- 1Institute of Immunology & Immunotherapy, University of Birmingham, Birmingham, United Kingdom
- 2Department of Clinical Science, Liverpool School of Tropical Medicine, Liverpool, United Kingdom
- 3College of Health Sciences, Infectious Diseases Institute, Makerere University, Kampala, Uganda
- 4Fungal Pathogenesis Section, Laboratory of Clinical Immunology & Microbiology, National Institute of Allergy & Infectious Diseases, National Institutes of Health, Bethesda, MD, United States
- 5Institute of Microbiology & Infection, University of Birmingham, Birmingham, United Kingdom
Cryptococcal meningitis (CM) is the leading cause of central nervous system (CNS) fungal infections in humans, with the majority of cases reported from the African continent. This is partly due to the high burden of HIV infection in the region and reduced access to standard-of-care including optimal sterilising antifungal drug treatments. As such, CM is responsible for 10-15% of all HIV-related mortality, with a large proportion being preventable. Immunity to the causative agent of CM, Cryptococcus neoformans, is only partially understood. IFNγ producing CD4+ T-cells are required for the activation of myeloid cells, especially macrophages, to enable fungal killing and clearance. However, macrophages may also act as a reservoir of the fungal yeast cells, shielding them from host immune detection thus promoting latent infection or persistent chronic inflammation. In this chapter, we review the epidemiology and pathogenesis of CNS fungal infections in Africa, with a major focus on CM, and the antifungal immune pathways operating to protect against C. neoformans infection. We also highlight the areas of research and policy that require prioritisation to help reduce the burden of CNS fungal diseases in Africa.
Introduction
Cryptococcal meningitis (CM) is the leading cause of fungal meningitis in humans worldwide, with the largest disease burden reported in Africa (1). The majority of CM cases are caused by members of the Cryptococcus neoformans and C. gattii species complex (2), encapsulated basidiomycetous yeasts that are prevalent in the environment, growing in soil, some plants (e.g. eucalyptus trees) and pigeon guano (3–5). CM is an AIDS defining illness, responsible for 10-15% of all HIV-related mortality globally, resulting in ~80,000 deaths annually of which nearly three-quarters (73%) occur in Africa, particularly sub-Saharan Africa where up to 60% of people with HIV reside (1). C. neoformans produces airborne spores that are acquired by inhalation. In healthy people, host defence mechanisms clear these spores from the alveoli in the lungs preventing symptomatic infection (6), although there is evidence to suggest that these spores may instead become dormant and reactivate during periods of immunosuppression (7). In immunocompromised hosts, these mechanisms fail allowing proliferation of C. neoformans in the lungs and subsequent dissemination to the CNS, causing meningitis/meningoencephalitis (6, 8). In particular, defects in T-cell immunity are highly associated with the development of CNS infection, demonstrating the important role of T-cell-mediated immunity against C. neoformans.
Fungal CNS infections, including CM, disproportionally affect patients in low-middle income countries, although their precise prevalence throughout the world is not well established. Global Action Fund for Fungal Infections (GAFFI) has estimated 47 million Africans suffer from fungal diseases each year (9). Across the continent, there is reduced access to gold-standard diagnostic tools and antifungal drugs for the treatment of CM (9). Moreover, it is clear that we currently have limited effective treatments for CM, since approximately one third of HIV-infected patients given antifungal prophylaxis will still go on to develop serious CNS infection (10). These worrying statistics have led to the development of a global initiative to end deaths from CM by 2030 (11), by implementing improved screening and education programs, tackling HIV management and further research into the pathogenesis of CM.
In this chapter, we discuss the epidemiology, clinical features and immunology of fungal CNS infections in Africa (focusing predominantly on CM), highlighting the areas of research that require prioritisation to help reduce the burden of these life-threatening fungal infections in Africa.
Epidemiology of CNS Fungal Infections in Africa
Human fungal infections of the CNS are an underrepresented group of invasive infections within the African population, occurring as opportunistic infections particularly in individuals living with HIV. The most common CNS infections reported in Africa are CM and histoplasmosis (12, 13). It was estimated in 2017 that ~160,000 people were diagnosed with CM in Africa, with 98% of these cases localised to the sub-Saharan region (1). In particular, most CM cases were reported from South Africa, Nigeria and Mozambique, which averaged 20,000 cases/year/country while North Africa accounted for the least number of CM cases within the continent (1). Although recent years have seen a decrease in the yearly incidence of CM (due to improved access to antifungal and antiretroviral therapy), the mortality rates in Africa still remain high reaching 44% in short-term outcomes in routine care (14, 15) and 73% in long-term follow up studies (16–18). CM cases have been reported infrequently in children (<2% cases) with most cases found in adults living with HIV (19–22). The molecular epidemiology of Cryptococcus species causing CM in Africa is still not well understood, despite recent advances in technologies. C. neoformans (VNI/AFLP1) has been the major genotype causing CM in Africa, identified in >80% of isolates collected (23–30). Other C. neoformans genotypes including AFLP1B/VNII and AFLP1A/VNB have also been isolated from clinical samples and found to cause 5-10% of total CM infections (3, 25, 31–33). Increasing cases of CM as a result of C. gattii species complex such as C. gattii (VGI/AFLP4), C. tetragattii (VGIV/AFLP7) and C. deuterogattii (VGII/AFLP6) have been isolated in countries such as Botswana, Ivory Coast, Kenya and Zimbabwe over the past few years (25, 27, 29, 31, 33). Of note, C. neoformans (AFLP1A/VNB) and C. tetragattii (VGIV/AFLP7) are more common in Southern Africa (3, 31, 34, 35), whilst C. deuterogattii (VGII/AFLP6) has so far been only isolated in Ivory Coast (24, 29, 33).
Besides Cryptococcus, other human fungal pathogens are capable of invading the brain and causing CNS disease in the setting of immunodeficiency and/or traumatic or inadvertent iatrogenic inoculation into the CNS during neurosurgical procedures. The susceptibility of patients to fungal CNS infection with species other than C. neoformans is heavily dependent on specific risk factors, geographic location and environmental exposure. For example, CNS infection with Candida species is associated with CARD9 deficiency, a primary immunodeficiency caused by inherited deleterious mutations in CARD9. Neutrophil influx into the Candida-infected CNS is protective and requires CARD9 expressed by microglia (discussed below) (36, 37). CARD9 deficiency is rare, although several cases have now been reported from Africa, predominantly in Algeria (38). Interestingly, the majority of these CARD9-deficient patients shared the same mutation whereas there was greater diversity in the type of CARD9 mutations in Asian patients (38), but whether genetic variation at the population level contributes towards the geographical distribution of invasive CNS fungal infections is unknown.
Another fungal CNS infection that has been emerging in Africa is histoplasmosis, caused by the dimorphic fungus Histoplasma capsulatum with the var. duboisii being characteristically prevalent in Africa (39, 40). This fungus is the most common pathogenic dimorphic fungus causing endemic infections in Central and West Africa and in the island of Madagascar (41). Indeed, the World Health Organisation (WHO) recently recognised histoplasmosis as a neglected tropical disease requiring further attention (9). Common risk factors for histoplasmosis include advanced HIV infection and iatrogenic immune suppression (41). CNS involvement occurs in 5-20% of patients, usually in patients with advanced infection and poor response to therapy (41, 42). Like CM, diagnosis and treatment of histoplasmosis in the African continent in hampered by availability to gold-standard diagnostic testing and antifungal drugs. Therefore, a global effort to reduce drug costs and improve accessibility will not only improve clinical outcomes in CM, but also for other life-threatening invasive fungal infections such as histoplasmosis.
Cryptococcal Meningitis: Diagnosis, Clinical Features and Treatment
CM can be diagnosed by the identification of encapsulated yeast cells in the cerebrospinal fluid (CSF) using India Ink staining (43). However, this method can often return false negative results and is generally insensitive. Newer tests based on the detection of Cryptococcal antigen (CrAg) are much more sensitive and can allow for a rapid and low cost diagnosis (44), which is critical since many cases of CM are localised to countries with limited resources. The CrAg test works by detecting the Cryptococcus polysaccharide capsule antigen in the CSF; the latest versions of which are based on a lateral flow assay using an immunochromatographic dipstick. This technique is much faster and simpler than culture and/or microscopy based diagnostic assays, and can be performed at the patients’ bedside (45), and is also superior to other CrAg-based detection assays (e.g. latex agglutination assay) that require specialised laboratory equipment and skilled personnel (46). The World Health Organisation (WHO) recommends CrAg screening is performed in HIV-infected patients with a CD4 count of less than 100-200 cells/μL. A study on the effectiveness of CrAg screening in sub-Saharan Africa showed that mortality was significantly decreased when a CrAg screening program was introduced (47). Moreover, plasma CrAg titters are correlated with mortality and can lead to early identification of patients at risk of developing severe CM and death, even when symptoms are absent (10). However, several countries in Africa have limited access to the CrAg test meaning that these effective screening programs are not fully implemented in areas where they would have the greatest benefit. Therefore, improving access to these diagnostic tests is a critical step to help introduce prophylactic antifungal therapy and reduce mortality.
CM can present in the CNS as meningitis, encephalitis, or meningoencephalitis and can also result in cerebral mass lesions called “cryptococcomas” which are typically found along the perivascular spaces. CM is hard to distinguish from other types of meningitis, as there is a lack of specific clinical symptoms. In general, patients present with headache, fever, confusion and/or neck stiffness (13). Several areas of the brain can be affected by CM, including the basal ganglia, the white matter of the cerebral hemispheres, and the cerebellum (48). Computed tomography scans of CM patients usually reveal non-specific features, with ~40% of patients returning normal scans. In contrast, MRI imaging seems to perform better at assessing dilated perivascular spaces and leptomeningeal enhancement, particularly in immunocompromised patients (48), which are among the most common imaging features observed in CM patients (49).
