia may pave the way for future investigations on how alterations in the extracellular matrix may be exacerbated by excessive alcohol use.
Extracellular Matrix in the Lung
The extracellular matrix is a dynamic environment, rich with proteins, carbohydrates, and other significant structural molecules. In diseased states, additional matrix deposition results in diminished intracellular communication and progression to fibrosis. AUD-associated risk of pneumonia and ARDS (3, 4) precedes pulmonary fibrosis and loss of function if unresolved (13).
Hyaluronic acid (HA), an extracellular matrix glycosaminoglycan, is essential for maintaining tissue structure, promoting cell survival, and regulating inflammation and leukocyte motility after pulmonary injury (14–19). Further, accumulation of HA fragments is associated with chronic pulmonary inflammation mediated by innate immune cells (20–27). Increased HA synthesis and fragmentation is commonly involved in pulmonary disease pathology including fibrotic diseases (27–30), excessive remodeling (14, 18, 31, 32), and inflammation (15, 24, 33–37). In non-pathologic conditions, HA is expressed at very low concentrations in bronchoalveolar lavage fluid (38, 39) but is increased during pulmonary inflammation and pneumonia infections from Klebsiella pneumoniae (40) and Escherichia coli (41, 42).
Bacterial pneumonia clearance depends on dynamic, but regulated, HA metabolism and HA binding protein signaling (36, 40–44). Regulation of HA size and signaling through cell surface immune receptors is necessary to mobilize leukocytes, including alveolar macrophages, for recognition and destruction of infectious pathogens in those with AUD. Remodeling after respiratory infections is crucial and involves a restoration of HA dynamics coinciding with decreases in bacterial colonization, inflammation, and leukocyte recruitment.
HA Signaling: Hyaladherins and HA-Protein Interactions
Hyaladherins are HA binding proteins that transmit changes in the extracellular matrix to cell signals for altered intra- or inter-immune cell function (14) through intermediate proteoglycans (45, 46) or by ionic HA binding to membrane proteins (47, 48). Although alcohol diminishes the ability of alveolar macrophages to recognize and clear pathogens, the role of HA on bacterial recognition during excessive alcohol use is largely unknown.
CD44 and CHI3L1
Cluster of differentiation 44 (CD44) is a hyaladherin that spans the cellular membrane, binds HA, and internalizes HA for lysosomal degradation by hyaluronidase enzymes (49, 50). CD44 is the primary cell surface receptor for HA binding in lymphocytes (51–53) and forms an anti-apoptotic coat of HA around alveolar macrophages (54). Therefore, CD44 is crucial for HA metabolism and signaling in leukocytes. Granulocyte-macrophage colony stimulating factor (GM-CSF) and peroxisome proliferator-activated receptor gamma (PPARγ) agonism induce expression of CD44 in monocytes that do not readily bind HA (54). However, chronic alcohol diminishes GM-CSF and PPARγ (11, 55) in primary alveolar macrophages, potentially decreasing their ability to form an anti-apoptotic HA coat for signaling with other hyaladherins.
Patients with eosinophilic pneumonia have high concentrations of CD44, HA, and interleukin-5 in their bronchoalveolar fluid. In contrast, CD44 deficient mice show decreased HA content after Streptococcus pneumoniae but increased HA in response to E. coli infection (41), suggesting that bacterial strains differentially influence host HA matrices. Yet, these studies do not address altered HA binding or signaling as mechanisms for worsened bacterial pneumonia. While altered CD44 expression following alcohol use may be one mechanism of bacterial pneumonia pathogenesis, altered HA molecular weight or indirect HA signaling may also impact inflammatory signaling and the innate immune response in leukocytes.
For indirect immune cell signaling, chitinase-3 like-protein-1 (CHI3L1) forms an intermediate bond between CD44 and HA (56). Through HA binding to CHI3L1 (57, 58), lysosomal degradation of HA by CD44 internalization is inhibited. Thus, CHI3L1 indirectly inhibits HA uptake and degradation through CD44 mediated internalization, suggesting CHI3L1 as an important regulator of HA metabolism. CHI3L1 is expressed in macrophages, neutrophils and endothelial cells and is necessary for antigen response, oxidant injury response, inflammation, and macrophage phenotype in the lung (59). Alcohol and high CHI3L1 levels have been linked to the progression of liver injury and fibrosis (60–62), but not yet in alcohol and bacterial pneumonia.
In bacterial pneumonia, CHI3L1 activity promotes innate immune defenses by sensing oxidant stress, cytokines, growth factors and miRNAs in the extracellular environment. Patients hospitalized with pneumonia have increased levels of CHI3L1 in serum (44, 63, 64). Additionally, S. pneumoniae induces CHI3L1 expression, but mice lacking CHI3L1 have reduced bacterial clearance and enhanced mortality following S. pneumoniae infection (43). These studies suggest CD44 and CHI3L1 as important regulators of innate immunity in the lung during bacterial pneumonia. Further, these studies provide CD44 and CHI3L1 as targetable mechanisms for treating bacterial pneumonia in those with AUD.
HA Heavy Chain Formation
Tumor necrosis factor-stimulated gene-6 (TSG-6) is secreted by immune cells (65) and catalyzes inter-α-trypsin-inhibitor (IαI)-heavy chain complex to HA through pentatraxin 3 (PTX3) (66). Together, these molecular components generate a heavy chain HA matrix involved in airway inflammation (67), hyperresponsiveness (68–71) and toll-like receptor 4 (TLR4)-mediated lung injury (35, 69), possibly through PTX3 stimulation by TLR signaling (72). IαI attenuates lung injury in a porcine model of lipopolysaccharide (LPS)-induced sepsis (73), and PTX3 deficiency worsens LPS-induced lung injury. TSG-6 expression in cultured U-937 monocytes is enhanced by Staphylococcus aureus and Chlamydia pneumoniae (74), suggesting enhanced expression in some strains of bacterial pneumonia. Further, PTX3 is involved in microbial recognition and innate immunity through recruitment of leukocytes and binding to K. pneumoniae, Pseudomonas aeruginosa, Salmonella enterica, S. aureus, Neisseria meningitidis, and S. pneumoniae (75–77). Altogether, there is sufficient evidence for the role of heavy chain HA matrices in bacterial pneumonia, but further studies are needed to elucidate if PTX3 involvement in heavy chain HA formation is due to production by host or pathogen.
Little is known about heavy chain HA formation during excessive alcohol use. If heavy chain HA formation is involved in lung injury amelioration during bacterial pneumonia, disruptions in this process may lead to further lung injury and possibly sepsis. The risk of developing sepsis from pneumonia increases from 35% to 60% in people with AUD (4). EtOH feeding to C57BL/6 mice significantly diminished survival rates and lung PTX3 expression in a model of sepsis, and delayed tumor necrosis factor α (TNFα) level increases in plasma (78). Similarly, in a binge drinking mouse model of gram-negative bacterial lung infection, plasma TNFα was suppressed even while bacterial colonization was increased (79). Overall, these studies suggest that sepsis after excessive alcohol use not due to lack of inflammatory TNFα signaling. Rather, alterations in PTX3 disrupt HA heavy matrix formation and may be a mechanism for deranged immune function in those with AUD.
Versican and TLRs
Lecticans are HA-binding proteoglycans, containing chondroitin sulfate side chains, that ionically bind to HA through clusters of positively charged amino acids forming the link domain (48, 53). Little is known about how lecticans are impacted in bacterial pneumonia; however, levels of hyaluronan and the lectican, versican, increase during lung injury (38, 80, 81), perhaps by HA synthase regulation (82, 83). Although rats exposed to fetal alcohol showed a decrease in synaptic versican (84), the role of versican in alcohol-induced lung derangements continue to be an active area of investigation.
TLRs bind to hyaladherins and are known mediators of the inflammatory response during bacterial pneumonia. Like HA, versican can act as a danger associated molecular pattern for TLR signaling in alveolar macrophages (85, 86). Versican is augmented in the lungs of adult mice exposed to P. aeruginosa and upon TLR agonism (87). Comparatively, conditional versican deficiency in myeloid cells reduced inflammatory cell recruitment to the lungs (88). LPS stimulation of the TLR4/Trif pathway increases HA and versican levels in bone marrow derived macrophages in vitro and in murine alveolar macrophages (42, 88), but there is a lack of similar studies with gram positive bacteria.
