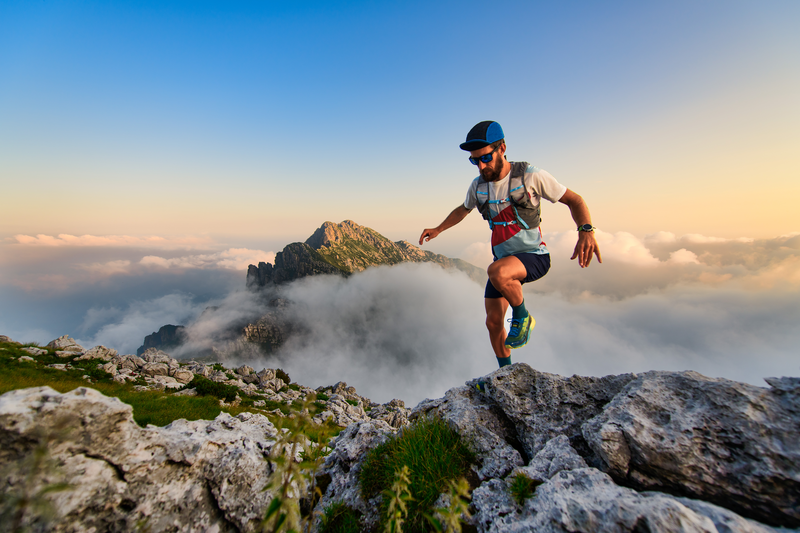
94% of researchers rate our articles as excellent or good
Learn more about the work of our research integrity team to safeguard the quality of each article we publish.
Find out more
ORIGINAL RESEARCH article
Front. Immunol. , 15 February 2022
Sec. Cytokines and Soluble Mediators in Immunity
Volume 13 - 2022 | https://doi.org/10.3389/fimmu.2022.837642
This article is part of the Research Topic The Role of Circulating Immune Mediators in the Crosstalk Between Cells of the Immune System and Cardiovascular Systems in CVDs View all 7 articles
Background: The level of systemic inflammation correlates with the severity of the clinical course of acute myocardial infarction (AMI). It has been shown that circulating cytokines and endothelial dysfunction play an important role in the process of clot formation. The aim of our study was to assess the concentration of various circulating cytokines, endothelial function and blood clotting in AMI patients depending on the blood flow through the infarction-related artery (IRA).
Methods: We included 75 patients with AMI. 58 presented with ST-elevation myocardial infarction (STEMI) and 17 had non-ST-elevation myocardial infarction (non-STEMI). A flow-mediated dilation test (FMD test), thrombodynamics and rotational thromboelastometry as well as assessment of 14 serum cytokines using xMAP technology were performed.
Findings: Non-STEMI-patients were characterized by higher levels of MDC, MIP-1β, TNF-α. Moreover, we observed that patients with impaired blood flow through the IRA (TIMI flow 0-1) had higher average and initial clot growth rates, earlier onset of spontaneous clots, C-reactive protein (CRP) and IL-10 compared to patients with preserved blood flow through the IRA (TIMI flow 2-3). Patients with TIMI 2-3 blood flow had higher level of IP-10. IL-10 correlated with CRP and pro-inflammatory cytokines levels, initial clot growth rate and clot lysis time in TIMI 0-1 patients. All these differences were statistically significant.
Interpretation: We demonstrated that concentrations of the inflammatory cytokines correlate not only with the form of myocardial infarction (STEMI or non-STEMI), but also with the blood flow through the infarct-related artery. Inflammatory response, functional state of endothelium, and clot formation are closely linked with each other. A combination of these parameters affects the patency of the infarct-related artery.
The cornerstone in the pathogenesis of acute myocardial infarction (AMI) is the interaction between the endothelium, hemostasis and the chronic inflammation that accompanies atherosclerosis (1, 2).
Currently, AMI is divided into ST-elevation myocardial infarction (STEMI) and non-ST-elevation myocardial infarction (non-STEMI) according to electrocardiogram at presentation (3). These types of AMI differ in the blood flow through the infarct-related artery (IRA): while the majority of patients with STEMI demonstrate occlusion of IRA on coronary angiography, the development of IRA occlusion in patients with non-STEMI is uncommon. Nevertheless, approximately 4-24% of STEMI cases are characterized by the development of spontaneous reperfusion (SR) of the IRA (4), while up to 25% of non-STEMI cases may be accompanied by occlusion (5).
Despite the fact that certain determinants of the patency of the IRA are well-known (younger age, the absence of significant concomitant diseases, a lower contents of lipoprotein (a), total cholesterol, homocysteine, etc.), the exact mechanism of blood flow preservation through the IRA remains unclear (6–10).
Earlier, we demonstrated that the functional state of endothelium as assessed with flow-mediated dilation test (FMD test) correlates with the patency of IRA (11). The higher activity of endogenous fibrinolysis, which is closely interconnected with the functional state of endothelium, is the most probable cause of SR (12–15). Also, several small studies demonstrated higher platelet reactivity in patients without SR of the IRA (16, 17).
The existing data show the general dependence of the occlusion of the IRA and long-term prognosis on the inflammatory status of AMI-patients. While various cytokines play an important role in inflammation, it is mostly evaluated from measurement of high sensitive C-reactive protein (hs-CRP) together with leukocytes counts. Little is known about the possible role of cytokines in the state of blood flow through the IRA (18).
Here, we attempted to fill in this gap. The aim of the current study was to investigate the links between the functional state of the endothelium, the cytokine spectrum, and critical parameters of thrombus formation in AMI-patients with differences in coronary blood flow through the IRA.
The study was performed at the Moscow City Clinical Hospital named after I.V. Davydovsky between January 2017 and October 2021. The study protocol was developed in accordance with the principles of the Helsinki Declaration and was approved by the Interuniversity Committee of Ethics. All participants signed an informed written consent.
We included 75 AMI patients in our study: 58 presented with STEMI, while 17 presented with non-STEMI. All patients underwent selective coronary angiography with subsequent primary percutaneous coronary intervention (PCI) (STEMI-patients directly upon admission, non-STEMI patients during the first 24 hours of hospitalization). Patency of the IRA was determined on TIMI Grade flow classification (Appleby et al., 2000). Of the patients, 45 had an impaired blood flow through the IRA (TIMI 0-1) and 30 were characterized by preserved blood flow (TIMI 2-3).
