- 1Department of Emergency Medicine, Zhongshan Hospital, Fudan University, Shanghai, China
- 2Endoscopy Center and Endoscopy Research Institute, Zhongshan Hospital, Fudan University, Shanghai, China
- 3 Shanghai Key Laboratory of Lung Inflammation and Injury, Shanghai, China
- 4Shanghai Institute of Infectious Disease and Biosecurity, School of Public Health, Fudan University, Shanghai, China
Background: Dysfunction of the immune system would disturb the intestinal homeostasis and lead to inflammatory bowel disease (IBD). Dendritic cells (DCs) help maintain intestinal homeostasis and immediately respond to pathogens or injuries once the mucosa barriers are destroyed during IBD. G protein-coupled receptors(GPR)174 is an essential regulator of immunity that is widely expressed in most immune cells, including DCs. However, the role of GPR174 in regulating the immune function of DC in colitis has not been investigated.
Methods: Dextran sodium sulfate (DSS) was administered to establish the mice colitis model. Data of weight, length of colon, disease activity index (DAI), and macroscopic scores were collected. The flow cytometry was used to detect the infiltrations of T cells and DCs, the mean fluorescence intensity (MFI) of CD80, CD86, CD40, and major histocompatibility complex-II (MHC-II). And T cells proliferataion was measured by carboxyfluorescein diacetate succinimidyl ester (CFSE). The expression of cytokines (tumor necrosis factor-α (TNF-α), interleukin-6 (IL-6), interleukin-10 (IL-10), interferon-γ (IFN-γ), interleukin -4 (IL-4)) and GPR174 mRNA were measured by Elisa, quantitative polymerase chain reaction (qPCR), and immunofluorescence. RNA of bone-marrow-derived dendritic cells (BMDCs) was extracted for sequencing. Adoptive transfer of BMDCs was administrated intravenously.
Results: Gpr174-/- mice exposed to 3% DSS showed significant alleviation characterized by reduced loss of weight, more minor colon damage, and better DAI and macroscopic scores. The expression of pro-inflammatory cytokines (TNF-α, IL-6) decreased, while anti-inflammatory cytokine (IL-10) increased compared with WT mice. In vitro, Gpr174-/- BMDCs showed less maturity, with a declined expression of MHC-II, CD80, CD86 and reduced TNF-α, higher IL-10 after LPS stimulation. Gpr174-/- BMDCs were less capable of activating OT-II naïve CD4+ T cells than WT BMDCs and induced more Th0 cells to differentiate into Treg while less into Th1. Furthermore, the transcriptome sequencing analysis exhibited that Gpr174 participated in TNF-α (NF-κB) signaling, leukocyte transendothelial migration, and Th1/Th2 cell differentiation pathways. Adoptive transfer of Gpr174-/- BMDCs to WT mice ameliorated DSS-induced colitis.
Conclusion: Our study indicated that GPR174 was involved in the pathogenesis of IBD by regulating the maturation of the dendritic cells to maintain immune homeostasis. TNF-α (NF-κB) signaling pathway, leukocyte transendothelial migration, and Th1/Th2 cell differentiation pathways may be the target pathway.
Introduction
Inflammatory bowel disease (IBD), consisting of Crohn’s disease (CD) and ulcerative colitis (UC), is a multifaceted disease resulting from genetic, autoimmune, and environmental factors, which in fact, is an inflammatory condition of the gastrointestinal tract (1). Although precise pathogenesis is not clear, the immune response caused by the invasion of intestinal pathogens has been regarded as a pathogenic factor. The sustaining and aberrant inflammation responses disturb the mucosa and mucosal immunity homeostasis, leading to inappropriate and magnified intestinal inflammation (2).
Dendritic cells (DCs) maintain intestinal homeostasis and respond at the first time to pathogens or injuries once the mucosa barriers are destroyed during IBD (3). Clinical evidence indicates that the numbers and subsets of CD103+ dendritic cells change in inflamed mucosa, display loss of tolerogenic function, and disorder of cytokine profiles in IBD patients (4, 5). Similarly, dextran sulfate sodium salt (DSS)-induced colitis is developed in severe combined immunodeficiency (SCID) mice, suggesting that acute DSS colitis did not require the presence of either T cells or B cells, while dendritic cells participate in the pathogenesis of IBD independently (6, 7).
G protein-coupled receptors (GPCRs) are critical signaling molecules in immune response, cell proliferation, inflammation regulation, and intestinal barrier maintenance (8). One of the GPCRs, GPR174, is widely expressed in the intestine, spleen, thymus, and lymph nodes (9), is widely expressed in most immune cells, including T/B lymphocytes, and DCs, and is involved in many infectious diseases, including sepsis (10, 11). Gpr174 has also been reported to play an important role in gender dimorphism of humoral immunity and be more susceptible to experimental autoimmune encephalomyelitis. It suppresses germinal center formation in male mice and is a chemokine receptor for the CCL21 ligand (12).
However, whether GPR174 could regulate the immune function of DCs in IBD has not been investigated. The present study aimed to identify GPR174 involved in colitis by both in vivo and vitro studies. In addition, we focused on the relationship between GPR174 and DCs function, which may play a critical role in regulating the intestinal injury of colitis.
Methods
Animals
Male wild-type C57BL/6 and male Gpr174 knockout mice (Gpr174-/-) (8-12 weeks, 20-25g) were obtained from the Southern Model Biological Technology Development Co. (Shanghai, China) and bred under pathogen-free conditions. Animals were housed separately in a temperature-controlled room with a 12-hour light/12-hour dark cycle and free food and water access. All experimental procedures and animal operations followed the international guidelines for the Care and Use of Laboratory Animals (NO. 201804001Z).
Induction and Assessment of DSS-Induced Colitis
The acute colitis mice model (n=25) was induced by oral administration of 3% DSS (35-50 kDa)(Sigma-Aldrich, St. Louis, MO, United States) for seven days and two days on regular drinking water (13). The control group of mice (n=25) was only fed with regular drinking water during the study. The health condition was monitored daily, and the disease activity index (DAI), including weight loss, stool consistency, and stool bleeding, was scored as described (14). All mice were sacrificed on day 9 after the intraperitoneal injection of avertin, and the colon was collected for length and gross macroscopic appearance (15).
Intestinal Permeability Assay
Intestinal permeability was measured by fluorescein isothiocyanate (FITC)-dextran (3,000-5,000 kDa) (Sigma-Aldrich, St. Louis, MO, United States) (16). Briefly, 9 days after oral administration of DSS, mice were fasted for 4 h and then gavaged with FITC-dextran (0.5 mg/g body weight at 125 mg/mL). Four hours later, blood taken from the abdominal aorta was centrifuged at 12,000 g for 5 min. The serum was collected to detect the FITC-dextran by a microplate reader with an excitation wavelength of 490 nm and an emission wavelength of 520 nm.
Myeloperoxidase (MPO) Assay
The MPO activity assay was applied to assess the neutrophil infiltration into the colon. The distal colon was homogenized in 4 volumes of MPO Assay buffer (Jiancheng Co. Ltd., Nanjing, China) and centrifuged at 13,000 g for 10 min at 4 °C. Prepare a Master Mix of the reagent according to the instructions, then add the reaction mix to each well containing the controls and samples. Mix and incubate at 25°C for hours. Add stop mix to all wells and incubate at room temperature and TNB for 5-10 min. At last, measure output (OD412 nm) on a microplate reader.
HE Staining and Histopathological Evaluation
About 2 cm in length, the colonic tissue was excised on the ninth day after drinking DSS-water, washed in phosphate-buffered saline (PBS), embedded in 4% formaldehyde in paraffin sectioned about 2 μm, and stained with hematoxylin and eosin (H&E) (17).
Quantitative RT-PCR
RNA was isolated from the whole colonic tissues using the Qiagen RNeasy Mini Kit following the manufacturer’s instructions. Quantitative polymerase chain reaction (qPCR) was performed for expressions of mRNAs using the primers for hGPR174, mIl-6, mIl-10, mTnf-α, mOccludin, mZo-1, and β-actin.
Bone Marrow-Derived Dendritic Cells (BMDCs)
Bone marrow cells were extracted from femurs and tibiae of C57BL/6(n=5) and Gpr174-/- mice(n=5). After incubated in Red blood cell (RBC) lysis buffer at 37°C for 5 min, cells were seeded in six-well plates at 106 cells/well concentration with RPMI-1640 medium supplemented with 20 ng/mL of granulocyte-macrophage colony-stimulating factor, 10% fetal bovine serum, 100 U/mL penicillin, and 100 mg/mL streptomycin. On day 3, the culture medium was changed to remove the nonadherent granulocyes without dislodging the cluster of dendritic cells and macrophages. On day 5, the suspension and loosely adherent dendritic cells were harvested by gently swirling the dishes to get rid of the bone-marrow derived macrophages which is tightly adherent to the dishes (18).
Flow Cytometry
An anti-FcR antibody was used to block the non-specific staining. Cells were subsequently immunostained with APC-conjugated anti-CD11c, APC-cy7-conjugated anti-CD86, and FITC-conjugated anti-MHC-II antibodies (Biolegend, USA).
BMDCs and Naïve CD4+ Cell In Vitro Co-Culture Assays
BMDCs were prepared as previously described and purified by CD11c+ magnetic beads. BMDCs were co-cultured with isolated OT-II naïve CD4+ T cells at a 1:10 ratio in complete RPMI in 96-well V-bottom plates. Cells were harvested 3 days later for further analysis. OVA peptide 323-339 (GenScript, USA) was added to wells at 1 mg/mL as a positive control.
CFSE Proliferation Assay
Isolate naïve CD4+ T cells from OT-II mice(n=5), label with carboxyfluorescein N-succinimidyl ester (CFSE, C34554; Life Technologies), and culture in the presence of WT BMDCs and Gpr174-/- DCs. Unstimulated T cells serve as a negative control. The percentage of divided cells was analyzed by FlowJo V10.
