- 1Reproductive Medicine Center, Department of Obstetrics and Gynecology, Renmin Hospital of Wuhan University, Wuhan, China
- 2Shenzhen Key Laboratory of Reproductive Immunology for Peri-implantation, Shenzhen Zhongshan Institute for Reproduction and Genetics, Shenzhen Zhongshan Urology Hospital, Shenzhen, China
- 3Department of Clinical Laboratory, Renmin Hospital of Wuhan University, Wuhan, China
Patients with recurrent pregnancy loss (RPL) account for approximately 1%-5% of women aiming to achieve childbirth. Although studies have shown that RPL is associated with failure of endometrial decidualization, placental dysfunction, and immune microenvironment disorder at the maternal-fetal interface, the exact pathogenesis remains unknown. With the development of high-throughput technology, more studies have focused on the genomics, transcriptomics, proteomics and metabolomics of RPL, and new gene mutations and new biomarkers of RPL have been discovered, providing an opportunity to explore the pathogenesis of RPL from different biological processes. Bioinformatics analyses of these differentially expressed genes, proteins and metabolites also reflect the biological pathways involved in RPL, laying a foundation for further research. In this review, we summarize the findings of omics studies investigating decidual tissue, villous tissue and blood from patients with RPL and identify some possible limitations of current studies.
Introduction
Recurrent pregnancy loss (RPL), defined as two or more consecutive clinically recognized spontaneous pregnancy losses before 20 weeks of gestation and includes embryonic or fetal loss, is a frequently occurring human infertility-related disease that affects 1-5% of parturients (1). Various factors have been proven to cause the occurrence and development of RPL, including chromosomal abnormalities, genital tract anomalies, immunological diseases, endocrine diseases, antiphospholipid syndrome, thrombophilic disorders and pathogen infections (2). Approximately 40-50% of cases remain unexplained, and the molecular mechanisms have not been fully explored. These cases are defined as unexplained recurrent pregnancy loss (URPL) (3). Although the diagnosis of RPL is relatively clear, the lack of standardized definitions, the uncertainties of its pathogenesis and the variable clinical manifestations still hamper progress in the treatment and prevention of RPL (4).
Omics studies generate a large amount of information concerning the biomarkers, molecular mechanisms and biological pathways involved in complex diseases (5). More recently, due to advances in the development and optimization of high-throughput techniques, numerous studies have applied omics approaches to the study of RPL (6–8). In this review, we summarize the results of omics-based studies conducted using human samples (decidua, villus, and blood) to explain the pathophysiological processes of RPL. The keywords in the included references were omics, omics technology, genomics, epigenomics, transcriptomics, proteomics, metabolomics, recurrent miscarriage, recurrent pregnancy loss, trophoblast, villi, decidua, and blood. We also attempted to perform an integrative analysis of omics data to obtain a global depiction of the complex relationships within and between different biological layers in RPL.
Overview of Omics
Omics technologies, including genomics, epigenomics, transcriptomics, proteomics (9, 10) and metabolomics (11), provide a holistic and integrative approach toward the study of biological systems (12). Through genomics technology, we can collectively characterize and quantitatively analyze all genes of an organism and then study the structure, function, location, and editing of the genome and their impact on the organism. In genomics, genotype arrays and next-generation sequencing are mostly used to obtain copy number variations, single nucleotide variations and small insertions or deletions (13, 14). Epigenomics refers to the study of all epigenetic modifications at the genome level, and epigenetic modifications are heritable. The most pivotal epigenetic modifications are DNA methylation, histone modifications and nucleosome remodeling. The current epigenetic studies investigating RPL mainly focus on DNA methylation (15). The main methods of DNA methylation analyses are bisulfite sequencing, array or bead hybridization, pyrosequencing, methylation-specific polymerase chain reaction (PCR), high -performance liquid chromatography-ultraviolet, and mass spectrometry (MS)-based approaches (16). The transcriptomics approach, including microarrays, bulk RNA sequencing (RNA-seq) and single-cell RNA-seq (scRNA-seq), is often used to study gene expression and regulation. Qualitative and quantitative information concerning mRNA and noncoding RNA is available due to these approaches (17). Proteomics is the systematic and holistic study of the types, structures and functions of proteins expressed in cells or tissues. Proteomics methods mainly include protein microarray, gel-based approaches [two-dimensional polyacrylamide gel electrophoresis (2-DE)], MS-based approaches (18), X-ray crystallography, nuclear magnetic resonance spectroscopy, SOMAmer-based technology, and quantitative techniques, including isotope-coded affinity tag labeling, stable isotope labeling with amino acids in cell culture and isobaric tags for relative and absolute quantitation (iTRAQ) (19, 20). However, most proteomics studies investigating RPL were performed using 2D-DIGE or quantitative techniques, such as iTRAQ combined with MS-based approaches. Metabolomics usually identifies and quantifies metabolites, such as amino acids, lipids, sugars and hormones, via nuclear magnetic resonance, gas chromatography-mass spectrometry and liquid chromatography-mass spectrometry. By exploring the relative relationship between metabolites and physiopathological changes, it is possible to find biomarkers for the diagnosis of disease. By studying and integrating data obtained using different omics approaches, knowledge of the underlying molecular interactions and associated longitudinal effects may be discovered and understood more deeply (21). In some studies investigating RPL, omics technologies were used in combination with bioinformatics analyses, and key genes and molecular pathways affecting RPL were identified. Upregulated or downregulated genes have been found to participate in a variety of biological processes important for embryonic/fetal development and fertility (22–24).
Establishment and Maintenance of Early Pregnancy
A normal pregnancy starts with the successful implantation of a promising embryo into the receptive endometrium (25). In preparation for implantation, the endometrium undergoes remodeling, which is regulated by estrogen and progesterone. During this timepoint, the endometrial epithelium becomes permissive to the adhesion of embryonic trophectoderm cells (the outer cells of the blastocyst), and then, the embryo implants into the uterus (26). After embryo implantation, the endometrium is gradually decidualized under the stimulation of ovarian hormones and other inducing factors. Decidualization is the differentiation of endometrial stromal cells into secretory decidual stromal cells. This process involves balancing pro- and anti- inflammatory cytokines (27, 28), angiogenesis, and uterine spiral artery remodeling (29). Meanwhile, the aggregation of immune cells (including uterine natural killer cells, macrophages, T cells, and dendritic cells) in the endometrium is involved in regulating the microenvironment that sustains pregnancy (30, 31). Placenta formation is the key process responsible for maintaining the growth and development of the embryo. During this process, the trophectoderm cells of the blastocyst differentiate into the extravillous trophoblast and the villous trophoblast (comprising the cytotrophoblast and syncytiotrophoblast), which form the major cell lineages of the placenta. Cytotrophoblasts can further differentiate into invasive extravillous trophoblasts (32). The proliferation and invasion of extravillous trophoblast cells into the decidua and the uterine myometrium are necessary for uterine spiral artery remodeling and establishing maternal-fetal circulation (33). The villous trophoblast contacts the maternal blood directly, which affects the material exchange among the mother, placenta and fetus (34). Extravillous trophoblast invasion and placental development are also controlled by decidualization. The establishment and maintenance of early pregnancy are represented in Figure 1.