Inclusion criteria: STEMI or non-STEMI patients; onset of symptoms during the 24 hours before admission; informed written consent.
Exclusion criteria: Age over 90 or under 18; more than 24 hours from the onset of symptoms, thrombolytic therapy, signs of cardiogenic shock, acute and chronic infectious diseases and inflammatory processes, severe anemia or ongoing bleeding, pregnancy, known oncological process, anticoagulant therapy.
In addition to the standard clinical examination, on admission, all patients underwent: a flow-mediated dilation test (FMD test) to evaluate endothelial function, thrombodynamics and rotational thromboelastometry (in NATEM mode) to evaluate plasma coagulation, thrombodynamics in fibrinolysis mode to evaluate endogenous fibrinolysis, and xMAP technology to measure concentration of 40 cytokines.
A 21-gauge needle was used with minimal stasis in order to avoid iatrogenic induction of platelet aggregation, and peripheral venous blood was drawn in the amount of 4.5 ml into a tube containing 0.105 M buffered sodium citrate anticoagulant. The whole blood was used for rotational thromboelastometry. Platelet-poor plasma was used for the thrombodynamics study. Blood was centrifuged at 1,600 g for 15 min to obtain platelet-poor plasma. Subsequently, the platelet-poor plasma was transferred to a new centrifugation tube and centrifuged at 10,000 g for 15 min to obtain platelet-free plasma.
We carried out the study using ROTEM (Roche). The study was performed in NATEM mode. The following indicators were used: clotting time (CT, sec) and thrombus amplitude at different time sections of the study (A10, A20, mm).
The study was performed on a T-2 Thrombodynamics Analyzer according to a standard technique (19). The following parameters of clot growth were used: clot growth rate (V, μm/min), initial clot growth rate (Vi, μm/min), and spontaneous clot formation time (Tsp, min). A standard activator with urokinase was added to induce thrombus lysis. The lysis was characterized by the time of lysis onset (LOT, min), clot lysis time (CLT, min), and the rate of lysis progression (LP, %/min).
To assess endothelial function, all patients underwent an FMD test according to the standard method (20). The results of FMD-test were expressed in %.
40 cytokines in serum were measured with a commercial kit, MILLIPLEX MAP Human Cytokine/Chemokine Magnetic Bead Panel (Merсk Millipore). The cytokine panel included interleukin-1α (IL-1α), IL-1β, IL-1RA (IL-1 receptor antagonist), IL-2, IL-3, IL-4, IL-5, IL-6, IL-7, IL-8, IL-9, IL-10, IL-12 (p40), IL-12 (p70), IL-13, IL-15, IL-17A, fractalkine (CX3CL1), growth-regulated alpha (GRO-α or CXCL1), interferon-γ-induced protein-10 (IP-10 or CXCL10), monocyte chemoattractant protein-1 (MCP-1 or CCL2), MCP-3 (CCL7), macrophage inflammatory protein-1α (MIP-1α or CCL3), MIP-1β (CCL4), eotaxin (CCL11), macrophage-derived chemokine (MDC or CCL22), soluble CD40-ligand (sCD40L), epidermal growth factor (EGF), fibroblast growth factor-2 (FGF-2), Fms-like tyrosine kinase 3 ligand (Flt-3L), vascular endothelial growth factor (VEGF), granulocyte colony-stimulating factor (G-CSF), granulocyte-macrophage colony-stimulating factor (GM-CSF), platelet-derived growth factor-AA (PDGF-AA), PDGF-AB/BB, transforming growth factor-α (TGF-α), interferon-α2 (IFN-α2), IFN-γ, tumor necrosis factor-α (TNF-α), and TNF-β. All cytokines were measured in pg/ml. Serum was diluted 4 times in assay buffer to reduce the matrix effect and added in a volume of 50 µl to each well. The standard curve was built up from 8 standard dilutions in triplicates, with the 1st-3rd standard dilutions with dilution factor 5 and the and 4th-8th dilutions with dilution factor 4. We used serum matrix diluted in assay buffer to mimic the matrix effect on the standard curve, controls, and blank wells. Standards and controls (25 µl) were diluted with 25 µl of serum matrix. We added 15 µl of 40-plex magnetic beads to each well and incubated for 18 h at 4°C and then for 30 min at 25°C. Beads were washed twice with automatic magnetic washer (Biotech ELx405) and incubated with detection antibodies for 1 h at 25°C. Antibodies were diluted with wash buffer in 1,93 times and added in the amount of 25 µl per well. After incubation, we added 15 µl of Streptavidin-PE solution to each well and incubated the final solution for 30 min at 25°C. Then, beads were washed twice, resuspended in the sheath fluid, and analyzed by means of the Luminex 200 system. For the analysis we collected 50 beads per region. Wells with fewer than 20 beads per region were excluded from analysis. During the analysis, we used 5PL fit for the standard curve.
During explanatory analysis we considered 26 from 40 initially measured cytokines in 40% of cases to be under the detection limit, and we decided to exclude them from analysis. The following cytokines were available for the final analysis: EGF, Eotaxin, GRO-α, IL-10, IL-8, IP-10, MCP-1, MDC, MIP-1β, PDGF-AA, PDGF-AB/BB, sCD40L, TGF-α, and TNF-α.
Statistical analysis was performed with R (4.0.5). The expression values obtained in the present study were in most cases not normally distributed, according to the Shapiro-Wilk test. For comparison of several groups, we used the Mann-Whitney rank test with continuity correction. For the analysis of categorical parameters, we used a two-tailed Fisher’s exact test with 2x2 frequency tables. In order to overcome errors from multiple comparisons we performed a Benjamini-Hochberg FDR-correction with calculation of critical values for each comparison matched with corresponding p-values; we calculated adjusted p-values and compared them with a critical value of 0.05, if not stated otherwise. For calculation of Spearman’s coefficient, we used a minimum 9 pairs of observations and a threshold of p.adjusted < 0.2 to keep the positive false discovery rate below 20%. 26% of the data was missing at random values in different parameters that did not result in sample bias by definition, so the pairwise deletion approach was used. The available data sets are sufficient to demonstrate significant differences in the cytokine levels using Mann-Whitney rank test, sig. level = 0.05, power = 0.8, and effect size = 0.8 which corresponds to ‘large’ effect size (21).
Compared with STEMI-patients, patients with non-STEMI were characterized by a significantly more frequent history of myocardial infarction (40% vs. 2.4%) and PCI (44% vs. 2.5%), and more often presented with multivessel disease (66.7% vs. 24.5%) (Table 1).
