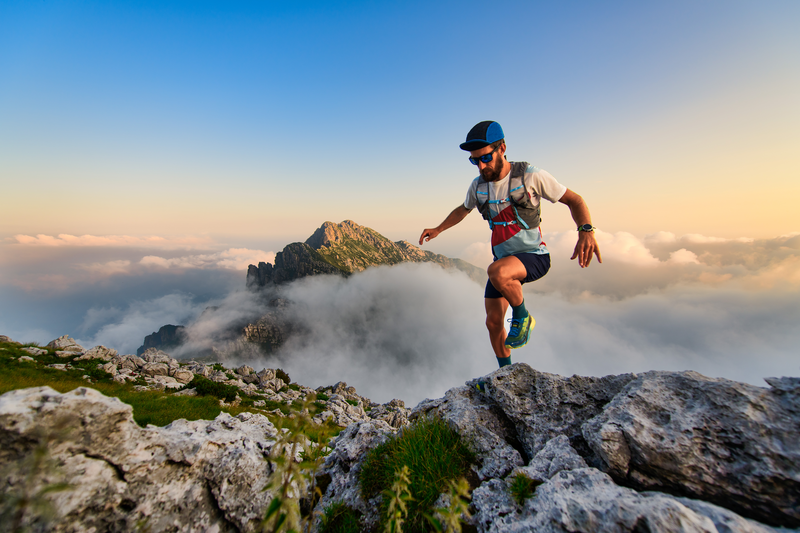
94% of researchers rate our articles as excellent or good
Learn more about the work of our research integrity team to safeguard the quality of each article we publish.
Find out more
ORIGINAL RESEARCH article
Front. Immunol. , 20 May 2022
Sec. Cancer Immunity and Immunotherapy
Volume 13 - 2022 | https://doi.org/10.3389/fimmu.2022.849468
This article is part of the Research Topic Combining Chemo/radio Therapy and Immunotherapy for Cancers—Perfect Mix of Old and New View all 49 articles
Objective: This study aims to investigate the potential prognostic significance of programmed death ligand-1 (PD-L1) protein expression in tumor cells of breast cancer patients received neoadjuvant chemotherapy (NACT).
Methods: Using semiquantitative immunohistochemistry, the PD-L1 protein expression in breast cancer tissues was analyzed. The correlations between PD-L1 protein expression and clinicopathologic characteristics were analyzed using Chi-square test or Fisher’s exact test. The survival curve was stemmed from Kaplan-Meier assay, and the log-rank test was used to compare survival distributions against individual index levels. Univariate and multivariate Cox proportional hazards regression models were accessed to analyze the associations between PD-L1 protein expression and survival outcomes. A predictive nomogram model was constructed in accordance with the results of multivariate Cox model. Calibration analyses and decision curve analyses (DCA) were performed for the calibration of the nomogram model, and subsequently adopted to assess the accuracy and benefits of the nomogram model.
Results: A total of 104 breast cancer patients received NACT were enrolled into this study. According to semiquantitative scoring for IHC, patients were divided into: low PD-L1 group (61 cases) and high PD-L1 group (43 cases). Patients with high PD-L1 protein expression were associated with longer disease free survival (DFS) (mean: 48.21 months vs. 31.16 months; P=0.011) and overall survival (OS) (mean: 83.18 months vs. 63.31 months; P=0.019) than those with low PD-L1 protein expression. Univariate and multivariate analyses indicated that PD-L1, duration of neoadjuvant therapy, E-Cadherin, targeted therapy were the independent prognostic factors for patients’ DFS and OS. Nomogram based on these independent prognostic factors was used to evaluate the DFS and OS time. The calibration plots shown PD-L1 based nomogram predictions were basically consistent with actual observations for assessments of 1-, 3-, and 5-year DFS and OS time. The DCA curves indicated the PD-L1 based nomogram had better predictive clinical applications regarding prognostic assessments of 3- and 5-year DFS and OS, respectively.
Conclusion: High PD-L1 protein expression was associated with significantly better prognoses and longer DFS and OS in breast cancer patients. Furthermore, PD-L1 protein expression was found to be a significant prognostic factor for patients who received NACT.
Breast cancer (BC) is one of the most common aggressive human cancers in the clinical setting, and is the primary cause of morbidity and mortality in women across the world (1). Due to a dearth of research, previously, breast cancer has been wrongly categorized as a non-immunogenic cancer (2). However, accumulating evidence continues to indicate otherwise, ranging from the presence of adaptive immune response that regulates breast cancer growth to the observance of a large number of heavy tumors infiltrated immune cells (3). Currently, treatment strategies for breast cancer, ranging from operation, chemotherapy, radiotherapy, endocrine therapy to targeted therapy, are commonly used in clinic and have definite curative effect (4, 5). Nowadays, cancer immunotherapy has become the new pillar of breast cancer treatment, and its use is approved for integrating with chemotherapy for first-line therapy (6, 7).
Immune checkpoint inhibitors (ICI), for instance, programmed cell death 1 (PD-1), programmed cell death ligand-1 (PD-L1), cytotoxic T lymphocyte antigen 4 (CTLA-4) have shown notably promise for the treatment of various cancers (8). And the crucial changes in these immune cells in cancerous tumors may contribute to the forecasting of the prognosis of cancer patients. As is known to all, PD-1 and PD-L1 are momentous immune checkpoint components that essentially regulate the function of tumor-infiltrating lymphocytes (TILs) and tumor cells (9). PD-1 is a type I transmembrane glycoprotein belonging to the immunoglobulin CD28 superfamily (10). As a cell surface receptor, PD-1 is predominantly expressed on the cell surface of T cells, B cells, Natural killer (NK) cells, monocytes, dendritic cells (DCs), tumor cells (11). Furthermore, PD-1 can negatively regulate the activity of T cells via interacting with its ligands PD-L1 (B7-H1) and PD-L2 (B7-DC) expressing on immune cells and tumor cells at some steps of the immune response (12). PD-L1 is a type I transmembrane glycoprotein, and is mainly expressed on the cell surface of T cells, B cells, DCs, macrophages, and tumor cells (13). Through binding PD-1, PD-L1 induces activated anti-tumor T cells, and plays a major role in the inhibition of T cell-mediated immune response (14). PD-1 and PD-L1 interact with each other attenuating local immune responses and shielding tumor cells from T cell-mediated killing (15).