RNA Sequencing and Functional Analysis
Treated with LPS for 12 h or left untreated, BMDCs were harvested for RNA extraction by QIAGEN RNeasy Mini Kit. The Agilent 4200 TapeStation System was used to check RNA integrity to ensure RNA integrity was ≥ 8.9. The TruSeq Stranded mRNA Library Prep Kit (Illumina) made libraries for RNASeq using 1-1.5 μg of RNA as input. The HiSeq 4000 Sequencing Systems (Illumina) was used to run across two lanes to yield 2 × 151 bp paired-end reads with an average yield of ~55 million reads/sample. a
Fisher’s exact test was used to calculate differential gene expression. If it met the cut-off criteria, it was considered significantly differentially. An R Bioconductor package heatmap3 was used to visualize the differential gene expression (https://cran.r-project.org/package=heatmap3). DAVID (version 6.8) was used for gene term enrichment.
Patient Enrollment
The Research and Ethics committee of Zhongshan Hospital, Fudan University, approved this study (NO: B2020-016R). All procedures were directed under guidelines. Samples from ten patients with ulcerative colitis (UC) collected under colonoscopy were used for qPCR and immunofluorescence.
Statistical Analysis
Data were expressed as mean ± standard error (SEM). One-way ANOVA, t-test followed by Bonferroni tests was performed using GraphPad Prism 7.0. Transcriptome analysis was visualized using the R prcomp function and ggplot package. A P value < 0.05 was considered statistically significant.
Results
Gpr174-/- Mice Were Resistant to DSS-Induced Colitis
To explore whether the GPR174 receptor is involved in the pathophysiology of IBD, we established a DSS-induced colitis animal model as previously described. After oral administration of DSS for 7 days and 2 days on regular drinking water, Gpr174 -/- mice showed improved acute colitis symptoms compared with WT mice. Gpr174-/- mice significantly decreased DAI scores (Figure 1B, P < 0.01), with less weight loss (Figure 1A, P < 0.05) and later appearance of diarrhea loose feces. Decreased loose bloody stools and a lower reduction in colon length were shown in Gpr174-/- mice compared with the WT mice (Figures 1C, D, P < 0.01). Furthermore, compared with the WT mice, Gpr174-/- mice exhibited minor architecture damage or epithelial barrier disruption, leading to the improved macroscopic scores with DSS treatment (Figures 1E, F, P < 0.01).
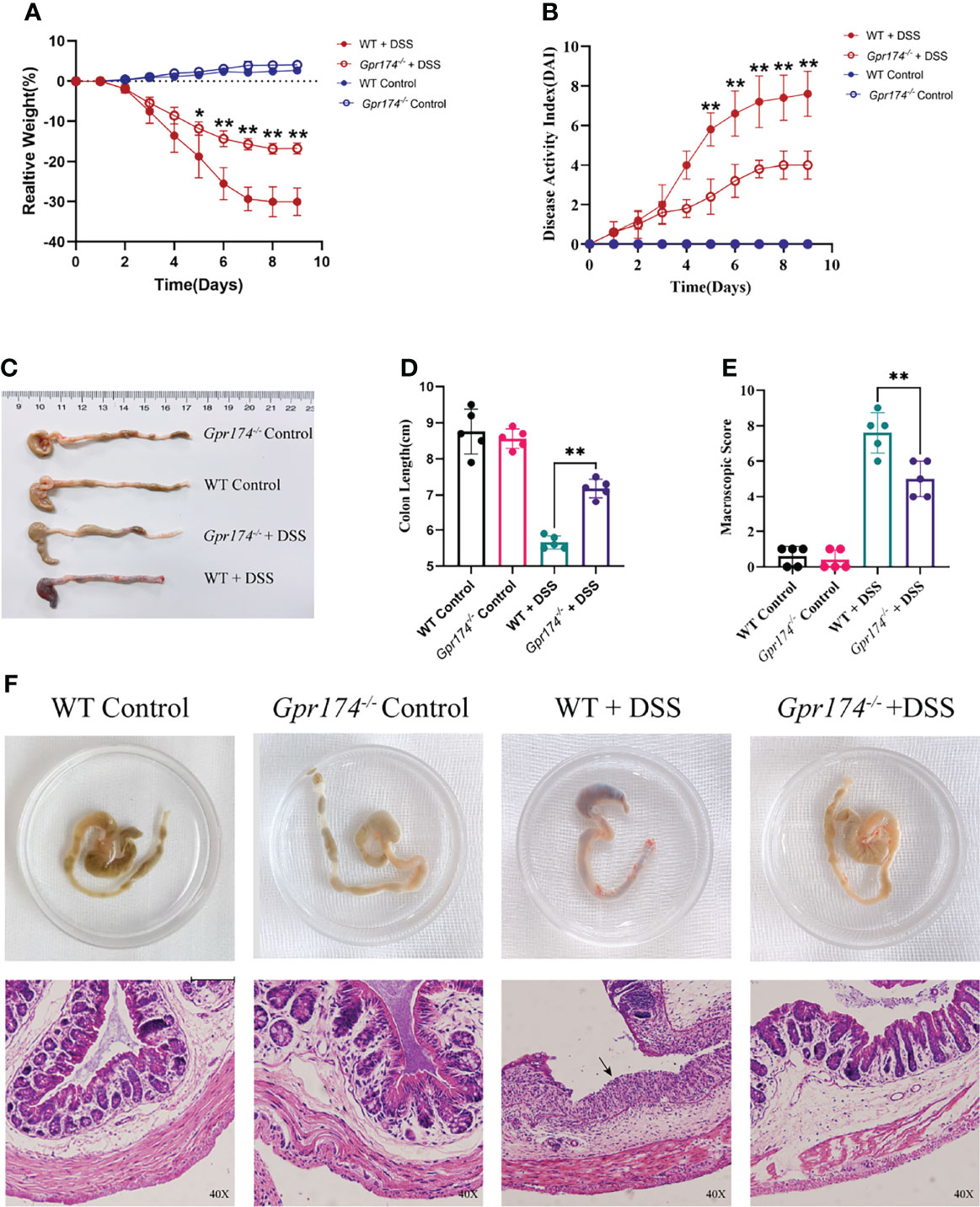
Figure 1 Gpr174-/- mice were resistant to DSS-induced colitis. (A) Body weight loss in each group, (B) DAI scores in each group, (C, D) Colon length of each group, (E) Macroscopic score of each group, (F) Morphology and HE of the intestine of each group. Data were exhibited as mean ± SEM of 5 mice per group. (Black arrow: epithelial barrier disruption. *P < 0.05, **P < 0.01).
Knockout of Gpr174 Reduced Gut Inflammatory Response and Maintained Intestinal Barrier in DSS-Induced Colitis
The intestinal barrier damage is caused by inflammatory responses and is characterized by epithelial barrier dysfunction and increased mucosal permeability. Myeloperoxidase (MPO), reflecting the degree of neutrophil infiltration, was decreased in the distal colonic tissue of Gpr174-/- mice compared with WT mice (Figure 2A, P < 0.01). To analyze why the Gpr174-/- mice reduced susceptibility to DSS, we evaluated the production of pro-inflammatory and anti-inflammatory cytokines in the colonic tissue. The results showed that Gpr174-/- mice treated with DSS demonstrated decreased levels of TNF-α (P < 0.01), IL-6 (P < 0.01), and increased levels of IL-10 (P < 0.001) compared with WT mice (Figure 2B). In addition, our study indicated that the expression of Zo-1 mRNA (Figure 2C, P < 0.05) and Occludin (Figure 2D, P < 0.01) in Gpr174-/- mice was significantly higher than that in WT mice. Besides, we observed that serum FITC-dextran was markedly decreased in Gpr174-/- mice compared with WT ones 6 h after gavaging with FITC-dextran (Figure 2E, P < 0.01), suggesting that epithelial permeability was reduced in the Gpr174-/- mice.
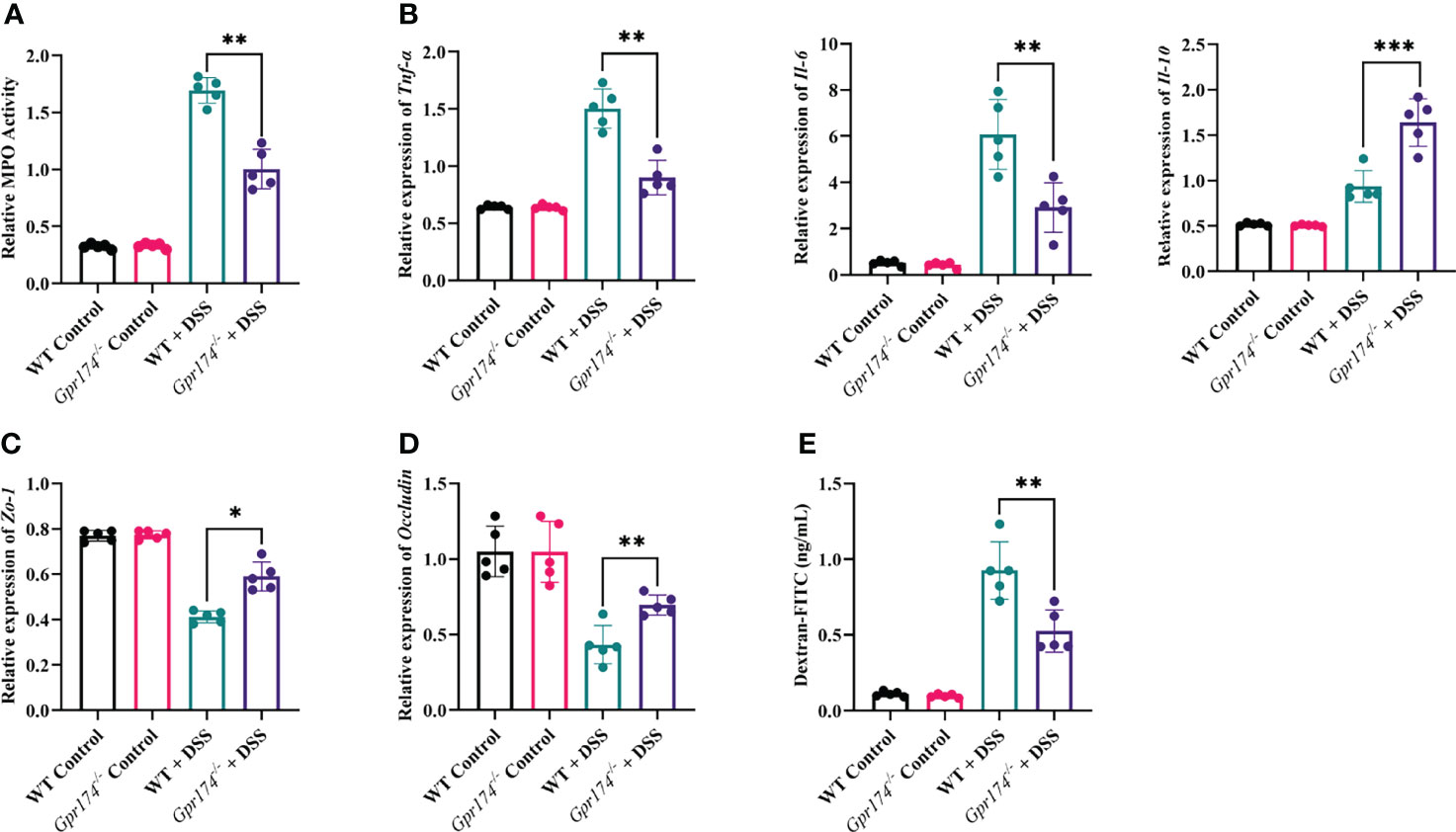
Figure 2 Gpr174 knockout reduced intestinal inflammation and protected the intestinal barrier. (A) Intestinal MPO expression in each group, (B) Intestinal cytokine expression of TNF-α, IL-6, IL-10 in each group, (C, D) Intestinal tight junctions of Zo-1 and Occludin mRNA expression in each group, (E) Intestinal permeability in each group. Data were exhibited as mean ± SEM of 5 mice per group. (*P < 0.05, **P < 0.01, ***P < 0.001).
