- Wits/South African Medical Research Council (SAMRC) Antiviral Gene Therapy Research Unit, School of Pathology, Faculty of Health Sciences, University of the Witwatersrand, Johannesburg, South Africa
Infection with the hepatitis B virus (HBV) continues to pose a major threat to public health as approximately 292 million people worldwide are currently living with the chronic form of the disease, for which treatment is non-curative. Chronic HBV infections often progress to hepatocellular carcinoma (HCC) which is one of the world’s leading causes of cancer-related deaths. Although the process of hepatocarcinogenesis is multifaceted and has yet to be fully elucidated, several studies have implicated numerous long non-coding RNAs (lncRNAs) as contributors to the development of HCC. These host-derived lncRNAs, which are often dysregulated as a consequence of viral infection, have been shown to function as signals, decoys, guides, or scaffolds, to modulate gene expression at epigenetic, transcriptional, post-transcriptional and even post-translational levels. These lncRNAs mainly function to promote HBV replication and oncogene expression or downregulate tumor suppressors. Very few lncRNAs are known to suppress tumorigenesis and these are often downregulated in HCC. In this review, we describe the mechanisms by which lncRNA dysregulation in HBV-related HCC promotes tumorigenesis and cancer progression.
1 Introduction
Once thought to be transcriptional noise by virtue of their apparent inability to encode proteins, long non-coding RNAs (lncRNAs) have since emerged as vital regulators of gene expression. The discovery and annotation of lncRNAs have picked up speed over the last few years, thanks to availability of deep sequencing techniques and bioinformatic tools. LncRNAs are larger than 200 bases, a feature that distinguishes them from other non-coding RNAs, although they may vary in size from a few hundred to a few thousand nucleotides. These diverse molecules are encoded at various regions of the genome and can be classified according to their orientation in relation to the nearby protein coding genes [reviewed in (1)]. Long intergenic non-coding RNAs, or lincRNAs, are the most abundant type of lncRNA and, as the name suggests, are encoded in the vast expanse of DNA that separates protein coding regions. The second most abundant type of lncRNA are the antisense lncRNAs which are transcribed from the opposite strand of protein coding genes. Thus, these lncRNAs possess the inherent ability to interact through complementary base pairing with mRNA transcribed from the same location and thereby regulate it. Less abundant sense lncRNAs are encoded within protein-coding genes in the same orientation as the gene, and intronic lncRNAs can be found within the introns of genes. Finally, the least abundant are bi-directional lncRNAs, which are located adjacent to protein-coding genes and are transcribed from the same promoter, but in an opposite direction. LncRNAs may act in cis on nearby genes or in trans on distant genes, mRNA or proteins (2). Like mRNAs, lncRNAs are transcribed by RNA polymerase II and may contain 5’ caps, polyadenylated tails, multiple exons, and can also undergo alternative splicing albeit to a lesser extent [reviewed in (2)].
2 Hepatitis B Virus (HBV)-Related Hepatocellular Carcinoma (HCC) and LncRNA Dysregulation
The development of lncRNA microarrays has facilitated comparative profiling of lncRNAs in cancerous and non-cancerous tissues. Numerous lncRNAs are now known to display differential expression profiles in most types of cancers, including HBV-related HCC [reviewed in (3–5)]. Chronic HBV infection is a major risk factor for development of HCC. Prolonged HBV replication promotes immune-mediated inflammation of the liver which results in cirrhosis and eventual malignant transformation of hepatocytes (6). In a previous review, we discussed the roles of several key lncRNAs in the etiology of HBV-related HCC (4). Subsequent studies have further characterized several of these lncRNAs providing valuable insight into their functionality, and potential as therapeutic targets or biomarkers. In addition, many other lncRNAs have been found to be upregulated during chronic HBV infection and have been implicated in progression to HCC. An updated summary of lncRNAs dysregulated in HBV-related HCC and their functions can be found in Table 1.
Certain lncRNAs, such as UCA1, DLEU2, HULC and DBH-AS1, can be directly or indirectly upregulated by the HBV x (HBx) protein (8, 12, 18, 26). However, these lncRNAs may not necessarily be specific to HBV-related HCC as several, including those not induced by HBx, are commonly dysregulated in a variety of other cancers (5). Differential lncRNA plasma concentrations are also observed in individuals with resolved, inactive, or chronic HBV infection (46). Certain lncRNAs, such as HOTTIP, MEG3 and PCAT32, are significantly upregulated in resolved cases, and may serve as early prognostic markers for resolution of HBV infection.
Whilst a few dysfunctional lncRNAs are common to HCCs of different viral etiologies, most are specific to the type of virus-induced HCC [reviewed in (4, 47)]. Since hepatitis virus-induced HCCs account for most cases, lncRNAs that are dysregulated in non-viral HCCs have not been fully investigated. A recent study shows that non-viral HCC also exhibits a differential expression profile when compared to hepatitis virus-induced HCC (48). However, lncRNAs such as HOTAIR and H19 have been found to be upregulated in obesity-induced HCC (49). These lncRNAs, along with MALAT1 and HULC, are also upregulated in non-alcoholic fatty liver disease which is a risk factor for HCC (50). Additional studies, taking HCC etiology into consideration, are required to determine which lncRNAs are commonly dysregulated.
LncRNAs are instrumental in the proliferation, metastasis, and invasion of cancerous cells, tumor angiogenesis, covalently closed circular DNA (cccDNA) stabilization, and modulation of HBV replication [reviewed in (3, 40, 51)]. Thus, lncRNAs have the potential to serve as novel therapeutic targets. To this end, understanding the mechanism of action is crucial to developing relevant therapies. Numerous studies have been conducted with the aim of elucidating the role of lncRNAs in various cancers. What can be gathered is that a single lncRNA can interact with several biomolecules to initiate or promote oncogenesis. This review will describe the mechanisms of action of a variety of dysregulated lncRNAs associated with HBV-related HCC.
3 Mechanisms of Action of LncRNAs
The molecular interactions between lncRNAs and their targets are quite diverse. Although not entirely clear, these interactions are most likely to be dependent on the sequence of the lncRNA. These sequences would influence DNA or RNA interactions through complementary base pairing, or the secondary structure of lncRNAs, which may enable interactions with target proteins [reviewed in (52)]. These molecular interactions are classified into four archetypes: scaffold, guide, decoy and signal [reviewed in (53)]. LncRNAs which form scaffolds support the formation and stabilization of protein complexes whereas guide lncRNAs direct their targets to specific regions of the genome. LncRNAs can also mimic the targets of transcription factors and microRNAs (miRNAs) and thus act as decoys to regulate gene expression. Signal lncRNAs are transcribed at precise stages of cell development and may act as signals for transcription factors to initiate transcription of developmental genes. These archetypes are not mutually exclusive as certain lncRNAs can exert gene regulatory functions by more than one type of molecular interaction.
The interacting partners of lncRNAs and the mechanism by which lncRNAs exert their functions largely depends on their cellular location [reviewed in (3)]. LncRNAs located in the nuclear compartment interact mainly with DNA or proteins to regulate gene expression at an epigenetic or translational level. Some nuclear lncRNAs interact with RNA and proteins to alter splicing patterns. Cellular lncRNAs can interact with RNA or proteins to regulate gene expression post-transcriptionally or post-translationally respectively. Post-transcriptional regulation is mediated by lncRNAs acting as competitive endogenous RNAs (ceRNAs) which sequester or sponge miRNAs to de-repress miRNA-mediated post-transcriptional silencing of certain genes. On the other hand, certain lncRNAs are precursors of miRNAs and can silence mRNA expression. LncRNAs can directly bind to and stabilize proteins, enhancing their bioavailability and functionality. Conversely, certain lncRNAs can also alter or inhibit the functionality of proteins. LncRNAs can also promote protein degradation by facilitating ubiquitination (40). The mechanisms by which HBV-specific lncRNAs exert their oncogenic functions are described below.
3.1 Transcriptional Regulation in the Nucleus
Oncogenes can be upregulated, or tumor suppressor genes downregulated by epigenetic modification of chromatin, which alters the accessibility of the DNA to transcription factors and proteins. Up to 38% of lincRNAs alter chromatin architecture, mainly by directing repressive chromatin modifying complexes to specific genomic sites (54). The Polycomb Repressor complex 2 (PRC2) is one such repressive chromatin modifying complex that is commonly modulated by HBV-dysregulated lncRNAs. PRC2 consists of three core subunits: the Enhancer of Zest Homolog 2 (EZH2), the Suppressor of Zest homolog 12 (SUZ12), and the Embryonic Ectoderm Development (EED) subunits (55). SUZ12 is responsible for the structural stability of the complex. EED guides the PRC2 to histone 3 (H3) lysine 27 (K27) and stimulates the methyltransferase activity of EZH2, which catalyzes the tri-methylation of H3K27 (H3K27me3). This action subsequently leads to chromatin compaction and transcriptional repression of the targeted genes, which are usually involved in the regulation of cell differentiation, development and fate during embryogenesis (56). Conversely, lncRNAs can promote open chromatin structure by preventing PRC2-mediated compaction or by recruiting activating chromatin modifiers to the sites of certain viral or host oncogenes, which can then be transcribed (Figure 1).
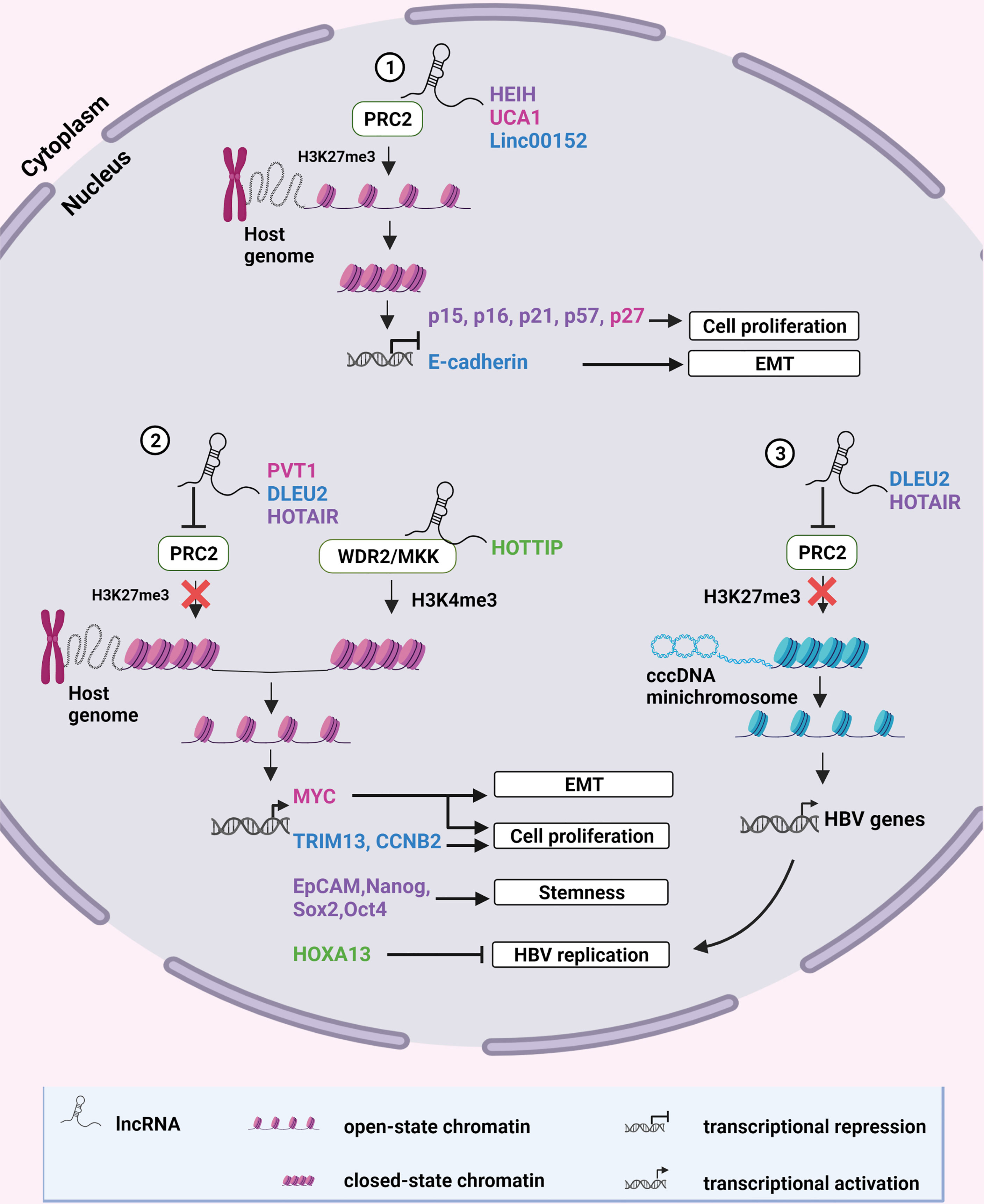
Figure 1 Epigenetic modification of chromatin by lncRNAs. (1) LncRNAs HEIH, UCA1 and Linc00152 recruit the repressive chromatin modifying complex, PRC2, to specific sites on the human genome to catalyze H3K27 trimethylation. This results in chromatin compaction and transcriptional repression of tumor suppressor genes. (2) LncRNAs PVT1 and HOTAIR inhibit formation of the PRC2 complex whereas DLEU2 displaces PRC2 from chromatin to prevent H3K27 methylation, allowing transcription of oncogenic host genes. HOTTIP recruits the activating chromatin-modifying complex WDR2/MKK to mediate H3K4 methylation of chromatin enabling the transcription of HOXA13 which suppresses HBV replication. (3) DLEU2 and HOTAIR can also inhibit PRC2 recruitment to HBV cccDNA, alleviating epigenetic silencing and promoting HBV replication. Color coding of names indicate a functional relationship between the lncRNA and its target. Created with BioRender.com.
Transcription of genes can also be enhanced or suppressed by modulating the activities of transcription factors that interact with the promoter regions of a gene. Certain lncRNAs regulate gene expression by direct interaction with the promoters of protein coding genes or by recruiting transcription factors to promoter sites (Figure 2) [reviewed in (51)]. The lncRNAs which employ epigenetic and/or direct transcriptional gene regulatory mechanisms to promote HBV replication, persistence and HCC are described below.