Recently, the PD-1/PD-L1 pathway regulates tumor microenvironment through the induction and maintenance of immune tolerance, and PD-1 and PD-L1 have been proved to be promising targets for the treatment of a large number of tumor types, such as hepatocellular carcinoma (HCC), pancreatic cancer, gastric neuroendocrine carcinomas, thymic carcinoma, non-small-cell lung cancer (NSCLC) (16–20). One study from Europe found that PD-1 positive immune cells correlated with longer disease free survival time in triple negative breast cancer (TNBC), and the density of TILs was notably associated with PD-1 and PD-L1 expression in immune cells (21). Research also indicated that PD-L1 was markedly enriched in basal-like breast cancer and was correlated with infiltrating lymphocytes, and improved disease-specific survival time in ER-negative disease (22). Moreover, another study performed that a positive correlation of CD8-positive T cells and PD-L1 expression in HER2-positive breast carcinoma patients, and might predict a favorable survival outcome (23).
However, the role of PD-L1 in breast cancer oncogenesis and treatment is still quite obscure currently. Against this backdrop, the potential clinical significance of PD-L1 protein expression in breast cancer patients who underwent neoadjuvant chemotherapy (NACT) have been very scarcely studied. Here, we aimed to identify the potential predictive and prognostic value of PD-L1 protein expression in breast cancer received NACT, and evaluate this would be useful as a predictor for estimating treatment response.
In the current study, we retrospectively enrolled 104 patients with breast cancer for whom formalin fixed paraffin embedded (FFPE) tissue specimens and 65 FFPE matching non-neoplastic background tissues had been handed in for safekeeping in Cancer Hospital Chinese Academy of Medical Sciences in China. All enrolled patients had complete clinical and demographic data from medical records, and were confirmed by histopathology as breast carcinoma. After NACT treatment, all patients have already undergone relevant surgeries, operations such as mastectomy and breast-conserving procedures.
The study involving human participants was reviewed and approved by the ethics committee of Cancer Hospital Chinese Academy of Medical Sciences. All processes performed in studies involving human participants were consistent with the standards of the institutional research committee and with the declaration of 1964 Helsinki as well as its later amendments or comparable ethical standards. The patients provided their written informed consent to participate in this study.
All patients underwent curative operation involving a mastectomy or breast-conserving surgery with axillary lymph node dissection (ALND) after NACT. The breast cancer tissues and adjacent normal breast tissues were fixed in methanol, embedded in paraffin, sectioned, and performed immunohistochemical analyses. According to the instructions of the manufacturer, the immunohistochemical staining was performed using a two-step kit, details of which have been described in our previously study (24). The PD-L1 levels were detected using the primary monoclonal antibody directed against PD-L1 (GB14132, dilution 1:500, Servicebio, China).
Patients’ PD-L1 protein expressions were measured by a semiquantitative scoring method. The arrays were scanned by the Aperio Image Scope system (Leica Biosystems, United States). Histological analyses were performed by combining the density and intensity of positive staining cells. The density classification of positive cells was itemized here below: 1) 0, the number of positive cells < 5%; 2) 1, the number of positive cells 5-25%; 3) 2, the number of positive cells 26-50%; 4) 3, the number of positive cells 51-75%; 5) 4, the number of positive cells > 75%. The intensity of positive staining cells was itemized here below: 1) 0, absence of staining; 2) 1, light yellow staining; 3) 2, brownish yellow staining; 4) 3, brownish brown staining. The immunoreactivity of the PD-L1 proteins were scored on the basis of the intensity and density of positive stained cells, and the scores were calculated and assessed by two independent investigators. All specimens were examined and evaluated by two investigators blinded to the clinical information of the patients.
All enrolled patients had routine follow-ups (e.g., outpatient, inpatient, or telephone consultations). Follow-up evaluations were performed every 3 months for the first to the second year after receiving the operation, every 6 months for the third to the fifth year after the operation, then yearly thereafter. disease free survival (DFS) was defined as the time from surgery to progression with regard to the distant disease metastasis, death from any cause or last follow-up. Overall survival (OS) was defined as the time from surgery to the date of death from any cause or last follow-up.
All statistical analyses were performed using the SPSS software (version 17.0; SPSS Inc., Chicago, IL, USA), GraphPad Prism software (version 8.0; GraphPad Inc., La Jolla, CA, USA), and R (version 3.6.0; Vienna, Austria. URL: http://www.R-project.org/). The correlations between PD-L1 protein expression and clinicopathologic characteristics were presented as absolute values and percentages (%), and tested using the Chi-square test or Fisher’s exact test. The survival curve was developed utilizing the Kaplan-Meier method, the log-rank test was used to compare survival distributions of individual index levels. Univariate and multivariate Cox proportional hazards regression models were accessed to analyze the associations between PD-L1 protein expression and survival outcomes. A predictive nomogram model was constructed in accordance with the results of the multivariate Cox model. Calibration analyses and decision curve analyses (DCA) were performed for the calibration of the nomogram model, and subsequently adopted to assess the accuracy and benefits of the nomogram model. Two-sided P values less than 0.05 were considered statistically significant.
PD-L1 was mainly expressed on the cytoplasm or membranous of tumor cells. According to the semiquantitative scoring method for IHC scores, we chose the median value of the IHC scores as the cutoff value. And these patients were subsequently divided into two groups, i.e., low PD-L1 group (61 cases) vs high PD-L1 group (43 cases). The Figure S1 shown the different expression status of PD-L1 by IHC.