Gpr174 Knockout Influenced CD11c+ Dendritic Cells Infiltration in Intestinal Lamina Propria(LP) in DSS-Induced Colitis
Due to intestinal inflammation, both WT and Gpr174-/- mice exhibited increased CD11c+ DCs accumulation in LP compared with the WT ones after model establishment. The number of DCs in LP showed no difference between WT and Gpr174-/- mice, while the percentage of CD11c+ DC, one of the most potent antigen-presenting cells, increased in Gpr174-/- mice than in WT mice (Figures 3A, B, P < 0.05). However, the expression of the MHC-II molecule decreased (Figure 3C).
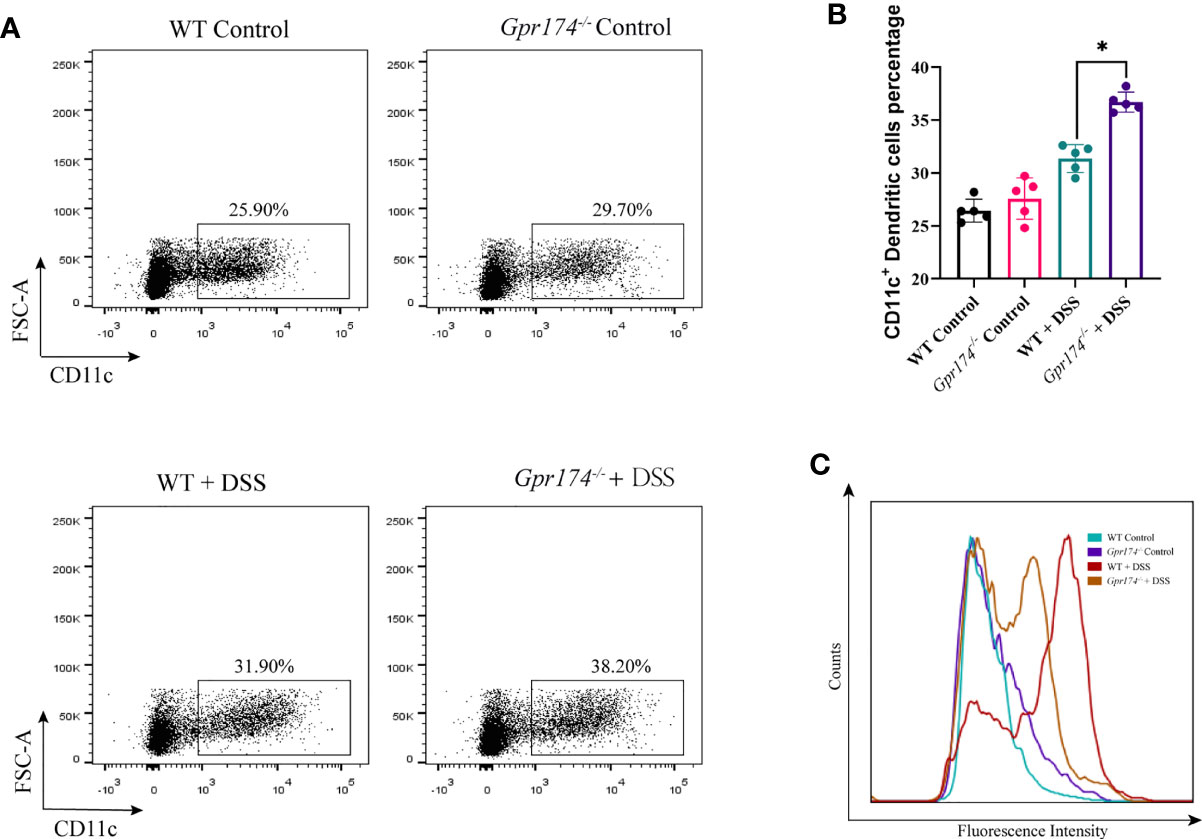
Figure 3 Gpr174 knockout increased CD11c+ dendritic cells infiltration while suppressed maturation in the lamina propria. (A, B) Infiltration of CD11c+ dendritic cells in the lamina propria in each group, (C) MHC-II expression of CD11c+ dendritic cells in each group. Data were exhibited as mean ± SEM of 5 mice per group. (*P < 0.05).
Gpr174 Knockout Led to Difficulty of BMDC Maturation
To further analyze the role of Gpr174 on the immune function of CD11c+ DC, we examined the expression of co-stimulatory molecules of BMDCs. BMDCs extracted from the bone marrow of Gpr174-/- mice were less prone to differentiate into CD11c+ cells, especially on the seventh day of culture (Figure 4A). Both Gpr174+/+ and Gpr174-/- BMDCs upregulated the expression of MHC II, CD80, CD86, and CD40 with LPS stimulation. However, the expression of MHC II, CD80, and CD86 increased much less in Gpr174-/- BMDCs than that in Gpr174+/+ (Figure 4B).
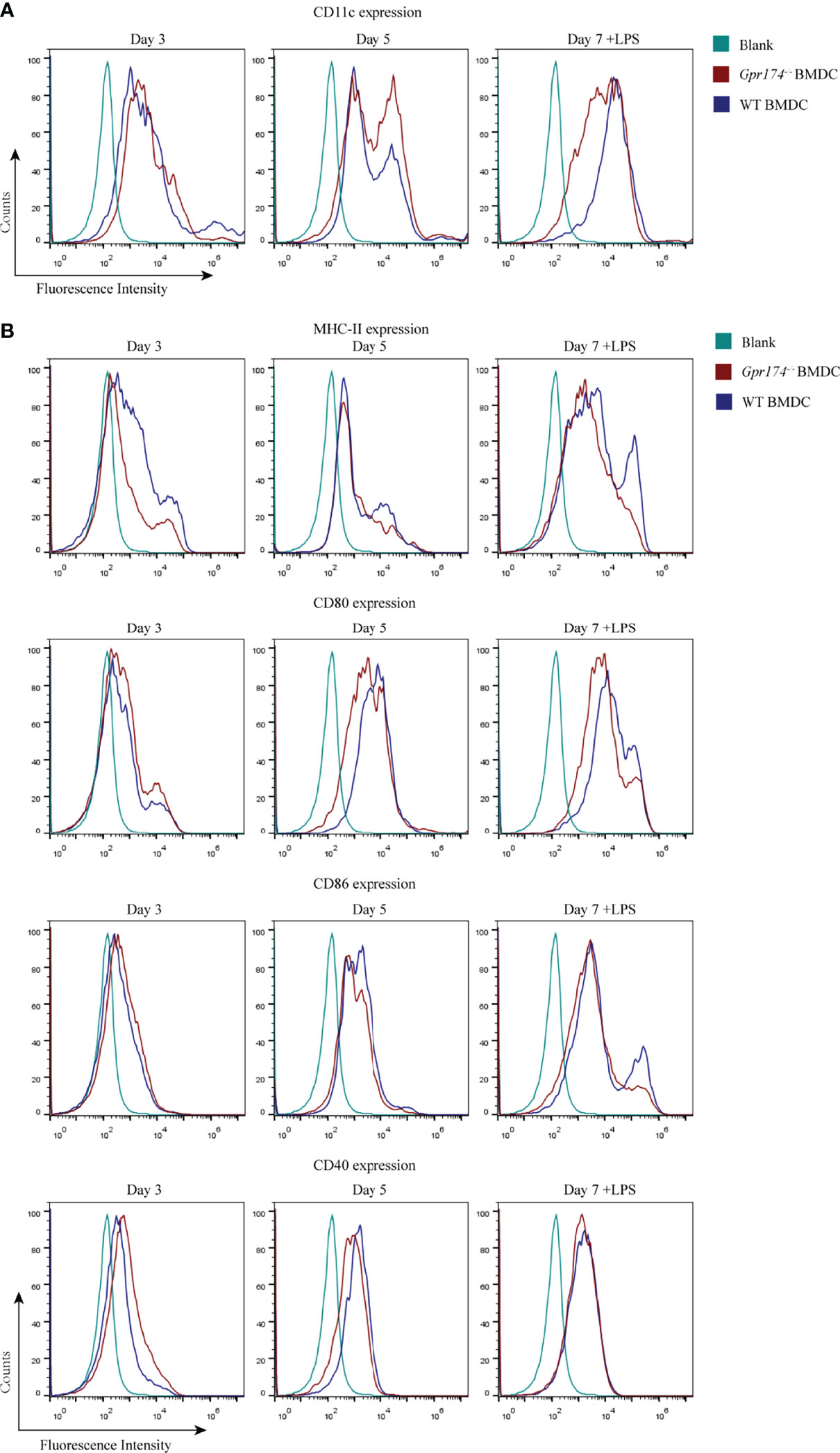
Figure 4 Gpr174 knockout inhibited maturation of BMDCs. (A) CD11c+ expression of BMDCs in each group, (B) Co-stimulatory molecular MHC-II, CD80, CD86, CD40 BMDCs. Data were exhibited as mean ± SEM of 5 mice per group.
