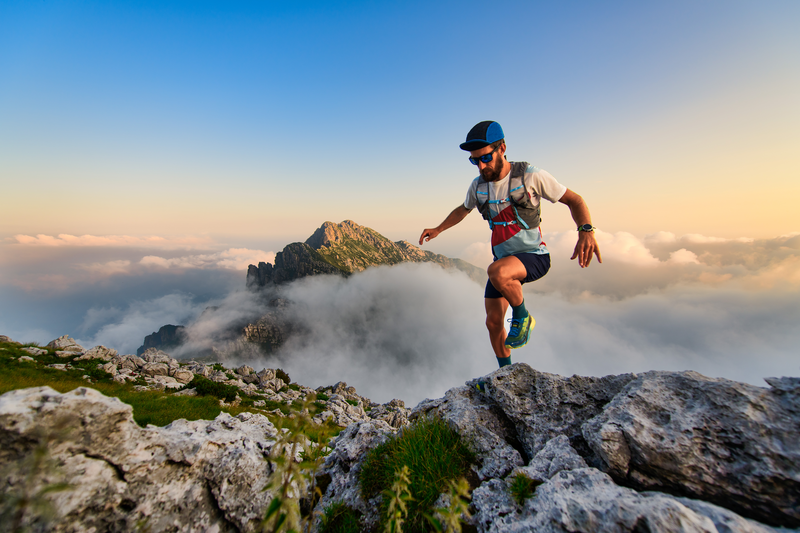
94% of researchers rate our articles as excellent or good
Learn more about the work of our research integrity team to safeguard the quality of each article we publish.
Find out more
ORIGINAL RESEARCH article
Front. Immunol. , 05 April 2022
Sec. Cytokines and Soluble Mediators in Immunity
Volume 13 - 2022 | https://doi.org/10.3389/fimmu.2022.818677
This article is part of the Research Topic IL-27 in Health and Disease View all 6 articles
In People with HIV (PWH), chronic immune activation and systemic inflammation are associated with increased risk to develop comorbidities including bone loss. Numerous cells of the immune system, namely, T cells are involved in the regulation of the bone homeostasis and osteoclasts (OCs) activity. IL-27, a cytokine that belongs to the IL-12 family can regulate the secretion of pro- and anti-inflammatory cytokines by T cells, however its role in the setting of HIV is largely unknown. In the present study, we determined the impact of OCs in T cell secretion of cytokines and whether IL-27 can regulate this function. We found that the presence of OCs in the T cell cultures significantly enhanced secretion of IFNγ, TNFα, IL-17, RANKL, and IL-10 in both PWH and healthy controls. In PWH, IL-27 inhibited IL-17 secretion and downregulated surface expression of RANKL in CD4 T cells. All together these results suggest that in the context of HIV infection IL-27 may favor IFNγ and TNFα secretion at the sites of bone remodeling.
In People with HIV (PWH), chronic immune activation and systemic inflammation are associated with an accelerated course of chronic diseases including osteoporosis, an important contributor of morbidity and mortality in these patients (1–5). Low bone mineral density (BMD) is multifactorial and represents a complex interplay between HIV infection, traditional risk factors of osteoporosis, and effects of the antiretroviral therapy. Reports have shown that HIV infection is a risk factor for low BMD in young (6–11) and older individuals (12–16). The molecular mechanisms linking HIV infection and bone disease are largely unknown.
In the bone, there is a coordinated balance between bone-forming osteoblast cells, and bone-resorbing osteoclasts (OCs) cells. OC precursors are monocyte/macrophage lineage and are recruited from the bone marrow and circulation into the sites of bone remodeling (17–23). During bone remodeling, OCs precursors undergo differentiation through signals provided by the macrophage colony-stimulating factor (M-CSF) and the receptor of activation of nuclear factor kappa B (NF-κB) (RANK) ligand (RANKL) (24). Osteoblasts were thought to be the main sources of RANKL promoting the differentiation of OCs precursors, however new evidence has determined that osteocytes produce RANKL and therefore are important players in regulating adult bone remodeling (25–28). The availability of RANKL in the microenvironment of the bone is regulated by Osteoprotegerin (OPG), which acts as a decoy receptor restricting osteoclastogenesis (17, 29–32).
Regulation of the bone homeostasis is modulated by cells of the immune system (33). B cells and T cells secrete RANKL and OPG, key factors involved in bone remodeling (34–38). Particularly, T cells are sources of RANKL and other cytokines, namely, IFNγ, TNFα, and IL-17 regulating osteoclasts differentiation and bone resorption (39). IL-17 synergizes with RANK–RANKL pathway promoting OCs activity (33, 36, 40–43). In contrast, IFNγ has dual effects by interfering with OCs differentiation; and indirectly IFNγ enhances OCs antigen presentation capacity promoting T cell activation and consequently the T cell secretion of pro-osteoclastogenic cytokines, including, RANKL and TNFα (44–47). In addition, IL-10 a regulatory cytokine has inhibitory effects both direct and indirect in osteoclastogenesis (48–51). In T cells, IL-10 has wide regulatory functions and inhibit production of pro-inflammatory cytokines (52).
In the setting of HIV infection, the role of T cell immune activation in bone homeostasis is not totally understood. Reports have shown that T cell reconstitution and immune activation is associated with bone loss (53–56). In PBMCs (Peripheral Blood Mononuclear Cells) from PWH, both CD4 and CD8 T cells express higher RANKL and decreased OPG, and its ratio is correlated with BMD suggesting a potential contribution of T cells in bone loss (53, 54, 57, 58).
IL-27 a cytokine that belongs to the IL-12 family has been shown to play a role in the bone remodeling (59). IL-27 is a heterodimer formed by the IL-27p28 chains and Epstein–Barr Virus-induced gene 3 (Ebi3) chain (60–63). IL-27 signals through a heterodimer receptor composed of IL-27Rα and gp130 and activates Janus kinase (JAK)-signal transducer and activator of transcription (STAT-1 and -3), and the mitogen activated protein kinase (MAPK) pathway (60, 63, 64). IL-27 plays a crucial role in immunity, balancing protective and inflammatory responses including development of helper T (Th)1 and inhibition of Th17 and Th2 differentiation (61, 65–67). In addition, IL-27 induces upregulation of PD-L1 expression, and generation of IL-10-producing type 1 regulatory T (Tr1) cells (64, 67–75).
In the bone, recent evidence has shown that IL-27 is expressed in both osteoblasts and osteoclasts and can directly modulate bone turnover by downregulating RANK expression (59, 76–78). In addition, in CD4 T cells, IL-27 inhibits RANKL secretion modulating their pro-osteoclastogenic function and suppressing T cell-mediated inflammatory bone destruction (79).
The impact of HIV driven T cell immune activation in the T cell–osteoclast (T:OCs) crosstalk and whether IL-27 can modulate T cell function in this setting is not well defined. In the present study, we developed an in vitro coculture system and investigated the cytokine networks involved, and their regulation by IL-27. We found that OCs significantly increased the secretion of cytokines (IL-17, TNFα, IFNγ, RANKL, and IL-10) by T cells from healthy controls and PWH. In T cells from PWH, IL-27 downregulated IL-17 and RANKL surface expression, suggesting that in the setting of HIV infection, IL-27 may favor a Th1 associated cytokines at the site of bone remodeling.
Participants were studied under a MedStar Georgetown University Hospital and an NIAID Institutional Review Board approved HIV clinical research studies. Characteristics of the study groups of PWH (n = 26) are described in Table 1. Healthy volunteers (n = 19) were obtained from the MedStar Georgetown University Hospital, and the NIH Blood Bank under an institutional review board approved protocol (Table 2). All study participants signed a written informed consent for the collection of samples.
