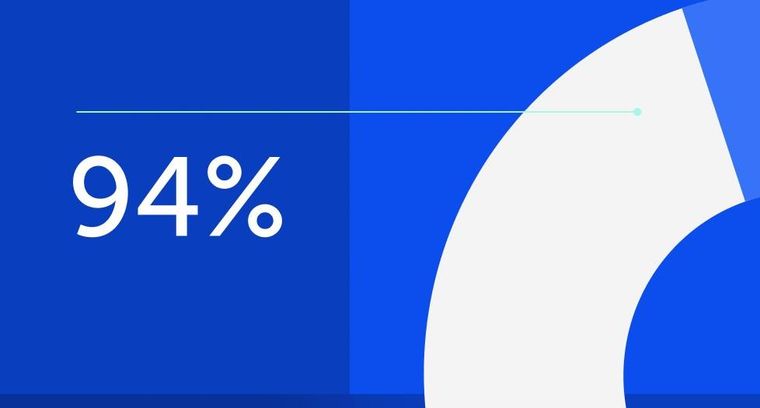
94% of researchers rate our articles as excellent or good
Learn more about the work of our research integrity team to safeguard the quality of each article we publish.
Find out more
REVIEW article
Front. Immunol., 27 April 2022
Sec. Alloimmunity and Transplantation
Volume 13 - 2022 | https://doi.org/10.3389/fimmu.2022.861583
This article is part of the Research TopicA Hill of Needs: Current State of the Art and Knowledge Gaps for Non-HLA Antibodies in Allograft TransplantationView all 6 articles
Transplantation is a treatment option for patients diagnosed with end-stage organ diseases; however, long-term graft survival is affected by rejection of the transplanted organ by immune and nonimmune responses. Several studies have demonstrated that both acute and chronic rejection can occur after transplantation of kidney, heart, and lungs. A strong correlation has been reported between de novo synthesis of donor-specific antibodies (HLA-DSAs) and development of both acute and chronic rejection; however, some transplant recipients with chronic rejection do not have detectable HLA-DSAs. Studies of sera from such patients demonstrate that immune responses to tissue-associated antigens (TaAgs) may also play an important role in the development of chronic rejection, either alone or in combination with HLA-DSAs. The synergistic effect between HLA-DSAs and antibodies to TaAgs is being established, but the underlying mechanism is yet to be defined. We hypothesize that HLA-DSAs damage the transplanted donor organ resulting in stress and leading to the release of extracellular vesicles, which contribute to chronic rejection. These vesicles express both donor human leukocyte antigen (HLA) and non-HLA TaAgs, which can activate antigen-presenting cells and lead to immune responses and development of antibodies to both donor HLA and non-HLA tissue-associated Ags. Extracellular vesicles (EVs) are released by cells under many circumstances due to both physiological and pathological conditions. Primarily employing clinical specimens obtained from human lung transplant recipients undergoing acute or chronic rejection, our group has demonstrated that circulating extracellular vesicles display both mismatched donor HLA molecules and lung-associated Ags (collagen-V and K-alpha 1 tubulin). This review focuses on recent studies demonstrating an important role of antibodies to tissue-associated Ags in the rejection of transplanted organs, particularly chronic rejection. We will also discuss the important role of extracellular vesicles released from transplanted organs in cross-talk between alloimmunity and autoimmunity to tissue-associated Ags after solid organ transplantation.
Emerging research shows that small extracellular vesicles (sEVs) are potential biomarkers and immune mediators in the field of transplantation (1–3). After lung transplantation (LTx), sEVs identified in the circulation and locally in the bronchoalveolar lavage fluid had distinct RNA profiles under normal and inflammatory conditions (4, 5). Our reports demonstrate the presence of mismatched donor human leukocyte antigens (HLAs) and lung associated-antigens on sEVs surfaces after LTx, suggesting that sEVs with lung TaAgs can be a biomarker for monitoring allograft rejection (6). SEVs derived from donor dendritic cells have been demonstrated to promote allograft-targeting immune responses by transferring immune-activating signals and donor HLA molecules (7). Studies have shown that the development of de novo antibodies (Abs) to mismatched donor-HLA is associated with chronic rejection after LTx, which is clinically diagnosed as bronchiolitis obliterans syndrome (BOS) (8, 9). Evidence also suggests that anti-donor responses leading to rejection are often due to de novo donor-specific antibodies (HLA-DSAs) and donor HLA reactive immune T cells (cellular rejection) (10). Recent evidence clearly demonstrates that Abs against donor HLA present either before transplantation or developed de novo after transplantation are strongly associated with antibody-mediated rejection (AMR), and repeated episodes of AMR are an important risk factor for chronic lung allograft dysfunction (CLAD) (11, 12). We have demonstrated that the development of Abs to TaAgs increases the risk for the development of HLA-DSAs and vice versa, indicating crosstalk between allo- and auto-immunity, both of which are implicated in the development of BOS (13). Abs to HLAs combined with a loss of the T regulatory cell population is also implicated in chronic rejection (14, 15). Several risk factors have been identified for chronic rejection, including donor organ ischemia, HLA mismatches, development of de novo HLA-DSAs, recurrent/refractory acute rejections, and viral infections (16, 17). We postulate that any of these risk factors can lead to inflammation and tissue remodeling, which facilitates the induction and release of extracellular vesicles (EVs), leading to immune responses against donor alloantigens and TaAgs and the development of allo- and auto-immunity. Although sEVs may have many relevant biological functions, including the induction of rejection and/or tolerance, data supporting the contribution of sEVs to these processes are currently limited. Therefore, the mechanisms by which sEVs regulate immune responses need further investigation.
Immune responses are recognized immediately after organ transplantation due to ischemia and reperfusion injury of the transplanted organ (18–21). Recent studies demonstrate a strong correlation between ischemia-reperfusion injury and de novo HLA-DSAs (22), thus increasing the risk for development of chronic rejection after human LTx (23–25), and many reports strongly support the concept that de novo HLA-DSAs after transplantation can lead to allograft dysfunction, including AMR (23, 26–28). It is also clear that de novo HLA-DSAs are an important player in allograft dysfunction during cellular rejection as many transplant recipients with cellular rejection have evidence of both T cells mediated immune response and Abs specific to mismatched HLA (29–31). De novo HLA-DSAs are an established biomarker for predicting AMR (32–35) and also play a significant role in the immune-pathogenesis of chronic rejection (36). Earlier studies have demonstrated that even a transient presence of de novo HLA-DSAs can be an important risk factor for the development of chronic rejection, indicating that persistent HLA-DSAs may not be necessary (37, 38).
Employing sera from LTx recipients diagnosed with BOS but without detectable HLA-DSAs in the circulation, we defined immune responses to lung tissue–associated Ags expressed on airway epithelial cells and their possible role in the development of BOS (39). Further, serial analysis of antibody development after LTx demonstrated that HLA-DSAs can induce immune responses to lung TaAgs, which may lead to the pathogenesis of chronic rejection (13). From these studies, we concluded that HLA-DSAs may induce immune responses to TaAgs, which either alone or in combination of both increases the risk of BOS. Significantly, primary graft dysfunction (PGD) is also a well-known risk factor for the development of BOS (40–42). Inflammation and tissue remodeling in the recipient and the surgical stress during transplantation can lead to the expression of sequestrated antigenic epitopes of TaAgs present in the lungs, and pre-existing immune responses to TaAgs can lead to PGD (43). Therefore, it is important to determine the role of pre-existing immune response to TaAgs after LTx so that rejection can be avoided.
Several well-studied non-HLA antigens may play a role in immune responses following organ transplantation. Immune responses against collagen V (Col-V) and K-alpha 1 tubulin (Kα1T) have been shown to play an important role in eliciting both cellular and humoral immune responses leading to lung allograft rejection (44, 45). Furthermore, Abs against cardiac myosin (MYO) and vimentin (VIM) have been reported to be present during cardiac allograft rejection (46, 47). After human kidney transplantation, Abs against perlecan (48), Col-IV, and fibronectin (FN) have been associated with rejection (49). In addition, immune responses against major histocompatibility complex (MHC) class I-related chain A (MICA) molecules have been identified in patients undergoing lung, kidney, and heart allograft rejection (50–52). These Abs to non-HLA antigens often precede the diagnosis of chronic rejection in lung, heart, and kidney recipients, suggesting a causal relationship (50–52). Recently, the International Society of Heart and Lung Transplantation guidelines proposed AMR as a distinct clinicopathological entity characterized by the presence of allograft dysfunction in concert with histological findings of capillary injury, positive immunofluorescence for C4d in lung tissue biopsies, and detection of circulating HLA-DSAs (11, 53). Accumulating evidence now suggests that early post-transplant events promote the development of inflammatory processes which subsequently lead to the development of de novo HLA-DSAs, increasing the risk for rejection of the transplanted organ (9, 27, 35). We demonstrated the role of HLA-DSAs to mismatched HLA molecules and Abs against two important lungs TaAgs (Col-V, Kα1T) in LTx recipients diagnosed with BOS (54–56). The presence of HLA-DSAs during AMR has also been associated with the development of Abs to Col-V and Kα1T in LTx recipients (55).
Col-V is a collagen intercalated within fibrils of type I collagen present in lung tissue. Yoshida et al. have demonstrated that Col-V is exposed and also released in bronchoalveolar lavage fluid due to ischemia-reperfusion injury during LTx (57). Iwata et al. have shown that graft injury mediated by HLA-DSAs can also modify Col-V and release Col-V fragments that serve as a major target in the transplanted organ for the development of Abs against TaAgs (58). Our laboratory analyzed pre-transplant sera from 317 LTx recipients between 2000 and 2011 with a diagnosis of chronic obstructive pulmonary disease, idiopathic pulmonary fibrosis, cystic fibrosis, or other end-stage lung diseases for Abs to Col-V and demonstrated that patients with idiopathic pulmonary fibrosis and cystic fibrosis had the highest prevalence of Abs to lung TaAgs. The Abs to lung TaAgs increased the risk for development of HLA-DSAs, PGD, and BOS (59). Tiriveedhi et al. demonstrated that Col-V epitopes shift from both α1 and α2 to only α1during BOS; this shift in immunodominant epitopes is correlated with a decreased expression of IL-10 and increased expression of IFN-γ (60). Col-V-specific Th17 cells and monocyte/macrophage accessory cells have been shown to cause progressive airway obliteration (61). De novo immunity to Col-V resulting in BOS was associated with donor-lung HLA-DR15+, suggesting that donor-derived HLA-DR15 presents novel self-peptides to recipient T cells (62).
We also demonstrated that administration of Abs to lung TaAgs (Col-V, Kα1T) in animal models of LTx resulted in antigenic epitope spreading between lung TaAgs and allo-MHC molecules leading to marked lung graft cellular infiltration, bronchiolar fibrosis, and loss of tolerance (63). Passive administration of Abs to Kα1T also led to cellular and humoral immune responses to Col-V, demonstrating a spreading of immune responses leading to the development of fibrosis in the transplanted organ and signifying the importance of immune responses to TaAgs in the pathogenesis of chronic rejection (45).
Studies have shown that tolerance to a lung allograft can be induced when CD4+ Col-V-specific regulatory T cells, which can downregulate Th17-mediated acute rejection, are administered after LTx (64). T regulatory cells that mediate Col-V-induced tolerance to lung allografts are phenotypically CD4+CD45RC high, lacking Smad7, and have not been associated with TGF-β-mediated signaling (65). We also investigated the role of B cells and their antigen-presenting properties in the induction of obliterative airway disease model where Abs to MHC class I were administered in B cell −/− and wild-type mice. Incidence of Kα1T- and Col-V-specific IL-17 cells significantly decreased in B−/− mice, and Abs against TaAgs and germinal center formation did not develop in B−/− mice (66). Our study has also described that induction of a transcription factor, zinc finger, and BTB domain-containing protein 7a (Zbtb7a) in alveolar macrophages were critical regulators of the inflammatory circuit associated with development of Abs to TaAgs in an obliterative airway disease model (67).
