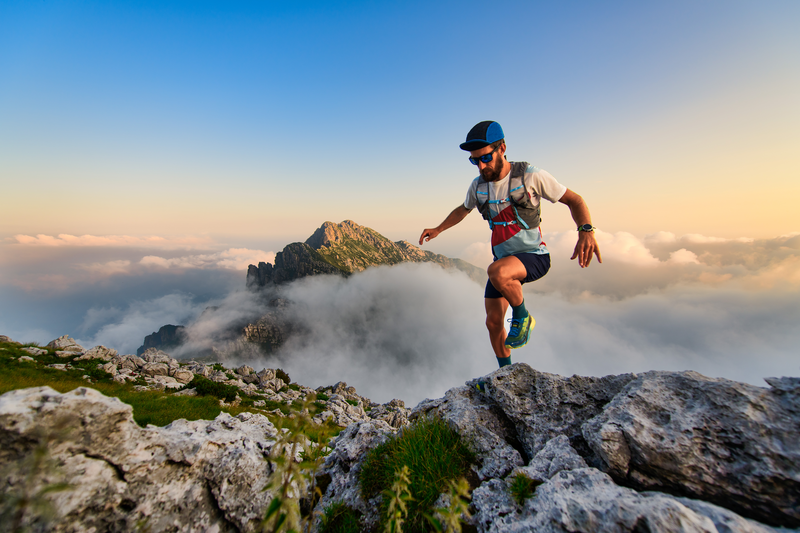
94% of researchers rate our articles as excellent or good
Learn more about the work of our research integrity team to safeguard the quality of each article we publish.
Find out more
ORIGINAL RESEARCH article
Front. Immunol. , 21 March 2022
Sec. Nutritional Immunology
Volume 13 - 2022 | https://doi.org/10.3389/fimmu.2022.860889
This article is part of the Research Topic Dietary Polyphenols for Improving Gut Health, volume II View all 14 articles
In aged animals, the physiological functions of the gastrointestinal tract (GIT) are reduced. Dietary intervention is necessary to re-activate GIT functions. The objective of this study was to investigate the impacts of dietary combination of quercetin (Q) and vitamin E (VE) on the intestinal structure and barrier integrity in aged breeder chickens. A sum of 400 (65-wks-old) Tianfu breeder hens were randomly allotted into four (4) groups with four (4) replicates, and fed with basal diet; basal diet supplemented with 0.4g/kg of Q; basal diet supplemented with 0.2g/kg of VE; and basal diet supplemented with the combination of Q (0.4 g/kg) and VE (0.2 g/kg) for 14 weeks. At the end of the 14th week, serum and gut segments were collected from eight hens per group for analyses. The results showed that Q+VE exerted synergistic effects on intestinal morphology by promoting villi height and crypt depth (P < 0.05), as well as mitigated the intestinal inflammatory damage of the aged hens, but decreased the concentration of serum D-lactate and diamine oxidase; and increased the levels of secretory immunoglobulin A (sIgA) and Mucin-2 mRNA (P < 0.05). Furthermore, the mRNA expression of intestinal tight junction proteins including occludin, ZO1, and claudin-1 was increased by Q+VE (P < 0.05). Moreover, Q+VE decreased the mRNA expression of the pro-inflammatory genes (TNF-α, IL-6, and IL-1β), and increased the expression of anti-inflammatory genes (IL-10 and IL-4) (P < 0.05). These results were consistent with the mRNA expression of Bax and Bcl-2. In addition, Q+VE protected the small intestinal tract from oxidative damage by increasing the levels of superoxide dismutase, total antioxidant capacity, glutathione peroxidase, catalase (P < 0.05), and the mRNA expression of SOD1 and GPx-2. However, Q+VE decreased malondialdehyde levels in the intestine compared to the control (P < 0.05). These results indicated that dietary Q+VE improved intestinal function in aged breeder hens, by protecting the intestinal structure and integrity. Therefore, Q+VE could act as an anti-aging agent to elevate the physiological functions of the small intestine in chickens.
The gastrointestinal tract (GIT) is the largest immunological organ in both animals and humans (1). The gut barrier is made up of several layers which consist of both external anatomical barrier and internal functional immunological barrier systems (2). In addition, tight junction proteins, including occludin, zona occludin-1 and claudins are critical for improving epithelial cell barrier immune functions (3–5). Moreover, the intestinal epithelial barrier interacts with the intestinal components, gut microbiome and immune cells to promote gut immunity (1). However, imbalances in the intestinal microenvironment and other factor such as age could cause disruption in the GIT of animals and humans (3), by reducing the concentration of tight junction proteins, hence, resulting in gut permeability (‘‘leaky gut’’) (1, 1, 6, 7) and several gut disorders, including intestinal inflammatory and autoimmune disorders (4, 8–10). In chickens, oxidative stress is a major factor that disrupts gut structure and integrity (11, 12), because the gut epithelium is prone to oxidative damage (12, 13).
The intestinal antioxidant capacity, gut microbiota and metabolite profile, motility in the intestinal tract regions, GIT transit time and pH, epithelial cell immune function (1, 14), cytokines (15), and intestinal mucus production (16, 17) depend on age and geography, which are influenced by diet (1). Moreover, during aging, there is a high occurrence of bacterial invasion of the intestinal epithelium in animals, thereby disrupting the intestinal barrier function (1, 18), leading to the emergence of gut disorders. Therefore, the intestinal structure and immune barrier integrity are correlated with age and diet (16, 19–21). Moreover, apoptosis of enterocytes and up-regulation of pro-inflammatory cytokines affects the integrity of the intestinal barrier, thereby causing intestinal permeability and hence, reduce the immunoregulatory roles of the intestinal barrier (22, 23). Aschoff et al. (24) reported that enterocytes towards the apex of the villi become increasingly susceptible to apoptosis, and found that Bax expression is related to intestinal cell damage (24). hence, alleviating excessive apoptosis and inflammation in the intestine of animals may promote the integrity of the intestinal barrier.
Therefore, a rapid development of dietary supplements to improve gut function and homeostasis in aged animals is imminent (25, 26). Vitamin E (VE) is a powerful dietary supplement characterized with a strong antioxidant property that attenuates the production and accumulation of reactive oxygen species (ROS) to prevent tissue damage caused by oxidative stress in chickens (27, 28). It is involved in muscular activity, tissue integrity and immune response by hindering the production of reactive oxygen species (29–33). Studies have shown that VE supplementation improves intestinal structure and mitigates intestinal inflammation in chickens (26). Moreover, VE has a synergistic effect with other dietary supplements such as flavonoids (27, 28, 34), selenium (35), and alpha lipoic acid (26, 36) to enhance antioxidant activity in animals. Vitamin E (alpha- and gamma-tocopherol) supplementation was reported to mitigate colitis, as well as protect the intestinal barrier function in mice by inhibiting colitis-induced loss of the tight junction protein occludin, and mitigates TNF-α/IFN-γ-induced impairment of trans-epithelial electrical resistance in human intestinal epithelial Caco-2 cell monolayer (37). In addition, supplementing vitamin E reduces phoxim (organophosphate pesticides) toxicity in rat intestinal tissues by alleviating phoxim-induced toxic effects on the intestinal oxidative stress, barrier function, and morphological changes (38). Furthermore, a study by Cadir et al. (39) showed that the supplementation of omeprazole and/or vitamin E exerts protective effects on the biochemical and histopathological intestinal damage induced by hypoxia/reoxygenation in newborn rats (39).
Dietary flavonoids such as catechin, resveratrol, rutin, and quercetin (Q) are polyphenolic compounds which are universally present in plants, and play major roles in chicken performance (40–42). Quercetin is a polyphenol belonging to the class of flavonoids obtained from fruits, vegetables, and beverages (27, 43). It is a potent antioxidant that enhances intestinal barrier function. Reports have indicated that quercetin promotes the concentration of tight junction proteins including claudin-1, claudin-4, occludin, and Zona occludin-1 (44, 45), thereby promoting barrier function and reducing inflammation. Furthermore, quercetin enhances barrier integrity in Caco-2 cells by inducing remodeling of epithelial tight junctions, as well as protects gastric mucosa against ulcerogenic agents (44). Yan et al. (46) reported that quercetin exhibits protective effect on indomethacininduced gastric mucosal injury in rats, by inducing mucus secretion (46). Quercetin also attenuates the effects of C. rodentium-induced colitis, inhibit the production of pro-inflammatory cytokines, as well as upregulates the expression of anti-inflammatory cytokines in the colon (47). In a high-fat-diet-fed mice model, quercetin exerts protective effect against immune/inflammatory responses and oxidative stress, and decreased intestinal lipid levels by attenuating atherosclerotic lesions (48). Moreover, in broiler chickens, quercetin supplementation alleviates oxidative stress and mitochondria damage induced by lipopolysaccharide (LPS)-induce via MAPK/Nrf2 signaling in the intestine, thereby increases the villus height and crypt depth in the duodenum, jejunum, and ileum (49). In addition, quercetin improves the intestinal health by decreasing serum endotoxin levels, reduces the intestinal ROS production, and increases the jejunal villi height and upregulated the mRNA expression of occludin and zonula occudens-1 in the jejunum of finishing pigs (50), as well as attenuates colon damage by upregulating the expression of Muc2 and ZO-1 in C57BL/6J mice (51).