Osteoclasts were differentiated from frozen PBMCs as described (80). Briefly, frozen PBMCs from healthy donors (HC, n = 19) and PWH (n = 26) were thawed and 1 × 106 cells were cultured in a 12-well plate in conditioned media MEM-α (Gibco, MA) containing 10% heat-inactivated FBS (Gemini, CA), and M-CSF (20 ng/ml, R&D System, MN) at 37°C and 5% CO2. Cells were fed with fresh media every three days. After 6 to 9 days of culture, osteoclast precursors were differentiated by the addition of RANKL (20 ng/ml, R&D System, MN) to the media (MEM-α containing M-CSF (20 ng/ml, R&D System, MN)). At day 6, osteoclast differentiation was determined by microscopy and measuring tartrate-resistance acid phosphatase (TRAP) activity as described (80, 81).
Cells were cultured in coverslips and fixed with 4% paraformaldehyde for 15 min and washed with PBS. Hoechst 33342 (Invitrogen, MA) and Alexa Fluor 488-Phalloidin (Molecular Probes, OR) were incubated in immunofluorescence staining buffer (PBS + 5% FBS (Gibco, MD) + 0.1% Triton X-100).
For TRAP staining reagents were purchased from Cosmo Bio Co, CA and used according to instructions of the manufacturer. Cells were stained for ~45 min with chromogenic substrate.
Hoechst and Phalloidin staining fluorescent microscopy images were collected using DAPI, GFP, and Phase Contrast filter sets (BioTek, VT). For TRAP Staining Color brightfield images of the resulting staining were collected. Both, images were collected using a Lionheart FX microscope (BioTek, VT) using a 20×/0.45 NA Plan Fluorite WD objective and Gen3.20 software.
Differentiated osteoclasts were collected by using trypsin (Corning, NY). A total of 30,000 osteoclasts were plated in an OsteoAssay™ Human Bone Plate (Lonza, CA) and cultured for 72 h at 37°C and 5% CO2. The media alone was used as background control. TRAP activity was measured in the supernatants using a TRAP solution containing a synthetic substrate (0.1 M sodium acetate (pH = 5.8), 1 mM ascorbic acid. 0.15 M KCL, 10 mM 4-Nitrophenyl Phosphate). Approximately 20 μl aliquot of culture supernatant was mixed with 80 μl TRAP solution and incubated at 37°C for 1 h. The reaction was stopped by addition 100 μl 0.3 N of NaOH. The absorbance was measured at 405 nm in a microplate reader SpectraMax iD3 (Molecular Devices, CA). The background absorbance was subtracted and the values were plotted and used to represent the activity of TRAP.
T cells were isolated from the non-adherent fraction of the osteoclast differentiation cultures and activated with CD3/CD28 mAbs coated beads (T cell TransAct™, Miltenyi Biotech, Auburn, CA). After 3 days of stimulation, IL-2 (50 U/ml, TECIN™, National Cancer Institute, Frederick, MD) was added to the media. At day 6, the phenotype of the expanded T cells was analyzed by flow cytometry. Prior to surface staining, T cells were stained with LIVE/DEAD staining (Invitrogen, MA), followed by incubation with 1 μg/ml human IgG (Sigma, MO) to block Fc receptors. Cell surface staining were performed using a cocktail of mAbs: CD3 (clone UCHT1) and CD8 (clone RPA-T8) both from BD Biosciences, CA. Cells were acquired using a BD FACS Symphony flow cytometer and analyzed using FlowJo.
T cells (100,000 cells) were cultured alone and in the presence of autologous osteoclasts (20,000 cells) at a ratio 5:1 in 96 well plates. Cells were stimulated with CD3/CD28 mAbs coated beads (Miltenyi Biotech, CA) and media as control. Cultures were performed in the presence or absence of IL-27 (50 ng/ml, PeproTech, NJ). Cells were cultured overnight at 37°C and 5% CO2 and the supernatants were collected to the analysis of cytokines IFNγ, TNFα, IL-10, IL-17A, and RANKL (LegendPlex™, Biolegend, CA).
For detection of IL-10 secreted by OCs, OCs cultured alone were in vitro stimulated with R848 (10 μM, In vivogen, CA) overnight and IL-10 measured in the supernatant (LegendPlex™, Biolegend, CA).
T cells were stimulated with CD3/CD28 mAbs overnight and stained with an IL-10 Secretion Assay-Detection Kit (Miltenyi Biotech, CA) or surface expression RANKL (clone MIH24, Biolegend, CA). Cells were acquired using a BD FACS Symphony flow cytometer and analyzed using FlowJo.
Statistical analysis was performed by GraphPad prism software. One-way ANOVA Friedman test and post hoc tests non-parametric Wilcoxon test for comparisons between the culture conditions and Mann–Whitney test for comparisons between study groups were used. Bonferroni test was used to adjust for multiple comparisons.
Correlations were performed using nonparametric Spearman correlation and p-value ≤0.01 was considered significant.
Osteoclasts (OCs) interaction with T cells leads to activation and subsequently the T-cell derived cytokines can influence OCs activity (36, 44–47). In this study, we hypothesized that in the setting of HIV infection, T cell immune activation alters the network of cytokines involved in the T cell–OCs crosstalk. To address this question, we developed an in vitro coculture system of activated T cells and in vitro differentiated autologous osteoclasts (OCs) from PBMCs to study their impact in T cell secretion of cytokines. We evaluated cytokines that has been shown to be “pro-osteoclastic”, TNFα, IL-17, and IFNγ; and IL-10 which modulates T cell function and have inhibitory properties in OCs (44–46, 48–52).
PBMCs from PWH (n = 26, red and blue symbols) have median CD4 counts of 524.5 (IQR: 442.3–678.5) cells/μl. The characteristics of the study groups is shown in Table 1. A set of participants (n = 17, Table 1, HIV+ (T), blue symbols) have measurements of bone mineral density (BMD). This group have a median age of 48 (IQR 42–53) years, median CD4 and CD8 T cell counts 525 (IQR: 449.5–689.0) and 718 (IQR: 571.0–1227.0) cells/μl respectively (Table 1, HIV+ (T)). PBMCs from healthy volunteers (n = 10, back symbols) have a median age 53 (IQR: 38 to 60) years were used as controls (Table 2).
Total T lymphocytes from healthy controls and PWH groups were expanded by TCR stimulation with CD3/CD28 mAbs. The frequency of CD4 and CD8 T cells from healthy controls were 67.6% (IQR: 34.85–73.25) and 27.80% (23.25–60.8) respectively (Figure S1A). In PWH, the frequency of CD4 and CD8 T cells were 44% (IQR: 23.8–61.85) and 51% (IQR: 29.5–68.95) respectively in PWH (Figure S1A). The frequency of expanded CD4 and CD8 T cells was not different between the groups (Figure S1A).
In vitro differentiation of OCs was monitored by microscopy for the formation of multinucleated osteoclasts by staining the actin cytoskeleton (Phallodin) and nuclei (Hoechst) before and after differentiation, and chromogenic staining for TRAP (Figures S1B, C). In addition, TRAP activity was measured in the supernatants of OCs cultured in bone plates (Figure S1D).
Activated T cells from PWH (n = 26) and healthy controls (n = 10) were cultured alone or in presence of OCs at 5:1 ratio (T cells:OCs) and stimulated with CD3/CD28 mAbs. After 24 h of culture, IFNγ, TNFα, IL-17, and IL-10 were measured in the supernatants (Figure 1). Unstimulated T cells cultured alone or in presence of OCs showed low levels of cytokine secretion in both healthy controls and PWH groups (Figures 1A-D). Similarly, unstimulated OCs cultured alone showed low basal level of cytokines secretion in the supernatants (Figure S2A).