Kα1T is an epithelial gap junction-associated protein expressed also in the lungs (36). In a clinical study of adult LTx recipients (N=142), we demonstrated that the presence of Abs to Kα1T pre-transplant led to an increase in proinflammatory cytokines IL-1 (2.1 fold increase), IL-2 (3.0 fold increase), IL-12 (2.5 fold increase), and IL-15 (3.0 fold increase) and chemokines IP-10 (3.9 fold increase) and MCP-1 (3.1 fold increase) and increased the risk of PGD and BOS (68).
Budding et al. demonstrated the presence of anti-ETAR and anti-AT1R auto-Abs in both pre- and post-transplant sera from 43 LTx patients transplanted due to chronic obstructive pulmonary disease, cystic fibrosis, or interstitial lung disease (69). Pre- and post-transplant sera from 162 LTx recipients at three centers between 2011 and 2013 were tested for Abs to AT1R and ETAR. Recipients with strong/intermediate binding of Abs to AT1R and ETAR had increased AMR onset and development of de novo HLA-DSA (70). After organ transplantation, development of Abs to AT1R and ETAR is likely due to damage to the endothelium of the host or transplanted organ followed by shedding of the extracellular particles of the receptors (71).
Abs to tissue-associated antigens have been validated as important mediators of rejection, allograft dysfunction, and cardiac allograft vasculopathy (CAV) after heart transplantation (72, 73). In heart transplant recipients, increased levels of AT1R have been associated with AMR, cell-mediated rejection, and early onset of micro vasculopathy at 1-year post-transplant (74). A recent study has demonstrated an association of VIM Ab with other non-HLA Abs in heart transplant recipients even when the patient is treated for AMR (75). Analysis using a non-HLA antigen multiplex panel has also documented, and validated, an important role for non-HLA Abs in heart transplantation (76).
An earlier study demonstrated allogeneic heart transplantation in mice resulted in a breakdown of immune tolerance to cardiac MYO, and pre-transplant sensitization of recipient mice with cardiac MYO caused a marked acceleration of rejection of allogeneic heart grafts. This strongly suggests that allogeneic transplant-induced autoimmunity to cardiac MYO contributes to cardiac graft rejection (77). Humoral autoimmune responses against cardiac MYO were associated with dilated cardiomyopathy and increased the frequency and severity of acute rejection after heart transplantation (78). Further studies that evaluated Abs to cardiac MYO in pre- and post-transplant sera from 41 adult cardiac allograft recipients suggested a pathogenic role for anti-MYO Abs in both acute and CAV-related heart transplant rejection (46, 79).
Earlier studies in nonhuman primates also demonstrated the role of humoral immunity to VIM and its association with cardiac allograft injury (80). Mahesh B, et al. (81, 82) demonstrated that autoimmune responses to VIM in conjunction with alloimmune responses accelerate CAV progression in murine cardiac allografts.
Tissue-associated Ags are cryptic epitopes that can serve as targets for the development of autoimmunity when exposed (83). Studies have demonstrated that Abs against kidney TaAgs contribute to the process of acute AMR after kidney transplantation (83, 84) and to the development of transplant glomerulopathy (49). Well-characterized renal TaAgs include AGT1R perlecan, Col-IV, and FN. Perlecan, a proteoglycan embedded within the vascular basement membrane, when degraded by metalloproteinases or cathepsin-L, releases endorepellin and a truncated C-terminal fragment harboring a laminin G motif (LG3) (85). Increased urinary levels of LG3 have also been observed in kidney transplant recipients with chronic rejection (86, 87). Endothelial apoptosis is associated with acute and chronic vascular rejection of solid allografts (88). Apoptotic endothelial cells release LG3, a regulator of obliterative vascular remodeling during rejection, which can lead to development of autoimmunity (86). Yang et al. demonstrated that the presence of pre-transplant LG3 was associated with reduced allograft function at 1 year in kidney transplant recipients; severity of renal injury at the time of transplantation had a negative impact on long-term outcomes (89). Recent studies have also demonstrated that Abs against perlecan can cause acute humoral rejection in pre-sensitized patients regardless of development of Abs to HLA after kidney transplantation (90).
Col-IV is a major constituent of glomerular basement membranes (91, 92). Studies have demonstrated that Col-IV is upregulated in acutely deteriorated renal allografts, and differential staining of Col-IV in glomerular basement membranes and the interstitium may help to diagnose chronic transplant nephropathy (93).
BK virus–associated nephropathy (94) and persistent BK viremia (defined as lasting >140 days) were also associated with de novo development of Abs against mismatched donor HLA antigens (HLA-DSA) (95). We demonstrated polyomavirus reactivation was associated with early de novo development of Abs to the kidney-associated Ag FN; the immune responses to FN along with Col-IV were associated with an increased risk of acute rejection (96). Dragun et al. first demonstrated the role of Abs to AT1R and its association in renal transplant recipients with acute vascular rejection with refractory hypertension (97). Abs to AT1R that bind to G-protein–coupled receptors can induce stress in endothelial cells via activation of distinct intracellular cell-signaling pathways (98). Several studies have associated pre- and post-transplant AT1R and ETAR Abs with AMR and adverse late graft outcomes in kidney transplantation (99–101).
Our group, including others, demonstrated that the development of Abs to mismatched donor HLA as well as immune responses to lung TaAgs are strongly associated with the development of BOS (55, 102, 103). Studies have shown that lung injury due to PGD, acute rejection, and respiratory viral infections, known risk factors for the development of CLAD, also induce circulatory sEVs with lung TaAgs (6, 104). sEVs (68, 105–107). Based on these results, we propose that stress to the transplanted organ either by PGD, respiratory viral infections, rejection episodes, or HLA-DSAs can release circulating sEVs with lung TaAgs. Persistence of these sEVs in the circulation can lead to continued immune activation and the development of allo- and auto-immunity, which can increase the risk of BOS. EVs interact with cells via numerous ligand–receptor interactions, and sEVs can activate not only via direct and indirect pathways of antigen presentation but also via the semidirect pathway, in which T cell activation occurs via donor-derived sEVs (Figure 1) (108, 109).
Figure 1 EVs interact with cells via numerous ligand–receptor interactions and sEVs can activate not only direct and indirect pathways of antigen presentation but also via the semidirect pathway in which T cell activation occurs via donor-derived sEVs.
EVs, or sEVs, are released by all cells, and EVs are heterogeneous in size and composition. EVs are classified by size: microvesicles (100–1000 nm in diameter), apoptotic bodies (>50-5000 nm in diameter), and sEVs (50–150 nm in diameter) (110, 111). All the secreted vesicles carry proteins/peptides, lipids, nucleic acids (DNA, RNA), and small metabolites; the composition and functional impact of vesicles depend on their cellular origin (112). The composition of sEVs is critical as they can be a potential biomarker for function in biological processes. In addition, there are certain markers, apart from size, are enriched in sEVs than other vesicles (e.g., TSG101, syntenin, tetraspanins, CD9, CD63, and CD81) (113, 114). Focus here is to discuss the role and importance of sEVs in the development of immune responses to TaAgs (autoimmunity) in lung allograft and other solid organ transplant recipients.
SEVs are involved in several biological and immune processes (115). Our group has recently validated the use of circulating sEVs with lung TaAgs as a potential biomarker in the early diagnosis of BOS after LTx (116). In addition, different clinical conditions after LTx that increase the risk for CLAD, e.g., PGD, AR, HLA-DSAs, and respiratory viral infections, can induce circulating sEVs with lung TaAgs (117). Increasing evidence supports a possible role of sEVs in the pathogenesis of various human diseases (108, 118–120). We have reported that sEVs isolated from LTx recipients contain increased levels of the lung TaAgs (Col-V, Kα1T) during acute and chronic rejection (6). Preliminary studies have also shown association of sEV’s containing cardiac TaAgs (VIM and MYO) in syngeneic cardiac transplant rejection induced by antibodies to cardiac myosin rejection (121). Our preliminary studies also demonstrated sEV’s containing kidney antigens (FN and Col-IV) in kidney transplant recipients was associated with transplant glomerulopathy and interstitial fibrosis and tubular atrophy (IFTA) (unpublished data) (Figure 2).
Figure 2 Composition of sEVs from lungs, heart and kidney transplant patients. (A) Col-V and Kα1T TAgs on lung specific sEVs (B) MYO and VIM TAgs on heart specific sEVs (C) Perlecan and Fibronectin TAgs on kidney specific sEVs.
The development of alloimmunity and Abs to TaAgs (autoimmunity) after transplantation is likely due to the induction and release of sEVs from the transplanted organ under varying conditions resulting in stress to the allograft. We and others have demonstrated that circulating sEVs released from the transplanted organ indeed play an important part in eliciting immune responses leading to rejection (7, 109, 122, 123). The presence of mismatched donor HLA and lung TaAgs (Col-V, Kα1T) in sEVs isolated from LTx recipients diagnosed with rejection demonstrates that the sEVs originated from the transplanted organ (6, 104). In a murine model of chronic lung allograft rejection (124) in which a single lung from B6D2F1 (H2b/d) mice was orthotopically transplanted into DBA/2(H2d) recipients, sEVs containing mismatched donor MHC (H2b) and lung TaAgs (Col-V, Kα1T) were released (days 14 and 28), and Abs to donor MHC developed, a known risk factor for pathogenesis of CLAD (12, 27). Furthermore, passive administration of these sEVs to DBA/2 recipient mice led to the development of Abs to donor MHC (H2b) and Abs to lung TaAgs, signifying that sEVs stimulate immune responses that lead to rejection (125). SEVs also contained MHC class II molecules; costimulatory molecules CD40, CD80, and CD86; and transcription factors class II MHC trans-activator, NF-kB, hypoxia inducible factor-1α, IL-1R–associated kinase 1, MyD88, and 20S proteasome (123). These molecules often increase in sEVs in LTx recipients with BOS in comparison to stable LTx recipients (123). We have also demonstrated that sEVs isolated from LTx recipients with BOS are immunogenic in vivo since immunization of mice with isolated sEVs resulted in humoral and cellular immune responses to lung TaAgs (6).
In a recent study, we demonstrated that sEVs from LTx recipients with BOS also contained NK cell markers (CD56, NKG2D) and cytotoxic molecules (perforin, FasL) (126) and, therefore, can play an important role in antiviral and antitumor immune surveillance (127). In the chronic murine LTx rejection model discussed above, we demonstrated that circulating sEVs are also derived from NK cells since isolated sEVs contain NK cell–associated molecules (NKp46, CD56, NKG2D) and cytotoxic molecules (perforin, FasL) (125). Further, NK cell depletion significantly reduced fibrosis in transplanted lung tissue, suggesting a role for NK cells in the pathogenesis of chronic rejection in this murine model of LTx rejection (125).
Recent studies demonstrated that the two phenotypes of CLAD (BOS and restrictive allograft syndrome) can be differentiated by levels of specific proteins in circulating sEVs (128). SEVs from patients with the restrictive allograft syndrome phenotype carry higher amounts of costimulatory marker CIITA, transcription factor NFkB, lung TaAg Kα1T, and class II HLA-DQ and DR molecules and 20S proteasome.