Treatment of CM remains challenging due to the limited selection of antifungal drugs available. Even with treatment, over 70% of patients surviving CM suffer from neurological and sensory impairment, leading to disability and reduced quality of life (50). The gold standard drug for CM treatment is the combination of Amphotericin B (AMB) with flucytosine (5-FC), however a typical course of AMB and 5-FC treatment costs approximately (US)$800 per patient (50), and is usually only available in countries with well-funded healthcare systems. In Africa, only a small number of countries are registered to provide 5-FC, and even when registered there is little evidence it has been prescribed to patients in some areas. Therefore, improving the affordability of 5-FC and enhancing awareness of the drug’s effectiveness is a crucial step towards ending CM deaths (51). In addition, the use of liposomal formulations of AMB is hindered by cost. Thus, because the use of AMB-deoxycholate (AMB-d) requires prolonged hospitalization for parenteral administration and is associated with renal and metabolic adverse effects, many resource-limited settings in Africa do not use AMB for the treatment of CM. Currently, the most commonly prescribed antifungal drug for CM in Africa is fluconazole, which has been shown to be inferior to AMB (52–55). There are now several reports of fluconazole resistance developing in C. neoformans, associated with chromosomal changes in the fungus (56), making management of CM especially difficult in settings where alternative options are not available. Thus, novel therapeutic approaches are needed. Adjunctive immune-based therapy with interferon gamma (IFNγ) has showed promising results in recent clinical trials (57, 58). Treatment with recombinant IFNγ combined with antifungal drugs showed that the addition of recombinant IFNγ resulted in improved clearance of fungi from the CSF compared to patients treated with antifungal drugs alone, although these studies were not large enough to determine if this correlated with improved survival (57, 59). Another experimental treatment that has been suggested is the use of corticosteroids to reduce immunopathology-associated neuroinflammation (see next section), such as dexamethasone, which has been shown to reduce mortality in patients with bacterial meningitis (60). However, dexamethasone treatment for CM in HIV-infected patients actually resulted in a higher mortality rate and disability than in the placebo group, and thus these trials were suspended for safety reasons (61). We therefore still require better antifungal treatments to improve clinical outcomes in patients with CM, which will depend on a better understanding of the immunology of CM (discussed below).
Cryptococcal Meningitis-Associated Immune Reconstitution Inflammatory Response Syndrome
HIV-associated CM management is often complicated by immune reconstitution inflammatory syndrome (IRIS) (62). In sub-Saharan Africa, where most CM infections and deaths occur, most individuals with CM have HIV infection with a profound decline in their CD4+ T cell counts. When antiretroviral therapy is initiated in individuals with this kind of severe immunosuppression, they undergo immune restoration albeit at varying rates (63, 64). This immune restoration occurring prior to pathogen clearance rescues pathogen specific immunity (65). These individuals then mount a pro-inflammatory response, a phenomenon termed IRIS. A similar pro-inflammatory response termed post infectious inflammatory response syndrome (PIIRS) occurs in non-HIV-associated cryptococcal meningitis (66, 67).
There are two kinds of HIV-associated CM-IRIS. First, unmasking CM-IRIS, which occurs following initiation of highly active antiretroviral therapy (HAART) in persons without any prior signs and symptoms of CM. Increased availability of HAART has not been matched by expanded CrAg screening for all individuals with advanced HIV disease, which has meant that unmasking CM-IRIS is on the increase (68). Second, paradoxical CM-IRIS, which occurs following initiation of HAART in persons previously treated for CM with documented microbiological recovery, and clinical resolution continues to decline from 20% - 30% to 3% - 6% as antifungal treatment regimens become more efficacious (69, 70). The median duration from HAART initiation to paradoxical IRIS diagnosis is 110 days (IQR, 73-227 days) (71). The main risk factors for paradoxical CM-IRIS is a high baseline CSF fungal load and a delay in CSF fungal clearance with poorly fungicidal drugs, low CD4 count, a rapid decline in HIV viral load following HAART, and early initiation of antiretroviral therapy following CM diagnosis (54, 62–69, 72, 73).
Diagnosis of CM-IRIS depends on demonstration of a rise in CSF white cell counts and protein levels, as well as evidence of inflammation on brain imaging in the setting of negative CSF fungal cultures. There is currently no definitive treatment for CM-IRIS. The recent IDSA guidelines recommend no specific treatment for minor IRIS presentation. However, for major IRIS complications manifesting with profound CSF pleocytosis and raised intracranial pressure, IDSA guidelines recommend 0.5–1.0 mg/kg per day of prednisone equivalent or higher doses of dexamethasone for severe manifestations tapered over 2 to 6 weeks (74). Although steroids have no role in treatment of active (culture positive) CM infection (see above), their use in HIV-associated IRIS is associated with improved outcomes (61, 75).
The immunopathogenesis of paradoxical CM-IRIS is better understood than unmasking CM-IRIS as summarized here. Type 1 immune responses are driven by Th1 CD4+ T cells secreting IFNγ, which polarizes macrophages to an M1 phenotype associated with production of pro-inflammatory cytokines (TNFα, IL-1β, IL-12, IL-6) and enhanced synthesis of nitric oxide (Figure 1). As a result, M1 macrophages are highly fungicidal to phagocytosed Cryptococcus. In contrast, type 2 responses are driven by IL-4/13-secreting Th2 CD4+ T cells which polarize macrophages to an M2 phenotype, characterized by secretion of anti-inflammatory cytokines (IL-10 and TGFβ) and arginase expression, which counters nitric oxide synthesis and thus impairs clearance of Cryptococcus (76). The protective and non-protective roles for Th1 and Th2, respectively, may be organ-specific however, since enhanced expression of Th1 and Th2-associated cytokines are both correlated with better survival in the CSF of patients with cryptococcal meningitis (72), indicating that while Th2 is strongly associated with promoting fungal infection in the lung (72), this may not be true for the CNS.
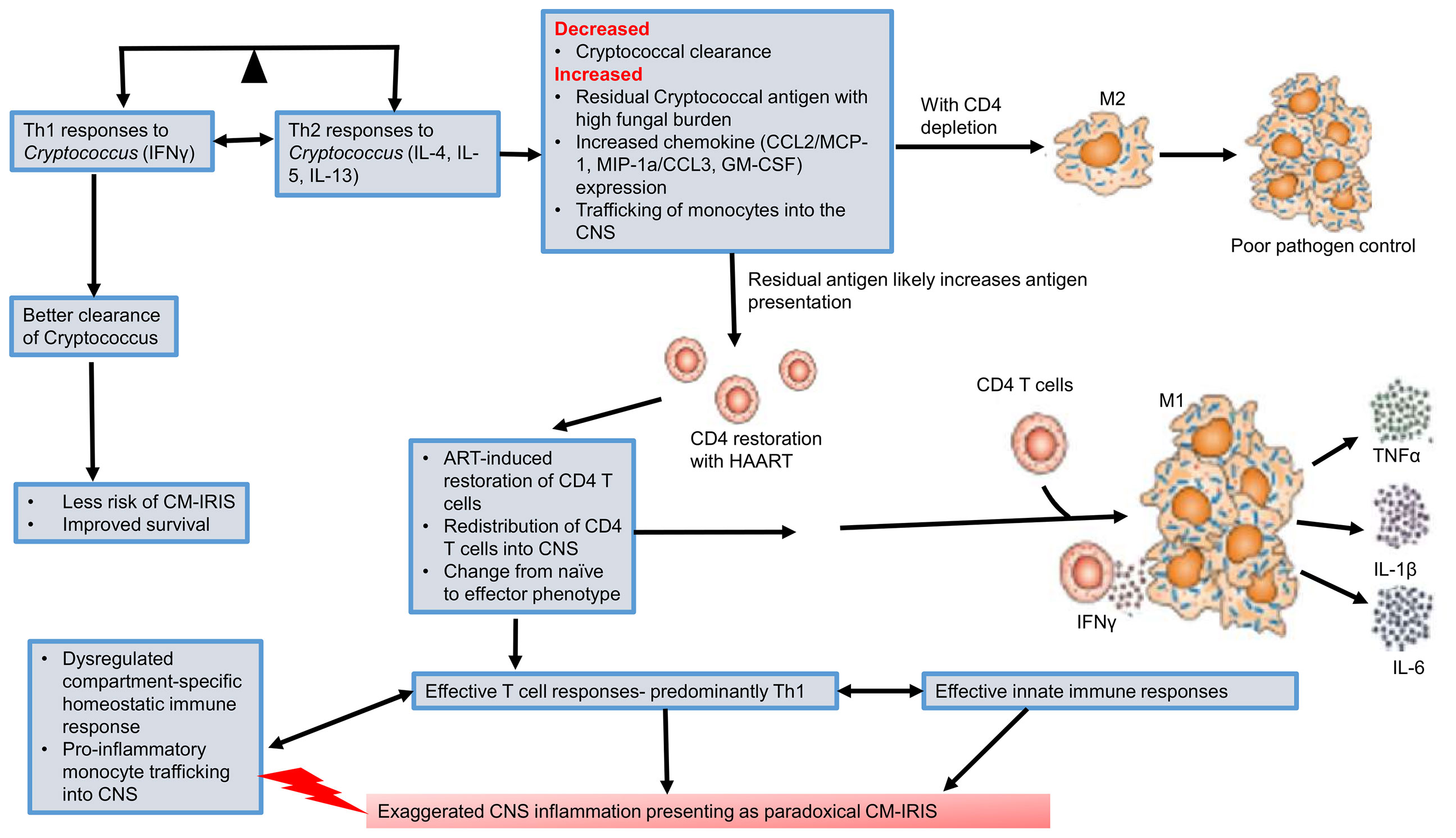
Figure 1 Pathogenesis of cryptococcal meningitis immune reconstitution inflammatory response syndrome (CM-IRIS). Th1 immune responses are required for better clearance of Cryptococcus. This reduces the risk of CM-IRIS and results in improved survival. However, there is an imbalance as the far as the Th1-Th2 paradigm is concerned with extreme HIV-associated CD4 depletion. A predominantly Th2 response is associated with M2 macrophages and poor pathogen clearance. Initiation of HAART restores CD4+ T cell counts. In a setting of poor pathogen clearance, the residual cryptococcal antigen induces an expansion of predominantly Th1 CD4+ T cells with secretion of IFNγ. This polarizes macrophages to an M1 phenotype which secretes pro-inflammatory cytokines and chemokines that recruit more innate immune cells. This predominantly Th1 immune response generates a dysregulated and exaggerated CNS inflammation that presents as paradoxical CM-IRIS. CNS, central nervous system; CM-IRIS, Cryptococcal meningitis immune reconstitution inflammatory response syndrome; HAART, highly active antiretroviral therapy.