All cases were female, and the median age was 46 years ranging 27 to 73 years old. In terms of diagnosis, prior to NACT, 3 patients (2.9%) were diagnosed with stage I breast cancer, 39 patients (37.5%) were stage II, and 62 patients (59.6%) were stage III. Post operation, 2 patients (3.8%) were diagnosed with stage Tis/T0, 16 patients (15.4%) were stage I, 38 patients (36.5%) were stage II, and 48 patients (46.2%) were stage III. After surgery, 79 (76.0%) cases received radiotherapy, 60 (57.7%) cases had undergone endocrine therapy, 32 (30.8%) cases received targeted therapy, and 74 (71.2%) cases were undergoing chemotherapy. The basic clinicopathological features of the patients could be found in Table 1. PD-L1 was related to ABO blood type (P=0.019).
Table 1 Patients’ characteristics for all patients according to programmed cell death ligand-1 (PD-L1).
Before NACT, patients with Luminal A were 8 cases (7.7%), Luminal B HER2 (+) were 14 cases (13.5%), Luminal B HER2 (-) were 35 cases (33.7%), HER2-enriched were 15 cases (14.4%), triple-negative were 32 cases (30.8%). After operation, patients with Luminal A were 17 cases (16.3%), Luminal B HER2 (+) were 9 cases (8.7%), Luminal B HER2 (-) were 23 cases (22.1%), HER2-enriched were 18 cases (17.3%), triple-negative were 37 cases (35.6%). However, no significant correlations between PD-L1 protein expression and pathology parameters were found (P>0.05). The detail information was shown in Table 2.
Table 2 Patients’ pathology parameters for all patients according to programmed cell death ligand-1 (PD-L1).
All patients were received NACT, and the effect of chemotherapy was determined after two cycles. The clinical response was assessed according to the Response Evaluation Criteria in Solid Tumors (RECIST) guidelines (25). 104 patients responded to NACT, including 60 cases (57.7%) partial responses (PRs), 43 cases achieved stable disease (SD) and one case had progressive disease (PD). The pathological response to chemotherapy was assessed by Miller-Payne grade (MPG) (a five-point histological grading system) (26). Nine patients (8.7%) were Grade 1 response, 42 patients (40.4%) were Grade 2 response, 48 patients (46.2%) were Grade 3 response, one patient (1.0%) was Grade 4 response, 4 patients (3.8%) were Grade 5 response. However, no significant correlations between PD-L1 protein expression and clinical response and pathological response were found (P>0.05). A summary of the patients’ chemotherapy results could be found in Table 3.
Table 3 Patients’ chemotherapy for all patients according to programmed cell death ligand-1 (PD-L1).
The common adverse events (AEs) (any-grade) during the NACT period were gastrointestinal reactions (included decreased appetite, nausea, vomiting, diarrhea, mouth ulcers, alopecia, peripheral neurotoxicity), hematologic reactions (anemia, leukopenia, neutropenia, thrombocytopenia), myelosuppression, and hepatic dysfunction. However, no significant correlations between PD-L1 and side effects of chemotherapy were found (P>0.05). The side effects of chemotherapy experienced by the patients could be found in Table 4.
Table 4 Patients’ side effects of chemotherapy for all patients according to programmed cell death ligand-1 (PD-L1).
Through PD-L1 protein expression on tumor cells, the DFS and OS were compared separately. The mean DFS and OS levels for patients in the low PD-L1 group were 31.16 months (range from 4.67 to 85.07 months) and 63.31 months (range from 6.43 to 133.60 months), while the mean DFS and OS values for patients in the high PD-L1 group were 48.21 months (range from 10.17 to 107.80 months) and 83.18 months (range from 14.47 to 148.00 months), respectively. Patients with high PD-L1 protein expression revealed significantly better DFS and OS than those with low PD-L1 protein expression (χ2 = 6.440, P=0.011; χ2 = 5.483, P=0.019; see in Figures 1A, B).
Figure 1 Kaplan-Meier curves for disease free survival (DFS) and overall survival (OS). (A) Kaplan-Meier curves for DFS for PD-L1 protein expression in tumor cells; (B) Kaplan-Meier curves for OS for PD-L1 protein expression in tumor cells.
According to the Cox proportional-hazards models for DFS, the univariate analysis suggested that PD-L1, tumor site, neoadjuvant chemotherapy regimen, duration of neoadjuvant therapy, pathology (ER, Ki-67, E-cad), postoperative endocrine therapy, and targeted therapy were related to the prognosis of breast cancer patients, however, the multivariate analysis found that only PD-L1, duration of neoadjuvant therapy, pathology E-cad, targeted therapy were the independent prognostic factors (Table 5).
Table 5 Univariate and multivariate Cox proportional hazards regression model for disease free survival (DFS) and overall survival (OS).
Through the Cox proportional-hazards models for OS, the univariate analysis indicated that PD-L1, BMI, family history, tumor site, neoadjuvant chemotherapy regimen, duration of neoadjuvant therapy, MPG, positive lymph node (PLN), pathology (ER, Ki-67, CK5/6, E-cad, EGFR, P53), postoperative chemotherapy, postoperative endocrine therapy, targeted therapy were related to the prognosis of breast cancer patients, however, the multivariate analysis found that only PD-L1, duration of neoadjuvant therapy, MPG, PLN, pathology E-cad, targeted therapy were the independent prognostic factors (Table 5).
As shown in Table 5 univariate and multivariate analyses, the PD-L1 protein expression in tumor cells was related to favorable DFS (HR=0.648, 95%CI: 0.442-0.952, P=0.027; HR=0.605, 95%CI: 0.411-0.891, P=0.011) and OS (HR=0.573, 95%CI: 0.335-0.979, P=0.042; HR=0.528, 95%CI: 0.359-0.777, P=0.001) survival of breast cancer patients.