A recent study demonstrated that EVs derived from vascular injury, ApoExo, as novel inducers of tertiary lymphoid structure (TLS) formation by attracting IL‐17‐producing gamma delta T cells to the allograft and ApoEXo proteasome activity was the important mediator of TLS formation (129). The formation of TLS in kidney allografts has been associated with severe and/or chronic forms of rejection. Earlier studies have demonstrated a stepwise breakdown of B-cell tolerance and formation auto antibodies during chronic rejection (130). The mechanism that occurs within the kidney graft during chronic rejection is due to changes in intragraft microenvironment which can be mediated by ApoExo released by local tissue injury (129, 131). A recent study demonstrating donor EVs were transported across the sub capsular sinus macrophages, and donor MHC molecules on the EVs were recognized by alloreactive B cells (132). This triggered B cell activation and DSA production, suggesting the EVs can activate immune response by semi direct pathway and does not always require donor leukocytes to directly interact for B cell allosensitization.
LKB1, also known as serine/threonine protein kinase 11, is a tumor suppressor gene (133, 134). By direct phosphorylation, LKB1 activates a family of 14 kinases related to the AMP-activated protein kinase (AMPK) (135–137). Most functions of LKB1 are attributed to its ability to activate AMPK, a central conserved regulator of metabolism and cell growth (138). AMPK regulates factors involved in cell metabolism, proliferation, survival, migration, and invasion (139–142). It has been demonstrated that loss of LKB1 is a biomarker for more aggressive biology in KRAS-mutant lung adenocarcinoma (6, 143). Studies have also demonstrated that expression of LKB1 inhibits epithelial-mesenchymal transition, tissue fibrosis, and malignant transformation (144, 145).
Recently, we demonstrated significantly reduced abundance of LKB1 in both mRNA/protein level in circulating sEVs isolated from LTx recipients diagnosed with BOS compared to sEVs isolated from stable LTx recipients (146). Our study also demonstrated novel findings indicating that sEV-mediated downregulation of a tumor suppressor gene, LKB1, in primary epithelial cells may also play an important role in chronic rejection after LTx by inducing epithelial-mesenchymal transition by upregulating VIM and α-SMA, leading to the pathogenesis of BOS (146).
A recent report by our group demonstrated that circulating sEVs isolated from plasma and bronchoalveolar lavage fluid from LTx recipients with BOS have donor HLA and significantly increased levels of lung TaAgs (Kα1T, Col-V) (6, 61). Based on this finding, we analyzed plasma samples collected from LTx recipients 6 and 12 months before the clinical diagnosis of BOS from two different LTx centers. Increased levels of lung TaAgs were detected in sEVs isolated from these samples 12 months before the clinical diagnosis of BOS, indicating that circulating sEVs with lung TaAgs can be a viable noninvasive biomarker for identifying patients at risk for developing CLAD (116). The determination of Col-V or Kα1T in circulating sEVs by western blot followed by semi-quantitation provides a higher positive predictive value with excellent sensitivity and specificity. During this study, the validation cohorts demonstrated that levels of sEVs containing Col-V had a sensitivity of 70% and a specificity of 80% at 6 months and a sensitivity of 60% and a specificity of 75% at 12 months before clinical diagnosis of BOS. For the lung TaAg, Kα1T, sensitivity was 60% and specificity was 80% at 6 months and sensitivity was 65% and specificity was 80% at 12 months. Early detection of patients at risk for developing chronic rejection provides an opportunity to develop strategies to prevent or intervene before the onset of irreversible damage to the transplanted organ.
We also found LKB1 expression was downregulated in circulating sEVs isolated from LTx recipients with BOS 6 months before the clinical diagnosis. Recently, it has been reported that aldehyde/sulfate latex beads can bind to sEVs and bead-sEVs can be detected by flow cytometry (147). Using this method, we demonstrated that LKB1 levels were significantly lower in sEVs isolated from LTx recipients 6 months before clinical diagnosis of BOS than in sEVs isolated from stable LTx recipients (146).
LTx recipients are prone to respiratory viral infections (respiratory syncytial virus, rhino, cytomegalovirus, and corona). Respiratory viral infections have been shown to increase the risk of CLAD, but the mechanisms remain largely unknown. One of the reports demonstrated that respiratory viral infections after LTx induce circulating sEVs that contain lung-associated Ags and viral antigens (117), and the viral antigens are on the surface of the isolated sEVs. These sEVs also induced immune responses to TaAgs when mice were immunized with isolated sEVs (123).
sEVs isolated from LTx recipients with respiratory viral infections also contained nucleic acid sequences of DNA and RNA viruses. These sequences in sEVs can induce innate immune signaling, cellular stress, and epithelial-mesenchymal transition both in vitro and in vivo through cGAS/STING and RIG1 pathways (148).
Recently, we demonstrated the physiological role of sEVs in eliciting immune responses during SARS-CoV-2 infection (149). SEVs carrying the SARS-CoV-2 spike protein were detectable in the circulation earlier than Abs to the SARS-CoV-2 spike protein in healthy individuals vaccinated with the mRNA BNT162b2 (Pfizer–BioNTech) vaccine (149). Mice immunized with the sEVs carrying the spike protein also developed Abs to the SARS-CoV-2 spike protein, indicating the immunogenicity of sEVs in vivo (Figure 3).
Figure 3 sEVs from SARS-CoV-2 infected and vaccinated patients. Presence of SARS-CoV-2 spike protein on sEVs and immunization of mice with the sEVs leads to development of SARS-CoV-2 antibodies in mice.
Several studies have demonstrated that microRNAs (miRNAs) play a major role in the regulation of allograft cells function in response to injury following solid organ transplantation (150–152). A recent integrative omics analysis of kidney allograft biopsies demonstrated several miRNA associated with microvascular inflammation-related pathways, in particular miRNA such as miR-142-3p/150-5p/155-5p/222-3p/223-3p (150). Earlier studies from our lab, and others, in organ transplantation have shown miRNA such as miR142-3p/5p (AMR) (153), miR155 (T cell activation and fibrosis) (154), miR223 (inflammation and aggravation of renal dysfunction) (151), miR10a (acute rejection) (155), let-7c (inflammation, cell migration, and proliferation) (156) can predict allograft status (157).
EVs are enriched in noncoding RNAs, of which miRNAs play an important role and the mechanisms regulating selective miRNA packaging into EVs are being explored (158). Earlier studies demonstrated in response to endotoxin stimulation, macrophages secrete EVPs containing miR-155, which in turn promotes inflammatory responses to LPS in vivo (159, 160). These studies provide evidence that miRNA transfer between immune cells facilitate the regulation of inflammatory responses (161). Dendritic cell derived EVs have been demonstrated to regulate innate immunity by EV-mediated transfer of miRNAs among DCs contributes to enhance their mutual activation during inflammation (162, 163). DC derived EVs with CD86, a costimulatory molecule, activates T cells through direct or semi-direct pathway (3, 164). A recent study in murine models of renal allografts demonstrated highly immature DC–derived EVs containing miR-682, suppressed CD4+ T cells and promoted regulatory T cell development, whereas mature DC–derived EVs induced T cell immunity (165). Treg-derived EVs have also been demonstrated to induce a tolerogenic phenotype of DC by transfer of miRNAs (miR-150-5p and miR-142-3p) (166). T cells also release sEVs and other EVs after T cell receptor activation having a dual role (immune suppression or activation) depending on the miRNA content and release (167, 168).
Recent studies have demonstrated that sEVs also play a role in the induction of tolerance after transplantation (169, 170). Donor-derived sEVs possess immunoregulatory molecules and also carry cell-derived antigens and donor HLA molecules. After infusion of donor monocyte-derived regulatory cells 7 days before transplantation, living liver transplant donors demonstrated transiently elevated levels of donor HLA, and immunoregulatory PD-L1, CD39 and CD73 molecules were detected in circulating small EVs, which can promote an immunosuppressed environment (171). Extracellular adenosine is a well-studied neurotransmitter, but it also exerts profound immune regulatory effects on the tolerogenic functions of DCs s by activity of CD73 and CD39 (172). PD-L1 and CD73 also can induce T cell anergy leading to tolerance (164, 173, 174). In an earlier study, circulating sEVs carrying PDL-1 and CD73 inhibited antitumor activities in patients with acute myeloid leukemia by delivering their suppressive cargos to immune recipient cells (175). Further, several reports have shown that allogeneic sEVs and T regulatory cell–derived sEVs can be used to promote tolerance to allografts (164, 176–178). A recent report demonstrated that T regulatory cell–derived IL-35-coated EVs can promote infectious tolerance (179).
Several clinical and pre-clinical studies have demonstrated that immune responses to mismatched donor HLA and tissue-associated non-HLA TaAgs play an important role in the rejection of solid organ transplants; however, many questions remain unanswered. Pre- and post-transplant HLA-DSAs are now routinely monitored not only to select histocompatible donors but also to identify transplant recipients at risk for rejection so that appropriate treatment strategies can be instituted. Identification of sensitization to non-HLA tissue-associated Ags both before and after transplantation is likely to improve the outcome of the transplanted organ. This may also impact long-term survival of allografts by preventing or delaying onset of chronic rejection, which remains a major problem following organ transplantation. Currently, only a limited number of non-HLA tissue-associated Ags have been identified, and their role in rejection has been proposed. Certainly, other molecules and receptors that play a role in allograft rejection are yet to be discovered. For example, muscarinic receptor is a G protein-coupled receptor-like AT1R, distributed in the lungs, that has a distinct functional role in lung physiology. An earlier study demonstrated that high levels of Abs to muscarinic receptors in sera altered immune regulation in patients with chronic fatigue syndrome (180). It is clear from the recent literature that sEVs released from donor organs play an important role in inducing rejection, in particular chronic rejection. Donor-derived sEVs possess immunoregulatory molecules and also carry cell-derived antigens and donor HLA molecules. Apart from intercellular communication, the mechanisms by which sEVs regulate immune responses after transplant is yet to be explored. However, it is evident that circulating sEVs with tissue-associated antigens synergistically mediate alloimmune and autoimmune responses. Therefore, it is important to define the role of circulating sEVs and identify strategies to selectively control the role of donor organ–derived sEVs since recipient sEVs are critical to normal physiology. Studies are also required to confirm the potential use of sEVs as a biomarker of allograft rejection, especially to identify transplant recipients at risk for developing chronic rejection so that strategies can be developed for preventing and treating this form of rejection, which remains a major impediment to long-term function of the transplanted organ, especially lung allografts. Finally, with further investigation, sEVs carrying viral antigens can likely play an important role in the early identification of various viral and bacterial infections affecting organ transplant recipients since circulating sEVs contain viral and bacterial antigens.
Concept and design: TM; Manuscript Drafting, writing, and Figures: RR, SB, MR, and TM; Critical review and support: AS, NS, TF, and AB. All authors contributed to the article and approved the submitted version.
This work was supported by NIH R21AI123034, NIH HL056643 and St. Joseph’s Foundation (TM).
The authors declare that the research was conducted in the absence of any commercial or financial relationships that could be construed as a potential conflict of interest.
All claims expressed in this article are solely those of the authors and do not necessarily represent those of their affiliated organizations, or those of the publisher, the editors and the reviewers. Any product that may be evaluated in this article, or claim that may be made by its manufacturer, is not guaranteed or endorsed by the publisher.
The authors would like to thank Kristine Nally and Billie Glasscock for their assistance in editing and submitting this manuscript.