Much as the Th1 response is appropriate for enhanced fungal clearance in both humans and murine models (see next section) (72, 77), the timing of this response and the balance with type 2 immunity is critical since dysregulated type 1 immune responses are thought to underlie the pathogenesis of IRIS (78). Current evidence shows that at paradoxical CM-IRIS diagnosis, there is a marked change in the number and phenotype of immune cells in CSF compared to when CM was diagnosed (71). For example, there is a significant increase in the number of T-cells within the CSF at the time of IRIS diagnosis, which exhibit a pro-inflammatory phenotype. Suppressive HAART rescues adaptive immune responses from the destructive effects of uncontrolled HIV replication, which had led to a decline in helper T cells. It is therefore conceivable that during paradoxical CM-IRIS, there is an increase in cryptococcal-specific peripheral blood and CSF activated (HLA-DR+) CD4+ and CD8+ T cells compared to when the initial CM diagnosis was made. Moreover, there is enhanced CXCR3/CXCL10 mediated signaling and trafficking of activated T cells into the CNS (79). Once within the CNS, recruited activated T cells secrete cytokines/chemokines (CCL2/MCP-1, MIP-1α/CCL3, GM-CSF) that enhance monocyte trafficking into the CNS and differentiation into inflammatory macrophages (80, 81). The recruited monocyte/macrophages are activated by Cryptococcus-specific Th1 cells (82). Indeed, the phenotype of CSF monocytes at the time of IRIS diagnosis has been found to have changed from a highly phagocytic classic (CD14++ CD16-) phenotype (observed at the time CM diagnosis), to more pro-inflammatory predominantly intermediate (CD14++ CD16+) and non-classical (CD14+ CD16++) phenotypes (71, 82). This shift is accompanied by an aberrant pro-inflammatory state characterized by enhanced production of TNFα, IL-1β, IL-6, and IFNγ (Figure 1). This exaggerated pro-inflammatory response results in damage to neurons with a rise in CSF neurofilament light chains during IRIS (83). A murine model for CM-IRIS shows that enhanced Th1 T cell infiltration in the CNS results in upregulation of astrocyte Aqp4 mRNA, which upregulates aquaporin-4 postulated to enhance brain edema and thus neuronal injury (84).
In the context of ‘Test and Treat’, where HAART is initiated as soon as individuals have a new HIV diagnosis and in the absence of CrAg screening for those with advanced HIV disease, one area that requires more data is whether persons recently initiated on HAART (<14 days) who present with unmasking cryptococcal IRIS have a higher risk of mortality compared with persons who develop CM after more than six months of HAART (85). Understanding the mechanisms for the immunopathogenesis of unmasking IRIS should be prioritized as well as determining whether interrupting HAART in persons who develop unmasking cryptococcal IRIS could have a survival benefit.
Cryptococcal Meningitis: Neuroimmunology
Like most invasive fungal infections, CM is largely a disease of immune-compromised patients. By studying the immune defects that promote susceptibility to CM, we are better able to understand how the mammalian immune system fights these fungal infections. This information is critical for understanding patient responses to adjunctive immune-based therapy and developing criteria to assess patient prognosis and clinical outcomes. The predominant risk factor for CM is loss of CD4 T-cells from advanced HIV infection (majority of CM cases) however there are increasing numbers of non-HIV CM being reported (66, 86). Several of these also associate with T cell dysfunction caused by various factors including lymphoma, autoimmune diseases (e.g. lupus, psoriasis, sarcoidosis), immunosuppressive therapy and idiopathic CD4+ lymphocytopenia (66, 87). As introduced above, T cells are essential for the activation of macrophages to kill C. neoformans and thus promote fungal clearance. In this section, we outline the mechanisms of fungal entry into the CNS, followed by the immunology of CM focusing on CNS-resident macrophages, astrocytes and brain-infiltrating lymphocytes, and how these different cell types contribute to protection and pathogenesis specifically within the Cryptococcus-infected CNS.
C. neoformans Entry to the CNS
The mechanisms governing C. neoformans entry into the CNS are thought to be largely mediated by two main pathways, the Trojan Horse method and transcellular migration. In this section, we will outline the evidence for each of these invasion mechanisms, although it should be noted that the relative dependence on each in vivo for different pathologic conditions (e.g. host immunosuppression, C. neoformans vs C. gattii), is not well understood.
The Trojan horse approach involves Cryptococcus yeast getting access to the CNS by transporting inside phagocytic cells, such as macrophages, monocytes, and neutrophils. In support of this hypothesis, a few research studies have shown that depletion of alveolar macrophages in mice decreased the dissemination of C. neoformans to CNS (88, 89). Another study compared dissemination to the CNS when mice were infected with bone marrow-derived monocytes previously infected with C. neoformans in vitro, or with free yeast. They found that the fungal burden was higher in the brain with infected bone marrow-derived monocytes compared to free yeast cells, suggesting that infected monocytes were more efficient at disseminating infection to the CNS than free yeast (90). Indeed, depleting circulating monocytes at a later stage of infection in mice reduced infection severity and reduced fungal burden by 40% in spleen, lungs, and brain (90), thus supporting the role of phagocytes in neuroinvasion. Moreover, depleting 99% of circulating monocytes in mice before infection abolished the development of CM and cerebral cryptococcomas and reduced fungal burden in the brain by ~90% (91). Neutrophils have also been shown to potentially promote transmission to the during Cryptococcus infection (92). Using intravital imaging, it was shown that neutrophils can expel C. neoformans within the brain vasculature, contributing towards brain infection (92), and depleting circulating neutrophils resulted in a reduced number of yeast cells in the perivascular space and reduced brain fungal burden by ~ 64% (91). Finally, the Trojan horse model has been modeled in vitro, where cultured brain microendothelial cells were challenged with yeast-containing macrophages. This in vitro model showed that C. neoformans bound CD44 on brain endothelium via hyaluronic acid. Mutant strains that were unable to make hyaluronic acid (cps1Δ) had a profound defect in cellular transmigration (discussed below), but could be transported within macrophages indicating that Trojan Horse mediated entry can enable transport of yeast that would otherwise be restricted from the CNS (93).
Transcellular migration across brain endothelium has also been observed to promote C. neoformans entry into the CNS (94–96). Intra-vital microscopy experiments in mice showed that free yeast cells were able to cross capillary walls, a process that was dependent on fungal-expressed urease since blocking urease reduced transmigration into the brain (96), although it should be noted that urease also promotes intracellular survival within phagocytes (97) indicating that urease blockade might prevent fungal CNS entry by Trojan Horse as well. Other C. neoformans virulence factors that promote CNS entry include the metalloprotease MPR1, hyaluronic acid synthase CPS1 (as mentioned above) and transcription factor HOB1. Mutants deficient in these factors are unable to invade a model blood-brain-barrier (BBB) in vitro, and are avirulent in mouse infection models with a reduced capacity to establish brain infection (98) (99). In order for transcellular migration to occur, C. neoformans yeast must first be internalised by endothelial cells. Interactions between CD44 and hyaluronic acid form part of this process, but it was recently demonstrated that endothelial-expressed EphA2-tyrosine kinase receptors also play a key role (100). Inhibiting EphA2 prevented transmigration of C. neoformans (100), and a similar dependence on EphA2 has been observed for CNS entry by several other pathogens including Chlamydia trachomatis, Epstein-Barr virus, and malaria parasites (101–103), indicating that ephA2 may generally be involved with BBB permeability and pathogen entry (104).
Microglia
The CNS is populated by tissue-resident macrophages that exist in distinct functional subsets and localise within specific anatomical compartments. The most numerous of these CNS-resident macrophages are called microglia, which are found throughout the brain parenchyma and are involved in immune surveillance and brain development (105). Microglia are equipped with an immune arsenal to protect against brain-invading pathogens, including the expression of multiple PRRs such as the C-type lectin recptors (CLRs) and toll-like recptors (TLRs), nitric oxide synthesising enzymes and components needed to process and present antigens to CD4+ T cells (Figure 2). In vitro studies showed that stimulating microglia using TLR agonists (e.g. Pam3 CSK4, LPS, and CpG) during C. neoformans infection drove the production of proinflammatory cytokines such as TNFα, IL-6, and IL-1β, which resulted in enhanced C. neoformans phagocytosis and prevented fungal intracellular replication within microglial phagosomes (106). Immortalised microglia have been shown to phagocytose C. neoformans leading to increased iNOS expression which is important for limiting fungal growth (107, 108). These antifungal actions are regulated by IFNγ, produced by infiltrating Th1-polarized CD4+ T cells. IFNγ has also been shown to induce the expression of MHC Class II by microglia in vitro, potentially allowing their interaction with infiltrating CD4+ T cells (Figure 2) (109–111). A study showed that immunomodulation with CD40 (a T-cell co-stimulatory molecule) and the cytokine IL-2 in C. neoformans-infected mice reduced the fungal burden in various organs including the brain, which correlated with an IFNγ-dependent increase of MHCII expression on microglia (112). Moreover, IFNγ knockout mice showed the critical role of IFNγ in activating microglia and inducing anti-cryptococcal activity (113, 114). Furthermore, patients with CM who feature neutralising IFNγ autoantibodies tend to have a persistent infection and lower survival rate (115). Despite these clear protective roles for microglia in controlling C. neoformans infections, some studies have shown that microglia are prone to latent intracellular infection, where C. neoformans survives and replicates inside microglial phagosomes (Figure 2) (116, 117). Indeed, post-mortem examinations of human brain tissue showed C. neoformans polysaccharide capsule is engulfed and localised inside microglia (116). Therefore, although microglia can engulf Cryptococcus yeast cells, the killing of yeast cells may not always occur in human microglia even when IFNγ is present (118).