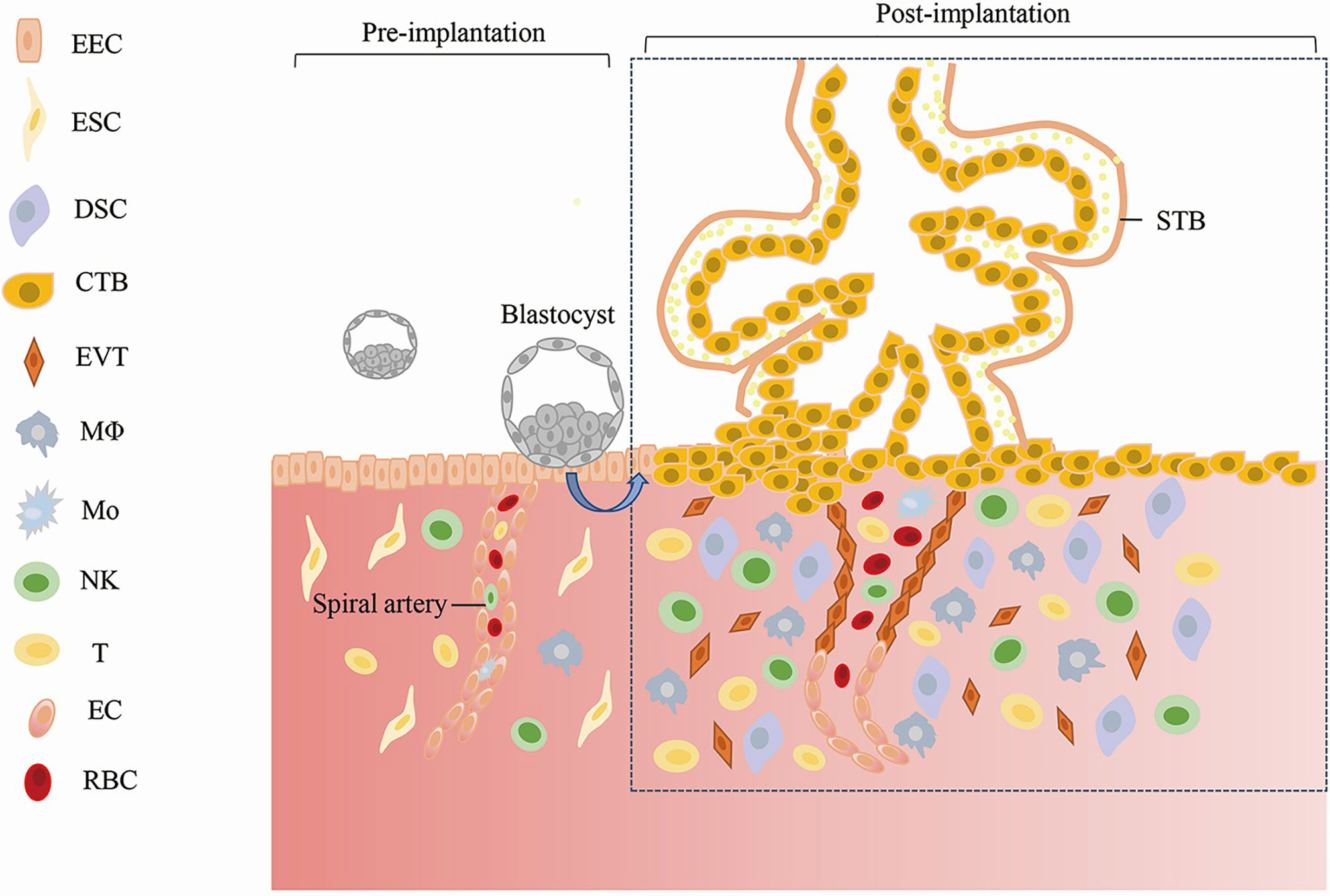
Figure 1 Establishment and Maintenance of Early Pregnancy. EEC, Endometrial Epithelial; Cell ESC, Endometrial Stromal Cell; DSC, Decidual Stromal Cell; CTB, Cytotrophoblast; STB, Syncytiotrophoblast; EVT, Extravillous Trophoblast; MΦ, Macrophages; Mo, Monocyte; NK, Natural Killer Cell; EC, Endothelial Cell; RBC, Red Blood Cell.
Omics Studies Investigating the Establishment and Maintenance of Early Pregnancy
During early pregnancy, extravillous trophoblasts move upstream along the arterial wall and migrate to, invade, and replace vascular smooth muscle cells and endothelial cells, thereby remodeling the uterine spiral arteries (35). Several cytokines produced by the placenta, such as vascular endothelial growth factor-A (VEGF-A), insulin-like growth factor, Krüppel-like factor 17, atrial natriuretic peptide may play important roles in the formation of the maternal-fetal vasculature (35, 36). Amin et al. found that the heterozygous genotype GA was significantly associated with the overexpression and underexpression of VEGF mRNA, while the homozygous variant genotype AA only decreased the VEGF mRNA levels in RPL patients by genotyping and quantitative real-time PCR (qRT–PCR-PCR) (37). Another similar study indicated that the 3’-untranslated region of VEGF resulted in susceptibility to RPL in Korean women (38). A recent study applying RNA sequencing of spontaneously hypertensive stroke-prone rats (SHRSP) found that the gene expression pattern of pregnant SHRSP uterine arteries was dominated by increased reactive oxygen species and downstream effectors of the renin-angiotensin-aldosterone system. The disrupted pathway involved may contribute to adverse vascular remodeling and the resultant placental ischemia and systemic vascular dysfunction (39). Uterine spiral artery remodeling is essential for promoting blood flow to the placenta and fetal development, and omics could help us better understand this process.
Successful decidualization is necessary for a normal pregnancy. By using DNA microarray and cytological verification, Lucas et al. demonstrated that in RPL, the loss of an epigenetic signature was related to the reduced expression of endometrial HMGB2 [associated with replicative senescence of human fibroblasts (40)], and then perturbed decidualization (41). Recently, scRNA-seq data of highly proliferative mesenchymal cells (hPMCs) in the midluteal human endometrium indicated that hPMC depletion was relevant to RPL. Vascular transmigration- and decidualization -related genes, including interleukin 1 receptor like 1 (42) and prolactin, were highly expressed in hPMC (43). hPMCs play an integral role in decidualization in pregnancy (44). A proteomic analysis also confirmed that decidualization in RPL patients differed from that in normal pregnant women. Dhaenens et al. identified 1416 differentially expressed proteins (DEPs), revealing the higher expression of serotransferrin in RPL samples compared with those in normal fertile samples (45). Another study by Harden et al. showed significant differences in endometrial metabolic profiles between decidualized and nondecidualized endometrium, which may be essential for successful embryo implantation (46).
The imbalance of immune tolerance at the maternal-fetal interface is an important factor for the occurrence of RPL (47). The immunologic events occurring at the maternal-fetal interface in early pregnancy are extremely complex and involve numerous immune cells and molecules with immunoregulatory properties (47). A genome-wide transcriptome profiling of splenic B cells in pregnant and nonpregnant mice found revealed 625 upregulated and 511 downregulated transcripts in B cells from pregnant mice compared with nonpregnant mice, suggesting that B cells acquire a state of hypo-responsiveness during gestation (48). Researchers performing single-cell transcriptomic profiling of decidual tissue revealed a dramatic difference in immune cell subsets and molecular properties in RPL cases (49). In RPL patients, a decidual NK (dNK) subset that supports embryonic growth was diminished in proportion, while the ratio of another dNK subset with cytotoxic and immune-active signatures (such as pro-inflammatory CD56+CD16+ dNK subset) was significantly increased (50). Chen et al. found that a subpopulation of CSF1+CD59+ KIR-expressing dNK cells was decreased in URPL decidua (51). Treg cells constitute another important immune cell type at the maternal-fetal interface, and the transcriptional and protein expression profiles of endometrial Tregs in RPL differ from those in normal pregnant women (52).
During early pregnancy, the changes in different genes, RNAs, proteins and metabolites associated with vascular remodeling, the abnormal expression of decidualized genes and phenotypic changes in various uterine immune cells all increase the possibility of the occurrence of RPL by affecting embryo implantation and blastocyst development (Figure 2). Although the pathogenesis of RPL is known to some extent, specific diagnostic biomarkers and candidate regulatory targets of RPL have not yet been identified. Thus, researchers have conducted various omics studies using decidual tissue, villi tissue, and blood from patients with RPL.