1. Tkach M, Thery C. Communication by Extracellular Vesicles: Where We Are and Where We Need to Go. Cell (2016) 164(6):1226–32. doi: 10.1016/j.cell.2016.01.043
2. Mastoridis S, Martinez-Llordella M, Sanchez-Fueyo A. Extracellular Vesicles as Mediators of Alloimmunity and Their Therapeutic Potential in Liver Transplantation. World J Transpl (2020) 10(11):330–44. doi: 10.5500/wjt.v10.i11.330
3. Quaglia M, Dellepiane S, Guglielmetti G, Merlotti G, Castellano G, Cantaluppi V. Extracellular Vesicles as Mediators of Cellular Crosstalk Between Immune System and Kidney Graft. Front Immunol (2020) 11:74. doi: 10.3389/fimmu.2020.00074
4. Admyre C, Grunewald J, Thyberg J, Gripenback S, Tornling G, Eklund A, et al. Exosomes With Major Histocompatibility Complex Class II and Co-Stimulatory Molecules Are Present in Human BAL Fluid. Eur Respir J (2003) 22(4):578–83. doi: 10.1183/09031936.03.00041703
5. Gregson AL, Hoji A, Injean P, Poynter ST, Briones C, Palchevskiy V, et al. Altered Exosomal RNA Profiles in Bronchoalveolar Lavage From Lung Transplants With Acute Rejection. Am J Resp Crit Care Med (2015) 192(12):1490–503. doi: 10.1164/rccm.201503-0558OC
6. Gunasekaran M, Xu Z, Nayak DK, Sharma M, Hachem R, Walia R, et al. Donor-Derived Exosomes With Lung Self-Antigens in Human Lung Allograft Rejection. Am J Transpl (2017) 17(2):474–84. doi: 10.1111/ajt.13915
7. Liu Q, Rojas-Canales DM, Divito SJ, Shufesky WJ, Stolz DB, Erdos G, et al. Donor Dendritic Cell-Derived Exosomes Promote Allograft-Targeting Immune Response. J Clin Invest (2016) 126(8):2805–20. doi: 10.1172/JCI84577
8. Itoda Y, Sato M, Thuita L, Niikawa H, Ayyat KS, Okamoto T, et al. Impact for Survival and Chronic Lung Allograft Dysfunction of ISHLT Consensus of Antibody Mediated Rejection After Lung Transplantation. J Heart Lung Transpl (2019) 38(4, Supplement):S405. doi: 10.1016/j.healun.2019.01.1031
9. Bery AI, Hachem RR. Antibody-Mediated Rejection After Lung Transplantation. Ann Trans Med (2020) 8(6):411. doi: 10.21037/atm.2019.11.86
10. Witt CA, Gaut JP, Yusen RD, Byers DE, Iuppa JA, Bennett Bain K, et al. Acute Antibody-Mediated Rejection After Lung Transplantation. J Heart Lung Transplant Off Publ Int Soc Heart Transpl (2013) 32(10):1034–40. doi: 10.1016/j.healun.2013.07.004
11. Roux A, Levine DJ, Zeevi A, Hachem R, Halloran K, Halloran PF, et al. Banff Lung Report: Current Knowledge and Future Research Perspectives for Diagnosis and Treatment of Pulmonary Antibody-Mediated Rejection (AMR). Am J Transpl (2019) 19(1):21–31. doi: 10.1111/ajt.14990
12. Tissot A, Danger R, Claustre J, Magnan A, Brouard S. Early Identification of Chronic Lung Allograft Dysfunction: The Need of Biomarkers. Front Immunol (2019) 10:1681. doi: 10.3389/fimmu.2019.01681
13. Saini D, Weber J, Ramachandran S, Phelan D, Tiriveedhi V, Liu M, et al. Alloimmunity-Induced Autoimmunity as a Potential Mechanism in the Pathogenesis of Chronic Rejection of Human Lung Allografts. J Heart Lung Transpl (2011) 30(6):624–31. doi: 10.1016/j.healun.2011.01.708
14. Chiu S, Fernandez R, Subramanian V, Sun H, DeCamp MM, Kreisel D, et al. Lung Injury Combined With Loss of Regulatory T Cells Leads to De Novo Lung-Restricted Autoimmunity. J Immunol (2016) 197(1):51–7. doi: 10.4049/jimmunol.1502539
15. Akbarpour M, Wu Q, Liu X, Sun H, Lecuona E, Tomic R, et al. Clinical Relevance of Lung-Restricted Antibodies in Lung Transplantation. Hum Immunol (2019) 80(8):595–601. doi: 10.1016/j.humimm.2019.04.016
16. Kroshus TJ, Kshettry VR, Savik K, John R, Hertz MI, Bolman RM. Risk Factors for the Development of Bronchiolitis Obliterans Syndrome After Lung Transplantation. J Thorac Cardiovasc Surg (1997) 114(2):195–202. doi: 10.1016/S0022-5223(97)70144-2
17. Stanbrook MB, Kesten S. Bronchial Hyperreactivity After Lung Transplantation Predicts Early Bronchiolitis Obliterans. Am J Respir Crit Care Med (1999) 160(6):2034–9. doi: 10.1164/ajrccm.160.6.9801037
18. Salvadori M, Rosso G, Bertoni E. Update on Ischemia-Reperfusion Injury in Kidney Transplantation: Pathogenesis and Treatment. World J Transpl (2015) 5(2):52–67. doi: 10.5500/wjt.v5.i2.52
19. Ochando J, Ordikhani F, Boros P, Jordan S. The Innate Immune Response to Allotransplants: Mechanisms and Therapeutic Potentials. Cell Mol Immunol (2019) 16(4):350–6. doi: 10.1038/s41423-019-0216-2
20. Mori DN, Kreisel D, Fullerton JN, Gilroy DW, Goldstein DR. Inflammatory Triggers of Acute Rejection of Organ Allografts. Immunol Rev (2014) 258(1):132–44. doi: 10.1111/imr.12146
21. Menke J, Sollinger D, Schamberger B, Heemann U, Lutz J. The Effect of Ischemia/Reperfusion on the Kidney Graft. Curr Opin Organ Transpl (2014) 19(4):395–400. doi: 10.1097/MOT.0000000000000090
22. Ius F, Sommer W, Tudorache I, Kuhn C, Avsar M, Siemeni T, et al. Early Donor-Specific Antibodies in Lung Transplantation: Risk Factors and Impact on Survival. J Heart Lung Transpl (2014) 33(12):1255–63. doi: 10.1016/j.healun.2014.06.015
23. Tikkanen JM, Singer LG, Kim SJ, Li Y, Binnie M, Chaparro C, et al. De Novo DQ Donor-Specific Antibodies Are Associated With Chronic Lung Allograft Dysfunction After Lung Transplantation. Am J Respir Crit Care Med (2016) 194(5):596–606. doi: 10.1164/rccm.201509-1857OC
24. Chen-Yoshikawa TF. Ischemia-Reperfusion Injury in Lung Transplantation. Cells (2021) 10(6):1333. doi: 10.3390/cells10061333
25. Roux A, Bendib Le Lan I, Holifanjaniaina S, Thomas KA, Picard C, Grenet D, et al. Characteristics of Donor-Specific Antibodies Associated With Antibody-Mediated Rejection in Lung Transplantation. Front Med (Lausanne) (2017) 4:155. doi: 10.3389/fmed.2017.00155
26. Levine DJ, Glanville AR, Aboyoun C, Belperio J, Benden C, Berry GJ, et al. Antibody-Mediated Rejection of the Lung: A Consensus Report of the International Society for Heart and Lung Transplantation. J Heart Lung Transpl (2016) 35(4):397–406. doi: 10.1016/j.healun.2016.01.1223
27. Verleden SE, Vanaudenaerde BM, Emonds MP, Van Raemdonck DE, Neyrinck AP, Verleden GM, et al. Donor-Specific and -Nonspecific HLA Antibodies and Outcome Post Lung Transplantation. Eur Respir J (2017) 50(5):1701248. doi: 10.1183/13993003.01248-2017
28. Hachem RR, Kamoun M, Budev MM, Askar M, Ahya VN, Lee JC, et al. Human Leukocyte Antigens Antibodies After Lung Transplantation: Primary Results of the HALT Study. Am J Transpl (2018) 18(9):2285–94. doi: 10.1111/ajt.14893
29. Nickeleit V, Zeiler M, Gudat F, Thiel G, Mihatsch MJ. Histological Characteristics of Interstitial Renal Allograft Rejection. Kidney Blood Press Res (1998) 21(2-4):230–2. doi: 10.1159/000025862
30. Sun Q, Liu ZH, Cheng Z, Chen J, Ji S, Zeng C, et al. Treatment of Early Mixed Cellular and Humoral Renal Allograft Rejection With Tacrolimus and Mycophenolate Mofetil. Kidney Int (2007) 71(1):24–30. doi: 10.1038/sj.ki.5001870
31. Christakoudi S, Runglall M, Mobillo P, Tsui TL, Duff C, Domingo-Vila C, et al. Development of a Multivariable Gene-Expression Signature Targeting T-Cell-Mediated Rejection in Peripheral Blood of Kidney Transplant Recipients Validated in Cross-Sectional and Longitudinal Samples. EBioMedicine (2019) 41:571–83. doi: 10.1016/j.ebiom.2019.01.060
32. Eskandary F, Bond G, Kozakowski N, Regele H, Marinova L, Wahrmann M, et al. Diagnostic Contribution of Donor-Specific Antibody Characteristics to Uncover Late Silent Antibody-Mediated Rejection-Results of a Cross-Sectional Screening Study. Transplantation (2017) 101(3):631–41. doi: 10.1097/TP.0000000000001195
33. Bartel G, Regele H, Wahrmann M, Huttary N, Exner M, Horl WH, et al. Posttransplant HLA Alloreactivity in Stable Kidney Transplant Recipients-Incidences and Impact on Long-Term Allograft Outcomes. Am J Transpl (2008) 8(12):2652–60. doi: 10.1111/j.1600-6143.2008.02428.x
34. Schinstock CA, Cosio F, Cheungpasitporn W, Dadhania DM, Everly MJ, Samaniego-Picota MD, et al. The Value of Protocol Biopsies to Identify Patients With De Novo Donor-Specific Antibody at High Risk for Allograft Loss. Am J Transpl (2017) 17(6):1574–84. doi: 10.1111/ajt.14161
35. Zhang R. Donor-Specific Antibodies in Kidney Transplant Recipients. Clin J Am Soc Nephrol CJASN (2018) 13(1):182–92. doi: 10.2215/CJN.00700117
36. Ramachandran S, Subramanian V, Mohanakumar T. Immune Responses to Self-Antigens (Autoimmunity) in Allograft Rejection. Clin Transpl (2012), 261–72.