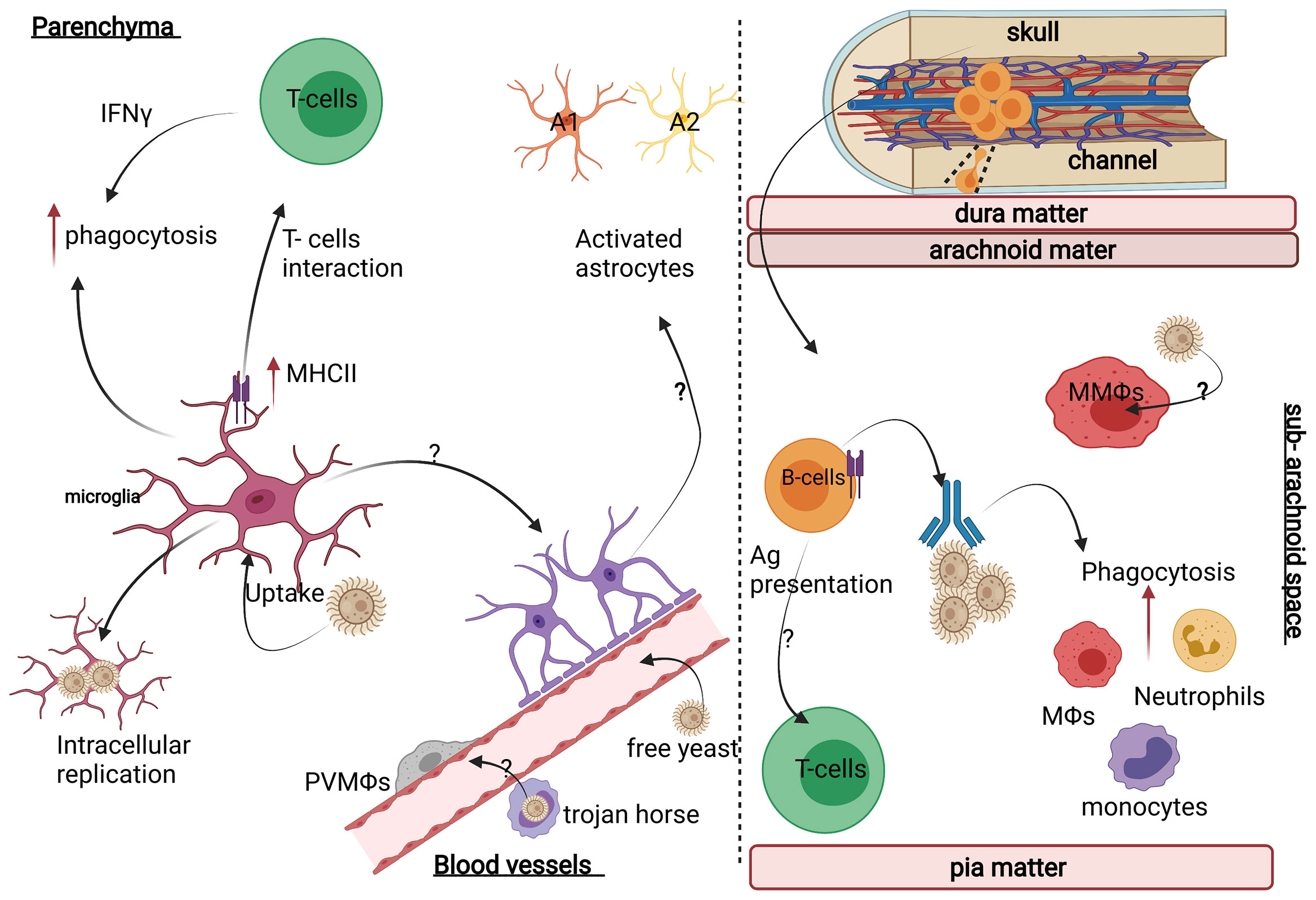
Figure 2 Neuroimmunology of C. neoformans infection. (Left panel) In the brain parenchyma, C. neoformans mostly interacts with brain-resident microglia and astrocytes, which differentiate into distinct functional states depending on the inflammatory context and infiltrating immune cells (e.g. T-cells). For example, astrocytes can develop into pro-inflammatory A1 or tissue-healing A2 subsets, but whether this occurs during CM is unknown. (Right panel) In the meninges, C. neoformans mostly localises to the sub-arachnoid space where it will encounter meningeal macrophages (MMΩs) and a variety of resident lymphocytes including B-cells (deriving from skull bone marrow and connecting channels) and T-cells. Image created with Biorender.com.
Although we can gain insights into anti-cryptococcal immunity using microglia cell lines and in vitro models, in vivo studies are needed to analyse the behaviour of microglia in their natural environment since microglia rapidly lose their tissue-resident identity when removed from their microenvironment. In vivo studies analysing antifungal activity of microglia are so far limited. In a murine model of CM-PIIRIS, full activation of microglia did not occur until 21 days post-infection, which coincided with a significant influx of infiltrating inflammatory myeloid cells and lymphocytes and a decrease in brain fungal burdens (77). A similar observation was made following acute infection with C. neoformans in mice, where microglia numbers expanded >1 week post-infection which coincided with an influx of monocytes and T-cells (91). Interestingly, these effects do not occur with C. gattii, which demonstrates a reduced capacity for entry into the CNS compared with C. neoformans, with C. gattii-infected animals typically succumbing to significant lung disease (91). In contrast, recent in vivo studies showed that C. albicans CNS infection results in a rapid activation of microglia (within 24h), which quickly initiate protective immunity upon C. albicans infection. Microglia highly express CARD9 (caspase recruitment domain-containing protein 9), a signaling adaptor protein downstream of the CLRs (37). Human CARD9 deficiency results in a profound susceptibility to CNS candidiasis, aspergillosis and phaeohyphomycosis but not cryptococcal meningitis (119–121). It was recently shown that CARD9 expression by microglia is required to sense the fungal toxin Candidalysin which is secreted by C. albicans (36). This toxin activated the production of IL-1β and CXCL1 from microglia, which in turn recruited CXCR2-expressing neutrophils to the brain to clear the fungus (36). CARD9 deficiency does not appear to promote susceptibility to CM in humans, and deficiency in CARD9-coupled CLRs do not promote susceptibility to CM in experimental mouse models (122, 123). Thus, microglia have an important role in antifungal immunity that is context-dependent. Future studies should focus on how microglia function during CM using the latest technologies, murine models and human samples in a bid to widen our understanding of immune regulation within the Cryptococcus-infected CNS and how this promotes fungal clearance, as well as associated inflammatory syndromes (see IRIS section above).
Non-Parenchymal CNS-Resident Macrophages
In addition to microglia, there are other CNS-resident macrophages found in the perivascular spaces (perivascular macrophages, PVMs), within the meninges (meningeal macrophages) and associated with the choroid plexus (choroid plexus macrophages) (Figure 2). Each of these populations are poorly studied in the context of CM, with many insights into the biology of these populations only gained in recent years with the advent of new technologies (e.g. single-cell RNA sequencing) that have allowed us to better define the markers and possible functions for these cells (124–126).
Analysis of human brain autopsy tissue showed that PVMs appear to harbour intracellular C. neoformans, indicating that these cells interact with and phagocytose C. neoformans (109). Indeed, the location of PVMs would ideally position them next to the main site of infection in CM (Figure 2). However, an extensive analysis of cryptococcal brain infection in mice showed that the main myeloid effector cells in the brain following C. neoformans infection were monocytes and neutrophils recruited from the blood, and that infection and inflammation were largely confined to the perivascular spaces where CNS-resident macrophages, including perivascular macrophages and microglia, were rare (91). Meningeal macrophages are also situated in the tissues most commonly involved in human CM. Yet, there is little research done to understand the specific contributions of these cells to fungal clearance and pathogenesis. Lastly, PVMs are the primary site for simian immunodeficiency virus (SIV) infection in the CNS, which affects the function of PVMs (127). This is important in the context of CM since it is not yet known how HIV infection (the predominant risk factor for CM in humans) affects the behaviour and function of CNS-resident macrophages such as PVMs and microglia, and the downstream consequences of this for susceptibility to cryptococcal infection. We therefore require a greater understanding of the interplay between HIV and fungal infection in these macrophage subsets and the impact of this on pathogenesis.
Astrocytes
Astrocytes are the most numerous glial cells within the CNS and the majority of studies on astrocyte function to date have focused on their roles in maintaining neuronal health and forming a major component of the blood-brain-barrier (BBB). In recent years, new studies have revealed that astrocytes perform important immune functions and contribute towards CNS pathologies (128). During infection, astrocytes undergo a poorly understood complex process known as ‘astrogliosis’, where structural and functional changes occur. These changes are controlled by the CNS microenvironment which give rise to functionally-differentiated phenotypes that are optimised for tissue repair or resistance to infection (Figure 2) (129–131). Whether fungal CNS infections affect astrocyte phenotype and/or function remains an open question. One study showed that murine astrocytes undergo astrogliosis following intravenous infection with C. neoformans (Figure 2) (130), confirming that astrocytes could play roles in the pathogenesis of CM. Furthermore, in vitro experiments using astrocyte cell lines found that C. neoformans can interact with and infect human astrocytes driving an increase in MHCII expression (132, 133), providing evidence that these cells might be involved in immunity during CM. It will be worth further investigating astrocyte behaviour in CM in future studies particularly as (1) astrocytes appear to become activated during human CM and this is blunted in HIV-infected patients (134–136), and (2) astrocytes regulate traffic through the BBB thus they might have significant role in prevention of C. neoformans invasion of the CNS.
T-Cells
There is growing evidence that T cells are present in the healthy CNS, which have a unique CNS-resident phenotype and are important for CNS homeostasis and animal behaviour (137–140). Mice deficient in adaptive immunity (e.g. Rag1-/-) have behavioural abnormalities, which was recently linked to their role in promoting microglial maturation in the developing brain (141–145). Moreover, T cells have been shown to promote pathology in several neurodegenerative diseases (146–148), as well as supress astrogliosis during ischaemic stroke (147). These lymphocytes are therefore integral to the outcome of CNS inflammation and important regulators of pathology in this tissue.
The specific functions of CD4+ T cells in the Cryptococcus-infected CNS remain poorly defined. As outlined above, these lymphocytes are thought to be required to activate antifungal killing pathways in myeloid cells but may also promote tissue pathology (149–151). CD4+ T cell recruitment to the cryptococcal-infected brain was recently shown to require CXCR3. Both human and murine T cells significantly upregulated CXCR3 in response to C. neoformans infection, and this chemokine receptor was required for Th1 polarization. Interestingly, Cxcr3-/- mice were protected from infection-associated CNS inflammation and thus had improved survival, but this did not correlate with reduced fungal burden. These studies therefore show that CXCR3+ Th1 T cells are not needed to help control fungal infection in the brain, at least in the context of an IRIS-like syndrome (152). Similarly, knockdown of CCR2 in mice was also shown to improve survival independently of fungal control in the CNS, although CCR2 was not involved in the direct recruitment of Th1 T cells to the CNS but acted indirectly by promoting the initial recruitment of inflammatory monocytes (153). Collectively, these studies indicate that T-cells have a complex role in CM, both for fungal clearance and mediating immunopathology, which is likely context- and time-dependent.
