- Department of Rheumatology and Immunology, Tianjin Medical University General Hospital, Tianjin, China
Mucosal-associated invariant T (MAIT) cells are an unconventional T cell subset expressing a semi-invariant TCR and recognize microbial riboflavin metabolites presented by major histocompatibility complex class 1-related molecule (MR1). MAIT cells serve as innate-like T cells bridging innate and adaptive immunity, which have attracted increasing attention in recent years. The involvement of MAIT cells has been described in various infections, autoimmune diseases and malignancies. In this review, we first briefly introduce the biology of MAIT cells, and then summarize their roles in rheumatic diseases including systemic lupus erythematosus, rheumatoid arthritis, primary Sjögren’s syndrome, psoriatic arthritis, systemic sclerosis, vasculitis and dermatomyositis. An increased knowledge of MAIT cells will inform the development of novel biomarkers and therapeutic approaches in rheumatology.
Introduction
Rheumatic diseases are a group of multisystem, immune-mediated disorders, including rheumatoid arthritis (RA), systemic lupus erythematosus (SLE), primary Sjögren’s syndrome (pSS), psoriatic arthritis (PsA), systemic sclerosis (SSc), large vessel vasculitis and systemic vasculitis. Although the pathogenesis of rheumatic diseases remains unclear, various immune cells, especially T cells, participate in the development of rheumatic diseases (1–5). Mucosal-associated invariant T (MAIT) cells are innate-like T lymphocytes that express a semi-invariant T cell receptor (TCR). MAIT cells are abundant in humans and can respond to pathogens. While many studies have focused on the roles of MAIT cells in infection, it is increasingly clear that they are also involved in autoimmune diseases. In this review, we first summarize the phenotype, distribution, development, activation and function of MAIT cells, and we then describe their roles in rheumatic diseases.
Phenotype of MAIT Cells
Vα7.2-Jα33 (TRAV1-2-TRAJ33) rearrangement was found to be enriched in human double-negative (CD4-CD8-, DN) α/β T cells and the phylogenetically conserved T cell population was described to express an invariant TCR combined with a limited set of Vβ chains in 1990s (6, 7). In mice, these T cells express the orthologous Vα19-Jα33 (TRAV1-TRAJ33) (7). Since 2003, the new T cell subsets were named mucosal-associated invariant T (MAIT) cells because they are present in mucosal tissues (8). Human MAIT cell subsets are mostly CD8α+ (median 79.7%) or DN (median 14.3%) with a minor subset being CD4+ cells (median 1.3%) (9), whereas most murine MAIT cells are DN although CD4+ and CD8+ subsets are detected in lower proportions and with varied frequencies in a tissue-specific manner (10). A recent study has found that human DN and CD8+ MAIT cells are functionally distinct subsets, and it is speculated that apoptosis-prone DN MAIT cells may be derived from the main CD8+ MAIT cell population (11). MAIT cells recognize metabolites from the bacterial riboflavin (vitamin B2) pathway that are presented by the major histocompatibility complex class 1-related molecule (MR1) (12, 13). MR1 tetramers have been developed for the specific identification of MAIT cells (14). MAIT cells have high surface expression of natural killer (NK) receptors such as C-type lectin CD161, NKG2D and NKP30 (15). The expression of surface markers, including CD45RO, CD95hi, CD27, CD26hi, CD44hi, CD122 and CD62Llo, identify MAIT cells as effector and memory phenotypes (16, 17). MAIT cells also possess high levels of specific homing receptors, such as chemokine CC receptor (CCR)6, CCR5, CCR9, chemokine CXC receptor (CXCR)6 and CXCR3, which are involved in cell migration to peripheral tissues, particularly the liver, intestine and inflammatory tissues (10, 15). However, the expression levels of CXCR2 (CD182), CXCR7, and lymph node homing receptor CCR7 (CD197) are low (18). Moreover, the high surface expression of a series of cytokine receptors such as interleukin (IL)-7, IL-12, IL-18 and IL-23 receptors is of great importance to MAIT cell activation in the absence of TCR stimulation (10, 19). In humans, the T-Box transcription factor TBX21 (T-bet), retinoicacid-related orphan receptor (ROR)γt and promyelocytic leukemia zinc finger (PLZF) transcription factors are coexpressed, suggesting the type1, type 17, and innate-like functionality of MAIT cells (10, 16, 17) (Figure 1).
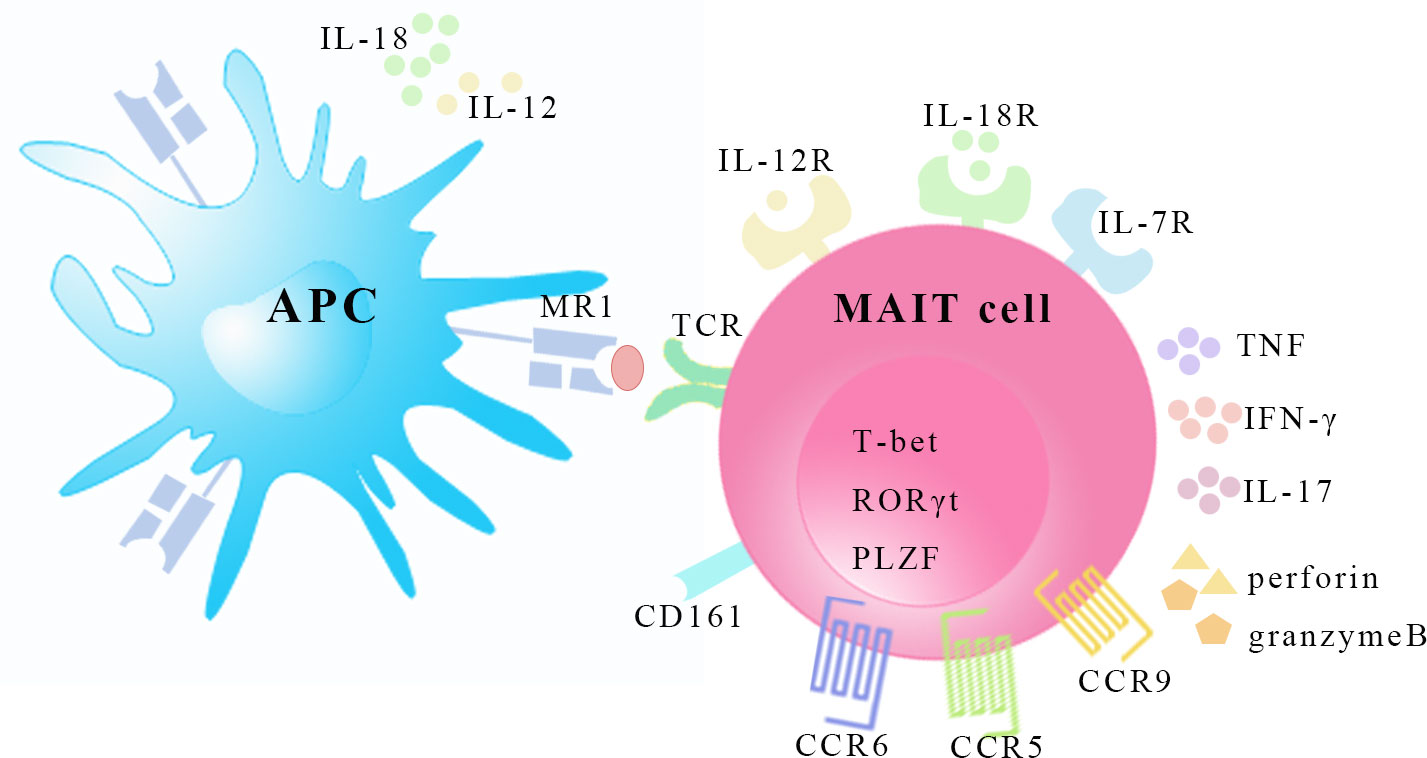
Figure 1 The characteristics of MAIT cells. MAIT cells recognize riboflavin-derived antigens presented by MR1 on surface of antigen presenting cell. MAIT cells express homing receptors CCR5, CCR6 and CCR9, and possess transcription factors T-bet, RORγt, and PLZF. MAIT cells can be activated in a TCR-dependent manner and cytokine-mediated manner by inflammatory cytokines like IL-12, IL-18 and IL-7. In response, MAIT cells secret Th1 and Th17 type cytokines and release granzyme B and perforin. APC, antigen presenting cell. MR1, major histocompatibility complex class 1-related molecule; TCR, T-cell receptor; CCR, CC-chemokine receptor; T-bet, T-Box transcription factor TBX21; RORγt, retinoic-acid-related orphan receptor γt; PLZF, promyelocytic leukemia zinc finger; IL, interleukin; TNF, tumor necrosis factor, IFN-γ, interferon-γ.