Table 1 Comparison of clinical data of patients in different groups, two types of stratification (STEMI vs. Non-STEMI; TIMI 0-1 vs. TIMI 2-3).
There was no significant difference in FMD-test values between STEMI and non-STEMI patients.
Non-STEMI patients had higher levels of MDC (779.13 [654.5; 1070.7]) vs. 513.63 [426.7; 807.95], p.adj < 0.05 in STEMI), MIP-1β (41.1 [34.0; 53.4]) vs. 18.1 [13.3; 34.1], p.adj < 0.05 in STEMI), TNF-α (10.2 [7.2; 14.8]) vs. 3.84 [2.9; 6.77], p.adj < 0.05 in STEMI) compared with STEMI-patients (Figure 1).
Figure 1 Differences in cytokine levels between STEMI and non-STEMI patients. Non-STEMI patients had elevated levels of MDC, MIP-1β, TNF-α. Asterix indicates p-adjusted < 0.05. ns, not significant.
There were no differences between STEMI and non-STEMI patients in parameters of clot formation and endogenous fibrinolysis. We observed a positive moderate strength correlation between TNF-α with LOT (r=0.4, p < 0.05, p.adj < 0.2) and CLT (r=0.5, p < 0.05, p.adj < 0.2).
Patients with preserved blood flow through the IRA had a history of myocardial infarction more frequently (29.1% vs. 0% in TIMI 0-1) (Table 1).
Patients with TIMI 2-3 blood flow were characterized by higher values of FMD test results (5.71 [4.3; 9.1] vs. 4.28 [2.5; 5.7] in TIMI 0-1, p.adj < 0.05).
Patients with TIMI 2-3 blood flow were characterized by a higher level of IP-10 (133.58 [103.7; 184] vs. 97.8 [70.7; 118.3] in TIMI 0-1, p < 0.05, p.adj < 0.2) while patients with TIMI 0-1 blood flow had higher levels of IL-10 (4.5 [2.3; 14.1] vs. 1.3 [0.9; 5.3] in TIMI 2-3, p< 0.05, p.adj < 0.2) (Figure 2A). Hs-CRP levels were higher in patients with TIMI 0-1 blood flow (5.4 [2.8; 18.2] vs. 0.1 [0.1; 0.95] in TIMI 2-3, p < 0.05). We analyzed the predictive value of studied cytokines and found IP-10 to be the most effective in separating between subject groups with TIMI 0-1 and 2-3, with ROC AUC = 0.72, and optimal sensitivity/specificity at 0.64/0.30, respectively (Figure S1).
Figure 2 Comparison of TIMI 0-1 and TIMI 2-3 groups. Differences in cytokine concentrations (A), clot formation and endogenous fibrinolysis (B). Asterix indicates p-adjusted < 0.05, ns, not significant.
Patients with TIMI 0-1 blood flow had higher average and initial clot growth rates (36.6 [31.9; 44.8] vs. 30.4 [27.9; 35.7] in TIMI 2-3, and 64.05 [59.8; 67.8] vs. 58.6 [54.6; 61.3] in TIMI 2-3, p.adj < 0.05) and earlier onset of spontaneous clots (31.8 [21.6; 44.4] vs. 63.6 [48; 85.4] in TIMI 2-3, p.adj < 0.05) (Figure 2B).
We observed significant positive correlations between IL-10 with the levels of hs-CRP and pro-inflammatory chemokines such as IL-8, GRO-α, MIP-1β, MCP-1, as well as with the Vi and CLT in TIMI 0-1 patients (Figure 3).
Figure 3 Correlation matrix of circulating markers of inflammation, clot formation and endogenous fibrinolysis and FMD. Included are 14 cytokines, hs-CRP, FMD and clot formation and endogenous fibrinolysis parameters in groups of patients with TIMI 0-1 and TIMI 2-3 blood flow, p < 0.05, p.adj < 0.2.
We performed an additional analysis of the influence of diabetes mellitus on the observed correlations by exclusion these patients from the cohort. The correlations described above were mostly preserved but tended to have lower correlation coefficients (Figure S2).
It is firmly established that chronic inflammation is the driving force of atherosclerosis (2, 22). There is a large body of evidence concerning the active involvement of leukocytes in the formation of atherosclerotic plaque (23, 24). Also, leukocytes promote proliferation of smooth muscle cells and their phenotypic switch, facilitating further plaque growth (25). The immune system plays a key role in the destabilization of atherosclerotic plaque, the most common cause of AMI, primarily through the massive release of matrix metalloproteinases by macrophages and impaired collagen synthesis (26–28). All the above processes are finely regulated by inflammatory and anti-inflammatory cytokines.
The level of systemic inflammation and prothrombotic state correlates with the severity of AMI (29). The positive effect of inhibition of systemic inflammation with statins and PCSK9 inhibitors both on reducing markers of systemic inflammation (CRP, etc.) and on major adverse cardiovascular events has been previously demonstrated in a number of clinical studies (30–34). Also, in a randomized double-blind clinical trial, CANTOS, a significant reduction in the number of adverse cardiovascular diseases was demonstrated under the influence of the specific IL-1β blocker, canakinumab (35). Considering this, the study of the effect of various cytokines both on the course of AMI and on the state of blood flow in the IRA facilitate understanding the mechanisms of relation of inflammation to AMI.
In pursuing this aim, we choose the panel of cytokines that are known to be associated with inflammation and haemostasis (36–40). Several cytokines in our panel such as IL-10, TNF-α, MCP-1, GRO-α, IP-10 and IL-8 were shown to be associated with the course and with the outcome of AMI (41–43). IL-10, TGF-α, IP-10, MIP-1β, IL-8, and Eotaxin were found to be predictors of AMI in the study by Hoogeveen et al. (44). Furthermore, as the goal of our study was also to investigate the influence of immune mechanisms on the prothrombotic state in AMI, we included in our study several cytokines that may not be directly connected to thrombosis but can be involved in the network and reflect the status of different cell types during AMI.
In the first part of our observational study we evaluated the levels of 14 circulatory cytokines in patients with STEMI and non-STEMI with differences in blood flow through the coronary artery and found significant correlations between these parameters. Non-STEMI patients in our study, as well as in the published data (45), were characterized by a longer history of coronary artery disease, repeated revascularization, and of multivascular involvement. In our study patients with non-STEMI demonstrated significantly higher levels of TNF-α, MDC and MIP-1β compared with STEMI patients.