B-Cells
B-cells produce anti-cryptococcal antibodies that are required for effective opsonisation of the fungus (particularly the capsule) and uptake by phagocytes, including macrophages (154). Patients with X-linked agammaglobulinemia (XLA), an inherited immune-deficiency caused by mutations in the BTK gene and characterised by an absence of B cells, have been reported to develop CM (155). Furthermore, reduced production of IgM in HIV+ patients has been correlated with a greater risk for developing CM (156). Treatment with the BTK inhibitor Ibrutinib, a drug used in the treatment of B-cell lymphomas, has been reported to promote CM in a small number of patients, although the exact underlying mechanism(s) and relative incidence of CM in Ibrutinib-treated patients remain unclear (157). Mice with B-cell and/or antibody deficiencies also have increased susceptibility to C. neoformans infection, characterized by higher brain fungal burden (158). Thus, B-cells provide critical support to phagocytes in the fight against CM and clearance of yeast cells from infected tissues (Figure 2).
CNS border tissues, such as the meninges, were recently shown to be populated by CNS-resident B cells which infiltrated the CNS from the skull bone marrow via a series of bone channels (Figure 2). These channels provide the meninges with a constant supply of CNS-resident B cells, which were shown to have an immunoregulatory phenotype and were optimised at recognising CNS-derived antigens (159–162). Furthermore, meningeal IgA-secreting plasma cells have been shown to curtail Candida invasion in the CNS (163), but whether these CNS-resident B-cells proliferate in response to C. neoformans infection and/or provide local protection against cryptococcal infection has not yet been determined.
Concluding Remarks
The majority of deaths from invasive fungal infections in humans occur in Africa, and many of these are preventable. Improving access and reducing cost of ‘gold-standard’ diagnostics and treatments is urgently needed to reduce the impact of fungal CNS infections on global human health. However, even with access to antifungal drugs, mortality and morbidity from fungal CNS infection remains high. Worryingly, we are also seeing more cases of fungal CNS infections reported particularly amongst non-HIV immunosuppressed populations. It is therefore clear that we require more insights into the pathogenesis of these diseases and adjunctive immune-based therapies that boost the effectiveness of antifungal drugs. Recent advances in neuroimmunology have led to the development of models and technologies leading to novel insights into how immune responses are initiated and regulated within the CNS. Many of these models and approaches have yet to be utilised by the fungal immunology field, but their application holds significant potential in terms of discovery and future therapeutic benefit. In summary, we hope that future studies focusing on CNS antifungal immunity will shed light on how these infections may be better managed and treated, which alongside enhancing public awareness and education on the impact of fungal CNS infections, may lead to reduced mortality and improved health across Africa.
Author Contributions
All authors wrote the manuscript, edited the final draft and approved the final submission.
Funding
This work was supported in part by the Medical Research Council (RD), the Academy of Medical Sciences (SM, RD), the Division of Intramural Research of the National Institute of Allergy & Infectious Diseases, National Institutes of Health (ML).
Conflict of Interest
The authors declare that the research was conducted in the absence of any commercial or financial relationships that could be construed as a potential conflict of interest.
Publisher’s Note
All claims expressed in this article are solely those of the authors and do not necessarily represent those of their affiliated organizations, or those of the publisher, the editors and the reviewers. Any product that may be evaluated in this article, or claim that may be made by its manufacturer, is not guaranteed or endorsed by the publisher.
References
1. Rajasingham R, Smith RM, Park BJ, Jarvis JN, Govender NP, Chiller TM, et al. Global Burden of Disease of HIV- Associated Cryptococcal Meningitis: An Updated Analysis. Lancet Infect Dis (2017) 17(8):873–81. doi: 10.1016/S1473-3099(17)30243-8
2. Hagen F, Lumbsch HT, Arsenijevic VA, Badali H, Bertout S, Billmyre RB, et al. Importance of Resolving Fungal Nomenclature: The Case of Multiple Pathogenic Species in the Cryptococcus Genus. mSphere (2017) 2(4):e00238–17. doi: 10.1128/mSphere.00238-17
3. Chen Y, Litvintseva AP, Frazzitta AE, Haverkamp MR, Wang L, Fang C, et al. Comparative Analyses of Clinical and Environmental Populations of Cryptococcus Neoformans in Botswana. Mol Ecol (2015) 24(14):3559–71. doi: 10.1111/mec.13260
4. Criseo G, Bolignano MS, De Leo F, Staib F, et al. Evidence of Canary Droppings as an Important Reservoir of Cryptococcus Neoformans. Zentralbl Bakteriol (1995) 282(3):244–54. doi: 10.1016/S0934-8840(11)80124-6
5. Spina-Tensini T, Dominguez Muro M, Queiroz-Telles F, Strozzi I, Talise Moraes S, Petterle RR, et al. Geographic Distribution of Patients Affected by Cryptococcus Neoformans/Cryptococcus Gattii Species Complexes Meningitis, Pigeon and Tree Populations in Southern Brazil. Mycoses (2017) 60(1):51–8. doi: 10.1111/myc.12550
6. Brown GD. Innate Antifungal Immunity: The Key Role of Phagocytes. Annu Rev Immunol (2011) 29:1–21. doi: 10.1146/annurev-immunol-030409-101229
7. Garcia-Hermoso D, Janbon G, Dromer F. Epidemiological Evidence for Dormant Cryptococcus Neoformans Infection. J Clin Microbiol (1999) 37(10):3204–9. doi: 10.1128/JCM.37.10.3204-3209.1999
8. Bojarczuk A, Miller KA, Hotham R, Lewis A, Ogryzko NV, Kamuyango AA, et al. Cryptococcus Neoformans Intracellular Proliferation and Capsule Size Determines Early Macrophage Control of Infection. Sci Rep (2016) 6:21489. doi: 10.1038/srep21489
9. Oladele RO, Akase IE, Fahal AH, Govender NP, Hoenigl M, Pierre Gangneux J, et al. Bridging the Knowledge Gap on Mycoses in Africa: Setting Up a Pan-African Mycology Working Group. Mycoses (2020) 63(3):244–9. doi: 10.1111/myc.13044
10. Rajasingham R, Boulware DR. Cryptococcal Antigen Screening and Preemptive Treatment—How Can We Improve Survival? Clin Infect Dis (2020) 70(8):1691–4. doi: 10.1093/cid/ciz488
11. Shroufi A, Chiller T, Jordan A, Denning DW, Harrison TS, Govender NP, et al. Ending Deaths From HIV-Related Cryptococcal Meningitis by 2030. Lancet Infect Dis (2021) 21(1):16–8. doi: 10.1016/S1473-3099(20)30909-9
12. Bongomin F, Adetona Fayemiwo S. Epidemiology of Fungal Diseases in Africa: A Review of Diagnostic Drivers. Curr Med Mycology (2021) 7(1):63–70. doi: 10.18502/cmm.7.1.6246
13. Baradkar V, De Mathur A, Kumar S, Rathi M, et al. Prevalence and Clinical Presentation of Cryptococcal Meningitis Among HIV Seropositive Patients. Indian J Sex Transm Dis AIDS (2009) 30(1):19–22. doi: 10.4103/0253-7184.55474
14. Kalata N, Ellis J, Kanyama C, Kuoanfank C, Temfack E, Mfinanga S, et al. Short-Term Mortality Outcomes of HIV-Associated Cryptococcal Meningitis in Antiretroviral Therapy-Naïve and -Experienced Patients in Sub-Saharan Africa. Open Forum Infect Dis (2021) 8(10):ofab397. doi: 10.1016/S1473-3099(17)30243-8
15. Tenforde MW, Gertz AM, Lawrence DS, Wills NK, Guthrie BL, Farquhar C, et al. Mortality From HIV-Associated Meningitis in Sub-Saharan Africa: A Systematic Review and Meta-Analysis. J Int AIDS Soc (2020) 23(1):e25416. doi: 10.1002/jia2.25416
16. Makadzange AT, Ndhlovu CE, Takarinda K, Reid M, Kurangwa M, Gona P, et al. Early Versus Delayed Initiation of Antiretroviral Therapy for Concurrent HIV Infection and Cryptococcal Meningitis in Sub-Saharan Africa. Clin Infect Dis (2010) 50(11):1532–8. doi: 10.1086/652652
17. Rothe C, Sloan DJ, Goodson P, Chikafa J, Mukaka M, Denis B, et al. A Prospective Longitudinal Study of the Clinical Outcomes From Cryptococcal Meningitis Following Treatment Induction With 800 Mg Oral Fluconazole in Blantyre, Malawi. PloS One (2013) 8(6):e67311. doi: 10.1371/journal.pone.0067311