37. Smith JD, Banner NR, Hamour IM, Ozawa M, Goh A, Robinson D, et al. De Novo Donor HLA-Specific Antibodies After Heart Transplantation Are an Independent Predictor of Poor Patient Survival. Am J Transpl (2011) 11(2):312–9. doi: 10.1111/j.1600-6143.2010.03383.x
38. Smith MA, Sundaresan S, Mohanakumar T, Trulock EP, Lynch JP, Phelan DL, et al. Effect of Development of Antibodies to HLA and Cytomegalovirus Mismatch on Lung Transplantation Survival and Development of Bronchiolitis Obliterans Syndrome. J Thorac Cardiovasc Surg (1998) 116(5):812–20. doi: 10.1016/S0022-5223(98)00444-9
39. Goers TA, Ramachandran S, Aloush A, Trulock E, Patterson GA, Mohanakumar T. De Novo Production of K-Alpha 1 Tubulin-Specific Abs: Role in Chronic Lung Allograft Rejection. J Immunol (2008) 180(7):4487–94. doi: 10.4049/jimmunol.180.7.4487
40. Huang HJ, Yusen RD, Meyers BF, Walter MJ, Mohanakumar T, Patterson GA, et al. Late Primary Graft Dysfunction After Lung Transplantation and Bronchiolitis Obliterans Syndrome. Am J Transpl (2008) 8(11):2454–62. doi: 10.1111/j.1600-6143.2008.02389.x
41. Shaver CM, Ware LB. Primary Graft Dysfunction: Pathophysiology to Guide New Preventive Therapies. Expert Rev Respir Med (2017) 11(2):119–28. doi: 10.1080/17476348.2017.1280398
42. Wilkey BJ, Abrams BA. Mitigation of Primary Graft Dysfunction in Lung Transplantation: Current Understanding and Hopes for the Future. Semin Cardiothorac Vasc Anesth (2020) 24(1):54–66. doi: 10.1177/1089253219881980
43. Bharat A, Mohanakumar T. Immune Responses to Tissue-Restricted Nonmajor Histocompatibility Complex Antigens in Allograft Rejection. J Immunol Res (2017) 2017:8. doi: 10.1155/2017/6312514
44. Bharat A, Fields RC, Steward N, Trulock EP, Patterson GA, Mohanakumar T. CD4+25+ Regulatory T Cells Limit Th1-Autoimmunity by Inducing IL-10 Producing T Cells Following Human Lung Transplantation. Am J Transpl (2006) 6(8):1799–808. doi: 10.1111/j.1600-6143.2006.01383.x
45. Bharat AC, Chiu S, Zheng Z, Sun H, Yeldandi A, DeCamp MM, et al. Lung-Restricted Antibodies Mediate Primary Graft Dysfunction and Prevent Allotolerance After Murine Lung Transplantation. Am J Respir Cell Mol Biol (2016) 55(4):532–41. doi: 10.1165/rcmb.2016-0077OC
46. Kalache S, Dinavahi R, Pinney S, Mehrotra A, Cunningham MW, Heeger PS. Anticardiac Myosin Immunity and Chronic Allograft Vasculopathy in Heart Transplant Recipients. J Immunol (2011) 187(2):1023–30. doi: 10.4049/jimmunol.1004195
47. Nath DS, Basha IH, Tiriveedhi V, Alur C, Phelan D, Ewald GA, et al. Characterization of Immune Responses to Cardiac Self-Antigens Myosin and Vimentin in Human Cardiac Allograft Recipients With Antibody Mediated Rejection and Cardiac Allograft Vasculopathy. J Heart Lung Transpl (2010) 29(11):1277–85. doi: 10.1016/j.healun.2010.05.025
48. Cardinal H, Dieude M, Brassard N, Qi S, Patey N, Soulez M, et al. Antiperlecan Antibodies Are Novel Accelerators of Immune-Mediated Vascular Injury. Am J Transpl (2013) 13(4):861–74. doi: 10.1111/ajt.12168
49. Angaswamy N, Klein C, Tiriveedhi V, Gaut J, Anwar S, Rossi A, et al. Immune Responses to Collagen-IV and Fibronectin in Renal Transplant Recipients With Transplant Glomerulopathy. Am J Transpl (2014) 14(3):685–93. doi: 10.1111/ajt.12592
50. Nath DS, Angaswamy N, Basha HI, Phelan D, Moazami N, Ewald GA, et al. Donor-Specific Antibodies to Human Leukocyte Antigens Are Associated With and Precede Antibodies to Major Histocompatibility Complex Class I-Related Chain A in Antibody-Mediated Rejection and Cardiac Allograft Vasculopathy After Human Cardiac Transplantation. Hum Immunol (2010) 71(12):1191–6. doi: 10.1016/j.humimm.2010.09.012
51. Zou Y, Stastny P, Susal C, Dohler B, Opelz G. Antibodies Against MICA Antigens and Kidney-Transplant Rejection. N Engl J Med (2007) 357(13):1293–300. doi: 10.1056/NEJMoa067160
52. Angaswamy N, Saini D, Ramachandran S, Nath DS, Phelan D, Hachem R, et al. Development of Antibodies to Human Leukocyte Antigen Precedes Development of Antibodies to Major Histocompatibility Class I-Related Chain A and Are Significantly Associated With Development of Chronic Rejection After Human Lung Transplantation. Hum Immunol (2010) 71(6):560–5. doi: 10.1016/j.humimm.2010.02.021
53. Wallace WD, Li N, Andersen CB, Arrossi AV, Askar M, Berry GJ, et al. Banff Study of Pathologic Changes in Lung Allograft Biopsy Specimens With Donor-Specific Antibodies. J Heart Lung Transplant Off Publ Int Soc Heart Transpl (2016) 35(1):40–8. doi: 10.1016/j.healun.2015.08.021
54. Hachem RR, Yusen RD, Meyers BF, Aloush AA, Mohanakumar T, Patterson GA, et al. Anti-Human Leukocyte Antigen Antibodies and Preemptive Antibody-Directed Therapy After Lung Transplantation. J Heart Lung Transpl (2010) 29(9):973–80. doi: 10.1016/j.healun.2010.05.006
55. Hachem RR, Tiriveedhi V, Patterson GA, Aloush A, Trulock EP, Mohanakumar T. Antibodies to K-Alpha 1 Tubulin and Collagen V Are Associated With Chronic Rejection After Lung Transplantation. Am J Transpl (2012) 12(8):2164–71. doi: 10.1111/j.1600-6143.2012.04079.x
56. Jaramillo A, Smith MA, Phelan D, Sundaresan S, Trulock EP, Lynch JP, et al. Development of ELISA-Detected Anti-HLA Antibodies Precedes the Development of Bronchiolitis Obliterans Syndrome and Correlates With Progressive Decline in Pulmonary Function After Lung Transplantation. Transplantation (1999) 67(8):1155–61. doi: 10.1097/00007890-199904270-00012
57. Yoshida S, Haque A, Mizobuchi T, Iwata T, Chiyo M, Webb TJ, et al. Anti-Type V Collagen Lymphocytes That Express IL-17 and IL-23 Induce Rejection Pathology in Fresh and Well-Healed Lung Transplants. Am J Transpl (2006) 6(4):724–35. doi: 10.1111/j.1600-6143.2006.01236.x
58. Iwata T, Philipovskiy A, Fisher AJ, Presson RG Jr., Chiyo M, Lee J, et al. Anti-Type V Collagen Humoral Immunity in Lung Transplant Primary Graft Dysfunction. J Immunol (2008) 181(8):5738–47. doi: 10.4049/jimmunol.181.8.5738
59. Tiriveedhi V, Gautam B, Sarma N, Askar M, Budev M, Aloush A, et al. Pre-Transplant Antibodies to K-alpha-1 Tubulin and Collagen-V in Lung Transplantation: Clinical Correlations. J Heeart Lung Transplant (2013) 32(8):807–14. doi: 10.1016/j.healun.2013.06.003
60. Tiriveedhi V, Angaswamy N, Brand D, Weber J, Gelman AG, Hachem R, et al. A Shift in the Collagen V Antigenic Epitope Leads to T Helper Phenotype Switch and Immune Response to Self-Antigen Leading to Chronic Lung Allograft Rejection. Clin Exp Immunol (2012) 167(1):158–68. doi: 10.1111/j.1365-2249.2011.04486.x
61. Burlingham WJ, Love RB, Jankowska-Gan E, Haynes LD, Xu Q, Bobadilla JL, et al. IL-17-Dependent Cellular Immunity to Collagen Type V Predisposes to Obliterative Bronchiolitis in Human Lung Transplants. J Clin Invest (2007) 117(11):3498–506. doi: 10.1172/JCI28031
62. Keller MR, Haynes LD, Jankowska-Gan E, Sullivan JA, Agashe VV, Burlingham SR, et al. Epitope Analysis of the Collagen Type V-Specific T Cell Response in Lung Transplantation Reveals an HLA-DRB1*15 Bias in Both Recipient and Donor. PloS One (2013) 8(11):e79601. doi: 10.1371/journal.pone.0079601
63. Subramanian V, Ramachandran S, Banan B, Bharat A, Wang X, Benshoff N, et al. Immune Response to Tissue-Restricted Self-Antigens Induces Airway Inflammation and Fibrosis Following Murine Lung Transplantation. Am J Transpl (2014) 14(10):2359–66. doi: 10.1111/ajt.12908
64. Braun RK, Molitor-Dart M, Wigfield C, Xiang Z, Fain SB, Jankowska-Gan E, et al. Transfer of Tolerance to Collagen Type V Suppresses T-Helper-Cell-17 Lymphocyte-Mediated Acute Lung Transplant Rejection. Transplantation (2009) 88(12):1341–8. doi: 10.1097/TP.0b013e3181bcde7b
65. Mizobuchi T, Yasufuku K, Zheng Y, Haque MA, Heidler KM, Woods K, et al. Differential Expression of Smad7 Transcripts Identifies the CD4+CD45RChigh Regulatory T Cells That Mediate Type V Collagen-Induced Tolerance to Lung Allografts. J Immunol (2003) 171(3):1140–7. doi: 10.4049/jimmunol.171.3.1140
66. Fukami N, Ramachandran S, Takenaka M, Saini D, Weber J, Mohanakumar T. An Obligatory Role for Antibodies to Self-Antigens and Lung Infiltrating B Cells in the Immunopathogenesis of Obliterative Airway Disease Induced by Antibodies to MHC Class I Molecules. Am J Transpl (2012) 12(4):867–76. doi: 10.1111/j.1600-6143.2011.03917.x
67. Nayak DK, Zhou F, Xu M, Huang J, Tsuji M, Yu J, et al. Zbtb7a Induction in Alveolar Macrophages Is Obligatory in Anti-Human Leukocyte Antigen-Mediated Lung Allograft Rejection. Sci Trans Med (2017) 9(398):eaal1243. doi: 10.1126/scitranslmed.aal1243
68. Bharat A, Saini D, Steward N, Hachem R, Trulock EP, Patterson GA, et al. Antibodies to Self-Antigens Predispose to Primary Lung Allograft Dysfunction and Chronic Rejection. Ann Thorac Surg (2010) 90:(1094–101). doi: 10.1016/j.athoracsur.2010.06.009
69. Budding K, van de Graaf EA, Hoefnagel T, Kwakkel-van Erp JM, van Kessel DA, Dragun D, et al. Anti-ETAR and Anti-AT1R Autoantibodies Are Elevated in Patients With Endstage Cystic Fibrosis. J Cyst Fibros (2015) 14(1):42–5. doi: 10.1016/j.jcf.2014.07.007
70. Reinsmoen NL, Mirocha J, Ensor CR, Marrari M, Chaux G, Levine DJ, et al. A 3-Center Study Reveals New Insights Into the Impact of Non-HLA Antibodies on Lung Transplantation Outcome. Transplantation (2017) 101(6):1215–21. doi: 10.1097/TP.0000000000001389
71. Philogene MC, Johnson T, Vaught AJ, Zakaria S, Fedarko N. Antibodies Against Angiotensin II Type 1 and Endothelin A Receptors: Relevance and Pathogenicity. Hum Immunol (2019) 80(8):561–7. doi: 10.1016/j.humimm.2019.04.012
72. Njue F, Chih S. The Importance of Non-HLA Antibodies After Heart Transplant. Curr Transplant Rep (2019) 6(4):300–6. doi: 10.1007/s40472-019-00254-1
73. Zhang X, Reinsmoen NL. Impact of Non-Human Leukocyte Antigen-Specific Antibodies in Kidney and Heart Transplantation. Front Immunol (2017) 8:434. doi: 10.3389/fimmu.2017.00434
74. Hiemann NE, Meyer R, Wellnhofer E, Schoenemann C, Heidecke H, Lachmann N, et al. Non-HLA Antibodies Targeting Vascular Receptors Enhance Alloimmune Response and Microvasculopathy After Heart Transplantation. Transplantation (2012) 94(9):919–24. doi: 10.1097/TP.0b013e3182692ad2
75. Zhang X, Levine R, Patel JK, Kittleson M, Czer L, Kobashigawa JA. Association of Vimentin Antibody and Other Non-HLA Antibodies With Treated Antibody Mediated Rejection in Heart Transplant Recipients. Hum Immunol (2020) 81(12):671–4. doi: 10.1016/j.humimm.2020.09.003
76. Butler CL, Hickey MJ, Jiang N, Zheng Y, Gjertson D, Zhang Q, et al. Discovery of Non-HLA Antibodies Associated With Cardiac Allograft Rejection and Development and Validation of a Non-HLA Antigen Multiplex Panel: From Bench to Bedside. Am J Transpl (2020) 20(10):2768–80. doi: 10.1111/ajt.15863
77. Fedoseyeva EV, Zhang F, Orr PL, Levin D, Buncke HJ, Benichou G. De Novo Autoimmunity to Cardiac Myosin After Heart Transplantation and Its Contribution to the Rejection Process. J Immunol (1999) 162(11):6836–42.
