- 1National Clinical Research Center for Hematologic Diseases, Jiangsu Institute of Hematology, The First Affiliated Hospital of Soochow University, Suzhou, China
- 2Institute of Blood and Marrow Transplantation, Collaborative Innovation Center of Hematology, Soochow University, Suzhou, China
- 3Key Laboratory of Thrombosis and Hemostasis of Ministry of Health, Institute of Blood and Marrow Transplantation, Suzhou, China
- 4Research and Development Department, Shanghai Unicar-Therapy Bio-Medicine Technology Co., Ltd., Shanghai, China
Background/Aims: Chimeric antigen receptor (CAR) T cells for refractory or relapsed (r/r) B-cell acute lymphoblastic leukemia (ALL) patients have shown promising clinical effectiveness. However, the factors impacting the clinical response of CAR-T therapy have not been fully elucidated. We here aimed to identify the independent factors of CAR-T treatment response and construct the models for predicting the complete remission (CR) and minimal residual disease (MRD)-negative CR in r/r B-ALL patients after CAR-T cell infusion.
Methods: Univariate and multivariate logistic regression analyses were conducted to identify the independent factors of CR and MRD-negative CR. The predictive models for the probability of remission were constructed based on the identified independent factors. Discrimination and calibration of the established models were assessed by receiver operating characteristic (ROC) curves and calibration plots, respectively. The predictive models were further integrated and validated in the internal series. Moreover, the prognostic value of the integration risk model was also confirmed.
Results: The predictive model for CR was formulated by the number of white blood cells (WBC), central neural system (CNS) leukemia, TP53 mutation, bone marrow blasts, and CAR-T cell generation while the model for MRD-negative CR was formulated by disease status, bone marrow blasts, and infusion strategy. The ROC curves and calibration plots of the two models displayed great discrimination and calibration ability. Patients and infusions were divided into different risk groups according to the integration model. High-risk groups showed significant lower CR and MRD-negative CR rates in both the training and validation sets (p < 0.01). Furthermore, low-risk patients exhibited improved overall survival (OS) (log-rank p < 0.01), higher 6-month event-free survival (EFS) rate (p < 0.01), and lower relapse rate after the allogeneic hematopoietic stem cell transplantation (allo-HSCT) following CAR-T cell infusion (p = 0.06).
Conclusions: We have established predictive models for treatment response estimation of CAR-T therapy. Our models also provided new clinical insights for the accurate diagnosis and targeted treatment of r/r B-ALL.
Introduction
Refractory or relapsed (r/r) B-cell acute lymphoblastic leukemia (ALL) remains one of the most fatal hematological malignancies with the reported median overall survival (OS) ranging from 3.0 to 8.4 months even after salvage chemotherapy or transplantation (1–4). Chimeric antigen receptor (CAR) T-cell therapy emerges as a new cellular immunotherapeutic strategy these years showing impressive efficacy in r/r B-ALL (5, 6). Published clinical trials have indicated a high complete remission (CR) rate of 67% to 93% in r/r B-ALL patients who received CAR-T cell infusion (7–13). However, there are still approximately 10% to 30% patients who had no response to the treatment and a large proportion of patients who relapsed soon after achieving CR (7–13). This is mainly because engineered T-cell therapy is an immensely individualized treatment due to the high heterogeneity of the malignancies, patients, and these functional T cells, which was originally collected from each patient (6, 14). Hence, to further improve therapeutic response, it is imperative for effective predictive tools to select the most benefited patients before infusion. In addition, on account of the considerable costs of CAR-T therapy, pretreatment evaluation is also of great significance to improve the cost-effectiveness as the application of this therapy is increasingly broadened (15, 16).
The influence factors of CAR-T therapeutic effect remain unclear. The results of several studies trying to identify these clinical factors varied (17–19). Besides, previous studies mainly centered on the resistance of cancer cells to the modified T cells and the lack of the persistence of CAR-T cells, irrespective of the clinical characteristics of the patients themselves (20, 21). Therefore, studies investigating the baseline characteristics as the potential predictive or prognostic factors of this novel therapy are warranted to help facilitate clinical prediction and guide personalized treatment. Here, we enrolled a large cohort of r/r B-ALL patients treated with CAR-T cells from three clinical trials and performed analyses to screen the independent factors of CAR-T treatment response. Also ultimately, in this study we first established the simple-to-use models for predicting the clinical outcome of these patients.
Materials and Methods
Patients and Data Collection
A total of 286 consecutive r/r B-ALL patients who received CAR-T cells enrolled on three clinical trials (www.clinicaltrials.gov, identifiers: NCT03919240, NCT03614858, and NCT03275493) from December 2015 to September 2021 were included in this study. The major inclusion criteria were as follows: (1) diagnosed as refractory/relapsed B-ALL; (2) Karnofsky performance status score ≥ 60 or Eastern Cooperative Oncology Group performance score ≤ 2; (3) estimated survival time ≥3 months; and (4) ineligible for or refusal to allogeneic hemopoietic stem cell transplantation (allo-HSCT). Relapsed disease was defined as >5% bone marrow blasts, reappearance of circulating blasts, or development of extramedullary disease. Refractory disease was defined as those patients who did not achieve CR after 2 courses of intensive induction chemotherapy (13, 22). All three clinical trials and this study were approved by the Institutional Ethics Committees of the First Affiliated Hospital of Soochow University and conformed to the provisions of the Declaration of Helsinki. Written informed consent was obtained from the patients or their legal guardians. Clinical data including the clinical characteristics of every subject and the baseline information of the CAR-T cells used for each time of infusion for each patient were collected. All the data were extracted from the electronic medical record system of the patients.
Study Design
The overall design of this study is as summarized in Figure 1. Herein, two main phases including constructing and validating the predictive models for r/r B-ALL patients after CAR-T therapy were conducted. Out of all the 286 subjects, 204 patients enrolled on the above three clinical trials from December 2015 to March 2020 were treated as the discovery dataset. Another 82 patients from April 2020 to September 2021 were taken as the independent validation set. During the discovery phase, the first time of CAR-T cell infusion of each subject from the discovery set was included for the model construction. Firstly, the univariate and multivariate logistic regression analyses were applied to screen and identify the independent CR- and minimal residual disease (MRD)-negative CR-related factors. Except binary variables, all the variables were transformed into categorical ones before putting into logistic models. Moreover, the cutoff values adopted for the transformed covariates were as shown in Table 1. In the meantime, the predictive models were generated from the forward stepwise multivariate analysis (likelihood ratio). Then, for model evaluation, receiver operating characteristic (ROC) curves, along with the corresponding area under the curve (AUC), were used to assess the discrimination ability. The calibration curves for estimation of the consistency between the actual observation and the model-predicted value, and the Hosmer–Lemeshow chi-square (χ2) test were carried out simultaneously for evaluating the accuracy and goodness of fit (23). In the validation analyses, the two models predicting CR and MRD-negative CR were integrated into one risk model for better prediction. First of all, we used all the infusions of 204 patients in the discovery set (some patients received more than one time of CAR-T cell infusion) as the training set to preliminarily validate the risk model. Furthermore, we also followed up the 204 participants for their survival status, 6-month event-free survival (EFS) status, subsequent treatments including transplantation, etc. The OS, 6-month EFS rate, and relapse rate of those who received allo-HSCT after CAR-T therapy were compared between different risk groups, respectively, to confirm the prognostic value of the modified model. Eventually, all the infusions of an independent patient cohort were used to further validate our risk model.
CAR-T Therapy
CAR-T cells were produced by Shanghai UniCAR Technology Co., Ltd. (UCT, Shanghai, China) (24, 25). Briefly, mononuclear cells for CAR-T cell production were obtained from the peripheral blood of the patients, transplant, or healthy donors by leukapheresis. Then, these cells were purified and transduced with lentiviral vector encoding chimeric T-cell antigen receptors. The CAR was finally composed of targeted single-chain variable fragment (scFv), intracellular domain including 4-1BB or CD28 as a co-stimulation signal, and a cytoplasmic signaling sequence, CD3ζ. Antigen receptors of these CAR-T cells contain CD19, CD22, and dual target, CD19+CD22.