Figure 1 T cells cocultured with osteoclasts (OCs) show enhanced secretion of IFNγ, TNFα, IL-17, and IL-10 upon TCR stimulation. Activated T cells from PWH (n = 9, red symbols, HIV+ (T) n = 17, opened blue symbols, Table 1) and healthy controls (HC, n = 10, black symbols) were cultured alone or in presence of in vitro differentiated autologous osteoclasts at a 5:1 ratio (T cell:OCs). T cells cultured in media were stimulated with CD3/CD28 mAbs overnight and the supernatants were collected to measure cytokines: (A) IFNγ, (B) TNFα, (C) IL-17, and (D) IL-10. In the graph, solid blue symbols represent individuals with viral loads >50 copies/ml. T cells cultured alone or in presence of OCs from PWH (n = 7, red symbols, HIV+ (T) n = 14, opened blue symbols, Table 1) and healthy controls (HC, n = 7, black symbols) were stimulated with CD3/CD28 mAbs in the presence and absence of IL-27 (50 ng/ml) stimulated T cells overnight and the supernatants were collected to measure cytokines: (E) IFNγ, (F) TNFα, (G) IL-17, and (H) IL-10. One-way ANOVA was used for comparisons between culture conditions. Post hoc non-parametric Wilcoxon was used for comparisons between culture conditions. Bonferroni test was used to adjust for multiple comparisons. P-value ≤0.003 was considered significant. Post hoc nonparametric unpaired Mann–Whitney test was used for comparisons between the groups adjusted by Bonferroni test. P-value ≤0.01 was considered significant.
TCR stimulation of T cells from healthy controls significantly increases secretion of IFNγ (p = 0.002), and it was enhanced by the presence of OCs in the cultures (p = 0.002) (Figure 1A). Similar effects were observed upon stimulation of T cells from PWH (p <0.001) when compared to T cells cultured alone (Figure 1A). The levels of IFNγ in the supernatants of the T cell cultures in the absence or the presence of OCs were similar between healthy controls and PWH (Figure 1A).
In addition, OCs also significantly enhanced the secretion of TNFα in healthy controls and PWH, p = 0.002 and p < 0.001 respectively (Figure 1B). Compared to stimulated T cells cultured alone, the ability of T cells to secrete IL-17A was also significantly enhanced by the presence of OCs in the cocultures in both PWH (p <0.001) and healthy controls (p = 0.006) (Figure 1C).
We next evaluated the secretion of the immunomodulatory cytokine IL-10 and asked whether OCs can modulate its secretion (47, 49–52). Stimulation of T cells in the presence of OCs significantly induced secretion of IL-10 relative to T cells cultured alone in PWH and healthy controls p <0.001 and p = 0.002 respectively (Figure 1D). T cells from PWH expressed lower basal levels of IL-10 and a trend was observed after stimulation of T cells cultured alone but did not reach statistical significance (Figure 1D).
These results suggest that OCs enhance the secretion of Th1 (IFNγ, TNFα) and Th17 (IL-17) associated cytokines in both healthy control and PWH groups. In addition to the enhancement of pro-inflammatory cytokines, OCs also promoted secretion of IL-10 in the T cell:OCs cocultures suggesting a complex interplay between OCs and T cells.
The above data suggest that OCs enhance activation of T cells promoting secretion of pro- and anti-inflammatory cytokines in the bone (33). IL-27 is an immune modulatory cytokine that inhibit OCs differentiation (76). In T cells, IL-27 promotes Th1 differentiation, suppresses Th17 differentiation and induces secretion of IL-10 by regulatory T cells (46, 49–57). Whether IL-27 can exert these functions in the setting of HIV infection is not well defined. We next evaluated the effects of IL-27 when T cells were TCR stimulated alone or in the presence of OCs (Figure 1, right panels).
IL-27 showed no effect on IFNγ and TNFα secretion by T cells from both study groups (Figures 1E, F). In contrast, IL-27 induced a significantly downregulation in IL-17 secretion in PWH but not in healthy volunteers (Figure 1G).
The secretion of IL-10 showed a trend of inhibition by IL-27, however did not reach statistical significance (Figure 1H). Because both T cells and OCs can secrete IL-10, to better understand their relative contribution and its regulation by IL-27 we examined the secretion of IL-10 in both cell types (47). T cells were evaluated using a flow cytometric cytokine capture assay in a set of HIV infected individuals (n = 10, Figures S2B, C). We found that both CD4 and CD8 T cells produce IL-10, and the frequency of IL-10+CD4 and IL-10+CD8 T cells was significantly increased in the cocultures with OCs, however IL-27 did not have an effect in either the frequency or median fluorescence intensity of IL-10 secreting T cells (Figures S2B, C). In addition, OCs cultured alone secrete low basal levels of IL-10 that was significantly increased upon stimulation with the TLR7/8 agonist in both healthy controls and PWH. In this culture condition, IL-27 showed no effect on IL-10 secretion (Figure S2D).
Altogether these data suggest that IL-27 may not modulate IFNγ, TNFα and IL-10 in activated T cells. In contrast in PWH, IL-27 showed downregulatory effect on IL-17 secretion and overcame the costimulatory effects of OCs in the cocultures.
The data above suggest that IL-27 can modulate IL-17, a cytokine that has been shown to synergized with RANKL, a major “pro-osteoclastic” factor secreted by activated T cells (59, 79, 82). To better understand the potential role of IL-27 in the regulation of cytokines in the setting of HIV infection, we evaluated RANKL expression in CD4 and CD8 T cells from PWH (n = 15) and healthy controls (n = 9) by flow cytometry (Figure 2). In PWH, both CD4 and CD8 T cells cultured alone showed upregulation of surface expression of RANKL upon TCR stimulation, and only a trend was noted in healthy controls (Figures 2A-C). The presence of OCs in the T cell cultures increased the frequency of RANKL+CD4 and CD8 T cells from PWH (Figures 2B, C). Similar observations were noted in the median fluorescence intensity of RANKL in T cells (Figures S3A, B)
Figure 2 IL-27 modulates the expression surface expression of RANKL in CD4 T cells from PWH. T cells from PWH (n = 3, red symbols, HIV+ (T) n = 12, opened blue symbols, Table 1) and healthy controls (HC, n = 9, black symbols) were cultured alone or in presence of OCs at a 5:1 ratio (T cell:OCs) were stimulated with CD3/CD28 mAbs. After overnight culture RANKL was measured by flow cytometry. (A) Gating strategy and representative contour plot of RANKL surface expression in T cells, (B) Surface expression of RANKL in CD4 T cells, and (C) Surface expression of RANKL in CD8 T cells. T cells cultured alone or in presence of OCs were stimulated with CD3/CD28 mAbs in the presence and absence of IL-27 (50 ng/ml) and RANKL surface expression was measure by flow cytometry: (D) CD4 T cells and (E) CD8 T cells. Graph representing RANKL surface expression in T cells. In the graph, solid blue symbols represent individuals with viral loads >50 copies/ml. One-way ANOVA was used for comparisons between culture conditions. Post hoc non-parametric Wilcoxon was used for comparisons between culture conditions. Bonferroni test was used to adjust for multiple comparisons. P-value ≤0.003 was considered significant. Post hoc nonparametric unpaired Mann–Whitney test was used for comparisons between the groups adjusted by Bonferroni test. P-value ≤0.01 was considered significant.
We next evaluated the effects of IL-27 on RANKL expression (Figures 2D, E). IL-27 showed an inhibitory effect on the frequency of RANKL+ T cells cultured alone from PWH (Figures 2D, E). IL-27 inhibitory effect was still observed in CD4 T cells stimulated in the presence of OCs (p <0.001, Figure 2D) but not in CD8 T cells. These effects were not observed in the median fluorescence intensity (Figures S3C, D). The effects of IL-27 on CD4 T cells were not reflected in the levels of RANKL detected in the supernatants (Figure S3E).
These data suggest that activated CD4 and CD8 T cells expressed surface RANKL and this expression is significantly enhanced by OCs in PWH. In addition, IL-27 inhibited RANKL expression in CD4 but not CD8 T cells cultured with OCs.
In the context of HIV infection, systemic inflammation and immune activation has been suggested as contributor of bone loss (83). The data above suggest that T cell function is enhanced by osteoclasts potentiating their ability to secrete pro-inflammatory cytokines, namely, IFNγ, TNFα, IL-17, RANKL, and the anti-inflammatory cytokine IL-10.