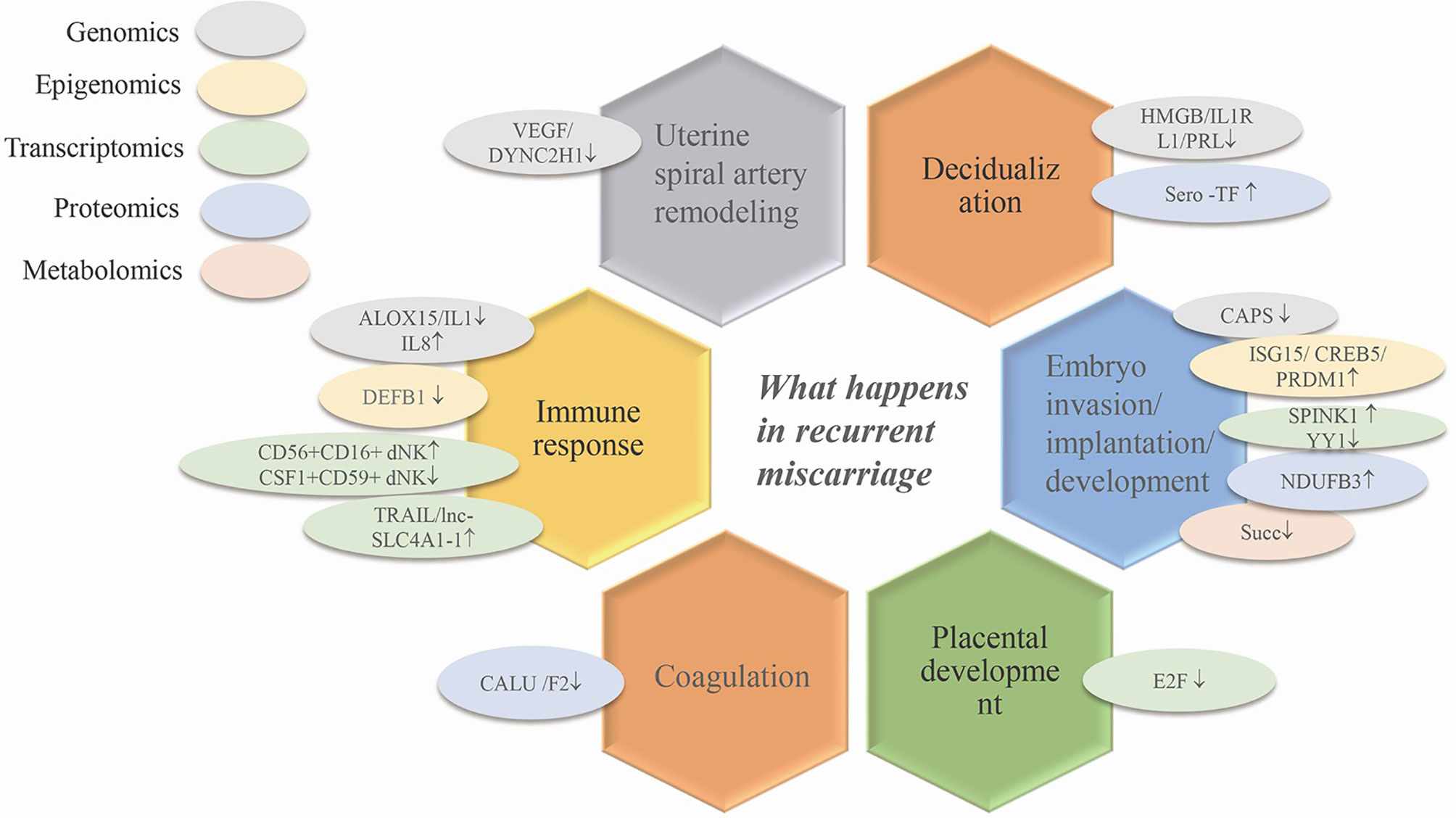
Figure 2 The application of omics techniques in recurrent miscarriage. Ovals of different colors represent different omics studies. VEGF, vascular endothelial growth factor; HMGB, high mobility group box; IL1RL1, interleukin 1 receptor-like 1; PRL, prolactin; ISG15, interferon stimulated gene 15; SPINK1, serine peptidase inhibitor Kazal-type 1; CREB5, cAMP responsive element binding protein5; CAPS, calcyphsine; PRDM1, positive regulatory domain 1; YY1, yin and yang 1 transcription factor; NDUFB3, NADH dehydrogenase (ubiquinone) 1 beta subcomplex 3; E2F, one transcription factor family; IL1, interleukin 1; IL8, IL1, interleukin 1; CD56+CD16+dNK/CSF1+CD59+dNK, transcriptomics via single cell sequencing; TRAIL, TNF-related apoptosis-inducing ligand; lnc-SLC4A1-1, solute carrier family 4, anion exchanger, member 1; DEFB1, defensin beta 1; Succ, succinate; CALU, calumenin; F2, coagulation factor II.
Omics Studies of Decidua in Recurrent Pregnancy Loss
It is of great significance to study the growth, degradation and functional regulation of maternal decidual tissue to clarify the mechanism of embryo implantation and fertility regulation. Epigenomics has verified that RPL is associated with abnormal DNA modification in decidual cells. In an animal model, the abnormal methylation of the decidua has been demonstrated to be associated with pregnancy failure (53). Li et al. conducted a genome-wide screening of DNA methylation in decidual samples from women with RPL (54). The findings showed that the differentially methylated genes (PRDM16, HLA-E, HLA-G, and ISG15) were closely related to embryonic development (55–57). QRT-PCR verification showed that the mRNA expression levels of ISG15, HLA-E, and HLA-G were increased in RPL. Li et al. also verified the upregulated expression of ISG15 in RPL by utilizing RNA-seq technology, and these authors found that ISG15 was involved in the type I interferon signaling pathway by Gene Ontology (GO) and Kyoto Encyclopedia of Genes and Genomes (KEGG) pathway enrichment analyses (54). CREB5 regulates cell growth, proliferation, differentiation and the cell cycle and belongs to the cAMP response element (CRE)-binding protein family (58). The upregulation of CREB5 has been shown to promote the invasion of tumor cells (59). Yu et al. conducted an analysis of DNA methylation and gene expression, and found that hypomethylation in the GREB5 promoter regions upregulated the mRNA and protein expression levels of CREB5 in RPL (60). These authors further proved that CREB5 increased migration and apoptosis in the HTR8-S/Vneo (human chorionic trophoblast cell line in vitro) and JEG-3 (human choriocarcinoma cell) cell lines and prolonged the cell cycle (61). Some studies have shown that CREB5 expression and methylation are related to the plasma interleukin-6 levels. Moreover, reduced CREB5 expression in monocytes could cause immunosuppression by increasing the tumor necrosis factor alpha (TNF-α) levels and decreasing the interleukin-10 levels in plasma (62, 63). However, the immune regulation of CREB5 at the maternal-fetal interface and its contribution to RPL require further verification.
The upregulation of matrix metallopeptidase 26 and the serine peptidase inhibitor Kazal-type 1 (64) are crucial for trophoblastic invasion by regulating the degradation of the extracellular matrix (65). Furthermore, Krieg et al. found that all genes in the interleukin-1 pathway (downregulated) and interleukin-8 pathway (upregulated) were differentially expressed (64), suggesting that the dysregulation of genes related to immunity might contribute to RPL. Transcriptomic data also suggest that the alteration of noncoding RNA (ncRNA) expression profiles in the decidua is related to RPL. NcRNAs refer to RNAs that do not have the potential to encode proteins, including microRNAs (miRNAs), long noncoding RNAs (lncRNAs) and circular RNAs (circRNAs). NcRNAs have complex types and functions that regulate cell activity by controlling gene expression, transcription, and translation processes (66). In the decidua of RPL patients, GO and KEGG pathway analyses suggest that differentially expressed miRNAs are involved in the ErbB signaling pathway and p53 signaling pathway. LncRNAs are involved in the peroxisome proliferator-activated receptor pathway. These signaling pathways participate in the progression of RPL (67, 68). However, the function of ncRNAs in decidual cells has not been further verified. Transcriptomics data have shown the abnormal expression of genes related to embryo invasion, implantation, and development, and immune responses of decidual cells. RPL may be the result of the coregulation of these differentially expressed genes (DEGs). Unfortunately, the regulatory mechanisms of most genes or regulatory factors have not been further explored. In addition, the function of some DEGs in the decidua was further verified in HTR8-S/Vneo and JEG-3 cell lines rather than decidual cells, which is less rigorous.
Proteomics studies investigating decidua also revealed numerous DEPs and pathways involved in the pathogenesis of RPL. The complex I subunit NDUFB3 was involved in mitochondrial respiratory chain function (69), which was significantly increased in the decidua of RPL. The overexpression of NDUFB3 induced oxidative stress and apoptosis in decidual stromal cells by participating in the process of oxidative phosphorylation and affecting mitochondrial function (70). Xiong et al. suggested that 50 DEPs in the decidua were important for embryonic development and revealed that angiotensinogen (AGT) was the most important upstream regulator (71). A proteomic analysis suggested changes in protein levels related to decidual cell growth and embryonic development in decidual tissue of RPL patients, identified more biomarkers of RPL, and helped us better understand the pathogenesis of RPL.
Metabolomics provides an opportunity to study the metabolic state of tissues. Metabolic abnormalities in the decidua could result in immune dysfunction and nutritional disorders, leading to pregnancy complications, including RPL. A recent study found differentially expressed metabolites, including lipids, amino acids and organic acids, in the decidua of RPL (72), indicatingabnormalities in glucose, lipid and amino acid metabolism in decidual cells. Metabolomics studies of RPL decidua are currently limited. However, differentially expressed metabolites in patients with RPL and subsequent functional analyses could be helpful for identifying biomarkers of RPL. Omics studies investigating decidual tissue in RPL are shown in Table 1.
Omics Studies of Villus in Recurrent Pregnancy Loss
Placental trophoblast cells can promote embryo implantation, uterine spiral artery remodeling and placentation via their proliferation, invasion, and migration. Furthermore, these cells secrete numerous active substances to regulate maternal-fetal interactions to ensure the normal growth and development of the embryo and fetus (17). It is important to understand the changes in trophoblast cell function in RPL to explain its pathogenesis. Whole-exome sequencing of villi indicate that different pathogenic genes played a vital role in RPL. For example, one patient was found to have compound heterozygous mutations in dynein cytoplasmic 2 heavy chain 1, while another patient exhibited compound heterozygous variants in arachidonate 15-lipoxygenase (74). Mutations in these genes may be individually or jointly involved in the occurrence of RPL by affecting inflammation, the oxidative stress response and angiogenesis (75, 76) at the maternal-fetal interface.,
Changes in DNA methylation affect the levels of gene expression. Positive regulatory domain 1 (PRDM1) is a transcription inhibitor that plays a role in embryonic development (95). In RPL, hypomethylation near the transcription start site of PRDM1 can upregulate the expression of PRDM1, leading to increased apoptosis and migration of trophoblast cells (78). PRDM1 hypomethylation plays a regulatory role by recruiting transcription factors, such as Forkhead boxA1 and GATA binding protein 2, and then regulating the differentiation and development of trophoblast cells (96). Another study found that in placental villus tissue of RPL patients, the β-defensinb1 gene involved in the innate immune response had decreased methylation at the promoter region (77, 97).