Reports indicated that quercetin supplementation increased the intestinal villi length, as well as enhanced the mucosal thickness of the intestine (52), and restored barrier function in antibiotic-treated mice through decreasing the expression of serum biomarkers such as D-lactic acid and serum diamine oxidase levels (53).
Dong et al. (54) reported that quercetin supplementation upregulated the mRNA expression of tight junction proteins (such as tight junction protein 1) and Mucin-2 in chickens (54). These studies have provided the basic evidence for the role of quercetin as an important supplement that can promote intestinal barriers function in animals. Becker et al. (34) reported that quercetin has a strong synergistic effect with other dietary supplements such as rutin and α-tocopherol without any detrimental effects (34). Moreover, quercetin has also been found to have interactive effects with dietary supplements including catechin (55), citrulline (25), and resveratrol (40) to promote physiological functions in animals.
However, to the best of our knowledge, no studies have reported the interactive effects of quercetin and vitamin E on gut function in aged animals. Hence, the aim of this current study was to determine the impacts of dietary quercetin and vitamin E, supplemented independently and in combination on the intestinal structure and immune barrier integrity of aged breeder hens.
A total of 400 Tianfu Breeder Hens (65 weeks old) obtained from the Chicken Breeding Unit, Sichuan Agricultural University (the characteristics of this chicken breed were described in our previous study (27) were randomly allotted into 4 treatments containing 100 birds each, with 4 replicates of 25 chickens each. The birds were housed in individual wire cages (width: 48.8 cm, depth: 38.1 cm, height: 38.1 cm) and the lighting system was controlled (16 h light per day) and optimal ventilation was maintained throughout the experiment. Quercetin (95%, High-Performance Liquid Chromatography (HPLC) and Vitamin E were supplied by Shaanxi Huike Plant Development Co., Ltd. (Xian, Shaanxi, China). We determined the purity (95%) of the quercetin using HPLC. The chickens were fed a basal diet (control group); basal diet supplemented with 0.4 g/kg quercetin powder (quercetin group); basal diet supplemented with 0.2 g/kg vitamin E (vitamin E group); and basal diet supplemented with the combination of 0.4 g/kg quercetin and 0.2 g/kg vitamin E (Q + VE group). The recommended levels of vitamin E and quercetin inclusion were chosen based on previous studies (56) and (43, 57), respectively. Throughout the experimental period, the hens were given 120 g feed/day/hen, under a photoperiod of 16L:8D, and water was provided ad libitum. The ingredient composition in percentages and the calculated nutritional values of the basal diet fed the aged hens have been reported (27). The feed intake and body weight of the birds were measured weekly throughout the experimental period. The average body weight and average daily feed intake were calculated.
The experiment lasted for 14 weeks, and at the end of the 14th week, we randomly selected 2 birds per replicate (8 birds per treatment, totaling 32) whose blood samples (5 mL) were collected via the wing vein. The blood samples were centrifuged at 3,000 rpm for 10 min at 4°C to obtain the serum, and then stored at -800C for further analyses. Subsequently, the selected chickens were euthanized, their viscera were excised, the intestine was discretely separated and the adherent materials were precisely removed. The intestinal segmental samples (duodenum, jejunum, and ileum: thus, the duodenum from the ventriculus to the pancreatobiliary ducts; jejunum from pancreo-biliary ducts to yolk stalk; and ileum from yolk stalk to ileocecal junction) were collected, and after squeezing out the contents, the remaining small intestine was cut and the mucosa was gently scraped using a clean glass slide, immediately frozen in liquid nitrogen, and stored at -80°C for subsequent RNA extraction and qRT-PCR analysis. Moreover, parts of the intestinal mucosa (duodenal, jejunal, ileal mucosa) were stored at -20°C for subsequent biochemical analysis. The middle portions of the three sections of the small intestine (duodenum, jejunum, and ileum) were collected, washed in PBS, and fixed in 4% paraformaldehyde for morphological analysis.
The intestinal mucosa obtained from the, duodenal, jejunal, and ileal tissues were homogenized in pre-cold 0.9% saline and centrifuged at 3,000 rpm for approximately 10 min at 4°C to obtain the supernatant. Later, the supernatant was stored at -20°C for biochemical analysis. In addition, the protein concentration in the supernatant was determined using a Total Protein Assay kit according to the manufacturer’s instructions (Nanjing Jiancheng Bioengineering Institute, Nanjing, China). Below are the parameters measured in the serum and intestinal mucosa (duodenal, jejunal, and ileal) tissue supernatants.
The activity and concentration of the serum diamine oxidase (DAO) and D-lactate were determined using the enzyme-linked immunosorbent assay (ELISA) kits following the manufacturer’s instructions (Baolai Biotechnology Co., Ltd., Yancheng, China).
The activities of superoxide dismutase (SOD), total antioxidant capacity (TAOC), glutathione peroxidase (GPx), catalase (CAT), and malondialdehyde (MDA) in the intestinal mucosa supernatants and plasma were determined using commercial biochemistry kits according the instructions provided by the manufacturer (Nanjing Jiancheng Bioengineering Institute, Nanjing, China). The parameters (TAOC, CAT, GPx, and SOD levels) determined in the tissue (intestinal) homogenates were expressed as units per milligram of protein, whereas the levels of the MDA was expressed as nanomoles per milligram of protein.
The expression levels of cytokines such as IL-1β, IL-6, IL-4, and IL-10, were measured in the supernatants of the intestinal mucosa (ileum, duodenum, and jejunum) and plasma using commercial biochemistry kits following the protocols provided by the manufacturer (Nanjing Jiancheng Bioengineering Institute, Nanjing, China). Moreover, the concentration of secretory IgA (sIgA) was determined in the intestinal mucosa (duodenum, jejunum, and ileum) using ELISA kits according to the guide provided by the manufacturer (Baolai Biotechnology Co., Ltd., Yancheng, China).
For morphological analysis, the duodenal, jejunal, and ileal tissue samples were stored in 4% paraformaldehyde for 48 h. Thereafter, we prepared the samples using paraffin embedding techniques. The sections (5µm) were stained using hematoxylin and eosin (HE) for morphological and structural observation. The dyed slices of the duodenum, jejunum, and ileum were used to examine the pathological status of the intestinal tissues under a light microscope (DP80Digital, Olympus, Tokyo, Japan) and images were captured. Furthermore, the morphological characteristics such as villus height (from the tip of the villus to the crypt opening/junction) and crypt depth (from the opening of the invagination to the base above the lamina muscularis mucosae, i.e., distance of the invagination between 2 adjacent villi) were measured using ImagePro Plus 6.0 software (Media Cybernetics). At least ten (10) views were selected from each intestinal sample for measurement (thus, 10 separate well-oriented villi and crypts were measured per slide), and the average of each index was recorded. Furthermore, we grade (score) the degree of duodenum, jejunum, and ileum tissue damage in accordance with the degree of inflammation described in previous studies (58, 59). Thus, the severity of the histological inflammation were scored as follows: 0 = none; 1 = mild; 2 = moderate; and 3 = severe. In addition, the inflammatory cell infiltration were scored as: 0 = normal; 1 = mucosal; 2 = submucosal; and 3 = osmotic transmural expansion, whereas, the scores for the epithelial lesions were; 0 = complete; 1 = crypt structure deformation; 2 = erosion; and 3 = ulcers. Moreover, the grades for the extent of lesions were; 0 = none; 1 and 2 = multifocal; and 3 = spread, while, the scores for edema were 0 = none; 1 = mild mucosal; 2 = submucosal; and 3 = mucosal). In all, these scores were computed to determine the damage scores for the various intestinal tissues.
Total RNA was extracted from the intestinal (duodenum, jejunum, and ileum) mucosal scrapings following previously described procedures (27, 28, 60), using TRIzol reagent (Takara, Dalian, China), according to the manufacturer’s instructions. Then, the concentration and purity of the extracted RNA were determined using Nanodrop 2000C (Thermo Fisher Scientific, Waltham, MA, USA) with an absorbance ratio of A260/280. Thereafter, the PrimeScript RT Reagent Kit (Takara, Dalian, China) was used to synthesize the singlestrand cDNA following the protocols provided by the manufacturer. Thereafter, we used the single-strand cDNA for the qRT-PCR analysis using the CFX96 Real-Time System (Bio-Rad, Hercules, CA, USA) under favorable conditions such as: 95°C for 3 min, 40 cycles of 95°C for 10 s and annealing temperature (Table 1) for 20 s, which was followed by a final extension at 72°C for 20 s, with a melt curve analysis performed at 65~95°C. The amplification efficiencies of the target genes ranged from 95% to 105%. We have performed each qRT-PCR reaction with the volumes of 15 µL containing 6.25 µL TB Green TM Premix (Takara), 0.3 µL forward and reverse primers, 1.5 µL cDNA, and 6.65 µL DNase/RNase-Free Deionized Water (Tiangen, Beijing, China). The samples were run in triplicate, and the expression level of β-actin was used to normalize the cycle threshold (Ct) values. The relative abundance of each transcript was normalized to that of β-actin. Gene expression was calculated using the 2−∆∆Ct method (61).