78. Warraich RS, Pomerance A, Stanley A, Banner NR, Dunn MJ, Yacoub MH. Cardiac Myosin Autoantibodies and Acute Rejection After Heart Transplantation in Patients With Dilated Cardiomyopathy. Transplantation (2000) 69(8):1609–17. doi: 10.1097/00007890-200004270-00015
79. Morgun A, Shulzhenko N, Unterkircher CS, Diniz RV, Pereira AB, Silva MS, et al. Pre- and Post-Transplant Anti-Myosin and Anti-Heat Shock Protein Antibodies and Cardiac Transplant Outcome. J Heart Lung Transpl (2004) 23(2):204–9. doi: 10.1016/S1053-2498(03)00114-1
80. Azimzadeh AM, Pfeiffer S, Wu GS, Schroder C, Zhou H, Zorn GL 3rd, et al. Humoral Immunity to Vimentin Is Associated With Cardiac Allograft Injury in Nonhuman Primates. Am J Transpl (2005) 5(10):2349–59. doi: 10.1111/j.1600-6143.2005.01022.x
81. Mahesh B, Leong HS, Nair KS, McCormack A, Sarathchandra P, Rose ML. Autoimmunity to Vimentin Potentiates Graft Vasculopathy in Murine Cardiac Allografts. Transplantation (2010) 90(1):4–13. doi: 10.1097/TP.0b013e3181dfa694
82. Mahesh B, Leong HS, McCormack A, Sarathchandra P, Holder A, Rose ML. Autoantibodies to Vimentin Cause Accelerated Rejection of Cardiac Allografts. Am J Pathol (2007) 170(4):1415–27. doi: 10.2353/ajpath.2007.060728
83. Zhang Q, Reed EF. The Importance of Non-HLA Antibodies in Transplantation. Nat Rev Nephrol (2016) 12(8):484–95. doi: 10.1038/nrneph.2016.88
84. Hesemann LE, Subramanian V, Mohanakumar T, Dharnidharka VR. De Novo Development of Antibodies to Kidney-Associated Self-Antigens Angiotensin II Receptor Type I, Collagen IV, and Fibronectin Occurs at Early Time Points After Kidney Transplantation in Children. Pediatr Transpl (2015) 19(5):499–503. doi: 10.1111/petr.12531
85. Cailhier JF, Sirois I, Laplante P, Lepage S, Raymond MA, Brassard N, et al. Caspase-3 Activation Triggers Extracellular Cathepsin L Release and Endorepellin Proteolysis. J Biol Chem (2008) 283(40):27220–9. doi: 10.1074/jbc.M801164200
86. Soulez M, Pilon EA, Dieude M, Cardinal H, Brassard N, Qi S, et al. The Perlecan Fragment LG3 Is a Novel Regulator of Obliterative Remodeling Associated With Allograft Vascular Rejection. Circ Res (2012) 110(1):94–104. doi: 10.1161/CIRCRESAHA.111.250431
87. O'Riordan E, Orlova TN, Mendelev N, Patschan D, Kemp R, Chander PN, et al. Urinary Proteomic Analysis of Chronic Allograft Nephropathy. Proteomics Clin Appl (2008) 2(7-8):1025–35. doi: 10.1002/prca.200780137
88. Dieude M, Cardinal H, Hebert MJ. Injury Derived Autoimmunity: Anti-Perlecan/LG3 Antibodies in Transplantation. Hum Immunol (2019) 80(8):608–13. doi: 10.1016/j.humimm.2019.04.009
89. Yang B, Dieude M, Hamelin K, Henault-Rondeau M, Patey N, Turgeon J, et al. Anti-LG3 Antibodies Aggravate Renal Ischemia-Reperfusion Injury and Long-Term Renal Allograft Dysfunction. Am J Transpl (2016) 16(12):3416–29. doi: 10.1111/ajt.13866
90. Riesco L, Irure J, Rodrigo E, Guiral S, Ruiz JC, Gomez J, et al. Anti-Perlecan Antibodies and Acute Humoral Rejection in Hypersensitized Patients Without Forbidden HLA Specificities After Kidney Transplantation. Transpl Immunol (2019) 52:53–6. doi: 10.1016/j.trim.2018.11.002
91. Odermatt BF, Lang AB, Ruttner JR, Winterhalter KH, Trueb B. Monoclonal Antibodies to Human Type IV Collagen: Useful Reagents to Demonstrate the Heterotrimeric Nature of the Molecule. Proc Natl Acad Sci USA (1984) 81(23):7343–7. doi: 10.1073/pnas.81.23.7343
92. Bulow RD, Boor P. Extracellular Matrix in Kidney Fibrosis: More Than Just a Scaffold. J Histochem Cytochem (2019) 67(9):643–61. doi: 10.1369/0022155419849388
93. Utsumi K, Shimizu A, Yamato M, Tojimbara T, Nakajima I, Adachi E, et al. Alteration of Collagen IV in Acutely Deteriorated Renal Allografts. Transplantation (2001) 71(12):1757–65. doi: 10.1097/00007890-200106270-00010
94. Hirsch HH, Randhawa P. Practice ASTIDCo. BK Polyomavirus in Solid Organ Transplantation. Am J Transpl (2013) 13 Suppl 4:179–88. doi: 10.1111/ajt.12110
95. Sawinski D, Forde KA, Trofe-Clark J, Patel P, Olivera B, Goral S, et al. Persistent BK Viremia Does Not Increase Intermediate-Term Graft Loss But Is Associated With De Novo Donor-Specific Antibodies. J Am Soc Nephrol (2015) 26(4):966–75. doi: 10.1681/ASN.2014010119
96. Seifert ME, Gunasekaran M, Horwedel TA, Daloul R, Storch GA, Mohanakumar T, et al. Polyomavirus Reactivation and Immune Responses to Kidney-Specific Self-Antigens in Transplantation. J Am Soc Nephrol (2017) 28(4):1314–25. doi: 10.1681/ASN.2016030285
97. Dragun D, Muller DN, Brasen JH, Fritsche L, Nieminen-Kelha M, Dechend R, et al. Angiotensin II Type 1-Receptor Activating Antibodies in Renal-Allograft Rejection. N Engl J Med (2005) 352(6):558–69. doi: 10.1056/NEJMoa035717
98. Dragun D, Catar R, Philippe A. Non-HLA Antibodies Against Endothelial Targets Bridging Allo- and Autoimmunity. Kidney Int (2016) 90(2):280–8. doi: 10.1016/j.kint.2016.03.019
99. Philogene MC, Bagnasco S, Kraus ES, Montgomery RA, Dragun D, Leffell MS, et al. Anti-Angiotensin II Type 1 Receptor and Anti-Endothelial Cell Antibodies: A Cross-Sectional Analysis of Pathological Findings in Allograft Biopsies. Transplantation (2017) 101(3):608–15. doi: 10.1097/TP.0000000000001231
100. Reinsmoen NL, Lai CH, Heidecke H, Haas M, Cao K, Ong G, et al. Anti-Angiotensin Type 1 Receptor Antibodies Associated With Antibody Mediated Rejection in Donor HLA Antibody Negative Patients. Transplantation (2010) 90(12):1473–7. doi: 10.1097/TP.0b013e3181fd97f1
101. Banasik M, Boratynska M, Koscielska-Kasprzak K, Kaminska D, Zmonarski S, Mazanowska O, et al. Non-HLA Antibodies: Angiotensin II Type 1 Receptor (Anti-AT1R) and Endothelin-1 Type A Receptor (Anti-ETAR) Are Associated With Renal Allograft Injury and Graft Loss. Transplant Proc (2014) 46(8):2618–21. doi: 10.1016/j.transproceed.2014.09.029
102. Kuo E, Maruyama T, Fernandez F, Mohanakumar T. Molecular Mechanisms of Chronic Rejection Following Transplantation. Immunol Res (2005) 32(1-3):179–85. doi: 10.1385/IR:32:1-3:179
103. Xu Z, Nayak D, Yang W, Baskaran G, Ramachandran S, Sarma N, et al. Dysregulated microRNA Expression and Chronic Lung Allograft Rejection in Recipients With Antobidies to Donor HLA. Am J Transpl (2015) 15(7):1933–47. doi: 10.1111/ajt.13185
104. Mohanakumar T, Sharma M, Bansal S, Ravichandran R, Smith MA, Bremner RM. A Novel Mechanism for Immune Regulation After Human Lung Transplantation. J Thorac Cardiovasc Surg (2019) 157(5):2096–106. doi: 10.1016/j.jtcvs.2018.12.105
105. Bharat A, Kuo E, Saini D, Steward N, Hachem R, Trulock EP, et al. Respiratory Virus-Induced Dysregulation of T-Regulatory Cells Leads to Chronic Rejection. Ann Thorac Surg (2010) 90(5):1637–44; discussion 44. doi: 10.1016/j.athoracsur.2010.06.048
106. Almaghrabi RS, Omrani AS, Memish ZA. Cytomegalovirus Infection in Lung Transplant Recipients. Expert Rev Respir Med (2017) 11:377–83. doi: 10.1080/17476348.2017.1317596
107. Fisher CE, Mohanakumar T, Limaye AP. Respiratory Virus Infections and Chronic Lung Allograft Dysfunction: Assessment of Virology Determinants. J Heart Lung Transplant (2016) 35:946–7. doi: 10.1016/j.healun.2016.04.004
108. Gonzalez-Nolasco B, Wang M, Prunevieille A, Benichou G. Emerging Role of Exosomes in Allorecognition and Allograft Rejection. Curr Opin Organ Transpl (2018) 23(1):22–7. doi: 10.1097/MOT.0000000000000489
109. Marino J, Babiker-Mohamed MH, Crosby-Bertorini P, Paster JT, LeGuem C, Germana S, et al. Donor Exosomes Rather Than Passenger Leukocytes Initiate Alloreactive T Cell Responses After Transplantation. Sci Immunol (2016) 1(1):aaf8759. doi: 10.1126/sciimmunol.aaf8759
110. van der Pol E, Boing AN, Harrison P, Sturk A, Nieuwland R. Classification, Functions, and Clinical Relevance of Extracellular Vesicles. Pharmacol Rev (2012) 64(3):676–705. doi: 10.1124/pr.112.005983
111. Cocucci E, Racchetti G, Meldolesi J. Shedding Microvesicles: Artefacts No More. Trends Cell Biol (2009) 19(2):43–51. doi: 10.1016/j.tcb.2008.11.003
112. Mathivanan S, Ji H, Simpson RJ. Exosomes: Extracellular Organelles Important in Intercellular Communication. J Proteomics (2010) 73(10):1907–20. doi: 10.1016/j.jprot.2010.06.006
113. Ravichandran R, Bansal S, Rahman M, Sharma M, Liu W, Bharat A, et al. The Role of Donor-Derived Exosomes in Lung Allograft Rejection. Hum Immunol (2019) 80(8):588–94. doi: 10.1016/j.humimm.2019.03.012
114. Thery C, Witwer KW, Aikawa E, Alcaraz MJ, Anderson JD, Andriantsitohaina R, et al. Minimal Information for Studies of Extracellular Vesicles 2018 (MISEV2018): A Position Statement of the International Society for Extracellular Vesicles and Update of the MISEV2014 Guidelines. J Extracell Vesicles (2018) 7(1):1535750. doi: 10.1080/20013078.2018.1535750