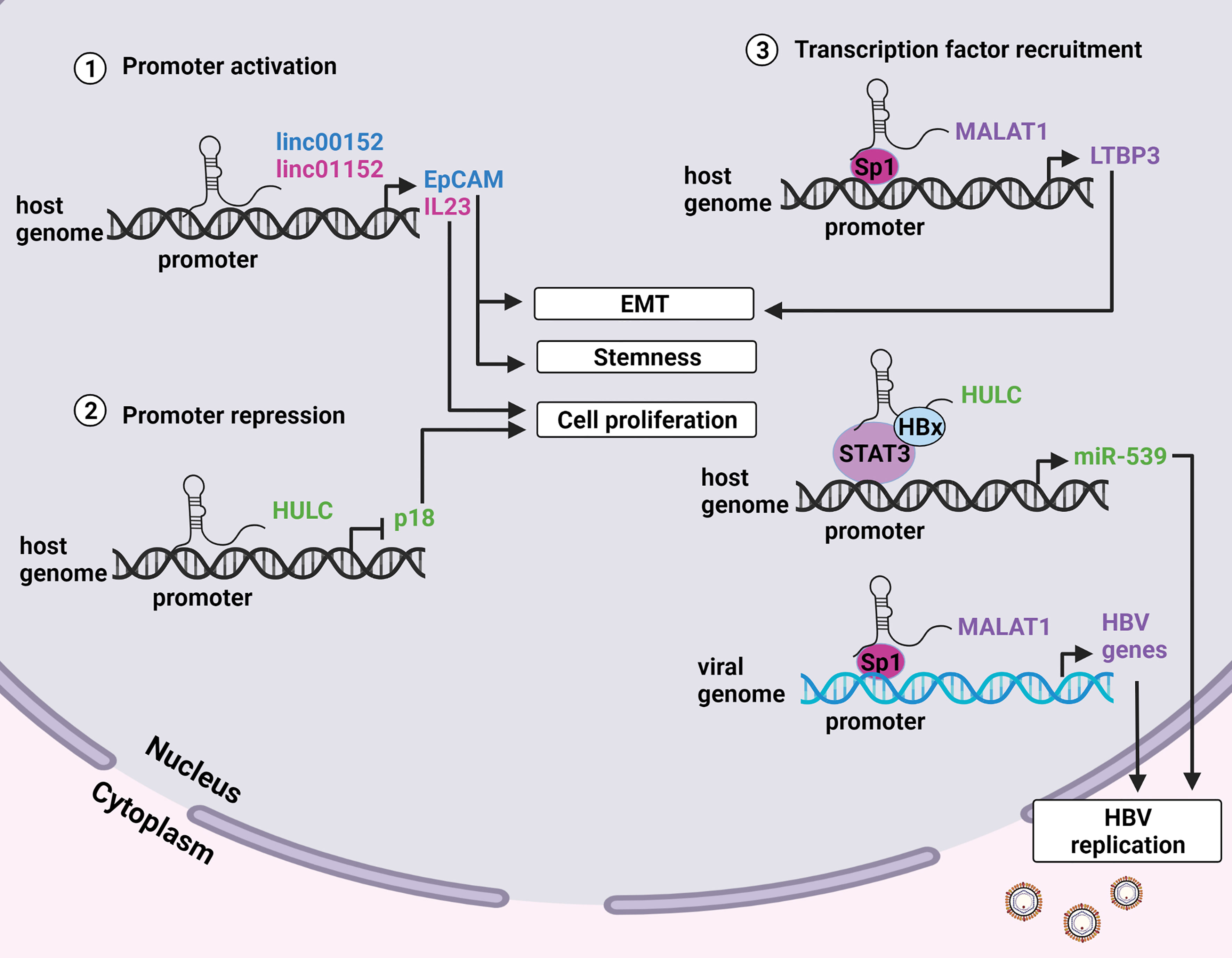
Figure 2 LncRNA modulation of promoter activity. (1) LncRNAs linc00152 and linc01152 have enhancer-like properties and interact with the promoters of the EpCAM and IL23 genes respectively. EpCAM positive cells display enhanced stemness and metastatic potential, and increased levels of IL23 promote proliferation. (2) LncRNA HULC interacts with the promoter of the tumor suppressor p18 to inhibit its transcription and promote cell proliferation. (3) Certain lncRNAs can guide transcription factors to the promoters of specific genes to enhance transcription. MALAT1 recruits the transcription factor Sp1 to the promoter of the LTBP3 gene on the host genome resulting in metastasis. HULC and HOTAIR recruit the transcription factors STAT3 and Sp1 to host and viral genomes respectively to enhance transcription of viral genes thus promoting HBV replication. Created with BioRender.com.
3.1.1 HEIH
High expression in hepatocellular carcinoma (HEIH) is a 1,781 nucleotide long, polyadenylated lincRNA that was first identified in HBV-related HCC tissues (7). Its transcription is upregulated by the transcription factor Sp1 in HCC and HBV-infected cirrhotic tissue specimens. HEIH associates with the EZH2 subunit of the PRC2 through its 5’ end and recruits EZH2 to the promoter regions of the cell-cycle regulatory proteins p15, p16, p21 and p57. These genes are subsequently silenced, leading to increased cell proliferation and ultimately HCC. Levels of HEIH are also known to increase as disease progresses from a chronic HBV infection to cirrhosis then HCC, and could be useful to estimate the stage of disease (57)
3.1.2 UCA1
Urothelial Cancer associated 1 (UCA1) is a 1,400 nucleotide long lincRNA that was first identified as a marker of bladder transitional cell carcinoma (58). It was also found to be upregulated by HBx in HBV-related HCC specimens and HBx-expressing cell lines (8). Its expression is associated with increased cell proliferation and inhibition of apoptosis. As with many other lincRNAs, UCA1 can recruit EZH2 to mediate epigenetic silencing. Its target is the tumor suppressor p27, which when suppressed, allows cyclin-dependent kinase 2 (CDK2) to accelerate G1/S cell cycle progression, cell proliferation and development of HCC.
3.1.3 Linc00152
Linc00152 is upregulated by HBx in HBV-related HCC tissues where it is localized mainly in the nucleus and promotes epithelial-mesenchymal transition (EMT) (9). The hallmark of EMT is switching from E-cadherin to N-cadherin expression, which increases cellular metastasis and invasiveness, and is associated with an overall poor patient outcome in many types of cancer [reviewed in (59)]. At an epigenetic level, linc00152 recruits EZH2 to the promoter of the gene encoding E-cadherin to induce its suppression, and promote EMT (9). As a cis-acting lincRNA in HCC-related tissues, linc00152 also enhances the promoter activity of the nearby Epithelial Cell Adhesion Molecule (EpCAM) gene, which results in the activation of mTOR signaling (10). EpCAM-positive HCC cells display stem cell-like properties, which promote tumor formation and proliferation (60). In vitro knockdown of linc00152 in HCC cells decreases the promoter activity of EpCAM, and increases expression of E-cadherin while suppressing N-cadherin to successfully decrease the proliferative and migratory capacity of HCC cells (9, 10). Targeting this lincRNA may reduce the likelihood of metastasis in HBV-related HCC.
3.1.4 PVT1
Plasmacytoma Variant Translocation 1 (PVT1) is a 1,716 nucleotide long lncRNA that is progressively upregulated by TGF-β1 in HBV-related HCC (11, 61). PVT1 alleviates PRC2-mediated repression of the downstream MYC oncogenes via two mechanisms (11). Firstly, it negatively regulates the expression of EZH2 to reduce its concentration in the nucleus, although the exact mechanism by which this occurs has not been determined. Secondly, it directly binds to EZH2 to prevent formation of the PRC2 complex and its recruitment to the MYC promoter. The resultant hypomethylation of this region results in active transcription of c-myc and promotes cell proliferation, migration, and invasion by HBV-positive Hep3B liver cell lines.
3.1.5 DLEU2
Deleted in Lymphocytic Leukemia 2 (DLEU2) is a large antisense lncRNA consisting of 15 exons which can be alternatively spliced to yield three isoforms, the largest of which partially overlaps the RFP2/LEU5 gene (62). It is known to function as a tumor suppressor gene since its deletion was associated with the development of chronic lymphocytic leukemia (63). However, the role of DLEU2 in HCC is quite the opposite. In cellular models of HBV infection, HBx was recently shown to directly promote the expression of DLEU2 by binding to its promoter (12). In addition to upregulating this lncRNA, HBx also directly interacts with DLEU2 at partially overlapping binding sites that are shared with EZH2 (12). Recruitment of HBx-DLEU2 to the viral cccDNA minichromosome thus interferes with EZH2-mediated repression of viral genes to increase viral replication. Similarly, HBx also recruits DLEU2 to host promoter sequences to relieve transcriptional repression of selected host genes, such as TRIM13 and CCNB2. This is achieved by displacing EZH2/PRC2 complexes.
3.1.6 HOTTIP
HOXA transcript at the distal tip (HOTTIP) is a 3,764 nucleotide long antisense lincRNA that is located at the 5’ terminus of the HOXA locus (64). As mentioned earlier, HOTTIP is upregulated in cases of resolved HBV and may thus have an important role in promoting the clearance of HBV. Its expression is also upregulated in an HBx-independent manner in HBV-related HCC tissues where it plays a role in suppressing HBV replication (13). In this instance, it is the HBV DNA polymerase which stabilizes the cAMP-responsive element binding (CREB) transcription factor to promote the expression of HOTTIP. HOTTIP then recruits the chromatin modifying complex WDR5/MKK to the HOXA gene locus through chromosomal looping to mediate H3K4 trimethylation and transcriptional activation of the downstream HOXA13 gene (64). HOXA13 thereafter binds to the HBV enhancer 1 and X promoter to restrain viral replication. In this manner, HBV polymerase negatively regulates replication, possibly to avoid host immune responses, and establish chronicity [reviewed in (65)].
Recently, HOTTIP was found to be significantly upregulated in exosomes obtained from the sera of chronically infected HBV patients who were treated with the nucleoside analog Tenofovir Alafenamide (TAF) (66). The exosomal presence of HOTTIP seemed to enhance the antiviral effects of TAF as evidenced by the significantly downregulated levels of HBV surface antigen (HBsAg) and HBV e antigen (HBeAg), and most importantly cccDNA in HepAD38 cells treated with patient derived exosomes. These promising results were observed in a small study and still require further in vivo investigation.
In non-HBV-related HCC specimens, upregulation of HOTTIP, and the associated increase in HOXA13, is an indication of metastatic potential, increased probability of disease recurrence following liver transplantation, and poor overall survival (67, 68). Upregulation of HOTTIP and HOXA13 was also confirmed in several HCC-derived cell lines. Thus, even though HOXA13 suppresses HBV replication, it may also play a role in promoting HBV-related HCC, although the exact mechanism is yet to be examined.
3.1.7 HOTAIR
HOX Transcript Antisense RNA (HOTAIR) is an approximately 2,200 nucleotide long lincRNA located in the HOXC locus (69). Under normal physiological conditions, HOTAIR acts as a scaffold to simultaneously bind PRC2 and a second repressive chromatin-modifying complex: the LSD1/Co-REST/HDAC1 complex. This occurs through binding PRC2 and the LSD1/Co-REST/HDAC1 complex at 5’ and 3’ ends of HOTAIR respectively (70). This 3’ bound complex is stabilized by the Zinc Finger protein 198 (ZNF198) and is responsible for the removal of gene activating histone acetylations, and H3K4 methylations (71). The RNA helicase DEAD-box helicase 5 (DDX5) also associates with PRC2 via the HOTAIR scaffold and is essential for maintaining the stability of its SUZ12 component (15). The coupling action of HOTAIR therefore reinforces gene repression by these two chromatin-modifying complexes.
During HBV infection, the cccDNA minichromosome is known to associate closely with HOTAIR-encoding chromatin, although the significance of this interaction is not quite clear (72). The HBV minichromosome assumes a chromatin-like state which is also subject to epigenetic silencing by PRC2 thus preventing viral replication (15). This repression is alleviated by miRNA-mediated silencing of the RNA helicase DDX5 (73). Loss of DDX5 subsequently leads to the destabilization of SUZ12 (15). HBx also activates polo-like kinase1 (PLK1), which then phosphorylates both SUZ12 and ZNF128 marking them for ubiquitination by the E3 ligase Mex3b (14, 15, 74). In this scenario, the HOTAIR scaffold is hijacked by Mex3b to facilitate ubiquitination of SUZ12 and ZNF198, targeting them for proteasomal degradation. Resulting destabilization of PRC2 leads to de-repression of viral chromatin and initiation of viral replication. PRC2-repressed genes, such as EpCAM and pluripotency genes (Nanog, Sox2 and Oct4), which are commonly expressed in progenitor hepatic cancer cells, are also upregulated through this mechanism, and are indicative of poor prognosis following tumor resection (15, 60).
In addition to relieving epigenetic repression of cccDNA, HOTAIR actively enhances HBV promoter functions. It does so by guiding the transcription factor SP1 to viral gene promoter sites to upregulate viral transcription and replication (16).
3.1.8 HULC
Highly upregulated in liver cancer (HULC) is another lincRNA that is progressively upregulated in HBV-related disease states (57). This polyadenylated 500 nucleotide transcript was originally found to be upregulated in HCC tissues and is mainly localized in the cytoplasm. However, it also plays vital roles in the nucleus (75). Transcription of HULC falls under the control of a CREB transcription factor-dependent promoter and since HBx can interact with and enhance the affinity of CREB to its promoter, HULC can be indirectly upregulated by HBx (18, 76).
Upregulation of HULC is important to stabilize cccDNA and promote HBV replication (40). Two antiviral cytidine deaminases, APOBEC3A and APOBEC3B, are essential for the degradation of cccDNA and prevention of persistent infections (77). HULC prevents this degradation by upregulating expression of miR-539, which post-transcriptionally silences APOBEC3B. This transcriptional activation of miR-539 is not directly mediated by HULC. Instead, HULC stimulates HBx to activate and recruit the transcription factor STAT3 to the miR-539 promoter.