Patients received fludarabine and cyclophosphamide (FC)-based lymphodepletion regimen prior to CAR-T cell infusion. Bridging chemotherapy before lymphodepletion included decitabine, rituximab, or other cytotoxic chemotherapy, such as MVP regimen (MVP: mitoxantrone, vincristine and prednisone). For one single time of infusion, CAR-T cells were infused on 3 consecutive days with 10%, 30%, and 60% of the total dose, respectively, or on 2 days, 40% for day +1 and 60% for day +2. The median cell dose of the infused cells was 0.5 × 107/kg (range, 0.05–67 × 107/kg). Philadelphia chromosome (Ph)-positive B-ALL patients were given tyrosine kinase inhibitors (TKI).
Response Assessment and Follow-Up
CR was defined as <5% blasts in bone marrow and absence of extramedullary disease. MRD-negative CR was defined as <0.01% blasts in bone marrow detected by multicolor flow cytometry and, also, no evidence of extramedullary disease (13, 19). Bone marrow examination was performed at least 28 days after CAR-T cell infusion for evaluation of treatment response. Cytokine release syndrome (CRS) including CAR-T cell-related encephalopathy syndrome (CRES) was graded according to the criteria proposed by Lee et al. (26, 27). The last follow-up of the long-term survival of the patients from the discovery group was on December 31, 2020. OS was defined as the interval between the date of the first infusion and the date of death of any cause, allotransplant, or the last follow-up. EFS was calculated from the date of the first infusion to the date of relapse, death, or the last follow-up. If a patient had no response to CAR-T therapy, EFS status was also defined as 1 (28).
Statistical Considerations
To prevent missing more clinically significant indexes, the p values less than 0.2 from univariate analysis were considered as the threshold for inclusion in multivariate analysis. The univariate and multivariate logistic regression analyses were carried out via SPSS 23.0 for Windows (SPSS, Chicago, IL). The ROC curves with AUC calculation, Kaplan–Meier curves, and log-rank tests were performed in GraphPad Prism, version 7.0. The Hosmer–Lemeshow test was conducted by SPSS software (version 23.0), and its p value more than 0.05 indicated that the difference between the expected and actually observed values was insignificant. For each infusion, the patient could obtain a probability score of CR or MRD-negative CR generated from the constructed models. The clinical outcome of each infusion was then evaluated as high probability of CR (Xhigh) or low probability of CR (Xlow), and high probability of MRD-negative CR (Yhigh) or low probability of MRD-negative CR (Ylow) based on the cutoff values generated from ROC curves when Youden’s indexes achieved maximum. The proportions of CR, MRD-CR, event-free, and relapse patients among different groups were compared by χ2 test or Fisher’s exact test using absolute numbers of subjects in SPSS software as well. p < 0.05 in this study was considered statistically significant, otherwise indicated.
Results
Clinical Characteristics of the Study Population
The baseline characteristics of 204 participants in the discovery set and the CAR-T cells they initially received are presented in Table 1. Out of them, 10 (4.9%) r/r B-ALL patients were switched from CML and 44 (21.6%) had at least one allo-HSCT before CAR-T therapy. The Philadelphia chromosome was detected positive in 63 (30.9%) samples. 42 (20.6%) participants had extremely high tumor burden with white blood cells (WBC) in peripheral blood more than 100 × 109/l when newly diagnosed, while nearly half of the patients (N = 99) had relatively low (<5% of blasts) tumor burden before lymphodepletion, because most of the patients were heavily treated before CAR-T infusion with a median previous therapy number of 4, leading to varying degrees of bone marrow hypocellularity. Extramedullary disease other than CNS involvement was found in 62 (30.4%) subjects at enrollment. 14 patients were diagnosed with central neural system (CNS) leukemia. CRS occurred in 155 (76.0%) patients including 51 (25.0%) with severe symptoms (grade ≥3). 17 (8.3%) patients developed CRES of grade 3 or higher. In all, 176 (86.3%) patients achieved CR after CAR-T therapy and among them, 145 (71.1%) achieved MRD-negative CR. The 1-year probability of survival was 71.8% with the median follow-up time of 16.2 months (range: 1.3–52.1 months).
Searching for the Factors Associated With CR and MRD-Negative CR in r/r B-ALL Patients After CAR-T Therapy
To search for the possible factors of r/r B-ALL patients receiving CAR-T treatment, we first conducted univariate logistic regression analysis on clinical characteristics of the patients (Table 2) and the CAR-T cells infused for the first time (Table 3). The univariate analytic results revealed that the number of WBC in peripheral blood detected at diagnosis, CNS leukemia, TP53 mutation, number of relapses, and bone marrow blasts detected before lymphodepletion or CAR-T cell infusion (for those without lymphodepletion) were significantly associated with CR after CAR-T cell infusion (p < 0.05, Tables 2, 3). Also, two factors, number of relapses and CRS grade, were shown to have significant relations to MRD-negative CR (p < 0.05, Tables 2, 3), while the relation between CRS grade and CR was marginally significant (p = 0.06, Table 3). The following factors, the number of WBC in peripheral blood detected at diagnosis (p = 0.09, Table 2) and bone marrow blasts detected before lymphodepletion or CAR-T cell infusion (p = 0.08, Table 3), were found to have marginally significant correlations with MRD-negative CR despite their statistically significant relations to CR.
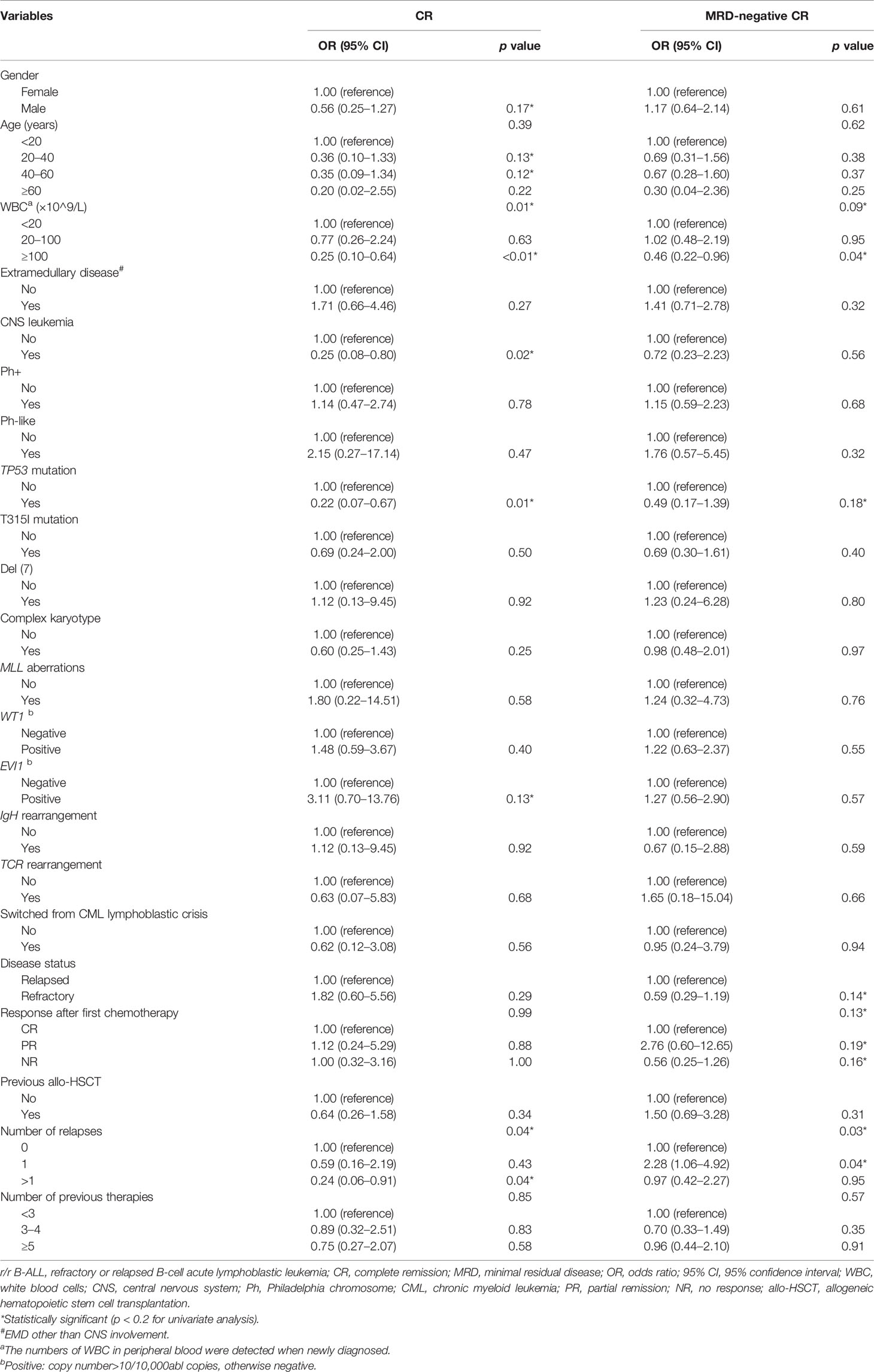
Table 2 Univariate logistic regression analyses of the clinical characteristics of r/r B-ALL patients associated with CR and MRD-negative CR.