Gpr174 Knockout Suppressed BMDCs to Naïve T Cell Proliferation and Differentiation
The naïve CD4+ T cells purified from the spleens of OT-II OVA-specific T cell receptor transgenic mice were used to examine the effects of Gpr174 on BMDCs to stimulate naïve T cell cytokine production. Gpr174-/- BMDCs induced less naïve T cell proliferation than Gpr174+/+ BMDCs (Figure 5A, P < 0.01). Co-culturing with Gpr174-/- BMDCs led to a significant reduction of IFN-γ (Figure 5B, P < 0.01) and an increase in the production of IL-10 in CD4+ T cells (Figure 5D, P < 0.05), while without any change in IL-4 (Figure 5C), indicating that Gpr174-/- BMDCs induced more naïve CD4+ T cells to differentiate into Treg cells, while less into Th1 cells.
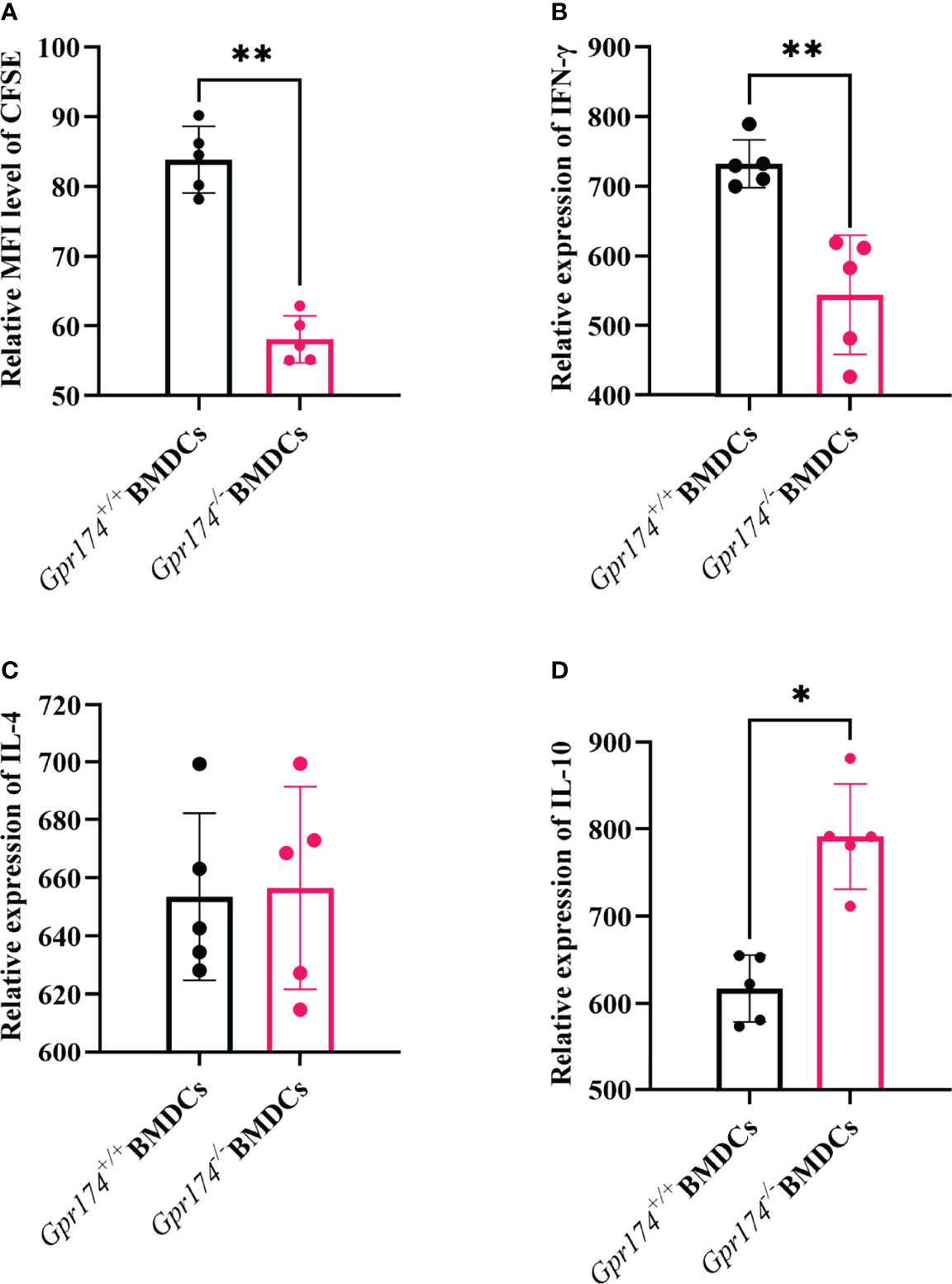
Figure 5 Gpr174 knockout inhibited T cell proliferation and influenced T cell differentiation. (A) Degree of naive T cells proliferation, which was labeled as CFSE after stimulation in each group, (B) IFN-γ expression of naive CD4+ T cells after stimulation in each group, (C) IL-4 expression of naive CD4+ T cells after stimulated in each group, (D) IL-10 expression of naive CD4+ T cells after stimulated in each group. Data were exhibited as mean ± SEM of 5 mice per group. (*P < 0.05, **P < 0.01).
Adoptively Transfer of Gpr174-/- BMDCs Alleviated DSS-Induced Colitis
To verify the function of Gpr174-/- BMDCs in alleviating DSS-induced colitis, we adoptively transfer Gpr174-/- BMDCs and Gpr174+/+ BMDCs into C57/BL6 mice intravenously at 5 days and 3 days before and 1 day after the induction of colitis, flow chart of procedure were shown in Figure 6C. Mice transferred with Gpr174-/- BMDCs were in a better condition after administration of DSS, characterized by the decreased weight loss (Figure 6A, P<0.05), ameliorative DAI scores (Figure 6B, P<0.01), better macroscopic scores (Figure 6D, P<0.01), and reduced shortening of colon length (Figures 6E, F P<0.05). Less disruption of the epithelium in mice treated with Gpr174-/- BMDCs than the Gpr174+/+ BMDCs was exhibited by H&E staining (Figure 6G). Besides, the inflammatory response was mitigated in the ones transferred with Gpr174-/- BMDCs, with MPO (Figure 7A, P < 0.05), TNF-α (Figure 7B, P < 0.05), and IL-6 (Figure 7C) declined, IL-10 (Figure 7D, P < 0.001) increased in the colon. In addition, T cells were reduced in the LP transferred with Gpr174-/- BMDCs (Figure 7E, P < 0.01)
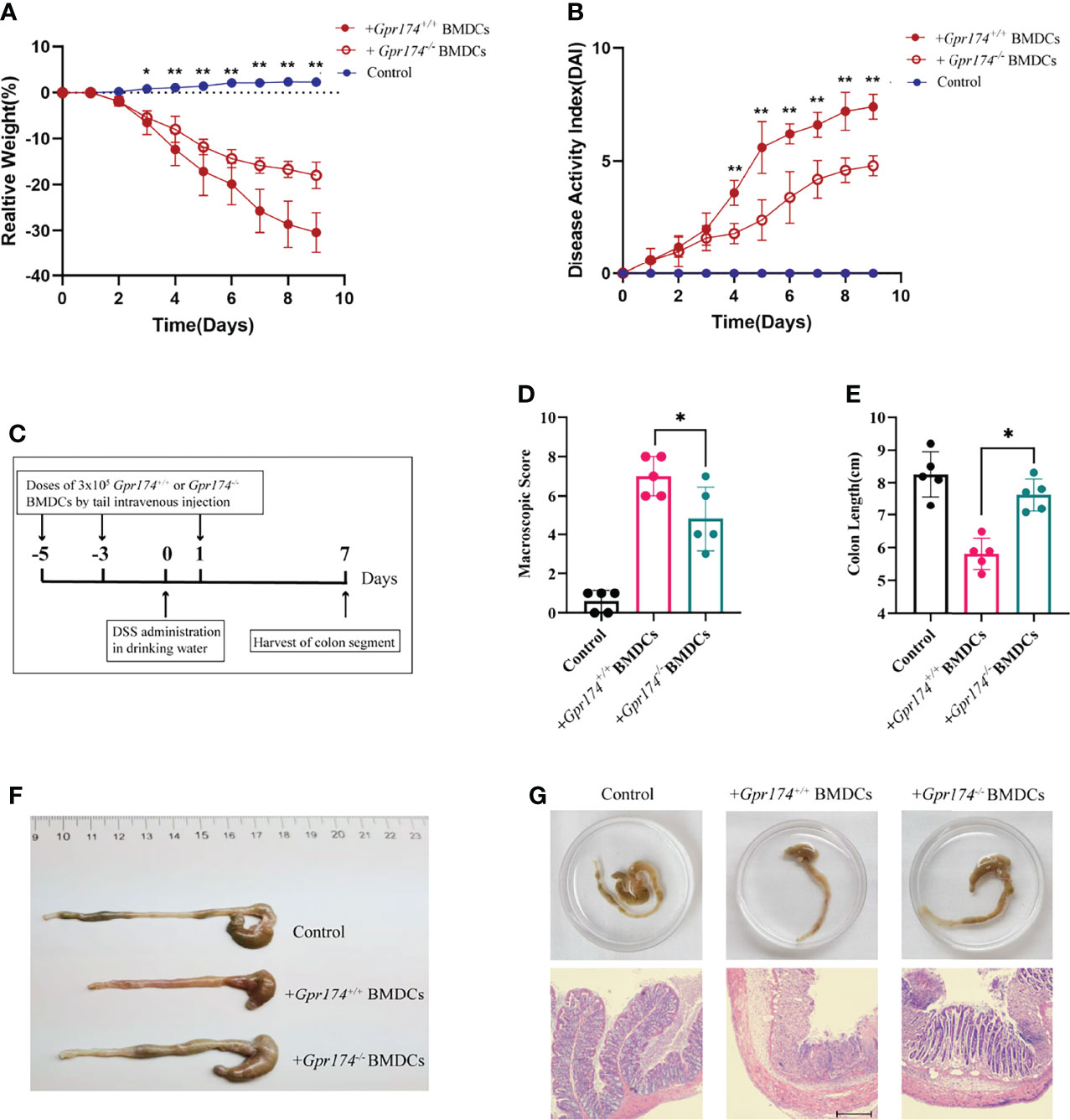
Figure 6 Adoptive transfer of Gpr174-/- BMDCs alleviated DSS-induced colitis. (A) Body weight loss in each group after adoptive transfer of Gpr174-/- DC, (B) DAI scores in each group, (C) Flow chart of the procedure of adoptive transfer of BMDCs and establishing DSS-induced colitis animal models, (D) Macroscopic score of each group, (E, F) Colon length of each group, (G) Morphology and HE of the intestine of each group. Data were exhibited as mean ± SEM of 5 mice per group. (*P < 0.05, **P < 0.01).