Nomograms were supposed to be an uncomplicated tool to provide personality risk assessment for each patient (27). We constructed an effective and novel nomogram for individualized assessment of DFS and OS after NACT and surgery. In accordance with the independent prognostic factors identified in the Cox proportional-hazards models, a nomogram was developed to predict the DFS probability of breast cancer at 1-, 3-, and 5- year after radical surgery, and OS probability of breast cancer at 1-year, 3-year, 5-year, and 10-year after radical surgery.
The nomogram for DFS had unique features, and integrated PD-L1, duration of neoadjuvant therapy, pathology E-cad, targeted therapy (Figure 2A). The nomogram for OS had distinguishing characteristics, including PD-L1, duration of neoadjuvant therapy, MPG, PLN, pathology E-cad, targeted therapy was an independent prognostic factor (Figure 2B).
Figure 2 PD-L1-based nomogram for predicting disease free survival (DFS) and overall survival (OS). A straight upward line is drawn to determine the points for every predictor. The sum of these points is situated on the total points axis, and a straight downward line shows the 1-year, 3-year, 5-year DFS estimated rates and 1-year, 3-year, 5-year, 10-year OS estimated rates. (A) PD-L1 based nomogram for predicting disease free survival (DFS); (B) PD-L1 based nomogram for predicting and overall survival (OS). E-cad, E-Cadherin; PLN, positive axillary lymph node.
Calibration curves (1000 bootstrap resamples) were established to check the concordance between the nomogram predicted and the actual probability of DFS and OS. The calibration plots for postoperative 1-year, 3-year, 5-year DFS survival indicated that PD-L1 based nomogram predictions were basically consistent with actual observations, especially in 5-year DFS survival (Figures 3A–C). The calibration plots for postoperative 1-year, 3-year, 5-year OS survival shown that PD-L1 based nomogram predictions were basically consistent with actual observations, especially in 3-year OS survival (Figures 3D–F). However, the calibration plots for postoperative 10-year OS survival revealed that PD-L1 based nomogram predictions were not well consistent with actual observations (Figure 3G).
Figure 3 The calibration curves for predicting the 1-year, 3-year, 5-year DFS rate and 1-year, 3-year, 5-year, 10-year OS rates in patients with breast cancer who underwent NACT. The X-axis presents the nomogram-predicted probability of disease free survival (DFS) and overall survival (OS); the Y-axis shows the actual DFS and OS. (A) The calibration curves for predicting the 1-year DFS rate in patients with breast cancer; (B) The calibration curves for predicting the 3-year DFS rate in patients with breast cancer; (C) The calibration curves for predicting the 5-year DFS rate in patients with breast cancer; (D) The calibration curves for predicting the 1-year OS rate in patients with breast cancer; (E) The calibration curves for predicting the 3-year OS rate in patients with breast cancer; (F) The calibration curves for predicting the 5-year OS rate in patients with breast cancer; (G) The calibration curves for predicting the 10-year OS rate in patients with breast cancer.
The decision curve analysis (DCA) was conducted to evaluate the clinical usefulness of the DFS and OS nomogram by quantifying the net benefits at different threshold probabilities. DCA was performed to compare the clinical usability and benefits of the 3-year, 5-year DFS and OS nomogram with that of the PD-L1. The DCA curves indicated that the nomogram 3-year, 5-year DFS and OS had better predictive clinical application than PD-L1 (Figure 4).
Figure 4 Decision curve analysis (DCA) of the nomograms and PD-L1 for predicting the disease free survival (DFS) and overall survival (OS). The X-axis represents threshold probability, and the Y-axis shows net benefit. The lines between the X-axis and the Y-axis display the benefit of different predictive variables. The red dotted line suggested that no patient has a poor prognosis, while the red line indicated that all patients have a poor prognosis. (A) DCA of the nomograms and PD-L1 for predicting the 3-year DFS; (B) DCA of the nomograms and PD-L1 for predicting the 5-year DFS; (C) DCA of the nomograms and PD-L1 for predicting the 3-year OS; (D) DCA of the nomograms and PD-L1 for predicting the 5-year OS.
There is growing evidence that the immune response status may be a critical determinant of influencing cancer progression and metastasis (28). It is universally acknowledged that anomalous immunosurveillance and immune escape of tumor cells play an important role in influencing antitumor immune response, aggressive growth and carcinogenesis of cancer cells (2, 29). Tumor tissue is not only composed of cancer cells, but also includes inflammatory cells, blood vessels, immune cells, fibrous tissue; and these components constitute a characteristic tumor immune microenvironment (TIME). Moreover, the TIME affects the prognosis and effectiveness of treatment, such as immunotherapy, and response to other treatments (30). That is to say, evaluating the TIME in each individual patient is helpful to predict the treatment response to different treatment patterns and anti-cancer drugs. In recent years, studies have been demonstrated that cancer immunotherapy is a major emerging treatment for breast cancer in clinical practice, following operation, chemotherapy, endocrine therapy, and radiotherapy (31).
The PD-1 and PD-L1 are two of the most critical biological inhibitors that allow cancer cells to escape host immunity. Blocking antibodies against PD-1 and PD-L1 can lead to local control and persistent response in patients with various tumors who are ineffective to standard treatment. The PD-1/PD-L1 pathway is also expected to effectively reverse the immunosuppression in tumors’ microenvironments (17). Thus, in light of the promising potential of the anti-PD-1 or anti-PD-L1 immunotherapy in cancer treatments (32), it is of vital importance to gain an in-depth and comprehensive understanding of the regulatory mechanisms of PD-1 and PD-L1 in carcinogenesis, progression, and metastasis. Previous studies have shown that PD-1 and PD-L1 protein expression were associated with the prognosis in different malignant tumors (33–35). However, due to significant limitations of these studies (e.g., Ahmed FS’s study (36), Wesolowski R’s study (37), and Loibl S’s study (38)), research into the potential significance of PD-1 and PD-L1 protein expression in breast cancer patients treated with NACT was often considered flawed or controversial. Therefore, in light of their research and practical significance, to bridge the research gap, the present study investigated the PD-L1 protein expression in post-NACT patients’ tumor tissues and examined the relationship between the PD-L1 protein expression and patients’ treatment efficacy.