Distribution and Development of MAIT Cells
MAIT cells are not only enriched in mucosal tissues, but are also abundant in the blood (0.1-9.2%), liver (20-40%) and peripheral lymphoid organs under physiological conditions (8, 9, 20, 21). However, MAIT cells are less frequent in laboratory strains of mice (C57BL/6 and BALB/c) at homeostasis. The proportion is approximately 0.6% of T cells in mouse livers (10). MAIT cells develop in a thymus-dependent manner, similar to humans and mice, and they can be divided into three stages (17). Stage 1 MAIT cells are present only in the thymus and are mostly either double positive (DP) or CD4+CD8- thymocytes, and they lack CD161 and CD27 surface markers (CD24+CD44- in mice) (17). A small subset of stage 1 MAIT cells express RORγt, which is attributed to vitamin B2 metabolite transferring into the thymus (22). Stage 2 MAIT cells are present at low frequency in the periphery and are mostly CD4+CD8- cells that express CD161-CD27+ (CD24-CD44- in mice) with a low expression of T-bet and RORγt (17). MiRNAs may participate in the transition from stage 1 to 2, because in the absence of miR-181a/b-1, thymic MAIT cells show low expressions of ROR γt and T -bet (23). The maturation from stage 2 to stage 3 and acquisition of effector function are PLZF dependent, and commensal bacteria along with IL-18 play an important role in the process (17). Stage 3 MAIT cells acquire functional potential and start to migrate to peripheral organs. At stage 3, MAIT cells expressing CD161+CD27pos-low (CD24-CD44+ in mice) exhibit interferon (IFN)-γ-producing T-bet+ (MAIT-1) sublineage or IL-17 A producing RORγt+ (MAIT-17) (24). IL-2/IL-15 receptor β chain, inducible costimulatory (ICOS), the transcription factor (TF) Bcl11b and miR-155 are involved in regulating MAIT1 and MAIT17 cells differentiation (25–27). Recently, MAIT2 cells that express PLZF have been defined using single-cell RNA sequencing analysis, which are considered as developmental intermediates of MAIT1 and MAIT17 cells (28). MAIT cells undergo further maturation and expansion after leaving the thymus, and microbes play an important role in regulating the number of MAIT cells in the periphery (29, 30).
Activation and Function of MAIT Cells
MAIT cells can be activated by two pathways as follows: via TCR signalling (MR1 dependent) or cytokine signalling alone (MR1 independent). Riboflavin derivatives presented by MR1 of antigen presenting cells (APCs) activate MAIT cells, while the folic acid product is a competitive inhibitor (31). Because riboflavin synthesis is broadly conserved among bacteria and fungi, MAIT cells respond to a diverse array of microbes, including known commensals (32, 33). Following MR1-mediated activation, MAIT cells rapidly produce a wide range of cytokines, including interferon (IFN)-γ, tumor necrosis factor (TNF), IL-17 and IL-22. These cytokines, either directly (IFN-γ, TNF and IL-17) or indirectly through cell proliferation (IL-22), promote cytolysis of the infected cells, thereby leading to the control of various infections (15, 32, 34, 35). MAIT cells can also be activated by inflammatory cytokines alone, such as IL-7, IL-12, IL-18, IL-15 and IFN-α/β, in a TCR-independent manner (19, 36, 37). MAIT cells are activated with no known antigens, for instance, during viral infections or sterile auto-immunities. Cytokine-dependent activation of MAIT cells leads to secretion of IFN-γ, TNF and granzyme B, which exert cytotoxic functions (36, 37). Transcriptomic analysis of E. coli- and TCR-activated MAIT cells has shown distinct transcriptional reprogramming, including altered pathways, transcription factors and effector molecules, which has been validated by proteomics and metabolomics (38). In E. coli -activated MAIT cells, the TWIST1 transcription factor has been identified as a key driver, showing high connectivity to cytokine and chemokine expression (38).
MAIT Cells in Rheumatic Diseases
Because MAIT cells are also involved in a number of inflammatory and autoimmune disorders as well as malignancies, they are attractive targets for therapeutic approaches (39–43). The present paper aims to review the evidence indicating that MAIT cells may contribute to the pathogenesis of rheumatic diseases due to the possible role of MAIT cells in SLE, RA, PsA, ankylosing spondylitis (AS), SSc, pSS, systemic vasculitis, polymyalgia rheumatica and dermatomyositis (Table 1).
Systemic Lupus Erythematosus
Systemic lupus erythematosus (SLE) is a systemic autoimmune disease characterized by the production of autoantibodies to nuclear antigens. Blood MAIT cell frequency and absolute number are significantly lower in SLE patients than in healthy controls (HCs) (44). Absolute MAIT cell numbers are correlated with age, lymphocyte count, and SLE disease activity index, indicating that MAIT cell levels may reflect disease activity in SLE. The percentage of IFN-γ+ MAIT cells is lower in SLE patients than in HCs, and IL-17+ or IL-4+ MAIT cell levels are similar between SLE patients and HCs (44). IFN-γ production by MAIT cells has been found to be mainly regulated by the nuclear factor of activated T cell (NFAT1) transcription factor, which is intrinsically defective in SLE patients. Moreover, MAIT cell frequency is significantly correlated with NKT cell frequency in SLE patients. In particular, α-GalCer–stimulated NKT cells show poor activation of MAIT cells in SLE patients in vitro, suggesting that NKT cell dysfunction influences MAIT cell dysfunction (44). Another study has found that the reduced frequency of MAIT cells in peripheral blood is due to increased cell death, and the expression of activation marker CD69 is correlated with disease activity (45). In addition, monocytes from patients with SLE have an increased capacity to present MR1 antigens and activate MAIT cells. But the antigens that activate MAIT cells in SLE patients remain to be identified. Cytokine analysis has revealed that the plasma levels of IL-6, IL-18 and IFN-α positively correlate with the activated state of MAIT cells in SLE and that MAIT cells are activated by cytokines, including IL-18 and IFN-α, in the absence of exogenous antigens. In the kidneys of SLE patients with class III and IV lupus nephritis, MAIT cells constitute a large proportion of CD3+ cells (46). In FcγRIIb-/-Yaa mice, an animal model of SLE, more MAIT cells are activated in the kidneys than in the spleen, and the proportions of IL-17- or IFN-γ-expressing MAIT cells are higher in the kidneys than in the spleen. Furthermore, in MR1-/- lupus mice, MAIT cell deficiency results in reduced disease severity, as evidenced by decreased autoantibody production and lower glomerulonephritis scores, and these effects are accompanied by reduced germinal center responses as well as reduced T cell and innate T cell responses (46). Researchers have also demonstrated that MAIT cells contribute to autoantibody production by B cells in vitro dependent on the CD40L-CD40 and TCR pathways (46).