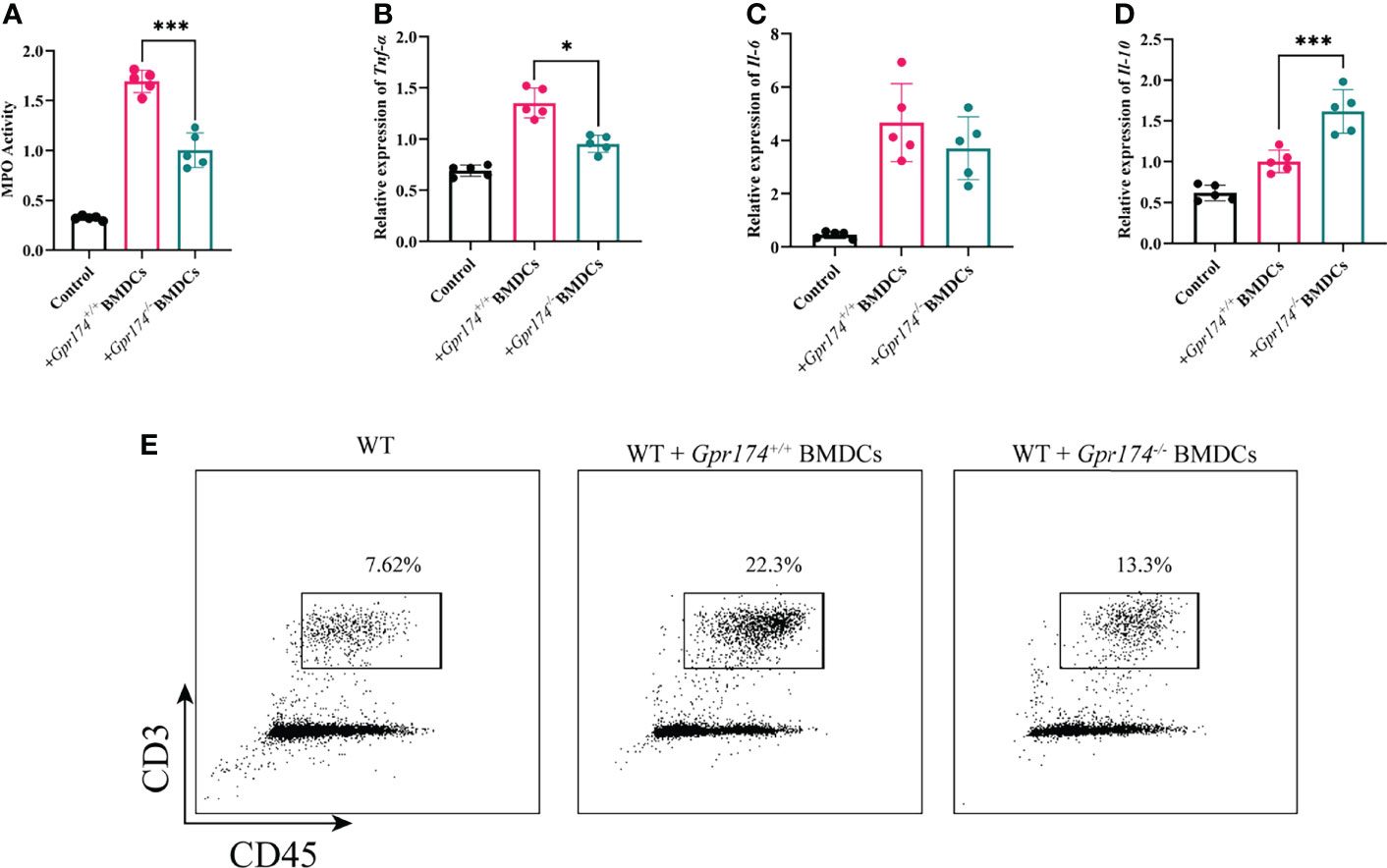
Figure 7 Adoptive transfer of Gpr174-/- BMDCs alleviated inflammatory response of colitis. (A) Colonic MPO expression in each group, (B) Colonic cytokine expression of TNF-α in each group, (C) Intestinal cytokine expression of IL-6 in each group, (D) Intestinal cytokine expression of IL-10 in each group, (E) CD3+ T cells infiltration in the lamina propria in each group (*P < 0.05, ***P < 0.001).
Gpr174 Knockout Altered Dendritic Cells Transcriptome After Maturation
Gpr174+/+ BMDCs and Gpr174-/- BMDCs were subjected to transcriptome analysis to reveal the role of Gpr174 in the differentiation and maturation of BMDCs. LPS led to significant modulation of gene expression between Gpr174-/- BMDCs and Gpr174+/+ BMDCs. However, no significant differences were observed between Gpr174-/- BMDCs and Gpr174+/+ BMDCs without LPS stimulation (Figures 8A, B). TNF-α was extracted from protein to protein interaction (PPI) network analysis of DEGs to be a hub gene (Figure 8C). The enrichment of KEGG analysis suggested that the TNF-α (NF-κB) signaling pathway and leukocyte transendothelial migration pathway may be the potential target pathway of Gpr174 (Figure 8D).
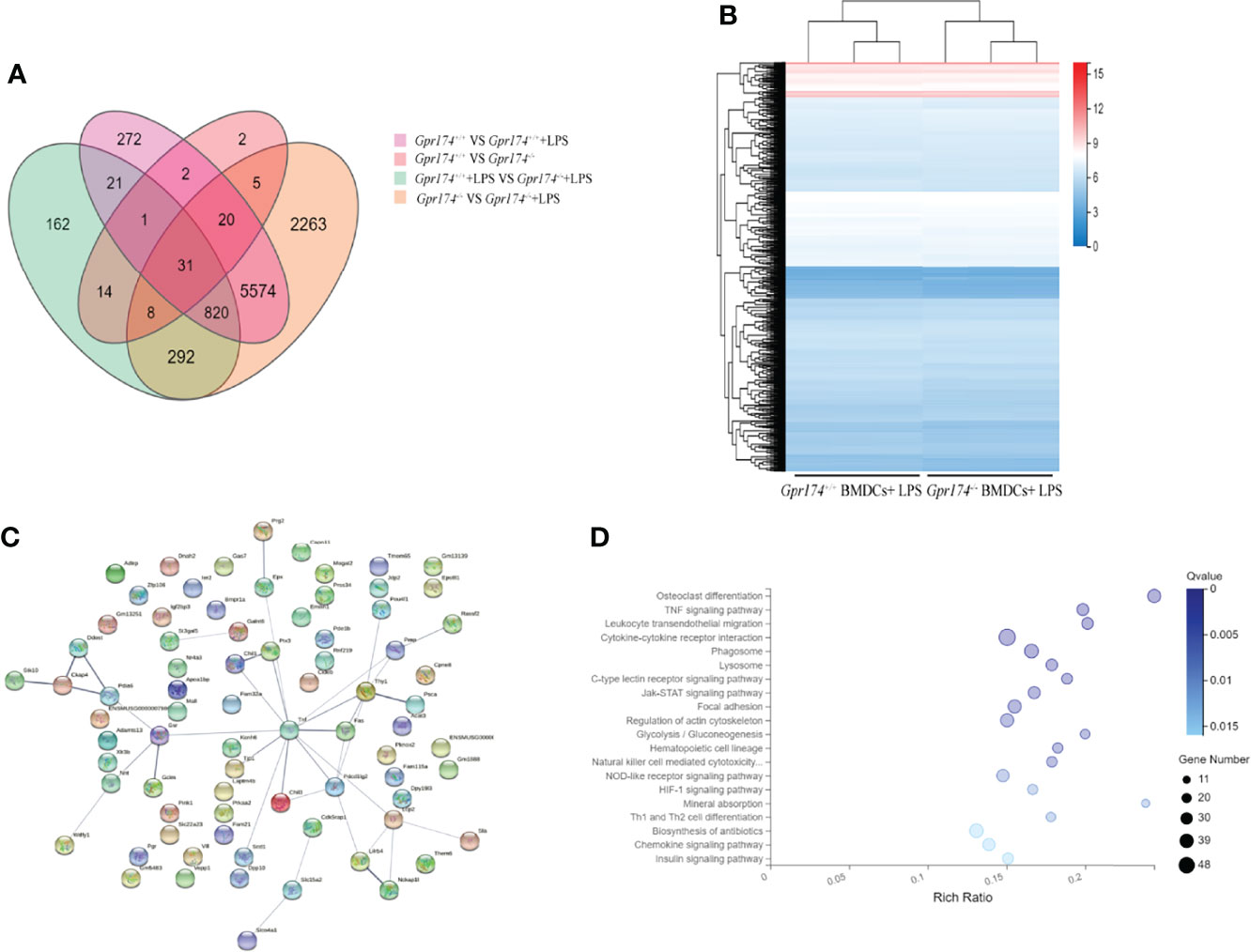
Figure 8 Gpr174 knockout influences BMDCs transcriptome after LPS stimulation. (A) Venn diagram of differential expression genes (DEGs) of four groups of BMDCs before and after LPS application, (B) Heatmap of DEGs of BMDCs in each group after LPS application, (C) Protein-protein interactions (PPI) networks analysis of DEGs of Gpr174-/- BMDCs and Gpr174+/+ BMDCs after LPS application, (D) KEGG analysis of DEGs of Gpr174-/- BMDCs and Gpr174+/+ BMDCs after LPS application (the first twenty 20 pathways with ease < 0.05, count ≥ 10, and FDR < 0.01).