The present study assessed how the protein expression of PD-L1 immune checkpoints affect the responses of breast cancer patients to NACT. By using IHC assay, we demonstrated that high PD-L1 protein expression at the protein level were related to better prognoses in breast cancer patients. Matikas A and associates found that PD-1 protein and gene expression seem to be promising prognostic factors in early breast cancer, and PD-L1 gene expression is a promising prognostic factor, especially in basal-like breast cancer (39, 40). Through the IHC score by semiquantitative scoring method, all patients were classified as two groups. High PD-L1 protein expression were associated with better prognosis and significantly longer DFS and OS, respectively. Patients with high PD-L1 protein expression had long-term DFS and OS survival, and PD-L1 protein expression was an independent prognostic factor. In Botti G’s study, PD-L1 protein expression was strongly correlated with better DFS, yet not associated with OS; and PD-L1 could be an important marker for prognostic stratification and planning immune checkpoint inhibitor treatment in TNBC patients (41). Other study shown that patients with PD-L1 positive expression in tumor cells had better good recurrence-free survival (RFS) and OS than those with PD-L1 negative expression in tumor cells; and PD-L1 expression was found to be an independent marker for favorable RFS and OS in TNBC patients (42). Another study on analyzing transcriptional data of breast cancer from TCGA, PD-1 and PD-L1 gene expression were associated with immune infiltration and immune checkpoints; and PD-1 expression was associated with favorable survival of breast cancer patients (43). These literatures were consistent with our study. However, in Asano Y’s study, high PD-1 and PD-L1 protein expression was associated with a poorer prognosis in breast cancer patient before undergoing NACT, and low PD-1 and PD-L1 protein expression in TNBC patients was be bound up with a higher pCR rate and significantly longer DFS (44). And Chen’s study indicated that high expression of PD-L1 had a bearing on worse survival in breast cancer patients after NACT, and was used as a prognostic marker in non-pCR patients (45). Thus, due to conflicting research findings in the literature, it is still unclear whether PD-L1 expression could accurately predict the prognosis of breast cancer. This might explain the results from our study: 1) the expression of PD-L1 might be related to TIL-mediated antitumor inflammatory response, and rather than tumor immune escape; 2) the expression of PD-L1 varied in different molecular types of breast cancer; 3) the expression of PD-L1 were at the protein level rather than at the mRNA level.
In this study, breast cancer patients were divided into low expression and high expression groups according to their IHC scores. We analyzed the relationship between PD-L1 with the patients’ characteristics, and the results revealed that PD-L1 was associated with ABO blood type. In Iwasaki K’s study, the PD-L1 expression was related to the ABO-I renal transplants when compared with those from ABO-identical/compatible transplants (46). Moreover, the results also indicated that no significant difference between PD-L1 and pathology parameters. Furthermore, our results also showed that there was no significant difference between PD-L1 and patients’ chemotherapy or side effects of chemotherapy.
The univariate and multivariate analyses revealed that PD-L1, duration of neoadjuvant therapy, pathology E-cad, targeted therapy were the independent prognostic factors for DFS; and PD-L1, duration of neoadjuvant therapy, MPG, PLN, pathology E-cad, targeted therapy were the independent prognostic factors for OS. Thus, we constructed a new nomogram based on the independent prognostic factors to evaluate the DFS and OS survival in breast cancer received NACT. And the calibration plots for postoperative 1-year, 3-year, 5-year DFS and OS survival shown that PD-L1 based nomogram predictions were basically consistent with actual observations, especially in 5-year DFS survival and 3-year OS survival. Moreover, the DCA curves indicated that the PD-L1 score-based nomogram offers prognostic assessment of 3-year, 5-year DFS and OS had better predictive clinical application after NACT, and might bring great benefits to clinical practice.
However, some limitations should be considered in our study. Firstly, this study is retrospective in nature, which means that it is vulnerable to potential selective bias. However, it is important to note that the retrospective research approach also ensures that the findings of our study are grounded in reality, as they capture and reflect the real-world experiences of actual breast cancer patients who underwent NACT in China. Secondly, it is possible that variations in the PD-L1 antibodies used, IHC scoring, and patient selection might have contributed to the high heterogeneity of the research findings. Thirdly, some potential important parameters related to clinical prognosis are not examined in the current study, and the constructed nomogram was developed based on limited independent factors. Moreover, the heterogeneity and molecular subtype of breast cancer also influence the PD-L1 expression. Finally, the nomogram was internally validated, and future studies focus on external validation using other populations.
High PD-L1 protein expressions were associated with significantly better prognoses and longer DFS and OS in breast cancer patients. Furthermore, PD-L1 protein expression was found to be a significant prognostic factor for patients who received NACT. Our study also suggested that nomogram analyses could provide more accurate individualized predictions of DFS and OS survival in patients, and in turn, have the potential to assist clinicians to make more informed decisions in clinical practice.
The original contributions presented in the study are included in the article/Supplementary Material. Further inquiries can be directed to the corresponding authors.
This study was approved by the ethics committee of Cancer Hospital Chinese Academy of Medical Sciences. The patients/participants provided their written informed consent to participate in this study.
Writing-original draft and writing-review and editing, LC, SH, and QL. Formal analysis, LC and XK. Data curation and investigation, LC and ZS. Methodology and supervision, YF and LZ. Resources, funding acquisition, and project administration, XL and JW. All authors contributed to the article and approved the submitted version.