115. Thery C, Ostrowski M, Segura E. Membrane Vesicles as Conveyors of Immune Responses. Nat Rev Immunol (2009) 9(8):581–93. doi: 10.1038/nri2567
116. Sharma M, Gunasekaran M, Ravichandran R, Fisher C, Limaye A, Hu C, et al. Circulating Exosomes With Lung Self-Antigens as a Biomarker for Chronic Lung Allograft Dysfunction: A Retrospective Analysis. J Heart Lung Transpl (2020) 39(11):1210–9. doi: 10.1016/j.healun.2020.07.001
117. Gunasekaran M, Bansal S, Ravichandran R, Sharma M, Perincheri S, Rodriguez F, et al. Respiratory Viral Infection in Lung Transplantation Induces Exosomes That Trigger Chronic Rejection. J Heart Lung Transplant (2020) 39(4):379–88. doi: 10.1016/j.healun.2019.12.009
118. De Toro J, Herschlik L, Waldner C, Mongini C. Emerging Roles of Exosomes in Normal and Pathological Conditions: New Insights for Diagnosis and Therapeutic Applications. Front Immunol (2015) 6:203. doi: 10.3389/fimmu.2015.00203
119. Hosseini HM, Fooladi AA, Nourani MR, Ghanezadeh F. The Role of Exosomes in Infectious Diseases. Inflamm Allergy Drug Target (2013) 12(1):29–37. doi: 10.2174/1871528111312010005
120. Zou J, Peng H, Liu Y. The Roles of Exosomes in Immunoregulation and Autoimmune Thyroid Diseases. Front Immunol (2021) 12:757674. doi: 10.3389/fimmu.2021.757674
121. Sharma M, Liu W, Perincheri S, Gunasekaran M, Mohanakumar T. Exosomes Expressing the Self-Antigens Myosin and Vimentin Play an Important Role in Syngeneic Cardiac Transplant Rejection Induced by Antibodies to Cardiac Myosin. Amer J Transpl (2018) 18:1626–35. doi: 10.1111/ajt.14650
122. Vallabhajosyula P, Korutla L, Habertheuer A, Yu M, Rostami S, Yuan CX, et al. Tissue-Specific Exosome Biomarkers for Noninvasively Monitoring Immunologic Rejection of Transplanted Tissue. J Clin Invest (2017) 127(4):1375–91. doi: 10.1172/JCI87993
123. Gunasekaran M, Sharma M, Hachem R, Bremner R, Smith MA, Mohanakumar T. Circulating Exosomes With Distinct Properties During Chronic Lung Allograft Rejection. J Immunol (2018) 200(8):2535–41. doi: 10.4049/jimmunol.1701587
124. Mimura T, Walker N, Aoki Y, Manning CM, Murdock BJ, Myers JL, et al. Local Origin of Mesenchymal Cells in a Murine Orthotopic Lung Transplantation Model of Bronchiolitis Obliterans. Am J Pathol (2015) 185(6):1564–74. doi: 10.1016/j.ajpath.2015.03.002
125. Ravichandran R, Itabashi Y, Liu W, Bansal S, Rahman M, Poulson C, et al. A Decline in Club Cell Secretory Proteins in Lung Transplantation Is Associated With Release of Natural Killer Cells Exosomes Leading to Chronic Rejection. J Heart Lung Transplant (2021) 40(12):1517–28. doi: 10.1016/j.healun.2021.08.016
126. Itabashi Y, Ravichandran R, Bansal S, Bharat A, Hachem R, Bremner R, et al. Decline in Club Cell Secretory Proteins, Exosomes Induction and Immune Responses to Lung Self-Antigens, Kalpha1 Tubulin and Collagen V, Leading to Chronic Rejection After Human Lung Transplantation. Transplantation (2020) 05(6):1337–46. doi: 10.1097/TP.0000000000003428
127. Lugini L, Cecchetti S, Huber V, Luciani F, Macchia G, Spadaro F, et al. Immune Surveillance Properties of Human NK Cell-Derived Exosomes. J Immunol (2012) 189(6):2833–42. doi: 10.4049/jimmunol.1101988
128. Bansal S, Arjuna A, Perincheri S, Poulson C, Bremner RM, Smith MA, et al. Restrictive Allograft Syndrome vs Bronchiolitis Obliterans Syndrome: Immunological and Molecular Characterization of Circulating Exosomes. J Heart Lung Transplant (2021) 41(1):24–33. doi: 10.1016/j.healun.2021.09.001
129. Dieude M, Turgeon J, Karakeussian Rimbaud A, Beillevaire D, Qi S, Patey N, et al. Extracellular Vesicles Derived From Injured Vascular Tissue Promote the Formation of Tertiary Lymphoid Structures in Vascular Allografts. Am J Transpl (2020) 20(3):726–38. doi: 10.1111/ajt.15707
130. Thaunat O, Graff-Dubois S, Fabien N, Duthey A, Attuil-Audenis V, Nicoletti A, et al. A Stepwise Breakdown of B-Cell Tolerance Occurs Within Renal Allografts During Chronic Rejection. Kidney Int (2012) 81(2):207–19. doi: 10.1038/ki.2011.317
131. Thaunat O, Patey N, Caligiuri G, Gautreau C, Mamani-Matsuda M, Mekki Y, et al. Chronic Rejection Triggers the Development of an Aggressive Intragraft Immune Response Through Recapitulation of Lymphoid Organogenesis. J Immunol (2010) 185(1):717–28. doi: 10.4049/jimmunol.0903589
132. Zeng F, Chen Z, Chen R, Shufesky WJ, Bandyopadhyay M, Camirand G, et al. Graft-Derived Extracellular Vesicles Transported Across Subcapsular Sinus Macrophages Elicit B Cell Alloimmunity After Transplantation. Sci Transl Med (2021) 13(585):eabb0122. doi: 10.1126/scitranslmed.abb0122
133. Zhou W, Zhang J, Marcus AI. LKB1 Tumor Suppressor: Therapeutic Opportunities Knock When LKB1 Is Inactivated. Genes Dis (2014) 1(1):64–74. doi: 10.1016/j.gendis.2014.06.002
134. Shaw RJ, Kosmatka M, Bardeesy N, Hurley RL, Witters LA, DePinho RA, et al. The Tmor Suppressor LKB1 Kinase Directly Activates AMP-Activated Kinase and Regulated Apoptosis in Response to Energy Stress. Proc Natl Acad Sci USA (2004) 101:3329–35. doi: 10.1073/pnas.0308061100
135. Herzig S, Shaw RJ. AMPK: Guardian of Metabolism and Mitochondrial Homeostasis. Nat Rev Mol Cell Biol (2018) 19(2):121–35. doi: 10.1038/nrm.2017.95
136. Shaw RJ, Kosmatka M, Bardeesy N, Hurley RL, Witters LA, DePinho RA, et al. The Tumor Suppressor LKB1 Kinase Directly Activates AMP-Activated Kinase and Regulates Apoptosis in Response to Energy Stress. Proc Natl Acad Sci USA (2004) 101(10):3329–35. doi: 10.1073/pnas.0308061100
137. Garcia D, Shaw RJ. AMPK: Mechanisms of Cellular Energy Sensing and Restoration of Metabolic Balance. Mol Cell (2017) 66(6):789–800. doi: 10.1016/j.molcel.2017.05.032
138. Mihaylova MM, Shaw RJ. The AMPK Signalling Pathway Coordinates Cell Growth, Autophagy and Metabolism. Nat Cell Biol (2011) 13(9):1016–23. doi: 10.1038/ncb2329
139. Chuang HC, Chou CC, Kulp SK, Chen CS. AMPK as a Potential Anticancer Target - Friend or Foe? Curr Pharm Des (2014) 20(15):2607–18. doi: 10.2174/13816128113199990485
140. Cunniff B, McKenzie AJ, Heintz NH, Howe AK. AMPK Activity Regulates Trafficking of Mitochondria to the Leading Edge During Cell Migration and Matrix Invasion. Mol Biol Cell (2016) 27(17):2662–74. doi: 10.1091/mbc.e16-05-0286
141. Saxena M, Balaji SA, Deshpande N, Ranganathan S, Pillai DM, Hindupur SK, et al. AMP-Activated Protein Kinase Promotes Epithelial-Mesenchymal Transition in Cancer Cells Through Twist1 Upregulation. J Cell Sci (2018) 131(14):jcs208314. doi: 10.1242/jcs.208314
142. Rout-Pitt N, Farrow N, Parsons D, Donnelley M. Epithelial Mesenchymal Transition (EMT): A Universal Process in Lung Diseases With Implications for Cystic Fibrosis Pathophysiology. Respir Res (2018) 19(1):136. doi: 10.1186/s12931-018-0834-8
143. Calles A, Sholl LM, Rodig SJ, Pelton AK, Hornick JL, Butaney M, et al. Immunohistochemical Loss of LKB1 Is a Biomarker for More Aggressive Biology in KRAS-Mutant Lung Adenocarcinoma. Clin Cancer Res (2015) 21(12):2851–60. doi: 10.1158/1078-0432.CCR-14-3112
144. Chen H, Liu H, Mao M, Tan Y, Mo X, Meng X, et al. Crosstalk Between Autophagy and Epithelial-Mesenchymal Transition and Its Application in Cancer Therapy. Mol Cancer (2019) 18(1):101. doi: 10.1186/s12943-019-1030-2
145. Thakur S, Viswanadhapalli S, Kopp JB, Shi Q, Barnes JL, Block K, et al. Activation of AMP-Activated Protein Kinase Prevents TGF-Beta1-Induced Epithelial-Mesenchymal Transition and Myofibroblast Activation. Am J Pathol (2015) 185(8):2168–80. doi: 10.1016/j.ajpath.2015.04.014
146. Rahman M, Ravichandran R, Bansal S, Sanborn K, Bowen S, Eschbacher J, et al. Novel Role for Tumor Suppressor Gene, Liver Kinase B1, in Epithelial Mesenchymal Transition Leading to Chronic Lung Allograft Dysfunction. Am J Transpl (2021) 22(3):843–52. doi: 10.1111/ajt.16903
147. Suarez H, Gamez-Valero A, Reyes R, Lopez-Martin S, Rodriguez MJ, Carrascosa JL, et al. A Bead-Assisted Flow Cytometry Method for the Semi-Quantitative Analysis of Extracellular Vesicles. Sci Rep (2017) 7(1):11271. doi: 10.1038/s41598-017-11249-2
148. Bansal S, Limaye AP, Lee J, Fleming T, Poulson C, Omar A, et al. Circulating Exosomes Induced by Respiratory Viral Infections in Lung Transplant Recipients Activate Cellular Stress, Innate Immune Pathways and Epithelial to Mesenchymal Transition. Transpl Immunol (2021) 69:101480. doi: 10.1016/j.trim.2021.101480
149. Bansal S, Perincheri S, Fleming T, Poulson C, Tiffany B, Bremner RM, et al. Cutting Edge: Circulating Exosomes With COVID Spike Protein Are Induced by BNT162b2 (Pfizer-BioNTech) Vaccination Prior to Development of Antibodies: A Novel Mechanism for Immune Activation by mRNA Vaccines. J Immunol (2021) 207(10):2405–10. doi: 10.4049/jimmunol.2100637