Another nuclear target of HULC is the downstream gene encoding the tumor suppressor protein p18 (18). In response to DNA damage, p18 is translocated to the nucleus to activate p53 and halt cell-cycle progression (78). However, in HCC tissues high levels of HULC were associated with low levels of p18. It was subsequently shown that HULC downregulates p18 by interacting with its promoter to repress transcription, leading to increased cell proliferation. Thus, in addition to promoting HBV persistence HULC also directly contributes to development of HCC by silencing the tumor suppressor p18.
3.1.9 MALAT1
Metastasis-associated Lung Adenocarcinoma Transcript 1 (MALAT1) is a multi-functional lincRNA that is upregulated in HCC tissues and even more so in HBx-expressing hepatocytes (79). It is initially transcribed as a single lncRNA but is cleaved into two separate molecules that are destined for different cellular compartments (80). The larger non-coding RNA is retained in the nucleus where it functions in a number of ways to promote various types of malignancies, whereas the smaller non-coding RNA is shuttled to the cytoplasm. Both RNAs can activate the ERK/MAPK pathway to promote metastasis in HCC, although the exact mechanism is yet to be elucidated (81).
In the nucleus, MALAT1 also functions as an enhancer-like lncRNA by recruiting the transcription factor Sp1 to the promoter of the Latent Transforming Growth Factor β-Binding Protein 3 (LTBP3) gene, which is closely located on the same chromosome, but transcribed in the opposite orientation (20). MALAT1 binds to both Sp1 and the LTBP3 promoter forming an RNA-protein-DNA triplex which guides the transcription factor to and stabilizes its interaction with the Sp1 consensus sequence located on the LTBP3 promoter. LTBP3 is a glycoprotein that is secreted into the extracellular matrix and promotes the proper folding and secretion of TGF-β (82). LTBP3 also enhances metastatic potential in several cancer cell lines and animal malignancy models (83). In HBx-expressing hepatocytes, upregulation of LTBP3 results in an increase in N-cadherin and vimentin, and a decrease in E-cadherin, which increases the invasive and migratory properties of hepatocytes in vitro and in vivo (79). This effect is mitigated by the knockdown of MALAT1, thus the HBx/MALAT1/LTBP3 axis could be considered a therapeutic target to prevent EMT.
3.1.10 Linc01152
Linc01152 was initially identified as a downregulated lncRNA in HBV-related HCC tumor tissues and was thought to be a tumor suppressor (47). However, a later study showed that the lncRNA is upregulated in HBx-expressing cell lines and can promote cell proliferation in vitro and in vivo (22). In HBV-related HCC patients, tissue and serum levels of IL-23 were positively correlated with levels of linc01152. In vitro experiments revealed that linc01152 binds directly to the IL-23 promoter and activates transcription of this pro-inflammatory cytokine. In a separate study, IL-23 levels correlated with HBV DNA levels, and were shown to mediate liver damage by expanding Th17 cells (21). Overexpression of linc01152 was associated with an upregulation of STAT3 and phosphorylated STAT3, whereas silencing of STAT3 reduced cell proliferation (22). Thus, linc01152 may promote HCC by activating the STAT3 pathway.
3.2 Alternative Splicing
Alternative splicing occurs in the nucleus and provides a means of producing different mature mRNAs from a single precursor mRNA. Transcriptome comparisons between HCC and normal hepatic tissues have shown differential alternative splicing patterns of certain precursor mRNAs, which gives rise to novel protein isoforms that promote tumorigenesis [reviewed in (84)]. LncRNAs are also subject to alternative splicing, but some have also demonstrated the ability to alter splicing patterns by interacting with splicing factors or precursor mRNAs [reviewed in (85)].
One such lncRNA, the Zinc finger E-box binding Homeobox 2 antisense RNA1 (ZEB2-AS1), may promote HBV-related HCC by this mechanism. ZEB2-AS1 overlaps the donor splice site of the ZEB2 gene (86). ZEB2 is a transcriptional repressor of E-cadherin and is thus a mediator of EMT (87). The mRNA that encodes ZEB2 has an unusually long 5’ UTR which contains an internal ribosome entry site (IRES) that is necessary for translation. In normal epithelial cells, this mRNA undergoes splicing to remove a major portion of its 5’ UTR including the IRES, leaving behind a shorter 5’ UTR which contains a sequence that inhibits translation. Thus, ZEB2 mRNA cannot be translated, and the cell maintains an epithelial phenotype.
In the case of HBV-related HCC, increased levels of HBx were associated with increased levels of ZEB2-AS1 and the transition into a mesenchymal phenotype (23). Being an antisense lncRNA, ZEB2-AS1 forms an RNA-RNA duplex through complementary base pairing with the 5’ splice site of the precursor mRNA encoding ZEB2 (86). This prevents binding of the spliceosome, and the longer IRES-containing 5’ UTR is retained in the mature transcript allowing for ZEB2 to be translated. Increased levels of ZEB2 then suppresses transcription of E-cadherin and promotes EMT (Figure 3).
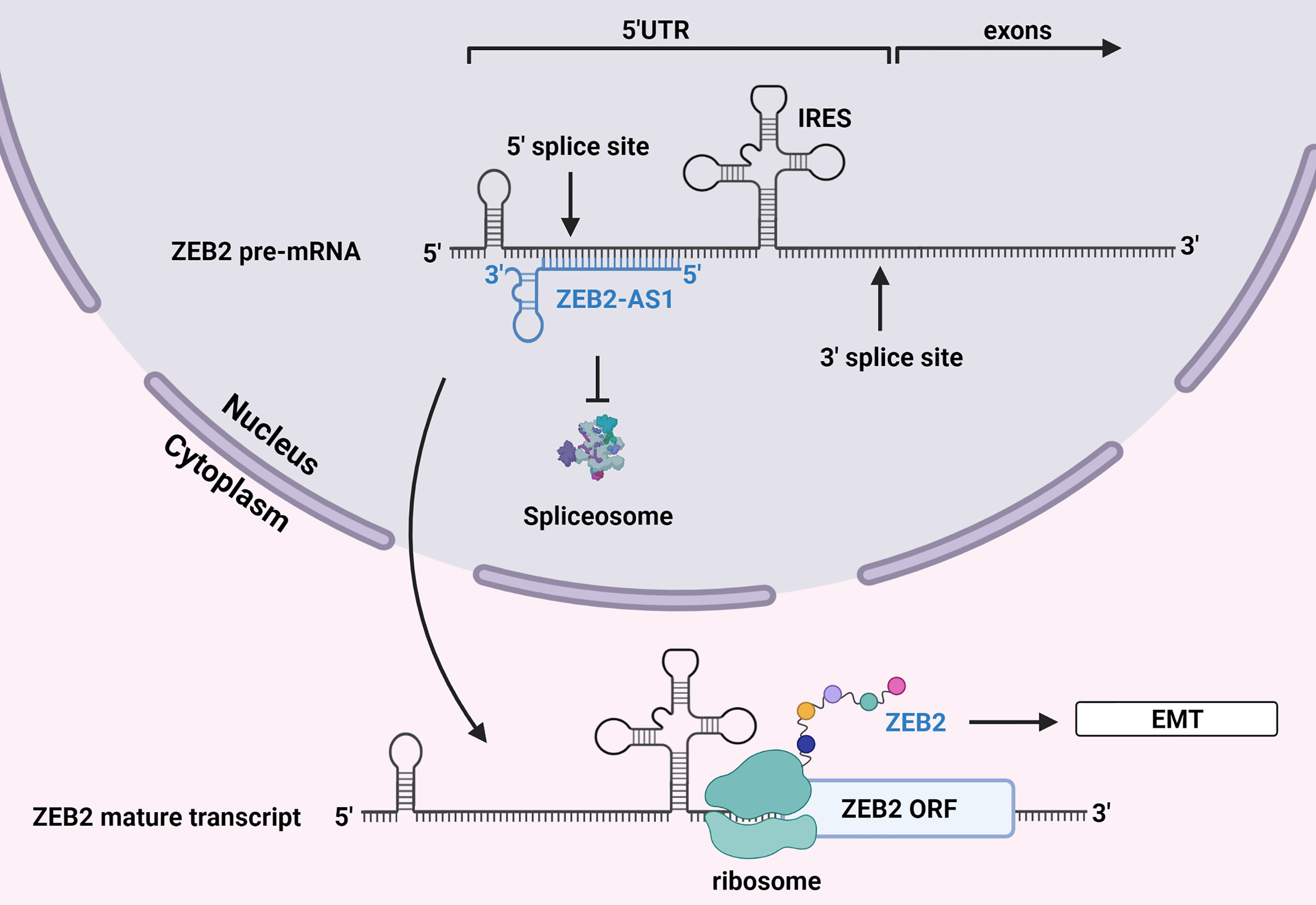
Figure 3 Alternative splicing by lncRNA ZEB2-AS1. The antisense lncRNA ZEB2-AS1 binds to the 5’ UTR donor splice site of mRNA encoding ZEB2, a transcriptional repressor of E-cadherin. This prevents the spliceosome from eliminating this region of the transcript, which contains an IRES that is necessary for translation. ZEB2 is subsequently translated, and its increased levels promote EMT. Created with BioRender.com.
3.3 Competitive Endogenous RNAs (ceRNAs)
ceRNAs are found in the cytoplasm and mediate post-transcriptional gene regulation. This type of lncRNA contains inherent miRNA response elements (MREs), allowing them to sequester or sponge complementary miRNAs (Figure 4). This action reduces availability of key miRNAs with tumor suppressor functions, thus facilitating de-repression of their oncogenic target molecules [reviewed in (88)].
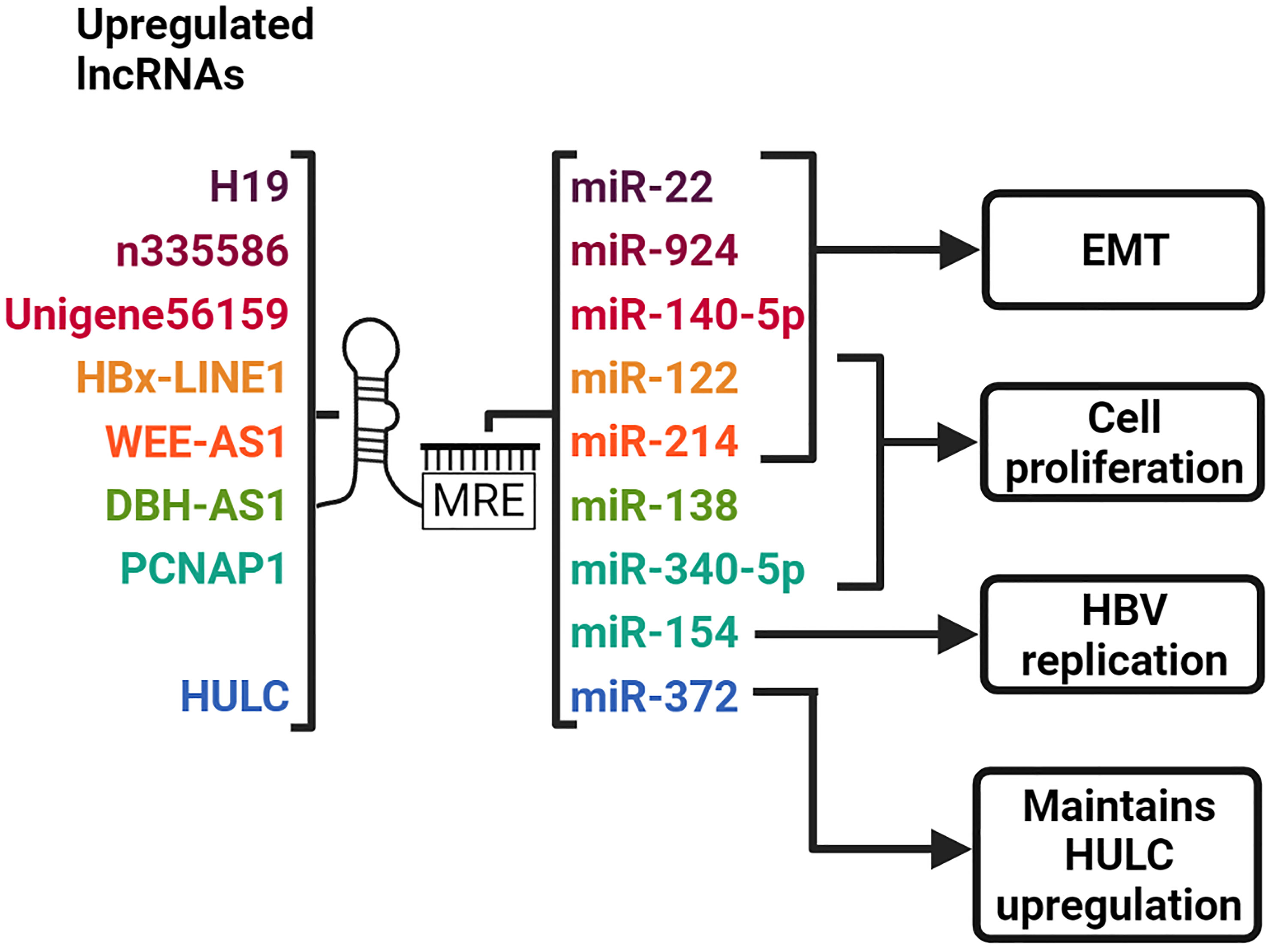
Figure 4 LncRNAs act as ceRNAs to sequester complementary miRNAs. LncRNAs harbor MREs which allow them to bind to miRNAs with complementary sequences. H19, n335586 and Unigene56159 promotes EMT by sequestering miR-22, miR-924 and miR-140-5p, respectively. HBx-LINE1 and WEE2-AS1 promotes EMT and cell proliferation by sponging miR-122 and miR-214, respectively. DBH-AS1 increases cell proliferation by sequestering miR-138. PCNAP1 sponges miR-340-5p and miR-154 to promote cell proliferation and HBV replication, respectively. HULC sequesters miR-372, thereby maintaining its upregulation. Created with BioRender.com.