GPR174 Expression Was Decreased in the Inflamed Mucosa of UC Patients
To further confirm the role of GPR174 in the pathogenesis of UC, the inflamed mucosas of the UC patients(n=10) were collected. The expression of GPR174 mRNA was relatively lower in the pathological site than in the healthy control (Figure 9A, P < 0.05). Likewise, the immunofluorescence showed less GPR174 expression in the inflamed tissues (Figure 9B).
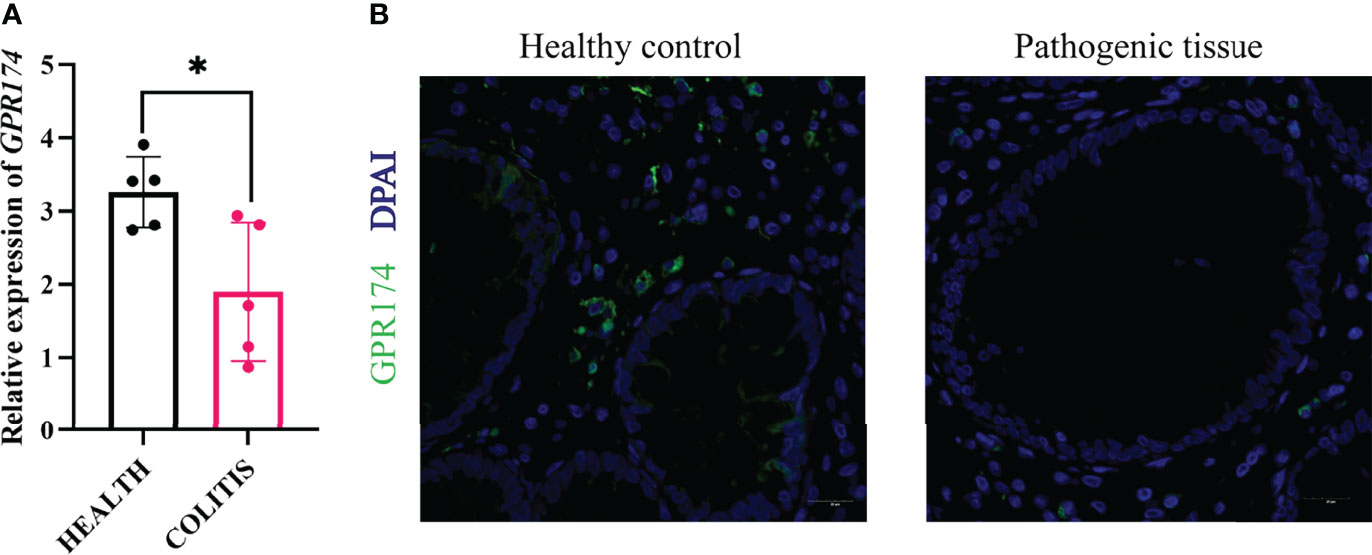
Figure 9 Expression of GPR174 between healthy and pathogenic tissues. (A) The mRNA expression of GPR174 between healthy and pathogenic tissues from the ulcerative patients(n=10), (B) The protein expression of GPR174 receptor between healthy and pathogenic tissues from the ulcerative patients (Blue: DAPI; Green: GPR174). (*P < 0.05).
Discussion
Our study showed that Gpr174 knockout reduced inflammatory response and altered the phenotype of DCs in colitis intestines. Gpr174 knockout lowered the expression of co-stimulatory molecules and influenced the ability of BDMCs to mature, which further altered the proliferation and differentiation of naïve T cells. T cell activation is a critical step in the intestinal immune system, and only the activated T cells exert an efficient mucosa immune response. Moreover, we adoptively transferred Gpr174-/- BMDCs to alleviate DSS-induced colitis and gut injury. The transcriptomic analysis revealed NF-κB signaling pathway participated in the process related to BMDCs maturation. Furthermore, the expression of GPR174 mRNA was decreased in the inflamed mucosa in IBD patients.
GPCRs are critical signaling molecules in immune response, inflammation regulation, cell proliferation (19), cell migration (20), and intestinal barrier maintenance (8, 21). Researchers have revealed that GPR43 could bind with short-chain fatty acids (SCFAs) to regulate the inflammatory responses in intestinal diseases, which indicated that GPCRs took a critical part in the pathogenesis of IBD. Our previous study demonstrated that Gpr174 knockout elevated the number of marginal zone B cells in the spleen of mice and promoted the function of regulatory T cells, cytotoxic T lymphocytes, and M2 macrophage polarization (11, 22). In this study, we found that deletion of Gpr174 could generally alleviate intestinal injury in the DSS-induced colitis, which considered Gpr174 participated in the pathogenesis of IBD.
Moreover, GPCRs also act as a navigator in the migration of dendritic cells from peripheral to draining Lymph nodes (LNs) and their maturation process (23). GPCRs such as chemokine receptors CCR7 and CCR4 are highly upregulated on DCs upon maturation. Moreover, GPCRs like GPR183 and CXCR5 help direct DCs to certain areas upon inflammation (24). DCs, a bridge between innate and acquired immune systems, maintain the homeostasis of intestinal immunity in the LP, were found to decrease colitis after Gpr174 knockout in this study.
We chose BMDCs to investigate the role of GPR174 in regulating the function of DCs because they could be abundantly generated in vitro and were widely applied as a model myeloid DC (25). In our study, Gpr174 modulated murine BMDCs maturation and migration. We found that deletion of the Gpr174 in BMDCs hardly affected the expression of CD86, CD80, or MHC-II on non-stimulated BMDCs. However, after being stimulated by LPS, Gpr174-/- BMDCs showed a different expression profile of co-stimulatory molecules, such as CD80, CD86, and MHC-II, which were significantly decreased, indicating that Gpr174-/- BMDCs remained an immature phenotype. Immature DCs possess a solid ability to phagocytose and digest antigens and then process and present antigens and gain a mature phenotype. Once BMDCs acquire mature phenotypes, they are empowered to activate naïve T/B cells (26, 27). Mature DCs migrate from LP to lymph nodes. They initiate naïve T/B cells and induce them to proliferate and differentiate. Many studies indicated that tolerant DCs could reduce the severity of DSS-induced colitis, probably due to their cytokine profiles and weakened immunity. Activated DCs also secrete pro-inflammatory cytokines to intercede the inflammatory regulation in UC by activating TLRs, which induce infiltration of neutrophils and activation of other innate immune cells (28). Like DCs and macrophages, cytokines released by APCs and macrophages trigger naïve T/B cell differentiation into various subsets. Consistent with the alteration of maturity, Gpr174 -/- BMDCs exhibited the impaired ability to stimulate naïve T cells to proliferation and differentiation.
Preliminary studies demonstrated that stimulation of OT-II CD4+ T cells co-cultured with DCs and 50 nM OVA (329-337 peptides) induced a high expression level of both IFN-γ and IL-4 a modest level of IL-10 (29, 30). In vitro experiments, our study indicated that Gpr174-/- BMDCs were less capable of stimulating naïve CD4+ T cells. Lumen antigens were endocytosed, digested by DCs, and then presented to naïve T/B cells to initiate systemic and mucosal immunity (31). Activated DCs in LP would acquire maturity and migrate to mesenteric lymph node (MLN) and secondary lymphoid tissues with antigens to translate innate to adaptive immune response. In addition, effector T cell subsets which were differentiated after binding with DCs also changed. Following activation, naïve CD4+ T cells differentiated into several types of effector T cells, subdivided into either Th1, Th2, or Treg cells according to cytokine profiles.
The pathogenesis of IBD may be an excessive activation of effector T cells and alteration of T cell-mediated tolerance, which is related to Treg development or alteration (32). An animal experiment showed that SAMP1/YitFc mice, which had massively expanded B cells in the intestine, developed transmural gut inflammation by blocking Tregs’ immune function (33). Helper T cells are critical mediators of the immune response, while regulatory T cells have a robust immune suppression function. Recent studies demonstrated that the immune imbalance between CD4+ T cells, Th1/Th2, and Treg might be the most direct and vital factor in the pathogenesis of IBD. It also played a role in mediating the occurrence of ulcerative colitis (34, 35). The current study suggested a reducing ratio of Th1/Th2 and Treg in LP alleviated inflammatory response in colonic tissue. Less effector T cells reduced pro-inflammatory factors release, thereby alleviating intestinal damage.
Pro-inflammatory cytokines released in the gut are involved in IBD and have different functions, including cellular adhesion, differentiation, and transmigration (36). Our results strongly suggested that increased pro-inflammatory cytokines in the gut of mice treated with DSS accounted for the observed exacerbation of colitis. Our study showed that Gpr174-/- mice with DSS treatment suppressed the pro-inflammatory factors like TNF-α and IL-6 and promoted the anti-inflammatory factor IL-10. A delicate balance of pro-and anti-inflammatory factors is necessary to preserve intestinal homeostasis. The increase of IL-10 in the Gpr174-/- mice might provide compensative mechanisms for improvements in inflammation status.