All data were analyzed by one-way analysis of variance (ANOVA) using GraphPad Prism version 6.01 statistical package for Windows (GraphPad Software Inc., San Diego, CA) and SPSS 20 Statistical Analysis Software (SPSS Inc., Chicago, IL, USA). Therefore, all the experimental data are indicated as the mean ± standard deviation (SD), and Tukey’s test was used to determine the differences among the treatment groups. Calculated Δ Ct (corrected sample) = mean value of target gene - mean value of internal reference gene, ΔΔ Ct = Δ Ct-mean value of control group. Values were significantly different at P < 0.05.
In this study, we observed the average daily feed intake and body weight per hen after 14 weeks of the experimental period. The feed intake recorded for the aged laying hens was significantly improved by the combination of Q + VE which is similar to that recorded in the VE group but differed from the Q and control groups (Figure 1A, P < 0.05). In addition, the body weight of the Q + VE group was significantly higher than those of the control group (Figure 1B, P < 0.05).
Figure 1 Effects of quercetin (Q), vitamin E (VE), and Q + VE on feed intake and body weight of aged laying hens fed 14 weeks. Bars without the same letter differed significantly (P < 0.05). (A) Feed intake; (B) body weight.
As shown in Figure 2, the concentration of serum diamine oxidase (DAO) in the Q + VE group was lower than that in the control and VE groups (Figure 2A, P < 0.05). In addition, the serum D-lactate (D-LA) level was significantly lower in the Q + VE group than in the other groups (Figure 2B, P<0.05). The Q and VE groups were significantly different from those of the control group (Figure 2B, P < 0.05). These results indicated that the combination of Q + VE had a synergistic effect on the intestinal barrier biomarker indices of aged breeder hens.
Figure 2 Effects of quercetin (Q), vitamin E (VE), and Q + VE on the activities of serum diamine oxidase (DAO) and D-lactate (D-LA) (intestinal biomarkers) after 14 weeks experimental feeding. Bars without the same letter differed significantly (P < 0.05). (A) serum DAO; (B) D-LA.
Table 2 summarizes the antioxidant indices in the intestinal segments (duodenum, jejunum, and ileum) and serum. The concentration of SOD in the serum was significantly higher in the Q + VE group than in the other groups (P < 0.05). In addition, there was a significant difference in the levels of SOD in the duodenum between the Q + VE and control groups. Meanwhile, the SOD level in the jejunum of the Q + VE group was significantly higher than those in the control and VE groups (P < 0.05). Interestingly, the ileal SOD level in the Q + VE group was significantly higher than that in the other groups (P < 0.05). Furthermore, the TAOC level in the serum of the Q + VE group was also higher than those in the other groups (Table 2; P < 0.05). The TAOC concentration in the duodenum was significantly different between the Q + VE and control groups (P < 0.05). In addition, serum GPx was significantly improved in the Q and Q + VE groups compared to the control group (p < 0.05). Again, the activity of GPx in the duodenum was increased by Q + VE compared with that in the control group (P < 0.05). Moreover, the level of GPx in the jejunum was improved by both the Q and Q + VE groups compared to the control and VE groups (P < 0.05). However, the Q + VE group had a higher concentration of GPx in the ileum than the other groups (P < 0.05). The CAT level in the serum was also increased by dietary Q + VE compared to the control and Q groups (P < 0.05). In the duodenum, CAT was significantly increased by the Q, VE, and Q + VE dietary treatments compared to the control group (P < 0.05). Moreover, CAT levels in the jejunum were significantly higher in the Q and Q + VE groups than in the control group (P < 0.05). Moreover, the Q + VE group was significantly higher than that of the control group (P < 0.05). Interestingly, the MDA levels in the serum, duodenum, jejunum, and ileum were significantly higher in the control group than in the other groups (P < 0.05).
Table 2 Effects of Q, VE, and Q + VE on antioxidant enzymes and MDA levels in the intestinal segments, and serum of aged breeder hens.
Table 3 summarizes the expression levels of pro-inflammatory (IL-6 and IL-1β) and anti-inflammatory cytokines (IL-10 and IL-4) in the intestinal mucosa and serum. The levels of pro-inflammatory cytokines (IL-6 and IL-1β) were significantly reduced in the Q + VE treatment group as compared with the control group (P < 0.05). However, in some instances, the individual Q and VE groups reduced the concentrations of these pro-inflammatory cytokines (IL-6 and IL-1β) in the intestinal mucosa and serum; for example; IL-1β was reduced in the three gut segments by the individual Q and VE. However, in some cases the individual Q and VE groups were similar to both the control and Q + VE groups (P > 0.05) across all the intestinal tissues (duodenum, jejunum, and ileum mucosa) and plasma tested. Moreover, the levels of the anti-inflammatory cytokines (IL-10 and IL-4) were highly elevated in the Q, VE, and Q + VE groups (with the highest level in the Q + VE group) compared with the control group (P < 0.05). This shows that the combination of Q + VE exerts synergistic effects in attenuating intestinal and systemic inflammation in aged breeder chickens.
Table 3 Effects of supplemental Q, VE, and Q + VE on pro- and anti-inflammatory cytokines in the intestinal mucosa and plasma of the aged breeder hens.
The activities of sIgA in the small intestinal mucosa are summarized in Table 4. The concentration of sIgA in the duodenum, jejunum, and ileum of the aged chickens was significantly increased in the Q, VE, and Q + VE groups compared to the control group (P < 0.05). However, in all small intestinal segments, sIgA levels were similar in the Q, VE, and Q + VE groups (P > 0.05).
Table 4 Effects of supplemental Q, VE, and Q + VE on the immune biomarker (sIgA) levels in the intestinal mucosa of the aged breeder hens.
As shown in Figure 3, the mRNA expression of the tight junction proteins [occludin, zona occludens 1 (ZO1), and Claudin 1] and barrier function (Mucin 2) related genes were significantly higher in the dietary supplement groups than in the control group (Figures 3A–D; P < 0.05). However, the combination group (Q + VE) showed an elevated concentration of occludin, ZO1, claudin 1, and Mucin 2 compared to the quercetin and vitamin E groups (Figures 3A–D; P < 0.05).
Figure 3 The impacts of quercetin (Q), vitamin E (VE), and Q + VE on the mRNA expression of the tight junction proteins and barrier function biomarker genes in the small intestinal mucosa of the aged breeder hens. mRNA expression of: (A) occludin; (B) claudin 1; (C) ZO1; and (D) Mucin 2 in the small intestinal mucosa. Bars without the same letter differed significantly (P < 0.05).
Figure 4 summarizes the relative mRNA expression of inflammation related cytokines in the intestinal mucosa. The levels of pro-inflammatory cytokines (TNF-α, IL-6, and IL-1β) were decreased in all the small intestinal segments due to the combinatory effects exerted by Q and VE as compared to all the other groups (Figures 4A–C; P < 0.05), whereas the combination of Q and VE significantly increased the levels of the anti-inflammatory cytokines (IL-10 and IL-4) in the mucosa of the duodenum, jejunum, and ileum as compared to the control group (Figures 4D, E; P < 0.05). Moreover, in most cases, levels of the pro- and anti-inflammatory cytokines in the control, quercetin, and vitamin E groups were similar (Figures 4A–E; P > 0.05).
Figure 4 Effects of Q, VE, and Q + VE on the mRNA expressions of pro- and anti-inflammation related cytokines (IL-6, IL-1β, and TFN-α; IL-10 and IL-4) related genes in the small intestinal mucosa of aged breeder hens. mRNA expressions of pro-inflammatory cytokines (A–C) and anti-inflammatory cytokines related genes (D, E). Bars without the same letter differed significantly (P < 0.05).
As shown in Figure 5, the dietary combination of Q + VE significantly increased the mRNA expression of the antioxidant related genes (SOD1 and GPx2) in the mucosa of the small intestine of the aged chickens as compared to the other groups (Figures 5A, B; P < 0.05). Furthermore, Q, VE, and Q + VE significantly increased the concentration of the anti-apoptotic gene (Bcl-2) and decreased the expression of Bax in the intestinal mucosa of the aged chickens compared to the control (Figures 5C, D; P < 0.05).