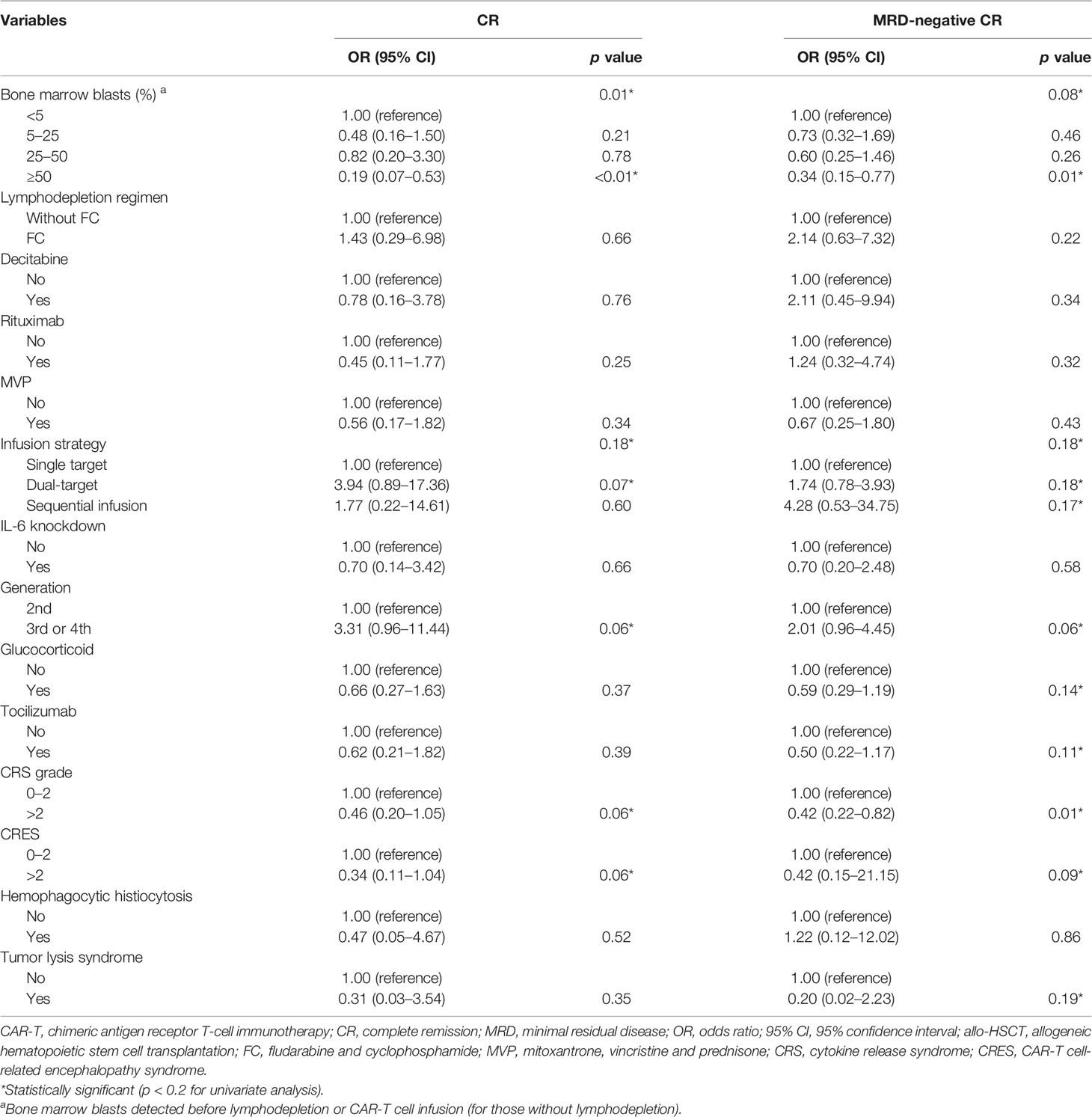
Table 3 Univariate logistic regression analyses of the baseline information of CAR-T therapy and CAR-T cells associated with CR and MRD-negative CR.
Identification of the Independent Factors Impacting CAR-T Therapeutic Response
In order to further identify the independent factors of the remission after CAR-T therapy, the possible influence factors (univariate logistic p < 0.2) from the above univariate analyses were incorporated into the following multivariate logistic regression analyses. The multivariate analytic results showed that the number of WBC in peripheral blood detected at diagnosis, CNS leukemia, TP53 mutation, bone marrow blasts detected before lymphodepletion, or CAR-T cell infusion and CAR-T cell generation were significant independent factors of CR (p < 0.05, Supplementary Table 1), while for MRD-negative CR, the independent factors were as follows: disease status (refractory or relapsed disease), bone marrow blasts detected before lymphodepletion, or CAR-T cell infusion and infusion strategy which referred to the choice of the infusion of single- or dual-target CAR-T cells or sequential infusion of two single-specific CAR-T cells (p < 0.05, Supplementary Table 2).
Construction of the Predictive Models for the Treatment Response of r/r B-ALL Patients Receiving CAR-T Cell Infusion
The models for predicting the probability of CR and MRD-negative CR of r/r B-ALL patients after CAR-T therapy were constructed based on the above stepwise multivariate logistic regression analysis. The predictive model for CR was as follows:
Definition and value:
PCR = the probability of CR;
X = the probability score of CR;
WBC: the number of WBC in peripheral blood detected at diagnosis; the value of WBC (×109/L): <20:1; 20–100:2; ≥100:3;
CNS leukemia: no:0; yes = 1;
TP53 mutation: no:0; yes = 1;
Blast: bone marrow blasts detected before lymphodepletion or CAR-T cell infusion (for those without lymphodepletion); the value of blast: <5:0; 5–25:1; 25–50:2; ≥50:3;
Generation: CAR-T cell generation used for this infusion; the value of generation: 2nd:1; 3rd or 4th:2.
The predictive model for MRD-negative CR was as follows:
Definition and value:
PMRD-CR = the probability of MRD-negative CR;
Y = the probability score of MRD-negative CR;
Disease_status: relapsed:0; refractory:1;
Blast: bone marrow blasts detected before lymphodepletion or CAR-T cell infusion (for those without lymphodepletion); the value of Blast: <5:0; 5–25:1; 25–50:2; ≥50:3;
Infusion_strategy: infusion of single- or dual-target CAR-T cells or sequential infusion of two single-specific CAR-T cells; single target:1; dual-target:2; sequential infusion: 3.
The discrimination ability of the established models was assessed by ROC curves. The CR-predicting model exhibited great performance in distinguishing CR patients from those not achieving CR with the AUC reaching 0.79 (95% CI: 0.69–0.89) (Figure 2A). The AUC of the ROC curves plotted based on the predictive model for MRD-negative CR was 0.66 (95% CI: 0.58–0.74) (Figure 2B). The accuracy of the two models was evaluated by calibration curves. The calibration plots showed good agreement when comparing the expected values generated from the constructed models and the observed values. The Hosmer–Lemeshow tests of the two models showed great goodness of fit with the p values, 0.62 and 0.68, respectively (Figures 2C, D).