These cytokines were reported to be directly connected to atherosclerosis development and progression. In particular, TNF-α is a pleiotropic cytokine that is involved in atherothrombosis in several pathways: TNF-α increases the expression of adhesion molecules by endothelium and as well enhances leukocyte adhesion and rolling (46), and it is involved in the implementation of endothelial dysfunction via increase of reactive oxygen species production and impairment of NO-mediated vasodilation (47–50). Finally, there is evidence of increased platelet aggregation upon TNF-α stimulation (51–54). MIP-1β was shown to be released by macrophages and smooth muscle cells in atherosclerotic plaques. Atherosclerotic patients had elevated levels of this circulating chemokine (55–57). It has been shown that TNF-α induces MIP-1β expression in THP-1 cells (58). Therefore, a possible mechanism of synergistic action of TNF-α and MIP-1β in AMI patients can be TNF-α-mediated induction of MIP-1β expression in monocytes and increased adhesion of leukocytes to endothelium pre-activated with TNF-α. MDC is predominantly secreted by macrophages and dendritic cells and is involved in migration of monocytes. MDC expression has been demonstrated in human atherosclerotic plaques and associated with M2 macrophages (59). One of the possible actions of MDC in cardiovascular diseases can be mediated by platelet activation as measured by platelet aggregation and calcium flux (60).
The course of AMI and its long-term prognosis depend on the baseline blood flow through the IRA. TIMI flow grade is commonly used to classify blood flow through the IRA (61). In the second part of our study, we investigated the link between cytokine spectrum, haemostasis, endothelium state and blood flow in AMI patients. We used the division of patients into a group with TIMI 0-1 blood flow (which indicates an almost complete absence of distal blood flow through the IRA) and a group with TIMI 2-3 blood flow, characterized by partially or fully preserved blood flow.
First, in these groups we analyzed blood clotting and endogenous fibrinolysis due to IRA blood flow dependence on clot formation and stability. Patients with impaired blood flow (TIMI 0-1) were characterized by more intensive clot formation compared with patients with preserved blood flow (TIMI 2-3). Our results are in agreement with the data on enhanced blood clotting in patients with impaired blood flow through the IRA (16, 17).
Functional state of endothelium and circulating cytokines have a direct influence on parameters of haemostasis. Earlier, it was shown that the FMD test can be a predictive factor of IRA patency and long-term prognosis in patients with AMI (11). In the present study we confirmed these results.
Furthermore, we compared levels of circulating cytokines in TIMI 0-1 and TIMI 2-3 groups of patients and analyzed their correlations with other markers of inflammation, specifically with parameters of coagulation and fibrinolysis. We found that the TIMI 0-1 and TIMI 2-3 groups had different patterns of correlations and most of the correlations were detected in the TIMI 0-1 group, probably because of massive intracoronary thrombosis in patients in this group.
In our study patients with impaired coronary blood flow (TIMI 0-1) had lower levels of IP-10 and higher levels of IL-10, compared with TIMI 2-3 patients. Moreover, IP-10 was found to be the most effective among other analyzed cytokines in separating subject groups with TIMI 0-1 and 2-3 blood flow. The predictive value and clinical implication of this result should be investigated in further prospective studies.
These results can be explained in the context of these cytokine functions. IP-10 is a chemokine belonging to the CXC chemokine family. It is secreted by cells of many types including smooth muscle cells, endothelial cells, and macrophages in atherosclerotic plaques; it acts as a chemoattractant predominantly for lymphocytes (62, 63), and can promote proliferation and migration of smooth muscle cells (38). Several studies have demonstrated upregulation of IP-10 during AMI, and it’s reverse correlation with the infarct size (64, 65). In our study, we have shown that IP-10 is elevated in patients with AMI with TIMI 2-3 coronary blood flow, compared with TIMI 0-1 patients. The patency of IRA can be associated with elevated levels of IP-10 and thus, with smaller infarct size in this group. IL-10 is an anti-inflammatory cytokine that can be released by various immune cells in response to pro-inflammatory cytokines and inhibits their action (66). The protective properties of this cytokine in relation to the development of atherosclerosis and the size of the zone of myocardial infarction were shown in several previous studies (67–70).
In our study, elevated levels of IL-10 can be a response to enhanced tissue injury and inflammation in TIMI 0-1 patients. This is confirmed by IL-10 correlation with the levels of hs-CRP and pro-inflammatory chemokines such as IL-8, GRO-α, MIP-1β, and MCP-1 in TIMI 0-1 patients (71). Elevated IL-10 levels can be not only a consequence of impaired coronary blood flow in TIMI 0-1 patients but can also be connected to the coagulation cascade. Despite the fact that several studies demonstrated an inhibitory effect of IL-10 on coagulation, we found a positive correlation of IL-10 with the initial clot growth rate (Vi) in patients with TIMI 0-1 (37). This correlation can be related to the association of coagulation with the pro-inflammatory response, where IL-10 can be induced by acute inflammation and be a bystander to increased coagulation. This seems to be confirmed by the correlation of IL-10 with pro-inflammatory chemokines such as GRO-α, IL-8, and MCP -1 and also by correlation of these chemokines with Vi (36). On the other hand, IL-10 can be involved in stabilization of the thrombus. Previously, it was shown that during endotoxemia, IL-10 inhibits not only coagulation, but also fibrinolysis (37). Alshehri et al. (72) showed that FXIII-A antigen was up-regulated in human monocytes in response to stimulation by IL-10, and treatment of monocytes with IL-10 stabilized FXIII-depleted thrombi from fibrinolytic degradation. On the basis of these facts taken together, it can be proposed that in AMI IL-10 not only inhibits pro-inflammatory stimuli leading to resolution of inflammation, but also stabilizes thrombus. In our study, this is evidenced by positive correlation of IL-10 with clot lysis time (CLT) in TIMI 0-1 patients. Due to the possible influence of metabolic disorders on the inflammatory status in AMI-patients, we performed an additional analysis excluding patients with diabetes mellitus. The most important correlations were preserved, but as expected, tended to have a lower correlation coefficients, which could be explained not only by the influence of diabetes mellitus, but by the smaller group size as well.
Thus, our findings demonstrated that the circulating cytokines may play an important role in the course of AMI by influencing endothelial function, clot formation, and fibrinolysis. Further research will determine the possible impact of cytokine spectrum on AMI treatment strategy.