Figure 5 Effects of dietary Q, VE, and Q + VE on the mRNA expressions of antioxidant and apoptosis related genes in the small intestine mucosa of aged breeder hens. mRNA expressions of antioxidant related genes (A, B) and apoptosis related genes (C, D). Bars without the same letter differed significantly (P < 0.05).
As shown in Figure 6, the histomorphological results showed that the duodenum, jejunum, and ileum of the aged hens in the control group exhibited shortening villi and infiltration of inflammatory cells, characterized with lymphocytes neutrophils, and macrophages (Figure 6, Control). However, in the individual quercetin and vitamin E groups, there were mild lesions observed in the duodenal, jejunal, and ileal tissue samples of the aged hens (Figure 6, quercetin and vitamin E). Moreover, the combination of quercetin and vitamin E (Q + VE) mitigated the intestinal damage induced by aging (Figure 6, Q + VE).
Figure 6 Effect of Q, VE, and Q + VE on intestinal inflammation in aged breeder hens. (Control, Q, and VE) Effects of dietary Q, VE, and Q + VE on intestinal histology in the Control group; quercetin group; vitamin E group; and Q + VE groups.
In addition, the histopathological scores obtained were consistent with the histological evaluation (Figure 7; P < 0.05). These results showed that the dietary combination of Q + VE could synergistically prevent intestinal structure damage by attenuating intestinal inflammation in aged breeder hens.
Figure 7 Histopathological severity scores of the four (4) groups. Bars without the same letter differed significantly (P < 0.05). Scale bar = 20um.
The results presented in Table 5 show that the villi height (VH) and crypt depth (CD) of the duodenal, jejunal and ileal sections of the small intestine in the Q, VE, and Q + VE groups were significantly higher than those in the control group (P < 0.05). However, there was no significant difference observed among the individual Q, VE, and control groups across all the small intestinal segments (P > 0.05). Therefore, this result suggested that, the synergism of Q + VE could improve the structure of the duodenum, jejunum, and ileum of aged breeder hens.
Table 5 Duodenal, jejunal, and ileal morphological characteristics of aged breeder chickens fed with Q, VE, and Q + VE.
The benefits of the intestinal health in animal wellbeing and welfare is unmeasurable. The importance of gut health in animal welfare and wellbeing is undisputable because of its important physiological functions (25). The current study elucidated the synergistic effects of dietary supplementation of quercetin and vitamin E on the intestinal structure and barrier integrity of aged breeder hens to promote immune response, gut homeostasis, and intestinal health. The results obtained in this study showed that the combination of quercetin and vitamin E promoted the palatability of the diet which promoted feed intake. In aged animals, the integrity of the intestinal barrier is compromised by pathogens (62–64), and these pathogens release certain biomarkers such as D-Lactate into the blood after gut damage. Plasma biomarkers such as DAO and D-Lac have been used as indicators of intestinal mucosal mass and integrity. DAO is an intracellular enzyme abundant in the epithelium of the small intestine (65), whereas, D-Lac is a product of intestinal bacteria (66) released into the blood during villi injury. Therefore, DAO and D-Lac are biomarkers used to assess gut permeability and mucosal damage (65).
In this study, we found that the combination of quercetin and vitamin E synergistically decreased plasma DAO and D-Lactate levels, indicating that intestinal mucosal integrity was protected by the attenuation of intestinal permeability. This was consistent with the study by Batista et al. (53), who reported that quercetin treatment significantly decreased plasma DAO activity, D-Lactate, and endotoxin levels in mice (53).
The intestinal mucosa is the first line of defense against oxidative stress, because it is composed of an extensive enzyme and non-enzymatic antioxidant system. However, in aged animals, due to imbalances in the antioxidant system, the intestinal mucosa experiences oxidative stress (25, 54). Previous studies have shown that aged animals fed without dietary antioxidant supplements experience oxidative stress responses (26, 54).
In this study, the antioxidant capacity was improved by the combination of quercetin and vitamin E in the intestinal mucosa and serum of aged breeder hens. Similar studies have shown that individual quercetin and vitamin E supplementation prevented the production and accumulation of ROS induced by proinflammatory cytokines; thereby, alleviating oxidative stress and apoptosis in animals (25, 43, 54, 67, 68).
In poultry, intestinal health and function are widely evaluated based on the characteristics of the gut morphological parameters such as villus height, crypt depth, and ratio of villus height to crypt depth (69–72). Longer villi length, deeper crypts, and a higher ratio of villus height to crypt depth increase nutrient absorption capacity, due to the large surface area (72–75). Thus, the higher the villi height, the higher the surface area for nutrient absorption in the small intestine. This process increases the action of digestive enzymes, and speeds up nutrient transportation (73, 76). Furthermore, the intestinal crypts are invaginations of the epithelium around the villi, and are lined by epithelial cells that secrete enzymes. The base of the crypts constantly divides to maintain the structure of the villi, therefore, an increase in crypt depth would produce more developed villi (73, 77, 78). Deeper crypts alternatively indicate fast tissue turnover because various types of special cells are present in the crypt, including absorptive, secretory and regenerative cells (79). Moreover, reducing the crypt depths of the intestinal villi may lead to a reduction in the absorption of nutrients (76, 78, 80). In this study, the villi height and crypt depth of the duodenum, jejunum, and ileum were enhanced by the combination of quercetin and vitamin E, indicating an improved absorption capacity in aged chickens. This was consistent with previous studies, which reported that quercetin significantly increased the depth of intestinal glands in rats and chickens, thereby improving intestinal morphology (52, 54, 81). Similarly, Wang et al. (26) reported that, vitamin E in combination with alpha lipoic acid promoted intestinal morphological characteristics in chickens (26). Therefore, the current study showed that the combination of dietary combination of quercetin and vitamin E promoted the epithelial structure of aged chickens.
The intestinal mucosa is made up of the membrane-bound and secreted mucins including Mucin 2 (MUC2) (82). MUC2 is responsible for preventing the internal invasion of pathogens, toxins, and foreign materials. Quercetin was reported to promote the upregulation of MUC2 through the protein kinase C alpha/extracellular regulated protein kinases 1-2 pathway (54). A study by Damiano et al. (83) showed that quercetin induces mRNA levels of MUC2 and MUC5AC via PKCα/ERK1-2 pathway in human intestinal epithelial Caco-2 cells. Hence, it shows that quercetin exerts protective effects on the intestinal mucosal barrier through regulating molecular mechanism that maintain secretory function of the intestinal goblet cells and mucin levels in the enterocytes of human (83). In addition, sIgA, a predominant immunoglobulin in the mucosal system, protects the mucosal surface against toxins, viruses, and enteropathogens; as well as inhibit pathogens from binding to the mucosal surface of the intestine, thereby enhancing immunity (84, 85). The results obtained in this study showed that the combination of quercetin and vitamin E promotes sIgA levels and mRNA expression of MUC2 in the intestinal mucosa, thereby improving intestinal immunity.
Tight junction proteins including claudin 1, ZO1, and occludin promote normal functioning of the intestinal mucosa barrier. The expression of these proteins is downregulated by conditions such as “Leaky gut’’ or inflammation (86–89), therefore, tight junction proteins are crucial for determining paracellular permeability (90). The results of the present study showed that the combination of quercetin and vitamin E increased the mRNA expression of claudin 1, occludin, and ZO1 in the duodenum, jejunum, and ileum of aged chickens. This indicated that the combination dietary between quercetin and vitamin E exerted intestinal homeostasis and immunity in aged chickens. This was consistent with the results of Valenzano et al. (44), Suzuki and Hara (45), and Dong et al. (54).
Cytokines, are endogenous mediators of the immune system and are responsible for controlling the occurrence of inflammatory reactions (74, 91–93). The intestinal barrier regulates the passage of microorganisms, pro-inflammatory molecules, antigens, and toxins (94), however, age-related changes induce oxidative stress, which eventually increases pro-inflammatory cytokines (95), resulting in intestinal epithelial cell damage and cellular apoptosis (96–98).
Dietary antioxidants suppress pro-inflammatory enzymes, thereby attenuating intestinal inflammation (99). In this study, the combination of dietary quercetin and vitamin E reduced the expression of pro-inflammatory cytokines, increased the expression of anti-inflammatory cytokines, decreased apoptosis, and attenuated oxidative stress in the small intestinal structure, thereby promoting immunoregulation in aged breeder hens. The expression levels of the apoptotic gene (Bax) in this study was reduced significantly, whereas Bcl-2 was significantly increased in the intestinal tissues of the chickens fed the combination of dietary quercetin and vitamin E. These results are consistent with previous studies that reported that a balanced enzymatic antioxidant system attenuates oxidative stress and hence, alleviates apoptosis; also dietary antioxidants such as quercetin reduces apoptosis in birds (100–102). This could be attributed to the beneficial effects of quercetin and vitamin E on the gut histomorphological results obtained. This was consistent with previous studies by Uyanga et al. (25), Abedi et al. (68), Yang et al. (102) and Shu et al. (42) who reported that dietary antioxidant supplementation improves immunity and antioxidant capacity in animals. Moreover, studies by Wang et al. (26) and Lewis et al. (103) showed that vitamin E supplementation positively mitigates intestinal inflammation and improves nutrient transport in chickens and mice, by suppressing pro-inflammatory cytokines and prostaglandin E2 (26, 103).