Defects in TLR signaling predispose an individual to immunodeficiency that can result in severe bacterial pneumonia (89). Further, the versican receptors TLR2 and TLR4 are affected by excessive alcohol use. TLR2 and TLR4 do not bind HA but have been hypothesized to interact with HA through clustering of other matrix or membrane proteins and proteoglycans, like versican. Individuals with alcohol use disorders showed significant increases in TLR2; those with AUD and cannabis use exhibited significant increases in TLR6 (90). No experimental groups had increased TLR4 expression in that study, but another study showed that alcohol exposure induced TLR4 endocytosis in alveolar macrophages, limiting TLR4 activity for the recognition of pathogens (11). These results suggest that TLR expression or signaling may compensate for impaired bacterial recognition in those who have an AUD and bacterial pneumonia. Other membrane hyaladherins can also bind HA simultaneously to influence leukocyte phenotype (91) and affect pro- or anti-inflammatory signaling depending on the binding protein. While it is not known if hyaluronan or any binding partners interact with the other TLRs, these studies identified multiple targets for therapeutic intervention.
RHAMM, HABP1 and HABP2
Receptor for HA mediated motility (RHAMM), and HA binding protein 1 and 2 (HABP1, HABP2) are expressed ubiquitously and have multiple binding partners, including HA (92, 93). RHAMM contains putative binding domains for HA (94), but RHAMM is mainly expressed intracellularly (93, 95–97) to participate in signaling excluding HA. However, it is possible that HA binds to hyaladherins within the cell membrane because several hyaladherins are expressed intracellularly. Upon HA interaction with RHAMM, cell migration is promoted, influencing tissue remodeling or immune cell trafficking (98). In mice, there is increased membrane expression of RHAMM following lung injury (99). Further, RHAMM can compensate for CD44 through increased HA binding without increased RHAMM expression, indicating convergence of HA signaling pathways (100).
RHAMM is implicated in acute lung injury (101), and alcohol use exacerbates acute lung injury (4, 8, 13, 102, 103). However, it is not yet known how alcohol consumption directly affects RHAMM in any organ system. Past work has shown that RHAMM and transforming growth factor beta (TGFβ) work collectively to promote cell motility (104). Alcohol use inhibits inflammatory cytokines while stimulating TGFβ, which acts as an inhibitory cytokine in human monocytes exposed to bacterial stimuli (105). In contrast, some studies show that alcohol induces lung injury through proinflammatory pathways and promote fibrosis by stimulating TGFβ1 activity (106, 107). In alveolar macrophages, alcohol-induced oxidative stress through TGFβ1 regulation of NADPH oxidases diminished alveolar macrophage function (108). Altogether, TGFβ1 is clearly involved in immune dysfunction following alcohol use, but more information is necessary to conclude that changes in TGFβ1 contribute to alterations in RHAMM signaling.
HABP1, also known as p32 or gClqR, can be found at the cell surface with higher affinity for HA corresponding to ionic strength and acidic environments (109), and HA binding to HABP1 can inhibit HA degradation by S. pneumoniae hyaluronidases (110). Bacteria express hyaluronidase proteins that degrade host HA matrices to allow for greater bacterial movement; thus, HABP1 activity is an endogenous antibacterial host defense. In humans, HABP1 assists in the regulation of HA metabolism in non-diseased states. While there is little known about HABP1 involvement in bacterial pneumonia, HABP1 activity is well described in cancer and mitochondrial biology. Alcohol exposure impairs alveolar macrophage ability to phagocytose pathogens (8–11) via increased cellular oxidative stress (111), mitochondrial redox imbalance (112, 113), and impaired mitochondrial bioenergetics (114). Mitochondrial HABP1 regulates oxidative phosphorylation (115, 116) by maintaining mitochondrial protein translation (117), and cleavage of HABP1 by caspase-1 shifts cancer cell phenotype toward glycolysis (118). In human lung cancers, HABP1 is highly expressed, leading to altered nuclear factor kappa B (NFκB) activity and cell proliferation (119), revealing a role for HABP1 in the lung microenvironment.
HABP2, also known as factor VII activating protease or plasma hyaluronan binding protein, is extracellular. High molecular weight HA inhibits HABP2’s activity to maintain barrier integrity while low molecular weight HA prevents a leaky barrier (120, 121). Normal barrier function prevents bacterial spread into the vasculature during bacterial pneumonia that would otherwise result in sepsis. Further, alcohol impairs pulmonary barrier function (122, 123). In the lung, HABP2 may be involved in LPS-induced lung injury (121) and ARDS (124) primarily through its role in modulating lung barrier integrity. In patients with ARDS, HABP2 levels and activity are increased in alveolar macrophage, epithelial, and endothelial cells (124), and chronic alcohol use elevates the risk for ARDS (4).
In vivo HABP2 silencing by small interfering RNA attenuated LPS-mediated lung injury and hyperpermeability, indicating a possible therapeutic strategy for bacterial pneumonia in those with AUD-induced barrier dysfunction. Additionally, HABP2 primarily binds to cell surface protease-activated receptors (PAR) (125), and silencing of PAR1 and PAR3 can attenuate LPS-mediated barrier dysfunction (121). Mice with PAR2 genetic deletions exhibited severe lung inflammation, neutrophil accumulation, and diminished macrophage and neutrophil bacterial phagocytosis in a model of P. aeruginosa. These alterations were attenuated by PAR2 activation (126), indicating a possible role for HABP2 in bacterial pneumonia clearance. Other studies show similar roles for PARs in bacterial pneumonia pathology (126–128); however, this mechanism needs to be further elucidated since HABP1 and the PARs each have multiple binding partners.
Discussion
This mini review addresses modulation of HA signaling by alcohol and bacterial pneumonia. CD44 and RHAMM are involved in HA metabolism, signaling, and intracellular communication. CHI3L1, IαI, TSG-6, PTX3, and versican all act as intermediates between HA and membrane signaling proteins, like CD44 and TLRs. Herein we also review how HA modulates cellular energy metabolism through HABP2 and intracellular signaling. Another hyaladherin, lymphatic vessel endothelial cell receptor 1 (LYVE-1), binds HA for immune cell motility and HA metabolism but was not discussed in detail due to its low expression in the lungs. Nevertheless, CD44 and LYVE-1 jointly assist in immune cell migration within the lymphatic system (129–131) to traffic cells to the lungs during bacterial pneumonia. HA-hyaladherin interactions additionally assist with leukocyte motility. In summary, changes in the extracellular matrix impact cellular signaling in bacterial pneumonia that can be exacerbated by excessive alcohol use but there is much to learn still. Nevertheless, targeting hyaladherins may be a potential therapeutic strategy for mitigating lung injury in those with alcohol use disorders. These pathways have been summarized in Figure 1.