Transcriptomics is widely used in the study of villi tissue of RPL. RNA sequencing results showed that Yin Yang 1 (YY1) mRNA expression was reduced in the trophoblasts of RPL (83). YY1 is a transcription factor involved in embryogenesis. YY1 can enhance the invasion and proliferation of trophoblasts by directly binding the promoter region of the matrix metalloproteinase 2 gene, which is involved in extracellular matrix remodeling during trophoblast invasion (83, 98, 99). The mRNA expression levels of placental TNF-related apoptosis-inducing ligand and S100 calcium binding protein A8 were confirmed to be elevated in RPL (84). Both genes are associated with cell apoptosis and the immune response (100, 101), and their increased expression levels indicate fetal rejection and abnormalities in trophoblasts. Another study found that most of DEGs in trophoblastic cells of RPL could bind the transcription Factor E2F (82). E2F plays an important role in maintaining trophoblastic cell function and placental development, and the absence of E2F causes disruption in the placental transcriptional network and leads to fetal death (102). Other studies have focused on the role of ncRNAs in RPL, and the differentially expressed ncRNAs participate in biological pathways, including immunity, apoptosis and hormonal regulation (79, 80). Huang et al. identified increased lnc-SLC4A1-1 expression in URPL patients, which activated interleukin-8 (IL-8), and then exacerbated the inflammatory response of trophoblastic cells by enhancing the release of TNF-α and IL-1β (81).Transcriptome studies have revealed that the altered expression of genes or regulatory factors was associated with trophoblast proliferation, invasion, migration, and apoptosis, resulting in placental dysfunction and embryo failure to survive. In addition, the function of trophoblasts during pregnancy is affected by the immune response at the maternal-fetal interface. DEGs involved in the balance between pro- and anti-inflammatory responses also contribute to the development of RPL.
Recently, researchers have attempted to use proteomics technology to screen proteins associated with RPL in villous tissue. Compared with the normal condition, numerous DEPs in RPL, including AGT, mitogen-activated protein kinase14 (MAPK14) and Prothrombin (F2), were associated with early embryonic development (85). Among these proteins, AGT belongs to the renin-angiotensin system, MAPK14 participates in MAPK pathways, and defects in F2 lead to susceptibility to thrombosis. All these biological pathways play a role in RPL (103–105). Proteins related to regulating the function of endothelial cells and coagulation, including calumenin (CALU) and enolase 1 (ENO1), were also differentially expressed in villi (86). Defects in CALU promote coagulation and thrombosis, and a lack of ENO1 renders the placenta intolerant to hypoxia, eventually resulting in RPL (106, 107).
Research concerning the metabolomics of villous tissue in RPL is limited. A recent study showed that low succinate accumulation in villi participated in the occurrence of RPL by reducing the invasion and migration of trophoblastic cells (87). Omics of the villi of RPL patients indicated new biomarkers for the diagnosis and treatment of RPL; however, further research is needed to reveal how these differential molecules work and how they are regulated. Omics studies investigating villous tissue in RPL are shown in Table 2.
Omics Studies of Blood in Recurrent Pregnancy Loss
To explore the mechanism of RPL, researchers have conducted omics studies using maternal blood from RPL patients to find disease-causing genes and biomarkers of RPL. Genomics data show that a homozygous frameshift mutation in calcyphosine (CAPS) might be the potential pathogenesis of RPL (90). CAPS encodes a Ca2+-binding protein and is involved in the crosstalk between Ca2+ signaling and cAMP-protein kinase A pathways, which is crucial for embryo implantation and pregnancy maintenance (108, 109). Another molecule associated with embryo adhesion, trophinin, has also been found to be mutated in RPL. Functional defects in trophinin led to failed implantation and eventually evolved into RPL (110, 111). Genomics is a good way to identify potential targets or biomarkers for the diagnosis and treatment of RPL. These mutated genes are involved in various pathways, including cell adhesion between trophoblasts and the endometrium, embryo implantation, angiogenesis, and extracellular matrix remodeling. However, the extent to which these genes play a role in RPL has not been further verified.
The study of epigenomics in the maternal blood of RPL females is limited. Only one study showed that the methylenetetrahydrofolate reductase (MTHFR) gene was specifically hypermethylated in RPL (91). MTHFR is a thrombophilic marker involved in global DNA methylation. The methylation of the MTHFR gene alters the transmethylation cycle and leads to other gene methylation abnormalities, ultimately contributing to the development of RPL (112).
Using a proteomics approach, researchers have found that DEPs, including CD45, pregnancy-specific glycoprotein 1, and peroxiredoxin-2, act as predictive and diagnostic biomarkers of RPL (92). These proteins affect the invasiveness of trophoblastic cells through the Fc gamma R-mediated phagocytosis pathway, and the regulation of reactive species oxygen. Furthermore, proteins, such as insulin-like growth factor-binding protein-related protein 1, dickkopf-related protein 3 and angiopoietin-2, are significantly decreased in RPL, but the mechanism by which these proteins contribute to RPL is unclear (93).
The study of metabolomics in patients with RPL is limited. The only data available show that the levels of metabolites related to the tricarboxylic acid cycle and phenylalanine metabolism in RPL females significantly differ from those in normal controls. Lactic acid was increased, while 5-methoxytryptamine was lower in RPL patients (94). Testing metabolomics in blood provides new clues for the understanding of disease. The dysfunction of glucose, lipid and amino acid metabolism in RPL can be revealed by comparing the metabolic profiles of blood in RPL with those in normal pregnant women. This disorder might cause dysfunction of the uterus and placenta and further inhibit the growth and development of the embryo. Additionally, analyzing biomarkers in blood could to some extent reflect the condition of the decidua and villi of females and provide a new perspective for explaining the abnormal function of trophoblasts and decidual cells in RPL patients. Omics studies investigating blood in RPL are shown in Table 3.
In this review, we attempted to show the common genes, proteins and metabolites by integrating and analyzing data from references (Table 4). However, due to the limited research available and the incomplete data provided in some references, the results we obtained are not optimistic. The commonly altered genes and proteins shown in Table 4 were derived from the results of two or more studies.
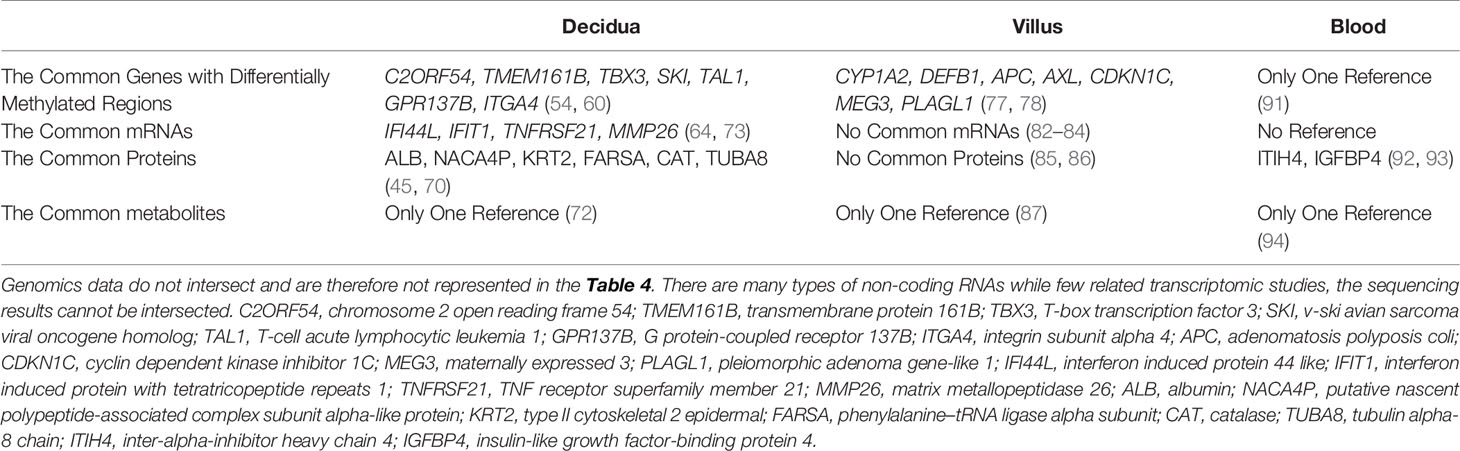
Table 4 The Common Genes, Proteins, Metabolites Changed in recurrent pregnancy loss patients compared with normal pregnancy females.
