- 1Hangzhou Key Laboratory of Animal Adaptation and Evolution, College of Life and Environmental Sciences, Hangzhou Normal University, Hangzhou, China
- 2Department of Entomology, China Agricultural University, Beijing, China
Locusta migratoria manilensis is one of the most important agricultural pests in China. The locust has high fecundity and consumes large quantities of food, causing severe damage to diverse crops such as corn, sorghum, and rice. Immunity against pathogens and reproductive success are two important components of individual fitness, and many insects have a trade-off between reproduction and immunity when resources are limited, which may be an important target for pest control. In this study, adult females L. migratoria manilensis were treated with different concentrations (5 × 106 spores/mL or 2 × 107 spores/mL) of the entomopathogenic fungus Paranosema locustae. Effects of input to immunity on reproduction were studied by measuring feeding amount, enzyme activity, vitellogenin (Vg) and vitellogenin receptor (VgR) production, ovary development, and oviposition amount. When infected by P. locustae, feeding rate and phenol oxidase and lysozyme activities increased, mRNA expression of Vg and VgR genes decreased, and yolk deposition was blocked. Weight of ovaries decreased, with significant decreases in egg, length and weight.Thus, locusts used nutritive input required for reproduction to resist invasion by microsporidia. This leads to a decrease in expression of Vg and VgR genes inhibited ovarian development, and greatly decreased total fecundity. P. locustae at 2 × 107 spores/mL had a more obvious inhibitory effect on the ovarian development in migratory locusts. This study provides a detailed trade-off between reproduction and immune input of the female, which provides a reliable basis to find pest targets for biological control from those trade-off processes.
Introduction
Paranosema (Nosema) locustae is an entomopathogenic fungus that only infects locusts. Since the 1980s, the fungus has shown great potential as a biological control in locust control (1, 2). Locusts infected by P. locustae can spread the pathogen to other nearby locusts through horizontal transmission. The fungus can also be transmited vertically, with microsporidia infecting offspring through locust eggs (3, 4). Recently, a study has found that microsporidia can also be transmitted through the feces of predators (5). After consumption by locusts, microsporidian spores germinate in the midgut lumen by everting the polar filament into cells, and the infection occurs through the sporoplasm to primarily infect the fat body (6). Microsporidia proliferate in the fat body and destroy normal host cell metabolism by capturing ATP and secreting hexokinase and hydrolase to deplete host glycogen (7–9). To resist the processes associated with infection, locusts must invest large amounts of energy.
Vitellogenesis, an imperative event of insect reproduction, is essential for insect fecundity (10, 11).In insects, vitellogenesis is a complex process involving two parts. First, vitellogenins (Vg) are biosynthesized in the fat body and released into the haemolymph. Maturing oocytes then absorb the vitellogenins from the haemolymph through endocytosis mediated by vitellogenin receptors (VgR) and accumulate yolk to form a mature egg, which increases in size as it matures (11, 12). In insects, juvenile hormone, insulin (or insulin-like peptide), and amino acid/target of rapamycin signaling pathways control vitellogenesis (13–16). Insulin and target of rapamycin signalling pathways regulate the terminal stages of oocyte development by regulating expression of vitellogenin (14). Total number of eggs produced and number of eggs in a single egg pod are affected by body size and life history of each individual (17). In L. migratoria manilensis, egg yolk biosynthesis is controlled by juvenile hormone (18) and is also dependent on feeding temperature. Vitellogenin first appears in the serum of adult females 5–9 days after emergence, with vitellogenin titer reaching 25 to 30 mg/mL in the second cycle of vitellogenin production (19).
In the face of predation, disease, and other hazards, the energy intake of an organism is generally insufficient for growth, reproduction, survival, and other important activities, which leads to trade-offs in allocation of resources (20, 21). Immunity and reproduction, are two critical processes that require a substantial investment of resources in female insects. Given that life-history evolution tends to optimize rather than maximize energy resources, reproduction and immunity can be mutually constraining (22). In Alloallomobilus socius, the mating process leads to a decrease in hemocyte load, lytic activity, and encapsulation and thus an increase in mortality (23). P. locustae infection in L. migratoria manilensis results in increased expression of defense genes, such as those for antimicrobial peptides, peroxiredoxin, and amine oxidase, increased investment in immunity, and consumption of energy materials for reproduction (2). In addition, microsporidia can inhibit phenol oxidase (PO) activity in locusts, which makes host melanization of pathogens difficult (2). In Orthoptera, crickets are frequently used as models to study the trade-off between immunity and reproduction.
The orthopteran L. migratoria manilensis, can be divided into aggregation and dispersion types, depending on their population density (24, 25). Locusts are a model orthopteran (26). However, they are also notorious because of the threat of mass migrations and are one of the most destructive agricultural pests worldwide (27). High locust fecundity is the basis of outbreaks. Plagues of locusts have been a historical problem in China and elsewhere. After nearly half a century of overuse of chemical pesticides, detrimental effects on environment have led to the recognition that more environmentally sound methods of pest control are needed (28). The use of P. locustae is one potentially sustainable approach to control locusts. The first report of microsporidia as a biological control tool was from the United States Department of Agriculture, which used Nosema acidophagus (29). P. locustae was isolated from locusts by Canning (30), and Henry (31) used Melanoplus bivittatus as a new host to increase spore numbers. Since then, P. locustae has since been used in locust control with remarkable success as a commercial, environmentally safe pest management tool. However, the continuous use of P. locustae has led to locusts developing a certain level of immunity. With the establishment of transgenic crops, the search for new gene targets is also an important research direction. In this study, the potential trade-off in investment between reproduction and immunity in locusts was explored. This study provides an important foundation to increase understanding of mechanisms of the trade-offs between insect immunity and other physiological functions and also to identify new potential targets for biological control.
Materials and methods
L. migratoria manilensis and P. locustae
Colonies of L. migratoria manilensis were maintained in the Key Laboratory of Animal Adaptation and Evolution at the Life and Environmental Science School of Hangzhou Normal University (Hangzhou, China). The feeding temperature was set at 30 ± 2°C with a photoperiod L16: D8 and 50% relative humidity. Locusts were hatched in small, clean boxes (10 cm × 15 cm × 20 cm) and then released into large, dry, well-ventilated cages of 50 cm × 50 cm × 50 cm at a density of 200 to 300 insects per cage. Locusts were provided fresh wheat seedlings and wheat bran for at least two generations before experimentation. A suspension of P. locustae provided by the China Agricultural University (Beijing, China) was diluted to either 5 × 106 spores/mL or 2 × 107 spores/mL and maintained at 4°C.
Treatment design and infection of L. migratoria manilensis
Fresh wheat seedlings were divided into three equal portions of 100g each. One portion was soaked with 5 × 106 spores/mL and another with 2 × 107 spores/mL of P. locustae, and both were dried at 37°C for 10 min. The third portion was used as a control treatment. Food was provided from emergence, and on days 1–4 post emergence, the average daily weight of wheat seedlings consumed was recorded. The three groups of locusts were reared in the same conditions, and males and females were paired. Each treatment included three biological replications of five locust individuals each.
PO and lysozyme activity in L. migratoria manilensis
There were three biological replicates of P. locustae-infection and control groups, with five individuals per replicate. In the three groups of locusts, fat bodies and ovaries were dissected at 2, 4, 6, 8, 10, 12, 14, 16, 18, and 20 d. Fat bodies and ovaries were frozen with liquid nitrogen and stored at −80°C.
To detect PO activity, 2 µL of serum was diluted to 20 µL using 0.9% normal saline, followed by addition of 40 μL of CAC buffer and 60 μL of 3 g/L L-dopa. Solutions were mixed and placed in a 25°C water bath for 30 min, and then, the OD595 nm value was determined. In a blank control, 60 μL of CAC buffer and 60 μL of 3 g/L L-dopa were used. Detection time of PO activity was 30 min. Total protein content was determined using a Coomassie bright blue protein kit following the manufacturer’s instructions (Nanjing Jiancheng Biological Company, Nanjing, China). The OD495 value was calculated as sample OD495 value − control OD495 value. One unit of PO activity (U) was defined as U = OD495 value/min/mg protein.