Cytokines could disrupt the intestinal epithelium, promote barrier permeability, and modulate the tight junctions (TJs). TJs are important for barrier integrity and play an essential role in intestinal homeostasis. It is reported that TNF-α on the intestinal epithelium could disrupt the epithelial barrier and decrease the tight junction proteins expression, including occludin and Zo-1 (37, 38). Once immune cells entered intestinal mucosa through broken TJs, the raised TNF-α and IFN-γ triggered cytokine storm made the intestinal barrier disruption heavier (39–41). This study showed increased occludin and Zo-1 mRNA levels and reduced macromolecule permeability in Gpr174-/- intestinal mucosa compared with Gpr174 +/+ ones, indicating Gpr174 knockout helped maintain the intensity of the intestinal mucosa in DSS treated mice.
To further validate whether Gpr174-/- BMDCs have protective effects on IBD, we transferred Gpr174-/- BMDCs into DSS-induced colitis animals. Adoptive transfer of Gpr174-/- BMDCs could significantly improve intestinal damage and suppress the inflammatory response in both innate and acquired immunity. Some clinical research revealed that patients with active IBD lacked immature peripheral blood plasmacytoid and myeloid DCs (42). DCs with immature phenotype could induce immune tolerance by inducing T regulatory cells and amplifying suppressor activity, which may have anti-inflammatory effects (43).
The progression of IBD is closely related to the immune system (44). Animal studies showed that the GPR174 was involved in IBD’s pathogenic course; some clinical evidence must be unearthed. Therefore, we took samples from ulcerative patients and set the non-lesion site as healthy controls to combine with clinical practice. The mRNA expression of GPR174 decreased in the inflamed tissues compared with healthy controls, which was consistent with the immunofluorescence results. This might be because the samples we collected were the patients in the late disease courses of colitis. We stimulated BMDCs of WT mice with LPS to validate this idea and found Gpr174 increased in the first 24h. Moreover, we collected the healthy controls’ PBMC to induce the monocyte-derived DCs (MODCs) and found the GPR174 began to increase in the 12h after LPS stimulation in vitro. Therefore, in the early course of LPS stimulation and colitis, the GPR174 might increase, while in the late course of the disease, the GPR174 expression decreased (Figure S1). In-depth studies are required to confirm these observations further.
Furthermore, we did some transcriptome analysis of Gpr174+/+ BMDCs and Gpr174-/- BMDCs, which enhanced the speculation that Gpr174 mainly participated in the complete maturation process. Studies showed that TNF-α cytokines and the NF-κB signaling pathway were indispensable elements in the differentiation and maturation process in BMDCs (45, 46). In this study, we found that the expression of TLR4, MYD88, IKKα, IκB-α, and p-NF-κB were decreased in Gpr174-/- with LPS stimulation. Taken together, we considered that deletion of Gpr174 might inhibit the NF-κB pathway and suppress BMDCs maturation.
In summary, our work revealed that the knockout of GPR174 could reduce intestinal inflammation and repair the epithelium barrier by suppressing DCs maturation and naïve T cell differentiation to alleviate DSS-induced colitis, which was probably through inhibiting the TNF-α (NF-κB) pathway.
Data Availability Statement
The datasets presented in this study can be found in online repositories. The names of the repository/repositories and accession number(s) can be found below: https://www.ncbi.nlm.nih.gov/bioproject/, PRJNA819571.
Ethics Statement
The studies involving human participants were reviewed and approved by NO:B2020-016R. The patients/participants provided their written informed consent to participate in this study. The animal study was reviewed and approved by NO. 201804001Z.
Author Contributions
ZS and WW designed the research plan. WW, SM and YH conducted the experiments, analyzed all data, and wrote the manuscript. WW, SM and YH were co-first authors. ZK helped with the mice experiment. YC and YL assisted with some data analysis. YZ and XW collaborated to collect endoscopy biopsies. CT and YY supervised the experimental work and data analysis. ZS, YY and YZ were co-corresponding authors. All authors participated in revising the manuscript and agreed to the final version.
Funding
This work was supported by the National Natural Science Foundation of China (Grant No. 82072214), National Key Research and Development Program of China (2021YFC2501800), Science and Technology of Shanghai Committee (Grant No. 20Y11900100, Grant No. 21MC1930400, Grant No.20DZ2261200), and Shanghai Municipal Health Bureau (Grant No. ZXYXZ-201906, Grant No. GWV-10.2-XD04).
Conflict of Interest Statement
The authors declare that the research was conducted in the absence of any commercial or financial relationships that could be construed as a potential conflict of interest.
Publisher’s Note
All claims expressed in this article are solely those of the authors and do not necessarily represent those of their affiliated organizations, or those of the publisher, the editors and the reviewers. Any product that may be evaluated in this article, or claim that may be made by its manufacturer, is not guaranteed or endorsed by the publisher.
Acknowledgments
The authors wish to thank all the patients enrolled in this study.
Supplementary Material
The Supplementary Material for this article can be found online at: https://www.frontiersin.org/articles/10.3389/fimmu.2022.841254/full#supplementary-material
Abbreviations
AAD, autoimmune Addison’s disease; BMDCs, bone-marrow-derived dendritic cells; DAI, disease activity index; DC, dendritic cells; GPCRs, G protein-coupled receptors; IBD, inflammatory bowel disease; SCFAs, short-chain fatty acids; TJ, tight junction.
References
1. Head KA, Jurenka JS. Inflammatory Bowel Disease Part 1: Ulcerative Colitis–Pathophysiology and Conventional and Alternative Treatment Options. Altern Med Rev (2003) 8:247–83.
2. Jiang LY, Zhang M, Zhou TE, Yang ZF, Wen LQ, Chang JX. Changes of the Immunological Barrier of Intestinal Mucosa in Rats With Sepsis. World J Emerg Med (2010) 1:138–43.
3. Stagg AJ. Intestinal Dendritic Cells in Health and Gut Inflammation. Front Immunol (2018) 9:2883–3. doi: 10.3389/fimmu.2018.02883
4. Middel P, Raddatz D, Gunawan B, Haller F, Radzun HJ. Increased Number of Mature Dendritic Cells in Crohn's Disease: Evidence for a Chemokine Mediated Retention Mechanism. Gut (2006) 55:220–7. doi: 10.1136/gut.2004.063008
5. Baumgart DC, Metzke D, Guckelberger O, Pascher A, Grötzinger C, Przesdzing I, et al. Aberrant Plasmacytoid Dendritic Cell Distribution and Function in Patients With Crohn's Disease and Ulcerative Colitis. Clin Exp Immunol (2011) 166:46–54. doi: 10.1111/j.1365-2249.2011.04439.x
6. Dieleman LA, Ridwan BU, Tennyson GS, Beagley KW, Bucy RP, Elson CO. Dextran Sulfate Sodium-Induced Colitis Occurs in Severe Combined Immunodeficient Mice. Gastroenterology (1994) 107:1643–52. doi: 10.1016/0016-5085(94)90803-6
7. Qualls JE, Tuna H, Kaplan AM, Cohen DA. Suppression of Experimental Colitis in Mice by CD11c+ Dendritic Cells. Inflammation Bowel Dis (2009) 15:236–47. doi: 10.1002/ibd.20733
8. Lämmermann T, Kastenmüller W. Concepts of GPCR-Controlled Navigation in the Immune System. Immunol Rev (2019) 289:205–31. doi: 10.1111/imr.12752
9. Chu X, Shen M, Xie F, Miao XJ, Shou WH, Liu L, et al. An X Chromosome-Wide Association Analysis Identifies Variants in GPR174 as a Risk Factor for Graves' Disease. J Med Genet (2013) 50:479–85. doi: 10.1136/jmedgenet-2013-101595
10. Napier C, Mitchell AL, Gan E, Wilson I, Pearce SH. Role of the X-Linked Gene GPR174 in Autoimmune Addison's Disease. J Clin Endocrinol Metab (2015) 100:E187–90. doi: 10.1210/jc.2014-2694
11. Zhu M, Li C, Song Z, Mu S, Wang J, Wei W, et al. The Increased Marginal Zone B Cells Attenuates Early Inflammatory Responses During Sepsis in Gpr174 Deficient Mice. Int Immunopharmacol (2020) 81:106034. doi: 10.1016/j.intimp.2019.106034
12. Zhao R, Chen X, Ma W, Zhang J, Guo J, Zhong X, et al. A GPR174-CCL21 Module Imparts Sexual Dimorphism to Humoral Immunity. Nature (2020) 577:416–20. doi: 10.1038/s41586-019-1873-0
13. Breynaert C, de Bruyn M, Arijs I, Cremer J, Martens E, Van Lommel L, et al. Genetic Deletion of Tissue Inhibitor of Metalloproteinase-1/TIMP-1 Alters Inflammation and Attenuates Fibrosis in Dextran Sodium Sulphate-Induced Murine Models of Colitis. J Crohns Colitis (2016) 10:1336–50. doi: 10.1093/ecco-jcc/jjw101