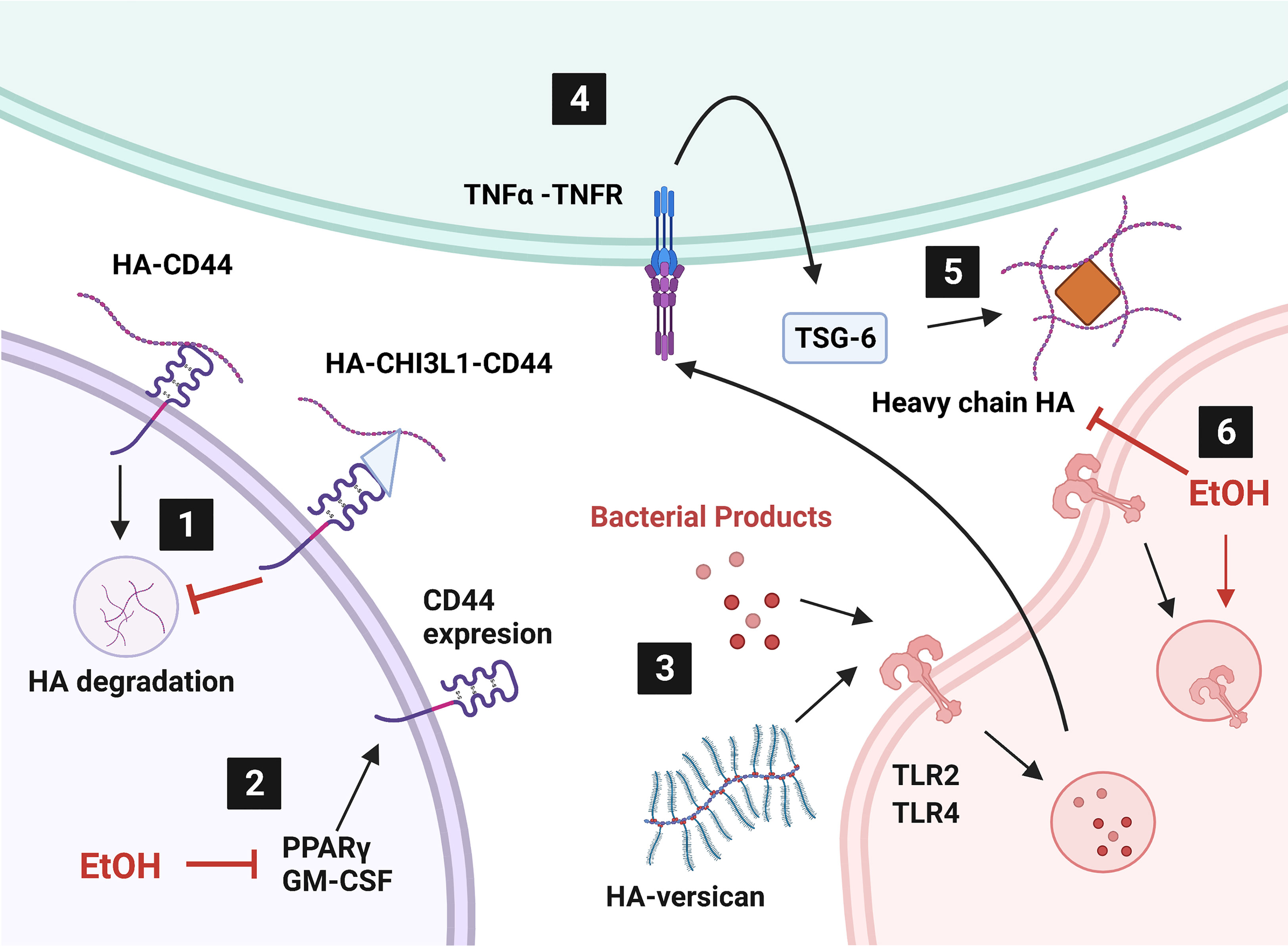
Figure 1 Alcohol affects hyaladherin signaling in the lung. 1) Internalization and degradation of hyaluronic acid (HA) is inhibited by overproduction of chitinase-3 like-protein-1 (CHI3L1). 2) Ethanol (EtOH) diminishes peroxisome proliferator activated receptor gamma (PPARγ) and granulocyte-macrophage colony stimulating factor (GM-CSF) levels. 3) HA-versican competes with bacterial products for toll-like receptor (TLR) signaling. 4) TLR signaling induces tumor necrosis factor alpha (TNFα) production. TNFα stimulates TNFα-stimulated gene-6 (TSG-6) expression. 5) TSG-6 catalyzes heavy chain HA matrix formation through pentatraxin 3 (PTX3, orange diamond). 6) EtOH induces TLR4 internalization and heavy chain formation by decreasing TNFα. Created with BioRender.com.
Controversies in the HA Field
Is increased HA production during lung disease pathological and does it need to be “fixed?” HA concentration increases, but average molecular weight decreases, in multiple pulmonary diseases involving immune dysfunction and inflammation. However, the mechanisms of HA signaling based on variations in molecular weight remain controversial in the field. Increased HA production appears to decrease leukocyte mobility and bacterial spread in pneumonia due to higher viscosity. However, increased HA production may aid in leukocyte motility through endogenous hyaladherins while preventing bacterial spread because of their lack of the same receptors.
Further, fragmented HA is thought to be pro-inflammatory while endogenous high molecular weight HA is anti-inflammatory (25, 34, 132). It is also clear that bacteria contain hyaluronidases to degrade host HA matrices, and fragmented HA can act as a danger associated molecular pattern for immune cell release of key immune factors. Our group has hypothesized that alcohol increases high molecular weight HA synthesis, thereby decreasing necessary pro-inflammatory signaling from fragmented HA. However, size classifications remain controversial in the field since “fragmented HA” or “low molecular weight HA” could range from HA chains of a few polysaccharides to 500 kD. Future studies should be done to clarify the immune response of leukocytes to different sized HA polymers to confirm past results.
Therapeutic Potential
Although the risk AUD individuals for getting sepsis and ARDS from pneumonia is approximately double that of non-AUD individuals (4), treatment strategies are comparable between AUD and non-AUD individuals. There are several FDA approved modulators of HA or HA binding proteins that are available by prescription or as a clinical treatment; however, additional studies on HA modulation in bacterial pneumonia and alcohol are needed before therapeutic targeting of these pathways in people with AUD can take place. Targeting bacterial protein influence in host HA matrices and barrier dysfunction go hand-in-hand. As bacteria spread and host lung cell apoptosis persists, cellular barriers are broken down. Use of current small molecule inhibitors of bacterial hyaluronidases are insufficient as a therapeutic strategy because they have low specificity and potency. Bacteria contain some hyaluronidases that are different than those in humans. Therefore, upregulation of host defenses against bacterial hyaluronidases, like HABP1, may work as an alternative treatment to prevent uncontrolled bacterial proliferation.
Proposed mechanisms of EtOH-induced oxidative stress in alveolar macrophage include loss of PPARγ activity (8, 11, 111), which is diminished following alcohol exposure (11, 55, 111). Rosiglitazone and pioglitazone, PPARγ agonists, improve EtOH-induced alveolar macrophage oxidative stress (9), mitochondrial-derived ROS (114), and dysfunctional phagocytosis and clearance of K. pneumoniae (11). Further, pioglitazone, reversed alcohol-induced derangements phagocytosis in alveolar macrophages (11, 55, 111). Because mitochondrial derived ATP is necessary for high energy processes, like phagocytosis, impaired mitochondrial function is one explanation for why alcohol impairs alveolar macrophage phagocytic ability. Identifying alcohol-induced mechanisms that impair HA signaling could further elucidate underlying mitochondrial dysfunction in alveolar macrophages.
In conclusion, AUDs increase the risk of respiratory infections and levels of the extracellular matrix component, HA, are increased in chronic respiratory diseases. HA signaling through hyaladherins are affected by alcohol use, which could modify inflammation and immune cell activity during bacterial pneumonia. The role of hyaladherins in alcohol-induced immune dysfunction is still largely unknown. This mini review highlights the necessity for future studies to provide insight into understanding the role of HA and its binding partners in host immune defense following excessive alcohol use.
Author Contributions
KMC outlined and prepared the manuscript; SMY outlined and prepared the manuscript. All authors contributed to the article and approved the submitted version.
Funding
This work was supported in part by grants from: the National Institute on Alcohol Abuse and Alcoholism (F31AA029938) to KMC (ORCID ID: 0000-0002-9461-4032) and (R01AA026086) to SMY (ORCID ID: 0000-0001-9309-0233) as well as the National Institute of General Medical Sciences (T32GM008602) to Randy A. Hall. The contents of this report do not represent the views of the Department of Veterans Affairs or the US Government.
Conflict of Interest
The authors declare that the research was conducted in the absence of any commercial or financial relationships that could be construed as a potential conflict of interest.
Publisher’s Note
All claims expressed in this article are solely those of the authors and do not necessarily represent those of their affiliated organizations, or those of the publisher, the editors and the reviewers. Any product that may be evaluated in this article, or claim that may be made by its manufacturer, is not guaranteed or endorsed by the publisher.
Acknowledgments
The authors would like to acknowledge the Emory University Molecular and Systems Pharmacology Program and the Atlanta VA Health Care System for their continued support.
Abbreviations
AUD, alcohol use disorder; ARDS, acute respiratory distress syndrome; HA, hyaluronic acid; EtOH, ethanol; CD44, Cluster of differentiation 44; GM-CSF, Granulocyte-macrophage colony stimulating factor; PPARγ, peroxisome proliferator activating receptor gamma; CHI3L1, chitinase-3 like-protein-1; TSG-6, Tumor necrosis factor-stimulated gene-6; PTX3, pentatraxin 3; TLR, Toll-like receptor; IαI, inter-α-trypsin-inhibitor; LPS, lipopolysaccharide; TNFα, tumor necrosis factor α; RHAMM, Receptor for HA mediated motility; HABP, hyaluronic acid binding protein; TGFβ, transforming growth factor beta; NFκB, nuclear factor kappa B; PAR, protease-activated receptors; LYVE-1, lymphatic vessel endothelial cell receptor 1.