The detection method for lysozyme activity was adjusted according to Hultmark (32). To prepare of the bacterial suspension, micrococcus dry powder (Nanjing Jiancheng Biological Company, Nanjing, China) was used as the substrate, 0.1 mol/L phosphoric acid buffer (pH 6.4) was used as the substrate solvent and activity was confirmed when the 570 nm light absorption value was greater than 0.35. To detect lysozyme activity, 200 μL of bacterial suspension was removed and placed in an ice bath for 10 min. Then, 4 μL of locust serum added and mixed, and a UV2000 was used to determine the absorbency (A) at 570 nm. A sample was placed in a 37°C water bath for 30 min, moved to ice to stop the reaction for 10 min, and the absorption value at 570 nm was determined (A0). Lysozyme activity (U) was calculated as U = (A0 − A)/A.
RNA extraction, cDNA synthesis and quantification of mRNA expression
From fat bodies and ovaries dissected imultaneously with collection of serum, total RNA was extracted using the TRIzol reagent (Invitrogen, Carlsbad, CA, USA) following the manufacturer’s instructions. Each treatment included three biological replicates of five locust individuals each. Dissecting time was 2, 4, 6, 8, 10, 12, 14, 16, 18, and 20 d after emergence. The RNA concentration was determined by measuring the absorbance at 260 nm with a spectrophotometer. First-strand cDNA synthesis was performed using a PrimeScript® RT Reagent Kit with gDNA Eraser (Takara, Dalian, China) following the manufacturer’s protocol. All primers were designed to determine expression of the genes VgA, VgB, VgR1, and VgR2 (Table 1). Expression levels of genes were normalized using the expression of actin. Reverse-transcription quantitative real-time PCR (RT-qPCR) was performed using a Bio-Rad CFX96 Real-Time PCR Detection System (Bio-Rad, Hercules, CA, USA) and Premix Ex Taq (SYBR Green) reagents (Takara). The 20 μL RT-qPCR reactions were subject to a thermal profile of 95°C for 3 min and then 40 cycles of 95°C for 10 s and 60°C for 30 s. The thermal melting profile was assessed using a final PCR cycle of 95°C for 30 s, with temperature increasing continuously from 60°C to 95°C. Relative gene expression levels were calculated using the 2–ΔΔCT method, with three replicates per sample.
Paraffin sectioning of ovaries
In controls and groups infected with P. locustae, ovarian tissue sections were prepared by a paraffin method. Female locusts 8 days after eclosion were immersed in 10% formaldehyde solution for 12 h. Fixed samples were then dehydrated in an ethanol series of 50%, 75%, 90%, and 100%, with at least 30 min in each step. Following dehydration, samples were cleared with xylene. Cleared samples were embedded in paraffin and sectioned. Sections were dewaxed with xylene and ethanol, hydrated, and stained with hematoxylin and eosin. After staining, samples were dehydrated with anhydrous alcohol for 30 s and 1 to 2 drops of neutral glue were added to seal the samples. Samples were observed and photographed under a microscope. Each treatment included three biological replicates of five locust individuals each.
L. migratoria manilensis reproductive and developmental indices
In controls and groups infected with P. locustae, the length and weight of egg pods and the length and width of each egg were measured. Each treatment included three biological replicates of eight individuals each. In an additional set of experiments, amount of oviposition was measured in the two treatments and control. Each treatment included three biological replicates of eight individuals each. After hatching, offspring were fed individually in transparent plastic feeding tubes and maintained in an artificial climate chamber. Each treatment included more than 30 individuals. Locust nymph development was recorded twice a day at 8:00 AM and 8:00 PM.
Statistical analyses
Statistical analyses were performed with the GraphPad Prism 8.3.0 software. Except for the data from sections of ovarian tissue, statistical significance analysis of the remaining data presented in this study were analyzed using one-way or two-way ANOVA followed by Tukey’s multiple comparisons test. The confidence interval was set to 95%, and P < 0.05 was considered statistically significant.
Results
Changes in food Intake of locusts infected with P. locustae
To understand changes in L. migratoria manilensis metabolism after microbial infection, effects of infection with different concentrations of P. locustae on locust feeding behavior were determined. Compared with uninfected control females, infected locusts consumed more food (F2,6 = 9.53; P = 0.0137). When infected with a concentration of 2 × 107 spores/mL, food intake increased significantly and was more than 1.48-fold higher than that in the control (Figure 1).
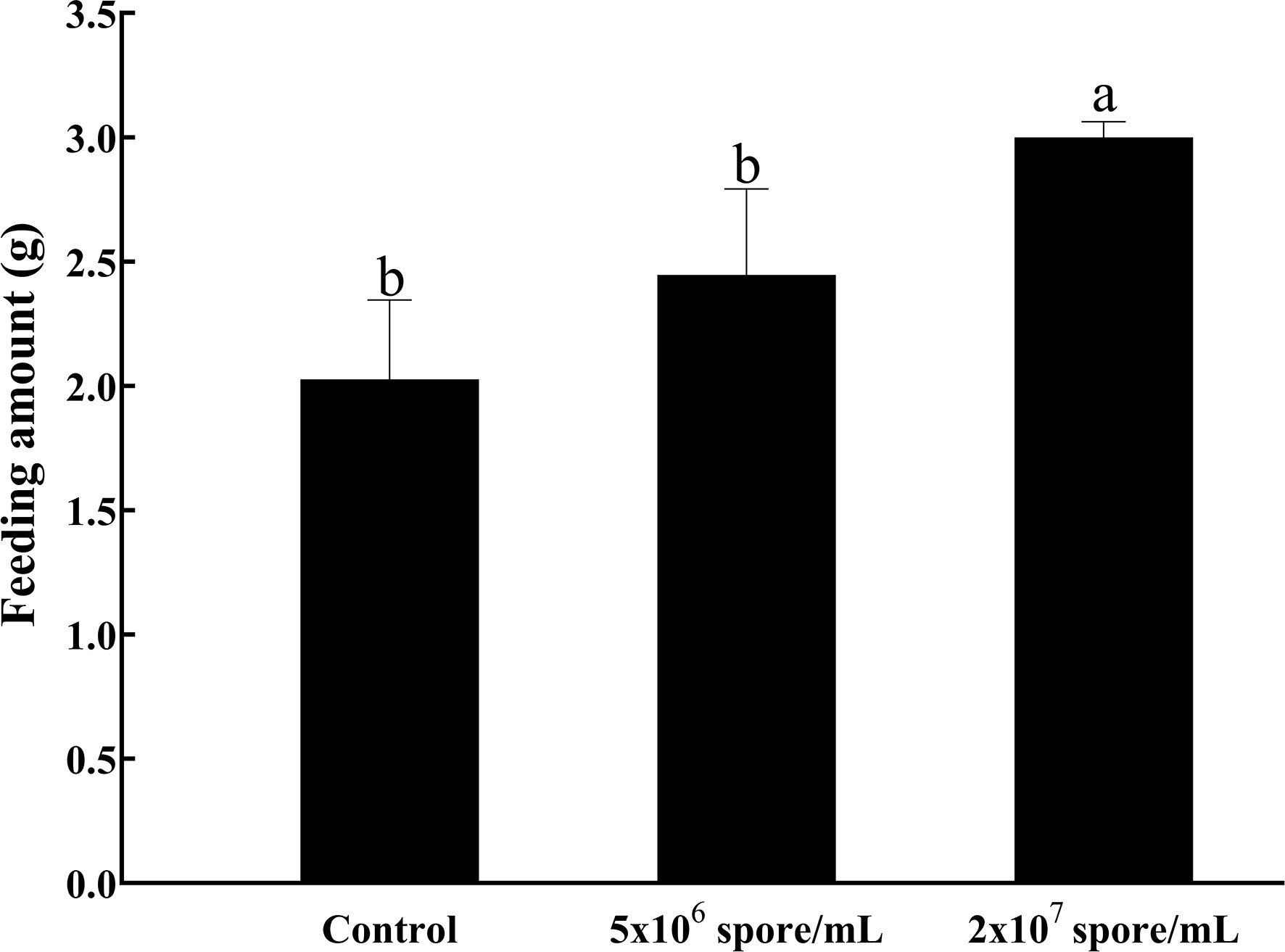
Figure 1 Weight (g) of food consumed by L. manilensis after infection with P. locustae. Average daily food intake of adult females on days 1–4 after eclosion. Each value is presented as mean ± SD, and different letters indicate significant differences among different treatments (one-way ANOVA followed by Tukey’s multiple comparisons test, P < 0.05).