3.3.1 HBx-LINE1
HBx-LINE1 is a unique chimeric lncRNA composed of the HBx and human LINE1 transcripts. It is formed by the integration of HBV DNA into the host LINE1 gene and activates the LINE1 sequence (24). When investigated in HBV-positive HCC cell lines, HBx-LINE1 was actively transcribed by the HBx promoter, from the site of viral integration on chr.8p11.21. HBx-LINE1 expression was also observed in 23.3% to 42.5% of HBV-positive HCC tumors, and correlated with reduced overall survival (24, 25). Furthermore, in vitro investigations revealed that HBx-LINE1 enhanced migration and invasion by inducing EMT, and promoted the translocation of β-catenin into the nucleus where it activates the Wnt signaling pathway.
In HBx-LINE1-positive HCC tissues and cells, the HBx-LINE1 transcript was shown to contain six confirmed binding sites for the tumor suppressor miR-122 (25). Specific binding of miR-122 to each of the corresponding HBx-LINE1 sites, and the inverse correlation between these transcripts, suggests that HBx-LINE1 acts as a molecular sponge that sequesters miR-122. Reduced levels of miR-122 in HBx-LINE1-positive HCC cells were associated with reduced E-cadherin and increased WNT1, phosphorylated β-catenin, migration, invasion and proliferation. Overexpression of miR-122 in these cells reversed these effects and significantly depleted HBx-LINE1 expression. When assessed in the mouse liver, HBx-LINE1 was found to sequester miR-122, which led to reduction of E-cadherin, promotion of β-catenin signaling and initiation of abnormal mitosis and liver injury. The evidence from these studies suggests that HBx-LINE1 sequesters miR-122 which leads to tumor progression by the induction of EMT and consequent activation of the Wnt/β-catenin signaling pathway (24, 25).
3.3.2 DBH-AS1
The HBx-induced expression of the lncRNA DBH-AS1 in HBV-related HCC is thought to involve the tumor suppressor protein p53. Studies have revealed a putative p53 binding site upstream of DBH-AS1 and have also shown that p53 suppression results in increased expression of DBS-AS1 (26). Interestingly, HBx is known to inhibit p53 to promote HCC (89). It is thus likely that HBx inhibition of p53 relieves transcriptional repression of DBH-AS1. Overexpression of DBH-AS1 in HBV-related HCC has been associated with decreased apoptosis and cell proliferation and is mediated by the activation of the ERK/p38/JNK/MAPK pathway, although the exact mechanism by which this occurs has not yet been elucidated. Activation of this pathway downregulates the cyclin-dependent kinase inhibitors p16, p21, and p27. The downstream effects of this suppression include the upregulation of the cyclin-dependent kinase CDK6, and cyclins D1 and E1, which promote G1/S and G2/M cell cycle progression with cell proliferation. A subsequent study uncovered that DBH-AS1 functions as a molecular sponge for miR-138, which leads to activation of the FAK/Src/ERK pathway and results in HCC (27). A correlation between DBH-AS1 and HBsAg levels in infected individuals and tumor size in HBV-induced HCC has been shown (26).
3.3.3 PCNAP1
The lncRNA PCNAP1 is a pseudogene of PCNA (90). PCNAP1 is highly expressed in HBV-positive HCC cells and patient tumor tissues (28, 29). Its upregulation was also observed in the livers of HBV-infected chimeric mice harboring human hepatocytes (28). This suggests that PCNAP1 expression is positively associated with HBV infection and HBV-related HCC. PCNAP1 has more than one target miRNA binding site. In the study performed by Feng et al, the results indicated that PCNAP1 acted as a ceRNA to sponge the tumor suppressor miR-154, thereby preventing it from inhibiting PCNA expression. This allows PCNA to bind cccDNA through interaction with the HBV core protein, promoting HBV replication and cccDNA accumulation. In the more recent study by He et al., PCNAP1 was suggested to promote HCC cell proliferation by sequestering the tumor suppressor miR-340-5p, and preventing it from directly suppressing ATF7 expression in HCC cells (29). Furthermore, PCNAP1 overexpression was significantly associated with reduced overall survival and may be a potential predictor of HCC prognosis.
3.3.4 WEE2-AS1
The antisense lncRNA WEE2-AS1 is upregulated in HBx-expressing HCC cell lines and tissues (30). It is associated with multiple hallmarks of HCC including increased cell proliferation, migration, invasion, hepatic vascular invasion, and decreased apoptosis (30). The Fermitin family member 3 (FERMT3) was identified as the downstream target of WEE2-AS1. Deregulation of FERMT3 is observed in various types of cancers, including HBV-related HCC, and involves activation of the Akt signaling pathway. The exact mechanism by which FERMT3 is upregulated by WEE2-AS1 is not yet known. However, molecular interaction prediction tools identified both WEE2-AS1- and FERMT3-encoding mRNA as targets for miR-214. In the case of glioblastoma, WEE2-AS1 is enriched in the cytoplasm and acts as a molecular sponge for other miRNAs, thus it is possible that it may act as a molecular sponge to sequester miR-214 and inhibit post-transcriptional silencing of FERMT3 (31).
3.3.5 Unigene56159
The lncRNA Unigene56159, of approximately 2,653 nucleotides in length, is located in the second intron of the ROBO1 gene (32). Its expression is significantly upregulated in HBV-related HCC tissues and cells, and it promotes cell migration, invasion, and EMT in vitro. Further investigation revealed that Unigene56159 may function as a ceRNA by acting as a sponge to sequester miR-140-5p and inhibit repressive activity on its target Slug, which is a repressor of the adhesion molecule E-cadherin (32, 33). The sequestration of miR-140-5p by Unigene56159 therefore promotes EMT which leads to hepatoma cell migration and invasion.
3.3.6 H19
H19 was one of the first lncRNAs to be discovered (91). It is approximately 2,300 nucleotides long and is an oncofetal lncRNA that is transcribed only from the maternal allele of the H19 gene during embryogenesis, and is suppressed postnatally (92). H19 expression is regulated by c-Myc which binds and promotes histone acetylation of E-boxes within the H19 promoter leading to initiation of transcription (93). Over the years, multiple studies have revealed that expression of this lncRNA is resumed in several cancers, where it exhibits either tumor suppressor or oncogenic properties [reviewed in (94)]. In HCC, the role of H19 is somewhat controversial and requires further examination. In the tumor tissue of patients with HBV-related HCC, H19 is significantly upregulated and is positively associated with lymph node invasion and distal metastasis, and negatively correlated with overall patient survival (95). Knockdown of H19 in HepG2.2.15 cells inhibited cell proliferation, migration, and invasion. This was accompanied by reduced expression of N-cadherin, Vimentin, β-catenin, and Matrix Metalloproteinase-9, all of which promote EMT. Using online prediction tools, miR-22 was identified as a putative target of H19 and was subsequently shown to be negatively regulated by H19 in HCC tissues and cell lines. The inhibition of migration and invasion induced by H19 knockdown was also rescued by the knockdown of miR-22. Thus, these results suggest that H19 promotes progression of HBV-related HCC by sponging miR-22 to increase the expression of EMT-associated proteins (95). Interestingly, H19 promoter-driven expression of the a diphtheria toxin has been evaluated as treatment for cancer [reviewed in (96)]. Since H19 is abundantly expressed in certain tumors and not surrounding tissues, delivery of a diphtheria toxin expressing plasmid under the transcriptional control of the H19 promoter has the potential to kill cancerous cells selectively.
In direct contrast to its role as a promoter of EMT in HBV-related HCC, H19 inhibited EMT in HCC in an earlier study (36). H19 expression was reduced in HCC tumor tissues compared to the adjacent peritumoral tissues, and lower H19 tumor/peritumoral ratios were associated with intrahepatic tumor metastasis, reduced tumor capsule integrity, and decreased disease-free survival. H19 expression was also reduced in invasive HCC specimens compared to non-invasive HCC specimens suggesting its importance in suppressing EMT. Investigation of HCC cell lines in vitro, revealed that H19 may reverse EMT by enhancing the expression of the EMT suppressor miR-200 family. This increase in miR-200 expression is brought about epigenetically through recruitment of the histone acetylase HnRNP U/PCAF/RNA PolII protein complex to chromatin regions upstream of the miR-200 family.
3.3.7 HULC
In addition to transcriptional silencing of p18, HULC acts as a molecular sponge for multiple cytoplasmic miRNAs. One of these targets is miR-372 which mediates translational suppression of a cAMP-dependent protein kinase, PRKACB (19). De-repression of PRKACB mRNA by miR-372 sponging increases the amount of PRKACB protein available to phosphorylate and activate CREB, which as mentioned earlier, is required for upregulating expression of HULC. Thus, HULC is part of an autoregulatory loop that serves to maintain its upregulation in HBV-related HCC.
3.3.8 n335586
LncRNA n335586 is located within the CKMT1A gene and has a length of approximately 270 nucleotides (37). This lncRNA is highly expressed in HBV-positive HCC tissues and cells. This is associated with decreased expression of E-cadherin and cytokeratin, and increased expression of vimentin pointing to a role in EMT. LncRNA n335586 functions in cis by upregulating expression of its host gene CKMT1A which was associated with increased migration and invasion of HCC cells. This finding, and the fact that n335586 overlaps with the downstream portion of the CDS and the 3’ UTR of the CKMT1A gene, suggests that n335586 may function as a ceRNA to prevent miRNA-mediated degradation of CKMT1A. The tumor suppressor miR-924 bound n335586 and CKMT1A transcripts competitively, which led to enhanced migration, invasion, and EMT in vitro and metastasis in vivo. Thus, n335586 promotes EMT by acting as a molecular sponge for miR-924 which alleviates post-transcriptional repression of the CKMT1A.
3.4 Protein Interactions
Apart from interacting with proteins that make up chromatin-modifying complexes in the nucleus, certain lncRNAs can post-translationally regulate oncogenic or tumor suppressor proteins found in both the nuclear and cytoplasmic compartments. They enhance protein stability by either binding directly to the target protein or by preventing ubiquitination and proteasomal degradation [reviewed in (51)]. Conversely, binding of lncRNAs can also inhibit protein functionality or promote protein degradation (Figure 5).
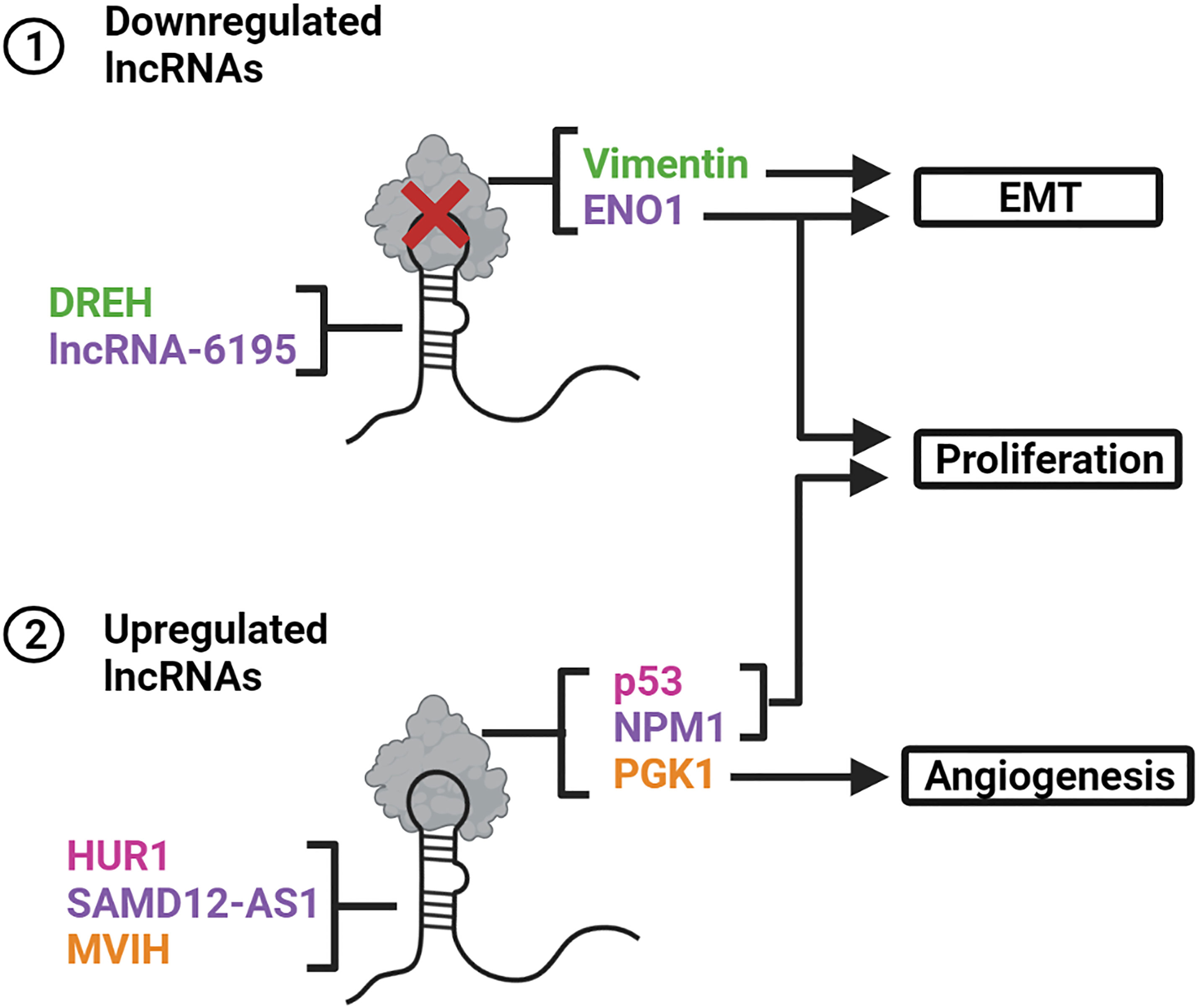
Figure 5 LncRNAs interact with proteins and alter their stability or functionality. (1) DREH and LncRNA-6195 are downregulated in HBV-related HCCs. The lack of interaction between DREH and the protein vimentin promotes EMT. Deficient binding of LncRNA-6195 to ENO1 promotes EMT and cell proliferation. (2) HUR1, SAMD12-AS1 and MVIH are upregulated in HBV-related HCCs. HUR1 and SAMD12-AS1 enhance cell proliferation by inhibiting p53 and NPM1, respectively. MVIH interacts with PGK1 to prevent its secretion thereby promoting angiogenesis. Created with BioRender.com.