References
1. Association, A. P. Diagnostic and Statistical Manual of Mental Disorders. 5th ed. Arlington, VA: American Psychiatric Association (2013).
2. Organization, W. H. Global Status Report on Alcohol and Health. Geneva: World Health Organization (2018).
3. Baker RC, Jerrells TR. Recent Developments in Alcoholism: Immunological Aspects. Recent Dev Alcohol (1993) 11:249–71. doi: 10.1007/978-1-4899-1742-3_15
4. Moss M, Parsons PE, Steinberg KP, Hudson LD, Guidot DM, Burnham EL, et al. Chronic Alcohol Abuse is Associated With an Increased Incidence of Acute Respiratory Distress Syndrome and Severity of Multiple Organ Dysfunction in Patients With Septic Shock. Crit Care Med (2003) 31:869–77. doi: 10.1097/01.CCM.0000055389.64497.11
5. Centers for Disease Control and Prevention, Released in 2020. Data are From the Multiple Cause of Death Files, 1999-2019, as Compiled From Data Provided by the 57 Vital Statistics Jurisdictions Through the Vital Statistics Cooperative Program (2021). Available at: http://wonder.cdc.gov/ucd-icd10.html.
6. Price ME, Gerald CL, Pavlik JA, Schlichte SL, Zimmerman MC, DeVasure JM, et al. Loss of cAMP-Dependent Stimulation of Isolated Cilia Motility by Alcohol Exposure is Oxidant-Dependent. Alcohol (2019) 80:91–8. doi: 10.1016/j.alcohol.2018.09.010
7. Wyatt TA, Gentry-Nielsen MJ, Pavlik JA, Sisson JH. Desensitization of PKA-Stimulated Ciliary Beat Frequency in an Ethanol-Fed Rat Model of Cigarette Smoke Exposure. Alcoholism Clin Exp Res (2004) 28:998–1004. doi: 10.1097/01.ALC.0000130805.75641.F4
8. Yeligar SM, Chen MM, Kovacs EJ, Sisson JH, Burnham EL, Brown LA. Alcohol and Lung Injury and Immunity. Alcohol (2016) 55:51–9. doi: 10.1016/j.alcohol.2016.08.005
9. Baughman RP, Roselle GA. Surfactant Deficiency With Decreased Opsonic Activity in a Guinea Pig Model of Alcoholism. Alcohol Clin Exp Res (1987) 11:261–4. doi: 10.1111/j.1530-0277.1987.tb01303.x
10. Greenberg SS, Zhao X, Hua L, Wang JF, Nelson S, Ouyang J. Ethanol Inhibits Lung Clearance of Pseudomonas Aeruginosa by a Neutrophil and Nitric Oxide-Dependent Mechanism, In Vivo. Alcohol Clin Exp Res (1999) 23:735–44. doi: 10.1111/j.1530-0277.1999.tb04177.x
11. Yeligar SM, Mehta AJ, Harris FL, Brown LA, Hart CM. Peroxisome Proliferator-Activated Receptor Gamma Regulates Chronic Alcohol-Induced Alveolar Macrophage Dysfunction. Am J Respir Cell Mol Biol (2016) 55:35–46. doi: 10.1165/rcmb.2015-0077OC
12. Yeligar SM, Harris FL, Hart CM, Brown LA. Glutathione Attenuates Ethanol-Induced Alveolar Macrophage Oxidative Stress and Dysfunction by Downregulating NADPH Oxidases. Am J Physiol Lung Cell Mol Physiol (2014) 306:L429–441. doi: 10.1152/ajplung.00159.2013
13. Sueblinvong V, Kerchberger VE, Saghafi R, Mills ST, Fan X, Guidot DM. Chronic Alcohol Ingestion Primes the Lung for Bleomycin-Induced Fibrosis in Mice. Alcohol Clin Exp Res (2014) 38:336–43. doi: 10.1111/acer.12232
14. Johnson P, Arif AA, Lee-Sayer SSM, Dong Y. Hyaluronan and Its Interactions With Immune Cells in the Healthy and Inflamed Lung. Front Immunol (2018) 9:2787. doi: 10.3389/fimmu.2018.02787
15. Noble PW, Jiang D. Matrix Regulation of Lung Injury, Inflammation, and Repair: The Role of Innate Immunity. Proc Am Thorac Soc (2006) 3:401–4. doi: 10.1513/pats.200604-097AW
16. Jiang D, Liang J, Fan J, Yu S, Luo Y, Chen S, et al. Regulation of Lung Injury and Repair by Toll-Like Receptors and Hyaluronan. Nat Med (2005) 11:1173–9. doi: 10.1038/nm1315
17. Jiang D, Liang J, Noble PW. Hyaluronan as an Immune Regulator in Human Diseases. Physiol Rev (2011) 91:221–64. doi: 10.1152/physrev.00052.2009
18. Jiang D, Liang J, Noble PW. Hyaluronan in Tissue Injury and Repair. Annu Rev Cell Dev Biol (2007) 23:435–61. doi: 10.1146/annurev.cellbio.23.090506.123337
19. Teder P, Vandivier RW, Jiang D, Liang J, Cohn L, Pure E, et al. Resolution of Lung Inflammation by CD44. Science (2002) 296:155–8. doi: 10.1126/science.1069659
20. Cantin AM, Larivee P, Begin RO. Extracellular Glutathione Suppresses Human Lung Fibroblast Proliferation. Am J Respir Cell Mol Biol (1990) 3:79–85. doi: 10.1165/ajrcmb/3.1.79
21. Cantor J, Ma S, Turino G. A Pilot Clinical Trial to Determine the Safety and Efficacy of Aerosolized Hyaluronan as a Treatment for COPD. Int J Chron Obstruct Pulmon Dis (2017) 12:2747–52. doi: 10.2147/COPD.S142156
22. Gebe JA, Yadava K, Ruppert SM, Marshall P, Hill P, Falk BA, et al. Modified High-Molecular-Weight Hyaluronan Promotes Allergen-Specific Immune Tolerance. Am J Respir Cell Mol Biol (2017) 56:109–20. doi: 10.1165/rcmb.2016-0111OC
23. Haserodt S, Aytekin M, Dweik RA. A Comparison of the Sensitivity, Specificity, and Molecular Weight Accuracy of Three Different Commercially Available Hyaluronan ELISA-Like Assays. Glycobiology (2011) 21:175–83. doi: 10.1093/glycob/cwq145
24. Papakonstantinou E, Roth M, Klagas I, Karakiulakis G, Tamm M, Stolz D. COPD Exacerbations Are Associated With Proinflammatory Degradation of Hyaluronic Acid. Chest (2015) 148:1497–507. doi: 10.1378/chest.15-0153
25. Sokolowska M, Chen LY, Eberlein M, Martinez-Anton A, Liu Y, Alsaaty S, et al. Low Molecular Weight Hyaluronan Activates Cytosolic Phospholipase A2alpha and Eicosanoid Production in Monocytes and Macrophages. J Biol Chem (2014) 289:4470–88. doi: 10.1074/jbc.M113.515106
26. Stern R, Asari AA, Sugahara KN. Hyaluronan Fragments: An Information-Rich System. Eur J Cell Biol (2006) 85:699–715. doi: 10.1016/j.ejcb.2006.05.009
27. Vistejnova L, Safrankova B, Nesporova K, Slavkovsky R, Hermannova M, Hosek P, et al. Low Molecular Weight Hyaluronan Mediated CD44 Dependent Induction of IL-6 and Chemokines in Human Dermal Fibroblasts Potentiates Innate Immune Response. Cytokine (2014) 70:97–103. doi: 10.1016/j.cyto.2014.07.006
28. Buonpensiero P, De Gregorio F, Sepe A, Di Pasqua A, Ferri P, Siano M, et al. Hyaluronic Acid Improves "Pleasantness" and Tolerability of Nebulized Hypertonic Saline in a Cohort of Patients With Cystic Fibrosis. Adv Ther (2010) 27:870–8. doi: 10.1007/s12325-010-0076-8
29. Liang J, Zhang Y, Xie T, Liu N, Chen H, Geng Y, et al. Hyaluronan and TLR4 Promote Surfactant-Protein-C-Positive Alveolar Progenitor Cell Renewal and Prevent Severe Pulmonary Fibrosis in Mice. Nat Med (2016) 22:1285–93. doi: 10.1038/nm.4192