The work is partly supported by research grants from The National Nature Science Foundation of China (No. 81872160, No. 82072940, No. 82103047, No. 82102887 and No.81802676), Beijing Nature Science Foundation of China (No. 7191009, No. 7204293), and National Key R&D Program of China (No. 2018YFC1312100), the China National Key R&D (or Research and Development) Program (No. 2020AAA0105000 and 2020AAA0105004), the Special Research Fund for Central Universities, Peking Union Medical College (No. 3332019053), the Beijing Hope Run Special Fund of Cancer Foundation of China (No. LC2020L01, No. LC2019B03, No. LC2019L07), Wuhan Youth Cadre Project (2017zqnlxr01 and 2017zqnlxr02), Clinical Research Physician Program of Tongji Medical College, HUST (5001540018), the Golden Bridge Project Seed Fund of Beijing Association for Science and Technology (No. ZZ20004), the Chinese Young Breast Experts Research project (No. CYBER-2021-005), the 2021 Chaoyang District Social Development Science and Technology Plan Project (Medical and Health Field) (No. CYSF2115), the Beijing Xisike Clinical Oncology Research Foundation (No. Y-Young2021-0017), the XianSheng Clinical Research Special Fund of China International Medical Foundation (No. Z-2014-06-2103).
The authors declare that the research was conducted in the absence of any commercial or financial relationships that could be construed as a potential conflict of interest.
All claims expressed in this article are solely those of the authors and do not necessarily represent those of their affiliated organizations, or those of the publisher, the editors and the reviewers. Any product that may be evaluated in this article, or claim that may be made by its manufacturer, is not guaranteed or endorsed by the publisher.
The Supplementary Material for this article can be found online at: https://www.frontiersin.org/articles/10.3389/fimmu.2022.849468/full#supplementary-material
Supplementary Figure 1 | Expression of PD-L1 in human breast cancer tissues.
1. Siegel RL, Miller KD, Fuchs HE, Jemal A. Cancer Statistics, 2021. CA Cancer J Clin (2021) 71(1):7–33. doi: 10.3322/caac.21654
2. Gil Del Alcazar CR, Alečković M, Polyak K. Immune Escape During Breast Tumor Progression. Cancer Immunol Res (2020) 8(4):422–7. doi: 10.1158/2326-6066.CIR-19-0786
3. Sun L, Kees T, Almeida AS, Liu B, He XY, Ng D, et al. Activating a Collaborative Innate-Adaptive Immune Response to Control Metastasis. Cancer Cell (2021) 39(10):1361–1374.e9. doi: 10.1016/j.ccell.2021.08.005
4. Pondé NF, Zardavas D, Piccart M. Progress in Adjuvant Systemic Therapy for Breast Cancer. Nat Rev Clin Oncol (2019) 16(1):27–44. doi: 10.1038/s41571-018-0089-9
5. Kawaji H, Kubo M, Yamashita N, Yamamoto H, Kai M, Kajihara A, et al. Comprehensive Molecular Profiling Broadens Treatment Options for Breast Cancer Patients. Cancer Med (2021) 10(2):529–39. doi: 10.1002/cam4.3619
6. Emens LA. Breast Cancer Immunotherapy: Facts and Hopes. Clin Cancer Res (2018) 24(3):511–20. doi: 10.1158/1078-0432.CCR-16-3001
7. Keenan TE, Tolaney SM. Role of Immunotherapy in Triple-Negative Breast Cancer. J Natl Compr Canc Netw (2020) 18(4):479–89. doi: 10.6004/jnccn.2020.7554
8. Wang X, Wang F, Zhong M, Yarden Y, Fu L. The Biomarkers of Hyperprogressive Disease in PD-1/PD-L1 Blockage Therapy. Mol Cancer (2020) 19(1):81. doi: 10.1186/s12943-020-01200-x
9. Baraibar I, Melero I, Ponz-Sarvise M, Castanon E. Safety and Tolerability of Immune Checkpoint Inhibitors (PD-1 and PD-L1) in Cancer. Drug Saf (2019) 42(2):281–94. doi: 10.1007/s40264-018-0774-8
10. Jin HT, Ahmed R, Okazaki T. Role of PD-1 in Regulating T-Cell Immunity. Curr Top Microbiol Immunol (2011) 350:17–37. doi: 10.1007/82_2010_116
11. Agata Y, Kawasaki A, Nishimura H, Ishida Y, Tsubata T, Yagita H, et al. Expression of the PD-1 Antigen on the Surface of Stimulated Mouse T and B Lymphocytes. Int Immunol (1996) 8(5):765–72. doi: 10.1093/intimm/8.5.765
12. Ralser DJ, Klümper N, Gevensleben H, Zarbl R, Kaiser C, Landsberg J, et al. Molecular and Immune Correlates of PDCD1 (PD-1), PD-L1 (CD274), and PD-L2 (PDCD1LG2) DNA Methylation in Triple Negative Breast Cancer. J Immunother (2021) 44(8):319–24. doi: 10.1097/CJI.0000000000000384
13. Cha JH, Chan LC, Li CW, Hsu JL, Hung MC. Mechanisms Controlling PD-L1 Expression in Cancer. Mol Cell (2019) 76(3):359–70. doi: 10.1016/j.molcel.2019.09.030
14. Poggio M, Hu T, Pai CC, Chu B, Belair CD, Chang A, et al. Suppression of Exosomal PD-L1 Induces Systemic Anti-Tumor Immunity and Memory. Cell (2019) 177(2):414–427.e13. doi: 10.1016/j.cell.2019.02.016
15. Zhou T, Xu D, Tang B, Ren Y, Han Y, Liang G, et al. Expression of Programmed Death Ligand-1 and Programmed Death-1 in Samples of Invasive Ductal Carcinoma of the Breast and Its Correlation With Prognosis. Anticancer Drugs (2018) 29(9):904–10. doi: 10.1097/CAD.0000000000000683