Changes in enzyme activity in locusts Infected with P. locustae
To understand changes in immune function in L. migratoria manilensis infected by the fungus, PO and lysozyme activities were examined. According to two-way ANOVA, PO activity was significantly affected by P. locustae concentration (F9,150 = 425.8; P < 0.001), duration of infection (F2,150 = 67.77; P < 0.001), and their interaction (F18,150 = 89.78; P < 0.001). PO activity in 5 × 106 and 2 × 107 spores/mL treatments was higher than that in the control from day 2 to 12 but was significantly lower from day 16 to 20. PO activity was 1.33-fold and 1.38-fold higher in 5 × 106 and 2 × 107 spores/mL treatments, respectively,than that in the control at 6 days (Figure 2A). According to two-way ANOVA, lysozyme activity was significantly affected by P. locustae concentration(F9,150 = 4,294; P < 0.001), duration of infection (F2,150 = 323.3; P < 0.001), and their interaction (F18,150 = 272; P < 0.001). Compared with the control, microsporidian infection increased lysozyme activity from day 2 to 8 but decreased it from day 10 to 20. Lysozyme activity was strongly induced at 4 days and was 1.28-fold (5 × 106 spores/mL) and 1.52-fold (2 × 107 spores/mL) higher than that in the control. Lysozyme activity at the high concentration (2 × 107 spores/mL) began to decrease significantly by day 10. However, enzyme activity at the low concentration (5 × 106 spores/mL) was not significantly different from that in the control from day 10 to 18 (Figure 2B). Therefore, a microsporidian solution with a concentration of 2 × 107 spores/mL led to the greatest stimulation in enzyme activity (Figure 2).
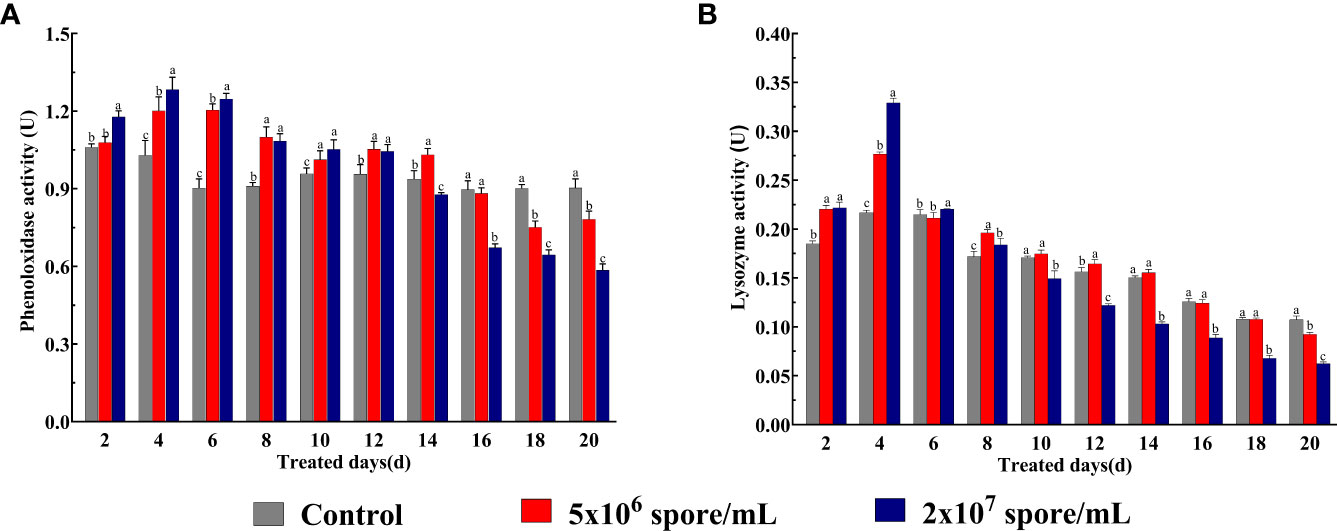
Figure 2 Phenol oxidase (PO) and lysozyme activities in L. migratoria manilensis infected with P. locustae. (A) PO activity of adult females were infected by P. locustae on days 2–20. (B) Lysozyme activity of adult females infected by P. locustae on days 2–20. Each value is presented as mean ± SD, and different letters indicate significant differences among different treatments at the same time point (two-way ANOVA followed by Tukey’s multiple comparisons test, P < 0.05).
Changes in expression of Vg and VgR genes in locusts Infected with P. locustae
To examine effects of P. locustae infection on locust reproductive capacity, mRNA expression of VgA and VgB and their receptors VgR1 and VgR2 was examined. Two-way ANOVA showed that expression of VgA, VgB, VgR1, and VgR2 was significantly affected by P. locustae concentration (VgA: F2,90 = 1871, P < 0.001; VgB: F2, 90 = 480.9, P < 0.001; VgR1: F2, 90 = 1987, P < 0.001; VgR2: F2, 90 = 531.8, P < 0.001), duration of infection(VgA: F9,90 = 310.3, P < 0.001; VgB: F9, 90 = 398.6, P < 0.001; VgR1: F9, 90 = 210.3, P < 0.001; VgR2: F9, 90 = 98.32, P < 0.001), and their interaction (VgA: F18,150 = 83.71, P < 0.001; VgB: F18, 90 = 51.18, P < 0.001; VgR1: F18, 90 = 51.91, P < 0.001; VgR2: F18, 90 = 67.63, P < 0.001) (Figure 3). Expression of VgA and VgR1 began to decrease significantly by day 4, and expression was significantly inhibited at 2 × 107 spores/mL at day 20 and was 20820.9-fold and 38111.9-fold lower, respectively, than that in the control (Figures 3A, C). Compared with control females, VgB expression was down-regulated on day 8 (Figure 3B) with P. locustae treatment. However, expression of VgR2 was significantly suppressed from day 2 (Figure 3D). The results indicated that P. locustae inhibited the transcription of Vg and VgR genes in locusts.