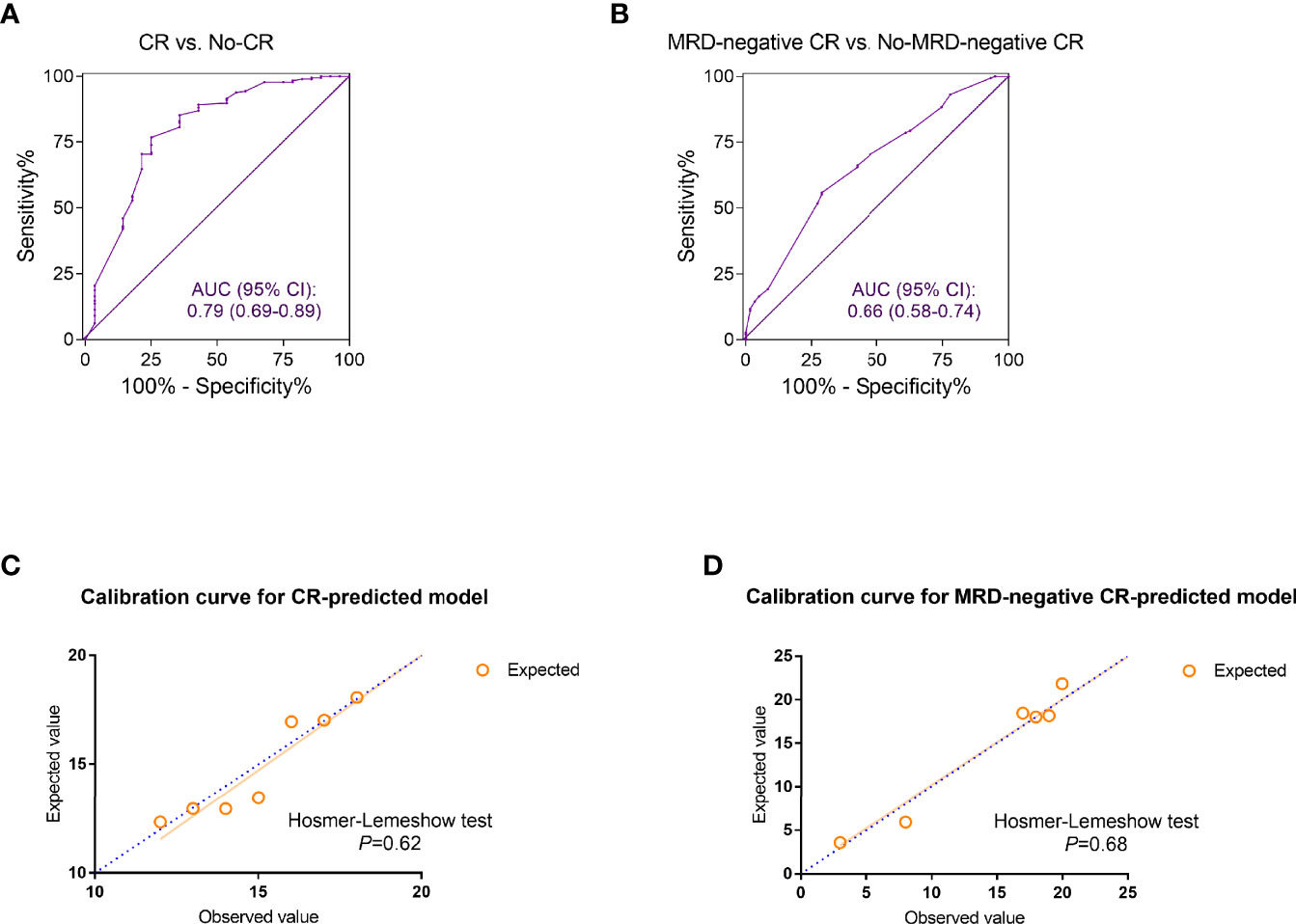
Figure 2 The discrimination and calibration evaluation of the complete remission (CR) and minimal residual disease (MRD)-negative CR predicted models. (A, B) The receiver operating characteristic (ROC) curves discriminating the refractory or relapsed (r/r) B-cell acute lymphoblastic leukemia (ALL) patients who achieved CR (A) or MRD-negative CR (B) from those who did not. The area under the curve (AUC) of ROC was calculated for evaluation. (C, D) Calibration curves for the estimation of CR (C) and MRD-negative CR (D). The observed and the model-expected numbers of events (CR and MRD-negative CR) were plotted on the x- and y-axes, respectively. p values generated from Hosmer–Lemeshow tests were also calculated for assessing the goodness of fit of the constructed models.
Development and Preliminary Validation of the Constructed Predictive Models
To better predict the treatment response of r/r B-ALL patients after CAR-T therapy, the above two predictive models were further modified and integrated as one risk model. In the validation analyses, the training group was composed of the 242 infusions which 204 patients had altogether. A total of 242 infusions were divided into Xhigh and Xlow groups, Yhigh and Ylow groups, by the cutoff value, 1.8 and 1.0, respectively. The treatment outcome of an infusion evaluated as Xhigh and Yhigh simultaneously was defined as the low-risk infusion while an infusion assessed as Xlow and Ylow was defined as high-risk, otherwise intermediate-risk (Figure 3A). The CR and MRD-negative CR rates were compared among three subgroups (high-risk, intermediate-risk, and low-risk groups). The results showed that three risk groups had significantly different remission proportions (p < 0.01, Figures 3B, C).
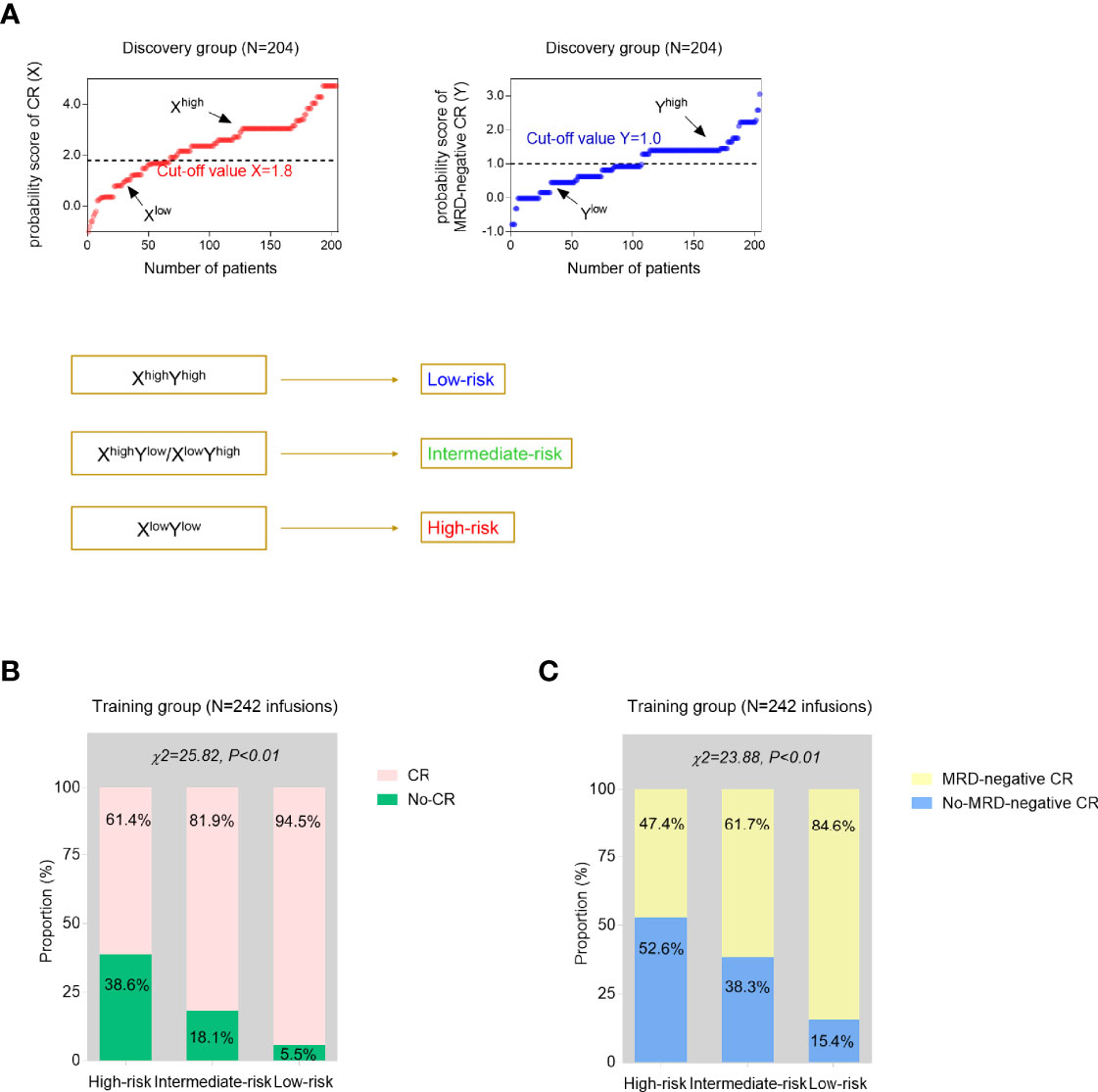
Figure 3 Development and validation analyses of the established models. (A) Using the CR-predicted and MRD-negative CR-predicted models, each time of CAR-T cell infusion could be evaluated as high probability of CR (Xhigh) or low probability of CR (Xlow), high probability of MRD-negative CR (Yhigh), or low probability of MRD-negative CR (Ylow) with the cutoff values generated from the discovery cohort. Then, two independent predictive models were further integrated as one risk stratification model based on the simultaneous estimation of the probability of CR and MRD-negative CR. (B, C) The proportions of CR (B) and MRD-negative CR (C) infusions were compared among three risk groups from the training group. p values were calculated via the chi-square (χ2) test.