Rheumatoid Arthritis
Rheumatoid arthritis (RA) is a systemic inflammatory disorder and is characterized by chronic, erosive synovitis of diarthrodial joints. Many studies have reported that the MAIT cell percentage is decreased in the peripheral blood of RA patients, and circulating absolute MAIT cell numbers can reflect disease activity in RA (44, 47, 48). The synovial fluid of patients with RA has a higher percentage of MAIT cells than peripheral blood (44, 47). In early, untreated RA patients, the distribution of MAIT cells shifts from CD8+ to CD4+ MAIT cells, and MAIT cells express lower levels of CD161. Furthermore, CD69 expression of MAIT cells is lower in RA patients compared to HCs, and MAIT cells are hyporesponsive as indicated by minimal upregulation of CD25 and CD69 in response to E. coli stimulation (48). Peripheral blood and synovial fluid MAIT cells of RA patients produce more IL-17A compared to osteoarthritis (OA) patients on stimulation (49). In a MAIT cell-deficient MR1-/- mouse model of arthritis, the disease severity of collagen-induced arthritis (CIA) is ameliorated, indicating that MAIT cells play a pathogenic role in RA (50). In addition, MAIT cells augment arthritis mainly by enhancing inflammation in arthritis rather than by a type II collagen-specific response. In vitro, it is revealed that IL-1β induces the proliferation of MAIT cells, and IL-23 promotes the production of IL-17 by MAIT cells in the absence of TCR stimulation, indicating that cytokine-mediated MAIT cell activation contributes to the exacerbation of arthritis (50).
Psoriatic Arthritis
Psoriatic arthritis (PsA) is a chronic inflammatory spondyloarthritis that occurs in combination with psoriasis and has related extraarticular manifestations. The frequency of MAIT cells in peripheral blood is lower in PsA patients than in OA patients, whereas a higher MAIT cell frequency is found in paired synovial fluid, suggesting that MAIT cells are recruited to joints (49). Most MAIT cells in the synovial fluid of PsA patients are of CD8+ and the memory (CD45RO+) phenotype. Synovial fluid MAIT cells of PsA patients produce significantly more IL-17A than those of RA and OA patients. Moreover, the expression of IL-23R in circulating MAIT cells is higher in PsA and RA patients than in OA patients and IL-23R is functionally active, as evidenced by the profound mitotic effect in the presence of rIL-23 (49). These findings indicate that MAIT cells are likely to be part of the IL-23/IL-17 cytokine network in the pathogenesis of PsA.
Ankylosing Spondylitis
Ankylosing spondylitis (AS) is a chronic inflammatory rheumatic disease characterized by abnormal ossification and ankylosis affecting the spine and sacroiliac joints. Peripheral blood MAIT cell frequency and number are reduced in AS patients compared with HCs; however, MAIT cell frequency does not correlate with disease activity (47, 51, 52). The results obtained by Gracey et al. show that the level of CD69 of blood MAIT cells in AS patients is equivalent to that in HCs, while Hayashi et al. have found that CD69 expression on circulating MAIT cells correlates with the Ankylosing Spondylitis Disease Activity Score in patients with AS (47, 51). The discrepancy may be due to some factors, such as different disease stages and different treatments. MAIT cells are enriched in synovial fluid with high expression of CCR6. There is a higher percentage of IL-17+ and IL-22+ MAIT cells and a lower percentage IFN-γ+ MAIT cells in the blood of AS patients; in the paired synovial fluid of AS patients, TNF+ and IFN-γ+ MAIT cells are decreased, and the granzyme B+ and IL-17+ MAIT cell frequencies are increased (47, 52). Furthermore, IL-17 elevation in circulating MAIT cells of AS patients depends on priming with IL-7 but not IL-23 or MR1-presenting antigens (47).
Primary Sjögren’s Syndrome
Primary Sjögren’s syndrome (pSS) is a chronic systemic autoimmune disease characterized by lymphocytic infiltration of the exocrine glands leading to glandular dysfunction. pSS patients have a reduced frequency of MAIT cells, and MAIT cells are unable to be identified as a distinct population by CD161 and TCRVα7.2 in half of pSS patients (53). The blood MAIT cells in pSS patients are enriched in the naïve (CD45RA+, CCR7+) population and show an increase in CD4+ subset as well as a decrease in CD8+ subset. Compared to MAIT cells in HCs, circulating MAIT cells in pSS patients show lower activation status with lower CD154 and CD69 expression, and they produce less TNF and IFN-γ. There is no difference in the expression of IL-17, Th2 cytokines, granzyme A, granzyme B or perforin between MAIT cells from pSS and control subjects (53). MAIT cells are present in the salivary gland tissue of pSS patients but undetectable in control biopsies (53). However, another study has demonstrated that MAIT cells are IL-17 polarized in the salivary glands of patients with pSS, and that in vitro IL-7 and IL-23 stimulation of MAIT cells induces IL-17 over-expression only in pSS patients. In addition, different molecular pathways are explored: IL-7 stimulation induced STAT3/HIF1alpha up-regulation and IL-23 stimulation induced RORc over-expression (54).
Systemic Sclerosis
Systemic sclerosis (SSc) is an immune-mediated rheumatic disease characterized by immune dysregulation and progressive fibrosis that affects the skin and internal organs. The peripheral blood MAIT cell frequency is decreased in patients with SSc compared to HCs (55). However, the circulating MAIT cell frequency and absolute numbers do not correlate with C reactive protein (CRP), brain natriuretic protein, pulmonary involvement, median skin fibrosis scale, steroid amount or disease duration in patients with SSc.
ANCA-Associated Vasculitis
Anti-neutrophil cytoplasmic autoantibodies (ANCA)-associated (AAV) is an autoimmune disease characterized by inflammation and necrosis of blood vessel walls, which is accompanied by the presence of ANCA antibodies in serum. AAV includes granulomatosis with polyangiitis (GPA), microscopic polyangiitis (MPA) and eosinophilic granulomatosis with polyangiitis (EGPA). The frequency of circulating MAIT cells is lower in patients with AAV compared to HCs, especially in MPA and GPA (56). MAIT cells in peripheral blood from AAV patients in the acute and remission phases exhibit an activated phenotype as judged by their increased expression of CD69 (57). The frequency of circulating MAIT cells that produce IFN-γ is increased in MPA and GPA patients, while the frequency of TNF and IL-17-producing MAIT cells is similar in AAV patients compared to controls (57).