Advantages and Limitations of Omics Studies of Recurrent Pregnancy Loss
With the development of high-throughput technology, different omics technologies have been continuously improved, providing a new perspective for the mechanistic study of RPL. The genomics technique accurately identifies the disease-causing genes of RPL and discovers the types of defective genes. Epigenomics studies do not change the DNA sequence but allow us to understand the mechanism of RPL from the perspective of gene modification. Transcriptomics suggests the regulatory mechanism of RPL-related RNA. Proteomics and metabolomics provide new biomarkers and analyze the biological pathways, in which these molecules participate. By combining these omics findings, we infer that most differentially expressed molecules associated with RPL are involved in the processes of decidualization, embryo implantation, trophoblast cell differentiation, invasion and apoptosis, placental development, fetal development, the immune response, and coagulation. Omics research investigating RPL is an emerging field, and there are still some shortcomings in the related research. First, the sample sizes in most studies are small, the reproducibility of the data is poor, and it cannot be ruled out that these experimental results are caused by individual differences. Therefore, further studies with a larger sample size are needed to support the current results. Second, most studies were only performed with omics combined with a bioinformatics analysis. The results only show the potential pathways or regulation modules in which these molecules participate. To illustrate the specific mechanisms of these molecules in RPL, more basic research is needed. Third, most current studies were conducted at the single-omics level, and few studies integrated multiomics to verify the pathogenesis of RPL. Subsequent studies should perform multiple omics tests in patients with RPL, integrate information from different omics, and delve deeper into the pathogenesis of RPL. Finally, no studies performed proteomics in RPL to determine the posttranslational modifications of proteins, such as phosphorylation, ubiquitination, acetylation, and glycosylation, which can verify the biological processes more precisely. In addition, isotope labeling in differential metabolites can be used in a metabolic flux analysis to better understand the dynamics of metabolic systems in RPL. In summary, there is still a long way to go for research investigating the etiology, treatment and prevention of RPL based on high-throughput omics.
Conclusion
The pathogenesis of RPL is complex, and related to hormonal, environmental, and genetic factors. Furthermore, the causes of approximately 50% of RPL cases are unknown, and its incidence rate is increasing. Although assisted reproductive technology can be used to promote the pregnancy rate, the success rate is not very satisfactory. RPL patients are often under tremendous physical and psychological pressure. Therefore, in future studies, it is indispensable to comprehensively analyze the pathogenesis of RPL from different biochemical and biophysical processes. As omics investigates pathomechanisms from multiple dimensions, such as DNA, RNA, proteins, and metabolites, we hope that multiomics could provide more clues regarding the diagnosis and treatment of PRL.
Author Contributions
JL, LW and JD contributed equally to the preparation and the writing of the manuscript. YC and LD critically provided critical feedback and helped shape the manuscript. LL, YZ and TY supervised and revised the manuscript. All authors approved the final version of the manuscript for publication.
Funding
The project was funded by National Key Research & Developmental Program of China (2018YFC1004601, 2018YFC1003900/04), Guangdong Basic and Applied Basic Research Foundation (2019A1515011315), Shenzhen Fundamental Research Program (JCYJ20190813161010761, JCYJ20210324123601004), and National Natural Science Foundation of China (No. 82071655, No. 81801540).
Conflict of Interest
The authors declare that the research was conducted in the absence of any commercial or financial relationships that could be construed as a potential conflict of interest.
Publisher’s Note
All claims expressed in this article are solely those of the authors and do not necessarily represent those of their affiliated organizations, or those of the publisher, the editors and the reviewers. Any product that may be evaluated in this article, or claim that may be made by its manufacturer, is not guaranteed or endorsed by the publisher.
References
1. Rai R, Regan L. Recurrent Miscarriage. Lancet (2006) 368(9535):601–11. doi: 10.1016/S0140-6736(06)69204-0
2. Li TC, Makris M, Tomsu M, Tuckerman E, Laird S. Recurrent Miscarriage: Aetiology, Management and Prognosis. Hum Reprod Update (2002) 8(5):463–81. doi: 10.1093/humupd/8.5.463
3. Allison JL, Schust DJ. Recurrent First Trimester Pregnancy Loss: Revised Definitions and Novel Causes. Curr Opin Endocrinol Diabetes Obes (2009) 16(6):446–50. doi: 10.1097/MED.0b013e3283327fc5
4. Dimitriadis E, Menkhorst E, Saito S, Kutteh WH, Brosens JJ. Recurrent Pregnancy Loss. Nat Rev Dis Primers (2020) 6(1):98. doi: 10.1038/s41572-020-00228-z
5. Karahalil B. Overview of Systems Biology and Omics Technologies. Curr Med Chem (2016) 23(37):4221–30. doi: 10.2174/0929867323666160926150617
6. Rull K, Nagirnaja L, Laan M. Genetics of Recurrent Miscarriage: Challenges, Current Knowledge, Future Directions. Front Genet (2012) 3:34. doi: 10.3389/fgene.2012.00034
7. Hong L, Zhu YC, Liu S, Wu T, Li Y, Ye L, et al. Multi-Omics Reveals a Relationship Between Endometrial Amino Acid Metabolism and Autophagy in Women With Recurrent Miscarriagedagger. Biol Reprod (2021) 105(2):393–402. doi: 10.1093/biolre/ioab101
8. Du L, Deng W, Zeng S, Xu P, Huang L, Liang Y, et al. Single-Cell Transcriptome Analysis Reveals Defective Decidua Stromal Niche Attributes to Recurrent Spontaneous Abortion. Cell Prolif (2021) 54(11):e13125. doi: 10.1111/cpr.13125
9. Hasin Y, Seldin M, Lusis A. Multi-Omics Approaches to Disease. Genome Biol (2017) 18(1):83. doi: 10.1186/s13059-017-1215-1
10. Manzoni C, Kia DA, Vandrovcova J, Hardy J, Wood NW, Lewis PA, et al. Genome, Transcriptome and Proteome: The Rise of Omics Data and Their Integration in Biomedical Sciences. Brief Bioinform (2018) 19(2):286–302. doi: 10.1093/bib/bbw114
11. Worheide MA, Krumsiek J, Kastenmuller G, Arnold M. Multi-Omics Integration in Biomedical Research - A Metabolomics-Centric Review. Anal Chim Acta (2021) 1141:144–62. doi: 10.1016/j.aca.2020.10.038
12. Conesa A, Beck S. Making Multi-Omics Data Accessible to Researchers. Sci Data (2019) 6(1):251. doi: 10.1038/s41597-019-0258-4
13. Boycott KM, Vanstone MR, Bulman DE, MacKenzie AE. Rare-Disease Genetics in the Era of Next-Generation Sequencing: Discovery to Translation. Nat Rev Genet (2013) 14(10):681–91. doi: 10.1038/nrg3555
14. Stamou MI, Cox KH, Crowley WF Jr. Discovering Genes Essential to the Hypothalamic Regulation of Human Reproduction Using a Human Disease Model: Adjusting to Life in the "-Omics" Era. Endocr Rev (2015) 36(6):603–21. doi: 10.1210/er.2015-1045
15. Zhou Q, Xiong Y, Qu B, Bao A, Zhang Y. DNA Methylation and Recurrent Pregnancy Loss: A Mysterious Compass? Front Immunol (2021) 12:738962. doi: 10.3389/fimmu.2021.738962
16. Kurdyukov S, Bullock M. DNA Methylation Analysis: Choosing the Right Method. Biol (Basel) (2016) 5(1):3. doi: 10.3390/biology5010003
17. Jaremek A, Jeyarajah MJ, Jaju Bhattad G, Renaud SJ. Omics Approaches to Study Formation and Function of Human Placental Syncytiotrophoblast. Front Cell Dev Biol (2021) 9:674162. doi: 10.3389/fcell.2021.674162
18. Aslam B, Basit M, Nisar MA, Khurshid M, Rasool MH. Proteomics: Technologies and Their Applications. J Chromatogr Sci (2017) 55(2):182–96. doi: 10.1093/chromsci/bmw167