To further explore the prognostic value of the predictive models, the long-term survival was compared among three risk groups. As seen from the Kaplan–Meier curves, low-risk patients had significantly better OS than high-risk and intermediate-risk patients (log-rank p < 0.01, Figure 4A). The 6-month EFS rate was also compared and significantly differed among three subgroups (p < 0.01, Figure 4B). In addition, the prognosis of the patients who received allo-HSCT after CAR-T cell infusion was assessed. 25 high-risk patients and 43 low-risk patients underwent allotransplant following CAR-T therapy altogether. The relapse rate in high-risk patients was higher than that in low-risk ones (p = 0.06, p value was insignificant probably because of the limited number of samples) (Figure 4C).
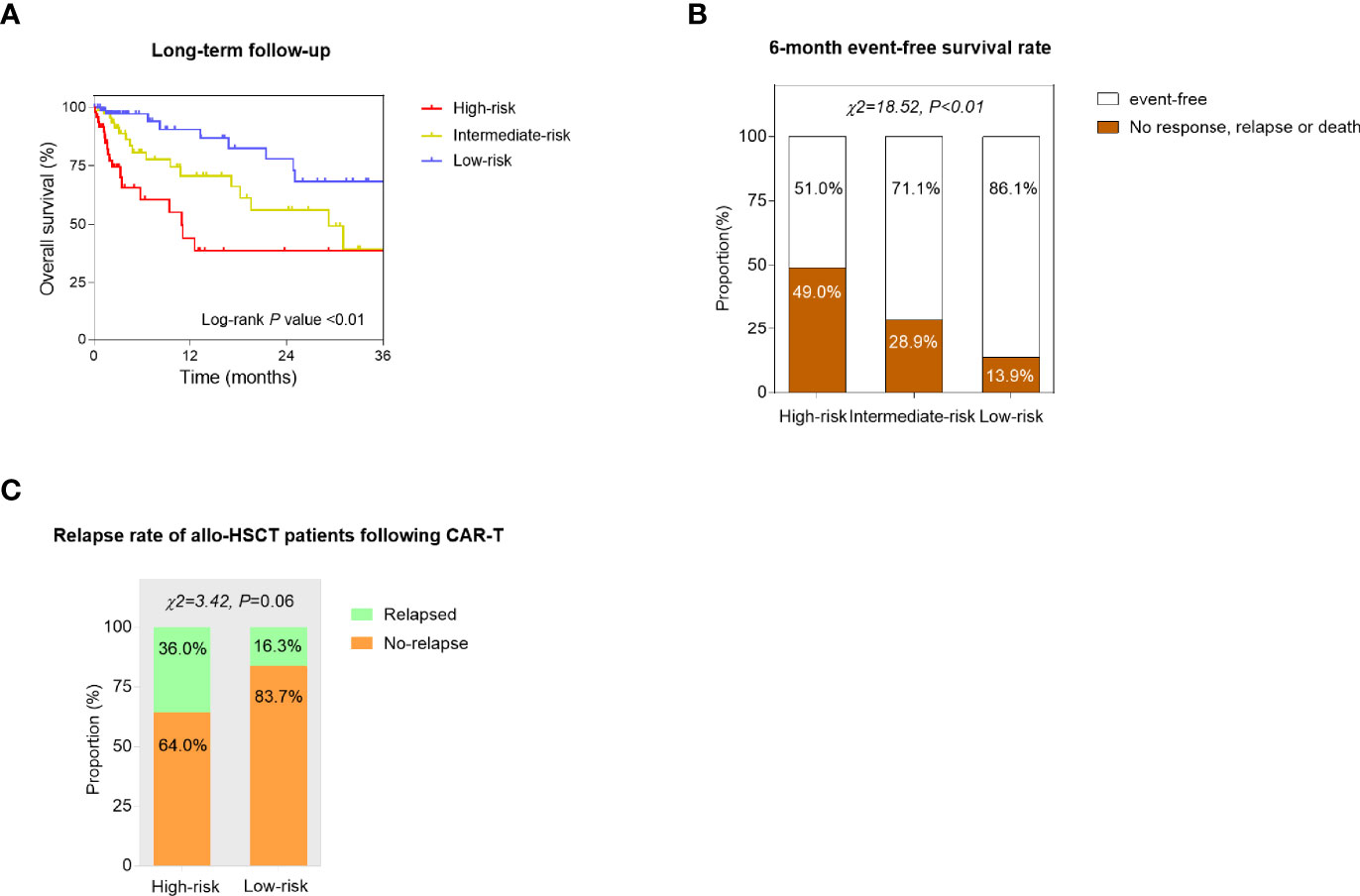
Figure 4 Confirmation of the prognostic value of the constructed models. (A) Kaplan–Meier curves of the high-risk, intermediate-risk, and low-risk r/r B-ALL patients. The overall survival (OS) was compared by the log-rank test. (B) The 6-month event-free survival (EFS) rate was compared among high-risk, intermediate-risk, and low-risk groups using the chi-square (χ2) test. (C) The relapse rate of the patients who received allogeneic hemopoietic stem cell transplantation (allo-HSCT) following CAR-T therapy was compared between high-risk and low-risk subgroups. p values were generated from the χ2 test.
Further Validation of the Risk Model Using the Independent Patient Group
The independent validation cohort consisted of 82 r/r B-ALL patients enrolled during a subsequent period of time. 82 patients had 84 infusions altogether (2 patients had twice). The baseline characteristics of the validation group are shown in Supplementary Table 3. According to the established predictive models for CR and MRD-negative CR, each time of CAR-T cell infusion could get an X and Y value. Then, based on the final risk model, all the 84 infusions (as the final validation dataset) could be stratified into high-risk, intermediate-risk, and low-risk groups (Figure 5A). The lower risk series showed higher CR and MRD-negative rates after CAR-T therapy, and the p value was statistically significant (p < 0.01, Figures 5B, C).