Giant Cell Arteritis and Polymyalgia Rheumatica
Giant cell arteritis (GCA) is an immune-mediated vasculitis characterized by granulomatous inflammation that affects medium-sized and large arteries. MAIT cells are present in the arterial wall of GCA patients while the percentage of circulating MAIT cells is similar between untreated GCA patients and controls (58). The MAIT cells in GCA patients show decreased expression of CXCR3 and increased expression of IFN-γ. The percentage of circulating IFN-γ+ MAIT cells does not decrease after glucocorticoid therapy. MAIT cells from GCA patients proliferate and produce higher amounts of IFN-γ after treatment of IL-12 and IL-18, while the production of granzyme B and TNF is higher after activation of the TCR pathway. Polymyalgia rheumatica (PMR) is an inflammatory rheumatic disease characterized by pain and stiffness in the neck and pelvic girdle, which can occur independently or in association with giant cell arteritis. Among MAIT cells, the percentages of IFN-γ+ cells tend to be higher in PMR patients with active disease compared to patients with inactive disease, while the percentages of IL-17+ cells are comparable between the active and inactive patient groups. MAIT cells show greater activation in patients with PMR in remission than in controls and active patients as evidenced by a higher frequency of HLA-DR+CD38+ MAIT cells and CD69+ MAIT cells. Moreover, the frequency of HLA-DR+CD38+ MAIT cells is positively correlated with the PMR activity score and CRP level in patients in remission (59).
Dermatomyositis
Dermatomyositis (DM) is an inflammatory disorder, characterized by classical cutaneous manifestations and inflammation of skeletal muscle. The frequency of MAIT cells is decreased in active DM patients in comparison with HCs, and the frequency increases after treatment (60). Reduced MAIT cell frequency is associated with increased expression of activation and exhaustion markers such as CD25, CD39 and CTLA4. There is no correlation between MAIT cell frequency and DM clinical scores or biological markers.
Discussion
MAIT cells were observed to be decreased with an activated phenotype in peripheral blood in patients with rheumatic diseases; whether these changes are a cause or an effect remains to be established. Some studies have found that the frequency or the activation level of MAIT cells is consistent with disease severity (44, 51, 59), indicating that MAIT cells may be potential biomarkers for rheumatic disease. However, longitudinal studies are needed to verify whether the MAIT cell population may recover after treatment. Homing of MAIT cells to inflamed tissues, as evidenced by high expression of chemokine receptors, and activation-induced cell death may contribute to the decrease in circulating MAIT cells. In patients with COVID-19, the frequency of pyroptotic MAIT cells rather than apoptotic MAIT cells is significantly increased, suggesting that activated MAIT cells may exhibit different forms of morbidity (61). Furthermore, a previous study has shown that the CD3+ MR1-tetramer+ MAIT cell frequency is similar in HCs and patients with RA and that CD161 is reduced in early RA patients, indicating that the gating strategy of CD3+CD161+Vα7.2+ might be partially responsible for the reduction in MAIT cells (48). Another study has also found CD161 is decreased in chronic HIV-1 infection and this Vα7.2+CD161– population does not contain MAIT cells (62, 63). Glucocorticoids have long been known to inhibit T cell proliferation and induce apoptosis (64). It has been reported that circulating MAIT cell frequency is associated with oral corticosteroids in asthma patients and MAIT cells are deficient in the airways of steroid-treated chronic obstructive pulmonary disease patients (65, 66). However, no significant correlations are found between circulating MAIT cell levels and use of steroids in SLE and GCA patients (44, 58). This inconsistency could be attributed to different doses of glucocorticoids. More in vitro experiments and animal studies are needed to verify the effects of corticosteroids on MAIT cells. MAIT cell activation is observed in some rheumatic diseases and pro-inflammatory cytokines have been identified as potential activator of MAIT cells (45, 57, 59). Is there the possibility that MR1 presents riboflavin derivatives or self-antigens to activate MAIT cells? The use of an inhibitory MR1 ligand to block MAIT cell activation and sterile environments may offer the potential to study the activation mechanism of MAIT cells in rheumatic diseases.
In most rheumatic diseases, IFN-γ and TNF produced by circulating MAIT cells decrease, while IL-17 produced by MAIT cells increases in blood and tissues (Figure 2), suggesting that MAIT cell proinflammatory IL-17 is biased in rheumatology similar to that in obesity and chronic liver disease (67–69). IL-17 plays different pathological roles in some rheumatic diseases, like the promotion and perpetuation of inflammation and the damage to the affected tissues (70, 71). Several therapeutic strategies targeting IL-17 have been approved for the treatment of PsA and AS. MAIT cells, as a source of IL-17, emerge in the mechanism of rheumatology and might be a promising therapeutic option. It remains to be determined whether MAIT cells are “friends or foes” in autoimmune responses. MAIT cells appeared with pathogenic roles in SLE and RA animal models; however, a protective role of MAIT cells in an animal model of multiple sclerosis has been observed (46, 50, 72). In type 1 diabetes, MAIT cells show dual roles in maintaining gut barrier integrity by secreting IL-17A and IL-22 but promote β-cell death in the pancreas (73). The effects of MAIT cells seems to be dependent on disease stages and their tissue localization, and MAIT cells may have other functions we do not yet know about due to the lack of known antigens of MAIT cells. More research is needed to further study the molecular mechanism of MAIT cells in rheumatic diseases, especially the MAIT cells recruited to affected organs. The cross-talk of MAIT cells and several immune cell subsets by secretion of cytokines and chemokines has been demonstrated (74). Our team has found MAIT cells induce anti-inflammatory macrophage polarization by producing regulatory cytokines in nonalcoholic fatty liver disease (75). MAIT cells increase plasmablasts and promote Ig production in vitro, and they are involved in autoantibody production by B cells in SLE (46, 76). We speculate that these MAIT-B cell interactions may occur in B cell/autoantibody-driven disorders, such as pSS and AAV. Further studies to understand how MAIT cells encounter autoreactive B cells are needed.