To better understand the impact of HIV infection in the bone, we investigated the relationship between cytokines and BMD (T-score), markers of systemic inflammation and coagulation, namely, CRP (C-reactive protein) and D-dimer (a bioproduct of fibrin degradation). The study participants (n = 17, Table 1 HIV+(T)) had CD4 T cell counts of CD4 of 525.0 (IQR: 449.5–689.0) cells/μl and CD8 T cell counts of 718.0 (571.0–1,227.0) cells/μl. The median T score was −0.3 (IQR: −0.95–1.55), and six out of 17 study participants had metabolic syndrome (Table 1). We found a weak trend of an inverse correlation between the T score and the ability of T cells to secrete IFNγ in absence of IL-27 (R = −0.67, p = 0.011), (Figure 3A). This inverse association was significant when T cells were cultured in the presence of IL-27 (R = −0.69, p = 0.008, Figure 3B) although the levels of IFNγ were not different between the culture conditions (Figure 1E). This association was not observed when the levels of IFNγ secretion increased as a result of the costimulatory function of the OCs (Figure 1E). In addition, the levels of IFNγ in the T cell:OCs cocultures showed a weak association with the biomarker of inflammation and coagulation D-dimer (R = 0.60, p = 0.010), (Figure 3C). No association was observed with CRP plasma levels.
Figure 3 IFNγ secretion is associated with BMD in PWH. Relationship between IFNγ secretion and BMD in T cells cultured: (A) alone (n=17), (B) in presence of IL-27 (n = 14). (C) Relationship between IFNγ secretion in the T cell:OCs cocultures (n = 17) and D-dimer. Correlations were performed using nonparametric Spearman correlation and p-value ≤0.01 was considered significant.
Low bone mineral density has been associated with higher lipid levels (84–88). Because some of the study participants had metabolic syndrome, we evaluated the relationship between lipids and cytokines. Total cholesterol and LDL and showed a positive trend with the levels of TNFα secretion by T cells (R = 0.60, p = 0.016 and R = 0.60, p = 0.012 respectively) but did not reach statistical significance (Figure S3F).
These data suggest that Th1 cytokines (IFNγ and TNFα) in PWH may be a contributing factor in maintaining levels of inflammation and increased risk of bone loss.
In PWH, systemic inflammation and immune activation is associated with comorbidities including bone loss. In the present study, in an in vitro coculture system of T cells and OCs from PWH and healthy controls, we evaluated the effect of T cell immune activation on cytokine secretion, and the potential regulatory effect of IL-27. We found that OCs can significantly enhance T cell function promoting the secretion of both pro- (IFNγ, TNFα, IL-17) and anti-inflammatory (IL-10) cytokines in healthy controls and PWH. In addition, the presence of OCs in the cocultures increased T cell surface expression of RANKL, a major pro-osteoclastic factor secreted by T cells. IL-27 showed no regulatory effects on the secretion of IFNγ, TNFα, and IL-10 by activated T cells. In contrast, IL-27 inhibited the secretion of IL-17 and surface RANKL expression in CD4 T cells from PWH. All together these results suggest that in the context of HIV infection IL-27 may favor Th1 associated cytokines (IFNγ and TNFα).
IL-27 has been shown to have direct and indirect effects in bone remodeling. In vitro, IL-27 inhibits RANKL dependent OCs differentiation of human monocytes and also inhibits bone resorption suggesting an anti-inflammatory function in bone homeostasis (76, 77, 89). In vivo, in a murine model of collagen-induced arthritis, IL-27 induced Th1 differentiation and IFNγ secretion constraining osteoclast differentiation (90–93). In contrast, IFNγ can stimulate osteoclast formation by promoting T cell activation and secretion of pro-osteoclastogenic factors including RANKL and TNFα leading to bone loss in in vivo models of bone resorption, namely, ovariectomy, LPS injection, and inflammation (45, 59). Our present study shows that in vitro OCs have a costimulatory effect on secretion of cytokines including IFNγ. The negative association of IFNγ levels with T-score may suggest a potential contribution of T cell immune activation. One limitation of this study is that the participants have normal T scores and future studies should further investigate the cytokine secretion networks involved in PWH with low BMD.
IL-27 showed no effects in the regulation of IFNγ in the cocultures. In our recent report we found that IL-27 promotes secretion of IFNγ in TIGIT+HIVGag specific T cells by upregulation of T-bet, a transcription factor associated with Th1 differentiation (94). It is possible that in the present studies the effects of IL-27 on IFNγ secretion were not fully appreciated in polyclonally activated T cells from healthy controls and PWH. In addition, recent reports have shown that OCs can be infected by HIV-1 (95, 96). OCs also can act as antigen presenting cells and activate CD4 and CD8 T cells (47). In this scenario, the interaction of T cells and OCs may contribute to maintain T cell immune activation, secretion of pro-osteoclastogenic cytokines and viral dissemination impacting bone homeostasis in PWH.
In contrast to IFNγ and TNFα, IL-27 inhibited IL-17 secretion in T cells cocultured with OCs. Th17 cells also produce RANKL and promote IL-17-dependent osteoclast differentiation by increasing expression of RANK receptor and promoting secretion of RANKL by osteoblasts (82, 97, 98). In our studies we found that IL-27 inhibited RANKL in CD4 T cells suggesting that IL-27 may has an anti-inflammatory effect during T cell interaction with OCs, whether RANKL inhibition was achieved in IFNγ and/or IL-17 secreting T cells needs to be determined.
IL-27 has been suggested to have an anti-inflammatory function; and in our in vitro coculture system, we found that in the context of HIV infection favors a Th1 associated cytokines by suppressing IL-17 secretion. The levels of IL-27 during HIV infection seems to be unmodulated; similar plasma levels have been reported in untreated, and successfully suppressed viremia with cART PWH, and healthy controls (99). In contrast, two small studies reported contradictory changes of plasma levels of IL-27 during HIV infection (100, 101).
In addition, to its immunomodulatory functions, in vitro IL-27 has antiviral properties, and inhibits HIV replication in CD4 T cells, monocyte-derived macrophages, and dendritic cells (102–104). It has been determined that OCs can be infected by HIV promoting their differentiation, whether IL-27 can protect OCs from HIV infection is largely unknown (95, 96).
Future studies should evaluate the expression of IL-27 in the bone and its potential overall impact on OCs activity at the sites of bone remodeling in PWH.
The original contributions presented in the study are included in the article/Supplementary Material. Further inquiries can be directed to the corresponding author.
The studies involving human participants were reviewed and approved by the MedStar Georgetown University Hospital and a NIAID Institutional Review Board. The patients/participants provided their written informed consent to participate in this study.
MC designed the study. TL performed the experiments. MC and TL analyzed and interpreted the data and write the manuscript. CH, JK, and PK were involved in recruitment of participants of the study and write the manuscript. JMW stained and imaged osteoclasts. JQ contributed with the statistical analysis. All authors listed have made a substantial, direct, and intellectual contribution to the work and approved it for publication.
This work supported by the Leidos Biomedical Research, Inc. has been funded in whole or in part with federal funds from the National Cancer Institute, NIH, under Contract HHSN261200800001E. The content of this publication does not necessarily reflect the views or policies of the Department of Health and Human Services, nor does mention of trade names, commercial products, or organizations imply endorsement by the U.S. Government. The funder was not involved in the study design, collection, analysis, interpretation of data, the writing of this article or the decision to submit it for publication. MC is also supported in part by the National Institute of Allergy and Infectious Diseases of the National Institutes of Health under award number NIH R01AI145549-02 and by the District of Columbia Center for AIDS Research, an NIH funded program (P30AI117970) which is supported by the following NIH Co-Funding and Participating Institutes and Centers: NIAID, NCI, NICHD, NHLBI, NIDA, NIMH, NIA, NIDDK, NIMHD, NIDCR, NINR, FIC and OAR. TGM is supported by the Intramural Research Program of the NIH. The work of JW was supported by the Intramural Research Program of the Eunice Kennedy Shriver National Institute of Child Health and Human Development, National Institutes of Health (ZIA Act HD001501 to L.V. Chernomordik).
The authors declare that the research was conducted in the absence of any commercial or financial relationships that could be construed as a potential conflict of interest.