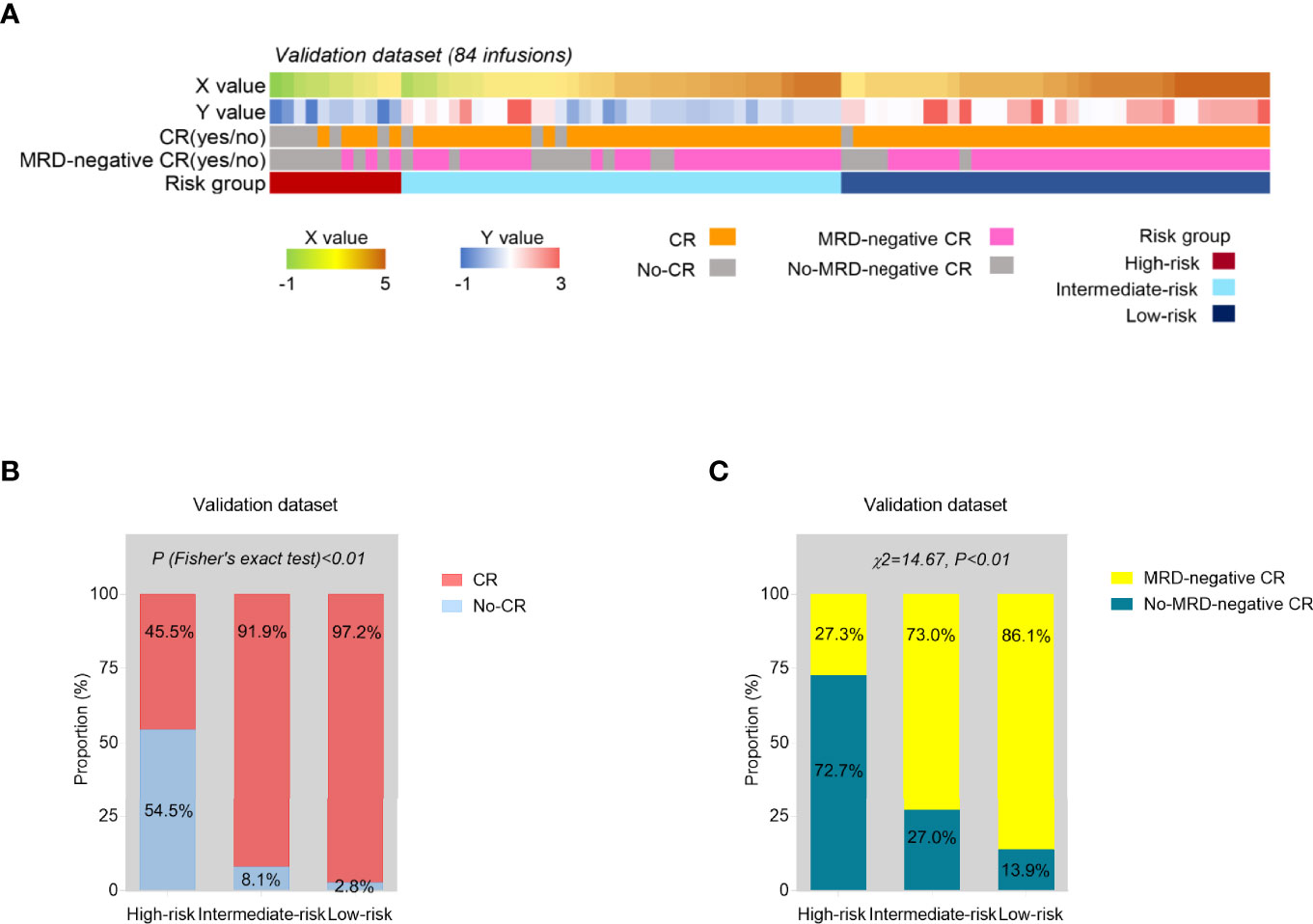
Figure 5 Internal validation of the risk model. (A) The predicted probability score of CR (X) and MRD-negative CR (Y) and risk group based on the constructed models, and the actual treatment response of each time of CAR-T cell infusion in the validation series (N = 84). (B, C) The proportions of CR (B) and MRD-negative CR (C) infusions were compared among three risk groups from the validation set. p values were calculated via Fisher’s exact test (B) and chi-square (χ2) test (C).
Discussion
In this study, we constructed the risk model of both predictive and prognostic values in r/r B-ALL patients receiving CAR-T therapy. Our final model integrated two independent predicting models for CR and MRD-negative CR as a second modification which can be expected of better predictive efficiency. Whether the integration model or the independent models were easy to use because all used variables could be conveniently obtained before the treatment, as they were all routine tests and baseline clinical data. Moreover, the contribution of each variable to the whole model could be quickly calculated according to their coefficients in the formulas which greatly increased its clinical applicability as well. Using the models, r/r B-ALL patients could be stratified into different risk groups, predicted of the treatment response in advance of CAR-T cell infusion, and provided with personalized therapeutic advice.
Consistent with the previous studies, our results also showed that high tumor burden especially marrow disease burden was related to poor treatment response of CAR-T therapy in r/r B-ALL patients (17, 19, 29). Multivariate analytic results demonstrated that the number of bone marrow blasts before lymphodepletion or CAR-T cell infusion was the independent factor of both CR and MRD-negative CR. The other direct marker of tumor burden, the number of WBC in peripheral blood when newly diagnosed, was an independent factor of CR. From univariate analysis, WBC ≥ 100 × 109/l was a significant risk factor (p = 0.04) of MRD-negative CR although it was not independently associated with MRD-negative CR. Therefore, pretreatment to lower leukemia burden particularly for those high-risk patients before infusion with CAR-T cells might be a cardinal approach to improve CAR-T therapeutic effects.
In terms of the other baseline characteristics of r/r B-ALL patients, our findings showed that TP53 mutation and CNS leukemia were the independent risk factors of CR. It has been demonstrated by numerous studies that TP53 mutation was associated with unfavorable outcome of patients with various cancers including hematological malignancies (30, 31). A recent large-cohort retrospective study by Zhang et al. also reported that TP53 mutation was independently correlated with the CR rate in B-ALL patients receiving CD19 CAR-T therapy (17). A non-randomized clinical trial involving 51 r/r B-ALL participants receiving CD19 CAR-T cells showed that extramedullary disease (EMD) other than CNS leukemia was independently associated with the poor survival of B-ALL patients after CAR-T therapy (18). However, in our study, we did not find significant relations between EMD and CR or MRD-negative CR, which was in accord with the results of Professor Zhang and her colleagues’ study (17). Anyway, these risk factors might greatly influence CAR-T treatment response and r/r B-ALL patients harboring these factors were probably not the appropriate candidates for the therapy.
Interestingly, we found that disease status (relapsed or refractory) right before infusion was the independent factor of MRD-negative CR in r/r B-ALL patients. Relapsed patients had a higher probability of MRD-CR remission after CAR-T therapy than refractory ones. To the best of our knowledge, clinical and experimental evidence is lacking in comparing the treatment response of these two subgroups at present. Our findings in this study led to speculation that relapsed patients might be more sensitive to antitumor therapy while refractory patients were more easily resistant to the treatment. However, more studies are needed to validate our results and reveal molecular biological mechanisms.
With respect to the characteristics of CAR-T cells used for each infusion, our study revealed that the third and fourth generations of CAR-T cells were associated with higher probability of remission compared to the second generation. Besides, for target recognition, either dual-target infusions or sequential infusions of two single-specific CAR-T cells demonstrated better clinical response than single-target infusions. At present, dual-target infusion is considered as a promising and effective strategy for the antigen-loss relapse after single-target CAR-T cells (32). A previous meeting abstract and a case report showed that sequential infusions could significantly improve the clinical outcome of r/r B-ALL patients as well (33, 34). Recently, sequential infusion of two single-specific CAR-T cells was proposed as a cocktail therapy in r/r B-ALL patients for its proved superior efficacy and safety in the clinical trial (35), while thus far, few clinical trials comparing the efficacy of dual-target and sequential infusions have been reported. In our study, we found that patients who received sequential infusions were more likely to achieve MRD-negative CR compared to those after dual-target infusions. However, more targets or latest generation indicated higher cost of this therapy. Accordingly, in order to reduce treatment expenses of patients, prioritize medical resources, and meanwhile, improve individual prognosis, based on our models, low-risk patients are highly recommended for latest and dual-targeting or sequential infusion of CAR-T cells.