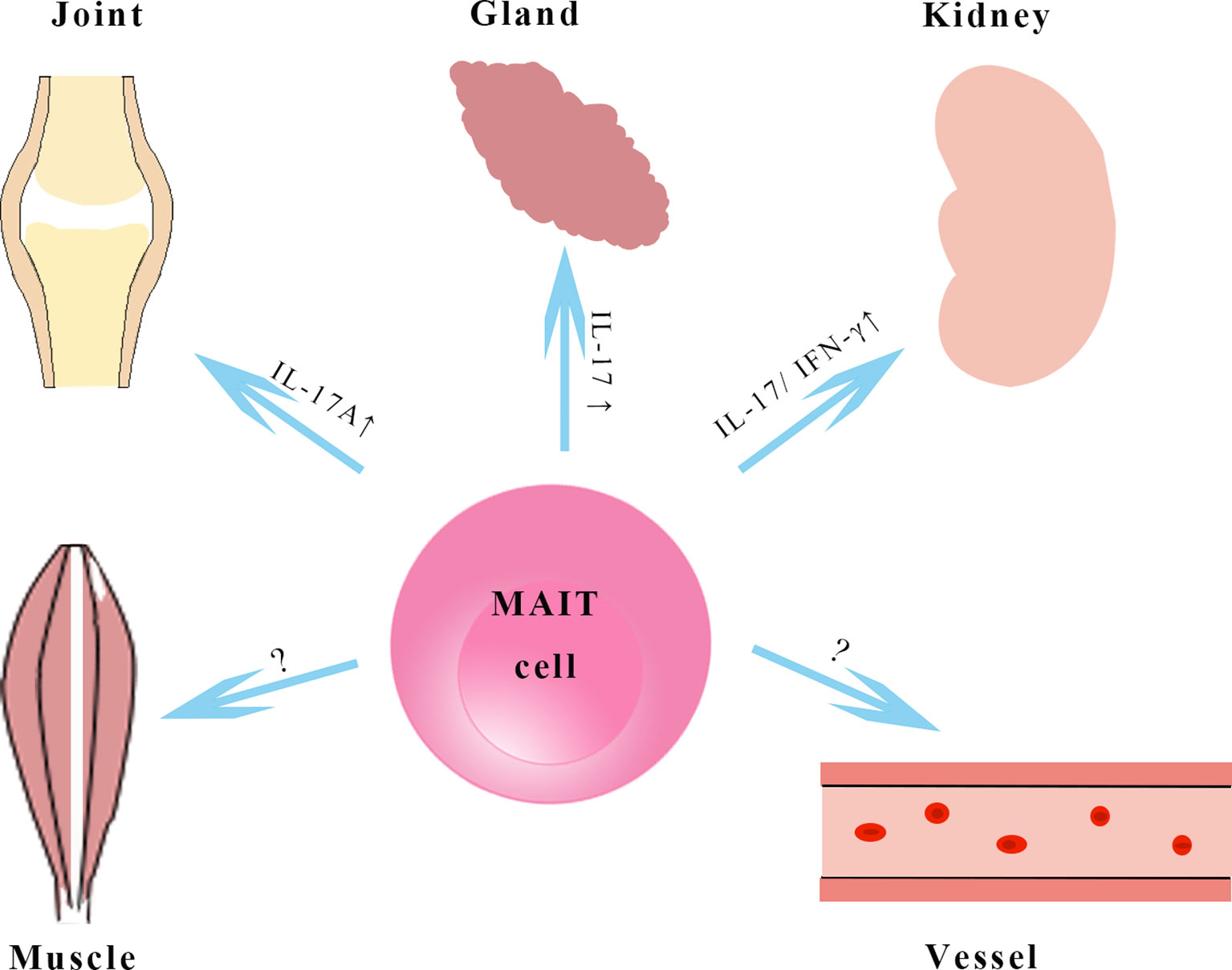
Figure 2 MAIT cells in rheumatological disorders. MAIT cells in kidney, joint, vessels and salivary glands in rheumatic diseases. MAIT cells in tissues produce higher amounts of IL-17 and may exhibit a pathogenic role in animal models with systemic lupus erythematosus and rheumatoid arthritis, however, the functions of MAIT cells in vessels and muscles remain to be elucidated. IL-17, interleukin-17; IFN-γ, interferon-γ.
There is increasing evidence that microbiomes and metabolites play a vital role in the immunopathogenesis of rheumatic diseases (77, 78). The reduced richness of microbial species and a reduced ratio of Firmicutes/Bacteroides in the gut are common features of rheumatic disease (79). As described above, microbiota-derived metabolites affect MAIT cell selection, expansion and function. In alcoholic liver disease, intestinal bacteria and metabolites drive selective MAIT cell depletion and dysfunction, whereas MAIT cells induce gut microbiota alterations in obesity model (80, 81). Thus, there are a few questions to be answered. It remains unknown whether the intestinal flora and metabolites change first to cause alternation in the functions of MAIT cells, thereby contributing to the diseases. It is important to understand how microbiota affect the phenotype of MAIT cells, and how microbiota-metabolite-MAIT cell interactions shape adaptive immunity. More in-depth investigations are required to study the correlations among dysbiosis, MAIT cell responses and clinical symptoms. The relative abundance of microbiota and metabolites in diseases can be determined by metagenomic and metabolomics analysis. And it would be interesting to investigate whether fecal microbiota transplantation from rheumatic patients in mouse models results in MAIT cell activation and disease exacerbation.
In this review, we have summarized current evidence supporting the emerging roles for MAIT cells in rheumatology. It is imperative that we continue to elucidate the activation mechanisms and functions of MAIT cells to better understand pathogenesis of rhematic diseases. MAIT cell deficiency (MR1-/-) and transgenic mice models, as wells as acetyl-6-formylpterin, a potent blocking MAIT cell molecule (82), are the new tools to be used for further investigations. The analysis of the crosstalk between MAIT cells and other immune cells and the interactions between MAIT cells and microbiota will identify therapeutic targets and approaches for clinical intervention in the future.
Author Contributions
YL and JD wrote the manuscript. WW provided the feedback. All authors contributed to the article and approved the submitted version.
Funding
This work was supported by the National Natural Science Foundation of China (grant 81900523).
Conflict of Interest
The authors declare that the research was conducted in the absence of any commercial or financial relationships that could be construed as a potential conflict of interest.
Publisher’s Note
All claims expressed in this article are solely those of the authors and do not necessarily represent those of their affiliated organizations, or those of the publisher, the editors and the reviewers. Any product that may be evaluated in this article, or claim that may be made by its manufacturer, is not guaranteed or endorsed by the publisher.
References
1. Szekanecz Z, McInnes IB. Autoinflammation and Autoimmunity Across Rheumatic and Musculoskeletal Diseases. Nat Rev Rheumatol (2021) 17(10):585–95. doi: 10.1038/s41584-021-00652-9
2. Gizinski AM, Fox DA. T Cell Subsets and Their Role in the Pathogenesis of Rheumatic Disease. Curr Opin Rheumatol (2014) 26(2):204–10. doi: 10.1097/bor.0000000000000036
3. Zhang F, Wei K, Slowikowski K, Fonseka CY, Rao DA, Kelly S, et al. Defining Inflammatory Cell States in Rheumatoid Arthritis Joint Synovial Tissues by Integrating Single-Cell Transcriptomics and Mass Cytometry. Nat Immunol (2019) 20(7):928–42. doi: 10.1038/s41590-019-0378-1
4. Nehar-Belaid D, Hong S, Marches R, Chen G. Mapping Systemic Lupus Erythematosus Heterogeneity at the Single-Cell Level. Nat Immunol (2020) 21(9):1094–106. doi: 10.1038/s41590-020-0743-0
5. Hong X, Meng S, Tang D, Wang T, Ding L, Yu H, et al. Single-Cell RNA Sequencing Reveals the Expansion of Cytotoxic CD4(+) T Lymphocytes and a Landscape of Immune Cells in Primary Sjögren’s Syndrome. Front Immunol (2020) 11:594658. doi: 10.3389/fimmu.2020.594658
6. Porcelli S, Yockey CE, Brenner MB, Balk SP. Analysis of T Cell Antigen Receptor (TCR) Expression by Human Peripheral Blood CD4-8- Alpha/Beta T Cells Demonstrates Preferential Use of Several V Beta Genes and an Invariant TCR Alpha Chain. J Exp Med (1993) 178(1):1–16. doi: 10.1084/jem.178.1.1
7. Tilloy F, Treiner E, Park SH, Garcia C, Lemonnier F, de la Salle H, et al. An Invariant T Cell Receptor Alpha Chain Defines a Novel TAP-Independent Major Histocompatibility Complex Class Ib-Restricted Alpha/Beta T Cell Subpopulation in Mammals. J Exp Med (1999) 189(12):1907–21. doi: 10.1084/jem.189.12.1907
8. Treiner E, Duban L, Bahram S, Radosavljevic M, Wanner V, Tilloy F, et al. Selection of Evolutionarily Conserved Mucosal-Associated Invariant T Cells by MR1. Nature (2003) 422(6928):164–9. doi: 10.1038/nature01433
9. Gherardin NA, Souter MN, Koay HF, Mangas KM, Seemann T, Stinear TP, et al. Human Blood MAIT Cell Subsets Defined Using MR1 Tetramers. Immunol Cell Biol (2018) 96(5):507–25. doi: 10.1111/imcb.12021
10. Rahimpour A, Koay HF, Enders A, Clanchy R, Eckle SB, Meehan B, et al. Identification of Phenotypically and Functionally Heterogeneous Mouse Mucosal-Associated Invariant T Cells Using MR1 Tetramers. J Exp Med (2015) 212(7):1095–108. doi: 10.1084/jem.20142110