The main limitation of our study was the relatively small number of patients included and available values, which led to the necessity of raising the threshold of adjusted p-value in some cases to reach the significant differences. Because 26 of the 40 initially measured cytokines in 40% of cases were under the detection limit, they were excluded from the final analysis. Nevertheless, these cytokines may also play an important role in AMI and should be analyzed in a larger cohort of patients.
We demonstrated that inflammatory cytokines correlate not only with the form of myocardial infarction (STEMI or non-STEMI), but also with the blood flow through the infarct-related artery. Inflammatory response, functional state of endothelium, and clot formation are closely linked with each other. A combination of these parameters affects the patency of the infarct-related artery.
The raw data supporting the conclusions of this article will be made available by the authors, without undue reservation.
The studies involving human participants were reviewed and approved by Davydovsky Moscow City Clinical Hospital local ethics committee. The patients/participants provided their written informed consent to participate in this study.
AK, OD, AL, DV, EM, LM, EV designed the study, contributed to the literature search, data collection, data analysis, data interpretation and writing of the manuscript. GR contributed to data analysis, data interpretation and writing of the manuscript. AS, LM, EV critically reviewed the manuscript. All authors contributed to the article and approved the submitted version.
The reported study was funded by RFBR, project number 20-315-70047. The work of LM was supported by the NICHD Intramural Program.
The authors declare that the research was conducted in the absence of any commercial or financial relationships that could be construed as a potential conflict of interest.
All claims expressed in this article are solely those of the authors and do not necessarily represent those of their affiliated organizations, or those of the publisher, the editors and the reviewers. Any product that may be evaluated in this article, or claim that may be made by its manufacturer, is not guaranteed or endorsed by the publisher.
We thank Dr. Barry Alpher for assistance in editing and improving the English style.
The Supplementary Material for this article can be found online at: https://www.frontiersin.org/articles/10.3389/fimmu.2022.837642/full#supplementary-material
1. Crea F, Libby P. Acute Coronary Syndromes: The Way Forward From Mechanisms to Precision Treatment. Circulation (2017) 136:1155–66. doi: 10.1161/CIRCULATIONAHA.117.029870
2. Libby P, Buring JE, Badimon L, Hansson GK, Deanfield J, Bittencourt MS, et al. Atherosclerosis. Nat Rev Dis Prim (2019) 5:1–18. doi: 10.1038/s41572-019-0106-z
3. Thygesen K, Alpert JS, Jaffe AS, Chaitman BR, Bax JJ, Morrow DA, et al. Executive Group on Behalf of the Joint European Society of Cardiology (ESC)/American College of Cardiology (ACC)/American Heart Association (AHA)/World Heart Federation (WHF) Task Force for the Universal Definition of Myocardial Infarction. Fourth Universal Definition of Myocardial Infarction (2018). Circulation (2018) 138:e618–51. doi: 10.1161/CIR.0000000000000617
4. Badings EA, Remkes WS, The SHK, Dambrink JHE, Tjeerdsma G, Rasoul S, et al. Early or Late Intervention in Patients With Transient ST-Segment Elevation Acute Coronary Syndrome: Subgroup Analysis of the ELISA-3 Trial. Catheter Cardiovasc Interv (2016) 88:755–64. doi: 10.1002/ccd.26719
5. Karwowski J, Poloński L, Gierlotka M, Ciszewski A, Hawranek M, Bćkowski M, et al. Total Coronary Occlusion of Infarct-Related Arteries in Patients With non-ST-Elevation Myocardial Infarction Undergoing Percutaneous Coronary Revascularisation. Kardiol Pol (Polish Hear Journal) (2017) 75:108–16. doi: 10.5603/KP.A2016.0130
6. Dagdelen S, Eren N, Akdemir I, Karabulut H, Ergelen M, Caglar N. The Effect of Lipoprotein-a on Thrombolytic Therapy and Spontaneous Reperfusion in Acute Myocardial Infarction. A Comparative Study. Int Angiol (2002) 21:384–9.
7. Lundergan CF, Reiner JS, McCarthy WF, Coyne KS, Califf RM, Ross AM. Clinical Predictors of Early Infarct-Related Artery Patency Following Thrombolytic Therapy: Importance of Body Weight, Smoking History, Infarct- Related Artery and Choice of Thrombolytic Regimen: The GUSTO-I Experience. J Am Coll Cardiol (1998) 32:641–7. doi: 10.1016/S0735-1097(98)00278-2
8. Moliterno DJ, Lange RA, Meidell RS, Willard JE, Leffert CC, Gerard RD, et al. Relation of Plasma Lipoprotein(a) to Infarct Artery Patency in Survivors of Myocardial Infarction. Circulation (1993) 88:935–40. doi: 10.1161/01.CIR.88.3.935
9. Wang J, He S. Clinical and Angiographic Characteristics of Patients With Spontaneous Reperfusion in ST-Segment Elevation Myocardial Infarction. Med (Baltimore) (2020) 99:e19267. doi: 10.1097/MD.0000000000019267
10. Li J, Zhou Y, Zhang Y, Zheng J. Admission Homocysteine is an Independent Predictor of Spontaneous Reperfusion and Early Infarct-Related Artery Patency Before Primary Percutaneous Coronary Intervention in ST-Segment Elevation Myocardial Infarction. BMC Cardiovasc Disord (2018) 18:1–7. doi: 10.1186/s12872-018-0868-3