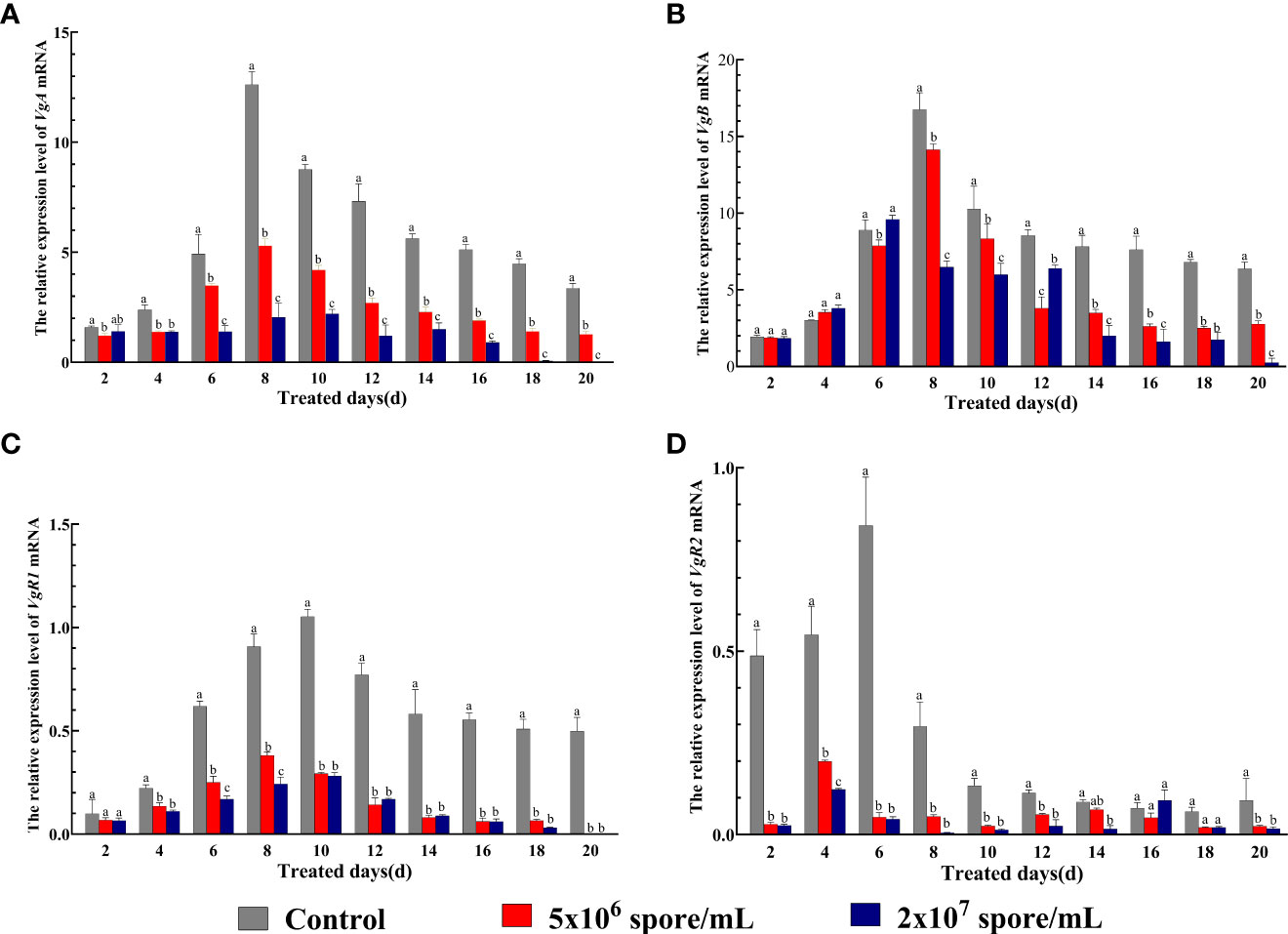
Figure 3 Expression of on Vg and VgR genes in expression after L. migratoria manilensis was infected by P. locustae. (A) Relative expression of VgA mRNA in adult females infected by P. locustae on days 2–20. (B) Relative expression of VgB mRNA in adult females infected by P. locustae on days 2–20. (C) Relative expression of VgR1 mRNA in adult females infected by P. locustae on days 2–20. (D) Relative expression of VgR2 mRNA in adult females infected by P. locustae on days 2–20. Each value is presented as mean ± SD, and different letters indicate significant differences among different treatments at the same time point (two-way ANOVA followed by Tukey’s multiple comparisons test, P < 0.05).
Changes in ovaries in locusts infected with P. locustae
Compared with control females, ovary size and number of well-developed eggs decreased slightly after day 8 at 5 × 106 spores/mL, but decreased significantly at 2 × 107 spores/mL (Figure 4). Therefore, ovarian development was abnormal with severe atrophy in locusts infected with P. locustaea.
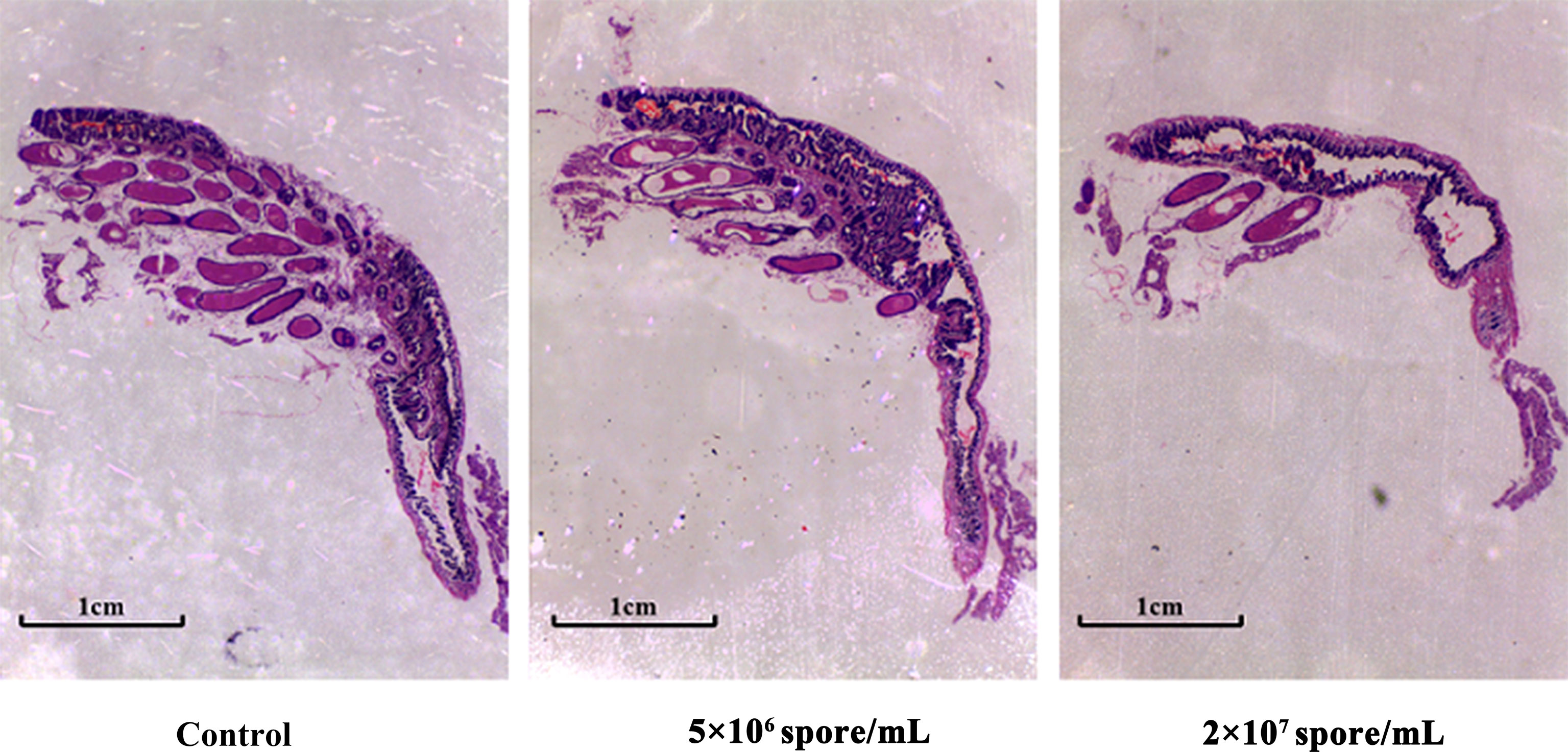
Figure 4 Effects of different spore concentrations of P. locustae on ovary development in L. migratoria manilensis. Longitudinal sections of locust ovarian tissue on day 8 after eclosion. Ovarian tissues were fixed with formaldehyde solution and then dehydrated in an ethanol series. After clearing with xylene, the samples were embedded in paraffin and stained with hematoxylin and eosin (H&E). Samples were observed and photographed under amicroscope. Scale bar = 50 μm.