3.4.1 DREH
LncRNA DREH was originally discovered in mice. Its expression is inhibited by HBx, as demonstrated by its significant downregulation in the livers of HBx-transgenic mice and HBx-expressing mouse liver cells (38). Suppression of DREH in mouse liver cells in vitro leads to increased cell proliferation, migration, and invasion, whereas in vivo investigations revealed DREH overexpression represses tumor growth and metastasis. DREH interacts with and inhibits the intermediate filament protein vimentin. Further analysis in murine hepatoma cells revealed that DREH may cause the cytoskeleton to reverse from a high to a low migration phenotype by altering the filamentous structure of vimentin. These results suggests that DREH acts as a tumor suppressor by interacting with the vimentin protein and reducing its expression, thereby altering the cytoskeleton structure to inhibit tumor metastasis.
Sequence analysis revealed hDREH, located on human chromosome 5, as a possible human ortholog of the murine DREH (38). The lncRNA hDREH transcribed from this locus was significantly downregulated in HBV-related HCC tissues. Its downregulation was associated with reduced recurrence-free survival, reduced overall survival, enhanced tumor size and increased HBsAg (38, 39). hDREH expression was inversely associated with HBx concentration in human HBV-related HCC tissues and significantly downregulated in HBx-expressing human hepatoma cells (39). Subsequent investigations revealed that hDREH inhibition of expression enhances cell proliferation in vitro and tumor growth in vivo. These observations further support a role for hDREH as a tumor suppressor in HBV-related HCC.
3.4.2 SAMD12-AS1
SAMD12-AS1 is an antisense lncRNA that is approximately 700 nucleotides long and shares the same locus as SAMD12 (40). This lncRNA is upregulated in HBV-positive HCC tissues and HBx-expressing cells and its transcription is enhanced by HBx. SAMD12-AS1 enhances proliferation and inhibits apoptosis, as indicated by its effect to reduce both caspase-3/7 activity and PARP-1 cleavage. The transcript primarily interacts with the nucleophosmin (NPM1) protein in the nucleus. NPM1 interacts with the E3 ubiquitin ligase HDM2 and prevents the degradation of p53 (97). Subsequent investigations revealed that p53 was rapidly degraded in SAMD12-AS1-expressing cells through increased ubiquitination of p53 by HDM2 (40). These results demonstrate that SAMD12-AS1 competitively binds to NPM1 to prevent its interaction with HDM2. This increases availability of HDM2 to mark p53 for proteasomal degradation, which ultimately promotes cell proliferation and inhibits apoptosis.
3.4.3 HUR1
The HUR1 gene transcribes the lncRNA HUR1, which is approximately 1,200 nucleotides in length (41). HUR1 upregulation was positively associated with HBV viral load in patients with HBV-related HCC. Its increased expression was also observed in HBx-expressing HepG2 cells, which indicated that HUR1 is upregulated by HBx. In HUR1-expressing HepG2 cells, HUR1 enhanced proliferation, as evidenced by the increased PCNA expression, and promoted G1/S transition. Enhanced proliferation was also observed in nude mice that were injected with these cells. Additional in vitro studies revealed that HUR1 enhances proliferation by interacting with the tumor suppressor p53 and inhibiting its transcriptional regulation of p21 and Bax. Furthermore, HUR1 promoted cell proliferation and liver regeneration in HUR1 transgenic mice that underwent partial hepatectomy, and promoted tumor progression in DEN-induced HCC mouse models.
3.4.4 MVIH
LncRNA associated with microvascular invasion in HCC (MVIH) is found within the intron of the RPS24 gene, however MVIH and RPS24 are independently transcribed (42). This lncRNA is highly upregulated in HBV-related HCC and is associated with greater microvascular invasion and advanced tumor node metastasis T stage. It is also an independent predictor of recurrence-free survival after hepatectomy. When examined in vivo, MVIH overexpression promoted tumor growth and intrahepatic metastasis. MVIH interacts directly with the angiogenesis inhibitor phosphoglycerate kinase (PGK1) and that there is a negative correlation between the two. This negative association was also observed in patient samples. Patients with greater MVIH expression in their tumor tissues showed reduced PGK1 serum levels. These results indicate that MVIH induces tumor angiogenesis by inhibiting the secretion of PGK1, thus promoting tumor growth and intrahepatic metastasis.
3.4.5 LncRNA-6195
LncRNA-6195 is significantly downregulated in HBV-related HCC tissues from patients (43). Its downregulation was correlated with HBx and decreased overall survival. LncRNA-6195 inhibited tumor growth in vitro and in vivo. RNA immunoprecipitation analysis revealed that lncRNA-6195 bound the substrate-binding site of ENO1 (aa 237-405). In lncRNA-6195-expressing hepatoma cells, lncRNA-6195 had no effect on ENO1 expression, but reduced ENO1 enzyme activity (43). The results demonstrate that lncRNA-6195 is a tumor suppressor which inhibits tumor growth by binding to the substrate region of ENO1. Resultant inhibition of enzyme activity represses energy metabolism in hepatoma cells.
3.5 miRNA Precursors
A lesser employed mechanism of post-transcriptional gene regulation is mRNA suppression by miRNA-encoding lncRNAs. These lncRNAs are transcribed as precursors of miRNAs and contain clusters of miRNAs (Figure 6). Subsequent downregulation of their target mRNAs may promote development of HCC.
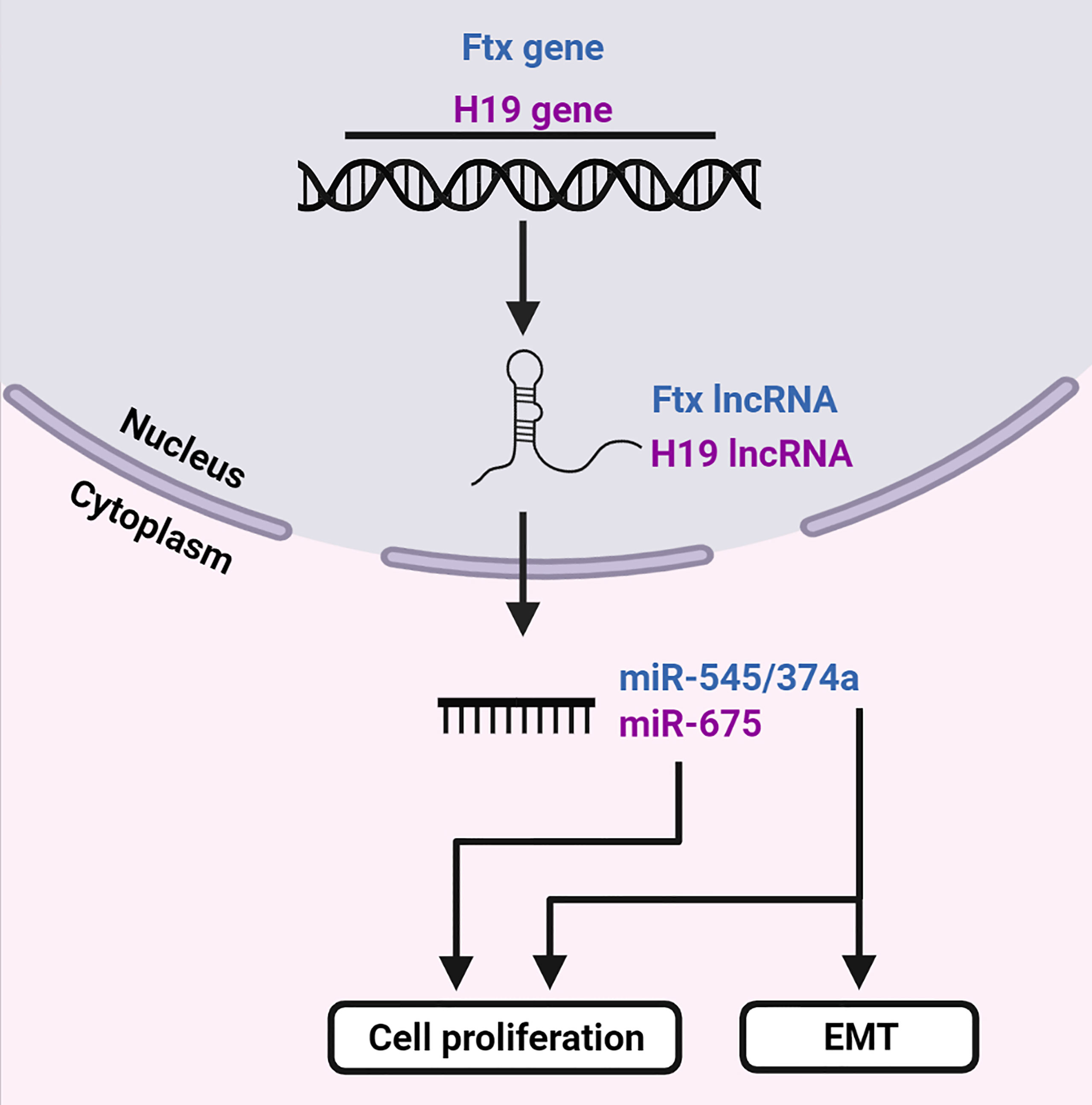
Figure 6 LncRNAs are precursors of miRNAs. The Ftx and H19 genes transcribe lncRNAs Ftx and H19, respectively. LncRNA Ftx encodes the miR-545/374a cluster, which subsequently promotes EMT and cell proliferation. LncRNA H19 transcribes miR-675 which enhances cell proliferation. Created with BioRender.com.
3.5.1 Ftx
The Ftx gene is located within the X-inactivation center of the X chromosome (98). It transcribes a 2,300 nucleotide long highly conserved lncRNA, which encodes four miRNAs in its introns. The miR-545/374a and miR-421/374b clusters are encoded by intron b and intron 12, respectively [reviewed in (99)]. In a study performed by Zhao et al., the miR-545/374a cluster was highly expressed in HBV-related HCC and was positively associated with histological differentiation and incomplete tumor capsule formation, whereas the upregulation of miR-374a was observed in patients with distal metastasis (45). When investigated in vitro, miR-545 and miR-374a promoted cell proliferation, migration and invasion which suggests a possible role in tumorigenesis. In HBV-related HCC patient tissue samples, expression of HBx correlated significantly with miR-545. In patients that were positive for HBeAg, miR-374a was highly expressed, whereas in patients that were positive for HBV DNA, both miRNAs were expressed. Furthermore, miR-545 was greatly increased in the HBV-positive cell lines and both miRNAs were significantly increased in cells transfected with HBV DNA. This positive association between the miR-545/miR-374a and HBV infection indicates that its expression may be dependent on HBx. Zhao et al. then revealed that expression of miR-545 and miR-374a in the HBV-related HCC tissues correlated with concentrations of the miRNAs in patient serum samples. After tumor resection, these concentrations decreased significantly, suggesting that the miRNAs are tumor-derived. Levels of miR-545 and miR-374a were also increased in the serum of patients with alpha-fetoprotein levels ≥20 ng/ml. Therefore, the detection of these miRNAs in the serum of patients infected with HBV may be useful as a marker of HCC. The levels of miR-545/374a were also significantly higher in HBV-related HCC from males compared to females (45). Low concentrations in females was hypothesized to be related to the estrogen-related gene ESRRG, which is a potential target of miR-545. In a study performed by Liu et al., where the majority of the patients with HCC were positive for HBsAg, Ftx and miR-545 were significantly upregulated in patient tumor tissues (44). This was associated with tumor size, high histological grade, advanced tumor stage, shorter overall survival and decreased disease-free survival, whereas increased expression of miR-545 alone was associated with venous infiltration.
3.5.2 H19
In addition to its previously mentioned functions, H19 was also identified as a precursor of miR-675 (100). The expression of H19 and miR-675 was significantly upregulated in the tissues of patients with chronic HBV and HBx-transfected LO2 cells. Inhibition of either of these transcripts within the HBx-transfected LO2 cells enhanced cell viability, suppressed HBx-induced apoptosis, inflammatory cytokine production, oxidative stress, and modulated energy metabolism. However, these effects were partially reversed by knocking down PPARα (40). PPARα was identified as a direct target of miR-675 and was downregulated in the tissue of patients with chronic HBV. PPARα expression was reduced by overexpression of HBx in LO2 cells. This suppression was (partially) reversed by inhibition of H19 or miR-675. These findings suggest that the overexpression of HBx induces H19 and miR-675 upregulation, leading to the inhibition of PPARα, which then promotes Akt/mTOR signaling.
3.6 LncRNAs With Unknown Mechanisms in HBV-Related HCC
The mechanisms of action of many lncRNAs dysregulated in HBV-related HCC remain largely unknown. Some have had their functions examined in other types of cancers. However, these lncRNAs may elicit other effects in HBV-related HCC. The proposed functions and targets of recently discovered lncRNAs have been predicted using in silico tools. A few of these lncRNAs are mentioned below.
The lncRNA TUC338 is upregulated in HBV-infected primary human hepatocytes as well as HCC cell lines and tissues, and has been shown to modulate expression of regulatory proteins involved in cell-cycle progression (12, 101). TUC338 associates with chromatin at multiple DNA motifs, the most enriched being those that are homologous to the binding sites of the tumor suppressors p53 and Pax6 (102). Thus, upregulation of TUC338 could potentially competitively inhibit p53 and Pax6, and subsequently suppress their downstream target genes. TUC338 also post-transcriptionally stabilizes mRNA encoding the Plasminogen Activator Inhibitor (PAI) protein, which is upregulated in HCC and contributes to transformed cell growth. This interaction is not sequence-specific, but rather mediated by the PAI-1 RNA binding protein (PAI-RBP1) which is also positively regulated by TUC338. This mechanism of tumorigenesis has not yet been confirmed in HBV-related HCC.