11. Vasilieva E, Urazovskaya I, Skrypnik D, Shpektor A. Total Occlusion of the Infarct-Related Coronary Artery Correlates With Brachial Artery Flow-Mediated Dilation in Patients With ST-Elevation Myocardial Infarction. Acute Card Care (2009) 11:155–9. doi: 10.1080/17482940902763372
12. Farag M, Spinthakis N, Gue YX, Srinivasan M, Sullivan K, Wellsted D, et al. Impaired Endogenous Fibrinolysis in ST-Segment Elevation Myocardial Infarction Patients Undergoing Primary Percutaneous Coronary Intervention Is a Predictor of Recurrent Cardiovascular Events: The RISK PPCI Study. Eur Heart J (2019) 40:295–305. doi: 10.1093/eurheartj/ehy656
13. Kalinskaya AI, Savvinova PP, Vasilieva EY, Shpektor AV. The Specifics of Clotting and Endogenic Fibrinolysis in Acute Coronary Syndrome Patients. Russ J Cardiol (2018) 23:12–6. doi: 10.15829/1560-4071-2018-9-12-16
14. Stone GW, Cox D, Garcia E, Brodie BR, Morice MC, Griffin J, et al. Normal Flow (TIMI-3) Before Mechanical Reperfusion Therapy is an Independent Determinant of Survival in Acute Myocardial Infarction: Analysis From the Primary Angioplasty in Myocardial Infarction Trials. Circulation (2001) 104:636–41. doi: 10.1161/hc3101.093701
15. Sumaya W, Wallentin L, James SK, Siegbahn A, Gabrysch K, Bertilsson M, et al. Fibrin Clot Properties Independently Predict Adverse Clinical Outcome Following Acute Coronary Syndrome: A PLATO Substudy. Eur Heart J (2018) 39:1078–85. doi: 10.1093/eurheartj/ehy013
16. Breet NJ, van Werkum JW, Bouman HJ, Kelder JC, Hackeng CM, ten Berg JM. The Relationship Between Platelet Reactivity and Infarct-Related Artery Patency in Patients Presenting With a St-Elevation Myocardial Infarction. Thromb Haemost (2011) 106:331–6. doi: 10.1160/TH10-08-0528
17. Skoric B, Milicic D, Lovric D, Gornik I, Skoric KN, Sertic J. Initial Patency of the Infarct-Related Artery in Patients With Acute ST Elevation Myocardial Infarction is Related to Platelet Response to Aspirin. Int J Cardiol (2010) 140:356–8. doi: 10.1016/j.ijcard.2008.11.031
18. Li X, Lin S, Chen X, Huang W, Li Q, Zhang H, et al. The Prognostic Value of Serum Cytokines in Patients With Acute Ischemic Stroke. Aging Dis (2019) 10:544–56. doi: 10.14336/AD.2018.0820
19. Balandina AN, Serebriyskiy II, Poletaev AV, Polokhov DM, Gracheva MA, Koltsova EM, et al. Thrombodynamics—A New Global Hemostasis Assay for Heparin Monitoring in Patients Under the Anticoagulant Treatment. PloS One (2018) 13:e0199900. doi: 10.1371/journal.pone.0199900
20. Mallat Z, Besnard S, Duriez M, Deleuze V, Emmanuel F, Bureau MF, et al. Protective Role of Interleukin-10 in Atherosclerosis. Circ Res (Circ Res) 85:1–8. doi: 10.1161/01.RES.85.8.E17
21. Cohen J. Statistical Power Analysis for the Behavioral Sciences. New York:Routledge (2013). doi: 10.4324/9780203771587
22. Libby P. Inflammation in Atherosclerosis. Arterioscler Thromb Vasc Biol (2012) 32:2045–51. doi: 10.1161/ATVBAHA.108.179705
23. Jones DP, True HD, Patel J. Leukocyte Trafficking in Cardiovascular Disease: Insights From Experimental Models. Mediators Inflamm (2017) 2017:1–9. doi: 10.1155/2017/9746169
24. Schrottmaier WC, Mussbacher M, Salzmann M, Assinger A. Platelet-Leukocyte Interplay During Vascular Disease. Atherosclerosis (2020) 307:109–20. doi: 10.1016/J.ATHEROSCLEROSIS.2020.04.018
25. Sorokin V, Vickneson K, Kofidis T, Woo CC, Lin XY, Foo R, et al. Role of Vascular Smooth Muscle Cell Plasticity and Interactions in Vessel Wall Inflammation. Front Immunol (2020) 11:599415/BIBTEX. doi: 10.3389/FIMMU.2020.599415/BIBTEX
26. Wang X, Khalil RA. Matrix Metalloproteinases, Vascular Remodeling, and Vascular Disease. Adv Pharmacol (2018) 81:241. doi: 10.1016/BS.APHA.2017.08.002
27. Fingleton B. Matrix Metalloproteinases as Regulators of Inflammatory Processes. Biochim Biophys Acta - Mol Cell Res (2017) 1864:2036–42. doi: 10.1016/J.BBAMCR.2017.05.010
28. Soehnlein O, Libby P. Targeting Inflammation in Atherosclerosis — From Experimental Insights to the Clinic. Nat Rev Drug Discov (2021) 20:589–610. doi: 10.1038/s41573-021-00198-1
29. Fang L, Moore XL, Dart AM, Wang LM. Systemic Inflammatory Response Following Acute Myocardial Infarction. J Geriatr Cardiol (2015) 12:305–12. doi: 10.11909/J.ISSN.1671-5411.2015.03.020
30. Goldberg RB, Mellies MJ, Sacks FM, Moyé LA, Howard BV, Howard WJ, et al. Cardiovascular Events and Their Reduction With Pravastatin in Diabetic and Glucose-Intolerant Myocardial Infarction Survivors With Average Cholesterol Levels: Subgroup Analyses in the Cholesterol And Recurrent Events (CARE) Trial. Circulation (1998) 98:2513–9. doi: 10.1161/01.CIR.98.23.2513
31. Mora S, Glynn RJ, Hsia J, MacFadyen JG, Genest J, Ridker PM. Statins for the Primary Prevention of Cardiovascular Events in Women With Elevated High-Sensitivity C-Reactive Protein or Dyslipidemia: Results From the Justification for the Use of Statins in Prevention: An Intervention Trial Evaluating Rosuvastatin (JUP. Circulation (2010) 121:1069–77. doi: 10.1161/CIRCULATIONAHA.109.906479
32. Cannon CP, Braunwald E, McCabe CH, Rader DJ, Rouleau JL, Belder R, et al. Intensive Versus Moderate Lipid Lowering With Statins After Acute Coronary Syndromes. N Engl J Med (2004) 350:1495–504. doi: 10.1056/nejmoa040583