18. Kambugu A, Meya DB, Rhein J, O'Brien M, Janoff EN, Ronald AR, et al. Outcomes of Cryptococcal Meningitis in Uganda Before and After the Availability of Highly Active Antiretroviral Therapy. Clin Infect Dis (2008) 46(11):1694–701. doi: 10.1086/587667
19. Nyazika TK, Masanganise F, Hagen F, Bwakura-Dangarembizi MF, Ticklay IMH, Robertson VJ, et al. Cryptococcal Meningitis Presenting as a Complication in HIV-Infected Children: A Case Series From Sub-Saharan Africa. Pediatr Infect Dis J (2016) 35(9):979–80. doi: 10.1097/INF.0000000000001212
20. Cotton MF, Rabie H, Nemes E, Mujuru H, Bobat R, Njau B, et al. A Prospective Study of the Immune Reconstitution Inflammatory Syndrome (IRIS) in HIV-Infected Children From High Prevalence Countries. PloS One (2019) 14(7):e0211155. doi: 10.1371/journal.pone.0211155
21. Miglia KJ, Govender NP, Rossouw J, Meiring S, Mitchell TG, the Group for Enteric, et al. Analyses of Pediatric Isolates of Cryptococcus Neoformans From South Africa. J Clin Microbiol (2011) 49(1):307–14. doi: 10.1128/JCM.01277-10
22. Meiring ST, Quan VC, Cohen C, Dawood H, Alan S K, McCarthy KM, et al. A Comparison of Cases of Paediatric-Onset and Adult-Onset Cryptococcosis Detected Through Population-Based Surveillance, 2005-2007. Aids (2012) 26(18):2307–14. doi: 10.1097/QAD.0b013e3283570567
23. Kassi FK, Bellet V, Drakulovski P, Krasteva D, Roger F, Valérie B-TA, et al. Comparative Typing Analyses of Clinical and Environmental Strains of the Cryptococcus Neoformans/Cryptococcus Gattii Species Complex From Ivory Coast. J Med Microbiol (2018) 67(1):87–96. doi: 10.1099/jmm.0.000654
24. Kassi FK, Drakulovski P, Virginie B, Krasteva D, Gatchitch F, Doumbia A, et al. Molecular Epidemiology Reveals Genetic Diversity Among 363 Isolates of Thecryptococcus Neoformansandcryptococcus Gattiispecies Complex in 61 Ivorian HIV-Positive Patients. Mycoses (2016) 59(12):811–7. doi: 10.1111/myc.12539
25. Nyazika TK, Hagen F, Machiridza T, Kutepa M, Masanganise F, Hendrickx M, et al. Cryptococcus Neoformans Population Diversity and Clinical Outcomes of HIV-Associated Cryptococcal Meningitis Patients in Zimbabwe. J Med Microbiol (2016) 65(11):1281–8. doi: 10.1099/jmm.0.000354
26. Beale MA, Sabiiti W, Robertson EJ, Fuentes-Cabrejo KM, O'Hanlon SJ, Jarvis JN, et al. Genotypic Diversity Is Associated With Clinical Outcome and Phenotype in Cryptococcal Meningitis Across Southern Africa. PloS Negl Trop Dis (2015) 9(6):e0003847. doi: 10.1371/journal.pntd.0003847
27. Kangogo M, Bader O, Boga H, Wanyoike W, Folba C, Worasilchai N, et al. Molecular Types of Cryptococcus Gattii/Cryptococcus Neoformans Species Complex From Clinical and Environmental Sources in Nairobi, Kenya. Mycoses (2015) 58(11):665–70. doi: 10.1111/myc.12411
28. Van Wyk M, Govender NP, Mitchell TG, Litvintseva AP, GERMS-SA, et al. Multilocus Sequence Typing of Serially Collected Isolates of Cryptococcus From HIV-Infected Patients in South Africa. J Clin Microbiol (2014) 52(6):1921–31. doi: 10.1128/JCM.03177-13
29. Kassi FK, Bellet V, Doumbia A, Krasteva D, Drakulovski P, Kouakou GA, et al. First Case of Mixed Infection With Cryptococcus Deuterogattii and Cryptococcus Neoformans VNI in an Ivorian HIV-Positive Patient. JMM Case Rep (2016) 3(4):e005037. doi: 10.1099/jmmcr.0.005037
30. Hurtado JC, Castillo P, Fernandes F, Navarro M, Lovane L, Casas I, et al. Mortality Due to Cryptococcus Neoformans and Cryptococcus Gattii in Low-Income Settings: An Autopsy Study. Sci Rep (2019) 9(1):7493. doi: 10.1038/s41598-019-43941-w
31. Litvintseva AP, Thakur R, Reller LB, Mitchell TG, et al. Prevalence of Clinical Isolates of Cryptococcus Gattii Serotype C Among Patients With AIDS in Sub-Saharan Africa. J Infect Dis (2005) 192(5):888–92. doi: 10.1086/432486
32. Rakotoarivelo RA, Raberahona M, Rasamoelina T, Rabezanahary A, Rakotomalala FA, Razafinambinintsoa T, et al. Epidemiological Characteristics of Cryptococcal Meningoencephalitis Associated With Cryptococcus Neoformans Var. Grubii From HIV-Infected Patients in Madagascar: A Cross-Sectional Study. PloS Negl Trop Dis (2020) 14(1):e0007984. doi: 10.1371/journal.pntd.0007984
33. Kassi FK, Drakulovski P, Bellet V, Roger F, Chabrol A, Krasteva D, et al. Cryptococcus Genetic Diversity and Mixed Infections in Ivorian HIV Patients: A Follow Up Study. PloS Negl Trop Dis (2019) 13(11):e0007812. doi: 10.1371/journal.pntd.0007812
34. Nyazika TK, Hagen F, Meis JF, Robertson VJ. Cryptococcus Tetragattii as a Major Cause of Cryptococcal Meningitis Among HIV-Infected Individuals in Harare, Zimbabwe. J Infect (2016) 72(6):745–52. doi: 10.1016/j.jinf.2016.02.018
35. Litvintseva AP, Mitchell TG. Population Genetic Analyses Reveal the African Origin and Strain Variation of Cryptococcus Neoformans Var Grubii. PloS Pathog (2012) 8(2):e1002495. doi: 10.1371/journal.ppat.1002495
36. Drummond RA, Swamydas M, Oikonomou V, Zhai B, Dambuza IM, Schaefer BC, et al. CARD9+ Microglia Promote Antifungal Immunity via IL-1β- and CXCL1-Mediated Neutrophil Recruitment. Nat Immunol (2019) 20(5):559–70. doi: 10.1038/s41590-019-0377-2
37. Drummond RA, Collar AL, Swamydas M, Rodriguez CA, Lim JK, Mendez LM, et al. CARD9-Dependent Neutrophil Recruitment Protects Against Fungal Invasion of the Central Nervous System. PloS Pathog (2015) 11(12):e1005293. doi: 10.1371/journal.ppat.1005293
38. Vaezi A, Fakhim H, Abtahian Z, Khodavaisy S, Geramishoar M, Alizadeh A, et al. Frequency and Geographic Distribution of CARD9 Mutations in Patients With Severe Fungal Infections. Front Microbiol (2018) 9(2434). doi: 10.3389/fmicb.2018.02434
39. Konan L, Drogba L, Brahima D, Mesfin FB, et al. A Case of Histoplasma Duboisii Brain Abscess and Review of the Literature. Cureus (2020) 12(2):e6984. doi: 10.7759/cureus.6984
40. Develoux M, Amona FM, Hennequin C. Histoplasmosis Caused by Histoplasma Capsulatum Var. Duboisii: A Comprehensive Review of Cases From 1993 to 2019. Clin Infect Dis (2021) 73(3):e543–9. doi: 10.1093/cid/ciaa1304
41. Veeravagu A, Ludwig C, Camara-Quintana JQ, Jiang B, Lad N, Shuer L, et al. Fungal Infection of a Ventriculoperitoneal Shunt: Histoplasmosis Diagnosis and Treatment. World Neurosurg (2013) 80(1-2):222.e5–13. doi: 10.1016/j.wneu.2012.12.016
42. Wheat LJ, Batteiger BE, Sathapatayavongs B. Histoplasma Capsulatum Infections of the Central Nervous System. A Clinical Review. Med (Baltimore) (1990) 69(4):244–60. doi: 10.1097/00005792-199007000-00006
43. Abassi M, Boulware DR, Rhein J. Cryptococcal Meningitis: Diagnosis and Management Update. Curr Trop Med Rep (2015) 2(2):90–9. doi: 10.1007/s40475-015-0046-y
44. Vidal JE, Boulware DR. Lateral Flow Assay for Cryptococcal Antigen: An Important Advance to Improve the Continuum of Hiv Care and Reduce Cryptococcal Meningitis-Related Mortality. Rev Do Inst Med Trop Sao Paulo (2015) 57 Suppl 19(Suppl 19):38–45. doi: 10.1590/S0036-46652015000700008
45. Boulware DR, Rolfes MA, Rajasingham R, von Hohenberg M, Qin Z, Taseera K, et al. Multisite Validation of Cryptococcal Antigen Lateral Flow Assay and Quantification by Laser Thermal Contrast. Emerg Infect Dis (2014) 20(1):45–53. doi: 10.3201/eid2001.130906
46. Binnicker MJ, Jespersen DJ, Bestrom JE, Rollins LO. Comparison of Four Assays for the Detection of Cryptococcal Antigen. Clin Vaccine Immunol (2012) 19(12):1988–90. doi: 10.1128/CVI.00446-12
47. Deiss R, Loreti CV, Gutierrez AG, Filipe E, Tatia M, Issufo S, et al. High Burden of Cryptococcal Antigenemia and Meningitis Among Patients Presenting at an Emergency Department in Maputo, Mozambique. PloS One (2021) 16(4):e0250195. doi: 10.1371/journal.pone.0250195
48. Xia S, Li X, Li H. Imaging Characterization of Cryptococcal Meningoencephalitis. Radiol Infect Dis (2016) 3(4):187–91. doi: 10.1016/j.jrid.2016.05.003
49. Duarte SBL, Oshima MM, do Amaral Mesquita JV, Pereira do Nascimento FB, de Azevedo PC, Reis F, et al. Magnetic Resonance Imaging Findings in Central Nervous System Cryptococcosis: Comparison Between Immunocompetent and Immunocompromised Patients. Radiol Bras (2017) 50(6):359–65. doi: 10.1590/0100-3984.2016.0017
50. Shiri T, Loyse A, Mwenge L, Chen T, Lakhi S, Chanda D, et al. Addition of Flucytosine to Fluconazole for the Treatment of Cryptococcal Meningitis in Africa: A Multicountry Cost-Effectiveness Analysis. Clin Infect Dis (2019) 70(1):26–9.