Our study has several limitations. Firstly, selection bias of the retrospective study was inevitable. Secondly, although the patients were from three clinical trials, they were treated in one similar medical center and were all Chinese. Therefore, larger patient groups, especially non-Chinese populations, from different medical centers are needed to further validate our models. Moreover, the application of our risk model was finally extended to each time of CAR-T cell infusion. However, the sample size of other infusions (not first-time infusion) was limited in this study. Thus, more real-world data of multiple CAR-T cell infusions of one single patient are need for validation as well. Besides, our models were constructed based on several common types of CAR-T cells. To further broaden the application of these models, more data of patients receiving the CAR-T cells of different targets, structures, and origins are needed to test and validate the models. Lastly, before putting into analyses, all the continuous variables were transformed into categorical ones to facilitate the application, which might simultaneously decrease the robustness of the constructed models. In spite of all the limitations, the predictive models for r/r B-ALL patients after CAR-T therapy, hereon we attempted to establish for the first time, displayed excellent clinical efficacy even in prognosis estimation.
In summary, we here developed and validated the predictive models for CAR-T therapeutic responses in r/r B-ALL patients and further confirmed the prognostic value of the risk model. The combined application of the two independent models for CR and MRD-negative CR estimation and the modified risk model can provide credible advice on the selection of the most benefited r/r B-ALL patients from this novel therapy and on the prevention treatment of these patients for clinicians and hematologists. What is more, as commercial products of CAR-T cells are increasingly reported these years, our models need to be tested and improved based on these multicenter real-world data. Nevertheless, our findings may also give clinical implications in improving CAR-T therapeutic effectiveness.
Data Availability Statement
The raw data supporting the conclusions of this article will be made available by the authors, without undue reservation.
Ethics Statement
The studies involving human participants were reviewed and approved by the Institutional Ethics Committees of the First Affiliated Hospital of Soochow University. Written informed consent to participate in this study was provided by the participants’ legal guardian/next of kin.
Author Contributions
XT, DW, and JG designed the research. JG, SL, HD, QC, JY, ZL, LK, HQ, YH, MM, SC, SX, YW, ZJ, XZ, and LY cared for the enrolled patients and conducted the medical visits. LK and LY were also responsible for the production of CAR-T cells. JG, WC, and SL collected and analyzed the patients’ data. HD, QC, JY, and ZL constructed the figures. JG and WC drafted the manuscript. XT and DW revised the manuscript. All authors contributed to the article and approved the submitted version.
Funding
This work was supported by research grants from the National Natural Science Foundation of China (81873443, 82070162, 81900175, 81400155, 81700139), Major Natural Science Research Projects in institutions of higher education of Jiangsu Province (19KJA210002), The Key Science Research Project of Jiangsu Commission of Health (K2019022), Translational Research Grant of NCRCH (2020ZKZC04), Natural Science Foundation of Jiangsu Province (BK20190181, BK20201169, BK20170360), the Frontier Clinical Technical Project of the Science and Technology Department of Jiangsu Province (BE2018652), and the Priority Academic Program Development of Jiangsu Higher Education Institutions (PAPD).
Conflict of Interest
Authors LK and LY are employed by Shanghai Unicar-Therapy Bio-Medicine Technology Co., Ltd.
The remaining authors declare that the research was conducted in the absence of any commercial or financial relationships that could be construed as a potential conflict of interest.
Publisher’s Note
All claims expressed in this article are solely those of the authors and do not necessarily represent those of their affiliated organizations, or those of the publisher, the editors and the reviewers. Any product that may be evaluated in this article, or claim that may be made by its manufacturer, is not guaranteed or endorsed by the publisher.
Acknowledgments
The authors thank the patients and their families, Uni CAR Technology Co., Ltd., Shanghai, China, and the First Affiliated Hospital of Soochow University for their strong support and close cooperation when performing this study.
Supplementary Material
The Supplementary Material for this article can be found online at: https://www.frontiersin.org/articles/10.3389/fimmu.2022.858590/full#supplementary-material
References
1. Gökbuget N, Stanze D, Beck J, Diedrich H, Horst HA, Hüttmann A, et al. Outcome of Relapsed Adult Lymphoblastic Leukemia Depends on Response to Salvage Chemotherapy, Prognostic Factors, and Performance of Stem Cell Transplantation. Blood (2012) 120:2032–41. doi: 10.1182/blood-2011-12-399287
2. Forman SJ, Rowe JM. The Myth of the Second Remission of Acute Leukemia in the Adult. Blood (2013) 121:1077–82. doi: 10.1182/blood-2012-08-234492
3. Kantarjian H, Stein A, Gökbuget N, Fielding AK, Schuh AC, Ribera JM, et al. Blinatumomab Versus Chemotherapy for Advanced Acute Lymphoblastic Leukemia. N Engl J Med (2017) 376:836–47. doi: 10.1056/NEJMoa1609783
4. O'Brien S, Thomas D, Ravandi F, Faderl S, Cortes J, Borthakur G, et al. Outcome of Adults With Acute Lymphocytic Leukemia After Second Salvage Therapy. Cancer (2008) 113:3186–91. doi: 10.1002/cncr.23919
5. Brentjens RJ, Rivière I, Park JH, Davila ML, Wang X, Stefanski J, et al. Safety and Persistence of Adoptively Transferred Autologous CD19-Targeted T Cells in Patients With Relapsed or Chemotherapy Refractory B-Cell Leukemias. Blood (2011) 118:4817–28. doi: 10.1182/blood-2011-04-348540
6. Lee DW, Barrett DM, Mackall C, Orentas R, Grupp SA. The Future Is Now: Chimeric Antigen Receptors as New Targeted Therapies for Childhood Cancer. Clin Cancer Res (2012) 18:2780–90. doi: 10.1158/1078-0432.ccr-11-1920
7. Lee DW, Kochenderfer JN, Stetler-Stevenson M, Cui YK, Delbrook C, Feldman SA, et al. T Cells Expressing CD19 Chimeric Antigen Receptors for Acute Lymphoblastic Leukaemia in Children and Young Adults: A Phase 1 Dose-Escalation Trial. Lancet (2015) 385:517–28. doi: 10.1016/s0140-6736(14)61403-3
8. Davila ML, Riviere I, Wang X, Bartido S, Park J, Curran K, et al. Efficacy and Toxicity Management of 19-28z CAR T Cell Therapy in B Cell Acute Lymphoblastic Leukemia. Sci Transl Med (2014) 6:224ra25. doi: 10.1126/scitranslmed.3008226
9. Maude SL, Frey N, Shaw PA, Aplenc R, Barrett DM, Bunin NJ, et al. Chimeric Antigen Receptor T Cells for Sustained Remissions in Leukemia. N Engl J Med (2014) 371:1507–17. doi: 10.1056/NEJMoa1407222
10. Turtle CJ, Hanafi LA, Berger C, Gooley TA, Cherian S, Hudecek M, et al. CD19 CAR-T Cells of Defined CD4+:CD8+ Composition in Adult B Cell ALL Patients. J Clin Invest (2016) 126:2123–38. doi: 10.1172/jci85309