All claims expressed in this article are solely those of the authors and do not necessarily represent those of their affiliated organizations, or those of the publisher, the editors and the reviewers. Any product that may be evaluated in this article, or claim that may be made by its manufacturer, is not guaranteed or endorsed by the publisher.
We thank Dr. Chernomordik for discussions and critical review of the manuscript.
The Supplementary Material for this article can be found online at: https://www.frontiersin.org/articles/10.3389/fimmu.2022.818677/full#supplementary-material
1. Yin MT, Zhang CA, McMahon DJ, Ferris DC, Irani D, Colon I, et al. Higher Rates of Bone Loss in Postmenopausal HIV-Infected Women: A Longitudinal Study. J Clin Endocrinol Metab (2012) 97:554–62. doi: 10.1210/jc.2011-2197
2. Amiel C, Ostertag A, Slama L, Baudoin C, N'Guyen T, Lajeunie E, et al. BMD is Reduced in HIV-Infected Men Irrespective of Treatment. J Bone Miner Res (2004) 19:402–9. doi: 10.1359/JBMR.0301246
3. Brown TT, Guaraldi G. Multimorbidity and Burden of Disease. Interdiscip Top Gerontol Geriatr (2017) 42:59–73. doi: 10.1159/000448544
4. Alvarez E, Belloso WH, Boyd MA, Inkaya AC, Hsieh E, Kambugu A, et al. Which HIV Patients Should be Screened for Osteoporosis: An International Perspective. Curr Opin HIV AIDS (2016) 11:268–76. doi: 10.1097/COH.0000000000000269
5. de Menezes EG, Machado AA, Barbosa F Jr., de Paula FJ, Navarro AM. Bone Metabolism Dysfunction Mediated by the Increase of Proinflammatory Cytokines in Chronic HIV Infection. J Bone Miner Metab (2017) 35:234–42. doi: 10.1007/s00774-016-0749-8
6. Bruera D, Luna N, David DO, Bergoglio LM, Zamudio J. Decreased Bone Mineral Density in HIV-Infected Patients is Independent of Antiretroviral Therapy. AIDS (London England) (2003) 17:1917–23. doi: 10.1097/00002030-200309050-00010
7. Carr A, Miller J, Eisman JA, Cooper DA. Osteopenia in HIV-Infected Men: Association With Asymptomatic Lactic Acidemia and Lower Weight Pre-Antiretroviral Therapy. AIDS (London England) (2001) 15:703–9. doi: 10.1097/00002030-200104130-00005
8. Dolan SE, Huang JS, Killilea KM, Sullivan MP, Aliabadi N, Grinspoon S. Reduced Bone Density in HIV-Infected Women. AIDS (London England) (2004) 18:475–83. doi: 10.1097/00002030-200402200-00014
9. Knobel H, Guelar A, Vallecillo G, Nogues X, Diez A. Osteopenia in HIV-Infected Patients: Is it the Disease or is it the Treatment? AIDS (London England) (2001) 15:807–8. doi: 10.1097/00002030-200104130-00022
10. Tebas P, Powderly W, Yarasheski K. Response to 'Accelerated Bone Mineral Loss in HIV-Infected Patients Receiving Potent Antiretroviral Therapy' by Drs Weil and Lenhard. AIDS (London England) (2000) 14:2417. doi: 10.1097/00002030-200010200-00031
11. Teichmann J, Stephan E, Lange U, Discher T, Friese G, Lohmeyer J, et al. Osteopenia in HIV-Infected Women Prior to Highly Active Antiretroviral Therapy. J Infect (2003) 46:221–7. doi: 10.1053/jinf.2002.1109
12. Arnsten JH, Freeman R, Howard AA, Floris-Moore M, Lo Y, Klein RS. Decreased Bone Mineral Density and Increased Fracture Risk in Aging Men With or at Risk for HIV Infection. AIDS (London England) (2007) 21:617–23. doi: 10.1097/QAD.0b013e3280148c05
13. Arnsten JH, Freeman R, Howard AA, Floris-Moore M, Santoro N, Schoenbaum EE. HIV Infection and Bone Mineral Density in Middle-Aged Women. Clin Infect Dis (2006) 42:1014–20. doi: 10.1086/501015
14. Jones S, Restrepo D, Kasowitz A, Korenstein D, Wallenstein S, Schneider A, et al. Risk Factors for Decreased Bone Density and Effects of HIV on Bone in the Elderly. Osteoporos Int (2008) 19:913–8. doi: 10.1007/s00198-007-0524-8
15. Yin M, Dobkin J, Brudney K, Becker C, Zadel JL, Manandhar M, et al. Bone Mass and Mineral Metabolism in HIV+ Postmenopausal Women. Osteoporos Int (2005) 16:1345–52. doi: 10.1007/s00198-005-1845-0
16. Guerri-Fernandez R, Vestergaard P, Carbonell C, Knobel H, Aviles FF, Castro AS, et al. HIV Infection is Strongly Associated With Hip Fracture Risk, Independently of Age, Gender, and Comorbidities: A Population-Based Cohort Study. J Bone Miner Res (2013) 28:1259–63. doi: 10.1002/jbmr.1874
17. Lacey DL, Timms E, Tan HL, Kelley MJ, Dunstan CR, Burgess T, et al. Osteoprotegerin Ligand is a Cytokine That Regulates Osteoclast Differentiation and Activation. Cell (1998) 93:165–76. doi: 10.1016/S0092-8674(00)81569-X
18. Yasuda H, Shima N, Nakagawa N, Yamaguchi K, Kinosaki M, Mochizuki S, et al. Osteoclast Differentiation Factor is a Ligand for Osteoprotegerin/Osteoclastogenesis-Inhibitory Factor and is Identical to TRANCE/RANKL. Proc Natl Acad Sci U S A (1998) 95:3597–602. doi: 10.1073/pnas.95.7.3597
19. Fuller K, Wong B, Fox S, Choi Y, Chambers TJ. TRANCE is Necessary and Sufficient for Osteoblast-Mediated Activation of Bone Resorption in Osteoclasts. J Exp Med (1998) 188:997–1001. doi: 10.1084/jem.188.5.997
20. Kahn AJ, Simmons DJ. Investigation of Cell Lineage in Bone Using a Chimaera of Chick and Quial Embryonic Tissue. Nature (1975) 258:325–7. doi: 10.1038/258325a0
21. Walker DG. Bone Resorption Restored in Osteopetrotic Mice by Transplants of Normal Bone Marrow and Spleen Cells. Science (New York N Y (1975) 190:784–5. doi: 10.1126/science.1105786
22. Walker DG. Spleen Cells Transmit Osteopetrosis in Mice. Science (New York N Y (1975) 190:785–7. doi: 10.1126/science.1198094
23. Matsuzaki K, Udagawa N, Takahashi N, Yamaguchi K, Yasuda H, Shima N, et al. Osteoclast Differentiation Factor (ODF) Induces Osteoclast-Like Cell Formation in Human Peripheral Blood Mononuclear Cell Cultures. Biochem Biophys Res Commun (1998) 246:199–204. doi: 10.1006/bbrc.1998.8586
24. Boyle WJ, Simonet WS, Lacey DL. Osteoclast Differentiation and Activation. Nature (2003) 423:337–42. doi: 10.1038/nature01658
25. Xiong J, Onal M, Jilka RL, Weinstein RS, Manolagas SC, O'Brien CA. Matrix-Embedded Cells Control Osteoclast Formation. Nat Med (2011) 17:1235–41. doi: 10.1038/nm.2448
26. Nakashima T, Hayashi M, Fukunaga T, Kurata K, Oh-Hora M, Feng JQ, et al. Evidence for Osteocyte Regulation of Bone Homeostasis Through RANKL Expression. Nat Med (2011) 17:1231–4. doi: 10.1038/nm.2452