33. Sabatine MS, Giugliano RP, Keech AC, Honarpour N, Wiviott SD, Murphy SA, et al. Evolocumab and Clinical Outcomes in Patients With Cardiovascular Disease. N Engl J Med (2017) 376:1713–22. doi: 10.1056/nejmoa1615664
34. Schwartz GG, Steg PG, Szarek M, Bhatt DL, Bittner VA, Diaz R, et al. Alirocumab and Cardiovascular Outcomes After Acute Coronary Syndrome. N Engl J Med (2018) 379:2097–107. doi: 10.1056/nejmoa1801174
35. Ridker PM, Everett BM, Thuren T, MacFadyen JG, Chang WH, Ballantyne C, et al. Antiinflammatory Therapy With Canakinumab for Atherosclerotic Disease. N Engl J Med (2017) 377:1119–31. doi: 10.1056/NEJMoa1707914
36. Osnes LTN, Westvik ÅB, Joø GB, Okkenhaug C, Kierulf P. Inhibition of IL-1 Induced Tissue Factor (TF) Synthesis and Procoagulant Activity (PCA) in Purified Human Monocytes by IL-4, IL-10 and IL-13. Cytokine (1996) 8:822–7. doi: 10.1006/CYTO.1996.0110
37. Pajkrt D, van der Poll T, Levi M, Cutler DL, Affrime MB, Van Den Ende A, et al. Interleukin-10 Inhibits Activation of Coagulation and Fibrinolysis During Human Endotoxemia. Blood (1997) 89:2701–5. doi: 10.1182/BLOOD.V89.8.2701
38. Wang X, Yue TL, Ohlstein EH, Sung CP, Feuerstein GZ. Interferon-Inducible Protein-10 Involves Vascular Smooth Muscle Cell Migration, Proliferation, and Inflammatory Response. J Biol Chem (1996) 271:24286–93. doi: 10.1074/jbc.271.39.24286
39. Esmon CT. Possible Involvement of Cytokines in Diffuse Intravascular Coagulation and Thrombosis. Bailliere’s Best Pract Res Clin Haematol (1999) 12:343–59. doi: 10.1053/beha.1999.0029
40. Christiansen SC, Næss IA, Cannegieter SC, Hammerstrøm J, Rosendaal FR, Reitsma PH. Inflammatory Cytokines as Risk Factors for a First Venous Thrombosis: A Prospective Population-Based Study. PloS Med (2006) 3:1414–9. doi: 10.1371/journal.pmed.0030334
41. Ong SB, Hernández-Reséndiz S, Crespo-Avilan GE, Mukhametshina RT, Kwek XY, Cabrera-Fuentes HA, et al. Inflammation Following Acute Myocardial Infarction: Multiple Players, Dynamic Roles, and Novel Therapeutic Opportunities. Pharmacol Ther (2018) 186:73–87. doi: 10.1016/j.pharmthera.2018.01.001
42. Hartman MHT, Groot HE, Leach IM, Karper JC, van der Harst P. Translational Overview of Cytokine Inhibition in Acute Myocardial Infarction and Chronic Heart Failure. Trends Cardiovasc Med (2018) 28:369–79. doi: 10.1016/J.TCM.2018.02.003
43. Sopova K, Park C, Al-Atta A, Bennaceur K, Mohammad A, Vlachogiannis N, et al. Interferon-Gamma Inducible Protein IP-10 and Left Ventricular Remodelling Post-Acute Myocardial Infarction: A Longitudinal Cardiovascular Magnetic Resonance Imaging Substudy of CAPRI Clinical Trial. Eur Heart J (2020) 41:1302. doi: 10.1093/ehjci/ehaa946.1302
44. Hoogeveen RM, Pereira JPB, Nurmohamed NS, Zampoleri V, Bom MJ, Baragetti A, et al. Improved Cardiovascular Risk Prediction Using Targeted Plasma Proteomics in Primary Prevention. Eur Heart J (2020) 41:3998–4007. doi: 10.1093/EURHEARTJ/EHAA648
45. Cohen M. Long-Term Outcomes in High-Risk Patients With Non-ST-Segment Elevation Myocardial Infarction. J Thromb Thromb (2016) 41:464–74. doi: 10.1007/s11239-015-1227-1
46. Chandrasekharan UM, Siemionow M, Unsal M, Yang L, Poptic E, Bohn J, et al. Tumor Necrosis Factor α (TNF-α) Receptor-II is Required for TNF-α–Induced Leukocyte-Endothelial Interaction In Vivo. Blood (2007) 109:1938–44. doi: 10.1182/BLOOD-2006-05-020875
47. Rolski F, Błyszczuk P. Complexity of TNF-α Signaling in Heart Disease. J Clin Med (2020) 9:3267. doi: 10.3390/JCM9103267
48. Zhang H, Park Y, Wu J, Chen XP, Lee S, Yang J, et al. Role of TNF-Alpha in Vascular Dysfunction. Clin Sci (Lond) (2009) 116:219–30. doi: 10.1042/CS20080196
49. Gao X, Belmadani S, Picchi A, Xu X, Potter BJ, Tewari-Singh N, et al. Tumor Necrosis Factor-α Induces Endothelial Dysfunction in Lepr Db Mice. Circulation (2007) 115:245–54. doi: 10.1161/CIRCULATIONAHA.106.650671
50. Aoki N, Siegfried M, Lefer AM. Anti-EDRF Effect of Tumor Necrosis Factor in Isolated, Perfused Cat Carotid Arteries. Am J Physiol - Hear Circ Physiol (1989) 256:1–4. doi: 10.1152/AJPHEART.1989.256.5.H1509
51. Pignatelli P, De Biase L, Lenti L, Tocci G, Brunelli A, Cangemi R, et al. Tumor Necrosis Factor-α as Trigger of Platelet Activation in Patients With Heart Failure. Blood (2005) 106:1992–4. doi: 10.1182/BLOOD-2005-03-1247
52. Page MJ, Bester J, Pretorius E. The Inflammatory Effects of TNF-α and Complement Component 3 on Coagulation. Sci Rep (2018) 8:1–9. doi: 10.1038/s41598-018-20220-8
53. Davizon-Castillo P, McMahon B, Aguila S, Bark D, Ashworth K, Allawzi A, et al. TNF-α–Driven Inflammation and Mitochondrial Dysfunction Define the Platelet Hyperreactivity of Aging. Blood (2019) 134:727–40. doi: 10.1182/BLOOD.2019000200
54. Qian H, Chen R, Wang B, Yuan X, Chen S, Liu Y, et al. Associations of Platelet Count With Inflammation and Response to Anti-TNF-α Therapy in Patients With Ankylosing Spondylitis. Front Pharmacol (2020) 11:559593/BIBTEX. doi: 10.3389/FPHAR.2020.559593/BIBTEX
55. Vistnes M. Macrophage Inflammatory Protein-1β: A Novel Prognostic Biomarker in Atherosclerosis? Cardiology (2012) 121:149–51. doi: 10.1159/000336484