As with many aforementioned lincRNAs (such as HEIH, UCA1 and linc00152), ULK4P2 associates with EZH2, but the exact gene target of ULK4P2 has not been elucidated (103). Analysis of a ULK4P2-mRNA co-expression network has pointed to its potential involvement in cell proliferation and metastasis. It is possible that ULK4P2 may mediate changes to chromatin architecture to enable transcription of genes involved in promoting cell proliferation and EMT. ANRIL was initially not correlated with HBV in HCC tissues (104, 105). However, this was contradicted in a more recent study, as ANRIL expression was significantly increased in patients with HBV-related HCC (15). The discrepancy may be attributed to differences in sample size between studies. The former compared 103 and 67 HBV-positive patients against 34 and 10 negative cases, while the latter compared 11 HBV-positive HCC cases against 52 negative cases.
LncRNAs PRC1-AS1, LINC00665 and AC092171.4 are highly upregulated in HBV-infected HCC cell lines (106). AF085935 and uc003wbd are significantly expressed in the serum of HBV-infected HCC patients suggesting utility as potential biomarkers for detection of the disease (107). LncRNAs AX800134 and uc001ncr are significantly upregulated in the serum and tumor tissue of patients with HBV-related HCC, including those with alpha-fetoprotein <400 ng/ml or early-stage disease (BCLC 0+A) (108, 109). Thus, these lncRNAs may be potential biomarkers for early diagnosis of the disease. AX800134 was upregulated in HepG2 cells which express HBx (109). Elevated levels of the pro-inflammatory cytokine TNF-α induced NF-κB signaling, which upregulated AX800134 in these cells. This upregulation was reversed by the reactive oxygen species scavenger, PDTC. Silencing of AX800134 did not decrease expression of the HBx protein but significantly inhibited cell growth rate and invasion, and enhanced apoptosis in serum-starved cells. This suggests that the presence of HBx and chronic inflammation may induce expression of AX800134, which in turn promotes hepatoma cell growth and invasion.
The Gene Expression Omnibus database was used to identify BAIAP2-AS1 as a lncRNA that was significantly upregulated in HBV-related HCC (110). Gene set enrichment analysis showed that transcripts encoding MAPKAP1, RAF1 and E2F3 were enriched with BAIAP2-AS1 expression. In silico sequence analysis of BAIAP2-AS1 identified binding sites for miR-491, miR-331 and miR-34A. These miRNAs target MAPKAP1, RAF1 and E2F3 respectively. Knockdown of BAIAP2-AS1 in HepG2 cells decreased MAPKAP1 and RAF1 expression, which suggests that BAIAP2-AS1 may function as a ceRNA.
RNA-sequencing data from The Cancer Genome Atlas (TGCA) database and microarray datasets from the European Bioinformatics Institute database revealed eight lncRNAs (TSPEARAS1, LINC00511, LINC01136, MKLN1-AS, LINC00506, KRTAP5-AS1, ZNF252P-AS1 and THUMPD3-AS1) that may be a collective prognostic biomarker for HBV-infected patients with HCC, as they were significantly correlated with overall survival (111). Further investigation into the mechanisms of these lncRNAs revealed that they may be involved in tumorigenesis by regulating cell cycle-related processes or pathways. Similarly, in another study which analyzed lncRNA expression using bioinformatics, seven lncRNAs (PVT1, LINC01138, LINC02499, AL355488.2, FGF14-AS2, MAFG-AS1 and LINC00261) were significantly associated with overall survival and collectively were identified as a prognostic signature for patients with HBV-related HCC (112).
MSC-AS1, POLR2J4, EIF3J-AS1, SERHL, RMST, and PVT1 were proposed to be a six-lncRNA prediction marker for HBV-HCC recurrence-free survival. This was determined by univariate COX regression analysis and validated in the TCGA database (113). Another study which utilized bioinformatics analysis revealed that FAM182B-miR-125b-5p-E2F2 and LINC00346-miR-10a-5p-CDK1/CCNE1 ceRNA axes enhanced tumorigenesis by promoting HBV-related HCC cell growth, thus making these lncRNAs attractive therapeutic targets and potential disease predictors (95). Deep sequencing analysis identified significant downregulation of n346077 expression in HBV-positive HCC tissues. Further in vitro investigations in HepG2 and QGY-7703 cell lines revealed that n346077 may act as a tumor suppressor by inhibiting hepatoma cell invasion and migration (114).
4 Conclusion
Over the past few years, there has been an unprecedented increase in the identification of dysregulated lncRNAs in HBV-related HCC. These lncRNAs are quite heterogenous in size, type, structure, cellular location, and functionality. Through an intricate network of interactions, involving epigenetic modifications to chromatin, modulation of transcription factors, sponging of miRNAs, protein interactions and miRNA production, these lncRNAs work in concert to promote tumorigenesis and cancer progression, while also modulating HBV replication. Some of these lncRNAs are common to many other types of cancers, however, the manner in which an individual lncRNA functions sometimes varies across cancers. Thus, the mechanisms described in this review are limited to those which have been confirmed in HBV-related HCC. Other reported mechanisms of lncRNA action may well be employed in HBV-related HCC, but this remains to be established. Not surprisingly, novel lncRNAs aberrantly expressed in HBV-related HCC tissues continue to be discovered. Future studies will undoubtedly shed more insight into their roles in promoting HBV-related HCC. LncRNAs may be useful as diagnostic or prognostic markers and could be considered as potential targets for the treatment of HBV-related HCC.
Author Contributions
CS and NS contributed equally to this work by writing the review article and creating the figures. AE, KB, and PA reviewed and edited the manuscript. All authors approved the submitted version.
Funding
Financial support from the South African National Research Foundation (Unique Grant Numbers: 118022 and 120383), The Poliomyelitis Research Foundation, and extramural unit baseline funding from the South African Medical Research Council is gratefully acknowledged.
Conflict of Interest
The authors declare that the research was conducted in the absence of any commercial or financial relationships that could be construed as a potential conflict of interest.
Publisher’s Note
All claims expressed in this article are solely those of the authors and do not necessarily represent those of their affiliated organizations, or those of the publisher, the editors and the reviewers. Any product that may be evaluated in this article, or claim that may be made by its manufacturer, is not guaranteed or endorsed by the publisher.
References
1. Tsagakis I, Douka K, Birds I, Aspden JL. Long Non-Coding RNAs in Development and Disease: Conservation to Mechanisms. J Pathol (2020) 250:480–95. doi: 10.1002/path.5405
2. Gil N, Ulitsky I. Regulation of Gene Expression by Cis-Acting Long non-Coding RNAs. Nat Rev Genet (2020) 21:102–17. doi: 10.1038/s41576-019-0184-5
3. Alfano V, Zeisel MB, Levrero M, Guerrieri F. The lncRNAs in HBV-Related HCCs: Targeting Chromatin Dynamics and Beyond. Cancers (2021) 13:3115. doi: 10.3390/cancers13133115
4. Moyo B, Nicholson SA, Arbuthnot PB. The Role of Long Non-Coding RNAs in Hepatitis B Virus-Related Hepatocellular Carcinoma. Virus Res (2016) 212:103–13. doi: 10.1016/j.virusres.2015.07.025
5. Zhang R, Xia LQ, Lu WW, Zhang J, Zhu J-S. LncRNAs and Cancer. Oncol Lett (2016) 12:1233–9. doi: 10.3892/ol.2016.4770
6. Chisari FV, Ferrari C. Hepatitis B Virus Immunopathogenesis. Annu Rev Immunol (1995) 13:29–60. doi: 10.1146/annurev.iy.13.040195.000333
7. Yang F, Zhang L, Huo X, Yuan J, Xu D, Yuan S, et al. Long Noncoding RNA High Expression in Hepatocellular Carcinoma Facilitates Tumor Growth Through Enhancer of Zeste Homolog 2 in Humans. Hepatol Baltim Md (2011) 54:1679–89. doi: 10.1002/hep.24563
8. Hu J-J, Song W, Zhang S-D, Shen X-H, Qiu X-M, Wu H-Z, et al. HBx-Upregulated lncRNA UCA1 Promotes Cell Growth and Tumorigenesis by Recruiting EZH2 and Repressing p27Kip1/CDK2 Signaling. Sci Rep (2016) 6:23521. doi: 10.1038/srep23521
9. Deng X, Zhao XF, Liang XQ, Chen R, Pan YF, Liang J. Linc00152 Promotes Cancer Progression in Hepatitis B Virus-Associated Hepatocellular Carcinoma. Biomed Pharmacother (2017) 90:100–8. doi: 10.1016/j.biopha.2017.03.031
10. Ji J, Tang J, Deng L, Xie Y, Jiang R, Li G, et al. LINC00152 Promotes Proliferation in Hepatocellular Carcinoma by Targeting EpCAM via the mTOR Signaling Pathway. Oncotarget (2015) 6:42813–24. doi: 10.18632/oncotarget.5970
11. Jiang B, Yang B, Wang Q, Zheng X, Guo Y, Lu W. lncRNA PVT1 Promotes Hepatitis B Virus−Positive Liver Cancer Progression by Disturbing Histone Methylation on the C−Myc Promoter. Oncol Rep (2019) 43:718–26. doi: 10.3892/or.2019.7444
12. Salerno D, Chiodo L, Alfano V, Floriot O, Cottone G, Paturel A, et al. Hepatitis B Protein HBx Binds the DLEU2 lncRNA to Sustain cccDNA and Host Cancer-Related Gene Transcription. Gut (2020) 69:2016–24. doi: 10.1136/gutjnl-2019-319637
13. Zhao X, Fan H, Chen X, Zhao X, Wang X, Feng Y, et al. Hepatitis B Virus DNA Polymerase Restrains Viral Replication Through the CREB1/HOXA Distal Transcript Antisense RNA Homeobox A13 Axis. Hepatology (2021) 73:503–19. doi: 10.1002/hep.31284
14. Zhang H, Diab A, Fan H, Mani SKK, Hullinger R, Merle P, et al. PLK1 and HOTAIR Accelerate Proteasomal Degradation of SUZ12 and ZNF198 During Hepatitis B Virus-Induced Liver Carcinogenesis. Cancer Res (2015) 75:2363–74. doi: 10.1158/0008-5472.CAN-14-2928
15. Zhang H, Xing Z, Mani SKK, Bancel B, Durantel D, Zoulim F, et al. RNA Helicase DEAD Box Protein 5 Regulates Polycomb Repressive Complex 2/Hox Transcript Antisense Intergenic RNA Function in Hepatitis B Virus Infection and Hepatocarcinogenesis. Hepatol Baltim Md (2016) 64:1033–48. doi: 10.1002/hep.28698
16. Ren F, Ren J-H, Song C-L, Tan M, Yu H-B, Zhou Y-J, et al. LncRNA HOTAIR Modulates Hepatitis B Virus Transcription and Replication by Enhancing SP1 Transcription Factor. Clin Sci (2020) 134:3007–22. doi: 10.1042/CS20200970
17. Liu Y, Feng J, Sun M, Yang G, Yuan H, Wang Y, et al. Long Non-Coding RNA HULC Activates HBV by Modulating HBx/STAT3/miR-539/APOBEC3B Signaling in HBV-Related Hepatocellular Carcinoma. Cancer Lett (2019) 454:158–70. doi: 10.1016/j.canlet.2019.04.008
18. Du Y, Kong G, You X, Zhang S, Zhang T, Gao Y, et al. Elevation of Highly Up-Regulated in Liver Cancer (HULC) by Hepatitis B Virus X Protein Promotes Hepatoma Cell Proliferation via Down-Regulating P18. J Biol Chem (2012) 287:26302–11. doi: 10.1074/jbc.M112.342113
19. Wang J, Liu X, Wu H, Ni P, Gu Z, Qiao Y, et al. CREB Up-Regulates Long non-Coding RNA, HULC Expression Through Interaction With microRNA-372 in Liver Cancer. Nucleic Acids Res (2010) 38:5366–83. doi: 10.1093/nar/gkq285
20. Li B, Chen P, Qu J, Shi L, Zhuang W, Fu J, et al. Activation of LTBP3 Gene by a Long Noncoding RNA (lncRNA) MALAT1 Transcript in Mesenchymal Stem Cells From Multiple Myeloma. J Biol Chem (2014) 289:29365–75. doi: 10.1074/jbc.M114.572693
21. Xia L, Tian D, Huang W, Zhu H, Wang J, Zhang Y, et al. Upregulation of IL-23 Expression in Patients With Chronic Hepatitis B Is Mediated by the HBx/ERK/NF-κb Pathway. J Immunol (2012) 188:753–64. doi: 10.4049/jimmunol.1101652
22. Chen T, Pei J, Wang J, Luo R, Liu L, Wang L, et al. HBx-Related Long Non-Coding RNA 01152 Promotes Cell Proliferation and Survival by IL-23 in Hepatocellular Carcinoma. Biomed Pharmacother (2019) 115:108877. doi: 10.1016/j.biopha.2019.108877
23. Jin Y, Wu D, Yang W, Weng M, Li Y, Wang X, et al. Hepatitis B Virus X Protein Induces Epithelial-Mesenchymal Transition of Hepatocellular Carcinoma Cells by Regulating Long Non-Coding RNA. Virol J (2017) 14:238. doi: 10.1186/s12985-017-0903-5
24. Lau C-C, Sun T, Ching AKK, He M, Li J-W, Wong AM, et al. Viral-Human Chimeric Transcript Predisposes Risk to Liver Cancer Development and Progression. Cancer Cell (2014) 25:335–49. doi: 10.1016/j.ccr.2014.01.030
25. Liang H-W, Wang N, Wang Y, Wang F, Fu Z, Yan X, et al. Hepatitis B Virus-Human Chimeric Transcript HBx-LINE1 Promotes Hepatic Injury via Sequestering Cellular microRNA-122. J Hepatol (2016) 64:278–91. doi: 10.1016/j.jhep.2015.09.013
26. Huang J, Ren T, Cao S, Zheng S, Hu X, Hu Y, et al. HBx-Related Long Non-Coding RNA DBH-AS1 Promotes Cell Proliferation and Survival by Activating MAPK Signaling in Hepatocellular Carcinoma. Oncotarget (2015) 6:33791–804. doi: 10.18632/oncotarget.5667