16. Mocan T, Sparchez Z, Craciun R, Bora CN, Leucuta DC. Programmed Cell Death Protein-1 (PD-1)/Programmed Death-Ligand-1 (PD-L1) Axis in Hepatocellular Carcinoma: Prognostic and Therapeutic Perspectives. Clin Transl Oncol (2019) 21(6):702–12. doi: 10.1007/s12094-018-1975-4
17. Nomi T, Sho M, Akahori T, Hamada K, Kubo A, Kanehiro H, et al. Clinical Significance and Therapeutic Potential of the Programmed Death-1 Ligand/Programmed Death-1 Pathway in Human Pancreatic Cancer. Clin Cancer Res (2007) 13(7):2151–7. doi: 10.1158/1078-0432.CCR-06-2746
18. Yang MW, Fu XL, Jiang YS, Chen XJ, Tao LY, Yang JY, et al. Clinical Significance of Programmed Death 1/Programmed Death Ligand 1 Pathway in Gastric Neuroendocrine Carcinomas. World J Gastroenterol (2019) 25(14):1684–96. doi: 10.3748/wjg.v25.i14.1684
19. Yokoyama S, Miyoshi H, Nakashima K, Shimono J, Hashiguchi T, Mitsuoka M, et al. Prognostic Value of Programmed Death Ligand 1 and Programmed Death 1 Expression in Thymic Carcinoma. Clin Cancer Res (2016) 22(18):4727–34. doi: 10.1158/1078-0432.CCR-16-0434
20. Li X, Lian Z, Wang S, Xing L, Yu J. Interactions Between EGFR and PD-1/PD-L1 Pathway: Implications for Treatment of NSCLC. Cancer Lett (2018) 418:1–9. doi: 10.1016/j.canlet.2018.01.005
21. Noske A, Möbus V, Weber K, Schmatloch S, Weichert W, Köhne CH, et al. Relevance of Tumour-Infiltrating Lymphocytes, PD-1 and PD-L1 in Patients With High-Risk, Nodal-Metastasised Breast Cancer of the German Adjuvant Intergroup Node-Positive Study. Eur J Cancer (2019) 114:76–88. doi: 10.1016/j.ejca.2019.04.010
22. Ali HR, Glont SE, Blows FM, Provenzano E, Dawson SJ, Liu B, et al. PD-L1 Protein Expression in Breast Cancer is Rare, Enriched in Basal-Like Tumours and Associated With Infiltrating Lymphocytes. Ann Oncol (2015) 26(7):1488–93. doi: 10.1093/annonc/mdv192
23. Hou Y, Nitta H, Wei L, Banks PM, Lustberg M, Wesolowski R, et al. PD-L1 Expression and CD8-Positive T Cells are Associated With Favorable Survival in HER2-Positive Invasive Breast Cancer. Breast J (2018) 24(6):911–9. doi: 10.1111/tbj.13112
24. Chen L, Zhou X, Kong X, Su Z, Wang X, Li S, et al. The Prognostic Significance of Anisomycin-Activated Phospho-C-Jun NH2-Terminal Kinase (P-JNK) in Predicting Breast Cancer Patients' Survival Time. Front Cell Dev Biol (2021) 9:656693. doi: 10.3389/fcell.2021.656693
25. Eisenhauer EA, Therasse P, Bogaerts J, Schwartz LH, Sargent D, Ford R, et al. New Response Evaluation Criteria in Solid Tumours: Revised RECIST Guideline (Version 1.1). Eur J Cancer (2009) 45(2):228–47. doi: 10.1016/j.ejca.2008.10.026
26. Ogston KN, Miller ID, Payne S, Hutcheon AW, Sarkar TK, Smith I, et al. A New Histological Grading System to Assess Response of Breast Cancers to Primary Chemotherapy: Prognostic Significance and Survival. Breast (2003) 12(5):320–7. doi: 10.1016/s0960-9776(03)00106-1
27. Park SY. Nomogram: An Analogue Tool to Deliver Digital Knowledge. J Thorac Cardiovasc Surg (2018) 155(4):1793. doi: 10.1016/j.jtcvs.2017.12.107
28. Basu A, Ramamoorthi G, Jia Y, Faughn J, Wiener D, Awshah S, et al. Immunotherapy in Breast Cancer: Current Status and Future Directions. Adv Cancer Res (2019) 143:295–349. doi: 10.1016/bs.acr.2019.03.006
29. Domschke C, Schneeweiss A, Stefanovic S, Wallwiener M, Heil J, Rom J, et al. Cellular Immune Responses and Immune Escape Mechanisms in Breast Cancer: Determinants of Immunotherapy. Breast Care (Basel) (2016) 11(2):102–7. doi: 10.1159/000446061
30. Hinshaw DC, Shevde LA. The Tumor Microenvironment Innately Modulates Cancer Progression. Cancer Res (2019) 79(18):4557–66. doi: 10.1158/0008-5472.CAN-18-3962
31. Sugie T. Immunotherapy for Metastatic Breast Cancer. Chin Clin Oncol (2018) 7(3):28. doi: 10.21037/cco.2018.05.05
32. Constantinidou A, Alifieris C, Trafalis DT. Targeting Programmed Cell Death -1 (PD-1) and Ligand (PD-L1): A New Era in Cancer Active Immunotherapy. Pharmacol Ther (2019) 194:84–106. doi: 10.1016/j.pharmthera.2018.09.008
33. Shen X, Zhao B. Efficacy of PD-1 or PD-L1 Inhibitors and PD-L1 Expression Status in Cancer: Meta-Analysis. BMJ (2018) 362:k3529. doi: 10.1136/bmj.k3529
34. Brody R, Zhang Y, Ballas M, Siddiqui MK, Gupta P, Barker C, et al. PD-L1 Expression in Advanced NSCLC: Insights Into Risk Stratification and Treatment Selection From a Systematic Literature Review. Lung Cancer (2017) 112:200–15. doi: 10.1016/j.lungcan.2017.08.005
35. Paver EC, Cooper WA, Colebatch AJ, Ferguson PM, Hill SK, Lum T, et al. Programmed Death Ligand-1 (PD-L1) as a Predictive Marker for Immunotherapy in Solid Tumours: A Guide to Immunohistochemistry Implementation and Interpretation. Pathology (2021) 53(2):141–56. doi: 10.1016/j.pathol.2020.10.007