56. Schecter AD, Calderon TM, Berman AB, McManus CM, Fallon JT, Rossikhina M, et al. Human Vascular Smooth Muscle Cells Possess Functional CCR5. J Biol Chem (2000) 275:5466–71. doi: 10.1074/jbc.275.8.5466
57. Cagnin S, Biscuola M, Patuzzo C, Trabetti E, Pasquali A, Laveder P, et al. Reconstruction and Functional Analysis of Altered Molecular Pathways in Human Atherosclerotic Arteries. BMC Genomics (2009) 10:1–15. doi: 10.1186/1471-2164-10-13
58. Ahmad R, Kochumon S, Chandy B, Shenouda S, Koshy M, Hasan A, et al. TNF-α Drives the CCl4 Expression in Human Monocytic Cells: Involvement of the SAPK/JNK and NF-κb Signaling Pathways. Cell Physiol Biochem (2019) 52:908–21. doi: 10.33594/000000063
59. Kimura S, Noguchi H, Nanbu U, Wang KY, Sasaguri Y, Nakayama T. Relationship Between CCL22 Expression by Vascular Smooth Muscle Cells and Macrophage Histamine Receptors in Atherosclerosis. J Atherosc Thromb (2018) 25:1240–54. doi: 10.5551/JAT.44297
60. Abi-Younes S, Si-Tahar M, Luster AD. The CC Chemokines MDC and TARC Induce Platelet Activation via CCR4. Thromb Res (2001) 101:279–89. doi: 10.1016/S0049-3848(00)00402-3
61. Appleby MA, Michaels AD, Chen M, Gibson CM. Importance of the TIMI Frame Count: Implications for Future Trials. Curr Control Trials Cardiovasc Med (2000) 1:31–4. doi: 10.1186/CVM-1-1-031/METRICS
62. Mach F, Sauty A, Iarossi AS, Sukhova GK, Neote K, Libby P, et al. Differential Expression of Three T Lymphocyte-Activating CXC Chemokines by Human Atheroma-Associated Cells. J Clin Invest (1999) 104:1041–50. doi: 10.1172/JCI6993
63. Van Den Borne P, Quax PHA, Hoefer IE, Pasterkamp G. The Multifaceted Functions of CXCL10 in Cardiovascular Disease. BioMed Res Int (2014) 2014:1–11. doi: 10.1155/2014/893106
64. Bujak M, Dobaczewski M, Gonzalez-Quesada C, Xia Y, Leucker T, Zymek P, et al. Induction of the CXC Chemokine Interferon-γ-Inducible Protein 10 Regulates the Reparative Response Following Myocardial Infarction. Circ Res (2009) 105:973–83. doi: 10.1161/CIRCRESAHA.109.199471
65. Koten K, Hirohata S, Miyoshi T, Ogawa H, Usui S, Shinohata R, et al. Serum Interferon-Gamma-Inducible Protein 10 Level was Increased in Myocardial Infarction Patients, and Negatively Correlated With Infarct Size. Clin Biochem (2008) 41:30–7. doi: 10.1016/J.CLINBIOCHEM.2007.10.001
66. Saraiva M, O’Garra A. The Regulation of IL-10 Production by Immune Cells. Nat Rev Immunol (2010) 10:170–81. doi: 10.1038/nri2711
67. Xu S, Zhang J, Liu J, Ye J, Xu Y, Wang Z, et al. The Role of Interleukin-10 Family Members in Cardiovascular Diseases. Int Immunopharmacol (2021) 94:107475. doi: 10.1016/J.INTIMP.2021.107475
68. Mallat Z, Besnard S, Duriez M, Deleuze V, Emmanuel F, Bureau MF, et al. Protective Role of Interleukin-10 in Atherosclerosis. Circ Res (1999) 85:1–8. doi: 10.1161/01.RES.85.8.E17
69. Pinderski Oslund LJ, Hedrick CC, Olvera T, Hagenbaugh A, Territo M, Berliner JA, et al. Interleukin-10 Blocks Atherosclerotic Events In Vitro and In Vivo. Arterioscler Thromb Vasc Biol (1999) 19:2847–53. doi: 10.1161/01.ATV.19.12.2847
70. Yang Z, Zingarelli B, Szabó C. Crucial Role of Endogenous Interleukin-10 Production in Myocardial Ischemia/Reperfusion Injury. Circulation (2000) 101:1019–26. doi: 10.1161/01.CIR.101.9.1019
71. Sproston NR, Ashworth JJ. Role of C-Reactive Protein at Sites of Inflammation and Infection. Front Immunol (2018) 9:754/BIBTEX. doi: 10.3389/FIMMU.2018.00754/BIBTEX
Keywords: myocardial infarction, inflammation, cytokines, clot formation, endothelium
Citation: Kalinskaya A, Dukhin O, Lebedeva A, Maryukhnich E, Rusakovich G, Vorobyeva D, Shpektor A, Margolis L and Vasilieva E (2022) Circulating Cytokines in Myocardial Infarction Are Associated With Coronary Blood Flow. Front. Immunol. 13:837642. doi: 10.3389/fimmu.2022.837642
Received: 16 December 2021; Accepted: 21 January 2022;
Published: 15 February 2022.
Edited by:
Daniele Catalucci, Institute of Genetic and Biomedical Research (CNR), ItalyReviewed by:
Carlos Ernesto Fernández-García, Princess University Hospital, SpainCopyright © 2022 Kalinskaya, Dukhin, Lebedeva, Maryukhnich, Rusakovich, Vorobyeva, Shpektor, Margolis and Vasilieva. This is an open-access article distributed under the terms of the Creative Commons Attribution License (CC BY). The use, distribution or reproduction in other forums is permitted, provided the original author(s) and the copyright owner(s) are credited and that the original publication in this journal is cited, in accordance with accepted academic practice. No use, distribution or reproduction is permitted which does not comply with these terms.
*Correspondence: Leonid Margolis, bWFyZ29saWxAbmloLmdvdg==
Disclaimer: All claims expressed in this article are solely those of the authors and do not necessarily represent those of their affiliated organizations, or those of the publisher, the editors and the reviewers. Any product that may be evaluated in this article or claim that may be made by its manufacturer is not guaranteed or endorsed by the publisher.
Research integrity at Frontiers
Learn more about the work of our research integrity team to safeguard the quality of each article we publish.