Changes in number and quality of eggs offspring development time in locusts infected with P. locustae
Total number of eggs laid by a single adult female decreased significantly when infected with P. locustae at 2 × 107 spores/mL, compared with the control (F2,30 = 30.83; P < 0.001). The low concentration treatment had no effect on oviposition amount, whereas at the high concentration, total number of eggs laid decreased by 17% (Figure 5A). Compared with control females, weight of egg pods (F2,27 = 15.27, P < 0.001; Figure 5B) and length of egg pods (F2,27 = 4.01, P = 0.03; Figure 5C) decreased significantly in females infected with P. locustae. In addition, length (F2,87 = 10.48; P < 0.001) and width (F2,87 = 25.78; P < 0.001) of eggs decreased significantly after P. locustae infection (Figures 5D, E). Infection of females with P. locustae did not significantly affect offspring development time from egg to adult (F2, 44 = 1.09, P = 0.34; Figure 6B). Only in the high concentration treatment, the time required for the 5th instar larvae to develop to adults was significantly shorter than that in the control (F2,44 = 4.21, P = 0.021; Figure 6A).
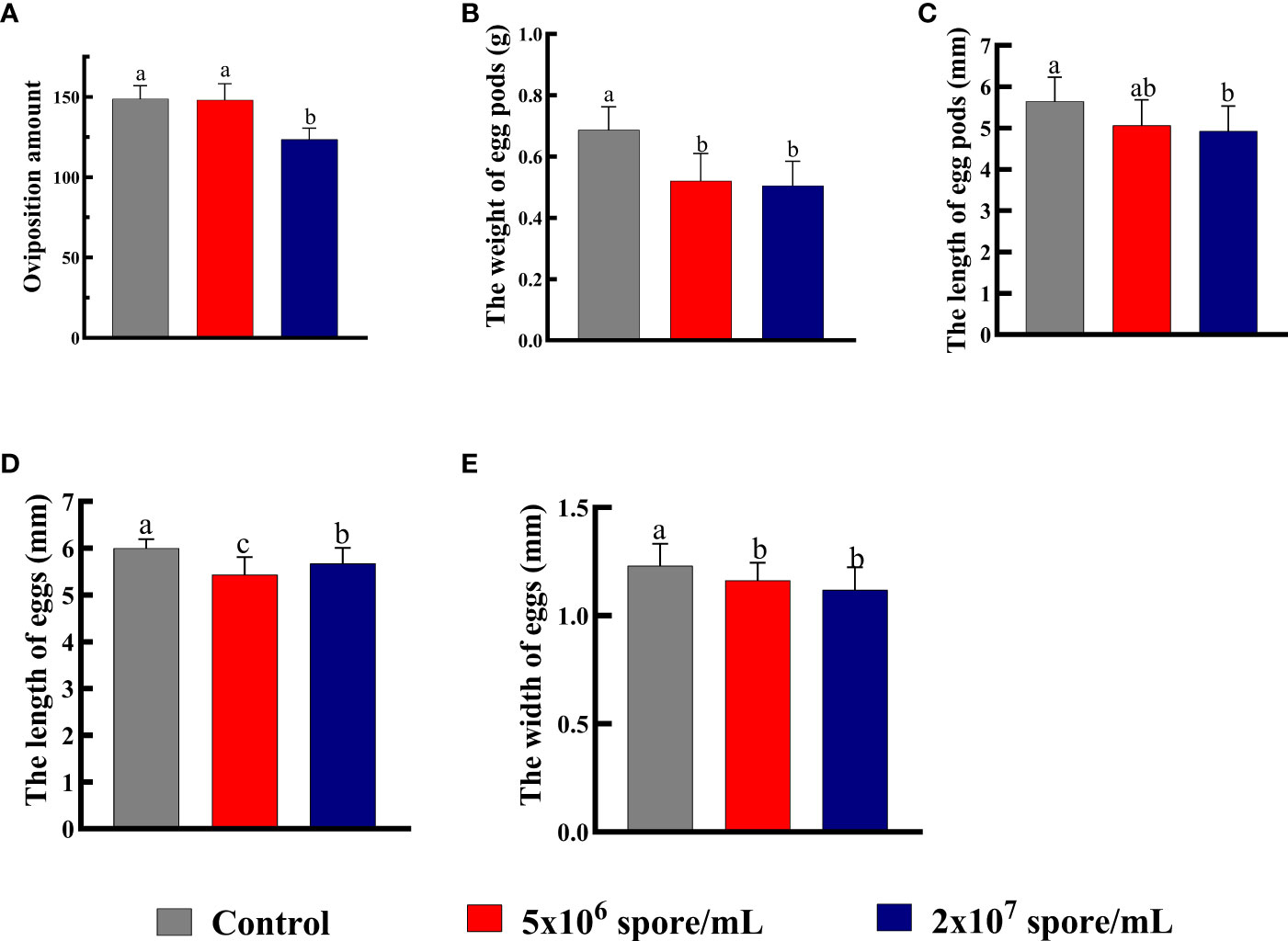
Figure 5 Number and quality of eggs laid by L. migratoria manilensis infected with P. locustae. (A) Oviposition amount or total number of eggs laid by a single adult female. (B) Average weight of egg pods laid by a single adult female. (C) Average length of egg pods laid by a single adult female. (D) Average length of eggs laid by a single adult female. (E) Average width of eggs laid by a single adult female. Each value is presented as mean ± SD, and the different letters indicate significant differences among different treatments (one-way ANOVA followed by Tukey’s multiple comparisons test, P < 0.05).
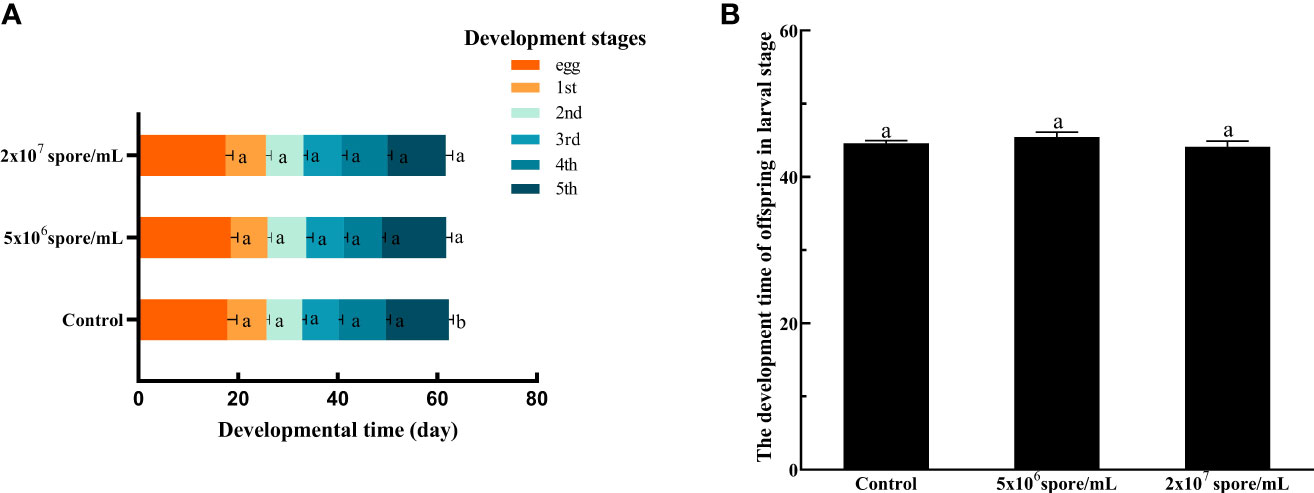
Figure 6 Development time of nymphs after L. migratoria manilensis infected with P. locustae. (A) Development time of each instar: egg, egg stage;1st, 1st instar nymph;2nd, 2nd instar nymph; 3rd, 3rd instar nymph; 4th, 4th instar nymph; 5th, 5th instar nymph. (B) Total time for offspring to develop from egg to adult. Each value is presented as mean ± SD, and different letters indicate significant differences among treatments (one-way ANOVA followed by Tukey’s multiple comparisons test, P < 0.05).
Discussion
The focus on trade-offs between reproduction and immunity in orthopteran has been on crickets. In dimorphic crickets, the immune response has a stronger effect on male sperm production than on female fertility (33–35), and there is an apparent lack of both physiological trade-offs and terminal reproductive investment in female crickets (36). However, similar studies on locusts have not been reported. In this study, lysozyme and PO activities in female L. migratoria manilensis infected with P. locustae were examined to assess the cost of immunity against pathogen invasion, and expression of vitellogenin, ovarian development, and development of offspring were examined to assess the reproductive changes in females when resources were invested in immunity.