30. Savani RC, Hou G, Liu P, Wang C, Simons E, Grimm PC, et al. A Role for Hyaluronan in Macrophage Accumulation and Collagen Deposition After Bleomycin-Induced Lung Injury. Am J Respir Cell Mol Biol (2000) 23:475–84. doi: 10.1165/ajrcmb.23.4.3944
31. Tseng V, Ni K, Allawzi A, Prohaska C, Hernandez-Lagunas L, Elajaili H, et al. Extracellular Superoxide Dismutase Regulates Early Vascular Hyaluronan Remodeling in Hypoxic Pulmonary Hypertension. Sci Rep (2020) 10:280. doi: 10.1038/s41598-019-57147-7
32. Vaday GG, Franitza S, Schor H, Hecht I, Brill A, Cahalon L, et al. Combinatorial Signals by Inflammatory Cytokines and Chemokines Mediate Leukocyte Interactions With Extracellular Matrix. J Leukoc Biol (2001) 69:885–92. doi: 10.1189/jlb.69.6.885
33. Bollyky PL, Lord JD, Masewicz SA, Evanko SP, Buckner JH, Wight TN, et al. Cutting Edge: High Molecular Weight Hyaluronan Promotes the Suppressive Effects of CD4+CD25+ Regulatory T Cells. J Immunol (2007) 179:744–7. doi: 10.4049/jimmunol.179.2.744
34. Johnson CG, Stober VP, Cyphert-Daly JM, Trempus CS, Flake GP, Cali V, et al. High Molecular Weight Hyaluronan Ameliorates Allergic Inflammation and Airway Hyperresponsiveness in the Mouse. Am J Physiol Lung Cell Mol Physiol (2018) 315:L787–98. doi: 10.1152/ajplung.00009.2018
35. Li Z, Potts-Kant EN, Garantziotis S, Foster WM, Hollingsworth JW. Hyaluronan Signaling During Ozone-Induced Lung Injury Requires TLR4, MyD88, and TIRAP. PloS One (2011) 6:e27137. doi: 10.1371/journal.pone.0027137
36. McKallip RJ, Ban H, Uchakina ON. Treatment With the Hyaluronic Acid Synthesis Inhibitor 4-Methylumbelliferone Suppresses LPS-Induced Lung Inflammation. Inflammation (2015) 38:1250–9. doi: 10.1007/s10753-014-0092-y
37. Wight TN, Frevert CW, Debley JS, Reeves SR, Parks WC, Ziegler SF. Interplay of Extracellular Matrix and Leukocytes in Lung Inflammation. Cell Immunol (2017) 312:1–14. doi: 10.1016/j.cellimm.2016.12.003
38. Nettelbladt O, Hallgren R. Hyaluronan (Hyaluronic Acid) in Bronchoalveolar Lavage Fluid During the Development of Bleomycin-Induced Alveolitis in the Rat. Am Rev Respir Dis (1989) 140:1028–32. doi: 10.1164/ajrccm/140.4.1028
39. Underhill CB, Nguyen HA, Shizari M, Culty M. CD44 Positive Macrophages Take Up Hyaluronan During Lung Development. Dev Biol (1993) 155:324–36. doi: 10.1006/dbio.1993.1032
40. Wang Q, Teder P, Judd NP, Noble PW, Doerschuk CM. CD44 Deficiency Is Associated With Increased Bacterial Clearance But Enhanced Lung Inflammation During Gram-Negative Pneumonia. Am J Pathol (2010) 177:2483–94. doi: 10.2353/ajpath.2010.100562
41. Wang Q, Teder P, Judd NP, Noble PW, Doerschuk CM. CD44 Deficiency Leads to Enhanced Neutrophil Migration and Lung Injury in Escherichia Coli Pneumonia in Mice. Am J Pathol (2002) 161:2219–28. doi: 10.1016/S0002-9440(10)64498-7
42. Chang MY, Tanino Y, Vidova V, Kinsella MG, Chan CK, Johnson PY, et al. A Rapid Increase in Macrophage-Derived Versican and Hyaluronan in Infectious Lung Disease. Matrix Biol (2014) 34:1–12. doi: 10.1016/j.matbio.2014.01.011
43. Dela Cruz CS, Liu W, He CH, Jacoby A, Gornitzky A, Ma B, et al. Chitinase 3-Like-1 Promotes Streptococcus Pneumoniae Killing and Augments Host Tolerance to Lung Antibacterial Responses. Cell Host Microbe (2012) 12:34–46. doi: 10.1016/j.chom.2012.05.017
44. Nordenbaek C, Johansen JS, Junker P, Borregaard N, Sorensen O, Price PA. YKL-40, a Matrix Protein of Specific Granules in Neutrophils, is Elevated in Serum of Patients With Community-Acquired Pneumonia Requiring Hospitalization. J Infect Dis (1999) 180:1722–6. doi: 10.1086/315050
45. Neame PJ, Christner JE, Baker JR. Cartilage Proteoglycan Aggregates. The Link Protein and Proteoglycan Amino-Terminal Globular Domains Have Similar Structures. J Biol Chem (1987) 262:17768–78. doi: 10.1016/S0021-9258(18)45445-2
46. Zimmermann DR, Ruoslahti E. Multiple Domains of the Large Fibroblast Proteoglycan, Versican. EMBO J (1989) 8:2975–81. doi: 10.1002/j.1460-2075.1989.tb08447.x
47. Goldstein LA, Zhou DF, Picker LJ, Minty CN, Bargatze RF, Ding JF, et al. A Human Lymphocyte Homing Receptor, the Hermes Antigen, is Related to Cartilage Proteoglycan Core and Link Proteins. Cell (1989) 56:1063–72. doi: 10.1016/0092-8674(89)90639-9
48. Kohda D, Morton CJ, Parkar AA, Hatanaka H, Inagaki FM, Campbell ID, et al. Solution Structure of the Link Module: A Hyaluronan-Binding Domain Involved in Extracellular Matrix Stability and Cell Migration. Cell (1996) 86:767–75. doi: 10.1016/s0092-8674(00)80151-8
49. Culty M, Nguyen HA, Underhill CB. The Hyaluronan Receptor (CD44) Participates in the Uptake and Degradation of Hyaluronan. J Cell Biol (1992) 116:1055–62. doi: 10.1083/jcb.116.4.1055
50. Culty M, O'Mara TE, Underhill CB, Yeager H Jr., Swartz RP. Hyaluronan Receptor (CD44) Expression and Function in Human Peripheral Blood Monocytes and Alveolar Macrophages. J Leukoc Biol (1994) 56:605–11. doi: 10.1002/jlb.56.5.605
51. Aruffo A, Stamenkovic I, Melnick M, Underhill CB, Seed B. CD44 is the Principal Cell Surface Receptor for Hyaluronate. Cell (1990) 61:1303–13. doi: 10.1016/0092-8674(90)90694-a
52. Miyake K, Underhill CB, Lesley J, Kincade PW. Hyaluronate can Function as a Cell Adhesion Molecule and CD44 Participates in Hyaluronate Recognition. J Exp Med (1990) 172:69–75. doi: 10.1084/jem.172.1.69
53. Toole BP. Hyaluronan and its Binding Proteins, the Hyaladherins. Curr Opin Cell Biol (1990) 2:839–44. doi: 10.1016/0955-0674(90)90081-o
54. Dong Y, Poon GFT, Arif AA, Lee-Sayer SSM, Dosanjh M, Johnson P. The Survival of Fetal and Bone Marrow Monocyte-Derived Alveolar Macrophages is Promoted by CD44 and its Interaction With Hyaluronan. Mucosal Immunol (2018) 11:601–14. doi: 10.1038/mi.2017.83
55. Yeligar SM, Mehta AJ, Harris FL, Brown LAS, Hart CM. Pioglitazone Reverses Alcohol-Induced Alveolar Macrophage Phagocytic Dysfunction. J Immunol (2021) 207:483–92. doi: 10.4049/jimmunol.2000565
56. Geng B, Pan J, Zhao T, Ji J, Zhang C, Che Y, et al. Chitinase 3-Like 1-CD44 Interaction Promotes Metastasis and Epithelial-to-Mesenchymal Transition Through Beta-Catenin/Erk/Akt Signaling in Gastric Cancer. J Exp Clin Cancer Res (2018) 37:208. doi: 10.1186/s13046-018-0876-2