27. Bao J, Chen X, Hou Y, Kang G, Li Q, Xu Y. LncRNA DBH-AS1 Facilitates the Tumorigenesis of Hepatocellular Carcinoma by Targeting miR-138 via FAK/Src/ERK Pathway. Biomed Pharmacother (2018) 107:824–33. doi: 10.1016/j.biopha.2018.08.079
28. Feng J, Yang G, Liu Y, Gao Y, Zhao M, Bu Y, et al. LncRNA PCNAP1 Modulates Hepatitis B Virus Replication and Enhances Tumor Growth of Liver Cancer. Theranostics (2019) 9:5227–45. doi: 10.7150/thno.34273
29. He M, Hu L, Bai P, Guo T, Liu N, Feng F, et al. LncRNA PCNAP1 Promotes Hepatoma Cell Proliferation Through Targeting miR-340-5p and Is Associated With Patient Survival. J Oncol (2021) 2021:e6627173. doi: 10.1155/2021/6627173
30. Hu Z, Huang P, Yan Y, Zhou Z, Wang J, Wu G. Hepatitis B Virus X Protein Related lncRNA WEE2-AS1 Promotes Hepatocellular Carcinoma Proliferation and Invasion. Biochem Biophys Res Commun (2019) 508:79–86. doi: 10.1016/j.bbrc.2018.11.091
31. Lin H, Zuo D, He J, Ji T, Wang J, Jiang T. Long Noncoding RNA WEE2-AS1 Plays an Oncogenic Role in Glioblastoma by Functioning as a Molecular Sponge for MicroRNA-520f-3p. Oncol Res (2021) 28:591–603. doi: 10.3727/096504020X15982623243955
32. Lv J, Fan H-X, Zhao X-P, Lv P, Fan J-Y, Zhang Y, et al. Long Non-Coding RNA Unigene56159 Promotes Epithelial-Mesenchymal Transition by Acting as a ceRNA of miR-140-5p in Hepatocellular Carcinoma Cells. Cancer Lett (2016) 382:166–75. doi: 10.1016/j.canlet.2016.08.029
33. Villarejo A, Cortés-Cabrera Á., Molina-Ortíz P, Portillo F, Cano A. Differential Role of Snail1 and Snail2 Zinc Fingers in E-Cadherin Repression and Epithelial to Mesenchymal Transition*. J Biol Chem (2014) 289:930–41. doi: 10.1074/jbc.M113.528026
34. Li L, Han T, Liu K, Lei C-G, Wang Z-C, Shi G-J. LncRNA H19 Promotes the Development of Hepatitis B Related Hepatocellular Carcinoma Through Regulating microRNA-22 via EMT Pathway. Eur Rev Med Pharmacol Sci (2019) 23:5392–401. doi: 10.26355/eurrev_201906_18208
35. Liu Y, Xu L, Lu B, Zhao M, Li L, Sun W, et al. LncRNA H19/microRNA-675/Pparα Axis Regulates Liver Cell Injury and Energy Metabolism Remodelling Induced by Hepatitis B X Protein via Akt/mTOR Signalling. Mol Immunol (2019) 116:18–28. doi: 10.1016/j.molimm.2019.09.006
36. Zhang L, Yang F, Yuan J, Yuan S, Zhou W, Huo X, et al. Epigenetic Activation of the MiR-200 Family Contributes to H19-Mediated Metastasis Suppression in Hepatocellular Carcinoma. Carcinogenesis (2013) 34:577–86. doi: 10.1093/carcin/bgs381
37. Fan H, Lv P, Mu T, Zhao X, Liu Y, Feng Y, et al. LncRNA N335586/miR-924/CKMT1A Axis Contributes to Cell Migration and Invasion in Hepatocellular Carcinoma Cells. Cancer Lett (2018) 429:89–99. doi: 10.1016/j.canlet.2018.05.010
38. Huang J, Guo Y, Zhao C, Yuan S, Wang Y, Tang G, et al. Hepatitis B Virus X Protein (HBx)-Related Long Noncoding RNA (lncRNA) Down-Regulated Expression by HBx (Dreh) Inhibits Hepatocellular Carcinoma Metastasis by Targeting the Intermediate Filament Protein Vimentin. Hepatol Baltim Md (2013) 57:1882–92. doi: 10.1002/hep.26195
39. Lv D, Wang Y, Zhang Y, Cui P, Xu Y. Downregulated Long non-Coding RNA DREH Promotes Cell Proliferation in Hepatitis B Virus-Associated Hepatocellular Carcinoma. Oncol Lett (2017) 14:2025–32. doi: 10.3892/ol.2017.6436
40. Liu Q, Liu N, Shangguan Q, Zhang F, Chai W, Tong X, et al. LncRNA SAMD12-AS1 Promotes Cell Proliferation and Inhibits Apoptosis by Interacting With NPM1. Sci Rep (2019) 9:11593. doi: 10.1038/s41598-019-48116-1
41. Liu N, Liu Q, Yang X, Zhang F, Li X, Ma Y, et al. Hepatitis B Virus-Upregulated LNC-HUR1 Promotes Cell Proliferation and Tumorigenesis by Blocking P53 Activity. Hepatol Baltim Md (2018) 68:2130–44. doi: 10.1002/hep.30098
42. Yuan S-X, Yang F, Yang Y, Tao Q-F, Zhang J, Huang G, et al. Long Noncoding RNA Associated With Microvascular Invasion in Hepatocellular Carcinoma Promotes Angiogenesis and Serves as a Predictor for Hepatocellular Carcinoma Patients’ Poor Recurrence-Free Survival After Hepatectomy. Hepatol Baltim Md (2012) 56:2231–41. doi: 10.1002/hep.25895
43. Yu S, Li N, Huang Z, Chen R, Yi P, Kang R, et al. A Novel lncRNA, TCONS_00006195, Represses Hepatocellular Carcinoma Progression by Inhibiting Enzymatic Activity of ENO1. Cell Death Dis (2018) 9:1–13. doi: 10.1038/s41419-018-1231-4
44. Liu Z, Dou C, Yao B, Xu M, Ding L, Wang Y, et al. Ftx Non Coding RNA-Derived miR-545 Promotes Cell Proliferation by Targeting RIG-I in Hepatocellular Carcinoma. Oncotarget (2016) 7:25350–65. doi: 10.18632/oncotarget.8129
45. Zhao Q, Li T, Qi J, Liu J, Qin C. The miR-545/374a Cluster Encoded in the Ftx lncRNA Is Overexpressed in HBV-Related Hepatocellular Carcinoma and Promotes Tumorigenesis and Tumor Progression. PloS One (2014) 9:e109782. doi: 10.1371/journal.pone.0109782
46. Susluer S, Kayabasi C, Ozmen Yelken B, Asik A, Celik D, Balci Okcanoglu T, et al. Analysis of Long Non-Coding RNA (lncRNA) Expression in Hepatitis B Patients. Bosn J Basic Med Sci (2018) 18:150–61. doi: 10.17305/bjbms.2018.2800
47. Zhang Q, Matsuura K, Kleiner DE, Zamboni F, Alter HJ, Farci P. Analysis of Long Noncoding RNA Expression in Hepatocellular Carcinoma of Different Viral Etiology. J Transl Med (2016) 14:328. doi: 10.1186/s12967-016-1085-4
48. Liu L, He C, Liu H, Wang G, Lv Z, Ni Y, et al. Transcriptomic Profiling of Long Non-Coding RNAs in Non-Virus Associated Hepatocellular Carcinoma. Cell Biochem Biophys (2020) 78:465–74. doi: 10.1007/s12013-020-00915-4
49. Yau MY-C, Xu L, Huang C-L, Wong C-M. Long Non-Coding RNAs in Obesity-Induced Cancer. Non-Coding RNA (2018) 4:19. doi: 10.3390/ncrna4030019
50. Shabgah AG, Norouzi F, Hedayati-Moghadam M, Soleimani D, Pahlavani N, Navashenaq JG. A Comprehensive Review of Long Non-Coding RNAs in the Pathogenesis and Development of non-Alcoholic Fatty Liver Disease. Nutr Metab (2021) 18:22. doi: 10.1186/s12986-021-00552-5
51. Zhang H, Chen X, Zhang J, Wang X, Chen H, Liu L, et al. Long Non−Coding RNAs in HBV−Related Hepatocellular Carcinoma (Review). Int J Oncol (2019) 56:18–32. doi: 10.3892/ijo.2019.4909
52. Moran VA, Perera RJ, Khalil AM. Emerging Functional and Mechanistic Paradigms of Mammalian Long non-Coding RNAs. Nucleic Acids Res (2012) 40:6391–400. doi: 10.1093/nar/gks296
53. Wang KC, Chang HY. Molecular Mechanisms of Long Noncoding RNAs. Mol Cell (2011) 43:904–14. doi: 10.1016/j.molcel.2011.08.018
54. Khalil AM, Guttman M, Huarte M, Garber M, Raj A, Rivea Morales D, et al. Many Human Large Intergenic Noncoding RNAs Associate With Chromatin-Modifying Complexes and Affect Gene Expression. Proc Natl Acad Sci USA (2009) 106:11667–72. doi: 10.1073/pnas.0904715106
55. Shi Y, Wang X, Zhuang Y, Jiang Y, Melcher K, Xu HE. Structure of the PRC2 Complex and Application to Drug Discovery. Acta Pharmacol Sin (2017) 38:963–76. doi: 10.1038/aps.2017.7
56. Bracken AP, Dietrich N, Pasini D, Hansen KH, Helin K. Genome-Wide Mapping of Polycomb Target Genes Unravels Their Roles in Cell Fate Transitions. Genes Dev (2006) 20:1123–36. doi: 10.1101/gad.381706
57. Ruan L, Huang L, Zhao L, Wang Q, Pan X, Zhang A, et al. The Interaction of lncRNA-HEIH and lncRNA-HULC With HBXIP in Hepatitis B Patients. Gastroenterol Res Pract (2018) 2018:1–6. doi: 10.1155/2018/9187316
58. Wang X-S, Zhang Z, Wang H-C, Cai J-L, Xu Q-W, Li M-Q, et al. Rapid Identification of UCA1 as a Very Sensitive and Specific Unique Marker for Human Bladder Carcinoma. Clin Cancer Res (2006) 12:4851–8. doi: 10.1158/1078-0432.CCR-06-0134
59. Loh C-Y, Chai JY, Tang TF, Wong WF, Sethi G, Shanmugam MK, et al. The E-Cadherin and N-Cadherin Switch in Epithelial-to-Mesenchymal Transition: Signaling, Therapeutic Implications, and Challenges. Cells (2019) 8:E1118. doi: 10.3390/cells8101118
60. Yamashita T, Ji J, Budhu A, Forgues M, Yang W, Wang H-Y, et al. EpCAM-Positive Hepatocellular Carcinoma Cells Are Tumor-Initiating Cells With Stem/Progenitor Cell Features. Gastroenterology (2009) 136:1012–24. doi: 10.1053/j.gastro.2008.12.004
61. Wang F, Yuan J-H, Wang S-B, Yang F, Yuan S-X, Ye C, et al. Oncofetal Long Noncoding RNA PVT1 Promotes Proliferation and Stem Cell-Like Property of Hepatocellular Carcinoma Cells by Stabilizing NOP2: WANG ET AL. Hepatology (2014) 60:1278–90. doi: 10.1002/hep.27239
62. Corcoran MM, Hammarsund M, Zhu C, Lerner M, Kapanadze B, Wilson B, et al. DLEU2 Encodes an Antisense RNA for the Putative Bicistronic RFP2/LEU5 Gene in Humans and Mouse: Antisense Relationship Between DLEU2 and RFP2/LEU5. Genes. Chromosomes Cancer (2004) 40:285–97. doi: 10.1002/gcc.20046
63. Klein U, Lia M, Crespo M, Siegel R, Shen Q, Mo T, et al. The DLEU2/miR-15a/16-1 Cluster Controls B Cell Proliferation and Its Deletion Leads to Chronic Lymphocytic Leukemia. Cancer Cell (2010) 17:28–40. doi: 10.1016/j.ccr.2009.11.019
64. Wang KC, Yang YW, Liu B, Sanyal A, Corces-Zimmerman R, Chen Y, et al. A Long Noncoding RNA Maintains Active Chromatin to Coordinate Homeotic Gene Expression. Nature (2011) 472:120–4. doi: 10.1038/nature09819
65. Zhao F, Xie X, Tan X, Yu H, Tian M, Lv H, et al. The Functions of Hepatitis B Virus Encoding Proteins: Viral Persistence and Liver Pathogenesis. Front Immunol (2021) 12:691766:691766. doi: 10.3389/fimmu.2021.691766
66. Liu Q-M, He Y-Y, Liu L-L, Wang L-K. Exosomal lncRNA HOTTIP Mediates Antiviral Effect of Tenofovir Alafenamide (TAF) on HBV Infection. J Inflamm Res Volume (2021) 14:5489–500. doi: 10.2147/JIR.S315716
67. Quagliata L, Matter MS, Piscuoglio S, Arabi L, Ruiz C, Procino A, et al. Long Noncoding RNA HOTTIP/HOXA13 Expression is Associated With Disease Progression and Predicts Outcome in Hepatocellular Carcinoma Patients. Hepatology (2014) 59:911–23. doi: 10.1002/hep.26740
68. Wu L, Yang Z, Zhang J, Xie H, Zhou L, Zheng S. Long Noncoding RNA HOTTIP Expression Predicts Tumor Recurrence in Hepatocellular Carcinoma Patients Following Liver Transplantation. Hepatobiliary Surg Nutr (2018) 7:429–39. doi: 10.21037/hbsn.2018.10.07
69. Rinn JL, Kertesz M, Wang JK, Squazzo SL, Xu X, Brugmann SA, et al. Functional Demarcation of Active and Silent Chromatin Domains in Human HOX Loci by Noncoding RNAs. Cell (2007) 129:1311–23. doi: 10.1016/j.cell.2007.05.022
70. Tsai M-C, Manor O, Wan Y, Mosammaparast N, Wang JK, Lan F, et al. Long Noncoding RNA as Modular Scaffold of Histone Modification Complexes. Science (2010) 329:689–93. doi: 10.1126/science.1192002
71. Gocke CB, Yu H. ZNF198 Stabilizes the LSD1-CoREST-HDAC1 Complex on Chromatin Through its MYM-Type Zinc Fingers. PloS One (2008) 3:e3255. doi: 10.1371/journal.pone.0003255
72. Moreau P, Cournac A, Palumbo GA, Marbouty M, Mortaza S, Thierry A, et al. Tridimensional Infiltration of DNA Viruses Into the Host Genome Shows Preferential Contact With Active Chromatin. Nat Commun (2018) 9:4268. doi: 10.1038/s41467-018-06739-4
73. Mani SKK, Yan B, Cui Z, Sun J, Utturkar S, Foca A, et al. Restoration of RNA Helicase DDX5 Suppresses Hepatitis B Virus (HBV) Biosynthesis and Wnt Signaling in HBV-Related Hepatocellular Carcinoma. Theranostics (2020) 10:10957–72. doi: 10.7150/thno.49629
74. Studach L, Wang W-H, Weber G, Tang J, Hullinger RL, Malbrue R, et al. Polo-Like Kinase 1 Activated by the Hepatitis B Virus X Protein Attenuates Both the DNA Damage Checkpoint and DNA Repair Resulting in Partial Polyploidy. J Biol Chem (2010) 285:30282–93. doi: 10.1074/jbc.M109.093963
75. Panzitt K, Tschernatsch MMO, Guelly C, Moustafa T, Stradner M, Strohmaier HM, et al. Characterization of HULC, a Novel Gene With Striking Up-Regulation in Hepatocellular Carcinoma, as Noncoding RNA. Gastroenterology (2007) 132:330–42. doi: 10.1053/j.gastro.2006.08.026
76. Williams JS, Andrisani OM. The Hepatitis B Virus X Protein Targets the Basic Region-Leucine Zipper Domain of CREB. Proc Natl Acad Sci USA (1995) 92:3819–23. doi: 10.1073/pnas.92.9.3819
77. Lucifora J, Xia Y, Reisinger F, Zhang K, Stadler D, Cheng X, et al. Specific and Nonhepatotoxic Degradation of Nuclear Hepatitis B Virus cccDNA. Science (2014) 343:1221–8. doi: 10.1126/science.1243462
78. Park B-J, Kang JW, Lee SW, Choi S-J, Shin YK, Ahn YH, et al. The Haploinsufficient Tumor Suppressor P18 Upregulates P53 via Interactions With ATM/ATR. Cell (2005) 120:209–21. doi: 10.1016/j.cell.2004.11.054
79. Hou Z, Xu X, Fu X, Tao S, Zhou J, Liu S, et al. HBx-Related Long non-Coding RNA MALAT1 Promotes Cell Metastasis via Up-Regulating LTBP3 in Hepatocellular Carcinoma. Am J Cancer Res (2017) 7:845–56.