19. Shiio Y, Aebersold R. Quantitative Proteome Analysis Using Isotope-Coded Affinity Tags and Mass Spectrometry. Nat Protoc (2006) 1(1):139–45. doi: 10.1038/nprot.2006.22
20. Kroksveen AC, Jaffe JD, Aasebo E, Barsnes H, Bjorlykke Y, Franciotta D, et al. Quantitative Proteomics Suggests Decrease in the Secretogranin-1 Cerebrospinal Fluid Levels During the Disease Course of Multiple Sclerosis. Proteomics (2015) 15(19):3361–9. doi: 10.1002/pmic.201400142
21. Sun YV, Hu YJ. Integrative Analysis of Multi-Omics Data for Discovery and Functional Studies of Complex Human Diseases. Adv Genet (2016) 93:147–90. doi: 10.1016/bs.adgen.2015.11.004
22. Filges I, Nosova E, Bruder E, Tercanli S, Townsend K, Gibson WT, et al. Exome Sequencing Identifies Mutations in KIF14 as a Novel Cause of an Autosomal Recessive Lethal Fetal Ciliopathy Phenotype. Clin Genet (2014) 86(3):220–8. doi: 10.1111/cge.12301
23. Tsurusaki Y, Yonezawa R, Furuya M, Nishimura G, Pooh RK, Nakashima M, et al. Whole Exome Sequencing Revealed Biallelic IFT122 Mutations in a Family With CED1 and Recurrent Pregnancy Loss. Clin Genet (2014) 85(6):592–4. doi: 10.1111/cge.12215
24. Ellard S, Kivuva E, Turnpenny P, Stals K, Johnson M, Xie W, et al. An Exome Sequencing Strategy to Diagnose Lethal Autosomal Recessive Disorders. Eur J Hum Genet (2015) 23(3):401–4. doi: 10.1038/ejhg.2014.120
25. Cha J, Sun X, Dey SK. Mechanisms of Implantation: Strategies for Successful Pregnancy. Nat Med (2012) 18(12):1754–67. doi: 10.1038/nm.3012
26. Evans J, Salamonsen LA, Winship A, Menkhorst E, Nie G, Gargett CE, et al. Fertile Ground: Human Endometrial Programming and Lessons in Health and Disease. Nat Rev Endocrinol (2016) 12(11):654–67. doi: 10.1038/nrendo.2016.116
27. Rytkonen KT, Erkenbrack EM, Poutanen M, Elo LL, Pavlicev M, Wagner GP. Decidualization of Human Endometrial Stromal Fibroblasts Is a Multiphasic Process Involving Distinct Transcriptional Programs. Reprod Sci (2019) 26(3):323–36. doi: 10.1177/1933719118802056
28. Erkenbrack EM, Maziarz JD, Griffith OW, Liang C, Chavan AR, Nnamani MC, et al. The Mammalian Decidual Cell Evolved From a Cellular Stress Response. PloS Biol (2018) 16(8):e2005594. doi: 10.1371/journal.pbio.2005594
29. Sato Y. Endovascular Trophoblast and Spiral Artery Remodeling. Mol Cell Endocrinol (2020) 503:110699. doi: 10.1016/j.mce.2019.110699
30. Wang F, Qualls AE, Marques-Fernandez L, Colucci F. Biology and Pathology of the Uterine Microenvironment and its Natural Killer Cells. Cell Mol Immunol (2021) 18(9):2101–13. doi: 10.1038/s41423-021-00739-z
31. Murata H, Tanaka S, Okada H. Immune Tolerance of the Human Decidua. J Clin Med (2021) 10(2):351. doi: 10.3390/jcm10020351
32. Lee BK, Kim J. Integrating High-Throughput Approaches and In Vitro Human Trophoblast Models to Decipher Mechanisms Underlying Early Human Placenta Development. Front Cell Dev Biol (2021) 9:673065. doi: 10.3389/fcell.2021.673065
33. Huppertz B. Traditional and New Routes of Trophoblast Invasion and Their Implications for Pregnancy Diseases. Int J Mol Sci (2019) 21(1). doi: 10.3390/ijms21010289
34. Lunghi L, Ferretti ME, Medici S, Biondi C, Vesce F. Control of Human Trophoblast Function. Reprod Biol Endocrinol (2007) 5:6. doi: 10.1186/1477-7827-5-6
35. Albrecht ED, Pepe GJ. Regulation of Uterine Spiral Artery Remodeling: A Review. Reprod Sci (2020) 27(10):1932–42. doi: 10.1007/s43032-020-00212-8
36. Wang C, Wang Z, He M, Zhou T, Niu Y, Sun S, et al. Kruppel-Like Factor 17 Upregulates Uterine Corin Expression and Promotes Spiral Artery Remodeling in Pregnancy. Proc Natl Acad Sci USA (2020) 117(32):19425–34. doi: 10.1073/pnas.2003913117
37. Amin I, Pandith AA, Manzoor U, Mir SH, Afroze D, Koul AM, et al. Implications of VEGF Gene Sequence Variations and its Expression in Recurrent Pregnancy Loss. Reprod BioMed Online (2021) 43(6):1035–44. doi: 10.1016/j.rbmo.2021.08.009
38. An HJ, Kim JH, Ahn EH, Kim YR, Kim JO, Park HS, et al. 3'-UTR Polymorphisms in the Vascular Endothelial Growth Factor Gene (VEGF) Contribute to Susceptibility to Recurrent Pregnancy Loss (RPL). Int J Mol Sci (2019) 20(13):3319. doi: 10.3390/ijms20133319
39. Scott K, Morgan HL, Delles C, Fisher S, Graham D, McBride MW. Distinct Uterine Artery Gene Expression Profiles During Early Gestation in the Stroke-Prone Spontaneously Hypertensive Rat. Physiol Genomics (2021) 53(4):160–71. doi: 10.1152/physiolgenomics.00159.2020
40. Taniguchi N, Carames B, Ronfani L, Ulmer U, Komiya S, Bianchi ME, et al. Aging-Related Loss of the Chromatin Protein HMGB2 in Articular Cartilage is Linked to Reduced Cellularity and Osteoarthritis. Proc Natl Acad Sci USA (2009) 106(4):1181–6. doi: 10.1073/pnas.0806062106
41. Lucas ES, Dyer NP, Murakami K, Lee YH, Chan YW, Grimaldi G, et al. Loss of Endometrial Plasticity in Recurrent Pregnancy Loss. Stem Cells (2016) 34(2):346–56. doi: 10.1002/stem.2222
42. Salker MS, Nautiyal J, Steel JH, Webster Z, Sucurovic S, Nicou M, et al. Disordered IL-33/ST2 Activation in Decidualizing Stromal Cells Prolongs Uterine Receptivity in Women With Recurrent Pregnancy Loss. PloS One (2012) 7(12):e52252. doi: 10.1371/journal.pone.0052252
43. Gellersen B, Brosens JJ. Cyclic Decidualization of the Human Endometrium in Reproductive Health and Failure. Endocr Rev (2014) 35(6):851–905. doi: 10.1210/er.2014-1045
44. Diniz-da-Costa M, Kong CS, Fishwick KJ, Rawlings T, Brighton PJ, Hawkes A, et al. Characterization of Highly Proliferative Decidual Precursor Cells During the Window of Implantation in Human Endometrium. Stem Cells (2021) 39(8):1067–80. doi: 10.1002/stem.3367
45. Dhaenens L, Lierman S, De Clerck L, Govaert E, Deforce D, Tilleman K, et al. Endometrial Stromal Cell Proteome Mapping in Repeated Implantation Failure and Recurrent Pregnancy Loss Cases and Fertile Women. Reprod BioMed Online (2019) 38(3):442–54. doi: 10.1016/j.rbmo.2018.11.022
46. Harden SL, Zhou J, Gharanei S, Diniz-da-Costa M, Lucas ES, Cui L, et al. Exometabolomic Analysis of Decidualizing Human Endometrial Stromal and Perivascular Cells. Front Cell Dev Biol (2021) 9:626619. doi: 10.3389/fcell.2021.626619
47. Ticconi C, Pietropolli A, Di Simone N, Piccione E, Fazleabas A. Endometrial Immune Dysfunction in Recurrent Pregnancy Loss. Int J Mol Sci (2019) 20(21):5332. doi: 10.3390/ijms20215332.
48. Valeff N, Muzzio DO, Matzner F, Dibo M, Golchert J, Homuth G, et al. B Cells Acquire a Unique and Differential Transcriptomic Profile During Pregnancy. Genomics (2021) 113(4):2614–22. doi: 10.1016/j.ygeno.2021.06.016
49. Guo C, Cai P, Jin L, Sha Q, Yu Q, Zhang W, et al. Single-Cell Profiling of the Human Decidual Immune Microenvironment in Patients With Recurrent Pregnancy Loss. Cell Discov (2021) 7(1):1. doi: 10.1038/s41421-020-00236-z
50. Wang F, Jia W, Fan M, Shao X, Li Z, Liu Y, et al. Single-Cell Immune Landscape of Human Recurrent Miscarriage. Genomics Proteomics Bioinf (2021) 19(2):208–22. doi: 10.1016/j.gpb.2020.11.002
51. Chen P, Zhou L, Chen J, Lu Y, Cao C, Lv S, et al. The Immune Atlas of Human Deciduas With Unexplained Recurrent Pregnancy Loss. Front Immunol (2021) 12:689019. doi: 10.3389/fimmu.2021.689019
52. Granne I, Shen M, Rodriguez-Caro H, Chadha G, O'Donnell E, Brosens JJ, et al. Characterisation of Peri-Implantation Endometrial Treg and Identification of an Altered Phenotype in Recurrent Pregnancy Loss. Mucosal Immunol (2021) 15(1):120–9. doi: 10.1038/s41385-021-00451-1.
53. Brown LY, Bonney EA, Raj RS, Nielsen B, Brown S. Generalized Disturbance of DNA Methylation in the Uterine Decidua in the CBA/J X DBA/2 Mouse Model of Pregnancy Failure. Biol Reprod (2013) 89(5):120. doi: 10.1095/biolreprod.113.113142
54. Pi L, Zhang Z, Gu Y, Wang X, Wang J, Xu J, et al. DNA Methylation Profiling in Recurrent Miscarriage. PeerJ (2020) 8:e8196. doi: 10.7717/peerj.8196
55. Gelmini GF, Costa CH, Nardi FD, Wowk PF, Mattar SB, Schuffner A, et al. Is HLA-E a Possible Genetic Marker Relevant for Natural Conception? Am J Reprod Immunol (2016) 76(6):439–42. doi: 10.1111/aji.12587.