51. Miot J, Leong T, Takuva S, Parrish A, Dawood H, et al. Cost-Effectiveness Analysis of Flucytosine as Induction Therapy in the Treatment of Cryptococcal Meningitis in HIV-Infected Adults in South Africa. BMC Health Serv Res (2021) 21(1):305. doi: 10.1186/s12913-021-06268-9
52. Ford N, Migone C, Calmy A, Kerschberger B, Kanters S, Nsanzimana S, et al. Benefits and Risks of Rapid Initiation of Antiretroviral Therapy. AIDS (Lond Engl) (2018) 32(1):17–23. doi: 10.1097/QAD.0000000000001671
53. Jarvis JN, Leeme TB, Molefi M, Chofle AA, Bidwell G, Tsholo K, et al. Short-Course High-Dose Liposomal Amphotericin B for Human Immunodeficiency Virus–Associated Cryptococcal Meningitis: A Phase 2 Randomized Controlled Trial. Clin Infect Dis (2019) 68(3):393–401. doi: 10.1093/cid/ciy515
54. Boulware DR, Meya DB, Muzoora C, Rolfes MA, Hullsiek KH, Musubire A, et al. Timing of Antiretroviral Therapy After Diagnosis of Cryptococcal Meningitis. N Engl J Med (2014) 370(26):2487–98. doi: 10.1056/NEJMoa1312884
55. Jarvis JN, Bicanic T, Loyse A, Namarika D, Jackson A, Nussbaum JC, et al. Determinants of Mortality in a Combined Cohort of 501 Patients With HIV-Associated Cryptococcal Meningitis: Implications for Improving Outcomes. Clin Infect Dis: an Off Publ Infect Dis Soc Am (2014) 58(5):736–45. doi: 10.1093/cid/cit794
56. Hope W, Stone NRH, Johnson A, McEntee L, Farrington N, Santoro-Castelazo A, et al. Fluconazole Monotherapy Is a Suboptimal Option for Initial Treatment of Cryptococcal Meningitis Because of Emergence of Resistance. mBio (2019) 10(6):e02575–19. doi: 10.1128/mBio.02575-19
57. Pappas PG, Bustamante B, Ticona E, Hamill RJ, Johnson PC, Reboli A, et al. Recombinant Interferon-γ1b as Adjunctive Therapy for AIDS-Related Acute Cryptococcal Meningitis. J Infect Dis (2004) 189(12):2185–91. doi: 10.1086/420829
58. Jarvis JN, Meintjes G, Rebe K, Williams GN, Bicanic T, Williams A, et al. Adjunctive Interferon-γ Immunotherapy for the Treatment of HIV-Associated Cryptococcal Meningitis: A Randomized Controlled Trial. AIDS (Lond Engl) (2012) 26(9):1105–13. doi: 10.1097/QAD.0b013e3283536a93
59. Jarvis JN, Meintjes G, Rebe K, Williams GN, Bicanic T, Williams A, et al. Adjunctive Interferon-γ Immunotherapy for the Treatment of HIV-Associated Cryptococcal Meningitis. AIDS (2012) 26(9):1105–13. doi: 10.1097/QAD.0b013e3283536a93
60. De Gans J, Van De Beek D. Dexamethasone in Adults With Bacterial Meningitis. N Engl J Med (2002) 347(20):1549–56. doi: 10.1056/NEJMoa021334
61. Beardsley J, Wolbers M, Kibengo FM, Ggayi A-BM, Kamali A, Cuc NT, et al. Adjunctive Dexamethasone in HIV-Associated Cryptococcal Meningitis. N Engl J Med (2016) 374(6):542–54. doi: 10.1056/NEJMoa1509024
62. Haddow LJ, Colebunders R, Meintjes G, Lawn SD, Elliott JH, Manabe YC, et al. Cryptococcal Immune Reconstitution Inflammatory Syndrome in HIV-1-Infected Individuals: Proposed Clinical Case Definitions. Lancet Infect Dis (2010) 10(11):791–802. doi: 10.1016/S1473-3099(10)70170-5
63. Sabin CA, Smith CJ, d'Arminio Monforte A, Battegay M, Gabiano C, Galli L, et al. Response to Combination Antiretroviral Therapy: Variation by Age. Aids (2008) 22(12):1463–73. doi: 10.1097/QAD.0b013e3282f88d02
64. Le Moing V, Thiébaut R, Chêne G, Leport C, Cailleton V, Michelet C, et al. Predictors of Long-Term Increase in CD4(+) Cell Counts in Human Immunodeficiency Virus-Infected Patients Receiving a Protease Inhibitor-Containing Antiretroviral Regimen. J Infect Dis (2002) 185(4):471–80. doi: 10.1086/338929
65. French MA, Lenzo N, John M, Mallal SA, McKinnon EJ, James IR, et al. Immune Restoration Disease After the Treatment of Immunodeficient HIV-Infected Patients With Highly Active Antiretroviral Therapy. HIV Med (2000) 1(2):107–15. doi: 10.1046/j.1468-1293.2000.00012.x
66. Williamson PR, Jarvis JN, Panackal AA, Fisher MC, Molloy SF, Loyse A, et al. Cryptococcal Meningitis: Epidemiology, Immunology, Diagnosis and Therapy. Nat Rev Neurol (2016) 13(1):13–24. doi: 10.1038/nrneurol.2016.167
67. Williamson PR. Post-Infectious Inflammatory Response Syndrome (PIIRS): Dissociation of T-Cell-Macrophage Signaling in Previously Healthy Individuals With Cryptococcal Fungal Meningoencephalitis. Macrophage (2015) 2:e1078. doi: 10.14800/Macrophage.1078
68. Rhein J, Hullsiek KH, Evans EE, Tugume L, Nuwagira E, Ssebambulidde K, et al. Detrimental Outcomes of Unmasking Cryptococcal Meningitis With Recent ART Initiation. Open Forum Infect Dis (2018) 5(8):ofy122. doi: 10.1093/ofid/ofy122
69. Rhein J, Hullsiek KH, Tugume L, Nuwagira E, Mpoza E, Evans EE, et al. Adjunctive Sertraline for HIV-Associated Cryptococcal Meningitis: A Randomised, Placebo-Controlled, Double-Blind Phase 3 Trial. Lancet Infect Dis (2019) 19(8):843–51. doi: 10.1016/S1473-3099(19)30127-6
70. Wiesner DL, Boulware DR. Cryptococcus-Related Immune Reconstitution Inflammatory Syndrome(IRIS): Pathogenesis and Its Clinical Implications. Curr Fungal Infect Rep (2011) 5(4):252–61. doi: 10.1007/s12281-011-0064-8
71. Meya DB, Okurut S, Zziwa G, Rolfes MA, Kelsey M, Cose S, et al. Cellular Immune Activation in Cerebrospinal Fluid From Ugandans With Cryptococcal Meningitis and Immune Reconstitution Inflammatory Syndrome. J Infect Dis (2015) 211(10):1597–606. doi: 10.1093/infdis/jiu664
72. Jarvis JN, Meintjes G, Bicanic T, Buffa V, Hogan L, Mo S, et al. Cerebrospinal Fluid Cytokine Profiles Predict Risk of Early Mortality and Immune Reconstitution Inflammatory Syndrome in HIV-Associated Cryptococcal Meningitis. PloS Pathog (2015) 11(4):e1004754–e1004754. doi: 10.1371/journal.ppat.1004754
73. Boulware DR, Meya DB, Bergemann TL, Wiesner DL, Rhein J, Musubire A, et al. Clinical Features and Serum Biomarkers in HIV Immune Reconstitution Inflammatory Syndrome After Cryptococcal Meningitis: A Prospective Cohort Study. PloS Med (2010) 7(12):e1000384. doi: 10.1371/journal.pmed.1000384
74. Perfect JR, et al. Clinical Practice Guidelines for the Management of Cryptococcal Disease: 2010 Update by the Infectious Diseases Society of America. Clin Infect Dis (2010) 50(3):291–322. doi: 10.1086/649858
75. Lesho E. Evidence Base for Using Corticosteroids to Treat HIV-Associated Immune Reconstitution Syndrome. Expert Rev Anti Infect Ther (2006) 4(3):469–78. doi: 10.1586/14787210.4.3.469
76. Stenzel W, Müller U, Köhler G, Heppner FL, Blessing M, McKenzie ANJ, et al. IL-4/IL-13-Dependent Alternative Activation of Macrophages But Not Microglial Cells Is Associated With Uncontrolled Cerebral Cryptococcosis. Am J Pathol (2009) 174(2):486–96. doi: 10.2353/ajpath.2009.080598
77. Neal LM, Xing E, Xu J, Kolbe JL, Osterholzer JJ, Segal BM, et al. CD4+ T Cells Orchestrate Lethal Immune Pathology Despite Fungal Clearance During Cryptococcus Neoformans Meningoencephalitis. mBio (2017) 8(6):e01415–17. doi: 10.2353/ajpath.2009.080598
78. Pirofski LA, Casadevall A. The Damage-Response Framework of Microbial Pathogenesis and Infectious Diseases. Adv Exp Med Biol (2008) 635:135–46. doi: 10.1007/978-0-387-09550-9_11
79. Davis MJ, et al. Macrophage M1/M2 Polarization Dynamically Adapts to Changes in Cytokine Microenvironments in Cryptococcus Neoformans Infection. mBio (2013) 4(3):e00264–13-e00264. doi: 10.1128/mBio.00264-13
80. Chang CC, Omarjee S, Lim A, Spelman T, Gosnell BI, Carr WH, et al. Chemokine Levels and Chemokine Receptor Expression in the Blood and the Cerebrospinal Fluid of HIV-Infected Patients With Cryptococcal Meningitis and Cryptococcosis-Associated Immune Reconstitution Inflammatory Syndrome. J Infect Dis (2013) 208(10):1604–12. doi: 10.1093/infdis/jit388
81. Longley N, Harrison TS, Jarvis JN. Cryptococcal Immune Reconstitution Inflammatory Syndrome. Curr Opin Infect Dis (2013) 26(1):26–34. doi: 10.1097/QCO.0b013e32835c21d1
82. Scriven JE, Rhein J, Hullsiek KH, von Hohenberg M, Linder G, Rolfes MA, et al. Early ART After Cryptococcal Meningitis Is Associated With Cerebrospinal Fluid Pleocytosis and Macrophage Activation in a Multisite Randomized Trial. J Infect Dis (2015) 212(5):769–78. doi: 10.1093/infdis/jiv067
83. Panackal AA, Wuest SC, Lin Y-C, Wu T, Zhang N, Kosa P, et al. Paradoxical Immune Responses in Non-HIV Cryptococcal Meningitis. PloS Pathog (2015) 11(5):e1004884. doi: 10.1371/journal.ppat.1004884
84. Khaw YM, et al. Th1-Dependent Cryptococcus-Associated Immune Reconstitution Inflammatory Syndrome Model With Brain Damage. Front Immunol (2020) 11:529219. doi: 10.3389/fimmu.2020.529219
85. Meya DB, Manabe YC, Boulware DR, Janoff EN. The Immunopathogenesis of Cryptococcal Immune Reconstitution Inflammatory Syndrome: Understanding a Conundrum. Curr Opin Infect Dis (2016) 29(1):10–22. doi: 10.1097/QCO.0000000000000224