80. Wilusz JE, Freier SM, Spector DL. 3’ End Processing of a Long Nuclear-Retained Noncoding RNA Yields a tRNA-Like Cytoplasmic RNA. Cell (2008) 135:919–32. doi: 10.1016/j.cell.2008.10.012
81. Xie S-J, Diao L-T, Cai N, Zhang L-T, Xiang S, Jia C-C, et al. mascRNA and its Parent lncRNA MALAT1 Promote Proliferation and Metastasis of Hepatocellular Carcinoma Cells by Activating ERK/MAPK Signaling Pathway. Cell Death Discovery (2021) 7:110. doi: 10.1038/s41420-021-00497-x
82. Miyazono K, Olofsson A, Colosetti P, Heldin CH. A Role of the Latent TGF-Beta 1-Binding Protein in the Assembly and Secretion of TGF-Beta 1. EMBO J (1991) 10:1091–101. doi: 10.1002/j.1460-2075.1991.tb08049.x
83. Deryugina EI, Zajac E, Zilberberg L, Muramatsu T, Joshi G, Dabovic B, et al. LTBP3 Promotes Early Metastatic Events During Cancer Cell Dissemination. Oncogene (2018) 37:1815–29. doi: 10.1038/s41388-017-0075-1
84. Lee SE, Alcedo KP, Kim HJ, Snider NT. Alternative Splicing in Hepatocellular Carcinoma. Cell Mol Gastroenterol Hepatol (2020) 10:699–712. doi: 10.1016/j.jcmgh.2020.04.018
85. Ouyang J, Zhong Y, Zhang Y, Yang L, Wu P, Hou X, et al. Long non-Coding RNAs are Involved in Alternative Splicing and Promote Cancer Progression. Br J Cancer (2021). doi: 10.1038/s41416-021-01600-w
86. Beltran M, Puig I, Peña C, García JM, Alvarez AB, Peña R, et al. A Natural Antisense Transcript Regulates Zeb2/Sip1 Gene Expression During Snail1-Induced Epithelial-Mesenchymal Transition. Genes Dev (2008) 22:756–69. doi: 10.1101/gad.455708
87. Comijn J, Berx G, Vermassen P, Verschueren K, van Grunsven L, Bruyneel E, et al. The Two-Handed E Box Binding Zinc Finger Protein SIP1 Downregulates E-Cadherin and Induces Invasion. Mol Cell (2001) 7:1267–78. doi: 10.1016/S1097-2765(01)00260-X
88. Leonardo S, Laura P, Yvonne T, Lev K, Pier Paolo P. A ceRNA Hypothesis: The Rosetta Stone of a Hidden RNA Language? Cell (2011) 146:353–8. doi: 10.1016/j.cell.2011.07.014
89. Ogden SK, Lee KC, Barton MC. Hepatitis B Viral Transactivator HBx Alleviates P53-Mediated Repression of α-Fetoprotein Gene Expression. J Biol Chem (2000) 275:27806–14. doi: 10.1074/jbc.M004449200
90. Stoimenov I, Lagerqvist A. The PCNA Pseudogenes in the Human Genome. BMC Res Notes (2012) 5:87. doi: 10.1186/1756-0500-5-87
91. Brannan CI, Dees EC, Ingram RS, Tilghman SM. The Product of the H19 Gene may Function as an RNA. Mol Cell Biol (1990) 10:28–36. doi: 10.1128/mcb.10.1.28-36.1990
92. Ariel I, Ayesh S, Perlman EJ, Pizov G, Tanos V, Schneider T, et al. The Product of the Imprinted H19 Gene is an Oncofetal RNA. Mol Pathol (1997) 50:34–44. doi: 10.1136/mp.50.1.34
93. Barsyte-Lovejoy D, Lau SK, Boutros PC, Khosravi F, Jurisica I, Andrulis IL, et al. The C-Myc Oncogene Directly Induces the H19 Noncoding RNA by Allele-Specific Binding to Potentiate Tumorigenesis. Cancer Res (2006) 66:5330–7. doi: 10.1158/0008-5472.CAN-06-0037
94. Ishiwata T. Expression and Role of Long non-Coding RNA H19 in Carcinogenesis. Front Biosci (2018) 23:614–25. doi: 10.2741/4608
95. Li H, Zhao X, Li C, Sheng C, Bai Z. Integrated Analysis of lncRNA-Associated ceRNA Network Reveals Potential Biomarkers for the Prognosis of Hepatitis B Virus-Related Hepatocellular Carcinoma. Cancer Manage Res (2019) 11:877–97. doi: 10.2147/CMAR.S186561
96. Smaldone MC, Davies BJ. BC-819, a Plasmid Comprising the H19 Gene Regulatory Sequences and Diphtheria Toxin A, for the Potential Targeted Therapy of Cancers. Curr Opin Mol Ther (2010) 12:607–16.
97. Kurki S, Peltonen K, Latonen L, Kiviharju TM, Ojala PM, Meek D, et al. Nucleolar Protein NPM Interacts With HDM2 and Protects Tumor Suppressor Protein P53 From HDM2-Mediated Degradation. Cancer Cell (2004) 5:465–75. doi: 10.1016/S1535-6108(04)00110-2
98. Chureau C, Prissette M, Bourdet A, Barbe V, Cattolico L, Jones L, et al. Comparative Sequence Analysis of the X-Inactivation Center Region in Mouse, Human, and Bovine. Genome Res (2002) 12:894–908. doi: 10.1101/gr.152902
99. Romito A, Rougeulle C. Origin and Evolution of the Long non-Coding Genes in the X-Inactivation Center. Biochimie Coding Non-coding: need they be exclusive? (2011) 93:1935–42. doi: 10.1016/j.biochi.2011.07.009
100. Cai X, Cullen BR. The Imprinted H19 Noncoding RNA is a Primary microRNA Precursor. RNA (2007) 13:313–6. doi: 10.1261/rna.351707
101. Braconi C, Valeri N, Kogure T, Gasparini P, Huang N, Nuovo GJ, et al. Expression and Functional Role of a Transcribed Noncoding RNA With an Ultraconserved Element in Hepatocellular Carcinoma. Proc Natl Acad Sci USA (2011) 108:786–91. doi: 10.1073/pnas.1011098108
102. Wen H-J, Walsh MP, Yan IK, Takahashi K, Fields A, Patel T. Functional Modulation of Gene Expression by Ultraconserved Long Non-Coding RNA TUC338 During Growth of Human Hepatocellular Carcinoma. iScience (2018) 2:210–20. doi: 10.1016/j.isci.2018.03.004
103. Yu T-T, Xu X-M, Hu Y, Deng J-J, Ge W, Han N-N, et al. Long Noncoding RNAs in Hepatitis B Virus-Related Hepatocellular Carcinoma. World J Gastroenterol (2015) 21:7208–17. doi: 10.3748/wjg.v21.i23.7208
104. Hua L, Wang C-Y, Yao K-H, Chen J-T, Zhang J-J, Ma W-L. High Expression of Long non-Coding RNA ANRIL is Associated With Poor Prognosis in Hepatocellular Carcinoma. Int J Clin Exp Pathol (2015) 8:3076–82.
105. Huang M, Chen W, Qi F, Xia R, Sun M, Xu T, et al. Long non-Coding RNA ANRIL is Upregulated in Hepatocellular Carcinoma and Regulates Cell Apoptosis by Epigenetic Silencing of KLF2. J Hematol Oncol.J Hematol Oncol (2015) 8:50. doi: 10.1186/s13045-015-0146-0
106. Xia J, Inagaki Y, Sawakami T, Song P, Cai Y, Hasegawa K, et al. Preliminary Investigation of Five Novel Long non-Coding RNAs in Hepatocellular Carcinoma Cell Lines. Biosci Trends (2016) 10:315–9. doi: 10.5582/bst.2016.01140
107. Lu J, Xie F, Geng L, Shen W, Sui C, Yang J. Investigation of Serum lncRNA-Uc003wbd and lncRNA-AF085935 Expression Profile in Patients With Hepatocellular Carcinoma and HBV. Tumour Biol J Int Soc Oncodevelopmental Biol Med (2015) 36:3231–6. doi: 10.1007/s13277-014-2951-4
108. Wang K, Guo WX, Li N, Gao CF, Shi J, Tang YF, et al. Serum LncRNAs Profiles Serve as Novel Potential Biomarkers for the Diagnosis of HBV-Positive Hepatocellular Carcinoma. PloS One (2015) 10:e0144934. doi: 10.1371/journal.pone.0144934
109. Zuo K, Kong L, Xue D, Yang Y, Xie L. The Expression and Role of lncRNA AX800134 in Hepatitis B Virus-Related Hepatocellular Carcinoma. Virus Genes (2018) 54:475–83. doi: 10.1007/s11262-018-1564-1
110. Gong X, Wei W, Chen L, Xia Z, Yu C. Comprehensive Analysis of Long non-Coding RNA Expression Profiles in Hepatitis B Virus-Related Hepatocellular Carcinoma. Oncotarget (2016) 7:42422–30. doi: 10.18632/oncotarget.9880
111. Zhao X, Bai Z, Li C, Sheng C, Li H. Identification of a Novel Eight-lncRNA Prognostic Signature for HBV-HCC and Analysis of Their Functions Based on Coexpression and ceRNA Networks. BioMed Res Int (2020) 2020:e8765461. doi: 10.1155/2020/8765461
112. Huang K, Lu Z, Li L, Peng G, Zhou W, Ye Q. Construction of a ceRNA Network and a Genomic-Clinicopathologic Nomogram to Predict Survival for HBV-Related HCC. Hum Cell (2021) 34:1830–42. doi: 10.1007/s13577-021-00607-y
113. Gu J-X, Zhang X, Miao R-C, Xiang X-H, Fu Y-N, Zhang J-Y, et al. Six-Long non-Coding RNA Signature Predicts Recurrence-Free Survival in Hepatocellular Carcinoma. World J Gastroenterol (2019) 25:220–32. doi: 10.3748/wjg.v25.i2.220
Keywords: HBV-related HCC, lncRNA, epigenetic regulation, transcriptional regulation, ceRNA, protein interactions, miRNA precursor processing
Citation: Samudh N, Shrilall C, Arbuthnot P, Bloom K and Ely A (2022) Diversity of Dysregulated Long Non-Coding RNAs in HBV-Related Hepatocellular Carcinoma. Front. Immunol. 13:834650. doi: 10.3389/fimmu.2022.834650
Received: 13 December 2021; Accepted: 10 January 2022;
Published: 28 January 2022.
Edited by:
Saikat Boliar, Cornell University, United StatesReviewed by:
James Ahodantin, University of Maryland, Baltimore, United StatesKazuo Kinoshita, Shizuoka Graduate University of Public Health, Japan
Copyright © 2022 Samudh, Shrilall, Arbuthnot, Bloom and Ely. This is an open-access article distributed under the terms of the Creative Commons Attribution License (CC BY). The use, distribution or reproduction in other forums is permitted, provided the original author(s) and the copyright owner(s) are credited and that the original publication in this journal is cited, in accordance with accepted academic practice. No use, distribution or reproduction is permitted which does not comply with these terms.
*Correspondence: Abdullah Ely, QWJkdWxsYWguRWx5QHdpdHMuYWMuemE=
†These authors have contributed equally to this work