27. Wilson C. Osteocytes, RANKL and Bone Loss. Nat Rev Endocrinol (2011) 7:693. doi: 10.1038/nrendo.2011.176
28. Xiong J, O'Brien CA. Osteocyte RANKL: New Insights Into the Control of Bone Remodeling. J Bone Miner Res (2012) 27:499–505. doi: 10.1002/jbmr.1547
29. Teitelbaum SL. Bone Resorption by Osteoclasts. Science (New York N Y (2000) 289:1504–8. doi: 10.1126/science.289.5484.1504
30. Min H, Morony S, Sarosi I, Dunstan CR, Capparelli C, Scully S, et al. Osteoprotegerin Reverses Osteoporosis by Inhibiting Endosteal Osteoclasts and Prevents Vascular Calcification by Blocking a Process Resembling Osteoclastogenesis. J Exp Med (2000) 192:463–74. doi: 10.1084/jem.192.4.463
31. Hofbauer LC, Khosla S, Dunstan CR, Lacey DL, Boyle WJ, Riggs BL. The Roles of Osteoprotegerin and Osteoprotegerin Ligand in the Paracrine Regulation of Bone Resorption. J Bone Miner Res (2000) 15:2–12. doi: 10.1359/jbmr.2000.15.1.2
32. Simonet WS, Lacey DL, Dunstan CR, Kelley M, Chang MS, Luthy R, et al. Osteoprotegerin: A Novel Secreted Protein Involved in the Regulation of Bone Density. Cell (1997) 89:309–19. doi: 10.1016/S0092-8674(00)80209-3
33. Weitzmann MN. Bone and the Immune System. Toxicol Pathol (2017) 45:911–24. doi: 10.1177/0192623317735316
34. Anderson DM, Maraskovsky E, Billingsley WL, Dougall WC, Tometsko ME, Roux ER, et al. A Homologue of the TNF Receptor and its Ligand Enhance T-Cell Growth and Dendritic-Cell Function. Nature (1997) 390:175–9. doi: 10.1038/36593
35. Feng X, Teitelbaum SL. Osteoclasts: New Insights. Bone Res (2013) 1:11–26. doi: 10.4248/BR201301003
36. Weitzmann MN, Ofotokun I. Physiological and Pathophysiological Bone Turnover - Role of the Immune System. Nat Rev Endocrinol (2016) 12:518–32. doi: 10.1038/nrendo.2016.91
37. Li Y, Toraldo G, Li A, Yang X, Zhang H, Qian WP, et al. B Cells and T Cells are Critical for the Preservation of Bone Homeostasis and Attainment of Peak Bone Mass In Vivo. Blood (2007) 109:3839–48. doi: 10.1182/blood-2006-07-037994
38. Pacifici R. T Cells, Osteoblasts, and Osteocytes: Interacting Lineages Key for the Bone Anabolic and Catabolic Activities of Parathyroid Hormone. Ann N Y Acad Sci (2016) 1364:11–24. doi: 10.1111/nyas.12969
39. Weitzmann MN, Pacifici R. T Cells: Unexpected Players in the Bone Loss Induced by Estrogen Deficiency and in Basal Bone Homeostasis. Ann N Y Acad Sci (2007) 1116:360–75. doi: 10.1196/annals.1402.068
40. Redlich K, Smolen JS. Inflammatory Bone Loss: Pathogenesis and Therapeutic Intervention. Nat Rev Drug Discov (2012) 11:234–50. doi: 10.1038/nrd3669
41. Fuller K, Murphy C, Kirstein B, Fox SW, Chambers TJ. TNFalpha Potently Activates Osteoclasts, Through a Direct Action Independent of and Strongly Synergistic With RANKL. Endocrinology (2002) 143:1108–18. doi: 10.1210/endo.143.3.8701
42. Sato K, Suematsu A, Okamoto K, Yamaguchi A, Morishita Y, Kadono Y, et al. Th17 Functions as an Osteoclastogenic Helper T Cell Subset That Links T Cell Activation and Bone Destruction. J Exp Med (2006) 203:2673–82. doi: 10.1084/jem.20061775
43. Gravallese EM, Schett G. Effects of the IL-23-IL-17 Pathway on Bone in Spondyloarthritis. Nat Rev Rheumatol (2018) 14:631–40. doi: 10.1038/s41584-018-0091-8
44. Takayanagi H, Ogasawara K, Hida S, Chiba T, Murata S, Sato K, et al. T-Cell-Mediated Regulation of Osteoclastogenesis by Signalling Cross-Talk Between RANKL and IFN-Gamma. Nature (2000) 408:600–5. doi: 10.1038/35046102
45. Gao Y, Grassi F, Ryan MR, Terauchi M, Page K, Yang X, et al. IFN-Gamma Stimulates Osteoclast Formation and Bone Loss In Vivo via Antigen-Driven T Cell Activation. J Clin Invest (2007) 117:122–32. doi: 10.1172/JCI30074
46. Mbalaviele G, Novack DV, Schett G, Teitelbaum SL. Inflammatory Osteolysis: A Conspiracy Against Bone. J Clin Invest (2017) 127:2030–9. doi: 10.1172/JCI93356
47. Li H, Hong S, Qian J, Zheng Y, Yang J, Yi Q. Cross Talk Between the Bone and Immune Systems: Osteoclasts Function as Antigen-Presenting Cells and Activate CD4+ and CD8+ T Cells. Blood (2010) 116:210–7. doi: 10.1182/blood-2009-11-255026
48. Lovibond AC, Haque SJ, Chambers TJ, Fox SW. TGF-Beta-Induced SOCS3 Expression Augments TNF-Alpha-Induced Osteoclast Formation. Biochem Biophys Res Commun (2003) 309:762–7. doi: 10.1016/j.bbrc.2003.08.068
49. Shin HH, Lee JE, Lee EA, Kwon BS, Choi HS. Enhanced Osteoclastogenesis in 4-1BB-Deficient Mice Caused by Reduced Interleukin-10. J Bone Miner Res (2006) 21:1907–12. doi: 10.1359/jbmr.060813
50. Dresner-Pollak R, Gelb N, Rachmilewitz D, Karmeli F, Weinreb M. Interleukin 10-Deficient Mice Develop Osteopenia, Decreased Bone Formation, and Mechanical Fragility of Long Bones. Gastroenterology (2004) 127:792–801. doi: 10.1053/j.gastro.2004.06.013
51. Xu LX, Kukita T, Kukita A, Otsuka T, Niho Y, Iijima T. Interleukin-10 Selectively Inhibits Osteoclastogenesis by Inhibiting Differentiation of Osteoclast Progenitors Into Preosteoclast-Like Cells in Rat Bone Marrow Culture System. J Cell Physiol (1995) 165:624–9. doi: 10.1002/jcp.1041650321
52. Mosser DM, Zhang X. Interleukin-10: New Perspectives on an Old Cytokine. Immunol Rev (2008) 226:205–18. doi: 10.1111/j.1600-065X.2008.00706.x
53. Ofotokun I, Titanji K, Vikulina T, Roser-Page S, Yamaguchi M, Zayzafoon M, et al. Role of T-Cell Reconstitution in HIV-1 Antiretroviral Therapy-Induced Bone Loss. Nat Commun (2015) 6:8282. doi: 10.1038/ncomms9282
54. Weitzmann MN, Vikulina T, Roser-Page S, Yamaguchi M, Ofotokun I. Homeostatic Expansion of CD4+ T Cells Promotes Cortical and Trabecular Bone Loss, Whereas CD8+ T Cells Induce Trabecular Bone Loss Only. J Infect Dis (2017) 216:1070–9. doi: 10.1093/infdis/jix444
55. Gazzola L, Bellistri GM, Tincati C, Ierardi V, Savoldi A, Del Sole A, et al. Association Between Peripheral T-Lymphocyte Activation and Impaired Bone Mineral Density in HIV-Infected Patients. J Trans Med (2013) 11:51. doi: 10.1186/1479-5876-11-51
56. Ofotokun I, McIntosh E, Weitzmann MN. HIV: Inflammation and Bone. Curr HIV/AIDS Rep (2012) 9:16–25. doi: 10.1007/s11904-011-0099-z
57. Titanji K, Vunnava A, Sheth AN, Delille C, Lennox JL, Sanford SE, et al. Dysregulated B Cell Expression of RANKL and OPG Correlates With Loss of Bone Mineral Density in HIV Infection. PloS Pathog (2014) 10:e1004497. doi: 10.1371/journal.ppat.1004497