11. Dias J, Boulouis C, Gorin JB, van den Biggelaar R, Lal KG, Gibbs A, et al. The CD4(-)CD8(-) MAIT Cell Subpopulation is a Functionally Distinct Subset Developmentally Related to the Main CD8(+) MAIT Cell Pool. Proc Natl Acad Sci U.S.A. (2018) 115(49):E11513–e11522. doi: 10.1073/pnas.1812273115
12. Kjer-Nielsen L, Patel O, Corbett AJ, Le Nours J, Meehan B, Liu L, et al. MR1 Presents Microbial Vitamin B Metabolites to MAIT Cells. Nature (2012) 491(7426):717–23. doi: 10.1038/nature11605
13. Corbett AJ, Eckle SB, Birkinshaw RW, Liu L, Patel O, Mahony J, et al. T-Cell Activation by Transitory Neo-Antigens Derived From Distinct Microbial Pathways. Nature (2014) 509(7500):361–5. doi: 10.1038/nature13160
14. Reantragoon R, Corbett AJ, Sakala IG, Gherardin NA, Furness JB, Chen Z, et al. Antigen-Loaded MR1 Tetramers Define T Cell Receptor Heterogeneity in Mucosal-Associated Invariant T Cells. J Exp Med (2013) 210(11):2305–20. doi: 10.1084/jem.20130958
15. Dusseaux M, Martin E, Serriari N, Péguillet I, Premel V, Louis D, et al. Human MAIT Cells are Xenobiotic-Resistant, Tissue-Targeted, CD161hi IL-17-Secreting T Cells. Blood (2011) 117(4):1250–9. doi: 10.1182/blood-2010-08-303339
16. Martin E, Treiner E, Duban L, Guerri L, Laude H, Toly C, et al. Stepwise Development of MAIT Cells in Mouse and Human. PloS Biol (2009) 7(3):e54. doi: 10.1371/journal.pbio.1000054
17. Koay HF, Gherardin NA, Enders A. A Three-Stage Intrathymic Development Pathway for the Mucosal-Associated Invariant T Cell Lineage. Nat Immunol (2016) 17(11):1300–11. doi: 10.1038/ni.3565
18. Dias J, Leeansyah E, Sandberg JK. Multiple Layers of Heterogeneity and Subset Diversity in Human MAIT Cell Responses to Distinct Microorganisms and to Innate Cytokines. Proc Natl Acad Sci U.S.A. (2017) 114(27):E5434–e5443. doi: 10.1073/pnas.1705759114
19. Tang XZ, Jo J, Tan AT, Sandalova E, Chia A, Tan KC, et al. IL-7 Licenses Activation of Human Liver Intrasinusoidal Mucosal-Associated Invariant T Cells. J Immunol (2013) 190(7):3142–52. doi: 10.4049/jimmunol.1203218
20. Fergusson JR, Hühn MH, Swadling L, Walker LJ, Kurioka A, Llibre A, et al. CD161(int)CD8+ T Cells: A Novel Population of Highly Functional, Memory CD8+ T Cells Enriched Within the Gut. Mucosal Immunol (2016) 9(2):401–13. doi: 10.1038/mi.2015.69
21. Gibbs A, Leeansyah E, Introini A, Paquin-Proulx D, Hasselrot K, Andersson E, et al. MAIT Cells Reside in the Female Genital Mucosa and are Biased Towards IL-17 and IL-22 Production in Response to Bacterial Stimulation. Mucosal Immunol (2017) 10(1):35–45. doi: 10.1038/mi.2016.30
22. Legoux F, Bellet D. Microbial Metabolites Control the Thymic Development of Mucosal-Associated Invariant T Cells. Science (2019) 366(6464):494–9. doi: 10.1126/science.aaw2719
23. Winter SJ, Kunze-Schumacher H, Imelmann E, Grewers Z, Osthues T, Krueger A. MicroRNA miR-181a/B-1 Controls MAIT Cell Development. Immunol Cell Biol (2019) 97(2):190–202. doi: 10.1111/imcb.12211
24. Godfrey DI, Koay HF. The Biology and Functional Importance of MAIT Cells. Nat Immunol (2019) 20(9):1110–28. doi: 10.1038/s41590-019-0444-8
25. Tao H, Pan Y. Differential Controls of MAIT Cell Effector Polarization by Mtorc1/Mtorc2 via Integrating Cytokine and Costimulatory Signals. Nat Commun (2021) 12(1):2029. doi: 10.1038/s41467-021-22162-8
26. Drashansky TT, Helm EY, Curkovic N, Cooper J, Cheng P, Chen X, et al. BCL11B is Positioned Upstream of PLZF and Rorγt to Control Thymic Development of Mucosal-Associated Invariant T Cells and MAIT17 Program. iScience (2021) 24(4):102307. doi: 10.1016/j.isci.2021.102307
27. Liu T, Wang J, Subedi K, Yi Q, Zhou L, Mi QS. MicroRNA-155 Regulates MAIT1 and MAIT17 Cell Differentiation. Front Cell Dev Biol (2021) 9:670531. doi: 10.3389/fcell.2021.670531
28. Lee M, Lee E. Single-Cell RNA Sequencing Identifies Shared Differentiation Paths of Mouse Thymic Innate T Cells. Nat Commun (2020) 11(1):4367. doi: 10.1038/s41467-020-18155-8
29. Chen Z, Wang H, D’Souza C, Sun S, Kostenko L, Eckle SB, et al. Mucosal-Associated Invariant T-Cell Activation and Accumulation After In Vivo Infection Depends on Microbial Riboflavin Synthesis and Co-Stimulatory Signals. Mucosal Immunol (2017) 10(1):58–68. doi: 10.1038/mi.2016.39
30. Legoux F, Salou M, Lantz O. MAIT Cell Development and Functions: The Microbial Connection. Immunity (2020) 53(4):710–23. doi: 10.1016/j.immuni.2020.09.009
31. Patel O, Kjer-Nielsen L, Le Nours J, Eckle SB, Birkinshaw R, Beddoe T, et al. Recognition of Vitamin B Metabolites by Mucosal-Associated Invariant T Cells. Nat Commun (2013) 4:2142. doi: 10.1038/ncomms3142
32. Le Bourhis L, Martin E, Péguillet I, Guihot A, Froux N, Coré M, et al. Antimicrobial Activity of Mucosal-Associated Invariant T Cells. Nat Immunol (2010) 11(8):701–8. doi: 10.1038/ni.1890
33. Constantinides MG, Link VM. MAIT Cells are Imprinted by the Microbiota in Early Life and Promote Tissue Repair. Science (2019) 366(6464):eaax6624. doi: 10.1126/science.aax6624
34. Leeansyah E, Loh L, Nixon DF, Sandberg JK. Acquisition of Innate-Like Microbial Reactivity in Mucosal Tissues During Human Fetal MAIT-Cell Development. Nat Commun (2014) 5:3143. doi: 10.1038/ncomms4143
35. Salou M, Franciszkiewicz K, Lantz O. MAIT Cells in Infectious Diseases. Curr Opin Immunol (2017) 48:7–14. doi: 10.1016/j.coi.2017.07.009
36. van Wilgenburg B, Scherwitzl I, Hutchinson EC. MAIT Cells are Activated During Human Viral Infections. Nat Commun (2016) 7:11653. doi: 10.1038/ncomms11653