P. locustae, first reported in desert locusts (37), has been used as a biological agent for pest control because it is harmless to humans and livestock (28). Infection with P. locustae activates host immunity, and then, insects must invest energy and resources to resist pathogen infection (38). In this study, after infection with P. locustae, feeding by locusts increased (Figure 1), indicating an increased need for energy to resist pathogens. Glycogen and fat are important energy reserves in insects and are primarily stored in the fat body. Microsporidia invade from the midgut and multiply in the fat body. Transcriptome profiles of P. locustae differ significantly in the fat body and midgut during middle and late stages of infection (2). In addition, late in the infection, the load of microsporidia in the fat body is much larger than that in the midgut, indicating the fat body is the eventual site of P. locustae eventually multiplication, and nodules form consisting of melanin deposits around heavily infected cells (2). Thus, microsporidia infection damages the fat body (39). After infection with microsporidia, locust glycogen and fat reserves are depleted, and there is rapid uptake of glucose by infected cells (7). However, because pathogens use energy from their hosts, an increase in food consumption may not necessarily increase host immunity (40). Insects lack the acquired immunity and therefore must rely on their innate immunity to resist the pathogens. In insects, the PO cascade, which induces expression of antimicrobial peptides, including lysozyme, is an important part of the humoral immunity (41). PO and prophenol oxidase are found in the sera of most arthropods, including crustaceans and insects, and are essential in defense responses. Insects can also resist bacterial infection through lysozyme shear activity (42).
In this study, PO activity increased significantly from day 2 to 12 (Figure 2A), whereas lysozyme activity increased from day 2 to 8 (Figure 2B). Those results demonstrated that infection with P. locustae activated expression of phenol oxidase and lysozyme, which could increase P. locustae mortality. However, PO activity decreased significantly from day 16 to 20, and lysozyme activity decreased significantly from day 10 to20, which indicated that expression of phenoloxidase and lysozyme was inhibited in the late stage of P. locustae infection. Reductions in melanization and lytic action against pathogens in locusts might be related to P. locustae ability to evade host immunity and contribute to rapid pathogen reproduction in the fat body. In many species, expression of host immunoreactive substances and level of immunity decrease in late phases of pathogen infection. For example, PO activity in honeybees infected with Spiroplasma melliferum increases initially and then decreases, and the decrease is associated with evasion of host immune defense by S. melliferum to improve its proliferation environment (43), and PO activity decreases sharply in the late stage of Charybdis japonica infection with Vibrio alginolyticus (44). In Spodoptera exigua, lysozyme and other antibacterial active substances are inhibited in the later stage of infection (45). Inhibitory effects of microsporidia on host antibacterial substances have also been observed in honeybees (46, 47). Therefore, microsporidia inhibition of PO the lysozyme activities in locusts could reduce melanization and enable parasites to escape immune responses and survive and proliferate.
Immunity and reproduction both consume large amounts of energy. In this study, female L. migratoria manilensis were infected with P. locustae early after eclosion, which might affect physiological indices related to reproduction. Activation of the immune system affects fecundity, which is a trade-off in resource allocation observed in many insects. For example, in fruit flies, activation of immunity reduces reproductive output (48). In Anopheles gambiae, immunization can induce melanization or humoral antimicrobial activity, and as a result, apoptosis begins in ovarian follicle cell epithelium, leading to a decrease in number of eggs produced (49). In crickets, immune responses can affect female survival rate and egg size (20). In this study, Vg expression levels in female L. migratoria manilensis decreased significantly with pathogen infection (Figures 3A, B), which would inhibit vitellin synthesis. In addition, expression of VgR genes decreased significantly (Figures 3C, D), which can result in abnormal deposition of vitellin and delayed ovarian development (50, 51). The results suggest that locusts increase energy investment in maintaining fluid circulation and survival after infection and therefore reduce investment in reproduction. In this study, ovaries of microsporidian-treated locusts showed significant atrophy (Figure 4), and as the concentration of P. locustae increased, the number of eggs laid decreased (Figure 5A).
Eggs size reflects reproductive input to offspring and is an important factor in matrilineal inheritance. In vitro-fertilized insects sperm combines with relatively large egg cells, and egg size determines an adaptability of the filial generation (52). Although the evolutionary importance of egg size has been debated, growth and survival rates and sometimes fecundity increase in large offspring (53). Resilience to environmental pressures, including larval competition (54), starvation (55, 56), drought (57) and nutritional stress (58, 59), also increases in large offspring. By contrasts, relatively small eggs tend to hatch faster but at a lower survival rate (54, 60). Egg production is also a measure of female fecundity because represents the number of offspring (61, 62). In this study, number of eggs laid (Figure 5A) and weight of egg pod (Figure 5B) decreased significantly after microsporidian infection. Egg length and width decreased significantly when the concentration reached 2 × 107 spores/mL (Figures 5D, E). Therefore, microsporidia infection decreased female fertility, and the decrease was related to microsporidia concentration. Development time of offspring in the 2 × 107 spores/mL treatment group was 1.02 days shorter than that of control offspring (Figure 6). This results suggested that high concentrations of P. locustae reduced time to hatch.
In this study, decreased expression of Vg and VgR in L. migratoria manilensis infected with P. locustae inhibited of vitelline deposition and ovarian development. Amount of oviposition (eggs per pod) decreased significantly, and nutritional status of eggs also decreased. Overall, the results of this study suggest the immune response to P. locustae reduced energy resources required for locust reproduction. In addition, new ideas were generated in this study for further studies on molecular mechanisms of the trade-offs between immunity and reproduction in locusts. Further studies are warranted on allocation of energy resources between immune responses and reproductive output and on identification of potential regulatory mechanisms, including energy-metabolizing and nutrient signaling, that could be targets for control of L. migratoria.
Data availability statement
The original contributions presented in the study are included in the article/supplementary material. Further inquiries can be directed to the corresponding authors.
Author contributions
S-GW and BT designed the work. Y-WH, G-QX, Y-JD and Q-YX carried out the experiments. Y-WH, S-HW, and YT performed the analysis. LZ provides Paranosema locustae for this research. Y-WH wrote the initial manuscript. S-HW, BT, LZ, S-GW and YT involved in interpreting data and revising manuscript. All authors read and approved the final manuscript.
Funding
This work was supported by National Key Research and Development Program of China (Grant No. 2017YFD0201000) and National Natural Science Foundation of China (Grant Nos. 31270459).
Acknowledgments
Thanks to China Agricultural University for providing Paranosema locustae for this research.
Conflict of interest
The authors declare that the research was conducted in the absence of any commercial or financial relationships that could be construed as a potential conflict of interest.
Publisher’s note
All claims expressed in this article are solely those of the authors and do not necessarily represent those of their affiliated organizations, or those of the publisher, the editors and the reviewers. Any product that may be evaluated in this article, or claim that may be made by its manufacturer, is not guaranteed or endorsed by the publisher.
Abbreviations
VgA, vitellogenin A; VgB, vitellogenin B; VgR, vitellogenin receptor; PO, phenol oxidase; RT-qPCR, reverse-transcription quantitative polymerase chain reaction.