58. Titanji K, Vunnava A, Foster A, Sheth AN, Lennox JL, Knezevic A, et al. T-Cell Receptor Activator of Nuclear factor-kappaB Ligand/Osteoprotegerin Imbalance is Associated With HIV-Induced Bone Loss in Patients With Higher CD4+ T-Cell Counts. AIDS (London England) (2018) 32:885–94. doi: 10.1097/QAD.0000000000001764
59. Adamopoulos IE, Pflanz S. The Emerging Role of Interleukin 27 in Inflammatory Arthritis and Bone Destruction. Cytokine Growth Factor Rev (2013) 24:115–21. doi: 10.1016/j.cytogfr.2012.10.001
60. Kastelein RA, Hunter CA, Cua DJ. Discovery and Biology of IL-23 and IL-27: Related But Functionally Distinct Regulators of Inflammation. Annu Rev Immunol (2007) 25:221–42. doi: 10.1146/annurev.immunol.22.012703.104758
61. Pflanz S, Timans JC, Cheung J, Rosales R, Kanzler H, Gilbert J, et al. IL-27, a Heterodimeric Cytokine Composed of EBI3 and P28 Protein, Induces Proliferation of Naive CD4+ T Cells. Immunity (2002) 16:779–90. doi: 10.1016/S1074-7613(02)00324-2
62. Devergne O, Hummel M, Koeppen H, Le Beau MM, Nathanson EC, Kieff E, et al. A Novel Interleukin-12 P40-Related Protein Induced by Latent Epstein-Barr Virus Infection in B Lymphocytes. J Virol (1996) 70:1143–53. doi: 10.1128/jvi.70.2.1143-1153.1996
63. Tait Wojno ED, Hunter CA, Stumhofer JS. The Immunobiology of the Interleukin-12 Family: Room for Discovery. Immunity (2019) 50:851–70. doi: 10.1016/j.immuni.2019.03.011
64. Hall AO, Silver JS, Hunter CA. The Immunobiology of IL-27. Adv Immunol (2012) 115:1–44. doi: 10.1016/B978-0-12-394299-9.00001-1
65. Takeda A, Hamano S, Yamanaka A, Hanada T, Ishibashi T, Mak TW, et al. Cutting Edge: Role of IL-27/WSX-1 Signaling for Induction of T-Bet Through Activation of STAT1 During Initial Th1 Commitment. J Immunol (2003) 170:4886–90. doi: 10.4049/jimmunol.170.10.4886
66. Owaki T, Asakawa M, Fukai F, Mizuguchi J, Yoshimoto T. IL-27 Induces Th1 Differentiation via P38 MAPK/T-Bet- and Intercellular Adhesion Molecule-1/LFA-1/ERK1/2-Dependent Pathways. J Immunol (2006) 177:7579–87. doi: 10.4049/jimmunol.177.11.7579
67. Hunter CA, Kastelein R. Interleukin-27: Balancing Protective and Pathological Immunity. Immunity (2012) 37:960–9. doi: 10.1016/j.immuni.2012.11.003
68. Bosmann M, Ward PA. Modulation of Inflammation by Interleukin-27. J Leukoc Biol (2013) 94:1159–65. doi: 10.1189/jlb.0213107
69. Seita J, Asakawa M, Ooehara J, Takayanagi S, Morita Y, Watanabe N, et al. Interleukin-27 Directly Induces Differentiation in Hematopoietic Stem Cells. Blood (2008) 111:1903–12. doi: 10.1182/blood-2007-06-093328
70. Artis D, Villarino A, Silverman M, He W, Thornton EM, Mu S, et al. The IL-27 Receptor (WSX-1) is an Inhibitor of Innate and Adaptive Elements of Type 2 Immunity. J Immunol (2004) 173:5626–34. doi: 10.4049/jimmunol.173.9.5626
71. Fitzgerald DC, Ciric B, Touil T, Harle H, Grammatikopolou J, Das Sarma J, et al. Suppressive Effect of IL-27 on Encephalitogenic Th17 Cells and the Effector Phase of Experimental Autoimmune Encephalomyelitis. J Immunol (2007) 179:3268–75. doi: 10.4049/jimmunol.179.5.3268
72. Stumhofer JS, Silver JS, Laurence A, Porrett PM, Harris TH, Turka LA, et al. Interleukins 27 and 6 Induce STAT3-Mediated T Cell Production of Interleukin 10. Nat Immunol (2007) 8:1363–71. doi: 10.1038/ni1537
73. Villarino AV, Gallo E, Abbas AK. STAT1-Activating Cytokines Limit Th17 Responses Through Both T-Bet-Dependent and -Independent Mechanisms. J Immunol (2010) 185:6461–71. doi: 10.4049/jimmunol.1001343
74. Anderson CF, Stumhofer JS, Hunter CA, Sacks D. IL-27 Regulates IL-10 and IL-17 From CD4+ Cells in Nonhealing Leishmania Major Infection. J Immunol (2009) 183:4619–27. doi: 10.4049/jimmunol.0804024
75. Zhang H, Madi A, Yosef N, Chihara N, Awasthi A, Pot C, et al. An IL-27-Driven Transcriptional Network Identifies Regulators of IL-10 Expression Across T Helper Cell Subsets. Cell Rep (2020) 33:108433. doi: 10.1016/j.celrep.2020.108433
76. Furukawa M, Takaishi H, Takito J, Yoda M, Sakai S, Hikata T, et al. IL-27 Abrogates Receptor Activator of NF-Kappa B Ligand-Mediated Osteoclastogenesis of Human Granulocyte-Macrophage Colony-Forming Unit Cells Through STAT1-Dependent Inhibition of C-Fos. J Immunol (2009) 183:2397–406. doi: 10.4049/jimmunol.0802091
77. Kalliolias GD, Zhao B, Triantafyllopoulou A, Park-Min KH, Ivashkiv LB. Interleukin-27 Inhibits Human Osteoclastogenesis by Abrogating RANKL-Mediated Induction of Nuclear Factor of Activated T Cells C1 and Suppressing Proximal RANK Signaling. Arthritis Rheum (2010) 62:402–13. doi: 10.1002/art.27200
78. Larousserie F, Bsiri L, Dumaine V, Dietrich C, Audebourg A, Radenen-Bussiere B, et al. Frontline Science: Human Bone Cells as a Source of IL-27 Under Inflammatory Conditions: Role of TLRs and Cytokines. J Leukoc Biol (2017) 101:1289–300. doi: 10.1189/jlb.3HI0616-280R
79. Kamiya S, Okumura M, Chiba Y, Fukawa T, Nakamura C, Nimura N, et al. IL-27 Suppresses RANKL Expression in CD4+ T Cells in Part Through STAT3. Immunol Lett (2011) 138:47–53. doi: 10.1016/j.imlet.2011.02.022
80. Henriksen K, Karsdal MA, Taylor A, Tosh D, Coxon FP. Generation of Human Osteoclasts From Peripheral Blood. Methods Mol Biol (2012) 816:159–75. doi: 10.1007/978-1-61779-415-5_11
81. Ghayor C, Correro RM, Lange K, Karfeld-Sulzer LS, Gratz KW, Weber FE. Inhibition of Osteoclast Differentiation and Bone Resorption by N-Methylpyrrolidone. J Biol Chem (2011) 286:24458–66. doi: 10.1074/jbc.M111.223297
82. Adamopoulos IE, Chao CC, Geissler R, Laface D, Blumenschein W, Iwakura Y, et al. Interleukin-17A Upregulates Receptor Activator of NF-kappaB on Osteoclast Precursors. Arthritis Res Ther (2010) 12:R29. doi: 10.1186/ar2936
83. McComsey GA, Tebas P, Shane E, Yin MT, Overton ET, Huang JS, et al. Bone Disease in HIV Infection: A Practical Review and Recommendations for HIV Care Providers. Clin Infect Dis (2010) 51:937–46. doi: 10.1086/656412
84. Poli A, Bruschi F, Cesana B, Rossi M, Paoletti R, Crosignani PG, et al. Plasma Low-Density Lipoprotein Cholesterol and Bone Mass Densitometry in Postmenopausal Women. Obstet Gynecol (2003) 102:922–6. doi: 10.1016/j.obstetgynecol.2003.07.004