37. Ussher JE, Bilton M, Attwod E, Shadwell J, Richardson R, de Lara C, et al. CD161++ CD8+ T Cells, Including the MAIT Cell Subset, are Specifically Activated by IL-12+IL-18 in a TCR-Independent Manner. Eur J Immunol (2014) 44(1):195–203. doi: 10.1002/eji.201343509
38. Schubert K, Karkossa I, Schor J, Engelmann B, Steinheuer LM, Bruns T, et al. A Multi-Omics Analysis of Mucosal-Associated-Invariant T Cells Reveals Key Drivers of Distinct Modes of Activation. Front Immunol (2021) 12:616967. doi: 10.3389/fimmu.2021.616967
39. Bertrand L, Lehuen A. MAIT Cells in Metabolic Diseases. Mol Metab (2019) 27s(Suppl):S114–s121. doi: 10.1016/j.molmet.2019.06.025
40. Chiba A, Murayama G, Miyake S. Mucosal-Associated Invariant T Cells in Autoimmune Diseases. Front Immunol (2018) 9:1333. doi: 10.3389/fimmu.2018.01333
41. Cogswell DT, Gapin L, Tobin HM, McCarter MD. MAIT Cells: Partners or Enemies in Cancer Immunotherapy? Cancers (Basel) (2021) 13(7):1502. doi: 10.3390/cancers13071502
42. Toubal A, Nel I, Lotersztajn S, Lehuen A. Mucosal-Associated Invariant T Cells and Disease. Nat Rev Immunol (2019) 19(10):643–57. doi: 10.1038/s41577-019-0191-y
43. Provine NM, Klenerman P. MAIT Cells in Health and Disease. Annu Rev Immunol (2020) 38:203–228. doi: 10.1146/annurev-immunol-080719-015428
44. Cho YN, Kee SJ, Kim TJ, Jin HM, Kim MJ, Jung HJ, et al. Mucosal-Associated Invariant T Cell Deficiency in Systemic Lupus Erythematosus. J Immunol (2014) 193(8):3891–901. doi: 10.4049/jimmunol.1302701
45. Chiba A, Tamura N, Yoshikiyo K, Murayama G, Kitagaichi M, Yamaji K, et al. Activation Status of Mucosal-Associated Invariant T Cells Reflects Disease Activity and Pathology of Systemic Lupus Erythematosus. Arthritis Res Ther (2017) 19(1):58. doi: 10.1186/s13075-017-1257-5
46. Murayama G, Chiba A, Suzuki H, Nomura A, Mizuno T, Kuga T, et al. A Critical Role for Mucosal-Associated Invariant T Cells as Regulators and Therapeutic Targets in Systemic Lupus Erythematosus. Front Immunol (2019) 10:2681. doi: 10.3389/fimmu.2019.02681
47. Gracey E, Qaiyum Z, Almaghlouth I, Lawson D, Karki S, Avvaru N, et al. IL-7 Primes IL-17 in Mucosal-Associated Invariant T (MAIT) Cells, Which Contribute to the Th17-Axis in Ankylosing Spondylitis. Ann Rheum Dis (2016) 75(12):2124–32. doi: 10.1136/annrheumdis-2015-208902
48. Koppejan H, Jansen D, Hameetman M, Thomas R, Toes REM, van Gaalen FA. Altered Composition and Phenotype of Mucosal-Associated Invariant T Cells in Early Untreated Rheumatoid Arthritis. Arthritis Res Ther (2019) 21(1):3. doi: 10.1186/s13075-018-1799-1
49. Raychaudhuri SK, Abria C, Mitra A, Raychaudhuri SP. Functional Significance of MAIT Cells in Psoriatic Arthritis. Cytokine (2020) 12:5154855. doi: 10.1016/j.cyto.2019.154855
50. Chiba A, Tajima R, Tomi C, Miyazaki Y, Yamamura T, Miyake S. Mucosal-Associated Invariant T Cells Promote Inflammation and Exacerbate Disease in Murine Models of Arthritis. Arthritis Rheum (2012) 64(1):153–61. doi: 10.1002/art.33314
51. Hayashi E, Chiba A, Tada K, Haga K, Kitagaichi M, Nakajima S, et al. Involvement of Mucosal-Associated Invariant T Cells in Ankylosing Spondylitis. J Rheumatol (2016) 43(9):1695–703. doi: 10.3899/jrheum.151133
52. Toussirot É, Laheurte C, Gaugler B, Gabriel D, Saas P. Increased IL-22- and IL-17a-Producing Mucosal-Associated Invariant T Cells in the Peripheral Blood of Patients With Ankylosing Spondylitis. Front Immunol (2018) 9:1610. doi: 10.3389/fimmu.2018.01610
53. Wang JJ, Macardle C, Weedon H, Beroukas D, Banovic T. Mucosal-Associated Invariant T Cells are Reduced and Functionally Immature in the Peripheral Blood of Primary Sjögren’s Syndrome Patients. Eur J Immunol (2016) 46(10):2444–53. doi: 10.1002/eji.201646300
54. Guggino G, Di Liberto D, Lo Pizzo M, Saieva L, Alessandro R, Dieli F, et al. IL-17 Polarization of MAIT Cells is Derived From the Activation of Two Different Pathways. Eur J Immunol (2017) 47(11):2002–3. doi: 10.1002/eji.201747140
55. Mekinian A, Mahevas T, Mohty M, Jachiet V, Rivière S, Fain O, et al. Mucosal-Associated Invariant Cells are Deficient in Systemic Sclerosis. Scand J Immunol (2017) 86(4):216–20. doi: 10.1111/sji.12585
56. Fazekas B, Moreno-Olivera A, Kelly Y, O’Hara P, Murray S, Kennedy A, et al. Alterations in Circulating Lymphoid Cell Populations in Systemic Small Vessel Vasculitis are non-Specific Manifestations of Renal Injury. Clin Exp Immunol (2018) 191(2):180–8. doi: 10.1111/cei.13058
57. Braudeau C, Amouriaux K, Néel A, Herbreteau G, Salabert N, Rimbert M, et al. Persistent Deficiency of Circulating Mucosal-Associated Invariant T (MAIT) Cells in ANCA-Associated Vasculitis. J Autoimmun (2016) 70:73–79. doi: 10.1016/j.jaut.2016.03.015
58. Ghesquière T, Ciudad M, Ramon A, Greigert H, Gerard C, Cladière C, et al. Mucosal-Associated Invariant T Cells in Giant Cell Arteritis. J Autoimmun (2021) 121:102652. doi: 10.1016/j.jaut.2021.102652
59. Nakajima S, Chiba A, Makiyama A, Hayashi E, Murayama G, Yamaji K, et al. Association of Mucosal-Associated Invariant T Cells With Different Disease Phases of Polymyalgia Rheumatica. Rheumatol (Oxford) (2020) 59(10):2939–46. doi: 10.1093/rheumatology/keaa054
60. Cassius C, Branchtein M, Battistella M, Amode R, Lepelletier C, Jachiet M, et al. Persistent Deficiency of Mucosal-Associated Invariant T Cells During Dermatomyositis. Rheumatol (Oxford) (2020) 59(9):2282–6. doi: 10.1093/rheumatology/kez564
61. Shi J, Zhou J, Zhang X, Hu W, Zhao JF, Wang S, et al. Single-Cell Transcriptomic Profiling of MAIT Cells in Patients With COVID-19. Front Immunol (2021) 12:700152. doi: 10.3389/fimmu.2021.700152