57. Malinda KM, Ponce L, Kleinman HK, Shackelton LM, Millis AJ. Gp38k, a Protein Synthesized by Vascular Smooth Muscle Cells, Stimulates Directional Migration of Human Umbilical Vein Endothelial Cells. Exp Cell Res (1999) 250:168–73. doi: 10.1006/excr.1999.4511
58. Nishikawa KC, Millis AJ. Gp38k (CHI3L1) is a Novel Adhesion and Migration Factor for Vascular Cells. Exp Cell Res (2003) 287:79–87. doi: 10.1016/s0014-4827(03)00069-7
59. Zhao T, Su Z, Li Y, Zhang X, You Q. Chitinase-3 Like-Protein-1 Function and its Role in Diseases. Signal Transduct Target Ther (2020) 5:201. doi: 10.1038/s41392-020-00303-7
60. Lee DH, Han JH, Lee YS, Jung YS, Roh YS, Yun JS, et al. Chitinase-3-Like-1 Deficiency Attenuates Ethanol-Induced Liver Injury by Inhibition of Sterol Regulatory Element Binding Protein 1-Dependent Triglyceride Synthesis. Metabolism (2019) 95:46–56. doi: 10.1016/j.metabol.2019.03.010
61. Nojgaard C, Johansen JS, Christensen E, Skovgaard LT, Price PA, Becker U, et al. Serum Levels of YKL-40 and PIIINP as Prognostic Markers in Patients With Alcoholic Liver Disease. J Hepatol (2003) 39:179–86. doi: 10.1016/s0168-8278(03)00184-3
62. Johansen JS, Christoffersen P, Moller S, Price PA, Henriksen JH, Garbarsch C, et al. Serum YKL-40 is Increased in Patients With Hepatic Fibrosis. J Hepatol (2000) 32:911–20. doi: 10.1016/s0168-8278(00)80095-1
63. Kronborg G, Ostergaard C, Weis N, Nielsen H, Obel N, Pedersen SS, et al. Serum Level of YKL-40 is Elevated in Patients With Streptococcus Pneumoniae Bacteremia and is Associated With the Outcome of the Disease. Scand J Infect Dis (2002) 34:323–6. doi: 10.1080/00365540110080233
64. Ostergaard C, Johansen JS, Benfield T, Price PA, Lundgren JD. YKL-40 is Elevated in Cerebrospinal Fluid From Patients With Purulent Meningitis. Clin Diagn Lab Immunol (2002) 9:598–604. doi: 10.1128/cdli.9.3.598-604.2002
65. Milner CM, Tongsoongnoen W, Rugg MS, Day AJ. The Molecular Basis of Inter-Alpha-Inhibitor Heavy Chain Transfer on to Hyaluronan. Biochem Soc Trans (2007) 35:672–6. doi: 10.1042/BST0350672
66. Salustri A, Garlanda C, Hirsch E, De Acetis M, Maccagno A, Bottazzi B, et al. PTX3 Plays a Key Role in the Organization of the Cumulus Oophorus Extracellular Matrix and in. Vivo fertilization Dev (2004) 131:1577–86. doi: 10.1242/dev.01056
67. Stober VP, Johnson CG, Majors A, Lauer ME, Cali V, Midura RJ, et al. TNF-Stimulated Gene 6 Promotes Formation of Hyaluronan-Inter-Alpha-Inhibitor Heavy Chain Complexes Necessary for Ozone-Induced Airway Hyperresponsiveness. J Biol Chem (2017) 292:20845–58. doi: 10.1074/jbc.M116.756627
68. Garantziotis S, Li Z, Potts EN, Kimata K, Zhuo L, Morgan DL, et al. Hyaluronan Mediates Ozone-Induced Airway Hyperresponsiveness in Mice. J Biol Chem (2009) 284:11309–17. doi: 10.1074/jbc.M802400200
69. Garantziotis S, Li Z, Potts EN, Lindsey JY, Stober VP, Polosukhin VV, et al. TLR4 is Necessary for Hyaluronan-Mediated Airway Hyperresponsiveness After Ozone Inhalation. Am J Respir Crit Care Med (2010) 181:666–75. doi: 10.1164/rccm.200903-0381OC
70. Lazrak A, Creighton J, Yu Z, Komarova S, Doran SF, Aggarwal S, et al. Hyaluronan Mediates Airway Hyperresponsiveness in Oxidative Lung Injury. Am J Physiol Lung Cell Mol Physiol (2015) 308:L891–903. doi: 10.1152/ajplung.00377.2014
71. Swaidani S, Cheng G, Lauer ME, Sharma M, Mikecz K, Hascall VC, et al. TSG-6 Protein is Crucial for the Development of Pulmonary Hyaluronan Deposition, Eosinophilia, and Airway Hyperresponsiveness in a Murine Model of Asthma. J Biol Chem (2013) 288:412–22. doi: 10.1074/jbc.M112.389874
72. Balhara J, Koussih L, Mohammed A, Shan L, Lamkhioued B, Gounni AS. PTX3 Deficiency Promotes Enhanced Accumulation and Function of CD11c(+)CD11b(+) DCs in a Murine Model of Allergic Inflammation. Front Immunol (2021) 12:641311. doi: 10.3389/fimmu.2021.641311
73. Jourdain M, Tournoys A, Leroy X, Mangalaboyi J, Fourrier F, Goudemand J, et al. Effects of N Omega-Nitro-L-Arginine Methyl Ester on the Endotoxin-Induced Disseminated Intravascular Coagulation in Porcine Septic Shock. Crit Care Med (1997) 25:452–9. doi: 10.1097/00003246-199703000-00014
74. Mandi Y, Endresz V, Mosolygo T, Burian K, Lantos I, Fulop F, et al. The Opposite Effects of Kynurenic Acid and Different Kynurenic Acid Analogs on Tumor Necrosis Factor-Alpha (TNF-Alpha) Production and Tumor Necrosis Factor-Stimulated Gene-6 (TSG-6) Expression. Front Immunol (2019) 10:1406. doi: 10.3389/fimmu.2019.01406
75. Bottazzi B, Bastone A, Doni A, Garlanda C, Valentino S, Deban L, et al. The Long Pentraxin PTX3 as a Link Among Innate Immunity, Inflammation, and Female Fertility. J Leukoc Biol (2006) 79:909–12. doi: 10.1189/jlb.1005557
76. Presta M, Camozzi M, Salvatori G, Rusnati M. Role of the Soluble Pattern Recognition Receptor PTX3 in Vascular Biology. J Cell Mol Med (2007) 11:723–38. doi: 10.1111/j.1582-4934.2007.00061.x
77. Deban L, Jaillon S, Garlanda C, Bottazzi B, Mantovani A. Pentraxins in Innate Immunity: Lessons From PTX3. Cell Tissue Res (2011) 343:237–49. doi: 10.1007/s00441-010-1018-0
78. Kasuda S, Kudo R, Yuui K, Sakurai Y, Hatake K. Acute Ethanol Intoxication Suppresses Pentraxin 3 Expression in a Mouse Sepsis Model Involving Cecal Ligation and Puncture. Alcohol (2017) 64:1–9. doi: 10.1016/j.alcohol.2017.04.003
79. Jimenez VM Jr., Settles EW, Currie BJ, Keim PS, Monroy FP. Persistence of Burkholderia Thailandensis E264 in Lung Tissue After a Single Binge Alcohol Episode. PloS One (2019) 14:e0218147. doi: 10.1371/journal.pone.0218147
80. Hallgren R, Samuelsson T, Laurent TC, Modig J. Accumulation of Hyaluronan (Hyaluronic Acid) in the Lung in Adult Respiratory Distress Syndrome. Am Rev Respir Dis (1989) 139:682–7. doi: 10.1164/ajrccm/139.3.682
81. Hallgren O, Nihlberg K, Dahlback M, Bjermer L, Eriksson LT, Erjefalt JS, et al. Altered Fibroblast Proteoglycan Production in COPD. Respir Res (2010) 11:55. doi: 10.1186/1465-9921-11-55
82. Evanko SP, Potter-Perigo S, Johnson PY, Wight TN. Organization of Hyaluronan and Versican in the Extracellular Matrix of Human Fibroblasts Treated With the Viral Mimetic Poly I:C. J Histochem Cytochem (2009) 57:1041–60. doi: 10.1369/jhc.2009.953802