References
1. Dakhel WH, Latchininsky AV, Jaronski ST. Efficacy of two entomopathogenic fungi, metarhizium brunneum, strain F52 alone and combined with paranosema locustae against the migratory grasshopper, melanoplus sanguinipes, under laboratory and greenhouse conditions. Insects (2019) 10:94. doi: 10.3390/insects10040094
2. Chen L, Gao X, Li R, Zhang L, Huang R, Wang L, et al. Complete genome of a unicellular parasite (Antonospora locustae) and transcriptional interactions with its host locust. Microb Genom (2020) 6:mgen000421. doi: 10.1099/mgen.0.000421
3. Tan S, Wang Y, Liu P, Ge Y, Li A, Xing, et al. Increase of albinistic hosts caused by gut parasites promotes self-transmission. Front Microbiol (2018) 9:1525. doi: 10.3389/fmicb.2018.01525
4. Gao X, Ban L. Genomics research progresses in microsporidia Antonospora locustae and its application status. J Plant Prot (2021) 48:60–4. doi: 10.13802/j.cnki.zwbhxb.2021.2021806
5. Wang-Peng S, Zheng X, Jia WT, Li AM, Camara I, Chen HX, et al. Horizontal transmission of paranosema locustae (Microsporidia) in grasshopper populations via predatory natural enemies. Pest Manag Sci (2018) 74:2589–93. doi: 10.1002/ps.5047
6. Lee SC, Corradi N, Doan S, Dietrich FS, Keeling PJ, Heitman J. Evolution of the sex-related locus and genomic features shared in microsporidia and fungi. PLoS One (2010) 5:e10539. doi: 10.1371/journal.pone.0010539
7. Méténier G, Vivarès CP. Molecular characteristics and physiology of microsporidia. Microbes Infect (2001) 3:407–15. doi: 10.1016/s1286-4579(01)01398-3
8. Tsaousis AD, Kunji ER, Goldberg AV, Lucocq JM, Hirt RP, Embley TM. A novel route for ATP acquisition by the remnant mitochondria of encephalitozoon cuniculi. Nature (2008) 453:553–6. doi: 10.1038/nature06903
9. Senderskiy IV, Timofeev SA, Seliverstova EV, Pavlova OA, Dolgikh VV. Secretion of antonospora (Paranosema) locustae proteins into infected cells suggests an active role of microsporidia in the control of host programs and metabolic processes. PLoS One (2014) 9:e93585. doi: 10.1371/journal.pone.0093585
10. Roy S, Saha TT, Zou Z, Raikhel AS. Regulatory pathways controlling female insect reproduction. Annu Rev Entomol (2018) 63:489–511. doi: 10.1146/annurev-ento-020117-043258
11. Zhu S, Liu F, Zeng H, Li N, Ren C, Su Y, et al. Insulin/IGF signaling and TORC1 promote vitellogenesis via inducing juvenile hormone biosynthesis in the American cockroach. Development (2020) 147:dev188805. doi: 10.1242/dev.188805
12. Seidelmann K, Helbing C, Göbeler N, Weinert H. Sequential oogenesis is controlled by an oviduct factor in the locusts locusta migratoria and schistocerca gregaria: Overcoming the doctrine that patency in follicle cells is induced by juvenile hormone. J Insect Physiol (2016) 90:1–7. doi: 10.1016/j.jinsphys.2016.03.008
13. Roy SG, Hansen IA, Raikhel AS. Effect of insulin and 20-hydroxyecdysone in the fat body of the yellow fever mosquito, aedes aegypti. Insect Biochem Mol Biol (2007) 37:1317–26. doi: 10.1016/j.ibmb.2007.08.004
14. Parthasarathy R, Palli SR. Molecular analysis of nutritional and hormonal regulation of female reproduction in the red flour beetle, tribolium castaneum. Insect Biochem Mol Biol (2011) 41:294–305. doi: 10.1016/j.ibmb.2011.01.006
15. Han B, Zhang T, Feng Y, Liu X, Zhang L, Chen H, et al. Two insulin receptors coordinate oogenesis and oviposition via two pathways in the green lacewing, chrysopa pallens. J Insect Physiol (2020) 123:104049. doi: 10.1016/j.jinsphys.2020.104049
16. Wu Z, Yang L, He Q, Zhou S. Regulatory mechanisms of vitellogenesis in insects. Front Cell Dev Biol (2021) 8:593613. doi: 10.3389/fcell.2020.593613
17. Chapman RF. The insects: Structure and function. Cambridge: Cambridge University Press (1998) p. 325–59.
18. Song J, Guo W, Jiang F, Kang L, Zhou S. Argonaute 1 is indispensable for juvenile hormone mediated oogenesis in the migratory locust, locusta migratoria. Insect Biochem Mol Biol (2013) 43:879–87. doi: 10.1016/j.ibmb.2013.06.004
19. Chinzei Y, Wyatt GR. Vitellogenin titre in haemolymph of Locusta migratoria in normal adults, after ovariectomy, and in response to methoprene. J Insect Physiol (1985) 31:0–445. doi: 10.1016/0022-1910(85)90090-3
20. Bascuñán-García AP, Lara C, Córdoba-Aguilar A. Immune investment impairs growth, female reproduction and survival in the house cricket, acheta domesticus. J Insect Physiol (2010) 56:204–11. doi: 10.1016/j.jinsphys.2009.10.005
21. Budischak SA, Hansen CB, Caudron Q, Garnier R, Kartzinel TR, Pelczer I, et al. Feeding immunity: Physiological and behavioral responses to infection and resource limitation. Front Immunol (2018) 8:1914. doi: 10.3389/fimmu.2017.01914
22. Schwenke RA, Lazzaro BP, Wolfner MF. Reproduction-immunity trade-offs in insects. Annu Rev Entomol (2016) 61:239–56. doi: 10.1146/annurev-ento-010715-023924
23. Fedorka KM, Zuk M, Mousseau TA. Immune suppression and the cost of reproduction in the ground cricket, allonemobius socius. Evolution (2004) 58:2478–85. doi: 10.1111/j.0014-3820.2004.tb00877.x
24. Wang X, Kang L. Molecular mechanisms of phase change in locusts. Annu Rev Entomol (2014) 59:225–44. doi: 10.1146/annurev-ento-011613-162019
25. Chen Q, He J, Ma C, Yu D, Kang L. Syntaxin 1A modulates the sexual maturity rate and progeny egg size related to phase changes in locusts. Insect Biochem Mol Biol (2015) 56:1–8. doi: 10.1016/j.ibmb.2014.11.001
26. Yu Y, Cao Y, Xia Y, Liu F. Wright-Giemsa staining to observe phagocytes in locusta migratoria infected with metarhizium acridum. J Invertebr Pathol (2016) 139:19–24. doi: 10.1016/j.jip.2016.06.009
27. Shi W, Guo Y, Xu C, Tan S, Miao J, Feng Y, et al. Unveiling the mechanism by which microsporidian parasites prevent locust swarm behavior. Proc Natl Acad Sci U.S.A. (2014) 111:1343–8. doi: 10.1073/pnas.1314009111
28. Lomer CJ, Bateman RP, Johnson DL, Langewald J, Thomas M. Biological control of locusts and grasshoppers. Annu Rev Entomol (2001) 46:667–702. doi: 10.1146/annurev.ento.46.1.667
29. Henry JE. Nosema acridophagus sp. n., a microsporidian isolated from grasshoppers. J Invertebr Pathol (1967) 9:331–41. doi: 10.1016/0022-2011(67)90067-5
30. Canning EU. The life cycle of Nosema locustae canning in Locusta migratoria migratorioides (Reiche and fairmaire), and its infectivity to other hosts. J Insect Pathol (1962) 4:237–47.