62. Leeansyah E, Ganesh A, Quigley MF, Sönnerborg A, Andersson J, Hunt PW, et al. Activation, Exhaustion, and Persistent Decline of the Antimicrobial MR1-Restricted MAIT-Cell Population in Chronic HIV-1 Infection. Blood (2013) 121(7):1124–35. doi: 10.1182/blood-2012-07-445429
63. Fernandez CS, Amarasena T, Kelleher AD, Rossjohn J, McCluskey J, Godfrey DI, et al. MAIT Cells are Depleted Early But Retain Functional Cytokine Expression in HIV Infection. Immunol Cell Biol (2015) 93(2):177–88. doi: 10.1038/icb.2014.91
64. Tuosto L, Cundari E, Gilardini Montani MS, Piccolella E. Analysis of Susceptibility of Mature Human T Lymphocytes to Dexamethasone-Induced Apoptosis. Eur J Immunol (1994) 24(5):1061–5. doi: 10.1002/eji.1830240508
65. Hinks TS, Wallington JC, Williams AP, Djukanović R, Staples KJ. Steroid-Induced Deficiency of Mucosal-Associated Invariant T Cells in the Chronic Obstructive Pulmonary Disease Lung. Implications for Nontypeable Haemophilus Influenzae Infection. Am J Respir Crit Care Med (2016) 194(10):1208–18. doi: 10.1164/rccm.201601-0002OC
66. Hinks TS, Zhou X, Staples KJ, Dimitrov BD, Manta A, Petrossian T, et al. Innate and Adaptive T Cells in Asthmatic Patients: Relationship to Severity and Disease Mechanisms. J Allergy Clin Immunol (2015) 136(2):323–33. doi: 10.1016/j.jaci.2015.01.014
67. Carolan E, Tobin LM, Mangan BA, Corrigan M, Gaoatswe G, Byrne G, et al. Altered Distribution and Increased IL-17 Production by Mucosal-Associated Invariant T Cells in Adult and Childhood Obesity. J Immunol (2015) 194(12):5775–80. doi: 10.4049/jimmunol.1402945
68. Hegde P, Weiss E, Paradis V, Wan J, Mabire M, Sukriti S, et al. Mucosal-Associated Invariant T Cells are a Profibrogenic Immune Cell Population in the Liver. Nat Commun (2018) 9(1):2146. doi: 10.1038/s41467-018-04450-y
69. Böttcher K, Rombouts K, Saffioti F, Roccarina D, Rosselli M, Hall A, et al. MAIT Cells are Chronically Activated in Patients With Autoimmune Liver Disease and Promote Profibrogenic Hepatic Stellate Cell Activation. Hepatology (2018) 68(1):172–86. doi: 10.1002/hep.29782
70. Lubberts E. The IL-23-IL-17 Axis in Inflammatory Arthritis. Nat Rev Rheumatol (2015) 11(7):415–29. doi: 10.1038/nrrheum.2015.53
71. Rafael-Vidal C, Pérez N, Altabás I, Garcia S. Blocking IL-17: A Promising Strategy in the Treatment of Systemic Rheumatic Diseases. Int J Mol Sci (2020) 21(19):7100. doi: 10.3390/ijms21197100
72. Croxford JL, Miyake S, Huang YY, Shimamura M, Yamamura T. Invariant V(alpha)19i T Cells Regulate Autoimmune Inflammation. Nat Immunol (2006) 7(9):987–94. doi: 10.1038/ni1370
73. Rouxel O, Da Silva J, Beaudoin L, Nel I, Tard C, Cagninacci L, et al. Cytotoxic and Regulatory Roles of Mucosal-Associated Invariant T Cells in Type 1 Diabetes. Nat Immunol (2017) 18(12):1321–31. doi: 10.1038/ni.3854
74. Ioannidis M, Cerundolo V, Salio M. The Immune Modulating Properties of Mucosal-Associated Invariant T Cells. Front Immunol (2020) 11:1556. doi: 10.3389/fimmu.2020.01556
75. Li Y, Huang B, Jiang X, Chen W, Zhang J, Wei Y, et al. Mucosal-Associated Invariant T Cells Improve Nonalcoholic Fatty Liver Disease Through Regulating Macrophage Polarization. Front Immunol (2018) 9:1994. doi: 10.3389/fimmu.2018.01994
76. Bennett MS, Trivedi S, Iyer AS, Hale JS, Leung DT. Human Mucosal-Associated Invariant T (MAIT) Cells Possess Capacity for B Cell Help. J Leukoc Biol (2017) 102(5):1261–9. doi: 10.1189/jlb.4A0317-116R
77. Abdollahi-Roodsaz S, Abramson SB, Scher JU. The Metabolic Role of the Gut Microbiota in Health and Rheumatic Disease: Mechanisms and Interventions. Nat Rev Rheumatol (2016) 12(8):446–55. doi: 10.1038/nrrheum.2016.68
78. Konig MF. The Microbiome in Autoimmune Rheumatic Disease. Best Pract Res Clin Rheumatol (2020) 34(1):101473. doi: 10.1016/j.berh.2019.101473
79. Mechelli R, Romano S. MAIT Cells and Microbiota in Multiple Sclerosis and Other Autoimmune Diseases. Nat Rev Immunol (2021) 9(6):1132. doi: 10.3390/microorganisms9061132
80. Riva A, Patel V, Kurioka A, Jeffery HC, Wright G, Tarff S, et al. Mucosa-Associated Invariant T Cells Link Intestinal Immunity With Antibacterial Immune Defects in Alcoholic Liver Disease. Gut (2018) 67(5):918–30. doi: 10.1136/gutjnl-2017-314458
81. Toubal A, Kiaf B, Beaudoin L, Cagninacci L, Rhimi M, Fruchet B, et al. Mucosal-Associated Invariant T Cells Promote Inflammation and Intestinal Dysbiosis Leading to Metabolic Dysfunction During Obesity. Nat Commun (2020) 11(1):3755. doi: 10.1038/s41467-020-17307-0
Keywords: mucosal-associated invariant T cell, rheumatic disease, systemic lupus erythematosus, rheumatoid arthritis, cytokine
Citation: Li Y, Du J and Wei W (2022) Emerging Roles of Mucosal-Associated Invariant T Cells in Rheumatology. Front. Immunol. 13:819992. doi: 10.3389/fimmu.2022.819992
Received: 22 November 2021; Accepted: 14 February 2022;
Published: 04 March 2022.
Edited by:
Agnieszka Paradowska-Gorycka, National Institute of Geriatrics, Rheumatology and Rehabilitation, PolandReviewed by:
Timothy Hinks, University of Oxford, United KingdomZhenjun Chen, The University of Melbourne, Australia
Andrew Chancellor, University of Basel, Switzerland
Copyright © 2022 Li, Du and Wei. This is an open-access article distributed under the terms of the Creative Commons Attribution License (CC BY). The use, distribution or reproduction in other forums is permitted, provided the original author(s) and the copyright owner(s) are credited and that the original publication in this journal is cited, in accordance with accepted academic practice. No use, distribution or reproduction is permitted which does not comply with these terms.
*Correspondence: Wei Wei, V2Vpd2VpX3RqbXVAMTYzLmNvbQ==
†These authors have contributed equally to this work and share first authorship