14. Ghia JE, Blennerhassett P, Collins SM. Impaired Parasympathetic Function Increases Susceptibility to Inflammatory Bowel Disease in a Mouse Model of Depression. J Clin Invest (2008) 118:2209–18. doi: 10.1172/JCI32849
15. Kimball ES, Wallace NH, Schneider CR, D'Andrea MR, Hornby PJ. Vanilloid Receptor 1 Antagonists Attenuate Disease Severity in Dextran Sulphate Sodium-Induced Colitis in Mice. Neurogastroenterol Motil (2004) 16:811–8. doi: 10.1111/j.1365-2982.2004.00549.x
16. An G, Wei B, Xia B, McDaniel JM, Ju T, Cummings RD, et al. Increased Susceptibility to Colitis and Colorectal Tumors in Mice Lacking Core 3-Derived O-Glycans. J Exp Med (2007) 204:1417–29. doi: 10.1084/jem.20061929
17. Fischer AH, Jacobson KA, Rose J, Zeller R. Hematoxylin and Eosin Staining of Tissue and Cell Sections. CSH Protoc (2008) 2008:pdb.prot4986. doi: 10.1101/pdb.prot4986
18. Janelsins BM, Mathers AR, Tkacheva OA, Erdos G, Shufesky WJ, Morelli AE, et al. Proinflammatory Tachykinins That Signal Through the Neurokinin 1 Receptor Promote Survival of Dendritic Cells and Potent Cellular Immunity. Blood (2009) 113:3017–26. doi: 10.1182/blood-2008-06-163121
19. Szekanecz Z, Koch AE. Successes and Failures of Chemokine-Pathway Targeting in Rheumatoid Arthritis. Nat Rev Rheumatol (2016) 12:5–13. doi: 10.1038/nrrheum.2015.157
20. Williams IR. CCR6 and CCL20: Partners in Intestinal Immunity and Lymphorganogenesis. Ann N Y Acad Sci (2006) 1072:52–61. doi: 10.1196/annals.1326.036
21. Ferrer-Picón E, Dotti I, Corraliza AM, Mayorgas A, Esteller M, Perales JC, et al. Intestinal Inflammation Modulates the Epithelial Response to Butyrate in Patients With Inflammatory Bowel Disease. Inflammation Bowel Dis (2020) 26:43–55. doi: 10.1093/ibd/izz119
22. Qiu D, Chu X, Hua L, Yang Y, Li K, Han Y, et al. Gpr174-Deficient Regulatory T Cells Decrease Cytokine Storm in Septic Mice. Cell Death Dis (2019) 10:233. doi: 10.1038/s41419-019-1462-z
23. Worbs T, Hammerschmidt SI, Förster R. Dendritic Cell Migration in Health and Disease. Nat Rev Immunol (2017) 17:30–48. doi: 10.1038/nri.2016.116
24. Woodruff MC, Heesters BA, Herndon CN, Groom JR, Thomas PG, Luster AD, et al. Trans-Nodal Migration of Resident Dendritic Cells Into Medullary Interfollicular Regions Initiates Immunity to Influenza Vaccine. J Exp Med (2014) 211:1611–21. doi: 10.1084/jem.20132327
25. Lutz MB, Kukutsch N, Ogilvie AL, Rössner S, Koch F, Romani N, et al. An Advanced Culture Method for Generating Large Quantities of Highly Pure Dendritic Cells From Mouse Bone Marrow. J Immunol Methods (1999) 223:77–92. doi: 10.1016/S0022-1759(98)00204-X
26. Hammer GE, Ma A. Molecular Control of Steady-State Dendritic Cell Maturation and Immune Homeostasis. Annu Rev Immunol (2013) 31:743–91. doi: 10.1146/annurev-immunol-020711-074929
27. Merad M, Sathe P, Helft J, Miller J, Mortha A. The Dendritic Cell Lineage: Ontogeny and Function of Dendritic Cells and Their Subsets in the Steady State and the Inflamed Setting. Annu Rev Immunol (2013) 31:563–604. doi: 10.1146/annurev-immunol-020711-074950
28. Geremia A, Biancheri P, Allan P, Corazza GR, Di Sabatino A. Innate and Adaptive Immunity in Inflammatory Bowel Disease. Autoimmun Rev (2014) 13:3–10. doi: 10.1016/j.autrev.2013.06.004
29. Connor LM, Tang SC, Camberis M, Le Gros G, Ronchese F. Helminth-Conditioned Dendritic Cells Prime CD4+ T Cells to IL-4 Production In Vivo. J Immunol (2014) 193:2709–17. doi: 10.4049/jimmunol.1400374
30. Keshavarz Shahbaz S, Varasteh AR, Koushki K, Ayati SH, Mashayekhi K, Sadeghi M, et al. Sublingual Dendritic Cells Targeting by Aptamer: Possible Approach for Improvement of Sublingual Immunotherapy Efficacy. Int Immunopharmacol (2020) 85:106603. doi: 10.1016/j.intimp.2020.106603
31. Neurath MF. Cytokines in Inflammatory Bowel Disease. Nat Rev Immunol (2014) 14:329–42. doi: 10.1038/nri3661
32. Larmonier CB, Shehab KW, Ghishan FK, Kiela PR. T Lymphocyte Dynamics in Inflammatory Bowel Diseases: Role of the Microbiome. BioMed Res Int (2015) 2015:504638. doi: 10.1155/2015/504638
33. Olson TS, Bamias G, Naganuma M, Rivera-Nieves J, Burcin TL, Ross W, et al. Expanded B Cell Population Blocks Regulatory T Cells and Exacerbates Ileitis in a Murine Model of Crohn Disease. J Clin Invest (2004) 114:389–98. doi: 10.1172/JCI200420855
34. Bai A, Lu N, Zeng H, Li Z, Zhou X, Chen J, et al. All-Trans Retinoic Acid Ameliorates Trinitrobenzene Sulfonic Acid-Induced Colitis by Shifting Th1 to Th2 Profile. J Interferon Cytokine Res (2010) 30:399–406. doi: 10.1089/jir.2009.0028
35. Li J, Hou W, Lin S, Wang L, Pan C, Wu F, et al. Polydopamine Nanoparticle-Mediated Dopaminergic Immunoregulation in Colitis. Adv Sci (Weinh) (2022) 9:e2104006. doi: 10.1002/advs.202104006
36. Marafini I, Sedda S, Dinallo V, Monteleone G. Inflammatory Cytokines: From Discoveries to Therapies in IBD. Expert Opin Biol Ther (2019) 19:1207–17. doi: 10.1080/14712598.2019.1652267
37. Ye X, Sun M. AGR2 Ameliorates Tumor Necrosis Factor-α-Induced Epithelial Barrier Dysfunction via Suppression of NF-κb P65-Mediated MLCK/p-MLC Pathway Activation. Int J Mol Med (2017) 39:1206–14. doi: 10.3892/ijmm.2017.2928
38. Watari A, Sakamoto Y, Hisaie K, Iwamoto K, Fueta M, Yagi K, et al. Rebeccamycin Attenuates TNF-α-Induced Intestinal Epithelial Barrier Dysfunction by Inhibiting Myosin Light Chain Kinase Production. Cell Physiol Biochem (2017) 41:1924–34. doi: 10.1159/000472367
39. Bruewer M, Luegering A, Kucharzik T, Parkos CA, Madara JL, Hopkins AM, et al. Proinflammatory Cytokines Disrupt Epithelial Barrier Function by Apoptosis-Independent Mechanisms. J Immunol (2003) 171:6164–72. doi: 10.4049/jimmunol.171.11.6164
40. Schmitz H, Fromm M, Bentzel CJ, Scholz P, Detjen K, Mankertz J, et al. Tumor Necrosis Factor-Alpha (TNFalpha) Regulates the Epithelial Barrier in the Human Intestinal Cell Line HT-29/B6. J Cell Sci (1999) 112(Pt 1):137–46. doi: 10.1242/jcs.112.1.137
41. Madara JL, Stafford J. Interferon-Gamma Directly Affects Barrier Function of Cultured Intestinal Epithelial Monolayers. J Clin Invest (1989) 83:724–7. doi: 10.1172/JCI113938
42. Baumgart DC, Metzke D, Schmitz J, Scheffold A, Sturm A, Wiedenmann B, et al. Patients With Active Inflammatory Bowel Disease Lack Immature Peripheral Blood Plasmacytoid and Myeloid Dendritic Cells. Gut (2005) 54:228–36. doi: 10.1136/gut.2004.040360
43. Gad M, Kristensen NN, Kury E, Claesson MH. Characterization of T-Regulatory Cells, Induced by Immature Dendritic Cells, Which Inhibit Enteroantigen-Reactive Colitis-Inducing T-Cell Responses In Vitro and In Vivo. Immunology (2004) 113:499–508. doi: 10.1111/j.1365-2567.2004.01977.x
44. Ungaro R, Mehandru S, Allen PB, Peyrin-Biroulet L, Colombel J-F. Ulcerative Colitis. Lancet (London England) (2017) 389:1756–70. doi: 10.1016/S0140-6736(16)32126-2
45. Berges C, Naujokat C, Tinapp S, Wieczorek H, Höh A, Sadeghi M, et al. A Cell Line Model for the Differentiation of Human Dendritic Cells. Biochem Biophys Res Commun (2005) 333:896–907. doi: 10.1016/j.bbrc.2005.05.171
Keywords: GPR174, inflammatory bowel disease, dendritic cells, intestinal barrier function, T cell activation
Citation: Wei W, Mu S, Han Y, Chen Y, Kuang Z, Wu X, Luo Y, Tong C, Zhang Y, Yang Y and Song Z (2022) Gpr174 Knockout Alleviates DSS-Induced Colitis via Regulating the Immune Function of Dendritic Cells. Front. Immunol. 13:841254. doi: 10.3389/fimmu.2022.841254
Received: 22 December 2021; Accepted: 25 April 2022;
Published: 20 May 2022.
Edited by:
Chih-Hao Chang, Jackson Laboratory, United StatesReviewed by:
Wenru Su, Sun Yat-Sen University, ChinaShubha Priyamvada, University of Illinois at Chicago, United States
Copyright © 2022 Wei, Mu, Han, Chen, Kuang, Wu, Luo, Tong, Zhang, Yang and Song. This is an open-access article distributed under the terms of the Creative Commons Attribution License (CC BY). The use, distribution or reproduction in other forums is permitted, provided the original author(s) and the copyright owner(s) are credited and that the original publication in this journal is cited, in accordance with accepted academic practice. No use, distribution or reproduction is permitted which does not comply with these terms.
*Correspondence: Zhenju Song, c29uZy56aGVuanVAenMtaG9zcGl0YWwuc2guY24=; Yilin Yang, eWFuZy55aWxpbkB6cy1ob3NwaXRhbC5zaC5jbg==; Yiqun Zhang, emhhbmcueWlxdW5AenMtaG9zcGl0YWwuc2guY24=
†These authors share first authorship