31. Henry JE. Experiment application of nosema locustae for control of grasshopper. J Invertebr Pathol (1971) 18:389–94. doi: 10.1016/0022-2011(71)90043-7
32. Hultmark D, Steiner H, Rasmuson T, Boman HG. Insect immunity. purification and properties of three inducible bactericidal proteins from hemolymph of immunized pupae of hyalophora cecropia. Eur J Biochem (1980) 106:7–16. doi: 10.1111/j.1432-1033.1980.tb05991.x
33. Tripet F. Sperm competition and its evolutionary consequences in the insects. Ann Entomological Soc America (2003) 96:170. doi: 10.1603/0013-8746(2003)096[0170:SCAIEC]2.0.CO;2
34. Meinhardt A, Hedger MP. Immunological, paracrine and endocrine aspects of testicular immune privilege. Mol Cell Endocrinol (2011) 335:60–8. doi: 10.1016/j.mce.2010.03.022
35. Simmons LW. Resource allocation trade-off between sperm quality and immunity in the field cricket, teleogryllus oceanicus. Behav Ecol (2011) 23:168–73. doi: 10.1093/beheco/arr170
36. Miyashita A, Lee TYM, McMillan LE, Easy R, Adamo SA. Immunity for nothing and the eggs for free: Apparent lack of both physiological trade-offs and terminal reproductive investment in female crickets (Gryllus texensis). PLoS One (2019) 14:e0209957. doi: 10.1371/journal.pone.0209957
37. Canning EU. A new microsporidian, nosema locustae N.Sp., from the fat body of the African migratory locust, locusta migratoria migratorioides r. & f. Parasitology (1953) 43:287–90. doi: 10.1017/s0031182000018655
38. Wang S, Liu X, Xia Z, Xie G, Tang B, Wang S. Transcriptome analysis of the molecular mechanism underlying immunity- and reproduction trade-off in locusta migratoria infected by micrococcus luteus. PLoS One (2019) 14(8):e0211605. doi: 10.1371/journal.pone.0211605
39. Tokarev YS, Sokolova YY, Entzeroth R. Microsporidia-insect host interactions: Teratoid sporogony at the sites of host tissue melanization. J Invertebr Pathol (2007) 94:70–3. doi: 10.1016/j.jip.2006.08.006
40. Cressler CE, Nelson WA, Day T, McCauley E. Disentangling the interaction among host resources, the immune system and pathogens. Ecol Lett (2014) 17:284–93. doi: 10.1111/ele.12229
41. Jiang H. The biochemical basis of antimicrobial responses in Manduca sexta. Insect Sci (2008) 15:53–66. doi: 10.1111/j.1744-7917.2008.00187.x
42. Elmogy M, Bassal TT, Yousef HA, Dorrah MA, Mohamed AA, Duvic B. Isolation, characterization, kinetics, and enzymatic and nonenzymatic microbicidal activities of a novel c-type lysozyme from plasma of schistocerca gregaria (Orthoptera: Acrididae). J Insect Sci (2015) 15:57. doi: 10.1093/jisesa/iev038
43. Yang D, Zha G, Li X, Gao H, Yu H. Immune responses in the haemolymph and antimicrobial peptide expression in the abdomen of apis mellifera challenged with spiroplasma melliferum CH-1. Microb Pathog (2017) 112:279–87. doi: 10.1016/j.micpath.2017.10.006
44. Xu SL, Qiu CG, Zhou W, Wang DL, Jia CY, Wang CL. Pathological analysis of hemolymphs of charybdis japonica infected with vibrio alginolyticus. Fish Shellfish Immunol (2013) 35:1577–84. doi: 10.1016/j.fsi.2013.08.025
45. Kim H, Choi D, Jung J, Kim Y. Eicosanoid mediation of immune responses at early bacterial infection stage and its inhibition by photorhabdus temperata subsp. temperata, an entomopathogenic bacterium. Arch Insect Biochem Physiol (2018) 99:e21502. doi: 10.1002/arch.21502
46. Chaimanee V, Chantawannakul P, Chen Y, Evans JD, Pettis JS. Differential expression of immune genes of adult honey bee (Apis mellifera) after inoculated by nosema ceranae. J Insect Physiol (2012) 58:1090–5. doi: 10.1016/j.jinsphys.2012.04.016
47. Branchiccela B, Arredondo D, Higes M, Invernizzi C, Martín-Hernández R, Tomasco I, et al. Characterization of nosema ceranae genetic variants from different geographic origins. Microb Ecol (2017) 73:978–87. doi: 10.1007/s00248-016-0880-z
48. Nystrand M, Dowling DK. Dose-dependent effects of an immune challenge at both ultimate and proximate levels in drosophila melanogaster. J Evol Biol (2014) 27:876–88. doi: 10.1111/jeb.12364
49. Ahmed AM, Hurd H. Immune stimulation and malaria infection impose reproductive costs in anopheles gambiae via follicular apoptosis. Microbes Infect (2006) 8:308–15. doi: 10.1016/j.micinf.2005.06.026
50. Shang F, Niu JZ, Ding BY, Zhang Q, Ye C, Zhang W, et al. Vitellogenin and its receptor play essential roles in the development and reproduction of the brown citrus aphid, aphis (Toxoptera) citricidus. Insect Mol Biol (2018) 27:221–33. doi: 10.1111/imb.12366
51. Yao Q, Xu S, Dong Y, Que Y, Quan L, Chen B. Characterization of vitellogenin and vitellogenin receptor of Conopomorpha sinensis Bradley and their responses to sublethal concentrations of insecticide. Front Physiol (2018) 9:1250. doi: 10.3389/fphys.2018.01250
52. Macfarlane CP, Hoysak DJ, Liley NR, Gage MJ. In vitro fertilization experiments using sockeye salmon reveal that bigger eggs are more fertilizable under sperm limitation. Proc Biol Sci (2009) 276:2503–7. doi: 10.1098/rspb.2009.0295
53. Marshall DJ, Keough MJ. The evolutionary ecology of offspring size in marine invertebrates. Adv Mar Biol (2007) 53:1–60. doi: 10.1016/S0065-2881(07)53001-4
54. Azevedo RB, French V, Partridge L. Life-history consequences of egg size in drosophila melanogaster. Am Nat (1997) 150:250–82. doi: 10.1086/286065
55. Carrière Y, Masaki S, Roff DA. The coadaptation of female morphology and offspring size: A comparative analysis in crickets. Oecologia (1997) 110:197–204. doi: 10.1007/s004420050150
56. Mappes J, Mappes T, Lappalainen T. Unequal maternal investment in offspring quality in relation to predation risk. Evol Ecol (1997) 11:237–43. doi: 10.1023/a:1018408201713
57. Sota T, Mogi M. Interspecific variation in desiccation survival time of aedes (Stegomyia) mosquito eggs is correlated with habitat and egg size. Oecologia (1992) 90:353–8. doi: 10.1007/BF00317691
58. Braby MF. The significance of egg size variation in butterflies in relation to host plant quality. Oikos (1994) 71:119–29. doi: 10.2307/3546179
59. Fox CW, Mousseau TA. Larval host plant affects fitness consequences of egg size variation in the seed beetle stator limbatus. Oecologia (1996) 107:541–8. doi: 10.1007/BF00333946
60. Fox CW. The influence of maternal age and mating frequency on egg size and offspring performance in callosobruchus maculatus (Coleoptera: Bruchidae). Oecologia (1993) 96:139–46. doi: 10.1007/BF00318042
61. Durham MF, Magwire MM, Stone EA, Leips J. Genome-wide analysis in drosophila reveals age-specific effects of SNPs on fitness traits. Nat Commun (2014) 5:4338. doi: 10.1038/ncomms5338
Keywords: immunity, reproduction, Locusta migratoria manilensis, Paranosema locustae, trade-off
Citation: Hu Y-W, Wang S-H, Tang Y, Xie G-Q, Ding Y-J, Xu Q-Y, Tang B, Zhang L and Wang S-G (2022) Suppression of yolk formation, oviposition and egg quality of locust (Locusta migratoria manilensis) infected by Paranosema locustae. Front. Immunol. 13:848267. doi: 10.3389/fimmu.2022.848267
Received: 04 January 2022; Accepted: 27 June 2022;
Published: 21 July 2022.
Edited by:
Erjun Ling, Shanghai Institutes for Biological Sciences (CAS), ChinaReviewed by:
Kai Wu, Shangrao Normal University, ChinaIoannis Eleftherianos, George Washington University, United States
Copyright © 2022 Hu, Wang, Tang, Xie, Ding, Xu, Tang, Zhang and Wang. This is an open-access article distributed under the terms of the Creative Commons Attribution License (CC BY). The use, distribution or reproduction in other forums is permitted, provided the original author(s) and the copyright owner(s) are credited and that the original publication in this journal is cited, in accordance with accepted academic practice. No use, distribution or reproduction is permitted which does not comply with these terms.
*Correspondence: Shi-Gui Wang, sgwang@hznu.edu.cn
†These authors have contributed equally to this work and share first authorship