11. Gardner RA, Finney O, Annesley C, Brakke H, Summers C, Leger K, et al. Intent-To-Treat Leukemia Remission by CD19 CAR T Cells of Defined Formulation and Dose in Children and Young Adults. Blood (2017) 129:3322–31. doi: 10.1182/blood-2017-02-769208
12. Maude SL, Laetsch TW, Buechner J, Rives S, Boyer M, Bittencourt H, et al. Tisagenlecleucel in Children and Young Adults With B-Cell Lymphoblastic Leukemia. N Engl J Med (2018) 378:439–48. doi: 10.1056/NEJMoa1709866
13. Park JH, Rivière I, Gonen M, Wang X, Sénéchal B, Curran KJ, et al. Long-Term Follow-Up of CD19 CAR Therapy in Acute Lymphoblastic Leukemia. N Engl J Med (2018) 378:449–59. doi: 10.1056/NEJMoa1709919
14. Hong M, Clubb JD, Chen YY. Engineering CAR-T Cells for Next-Generation Cancer Therapy. Cancer Cell (2020) 38:473–88. doi: 10.1016/j.ccell.2020.07.005
15. Singh AK, McGuirk JP. CAR T Cells: Continuation in a Revolution of Immunotherapy. Lancet Oncol (2020) 21:e168–78. doi: 10.1016/s1470-2045(19)30823-x
16. Lin JK, Muffly LS, Spinner MA, Barnes JI, Owens DK, Goldhaber-Fiebert JD. Cost Effectiveness of Chimeric Antigen Receptor T-Cell Therapy in Multiply Relapsed or Refractory Adult Large B-Cell Lymphoma. J Clin Oncol (2019) 71:689–703. doi: 10.1200/jco.18.02079
17. Zhang X, Yang J, Li J, Li W, Song D, Lu XA, et al. Factors Associated With Treatment Response to CD19 CAR-T Therapy Among a Large Cohort of B Cell Acute Lymphoblastic Leukemia. Cancer Immunol Immunother (2021). doi: 10.1007/s00262-021-03009-z
18. An F, Wang H, Liu Z, Wu F, Zhang J, Tao Q, et al. Influence of Patient Characteristics on Chimeric Antigen Receptor T Cell Therapy in B-Cell Acute Lymphoblastic Leukemia. Nat Commun (2020) 11:5928. doi: 10.1038/s41467-020-19774-x
19. Hay KA, Gauthier J, Hirayama AV, Voutsinas JM, Wu Q, Li D, et al. Factors Associated With Durable EFS in Adult B-Cell ALL Patients Achieving MRD-Negative CR After CD19 CAR T-Cell Therapy. Blood (2019) 133:1652–63. doi: 10.1182/blood-2018-11-883710
20. Shah NN, Fry TJ. Mechanisms of Resistance to CAR T Cell Therapy. Nat Rev Clin Oncol (2019) 16:372–85. doi: 10.1038/s41571-019-0184-6
21. Mueller KT, Maude SL, Porter DL, Frey N, Wood P, Han X, et al. Cellular Kinetics of CTL019 in Relapsed/Refractory B-Cell Acute Lymphoblastic Leukemia and Chronic Lymphocytic Leukemia. Blood (2017) 130:2317–25. doi: 10.1182/blood-2017-06-786129
22. Döhner H, Estey E, Grimwade D, Amadori S, Appelbaum FR, Büchner T, et al. Diagnosis and Management of AML in Adults: 2017 ELN Recommendations From an International Expert Panel. Blood (2017) 129:424–47. doi: 10.1182/blood-2016-08-733196
23. Tessler MJ, Charland L, Wang NN, Correa JA. The Association of Time of Emergency Surgery - Day, Evening or Night - With Postoperative 30-Day Hospital Mortality. Anaesthesia (2018) 73:1368–71. doi: 10.1111/anae.14329
24. Hua J, Zhang J, Zhang X, Wu X, Zhou L, Bao X, et al. Donor-Derived Anti-CD19 CAR T Cells Compared With Donor Lymphocyte Infusion for Recurrent B-ALL After Allogeneic Hematopoietic Stem Cell Transplantation. Bone Marrow Transplant (2021) 56:1056–64. doi: 10.1038/s41409-020-01140-6
25. Yang X, Dai H, Kang L, Qu C, Li Z, Yin J, et al. Donor Origin CAR19 T Cell Infusion for B-ALL Relapsed After Allogeneic Hematopoietic Stem Cell Transplantation. Hematol Oncol (2019) 37:655–8. doi: 10.1002/hon.2682
26. Lee DW, Gardner R, Porter DL, Louis CU, Ahmed N, Jensen M, et al. Current Concepts in the Diagnosis and Management of Cytokine Release Syndrome. Blood (2014) 124:188–95. doi: 10.1182/blood-2014-05-552729
27. Lee DW, Santomasso BD, Locke FL, Ghobadi A, Turtle CJ, Brudno JN, et al. ASTCT Consensus Grading for Cytokine Release Syndrome and Neurologic Toxicity Associated With Immune Effector Cells. Biol Blood Marrow Transplant (2019) 25:625–38. doi: 10.1016/j.bbmt.2018.12.758
28. Zhang C, Wang XQ, Zhang RL, Liu F, Wang Y, Yan ZL, et al. Donor-Derived CD19 CAR-T Cell Therapy of Relapse of CD19-Positive B-ALL Post Allotransplant. Leukemia (2021) 35:1563–70. doi: 10.1038/s41375-020-01056-6
29. Zhang X, Lu XA, Yang J, Zhang G, Li J, Song L, et al. Efficacy and Safety of Anti-CD19 CAR T-Cell Therapy in 110 Patients With B-Cell Acute Lymphoblastic Leukemia With High-Risk Features. Blood Adv (2020) 4:2325–38. doi: 10.1182/bloodadvances.2020001466
30. Stengel A, Kern W, Haferlach T, Meggendorfer M, Fasan A, Haferlach C. The Impact of TP53 Mutations and TP53 Deletions on Survival Varies Between AML, ALL, MDS and CLL: An Analysis of 3307 Cases. Leukemia (2017) 31:705–11. doi: 10.1038/leu.2016.263
31. Stengel A, Schnittger S, Weissmann S, Kuznia S, Kern W, Kohlmann A, et al. TP53 Mutations Occur in 15.7% of ALL and Are Associated With MYC-Rearrangement, Low Hypodiploidy, and a Poor Prognosis. Blood (2014) 124:251–8. doi: 10.1182/blood-2014-02-558833
32. Ruella M, Barrett DM, Kenderian SS, Shestova O, Hofmann TJ, Perazzelli J, et al. Dual CD19 and CD123 Targeting Prevents Antigen-Loss Relapses After CD19-Directed Immunotherapies. J Clin Invest (2016) 126:3814–26. doi: 10.1172/jci87366
33. Liu SY, Deng BP, Lin YH, Yin ZC, Pan J, Wu T, et al. Sequential CD19-And CD22-CART Cell Therapies for Relapsed B-Cell Acute Lymphoblastic Leukemia After Allogeneic Hematopoietic Stem Cell Transplantation. Blood (2018) 132(Supplement 1):2126–2126. doi: 10.1182/blood-2018-99-111856
34. Hua J, Qian W, Wu X, Zhou L, Yu L, Chen S, et al. Sequential Infusion of Anti-CD22 and Anti-CD19 Chimeric Antigen Receptor T Cells for a Pediatric Ph-Like B-ALL Patient That Relapsed After CART-Cell and Haplo-HSCT Therapy: A Case Report and Review of Literature. Onco Targets Ther (2020) 13:2311–7. doi: 10.2147/ott.s235882
Keywords: chimeric antigen receptor T cells, refractory or relapsed B-cell acute lymphoblastic leukemia, predictive models, complete remission, MRD-negative complete remission
Citation: Gu J, Liu S, Cui W, Dai H, Cui Q, Yin J, Li Z, Kang L, Qiu H, Han Y, Miao M, Chen S, Xue S, Wang Y, Jin Z, Zhu X, Yu L, Wu D and Tang X (2022) Identification of the Predictive Models for the Treatment Response of Refractory/Relapsed B-Cell ALL Patients Receiving CAR-T Therapy. Front. Immunol. 13:858590. doi: 10.3389/fimmu.2022.858590
Received: 20 January 2022; Accepted: 21 February 2022;
Published: 17 March 2022.
Edited by:
Wei Sang, The Affiliated Hospital of Xuzhou Medical University, ChinaReviewed by:
Kourong Miao, Nanjing Medical University, ChinaXiao-Dong Mo, Peking University People’s Hospital, China
Heng Mei, Huazhong University of Science and Technology, China
Copyright © 2022 Gu, Liu, Cui, Dai, Cui, Yin, Li, Kang, Qiu, Han, Miao, Chen, Xue, Wang, Jin, Zhu, Yu, Wu and Tang. This is an open-access article distributed under the terms of the Creative Commons Attribution License (CC BY). The use, distribution or reproduction in other forums is permitted, provided the original author(s) and the copyright owner(s) are credited and that the original publication in this journal is cited, in accordance with accepted academic practice. No use, distribution or reproduction is permitted which does not comply with these terms.
*Correspondence: Xiaowen Tang, eHd0YW5nMTAyMEAxNjMuY29t; Depei Wu, ZHJ3dWRlcGVpQDE2My5jb20=; d3VkZXBlaUBzdWRhLmVkdS5jbg==
†These authors share first authorship