83. Chang MY, Kang I, Gale M Jr, Manicone AM, Kinsella MG, Braun KR, et al. Versican is Produced by Trif- and Type I Interferon-Dependent Signaling in Macrophages and Contributes to Fine Control of Innate Immunity in Lungs. Am J Physiol Lung Cell Mol Physiol (2017) 313:L1069–86. doi: 10.1152/ajplung.00353.2017
84. Elibol B, Beker M, Jakubowska-Dogru E, Kilic U. Fetal Alcohol and Maternal Stress Modify the Expression of Proteins Controlling Postnatal Development of the Male Rat Hippocampus. Am J Drug Alcohol Abuse (2020) 46:718–30. doi: 10.1080/00952990.2020.1780601
85. Wang W, Xu GL, Jia WD, Ma JL, Li JS, Ge YS, et al. Ligation of TLR2 by Versican: A Link Between Inflammation and Metastasis. Arch Med Res (2009) 40:321–3. doi: 10.1016/j.arcmed.2009.04.005
86. Zhang Z, Miao L, Wang L. Inflammation Amplification by Versican: The First Mediator. Int J Mol Sci (2012) 13:6873–82. doi: 10.3390/ijms13066873
87. Snyder JM, Washington IM, Birkland T, Chang MY, Frevert CW. Correlation of Versican Expression, Accumulation, and Degradation During Embryonic Development by Quantitative Immunohistochemistry. J Histochem Cytochem (2015) 63:952–67. doi: 10.1369/0022155415610383
88. Kang I, Harten IA, Chang MY, Braun KR, Sheih A, Nivison MP, et al. Versican Deficiency Significantly Reduces Lung Inflammatory Response Induced by Polyinosine-Polycytidylic Acid Stimulation. J Biol Chem (2017) 292:51–63. doi: 10.1074/jbc.M116.753186
89. Currie AJ, Davidson DJ, Reid GS, Bharya S, MacDonald KL, Devon RS, et al. Primary Immunodeficiency to Pneumococcal Infection Due to a Defect in Toll-Like Receptor Signaling. J Pediatr (2004) 144:512–8. doi: 10.1016/j.jpeds.2003.10.034
90. Bailey KL, Wyatt TA, Katafiasz DM, Taylor KW, Heires AJ, Sisson JH, et al. Alcohol and Cannabis Use Alter Pulmonary Innate Immunity. Alcohol (2019) 80:131–8. doi: 10.1016/j.alcohol.2018.11.002
91. Wight TN, Kang I, Merrilees MJ. Versican and the Control of Inflammation. Matrix Biol (2014) 35:152–61. doi: 10.1016/j.matbio.2014.01.015
92. D'Souza M, Datta K. Evidence for Naturally Occurring Hyaluronic Acid Binding Protein in Rat Liver. Biochem Int (1985) 10:43–51.
93. Turley EA, Noble PW, Bourguignon LY. Signaling Properties of Hyaluronan Receptors. J Biol Chem (2002) 277:4589–92. doi: 10.1074/jbc.R100038200
94. Yang B, Zhang L, Turley EA. Identification of Two Hyaluronan-Binding Domains in the Hyaluronan Receptor RHAMM. J Biol Chem (1993) 268:8617–23. doi: 10.1016/S0021-9258(18)52919-7
95. Assmann V, Jenkinson D, Marshall JF, Hart IR. The Intracellular Hyaluronan Receptor RHAMM/IHABP Interacts With Microtubules and Actin Filaments. J Cell Sci 112 ( Pt (1999) 22):3943–54. doi: 10.1242/jcs.112.22.3943
96. Entwistle J, Hall CL, Turley EA. HA Receptors: Regulators of Signalling to the Cytoskeleton. J Cell Biochem (1996) 61:569–77. doi: 10.1002/(sici)1097-4644(19960616)61:4<569::aid-jcb10>3.0.co;2-b
97. Lynn BD, Turley EA, Nagy JI. Subcellular Distribution, Calmodulin Interaction, and Mitochondrial Association of the Hyaluronan-Binding Protein RHAMM in Rat Brain. J Neurosci Res (2001) 65:6–16. doi: 10.1002/jnr.1122
98. Sherman L, Sleeman J, Herrlich P, Ponta H. Hyaluronate Receptors: Key Players in Growth, Differentiation, Migration and Tumor Progression. Curr Opin Cell Biol (1994) 6:726–33. doi: 10.1016/0955-0674(94)90100-7
99. Zaman A, Cui Z, Foley JP, Zhao H, Grimm PC, Delisser HM, et al. Expression and Role of the Hyaluronan Receptor RHAMM in Inflammation After Bleomycin Injury. Am J Respir Cell Mol Biol (2005) 33:447–54. doi: 10.1165/rcmb.2004-0333OC
100. Nedvetzki S, Gonen E, Assayag N, Reich R, Williams RO, Thurmond RL, et al. RHAMM, a Receptor for Hyaluronan-Mediated Motility, Compensates for CD44 in Inflamed CD44-Knockout Mice: A Different Interpretation of Redundancy. Proc Natl Acad Sci U.S.A. (2004) 101:18081–6. doi: 10.1073/pnas.0407378102
101. Liang J, Jiang D, Jung Y, Xie T, Ingram J, Church T, et al. Role of Hyaluronan and Hyaluronan-Binding Proteins in Human Asthma. J Allergy Clin Immunol (2011) 128:403–411 e403. doi: 10.1016/j.jaci.2011.04.006
102. Esposito AJ, Bhatraju PK, Stapleton RD, Wurfel MM, Mikacenic C. Hyaluronic Acid is Associated With Organ Dysfunction in Acute Respiratory Distress Syndrome. Crit Care (2017) 21:304. doi: 10.1186/s13054-017-1895-7
103. Liang Y, Yeligar SM, Brown LA. Chronic-Alcohol-Abuse-Induced Oxidative Stress in the Development of Acute Respiratory Distress Syndrome. ScientificWorldJournal (2012) 2012:740308. doi: 10.1100/2012/740308
104. Samuel SK, Hurta RA, Spearman MA, Wright JA, Turley EA, Greenberg AH, et al. TGF-Beta 1 Stimulation of Cell Locomotion Utilizes the Hyaluronan Receptor RHAMM and Hyaluronan. J Cell Biol (1993) 123:749–58. doi: 10.1083/jcb.123.3.749
105. Szabo G, Mandrekar P, Girouard L, Catalano D. Regulation of Human Monocyte Functions by Acute Ethanol Treatment: Decreased Tumor Necrosis Factor-Alpha, Interleukin-1 Beta and Elevated Interleukin-10, and Transforming Growth Factor-Beta Production. Alcohol Clin Exp Res (1996) 20:900–7. doi: 10.1111/j.1530-0277.1996.tb05269.x
106. Sueblinvong V, Tseng V, Smith T, Saghafi R, Mills ST, Neujahr DC, et al. TGFbeta1 Mediates Alcohol-Induced Nrf2 Suppression in Lung Fibroblasts. Alcoholism Clin Exp Res (2014) 38:2731–42. doi: 10.1111/acer.12563
107. Kaphalia L, Calhoun WJ. Alcoholic Lung Injury: Metabolic, Biochemical and Immunological Aspects. Toxicol Lett (2013) 222:171–9. doi: 10.1016/j.toxlet.2013.07.016
108. Brown SD, Brown LA. Ethanol (EtOH)-Induced TGF-Beta1 and Reactive Oxygen Species Production are Necessary for EtOH-Induced Alveolar Macrophage Dysfunction and Induction of Alternative Activation. Alcoholism Clin Exp Res (2012) 36:1952–62. doi: 10.1111/j.1530-0277.2012.01825.x
109. Jha BK, Mitra N, Rana R, Surolia A, Salunke DM, Datta K. pH and Cation-Induced Thermodynamic Stability of Human Hyaluronan Binding Protein 1 Regulates its Hyaluronan Affinity. J Biol Chem (2004) 279:23061–72. doi: 10.1074/jbc.M310676200