In summary, the synergy between Quercetin and Vitamin E attenuated age-induced intestinal permeability, inflammation, and oxidative stress by promoting the activities of intestinal antioxidant biomarkers, anti-inflammatory cytokines, and elevation of intestinal tight junction proteins; as well as mRNA expression of genes related to improving the intestinal integrity and structure in aged breeder hens. Taken together, these findings provide novel insights into the use of a combination of dietary Quercetin and Vitamin E as anti-aging agents in the development of therapeutic and preventive strategies for age-induced intestinal barrier disruption in aged breeder hens.
The original contributions presented in the study are included in the article/supplementary material. Further inquiries can be directed to the corresponding author.
The animal study was reviewed and approved by Animal Care and Use Committee of Sichuan Agricultural University, China. Animals used in this experiment were cared for under the guidelines stated in the Guide for the Care and Use of Agricultural Animals in Agricultural Research and Teaching of Sichuan Province, China (No. 2019502005).
The authors’ contributions are as follows: FKA, XZ, and GS designed and conceived this study. FKA, ZC, and XD conducted the experiments. FKA, ZC, XD, ZN, XD, DX, GS, YHW, XC, WS, and YT collected the samples and performed the analysis of samples. FKA, ZC, DL, YW, YZ, XHD, QZ, and XH analyzed the data. FKA wrote the manuscript. FA, XZ, QZ, DL, YW, and XHD revised and edited the manuscript. All authors contributed to the article and approved the submitted version.
The authors thank National Natural Science Foundation of China (Grant No. 31872347), the Science and Technology Innovation and Entrepreneurship Seedling Project of the Sichuan Science and Technology Program (2020JDRC0104), the Key Research & Development Plan of the Department of Science and Technology of Tibet Autonomous Region (XZ202101ZY0002N), the Local Projects Guided by the Central Government from Razi County, Tibet Autonomous Region, and the Projects Funded by the Central Government to Guide Local Scientific and Technological Development from Guizhou province (QIANKEZHONGYINDI[2021]4003) for funding this work.
The authors declare that the research was conducted in the absence of any commercial or financial relationships that could be construed as a potential conflict of interest.
All claims expressed in this article are solely those of the authors and do not necessarily represent those of their affiliated organizations, or those of the publisher, the editors and the reviewers. Any product that may be evaluated in this article, or claim that may be made by its manufacturer, is not guaranteed or endorsed by the publisher.
We deeply appreciate Xi Peng from Chengdu University for her professional comments which greatly improved our paper quality.
1. Takiishi T, Fenero CIM, Câmara NOS. Intestinal Barrier and Gut Microbiota: Shaping Our Immune Responses Throughout Life. Tissue Barriers (2017) 5:e1373208. doi: 10.1080/21688370.2017.1373208
2. Zheng JD, He Y, Yu HY, Liu YL, Ge YX, Li XT. Unconjugated Bilirubin Alleviates Experimental Ulcerative Colitis by Regulating Intestinal Barrier Function and Immune Inflammation. World J Gastroenterol (2019) 25:1865–78. doi: 10.3748/wjg.v25.i15.1865
3. Odenwald M, Turner J. The Intestinal Epithelial Barrier: A Therapeutic Target? Nat Rev Gastroenterol Hepatol (2017) 14:9–21. doi: 10.1038/nrgastro.2016.169
4. Landy J, Ronde E, English N, Clark SK, Hart AL, Knight SC, et al. Tight Junctions in Inflammatory Bowel Diseases and Inflammatory Bowel Disease Associated Colorectal Cancer. World J Gastroenterol (2016) 22:3117–26. doi: 10.3748/wjg.v22.i11.3117
5. Schulzke JD, Ploeger S, Amasheh M, Fromm A, Zeissig S, Troeger H, et al. Epithelial Tight Junctions in Intestinal Inflammation. Ann N.Y. Acad Sci (2009) 1165:294–300. doi: 10.1111/j.1749-6632.2009.04062.x
6. Thrasivoulou C, Soubeyre V, Ridha H, Giuliani D, Giaroni C, Michael GJ, et al. Reactive Oxygen Species, Dietary Restriction and Neurotrophic Factors in Age-Related Loss of Myenteric Neurons. Aging Cell (2006) 5:247–57. doi: 10.1111/j.1474-9726.2006.00214.x
7. Singh R, Chandrashekharappa S, Bodduluri SR, Baby BV, Hegde B, Kotla NG, et al. Enhancement of the Gut Barrier Integrity by a Microbial Metabolite Through the Nrf2 Pathway. Nat Commun (2019) 10:89. doi: 10.1038/s41467-018-07859-7
8. Vindigni SM, Zisman TL, Suskind DL, Damman CJ. The Intestinal Microbiome, Barrier Function, and Immune System in Inflammatory Bowel Disease: A Tripartite Pathophysiological Circuit With Implications for New Herapeutic Directions. Ther Adv Gastroenterol (2016) 9:606–25. doi: 10.1177/1756283X16644242
9. De Mey J, Freund J. Understanding Epithelial Homeostasis in the Intestine: An Old Battle Field of Ideas, Recent Breakthroughs and Remaining Controversies. Tissue Barriers (2013) 1:e24965. doi: 10.4161/tisb.24965
10. Catalioto R, Maggi C, Giuliani S. Intestinal Epithelial Barrier Dysfunction in Disease and Possible Therapeutical Interventions. Curr Med Chem (2011) 18:398–426. doi: 10.2174/092986711794839179
11. Lin WC, Lee MT, Chang SC, Chang YL, Shih CH, Yu B, et al. Effects of Mulberry Leaves on Production Performance and the Potential Modulation of Antioxidative Status in Laying Hens. Poult Sci (2017) 96:1191. doi: 10.3382/ps/pew350
12. Mishra B, Jha R. Oxidative Stress in the Poultry Gut: Potential Challenges and Interventions. Fron Vet Sci (2019) 6:60. doi: 10.3389/fvets.2019.00060
13. Circu ML, Aw TY. Intestinal Redox Biology and Oxidative Stress. Semin. Cell Dev Biol (2012) 23:729–37. doi: 10.1016/j.semcdb.2012.03.014
14. Saffrey MJ. Aging of the Mammalian Gastrointestinal Tract: A Complex Organ System. Age (Dordr) (2014) 36:9603. doi: 10.1007/s11357-013-9603-2
15. Ershler WB. Biological Interactions of Aging and Anemia: A Focus on Cytokines. J Am Geriatr Soc (2003) 51:S18–21. doi: 10.1046/j.1532-5415.51.3s.2.x
16. Kumar M, Babaei P, Ji B, Nielsen J. Human Gut Microbiota and Healthy Aging: Recent Developments and Future Prospective. Nutr Healthy Aging (2016) 4:3–16. doi: 10.3233/NHA-150002
17. Johansson MEV, Sjövall H, Hansson GC. The Gastrointestinal Mucus System in Health and Disease. Nat Rev Gastroenterol Hepatol (2013) 10:352–61. doi: 10.1038/nrgastro.2013.35
18. Junyuan Z, Hui X, Chunlan H, Junjie F, Qixiang M, Yingying L, et al. Quercetin Protects Against Intestinal Barrier Disruption and Inflammation in Acute Necrotizing Pancreatitis Through TLR4/MyD88/p38 MAPK and ERS Inhibition. Pancreatology (2018) 18:742–52. doi: 10.1016/j.pan.2018.08.001
19. Mahmoud KZ, Edens FW. Breeder Age Affects Small Intestine Development of Broiler Chicks With Immediate or Delayed Access to Feed. Br Poult Sci (2012) 53:32–41. doi: 10.1080/00071668.2011.652596
20. Schiffrin EJ, Morley JE, Donnet-Hughes A, Guigoz Y. The Inflammatory Status of the Elderly: The Intestinal Contribution. Mutat Res (2010) 690:50–6. doi: 10.1016/j.mrfmmm.2009.07.011
21. Biagi E, Nylund L, Candela M, Ostan R, Bucci L, Pini E, et al. Hrough Ageing, and Beyond: Gut Microbiota and Inflammatory Status in Eniors and Centenarians. PloS One (2010) 5:e10667. doi: 10.1371/journal.pone.0010667
22. Chen WY, Wang M, Zhang J, Barve SS, McClain CJ, Joshi-Barve S. Acrolein Disrupts Tight Junction Proteins and Causes Endoplasmic Reticulum Stress-Mediated Epithelial Cell Death Leading to Intestinal Barrier Dysfunction and Permeability. Am J Pathol (2017) 187:2686–97. doi: 10.1016/j.ajpath.2017.08.015
23. Duan C, Wang J, Liu Y, Zhang J, Si J, Hao Z, et al. Antiviral Effects of Ergosterol Peroxide in a Pig Model of Porcine Deltacoronavirus (PDCoV) Infection Involves Modulation of Apoptosis and Tight Junction in the Small Intestine. Vet Res (2021) 52:86. doi: 10.1186/s13567-021-00955-5