36. Ahmed FS, Gaule P, McGuire J, Patel K, Blenman K, Pusztai L, et al. PD-L1 Protein Expression on Both Tumor Cells and Macrophages are Associated With Response to Neoadjuvant Durvalumab With Chemotherapy in Triple-Negative Breast Cancer. Clin Cancer Res (2020) 26(20):5456–61. doi: 10.1158/1078-0432.CCR-20-1303
37. Wesolowski R, Stiff A, Quiroga D, McQuinn C, Li Z, Nitta H, et al. Exploratory Analysis of Immune Checkpoint Receptor Expression by Circulating T Cells and Tumor Specimens in Patients Receiving Neo-Adjuvant Chemotherapy for Operable Breast Cancer. BMC Cancer (2020) 20(1):445. doi: 10.1186/s12885-020-06949-4
38. Loibl S, Untch M, Burchardi N, Huober J, Sinn BV, Blohmer JU, et al. A Randomised Phase II Study Investigating Durvalumab in Addition to an Anthracycline Taxane-Based Neoadjuvant Therapy in Early Triple-Negative Breast Cancer: Clinical Results and Biomarker Analysis of GeparNuevo Study. Ann Oncol (2019) 30(8):1279–88. doi: 10.1093/annonc/mdz158
39. Matikas A, Zerdes I, Lövrot J, Sifakis E, Richard F, Sotiriou C, et al. PD-1 Protein and Gene Expression as Prognostic Factors in Early Breast Cancer. ESMO Open (2020) 5(6):e001032. doi: 10.1136/esmoopen-2020-001032
40. Matikas A, Zerdes I, Lövrot J, Richard F, Sotiriou C, Bergh J, et al. Prognostic Implications of PD-L1 Expression in Breast Cancer: Systematic Review and Meta-Analysis of Immunohistochemistry and Pooled Analysis of Transcriptomic Data. Clin Cancer Res (2019) 25(18):5717–26. doi: 10.1158/1078-0432.CCR-19-1131
41. Botti G, Collina F, Scognamiglio G, Rao F, Peluso V, De Cecio R, et al. Programmed Death Ligand 1 (PD-L1) Tumor Expression Is Associated With a Better Prognosis and Diabetic Disease in Triple Negative Breast Cancer Patients. Int J Mol Sci (2017) 18(2):459. doi: 10.3390/ijms18020459
42. Parvathareddy SK, Siraj AK, Ahmed SO, Ghazwani LO, Aldughaither SM, Al-Dayel F, et al. PD-L1 Protein Expression in Middle Eastern Breast Cancer Predicts Favorable Outcome in Triple-Negative Breast Cancer. Cells (2021) 10(2):229. doi: 10.3390/cells10020229
43. Jiang C, Cao S, Li N, Jiang L, Sun T. PD-1 and PD-L1 Correlated Gene Expression Profiles and Their Association With Clinical Outcomes of Breast Cancer. Cancer Cell Int (2019) 19:233. doi: 10.1186/s12935-019-0955-2
44. Asano Y, Kashiwagi S, Goto W, Takada K, Takahashi K, Morisaki T, et al. Prediction of Treatment Responses to Neoadjuvant Chemotherapy in Triple-Negative Breast Cancer by Analysis of Immune Checkpoint Protein Expression. J Transl Med (2018) 16(1):87. doi: 10.1186/s12967-018-1458-y
45. Chen S, Wang RX, Liu Y, Yang WT, Shao ZM. PD-L1 Expression of the Residual Tumor Serves as a Prognostic Marker in Local Advanced Breast Cancer After Neoadjuvant Chemotherapy. Int J Cancer (2017) 140(6):1384–95. doi: 10.1002/ijc.30552
Keywords: breast cancer, immune checkpoint inhibitors, programmed cell death 1 (PD-1), programmed cell death ligand-1 (PD-L1), neoadjuvant chemotherapy
Citation: Chen L, Huang S, Liu Q, Kong X, Su Z, Zhu M, Fang Y, Zhang L, Li X and Wang J (2022) PD-L1 Protein Expression Is Associated With Good Clinical Outcomes and Nomogram for Prediction of Disease Free Survival and Overall Survival in Breast Cancer Patients Received Neoadjuvant Chemotherapy. Front. Immunol. 13:849468. doi: 10.3389/fimmu.2022.849468
Received: 06 January 2022; Accepted: 26 April 2022;
Published: 20 May 2022.
Edited by:
Jian Zhang, Southern Medical University, ChinaReviewed by:
Rui Sha, Shanghai Jiao Tong University, ChinaCopyright © 2022 Chen, Huang, Liu, Kong, Su, Zhu, Fang, Zhang, Li and Wang. This is an open-access article distributed under the terms of the Creative Commons Attribution License (CC BY). The use, distribution or reproduction in other forums is permitted, provided the original author(s) and the copyright owner(s) are credited and that the original publication in this journal is cited, in accordance with accepted academic practice. No use, distribution or reproduction is permitted which does not comply with these terms.
*Correspondence: Lin Zhang, dG9ueTE5ODIxMTBAZ21haWwuY29t; Xingrui Li, bGl4aW5ncnVpQHRqaC50am11LmVkdS5jbg==; Jing Wang, d2FuZ2ppbmdAY2ljYW1zLmFjLmNu
†ORCID: Li Chen, orcid.org/0000-0002-6989-1177
‡These authors have contributed equally to this work
Disclaimer: All claims expressed in this article are solely those of the authors and do not necessarily represent those of their affiliated organizations, or those of the publisher, the editors and the reviewers. Any product that may be evaluated in this article or claim that may be made by its manufacturer is not guaranteed or endorsed by the publisher.
Research integrity at Frontiers
Learn more about the work of our research integrity team to safeguard the quality of each article we publish.