56. Verloes A, Spits C, Vercammen M, Geens M, LeMaoult J, Sermon K, et al. The Role of Methylation, DNA Polymorphisms and microRNAs on HLA-G Expression in Human Embryonic Stem Cells. Stem Cell Res (2017) 19:118–27. doi: 10.1016/j.scr.2017.01.005
57. Horn KH, Warner DR, Pisano M, Greene RM. PRDM16 Expression in the Developing Mouse Embryo. Acta Histochem (2011) 113(2):150–5. doi: 10.1016/j.acthis.2009.09.006
58. He S, Deng Y, Liao Y, Li X, Liu J, Yao S. CREB5 Promotes Tumor Cell Invasion and Correlates With Poor Prognosis in Epithelial Ovarian Cancer. Oncol Lett (2017) 14(6):8156–61. doi: 10.3892/ol.2017.7234
59. Qi L, Ding Y. Involvement of the CREB5 Regulatory Network in Colorectal Cancer Metastasis. Yi Chuan (2014) 36(7):679–84. doi: 10.3724/SP.J.1005.2014.0679
60. Yu M, Du G, Xu Q, Huang Z, Huang X, Qin Y, et al. Integrated Analysis of DNA Methylome and Transcriptome Identified CREB5 as a Novel Risk Gene Contributing to Recurrent Pregnancy Loss. EBioMedicine (2018) 35:334–44. doi: 10.1016/j.ebiom.2018.07.042
61. Nevalainen T, Kananen L, Marttila S, Jylha M, Hervonen A, Hurme M, et al. Transcriptomic and Epigenetic Analyses Reveal a Gender Difference in Aging-Associated Inflammation: The Vitality 90+ Study. Age (Dordr) (2015) 37(4):9814. doi: 10.1007/s11357-015-9814-9
62. Long X, Li Y, Qiu S, Liu J, He L, Peng Y. MiR-582-5p/miR-590-5p Targeted CREB1/CREB5-NF-kappaB Signaling and Caused Opioid-Induced Immunosuppression in Human Monocytes. Transl Psychiatry (2016) 6:e757. doi: 10.1038/tp.2016.4
63. Lv XF, Sun LL, Cui CL, Han JS. NAc Shell Arc/Arg3.1 Protein Mediates Reconsolidation of Morphine CPP by Increased GluR1 Cell Surface Expression: Activation of ERK-Coupled CREB is Required. Int J Neuropsychopharmacol (2015) 18(9):pyv030. doi: 10.1093/ijnp/pyv030.
64. Krieg SA, Fan X, Hong Y, Sang QX, Giaccia A, Westphal LM, et al. Global Alteration in Gene Expression Profiles of Deciduas From Women With Idiopathic Recurrent Pregnancy Loss. Mol Hum Reprod (2012) 18(9):442–50. doi: 10.1093/molehr/gas017
65. Li Q, Wang H, Zhao Y, Lin H, Sang QA, Zhu C. Identification and Specific Expression of Matrix Metalloproteinase-26 in Rhesus Monkey Endometrium During Early Pregnancy. Mol Hum Reprod (2002) 8(10):934–40. doi: 10.1093/molehr/8.10.934
66. Beermann J, Piccoli MT, Viereck J, Thum T. Non-Coding RNAs in Development and Disease: Background, Mechanisms, and Therapeutic Approaches. Physiol Rev (2016) 96(4):1297–325. doi: 10.1152/physrev.00041.2015
67. Wang JM, Gu Y, Zhang Y, Yang Q, Zhang X, Yin L, et al. Deep-Sequencing Identification of Differentially Expressed miRNAs in Decidua and Villus of Recurrent Miscarriage Patients. Arch Gynecol Obstet (2016) 293(5):1125–35. doi: 10.1007/s00404-016-4038-5
68. Huang Z, Yu H, Du G, Han L, Huang X, Wu D, et al. Enhancer RNA lnc-CES1-1 Inhibits Decidual Cell Migration by Interacting With RNA-Binding Protein FUS and Activating PPARgamma in URPL. Mol Ther Nucleic Acids (2021) 24:104–12. doi: 10.1016/j.omtn.2021.02.018
69. Calvo SE, Compton AG, Hershman SG, Lim SC, Lieber DS, Tucker EJ, et al. Molecular Diagnosis of Infantile Mitochondrial Disease With Targeted Next-Generation Sequencing. Sci Transl Med (2012) 4(118):118ra10. doi: 10.1126/scitranslmed.3003310.
70. Yin XJ, Hong W, Tian FJ, Li XC. Proteomic Analysis of Decidua in Patients With Recurrent Pregnancy Loss (RPL) Reveals Mitochondrial Oxidative Stress Dysfunction. Clin Proteomics (2021) 18(1):9. doi: 10.1186/s12014-021-09312-2
71. Xiong YM, Pan HT, Ding HG, He Y, Zhang J, Zhang F, et al. Proteomic and Functional Analysis of Proteins Related to Embryonic Development of Decidua in Patients With Recurrent Pregnancy Lossdagger. Biol Reprod (2021) 105(5):1246–56. doi: 10.1093/biolre/ioab140
72. Wang LL, Liu H, Zhao SJ, Shen L, Xie T, Luo J, et al. The Metabolic Landscape of Decidua in Recurrent Pregnancy Loss Using a Global Metabolomics Approach. Placenta (2021) 112: 45–53. doi: 10.1016/j.placenta.2021.07.001
73. Li Y, Wang R, Wang M, Huang W, Liu C, Fang Z, et al. RNA Sequencing of Decidua Reveals Differentially Expressed Genes in Recurrent Pregnancy Loss. Reprod Sci (2021) 28(8):2261–9. doi: 10.1007/s43032-021-00482-w
74. Qiao Y, Wen J, Tang F, Martell S, Shomer N, Leung PC, et al. Whole Exome Sequencing in Recurrent Early Pregnancy Loss. Mol Hum Reprod (2016) 22(5):364–72. doi: 10.1093/molehr/gaw008
75. Goetz SC, Anderson KV. The Primary Cilium: A Signalling Centre During Vertebrate Development. Nat Rev Genet (2010) 11(5):331–44. doi: 10.1038/nrg2774
76. Ivanov I, Kuhn H, Heydeck D. Structural and Functional Biology of Arachidonic Acid 15-Lipoxygenase-1 (ALOX15). Gene (2015) 573(1):1–32. doi: 10.1016/j.gene.2015.07.073
77. Hanna CW, McFadden DE, Robinson WP. DNA Methylation Profiling of Placental Villi From Karyotypically Normal Miscarriage and Recurrent Miscarriage. Am J Pathol (2013) 182(6):2276–84. doi: 10.1016/j.ajpath.2013.02.021
78. Du G, Yu M, Xu Q, Huang Z, Huang X, Han L, et al. Hypomethylation of PRDM1 is Associated With Recurrent Pregnancy Loss. J Cell Mol Med (2020) 24(12):7072–7. doi: 10.1111/jcmm.15335
79. Wang L, Tang H, Xiong Y, Tang L. Differential Expression Profile of Long Noncoding RNAs in Human Chorionic Villi of Early Recurrent Miscarriage. Clin Chim Acta (2017) 464:17–23. doi: 10.1016/j.cca.2016.11.001
80. Tang L, Gao C, Gao L, Cui Y, Liu J. Expression Profile of Micro-RNAs and Functional Annotation Analysis of Their Targets in Human Chorionic Villi From Early Recurrent Miscarriage. Gene (2016) 576(1 Pt 2):366–71. doi: 10.1016/j.gene.2015.10.047.