24. Aschoff AP, Ott U, Fünfstück R, Stein G, Jirikowski GF. Colocalization of BAX and BCL-2 in Small Intestine and Kidney Biopsies With Different Degrees of DNA Fragmentation. Cell Tissue Res (1999) 296:351–7. doi: 10.1007/s004410051295
25. Uyanga VA, Amevor FK, Liu M, Cui Z, Zhao X, Lin H. Potential Implications of Citrulline and Quercetin on Gut Functioning of Monogastric Animals and Humans: A Comprehensive Review. Nutrients (2021) 13:3782. doi: 10.3390/nu13113782
26. Wang J, Clark DL, Jacobi SK, Velleman SG. Effect of Vitamin E and Alpha Lipoic Acid on Intestinal Development Associated With Wooden Breast Myopathy in Broilers. Poult Sci (2021) 100:100952. doi: 10.1016/j.psj.2020.12.049
27. Amevor FK, Cui Z, Du X, Ning Z, Shu G, Jin N, et al. Combination of Quercetin and Vitamin E Supplementation Promotes Yolk Precursor Synthesis and Follicle Development in Aging Breeder Hens via Liver–Blood–Ovary Signal Axis. Animals (2021) 11:1915. doi: 10.3390/ani11071915
28. Amevor FK, Cui Z, Ning Z, Du X, Jin N, Shu G, et al. Synergistic Effects of Quercetin and Vitamin E on Egg Production, Egg Quality, and Immunity in Aging Breeder Hens. Poult Sci (2021) 100:101481. doi: 10.1016/j.psj.2021.101481
29. Pekmezci D. Vitamin E and Immunity. Vitam Horm (2011) 86:179–215. doi: 10.1016/B978-0-12-386960-9.00008-3
30. Ebeid TA, Zeweil HS, Basyony MM, Dosoky WM, Badry H. Fortification of Rabbit Diets With Vitamin E or Selenium Affects Growth Performance, Lipid Peroxidation, Oxidative Status and Immune Response in Growing Rabbits. Livest Sci (2013) 155:323–31. doi: 10.1016/j.livsci.2013.05.011
31. Tufarelli V, Laudadio V. Antioxidant Activity of Vitamin E and its Role in Avian Reproduction. J Exp Biol Agric Sci (2016) 4:267–72. doi: 10.18006/2016.4(3S).266.272
32. Michalczuk M, Damaziak K, Niemiec J. Dietary Vitamin E Supplementation on Cholesterol, Vitamin E Content, and Fatty Acid Profile in Chicken Muscles. Can. J Anim Sci (2016) 120:114–20. doi: 10.1139/cjas-2015-0103
33. Fusco D, Colloca G, Monaco MRL, Cesari M. Effects of Antioxidant Supplementation on the Aging Process. Clin Interv Aging (2007) 2:377–87.
34. Becker EM, Ntouma G, Skibsted LH. Synergism and Antagonism Between Quercetin and Other Chain-Breaking Antioxidants in Lipid Systems of Increasing Structural Organization. Food Chem (2007) 103:1288–96.
35. Dalia AM, Loh TC, Sazili AQ, Jahromi MF, Samsudin AA. Effects of Vitamin E, Inorganic Selenium, Bacterial Organic Selenium, and Their Combinations on Immunity Response in Broiler Chickens. BMC Vet Res (2018) 14:249. doi: 10.1186/s12917-018-1578-x
36. Gonzalez-Perez O, Gonzalez-Castaneda RE. Therapeutic Perspectiveson the Combination of a-Lipoic Acid and Vitamin. E. Nutr Res (2006) 26:1–5. doi: 10.1016/j.nutres.2005.11.011
37. Liu KY, Nakatsu CH, Jones-Hall Y, Kozik A, Jiang Q. Vitamin E Alpha- and Gamma-Tocopherol Mitigate Colitis, Protect Intestinal Barrier Function and Modulate the Gut Microbiota in Mice. Free Radic Biol Med (2021) 1(163):180–9. doi: 10.1016/j.freeradbiomed.2020.12.017
38. Sun Y, Zhang J, Song W, Shan A. Vitamin E Alleviates Phoxim-Induced Toxic Effects on Intestinal Oxidative Stress, Barrier Function, and Morphological Changes in Rats. Environ Sci Pollut Res Int (2018) 25:26682–92. doi: 10.1007/s11356-018-2666-y
39. Cadir FO, Bicakci U, Tander B, Kilicoglu-Aydin B, Rizalar R, Ariturk E, et al. Protective Effects of Vitamin E and Omeprazole on the Hypoxia/Reoxygenation Induced Intestinal Injury in Newborn Rats. Pediatr Surg Int (2008) 24:809–13. doi: 10.1007/s00383-008-2157-1
40. Zhao L, Zhang Q, Ma W, Tian F, Shen H, Zhou MA. Combination of Quercetin and Resveratrol Reduces Obesity in High-Fat Diet-Fed Rats by Modulation of Gut Microbiota. Food Funct (2017) 8:4644–56. doi: 10.1039/C7FO01383C
41. Zhao C, Yang CF, Liu B, Lin B, Sarker LD, Nahar SL, et al. Bioactive Compounds From Marine Macroalgae and Their Hypoglycemic Benefits. Trends Food Sci Technol (2018) 72:1–12. doi: 10.1016/j.tifs.2017.12.001
42. Shu G, Kong F, Xu D, Yin L, He C, Lin J, et al. Bamboo Leaf Flavone Changed the Community of Cecum Microbiota and Improved the Immune Function in Broilers. Sci Rep (2020) 10:12324. doi: 10.1038/s41598-020-69010-1
43. Yang J, Ding X, Bai S, Wang J, Zeng Q, Peng H, et al. Effects of Maternal Dietary Vitamin E on the Egg Characteristics, Hatchability and Offspring Quality of Prolonged Storage Eggs of Broiler Breeder Hens. J Anim Physiol Anim Nutr (Berl) (2018) 104:1384–91. doi: 10.1111/jpn.13371
44. Valenzano MC, Di Guilio K, Mercado J, Teter M, To J, Ferraro B, et al. Remodeling of Tight Junctions and Enhancement of Barrier Integrity of the CACO-2 Intestinal Epithelial Cell Layer by Micronutrients. PloS One (2015) 10:e0133926. doi: 10.1371/journal.pone.0133926
45. Suzuki T, Hara H. Quercetin Enhances Intestinal Barrier Function Through the Assembly of Zonula [Corrected] Occludens-2, Occludin, and Claudin-1 and the Expression of Claudin-4 in Caco-2 Cells. J Nutr (2009) 139:965–74. doi: 10.3945/jn.108.100867
46. Yan XM, Joo MJ, Lim JC, Whang WK, Sim SS, Im C, et al. The Effect of Quercetin-3-O-β-D-Glucuronopyranoside on Indomethacininduced Gastric Damage in Rats via Induction of Mucus Secretion and Down-Regulation of ICAM-1 Expression. Arch Pharm Res (2011) 34:1527–34. doi: 10.1007/s12272-011-0915-4
47. Lin R, Piao M, Song Y. Dietary Quercetin Increases Colonic Microbial Diversity and Attenuates Colitis Severity in Citrobacter Rodentium-Infected Mice. Front Microbiol (2019) 10:1092. doi: 10.3389/fmicb.2019.01092
48. Nie J, Zhang L, Zhao G, Du X. Quercetin Reduces Atherosclerotic Lesions by Altering the Gut Microbiota and Reducing Atherogenic Lipid Metabolites. J Appl Microbiol (2019) 127:1824–34. doi: 10.1111/jam.14441
49. Sun L, Xu G, Dong Y, Li M, Yang L, Lu W. Quercetin Protects Against Lipopolysaccharide-Induced Intestinal Oxidative Stress in Broiler Chickens Through Activation of Nrf2 Pathway. Molecules (2020) 25:1053. doi: 10.3390/molecules25051053
50. Zou Y, Wei HK, Xiang QH, Wang J, Zhou YF, Peng J. Protective Effect of Quercetin on Pig Intestinal Integrity After Transport Stress is Associated With Regulation Oxidative Status and Inflammation. J Vet Med Sci (2016) 78:1487–94. doi: 10.1292/jvms.16-0090
51. Zhao L, Zhu X, Xia M, Li J, Guo AY, Zhu Y, et al. Quercetin Ameliorates Gut Microbiota Dysbiosis That Drives Hypothalamic Damage and Hepatic Lipogenesis in Monosodium Glutamate-Induced Abdominal Obesity. Front Nutr (2021) 8:671353. doi: 10.3389/fnut.2021.671353
52. Shi T, Bian X, Yao Z, Wang Y, Gao W, Guo C. Quercetin Improves Gut Dysbiosis in Antibiotic-Treated Mice. Food Funct (2020) 11:8003–13. doi: 10.1039/D0FO01439G
53. Batista MA, Nicoli JR, dos Santos Martins F, Nogueira Machado JA, Esteves Arantes RM, Pacífifico Quirino IE, et al. Pretreatment With Citrulline Improves Gut Barrier After Intestinal Obstruction in Mice. J Parenter Enter Nutr (2012) 36:69–76. doi: 10.1177/0148607111414024
54. Dong Y, Lei J, Zhang B. Effects of Dietary Quercetin on the Antioxidative Status and Cecal Microbiota in Broiler Chickens Fed With Oxidized Oil. Poult Sci (2020) 99:4892–903. doi: 10.1016/j.psj.2020.06.028
55. Vazquez Prieto MA, Bettaieb A, Rodriguez Lanzi C, Soto VC, Perdicaro DJ, Galmarini ,CR, et al. Catechin and Quercetin Attenuate Adipose Inflammation in Fructose-Fed Rats and 3T3-L1 Adipocytes. Mol Nutr Food Res (2015) 59:622–33. doi: 10.1002/mnfr.201400631
56. Yang JX, Maria TC, Zhou B, Xiao FL, Wang M, Mao YJ, et al. Quercetin Improves Immune Function in Arbor Acre Broilers Through Activation of NF-κB Signaling Pathway. Poult Sci (2020) 99:2305.