150. Tinel C, Lamarthee B, Callemeyn J, Van Loon E, Sauvaget V, Morin L, et al. Integrative Omics Analysis Unravels Microvascular Inflammation-Related Pathways in Kidney Allograft Biopsies. Front Immunol (2021) 12:738795. doi: 10.3389/fimmu.2021.738795
151. Anglicheau D, Sharma VK, Ding R, Hummel A, Snopkowski C, Dadhania D, et al. MicroRNA Expression Profiles Predictive of Human Renal Allograft Status. Proc Natl Acad Sci USA (2009) 106(13):5330–5. doi: 10.1073/pnas.0813121106
152. Soltaninejad E, Nicknam MH, Nafar M, Ahmadpoor P, Pourrezagholi F, Sharbafi MH, et al. Differential Expression of microRNAs in Renal Transplant Patients With Acute T-Cell Mediated Rejection. Transpl Immunol (2015) 33(1):1–6. doi: 10.1016/j.trim.2015.05.002
153. Xu Z, Nayak D, Yang W, Baskaran G, Ramachandran S, Sarma N, et al. Dysregulated MicroRNA Expression and Chronic Lung Allograft Rejection in Recipients With Antibodies to Donor HLA. Am J Transpl (2015) 15(7):1933–47. doi: 10.1111/ajt.13185
154. Xu Z, Yang W, Steward N, Sweet SC, Danziger-Isakov L, Heeger PS, et al. Role of Circulating MicroRNAs in the Immunopathogenesis of Rejection After Pediatric Lung Transplantation. Transplantation (2017) 101(10):2461–8. doi: 10.1097/TP.0000000000001595
155. van den Akker EK, Dor FJ JN, de Bruin RW. MicroRNAs in Kidney Transplantation: Living Up to Their Expectations? J Transplant (2015) 2015:354826. doi: 10.1155/2015/354826
156. Franco-Acevedo A, Melo Z, Echavarria R. Diagnostic, Prognostic, and Therapeutic Value of Non-Coding RNA Expression Profiles in Renal Transplantation. Diagnostics (Basel) (2020) 10(2):60. doi: 10.3390/diagnostics10020060
157. Li F, Qian W, Quan X, Yang H, Zhao G, Wei L. Differential MicroRNA Expressions in Human Peripheral Blood Mononuclear Cells Are Predictive of Renal Allograft Function. Transplant Proc (2019) 51(3):715–21. doi: 10.1016/j.transproceed.2019.01.051
158. Clancy JW, Zhang Y, Sheehan C, D'Souza-Schorey C. An ARF6-Exportin-5 Axis Delivers pre-miRNA Cargo to Tumour Microvesicles. Nat Cell Biol (2019) 21(7):856–66. doi: 10.1038/s41556-019-0345-y
159. Alexander M, Hu R, Runtsch MC, Kagele DA, Mosbruger TL, Tolmachova T, et al. Exosome-Delivered microRNAs Modulate the Inflammatory Response to Endotoxin. Nat Commun (2015) 6:7321. doi: 10.1038/ncomms8321
160. Vigorito E, Kohlhaas S, Lu D, Leyland R. miR-155: An Ancient Regulator of the Immune System. Immunol Rev (2013) 253(1):146–57. doi: 10.1111/imr.12057
161. Pelissier Vatter FA, Cioffi M, Hanna SJ, Castarede I, Caielli S, Pascual V, et al. Extracellular Vesicle- and Particle-Mediated Communication Shapes Innate and Adaptive Immune Responses. J Exp Med (2021) 218(8):e20202579. doi: 10.1084/jem.20202579
162. Golebiewska JE, Wardowska A, Pietrowska M, Wojakowska A, Debska-Slizien A. Small Extracellular Vesicles in Transplant Rejection. Cells (2021) 10(11):2989. doi: 10.3390/cells10112989
163. Montecalvo A, Larregina AT, Shufesky WJ, Stolz DB, Sullivan ML, Karlsson JM, et al. Mechanism of Transfer of Functional microRNAs Between Mouse Dendritic Cells via Exosomes. Blood (2012) 119(3):756–66. doi: 10.1182/blood-2011-02-338004
164. Bracamonte-Baran W, Florentin J, Zhou Y, Jankowska-Gan E, Haynes WJ, Zhong W, et al. Modification of Host Dendritic Cells by Microchimerism-Derived Extracellular Vesicles Generates Split Tolerance. Proc Natl Acad Sci USA (2017) 114(5):1099–104. doi: 10.1073/pnas.1618364114
165. Pang XL, Wang ZG, Liu L, Feng YH, Wang JX, Xie HC, et al. Immature Dendritic Cells Derived Exosomes Promotes Immune Tolerance by Regulating T Cell Differentiation in Renal Transplantation. Aging (Albany NY) (2019) 11(20):8911–24. doi: 10.18632/aging.102346
166. Tung SL, Boardman DA, Sen M, Letizia M, Peng Q, Cianci N, et al. Regulatory T Cell-Derived Extracellular Vesicles Modify Dendritic Cell Function. Sci Rep (2018) 8(1):6065. doi: 10.1038/s41598-018-24531-8
167. de Candia P, De Rosa V, Casiraghi M, Matarese G. Extracellular RNAs: A Secret Arm of Immune System Regulation. J Biol Chem (2016) 291(14):7221–8. doi: 10.1074/jbc.R115.708842
168. Lema DA, Burlingham WJ. Role of Exosomes in Tumour and Transplant Immune Regulation. Scand J Immunol (2019) 90(5):e12807. doi: 10.1111/sji.12807
169. Li X, Li JJ, Yang JY, Wang DS, Zhao W, Song WJ, et al. Tolerance Induction by Exosomes From Immature Dendritic Cells and Rapamycin in a Mouse Cardiac Allograft Model. PloS One (2012) 7(8):e44045. doi: 10.1371/journal.pone.0044045
170. Song J, Huang J, Chen X, Teng X, Song Z, Xing Y, et al. Donor-Derived Exosomes Induce Specific Regulatory T Cells to Suppress Immune Inflammation in the Allograft Heart. Sci Rep (2016) 7:20077. doi: 10.1038/srep20077
171. Antonioli L, Pacher P, Vizi ES, Hasko G. CD39 and CD73 in Immunity and Inflammation. Trends Mol Med (2013) 19(6):355–67. doi: 10.1016/j.molmed.2013.03.005
172. Silva-Vilches C, Ring S, Mahnke K. ATP and Its Metabolite Adenosine as Regulators of Dendritic Cell Activity. Front Immunol (2018) 9:2581. doi: 10.3389/fimmu.2018.02581
173. Poggio M, Hu T, Pai CC, Chu B, Belair CD, Chang A, et al. Suppression of Exosomal PD-L1 Induces Systemic Anti-Tumor Immunity and Memory. Cell (2019) 177(2):414–27 e13. doi: 10.1016/j.cell.2019.02.016
174. Smyth LA, Ratnasothy K, Tsang JY, Boardman D, Warley A, Lechler R, et al. CD73 Expression on Extracellular Vesicles Derived From CD4+ CD25+ Foxp3+ T Cells Contributes to Their Regulatory Function. Eur J Immunol (2013) 43(9):2430–40. doi: 10.1002/eji.201242909
175. Hong CS, Sharma P, Yerneni SS, Simms P, Jackson EK, Whiteside TL, et al. Circulating Exosomes Carrying an Immunosuppressive Cargo Interfere With Cellular Immunotherapy in Acute Myeloid Leukemia. Sci Rep (2017) 7(1):14684. doi: 10.1038/s41598-017-14661-w
176. Yu X, Huang C, Song B, Xiao Y, Fang M, Feng J, et al. CD4+CD25+ Regulatory T Cells-Derived Exosomes Prolonged Kidney Allograft Survival in a Rat Model. Cell Immunol (2013) 285(1-2):62–8. doi: 10.1016/j.cellimm.2013.06.010
177. Agarwal A, Fanelli G, Letizia M, Tung SL, Boardman D, Lechler R, et al. Regulatory T Cell-Derived Exosomes: Possible Therapeutic and Diagnostic Tools in Transplantation. Front Immunol (2014) 5:555. doi: 10.3389/fimmu.2014.00555
178. Aiello S, Rocchetta F, Longaretti L, Faravelli S, Todeschini M, Cassis L, et al. Extracellular Vesicles Derived From T Regulatory Cells Suppress T Cell Proliferation and Prolong Allograft Survival. Sci Rep (2017) 7(1):11518. doi: 10.1038/s41598-017-08617-3
179. Sullivan JA, Tomita Y, Jankowska-Gan E, Lema DA, Arvedson MP, Nair A, et al. Treg-Cell-Derived IL-35-Coated Extracellular Vesicles Promote Infectious Tolerance. Cell Rep (2020) 30(4):1039–51.e5. doi: 10.1016/j.celrep.2019.12.081
Keywords: extracellular vesicles, transplantation, immune responses, tissue-associated self-antigens, antibodies
Citation: Ravichandran R, Bansal S, Rahman M, Sureshbabu A, Sankpal N, Fleming T, Bharat A and Mohanakumar T (2022) Extracellular Vesicles Mediate Immune Responses to Tissue-Associated Self-Antigens: Role in Solid Organ Transplantations. Front. Immunol. 13:861583. doi: 10.3389/fimmu.2022.861583
Received: 24 January 2022; Accepted: 28 March 2022;
Published: 27 April 2022.
Edited by:
Mélanie Dieudé, Université de Montréal, CanadaReviewed by:
Dany Anglicheau, U1151 Institut Necker Enfants Malades (INSERM), FranceCopyright © 2022 Ravichandran, Bansal, Rahman, Sureshbabu, Sankpal, Fleming, Bharat and Mohanakumar. This is an open-access article distributed under the terms of the Creative Commons Attribution License (CC BY). The use, distribution or reproduction in other forums is permitted, provided the original author(s) and the copyright owner(s) are credited and that the original publication in this journal is cited, in accordance with accepted academic practice. No use, distribution or reproduction is permitted which does not comply with these terms.
*Correspondence: Thalachallour Mohanakumar, dG0ua3VtYXJAZGlnbml0eWhlYWx0aC5vcmc=
†These authors have contributed equally to this work
Disclaimer: All claims expressed in this article are solely those of the authors and do not necessarily represent those of their affiliated organizations, or those of the publisher, the editors and the reviewers. Any product that may be evaluated in this article or claim that may be made by its manufacturer is not guaranteed or endorsed by the publisher.
Research integrity at Frontiers
Learn more about the work of our research integrity team to safeguard the quality of each article we publish.