110. Yadav G, Prasad RL, Jha BK, Rai V, Bhakuni V, Datta K. Evidence for Inhibitory Interaction of Hyaluronan-Binding Protein 1 (HABP1/p32/gC1qR) With Streptococcus Pneumoniae Hyaluronidase. J Biol Chem (2009) 284:3897–905. doi: 10.1074/jbc.M804246200
111. Wagner MC, Yeligar SM, Brown LA, Michael Hart C. PPARgamma Ligands Regulate NADPH Oxidase, eNOS, and Barrier Function in the Lung Following Chronic Alcohol Ingestion. Alcohol Clin Exp Res (2012) 36:197–206. doi: 10.1111/j.1530-0277.2011.01599.x
112. Liang Y, Harris FL, Jones DP, Brown LAS. Alcohol Induces Mitochondrial Redox Imbalance in Alveolar Macrophages. Free Radic Biol Med (2013) 65:1427–34. doi: 10.1016/j.freeradbiomed.2013.10.010
113. Liang Y, Harris FL, Brown LA. Alcohol Induced Mitochondrial Oxidative Stress and Alveolar Macrophage Dysfunction. BioMed Res Int (2014) 2014:371593. doi: 10.1155/2014/371593
114. Morris NL, Harris FL, Brown LAS, Yeligar SM. Alcohol Induces Mitochondrial Derangements in Alveolar Macrophages by Upregulating NADPH Oxidase 4. Alcohol (2021) 90:27–38. doi: 10.1016/j.alcohol.2020.11.004
115. Fogal V, Richardson AD, Karmali PP, Scheffler IE, Smith JW, Ruoslahti E. Mitochondrial P32 Protein is a Critical Regulator of Tumor Metabolism via Maintenance of Oxidative Phosphorylation. Mol Cell Biol (2010) 30:1303–18. doi: 10.1128/MCB.01101-09
116. Muta T, Kang D, Kitajima S, Fujiwara T, Hamasaki N. P32 Protein, a Splicing Factor 2-Associated Protein, is Localized in Mitochondrial Matrix and is Functionally Important in Maintaining Oxidative Phosphorylation. J Biol Chem (1997) 272:24363–70. doi: 10.1074/jbc.272.39.24363
117. Yagi M, Uchiumi T, Takazaki S, Okuno B, Nomura M, Yoshida S, et al. P32/Gc1qr is Indispensable for Fetal Development and Mitochondrial Translation: Importance of its RNA-Binding Ability. Nucleic Acids Res (2012) 40:9717–37. doi: 10.1093/nar/gks774
118. Sunderhauf A, Raschdorf A, Hicken M, Schlichting H, Fetzer F, Brethack AK, et al. GC1qR Cleavage by Caspase-1 Drives Aerobic Glycolysis in Tumor Cells. Front Oncol (2020) 10:575854. doi: 10.3389/fonc.2020.575854
119. Sun YJ, Shi GH, Zhao WW, Zheng SY. HABP1 Promotes Proliferation and Invasion of Lung Adenocarcinoma Cells Through NFkappaB Pathway. Neoplasma (2021) 69:155–64. doi: 10.4149/neo_2021_210904N1271
120. Sumiya J, Asakawa S, Tobe T, Hashimoto K, Saguchi K, Choi-Miura NH, et al. Isolation and Characterization of the Plasma Hyaluronan-Binding Protein (PHBP) Gene (HABP2). J Biochem (1997) 122:983–90. doi: 10.1093/oxfordjournals.jbchem.a021861
121. Mambetsariev N, Mirzapoiazova T, Mambetsariev B, Sammani S, Lennon FE, Garcia JG, et al. Hyaluronic Acid Binding Protein 2 Is a Novel Regulator of Vascular Integrity. Arterioscler Thromb Vasc Biol (2010) 30:483–90. doi: 10.1161/ATVBAHA.109.200451
122. Burnham EL, Brown LA, Halls L, Moss M. Effects of Chronic Alcohol Abuse on Alveolar Epithelial Barrier Function and Glutathione Homeostasis. Alcoholism Clin Exp Res (2003) 27:1167–72. doi: 10.1097/01.ALC.0000075821.34270.98
123. Pelaez A, Bechara RI, Joshi PC, Brown LA, Guidot DM. Granulocyte/macrophage Colony-Stimulating Factor Treatment Improves Alveolar Epithelial Barrier Function in Alcoholic Rat Lung. Am J Physiol Lung Cell Mol Physiol (2004) 286:L106–111. doi: 10.1152/ajplung.00148.2003
124. Wygrecka M, Markart P, Fink L, Guenther A, Preissner KT. Raised Protein Levels and Altered Cellular Expression of Factor VII Activating Protease (FSAP) in the Lungs of Patients With Acute Respiratory Distress Syndrome (ARDS). Thorax (2007) 62:880–8. doi: 10.1136/thx.2006.069658
125. Byskov K, Etscheid M, Kanse SM. Cellular Effects of Factor VII Activating Protease (FSAP). Thromb Res (2020) 188:74–8. doi: 10.1016/j.thromres.2020.02.010
126. Moraes TJ, Martin R, Plumb JD, Vachon E, Cameron CM, Danesh A, et al. Role of PAR2 in Murine Pulmonary Pseudomonal Infection. Am J Physiol Lung Cell Mol Physiol (2008) 294:L368–377. doi: 10.1152/ajplung.00036.2007
127. Kager LM, Schouten M, Wiersinga WJ, de Boer JD, Lattenist LC, Roelofs JJ, et al. Overexpression of the Endothelial Protein C Receptor is Detrimental During Pneumonia-Derived Gram-Negative Sepsis (Melioidosis). PloS Negl Trop Dis (2013) 7:e2306. doi: 10.1371/journal.pntd.0002306
128. Schouten M, van't Veer C, Roelofs JJ, Levi M, van der Poll T. Protease-Activated Receptor-1 Impairs Host Defense in Murine Pneumococcal Pneumonia: A Controlled Laboratory Study. Crit Care (2012) 16:R238. doi: 10.1186/cc11910
129. Johnson LA, Banerji S, Lawrance W, Gileadi U, Prota G, Holder KA, et al. Dendritic Cells Enter Lymph Vessels by Hyaluronan-Mediated Docking to the Endothelial Receptor LYVE-1. Nat Immunol (2017) 18:762–70. doi: 10.1038/ni.3750
130. Banerji S, Ni J, Wang SX, Clasper S, Su J, Tammi R, et al. LYVE-1, a New Homologue of the CD44 Glycoprotein, is a Lymph-Specific Receptor for Hyaluronan. J Cell Biol (1999) 144:789–801. doi: 10.1083/jcb.144.4.789
131. Jackson DG. Leucocyte Trafficking via the Lymphatic Vasculature- Mechanisms and Consequences. Front Immunol (2019) 10:471. doi: 10.3389/fimmu.2019.00471
Keywords: hyaluronan, alcohol use disorder, pneumonia, hyaladherin, immunity
Citation: Crotty KM and Yeligar SM (2022) Hyaladherins May be Implicated in Alcohol-Induced Susceptibility to Bacterial Pneumonia. Front. Immunol. 13:865522. doi: 10.3389/fimmu.2022.865522
Received: 30 January 2022; Accepted: 15 April 2022;
Published: 12 May 2022.
Edited by:
Jean-Michel Constantin, Hôpital Pitié-Salpêtrière, FranceReviewed by:
Gavin Arteel, University of Pittsburgh, United StatesCopyright © 2022 Crotty and Yeligar. This is an open-access article distributed under the terms of the Creative Commons Attribution License (CC BY). The use, distribution or reproduction in other forums is permitted, provided the original author(s) and the copyright owner(s) are credited and that the original publication in this journal is cited, in accordance with accepted academic practice. No use, distribution or reproduction is permitted which does not comply with these terms.
*Correspondence: Samantha M. Yeligar, c3llbGlnYUBlbW9yeS5lZHU=
†ORCID: Kathryn M. Crotty, orcid.org/0000-0002-9461-4032
Samantha M. Yeligar, orcid.org/0000-0001-9309-0233