57. Liu Y, Li Y, Liu HN, Suo YL, Hu LL, Feng XA, et al. Effect of Quercetin on Performance and Egg Quality During the Late Laying Period of Hens. Br Poult Sci (2013) 54:510–4. doi: 10.1080/00071668.2013.799758
58. Vukelic I, Detel D, Pucar LB, Potocnjak I, Buljevic S, Domitrovic R. Chlorogenic Acid Ameliorates Experimental Colitis in Mice by Suppressing Signaling Pathways Involved in Inflammatory Response and Apoptosis. Food Chem Toxicol Int J (2018) 121:140–50. doi: 10.1016/j.fct.2018.08.061
59. Ding S, Ma Y, Liu G, Yan W, Jiang H, Fang J. Lactobacillus Brevis Alleviates DSS-Induced Colitis by Reprograming Intestinal Microbiota and Influencing Serum Metabolome in Murine Model. Front Physiol (2019) 10:1152. doi: 10.3389/fphys.2019.01152
60. Cui Z, Amevor FK, Feng Q, Kang ,X, Song W, Zhu ,Q, et al. Sexual Maturity Promotes Yolk Precursor Synthesis and Follicle Development in Hens via Liver-Blood-Ovary Signal Axis. Anim (Basel) (2020) 10:2348. doi: 10.3390/ani10122348
61. Livak KJ, Schmittgen TD. Analysis of Relative Gene Expression Data Using RT-PCR and the 2 (–Delta C (T)) Methods. Methods (2001) 25:402–40. doi: 10.1006/meth.2001.1262
62. de La Serre CB, Ellis CL, Lee J, Hartman AL, Rutledge JC, Raybould H.E, et al. Propensity to High-Fat Diet-Induced Obesity in Rats is Associated With Changes in the Gut Microbiota and Gut Inflammation. Am J Physiol Gastrointest Liver Physiol (2010) 99:G440–8. doi: 10.1152/ajpgi.00098.2010
63. Brun P, Castagliuolo I, Di Leo V, Buda A, Pinzani M, Palù G, et al. Increased Intestinal Permeability in Obese Mice: New Evidence in the Pathogenesis of Nonalcoholic Steatohepatitis. Am J Physiol Gastrointest Liver Physiol (2007) 92:518–25. doi: 10.1152/ajpgi.00024.2006
64. Chen X, Zhao HX, Fu XS, Li CP, Zhong XL. Glucagonlike Peptide 2 Protects Intestinal Barrier in Severe Acute Pancreatitis Through Regulating Intestinal Epithelial Cell Proliferation and Apoptosis. Pancreas (2012) 41:1080–5. doi: 10.1097/MPA.0b013e31824966b0
65. Fukudome I, Kobayashi M, Dabanaka K, Maeda H, Okamoto K, Okabayashi T, et al. Diamine Oxidase as a Marker of Intestinal Mucosal Injury and the Effect of Soluble Dietary Fiber on Gastrointestinal Tract Toxicity After Intravenous 5-Fluorouracil Treatment in Rats. Med Mol Morphol (2014) 47:100–7. doi: 10.1007/s00795-013-0055-7
66. Liu S, Zhang D, Liu Y, Zhou D, Yang H, Zhang K, et al. Circular RNA Circ_0001105 Protects the Intestinal Barrier of Septic Rats by Inhibiting Inflammation and Oxidative Damage and YAP1 Expression. Gene (2020) 755:144897. doi: 10.1016/j.gene.2020.144897
67. Panda AK, Cherian G. Role of Vitamin E in Counteracting Oxidative Stress in Poultry. J Poult Sci (2014) 51:109–17. doi: 10.2141/jpsa.0130134
68. Abedi P, Tabatabaei VS, Mamouei M, Aghaei A. Effect of Different Levels of Dietary Vitamin E on Reproductive and Productive Performances in Japanese Quails (Coturnix Coturnix Japonica). Vet Res Forum (2017) 8:353–9.
69. Feng Z, Li T, Wu C, Tao L, Blachier F, Yin Y. Monosodium L-Glutamate and Dietary Fat Exert Opposite Effects on the Proximal and Distalintestinal Health in Growing Pigs. Appl Physiol Nutr Metab (2016) 40:353–63. doi: 10.1139/apnm-2014-0434
70. Ducatelle R, Goossens E, De Meyer F, Eeckhaut V, Antonissen G, Haesebrouck F, et al. Biomarkers for Monitoring Intestinal Health in Poultry: Present Status and Future Perspectives. Vet Res (2018) 49:43. doi: 10.1186/s13567-018-0538-6
71. Hosseini-Vashan SJ, Safdari-Rostamabad M, Piray AH, Sarir H. The Growth Performance, Plasma Biochemistry Indices, Immune System, Antioxidant Status, and Intestinal Morphology of Heat-Stressed Broiler Chickens Fed Grape (Vitis Vinifera) Pomace. Anim Feed Sci Technol (2020) 259:114343. doi: 10.1016/j.anifeedsci.2019.114343
72. Cui Y, Okyere SK, Gao P, Wen J, Cao S, Wang Y, et al. Ageratina Adenophora Disrupts the Intestinal Structure and Immune Barrier Integrity in Rats. Toxins (Basel) (2021) 13:651. doi: 10.3390/toxins13090651
73. Chwen L, Foo H, Thanh N, Choe D. Growth Performance, Plasma Fatty Acids, Villous Height and Crypt Depth of Preweaning Piglets Fed With Medium Chain Triacylglycerol. Asian-australas J Anim Sci (2013) 26:700–4. doi: 10.5713/ajas.2012.12561
74. Wang X, Xue Y, Li Y, Liu F, Yan Y, Zhang H, et al. Effects of Isatis Root Polysaccharide in Mice Infected With H3N2 Swine Influenza Virus. Res Vet Sci (2018) 119:91–8. doi: 10.1016/j.rvsc.2018.04.012
75. Qin S, Zhang MKY, Ding XM, Bai SP, Wang JP, Zeng QF. Effect of Dietary Graded Resistant Potato Starch Levels on Growth Performance, Plasma Cytokines Concentration, and Intestinal Health in Meat Ducks. Poult Sci (2019) 98:3523–32. doi: 10.3382/ps/pez186
76. Wang K, Cao G, Zhang H, Li Q, Yang C. Effects of Clostridium Butyricum and Enterococcus Faecalis on Growth Performance, Immune Function, Intestinal Morphology, Volatile Fatty Acids, and Intestinal Flora in a Piglet Model. Food Funct (2019) 10:7844–54. doi: 10.1039/C9FO01650C
77. Jayaraman S, Thangavel G, Kurian H, Mani R, Mukkalil R, Chirakkal H. Bacillus Subtilis PB6 Improves Intestinal Health of Broiler Chickens Challenged With Clostridium Perfringens-Induced Necrotic Enteritis. Poult Sci (2013) 92:370–4. doi: 10.3382/ps.2012-02528
78. Okyere SK, Wen J, Cui Y, Xie L, Gao P, Zhang M, et al. Bacillus Toyonensis SAU-19 and SAU-20 Isolated From Ageratina Adenophora Alleviates the Intestinal Structure and Integrity Damage Associated With Gut Dysbiosis in Mice Fed High Fat Mice. Front Microbiol (2022) 13:820236. doi: 10.3389/fmicb.2022.820236
79. Awad WA, Ghareeb K, Abdel-Raheem S, Böhm J. Effects of Dietary Inclusion of Probiotic and Synbiotic on Growth Performance, Organ Weights, and Intestinal Histomorphology of Broiler Chickens. Poult Sci (2009) 88:49–56. doi: 10.3382/ps.2008-00244
80. Saeid J, Mohamed A, Al-Baddy M. Effect of Garlic Powder (Allium Sativum) and Black Seed (Nigella Sativa) on Broiler Growth Performance and Intestinal Morphology. Iran J Appl Anim Sci (2013) 3:185–8.