81. Huang Z, Du G, Huang X, Han L, Han X, Xu B, et al. The Enhancer RNA lnc-SLC4A1-1 Epigenetically Regulates Unexplained Recurrent Pregnancy Loss (URPL) by Activating CXCL8 and NF-kB Pathway. EBioMedicine (2018) 38:162–70. doi: 10.1016/j.ebiom.2018.11.015
82. Sober S, Rull K, Reiman M, Ilisson P, Mattila P, Laan M. RNA Sequencing of Chorionic Villi From Recurrent Pregnancy Loss Patients Reveals Impaired Function of Basic Nuclear and Cellular Machinery. Sci Rep (2016) 6:38439. doi: 10.1038/srep38439
83. Tian FJ, Cheng YX, Li XC, Wang F, Qin CM, Ma XL, et al. The YY1/MMP2 Axis Promotes Trophoblast Invasion at the Maternal-Fetal Interface. J Pathol (2016) 239(1):36–47. doi: 10.1002/path.4694
84. Rull K, Tomberg K, Koks S, Mannik J, Mols M, Sirotkina M, et al. Increased Placental Expression and Maternal Serum Levels of Apoptosis-Inducing TRAIL in Recurrent Miscarriage. Placenta (2013) 34(2):141–8. doi: 10.1016/j.placenta.2012.11.032
85. Pan HT, Ding HG, Fang M, Yu B, Cheng Y, Tan YJ, et al. Proteomics and Bioinformatics Analysis of Altered Protein Expression in the Placental Villous Tissue From Early Recurrent Miscarriage Patients. Placenta (2018) 61:1–10. doi: 10.1016/j.placenta.2017.11.001
86. Gharesi-Fard B, Zolghadri J, Kamali-Sarvestani E. Alteration in the Expression of Proteins in Unexplained Recurrent Pregnancy Loss Compared With in the Normal Placenta. J Reprod Dev (2014) 60(4):261–7. doi: 10.1262/jrd.2013-096
87. Wang XH, Xu S, Zhou XY, Zhao R, Lin Y, Cao J, et al. Low Chorionic Villous Succinate Accumulation Associates With Recurrent Spontaneous Abortion Risk. Nat Commun (2021) 12(1):3428. doi: 10.1038/s41467-021-23827-0
88. Quintero-Ronderos P, Mercier E, Fukuda M, Gonzalez R, Suarez CF, Patarroyo MA, et al. Novel Genes and Mutations in Patients Affected by Recurrent Pregnancy Loss. PloS One (2017) 12(10):e0186149. doi: 10.1371/journal.pone.0186149
89. Maddirevula S, Awartani K, Coskun S, AlNaim LF, Ibrahim N, Abdulwahab F, et al. A Genomics Approach to Females With Infertility and Recurrent Pregnancy Loss. Hum Genet (2020) 139(5):605–13. doi: 10.1007/s00439-020-02143-5
90. Pan H, Xiang H, Wang J, Wei Z, Zhou Y, Liu B, et al. CAPS Mutations Are Potentially Associated With Unexplained Recurrent Pregnancy Loss. Am J Pathol (2019) 189(1):124–31. doi: 10.1016/j.ajpath.2018.09.010
91. Mishra J, Talwar S, Kaur L, Chandiok K, Yadav S, Puri M, et al. Differential Global and MTHFR Gene Specific Methylation Patterns in Preeclampsia and Recurrent Miscarriages: A Case-Control Study From North India. Gene (2019) 704:68–73. doi: 10.1016/j.gene.2019.04.036
92. Cui Y, He L, Yang CY, Ye Q. iTRAQ and PRM-Based Quantitative Proteomics in Early Recurrent Spontaneous Abortion: Biomarkers Discovery. Clin Proteomics (2019) 16:36. doi: 10.1186/s12014-019-9256-y
93. Wu Y, He J, Guo C, Zhang Y, Yang W, Xin M, et al. Serum Biomarker Analysis in Patients With Recurrent Spontaneous Abortion. Mol Med Rep (2017) 16(3):2367–78. doi: 10.3892/mmr.2017.6890
94. Li X, Yin M, Gu J, Hou Y, Tian F, Sun F. Metabolomic Profiling of Plasma Samples From Women With Recurrent Spontaneous Abortion. Med Sci Monit (2018) 24:4038–45. doi: 10.12659/MSM.907653
95. Ohinata Y, Payer B, O'Carroll D, Ancelin K, Ono Y, Sano M, et al. Blimp1 is a Critical Determinant of the Germ Cell Lineage in Mice. Nature (2005) 436(7048):207–13. doi: 10.1038/nature03813
96. Paul S, Home P, Bhattacharya B, Ray S. GATA Factors: Master Regulators of Gene Expression in Trophoblast Progenitors. Placenta (2017) 60(Suppl 1):S61–6. doi: 10.1016/j.placenta.2017.05.005
97. King AE, Kelly RW, Sallenave JM, Bocking AD, Challis JR. Innate Immune Defences in the Human Uterus During Pregnancy. Placenta (2007) 28(11-12):1099–106. doi: 10.1016/j.placenta.2007.06.002
98. Calame K, Atchison M. YY1 Helps to Bring Loose Ends Together. Genes Dev (2007) 21(10):1145–52. doi: 10.1101/gad.1559007
99. Ferretti C, Bruni L, Dangles-Marie V, Pecking AP, Bellet D. Molecular Circuits Shared by Placental and Cancer Cells, and Their Implications in the Proliferative, Invasive and Migratory Capacities of Trophoblasts. Hum Reprod Update (2007) 13(2):121–41. doi: 10.1093/humupd/dml048
100. Bai X, Williams JL, Greenwood SL, Baker PN, Aplin JD, Crocker IP. A Placental Protective Role for Trophoblast-Derived TNF-Related Apoptosis-Inducing Ligand (TRAIL). Placenta (2009) 30(10):855–60. doi: 10.1016/j.placenta.2009.07.006
101. Kostakis ID, Cholidou KG, Kallianidis K, Perrea D, Antsaklis A. The Role of Calprotectin in Obstetrics and Gynecology. Eur J Obstet Gynecol Reprod Biol (2010) 151(1):3–9. doi: 10.1016/j.ejogrb.2010.03.006
102. Ouseph MM, Li J, Chen HZ, Pecot T, Wenzel P, Thompson JC, et al. Atypical E2F Repressors and Activators Coordinate Placental Development. Dev Cell (2012) 22(4):849–62. doi: 10.1016/j.devcel.2012.01.013
103. Tyers M, Mann M. From Genomics to Proteomics. Nature (2003) 422(6928):193–7. doi: 10.1038/nature01510
104. Jeon YJ, Kim JH, Lee BE, Rah H, Shin JE, Kang H, et al. Association Between Polymorphisms in the Renin-Angiotensin System Genes and Prevalence of Spontaneously Aborted Fetuses. Am J Reprod Immunol (2013) 70(3):238–45. doi: 10.1111/aji.12110
105. Bell CE, Lariviere NM, Watson PH, Watson AJ. Mitogen-Activated Protein Kinase (MAPK) Pathways Mediate Embryonic Responses to Culture Medium Osmolarity by Regulating Aquaporin 3 and 9 Expression and Localization, as Well as Embryonic Apoptosis. Hum Reprod (2009) 24(6):1373–86. doi: 10.1093/humrep/dep010
106. Coppinger JA, Cagney G, Toomey S, Kislinger T, Belton O, McRedmond JP, et al. Characterization of the Proteins Released From Activated Platelets Leads to Localization of Novel Platelet Proteins in Human Atherosclerotic Lesions. Blood (2004) 103(6):2096–104. doi: 10.1182/blood-2003-08-2804
107. Pringle KG, Kind KL, Sferruzzi-Perri AN, Thompson JG, Roberts CT. Beyond Oxygen: Complex Regulation and Activity of Hypoxia Inducible Factors in Pregnancy. Hum Reprod Update (2010) 16(4):415–31. doi: 10.1093/humupd/dmp046
108. Kusama K, Yoshie M, Tamura K, Imakawa K, Isaka K, Tachikawa E. Regulatory Action of Calcium Ion on Cyclic AMP-Enhanced Expression of Implantation-Related Factors in Human Endometrial Cells. PloS One (2015) 10(7):e0132017. doi: 10.1371/journal.pone.0132017
109. Salker MS, Singh Y, Durairaj RRP, Yan J, Alauddin M, Zeng N, et al. LEFTY2 Inhibits Endometrial Receptivity by Downregulating Orai1 Expression and Store-Operated Ca(2+) Entry. J Mol Med (Berl) (2018) 96(2):173–82. doi: 10.1007/s00109-017-1610-9
110. Lessey BA. Adhesion Molecules and Implantation. J Reprod Immunol (2002) 55(1-2):101–12. doi: 10.1016/s0165-0378(01)00139-5
111. Quintero-Ronderos P, Mercier E, Fukuda M, González R, Suárez CF, Patarroyo MA, et al. Novel Genes and Mutations in Patients Affected by Recurrent Pregnancy Loss. PLoS One (2017) 12(10):e0186149. doi: 10.1371/journal.pone.0186149
Keywords: omics, villus, decidua, blood, recurrent pregnancy loss
Citation: Li J, Wang L, Ding J, Cheng Y, Diao L, Li L, Zhang Y and Yin T (2022) Multiomics Studies Investigating Recurrent Pregnancy Loss: An Effective Tool for Mechanism Exploration. Front. Immunol. 13:826198. doi: 10.3389/fimmu.2022.826198
Received: 30 November 2021; Accepted: 31 March 2022;
Published: 27 April 2022.
Edited by:
Udo Rudolf Markert, University Hospital Jena, GermanyReviewed by:
Nandor Gabor Than, Hungarian Academy of Sciences (MTA), HungaryFederico Jensen, University of Buenos Aires, Argentina
Copyright © 2022 Li, Wang, Ding, Cheng, Diao, Li, Zhang and Yin. This is an open-access article distributed under the terms of the Creative Commons Attribution License (CC BY). The use, distribution or reproduction in other forums is permitted, provided the original author(s) and the copyright owner(s) are credited and that the original publication in this journal is cited, in accordance with accepted academic practice. No use, distribution or reproduction is permitted which does not comply with these terms.
*Correspondence: Tailang Yin, reproductive@whu.edu.cn; Yan Zhang, peneyyan@mail.ustc.edu.cn; Longfei Li, lilongfei2005@163.com
†These authors have contributed equally to this work
‡These authors jointly directed the study