85. Makovey J, Chen JS, Hayward C, Williams FM, Sambrook PN. Association Between Serum Cholesterol and Bone Mineral Density. Bone (2009) 44:208–13. doi: 10.1016/j.bone.2008.09.020
86. Kim KC, Shin DH, Lee SY, Im JA, Lee DC. Relation Between Obesity and Bone Mineral Density and Vertebral Fractures in Korean Postmenopausal Women. Yonsei Med J (2010) 51:857–63. doi: 10.3349/ymj.2010.51.6.857
87. Kim YH, Nam GE, Cho KH, Choi YS, Kim SM, Han BD, et al. Low Bone Mineral Density is Associated With Dyslipidemia in South Korean Men: The 2008-2010 Korean National Health and Nutrition Examination Survey. Endocr J (2013) 60:1179–89. doi: 10.1507/endocrj.EJ13-0224
88. Jeong TD, Lee W, Choi SE, Kim JS, Kim HK, Bae SJ, et al. Relationship Between Serum Total Cholesterol Level and Serum Biochemical Bone Turnover Markers in Healthy Pre- and Postmenopausal Women. BioMed Res Int (2014) 2014:398397. doi: 10.1155/2014/398397
89. Kamiya S, Nakamura C, Fukawa T, Ono K, Ohwaki T, Yoshimoto T, et al. Effects of IL-23 and IL-27 on Osteoblasts and Osteoclasts: Inhibitory Effects on Osteoclast Differentiation. J Bone Miner Metab (2007) 25:277–85. doi: 10.1007/s00774-007-0766-8
90. Hibbert L, Pflanz S, De Waal Malefyt R, Kastelein RA. IL-27 and IFN-Alpha Signal via Stat1 and Stat3 and Induce T-Bet and IL-12Rbeta2 in Naive T Cells. J Interferon Cytokine Res (2003) 23:513–22. doi: 10.1089/10799900360708632
91. Lucas S, Ghilardi N, Li J, de Sauvage FJ. IL-27 Regulates IL-12 Responsiveness of Naive CD4+ T Cells Through Stat1-Dependent and -Independent Mechanisms. Proc Natl Acad Sci U S A (2003) 100:15047–52. doi: 10.1073/pnas.2536517100
92. Yoshida H, Hamano S, Senaldi G, Covey T, Faggioni R, Mu S, et al. WSX-1 is Required for the Initiation of Th1 Responses and Resistance to L. Major Infection. Immunity (2001) 15:569–78. doi: 10.1016/S1074-7613(01)00206-0
93. Park JS, Jung YO, Oh HJ, Park SJ, Heo YJ, Kang CM, et al. Interleukin-27 Suppresses Osteoclastogenesis via Induction of Interferon-Gamma. Immunology (2012) 137:326–35. doi: 10.1111/j.1365-2567.2012.03622.x
94. Cheng J, Myers TG, Levinger C, Kumar P, Kumar J, Goshu BA, et al. IL-27 Induces IFN/STAT1-Dependent Genes and Enhances Function of TIGIT(+) HIVGag-Specific T Cells. iScience (2022) 25:103588. doi: 10.1016/j.isci.2021.103588
95. Gohda J, Ma Y, Huang Y, Zhang Y, Gu L, Han Y, et al. HIV-1 Replicates in Human Osteoclasts and Enhances Their Differentiation In Vitro. Retrovirology (2015) 12:12. doi: 10.1186/s12977-015-0139-7
96. Raynaud-Messina B, Bracq L, Dupont M, Souriant S, Usmani SM, Proag A, et al. Bone Degradation Machinery of Osteoclasts: An HIV-1 Target That Contributes to Bone Loss. Proc Natl Acad Sci U S A (2018) 115:E2556–65. doi: 10.1073/pnas.1713370115
97. Tyagi AM, Srivastava K, Mansoori MN, Trivedi R, Chattopadhyay N, Singh D. Estrogen Deficiency Induces the Differentiation of IL-17 Secreting Th17 Cells: A New Candidate in the Pathogenesis of Osteoporosis. PloS One (2012) 7:e44552. doi: 10.1371/journal.pone.0044552
98. Kotake S, Udagawa N, Takahashi N, Matsuzaki K, Itoh K, Ishiyama S, et al. IL-17 in Synovial Fluids From Patients With Rheumatoid Arthritis is a Potent Stimulator of Osteoclastogenesis. J Clin Invest (1999) 103:1345–52. doi: 10.1172/JCI5703
99. Swaminathan S, Hu Z, Rupert AW, Higgins JM, Dewar RL, Stevens R, et al. Plasma Interleukin-27 (IL-27) Levels are Not Modulated in Patients With Chronic HIV-1 Infection. PloS One (2014) 9:e98989. doi: 10.1371/journal.pone.0098989
100. Ruiz-Riol M, Berdnik D, Llano A, Mothe B, Galvez C, Perez-Alvarez S, et al. Identification of Interleukin-27 (IL-27)/IL-27 Receptor Subunit Alpha as a Critical Immune Axis for In Vivo HIV Control. J Virol (2017) 91(16):e00441–17. doi: 10.1128/JVI.00441-17
101. Zheng YH, Xiao SL, He B, He Y, Zhou HY, Chen Z, et al. The Role of IL-27 and its Receptor in the Pathogenesis of HIV/AIDS and Anti-Viral Immune Response. Curr HIV Res (2017) 15:279–84. doi: 10.2174/1570162X15666170517130339
102. Chen Q, Swaminathan S, Yang D, Dai L, Sui H, Yang J, et al. Interleukin-27 is a Potent Inhibitor of Cis HIV-1 Replication in Monocyte-Derived Dendritic Cells via a Type I Interferon-Independent Pathway. PloS One (2013) 8:e59194. doi: 10.1371/journal.pone.0059194
103. Dai L, Lidie KB, Chen Q, Adelsberger JW, Zheng X, Huang D, et al. IL-27 Inhibits HIV-1 Infection in Human Macrophages by Down-Regulating Host Factor SPTBN1 During Monocyte to Macrophage Differentiation. J Exp Med (2013) 210:517–34. doi: 10.1084/jem.20120572
Keywords: IL27, HIV, T cell immune activation, Th1, T cell:osteoclast
Citation: Li T, Hadigan C, Whitlock JM, Qin J, Kumar J, Kumar P and Catalfamo M (2022) IL-27 Modulates the Cytokine Secretion in the T Cell–Osteoclast Crosstalk During HIV Infection. Front. Immunol. 13:818677. doi: 10.3389/fimmu.2022.818677
Received: 19 November 2021; Accepted: 07 March 2022;
Published: 05 April 2022.
Edited by:
Katrina Gee, Queen's University, CanadaReviewed by:
Brigitte Raynaud-Messina, UMR5089 Institut de Pharmacologie et de Biologie Structurale (IPBS), FranceCopyright © 2022 Li, Hadigan, Whitlock, Qin, Kumar, Kumar and Catalfamo. This is an open-access article distributed under the terms of the Creative Commons Attribution License (CC BY). The use, distribution or reproduction in other forums is permitted, provided the original author(s) and the copyright owner(s) are credited and that the original publication in this journal is cited, in accordance with accepted academic practice. No use, distribution or reproduction is permitted which does not comply with these terms.
*Correspondence: Marta Catalfamo, bWMyMTUxQGdlb3JnZXRvd24uZWR1
Disclaimer: All claims expressed in this article are solely those of the authors and do not necessarily represent those of their affiliated organizations, or those of the publisher, the editors and the reviewers. Any product that may be evaluated in this article or claim that may be made by its manufacturer is not guaranteed or endorsed by the publisher.
Research integrity at Frontiers
Learn more about the work of our research integrity team to safeguard the quality of each article we publish.