81. Curgali K, Toth S, Jonecova Z, Maretta M, Kalpakidis T, Petriskova I, et al. Quercetin Protects Jejunal Mucosa From Experimental Intestinal Ischemia Reperfusion Injury by Activation of CD68 Positive Cells. Acta Histochem (2018) 120:28–32. doi: 10.1016/j.acthis.2017.11.001
82. Gonzales GB, Van Camp J, Smagghe G, Raes K, Mackie A. Flavonoid-Gastrointestinal Mucus Interaction and its Potential Role in Regulatingflavonoid Bioavailability and Mucosal Biophysical Properties. Food Res Int (2016) 88:342–7. doi: 10.1016/j.foodres.2015.12.023
83. Damiano S, Sasso A, De Felice B, Di Gregorio I, La Rosa G, Lupoli GA, et al. Quercetin Increases MUC2 and MUC5AC Gene Expression and Secretion in Intestinal Goblet Cell-Like LS174T via PLC/Pkcα/ERK1-2 Pathway. Front Physiol (2018) 6(9):357. doi: 10.3389/fphys.2018.00357
84. Lammers A, Wieland WH, Kruijt L, Jansma A, Straetemans T, Schots A, et al. Successive Immunoglobulin and Cytokine Expression in the Small Intestine of Juvenile Chicken. Dev Comp Immunol (2010) 34:1254–62. doi: 10.1016/j.dci.2010.07.001
85. Lindner C, Wahl B, Fohse L, Suerbaum S, Macpherson AJ, Prinz I, et al. Age, Microbiota, and T Cells Shape Diverse Individual IgA Repertoires in the Intestine. J Exp Med (2012) 209:365–77. doi: 10.1084/jem.20111980
86. Buschmann MM, Shen L, Rajapakse H, Raleigh DR, Wang Y, Wang Y, et al. Occludin OCEL-Domain Interactions are Required for Maintenance and Regulation of the Tight Junction Barrier to Macromolecular Flux. Mol Biol Cell (2013) 24:3056–68. doi: 10.1091/mbc.e12-09-0688
87. Gong Y, Li H, Li Y. Effects of Bacillus Subtilis on Epithelial Tight Junctions of Mice With Inflammatory Bowel Disease. J Interferon Cytokine Res (2016) 36:75–85. doi: 10.1089/jir.2015.0030
88. Xu CM, Li XM, Qin BZ, Liu B. Effect of Tight Junction Protein of Intestinal Epithelium and Permeability of Colonic Mucosa in Pathogenesis of Injured Colonic Barrier During Chronic Recovery Stage of Rats With Inflammatory Bowel Disease. Asian Pac J Trop Med (2016) 9:148–52. doi: 10.1016/j.apjtm.2016.01.001
89. Wu QJ, Liu N, Wu XH, Wang GY, Lin L. Glutamine Alleviates Heat Stress-Induced Impairment of Intestinal Morphology, Intestinal Inflammatory Response, and Barrier Integrity in Broilers. Poult Sci (2018) 97:2675–83. doi: 10.3382/ps/pey123
90. Jeong CH, Kwon HC, Kim DH, Cheng WN, Kang S, Shin DM, et al. Effects of Aluminum on the Integrity of the Intestinal Epithelium: An In Vitro and In Vivo Study. Environ Health Perspect (2020) 128:17013. doi: 10.1289/EHP5701
91. Suzuki T, Hara H. Role of Flavonoids in Intestinal Tight Junction Regulation. J Nutr Biochem (2011) 22:401–8. doi: 10.1016/j.jnutbio.2010.08.001
92. Guo S, Nighot M, Al-Sadi R, Alhmoud T, Nighot P, Ma TY. Lipopolysaccharide Regulation of Intestinal Tight Junction Permeability is Mediated by TLR4 Signal Transduction Pathway Activation of FAK and Myd88. J Immunol (2015) 195:4999–5010. doi: 10.4049/jimmunol.1402598
93. Jiang N, Li Y, Shu T, Wang J. Cytokines and Inflammation in Adipogenesis: An Updated Review. Front Med (2019) 13:314–29. doi: 10.1007/s11684-018-0625-0
94. Farré R, Fiorani M, Abdu Rahiman S, Matteoli G. Intestinal Permeability, Inflammation and the Role of Nutrients. Nutrients (2020) 12:1185. doi: 10.3390/nu12041185
95. Zhang K, Shi S, Han W. Research Progress in Cytokines With Chemokine-Like Function. Cell Mol Immunol (2018) 15:660–2. doi: 10.1038/cmi.2017.121
96. Medzhitov R. Origin and Physiological Roles of Inflammation. Nature (2008) 454:428–35. doi: 10.1038/nature07201
98. Van Kaer L, Olivares-Villagomez D. Development, Homeostasis, and Functions of Intestinal Intraepithelial Lymphocytes. J Immunol (2018) 200:2235–44. doi: 10.1016/j.it.2017.11.003
99. Nunes C, Ferreira E, Freitas V, Almeida L, Barbosa RM, Laranjinha J. Intestinal Anti-Inflammatory Activity of Red Wine Extract: Unveiling the Mechanisms in Colonic Epithelial Cells. Food Funct (2013) 4:373–83. doi: 10.1039/C2FO30233K
100. Devine PJ, Perreault SD, Luderer U. Roles of Reactive Oxygen Species and Antioxidants in Ovarian Toxicity. Biol Reprod (2012) 86:27. doi: 10.1095/biolreprod.111.095224
101. Li H, Wang T, Xu C, Wang D, Ren J, Li Y, et al. Transcriptome Profifile of Liver at Different Physiological Stages Reveals Potential Mode for Lipid Metabolism in Laying Hens. BMC Genom (2015) 16:763–75. doi: 10.1186/s12864-015-1943-0
102. Yang JX, Chaudhry MT, Yao JY, Wang SN, Zhou B, Wang M, et al. Effects of Phyto-Oestrogen Quercetin on Productive Performance, Hormones, Reproductive Organs and Apoptotic Genes in Laying Hens. J Anim Physiol Anim Nutr (Berl) (2018) 102:505–13. doi: 10.1111/jpn.12778
Keywords: chicken, antioxidants, intestinal immunity, inflammation, oxidative stress
Citation: Amevor FK, Cui Z, Du X, Ning Z, Deng X, Xu D, Shu G, Wu Y, Cao X, Shuo W, Tian Y, Li D, Wang Y, Zhang Y, Du X, Zhu Q, Han X and Zhao X (2022) Supplementation of Dietary Quercetin and Vitamin E Promotes the Intestinal Structure and Immune Barrier Integrity in Aged Breeder Hens. Front. Immunol. 13:860889. doi: 10.3389/fimmu.2022.860889
Received: 23 January 2022; Accepted: 21 February 2022;
Published: 21 March 2022.
Edited by:
Wenkai Ren, South China Agricultural University, ChinaReviewed by:
Xin Wu, Chinese Academy of Sciences (CAS), ChinaCopyright © 2022 Amevor, Cui, Du, Ning, Deng, Xu, Shu, Wu, Cao, Shuo, Tian, Li, Wang, Zhang, Du, Zhu, Han and Zhao. This is an open-access article distributed under the terms of the Creative Commons Attribution License (CC BY). The use, distribution or reproduction in other forums is permitted, provided the original author(s) and the copyright owner(s) are credited and that the original publication in this journal is cited, in accordance with accepted academic practice. No use, distribution or reproduction is permitted which does not comply with these terms.
*Correspondence: Xiaoling Zhao, emhhb3hpYW9saW5nQHNpY2F1LmVkdS5jbg==
Disclaimer: All claims expressed in this article are solely those of the authors and do not necessarily represent those of their affiliated organizations, or those of the publisher, the editors and the reviewers. Any product that may be evaluated in this article or claim that may be made by its manufacturer is not guaranteed or endorsed by the publisher.
Research integrity at Frontiers
Learn more about the work of our research integrity team to safeguard the quality of each article we publish.