86. Snarr BD, Drummond RA, Lionakis MS. It’s All in Your Head: Antifungal Immunity in the Brain. Curr Opin Microbiol (2020) 58:41–6. doi: 10.1016/j.mib.2020.07.011
87. Malik A, et al. Rapid Development of IRIS in the Form of Cryptococcal Meningitis After Beginning ART. Curr Opinion Microbiol (2012) 1(1):56–8. doi: 10.1016/j.mmcr.2012.07.002
88. Kechichian TB, Shea J, Del Poeta M. Depletion of Alveolar Macrophages Decreases the Dissemination of a Glucosylceramide-Deficient Mutant of Cryptococcus Neoformans in Immunodeficient Mice. Infect Immun (2007) 75(10):4792–8. doi: 10.1128/IAI.00587-07
89. Denham ST, Brown JCS. Mechanisms of Pulmonary Escape and Dissemination by Cryptococcus Neoformans. J Fungi (Basel) (2018) 4(1):56–8. doi: 10.3390/jof4010025
90. Charlier C, Nielsen K, Daou S, Brigitte M, Chretien F, Dromer F, et al. Evidence of a Role for Monocytes in Dissemination and Brain Invasion by Cryptococcus Neoformans. Infect Immun (2009) 77(1):120–7. doi: 10.1128/IAI.01065-08
91. Kaufman-Francis K, Djordjevic JT, Juillard P-G, Lev S, Desmarini D, Grau GER, et al. The Early Innate Immune Response to, and Phagocyte-Dependent Entry of, Cryptococcus Neoformans Map to the Perivascular Space of Cortical Post-Capillary Venules in Neurocryptococcosis. Am J Pathol (2018) 188(7):1653–65. doi: 10.1016/j.ajpath.2018.03.015
92. Yang X, Wang H, Hu F, Chen X, Zhang M. Nonlytic Exocytosis of Cryptococcus Neoformans From Neutrophils in the Brain Vasculature. Cell Commun Signal (2019) 17(1):120–7. doi: 10.1186/s12964-019-0429-0
93. Santiago-Tirado FH, Onken MD, Cooper JA, Klein RS, Doering TL. Trojan Horse Transit Contributes to Blood-Brain Barrier Crossing of a Eukaryotic Pathogen. mBio (2017) 8(1):e02183–16. doi: 10.1128/mBio.02183-16
94. Chretien F, Lortholary O, Kansau I, Neuville S, Gray F, Dromer F, et al. Pathogenesis of Cerebral Cryptococcus Neoformans Infection After Fungemia. J Infect Dis (2002) 186(4):522–30. doi: 10.1086/341564
95. Chang YC, Stins MF, McCaffery MJ, Miller GF, Pare DR, Dam T, et al. Cryptococcal Yeast Cells Invade the Central Nervous System via Transcellular Penetration of the Blood-Brain Barrier. Infect Immun (2004) 72(9):4985–95. doi: 10.1128/IAI.72.9.4985-4995.2004
96. Shi M, Li SS, Zheng C, Jones GJ, Kim KS, Zhou H, et al. Real-Time Imaging of Trapping and Urease-Dependent Transmigration of Cryptococcus Neoformans in Mouse Brain. J Infect Dis (2010) 120(5):1683–93. doi: 10.1172/JCI41963
97. Fu MS, Coelho C, De Leon-Rodriguez CM, Rossi DCP, Camacho E, Jung EH, et al. Cryptococcus Neoformans Urease Affects the Outcome of Intracellular Pathogenesis by Modulating Phagolysosomal Ph. PloS Pathog (2018) 14(6):e1007144. doi: 10.1371/journal.ppat.1007144
98. Lee K-T, Hong J, Lee D-G, Lee M, Cha S, Lim Y-G, et al. Fungal Kinases and Transcription Factors Regulating Brain Infection in Cryptococcus Neoformans. Nat Commun (2020) 11(1):1683–93. doi: 10.1038/s41467-020-15329-2
99. Vu K, Tham R, Uhrig JP, Thompson GR 3rd, Pombejra SN, Jamklang M, et al. Invasion of the Central Nervous System by Cryptococcus Neoformans Requires a Secreted Fungal Metalloprotease. mBio (2014) 5(3):e01101–14. doi: 10.1371/journal.ppat.1007144
100. Aaron PA, Jamklang M, Uhrig JP, Gelli A, et al. The Blood-Brain Barrier Internalises Cryptococcus Neoformans via the Epha2-Tyrosine Kinase Receptor. Cell Microbiol (2018) 20(3):1521. doi: 10.1111/cmi.12811
101. Subbarayal P, Karunakaran K, Winkler A-C, Rother M, Gonzalez E, Meyer TF, et al. EphrinA2 Receptor (EphA2) Is an Invasion and Intracellular Signaling Receptor for Chlamydia Trachomatis. Am Soc Microbiol (2015) 11(4):e1004846. doi: 10.1371/journal.ppat.1004846
102. Chen J, et al. Ephrin Receptor A2 is a Functional Entry Receptor for Epstein-Barr Virus. Nat Microbiol (2018) 3(2):172–80. doi: 10.1038/s41564-017-0081-7
103. Kaushansky A, Douglass AN, Arang N, Vigdorovich V, Dambrauskas N, Kain HS, et al. Malaria Parasites Target the Hepatocyte Receptor Epha2 for Successful Host Infection. Science (2015) 350(6264):1089–92. doi: 10.1126/science.aad3318
104. Pasquale EB. Eph Receptor Signalling Casts a Wide Net on Cell Behaviour. Nat Rev Mol Cell Biol (2005) 6(6):462–75. doi: 10.1038/nrm1662
105. Bachiller S, Jiménez-Ferrer I, Paulus A, Yang Y, Swanberg M, Deierborg T. Microglia in Neurological Diseases: A Road Map to Brain-Disease Dependent-Inflammatory Response. Front Cell Neurosci (2018) 12(488). doi: 10.3389/fncel.2018.00488
106. Redlich S, et al. Toll-Like Receptor Stimulation Increases Phagocytosis of Cryptococcus Neoformans by Microglial Cells. Nat Rev Mol Cell Biol (2013) 10(1):71. doi: 10.1186/1742-2094-10-71
107. Adami C, et al. S100B Expression in and Effects on Microglia. Glia (2001) 33(2):131–42. doi: 10.1002/1098-1136(200102)33:2<131::AID-GLIA1012>3.0.CO;2-D
108. Song X. Fc Receptor Signaling in Primary Human Microglia: Differential Roles of PI-3K and Ras/ERK MAPK Pathways in Phagocytosis and Chemokine Induction. J Neuroinflamm (2004) 75(6):1147–55. doi: 10.1186/1742-2094-10-71
109. Aguirre K, Miller S. MHC Class II-Positive Perivascular Microglial Cells Mediate Resistance Tocryptococcus Neoformans Brain Infection. Glia (2002) 39(2):184–8. doi: 10.1002/glia.10093
110. Blasi E. Role of Nitric Oxide and Melanogenesis in the Accomplishment of Anticryptococcal Activity by the BV-2 Microglial Cell Line. J Neuroimmunol (1995) 58(1):111–6. doi: 10.1016/0165-5728(95)00016-U
111. Panek RB, Benveniste EN. Class II MHC Gene Expression in Microglia. Regulation by the Cytokines IFN-Gamma, TNF-Alpha, and TGF-Beta. J Immunol (1995) 154(6):2846. doi: 10.1002/glia.10093
112. Zhou Q, Gault RA, Kozel TR, Murphy WJ. Immunomodulation With CD40 Stimulation and Interleukin-2 Protects Mice From Disseminated Cryptococcosis. Infect Immun (2006) 74(4):2161. doi: 10.1128/IAI.74.4.2161-2168.2006
113. Zhou Q, Gault RA, Kozel TR, Murphy WJ. Protection From Direct Cerebral Cryptococcus Infection by Interferon-γ-Dependent Activation of Microglial Cells. J Immunol (2007) 178(9):5753–61. doi: 10.4049/jimmunol.178.9.5753
114. Wormley FL Jr, Perfect JR, Steele C, Cox GM. Protection Against Cryptococcosis by Using a Murine Gamma Interferon-Producing Cryptococcus Neoformans Strain. Infect Immun (2007) 75(3):1453–62. doi: 10.1128/IAI.00274-06
115. Zeng W, Qiu Y, Tang S, Zhang J, Pan M, Zhong X. Characterization of Anti-Interferon-γ Antibodies in HIV-Negative Patients Infected With Disseminated Talaromyces Marneffei and Cryptococcosis. Open Forum Infect Dis (2019) 6(10):ofz208–8. doi: 10.1093/ofid/ofz208
116. Lee SC, Kress Y, Zhao ML, Dickson DW, Casadevall A. Cryptococcus Neoformans Survive and Replicate in Human Microglia. Lab Invest (1995) 73(6):871–9. doi: 10.1128/IAI.00274-06
117. Lee SC, et al. Human Microglia Mediate Anti-Cryptococcus Neoformans Activity in the Presence of Specific Antibody. J Neuroimmunol (1995) 62(1):43–52. doi: 10.1016/0165-5728(95)00097-L
118. Lipovsky MM, Juliana AE, Gekker G, Hu S, Hoepelman AI, Peterson PK. Effect of Cytokines on Anticryptococcal Activity of Human Microglial Cells. Clin Diagn Lab Immunol (1998) 5(3):410. doi: 10.1128/CDLI.5.3.410-411.1998
119. Drummond RA, Franco LM, Lionakis MS. Human CARD9: A Critical Molecule of Fungal Immune Surveillance. Front Immunol (2018) 9:1836. doi: 10.3389/fimmu.2018.01836
120. Rieber N, Gazendam RP, Freeman AF, Hsu AP, Collar AL, Sugui JA. Extrapulmonary Aspergillus Infection in Patients With CARD9 Deficiency. JCI Insight (2016) 1(17):e89890. doi: 10.26226/morressier.57bc1756d462b80290b4db0e
121. Lanternier F, et al. Inherited CARD9 Deficiency in 2 Unrelated Patients With Invasive Exophiala Infection. J Infect Dis (2015) 211(8):1241–50. doi: 10.1093/infdis/jiu412
122. Campuzano A, et al. CARD9 is Required for Classical Macrophage Activation and the Induction of Protective Immunity Against Pulmonary Cryptococcosis. mBio (2020) 11(1):e03005–19. doi: 10.1128/mBio.03005-19
123. Campuzano A, Wormley F. Innate Immunity Against Cryptococcus, From Recognition to Elimination. J Fungi (2018) 4(1):33. doi: 10.3390/jof4010033
124. Alves De Lima K, Rustenhoven J, Kipnis J. Meningeal Immunity and its Function in Maintenance of the Central Nervous System in Health and Disease. Annu Rev Immunol (2020) 38(1):597–620. doi: 10.1146/annurev-immunol-102319-103410
125. Faraco G, Park L, Anrather J, Iadecola C. Brain Perivascular Macrophages: Characterization and Functional Roles in Health and Disease. J Mol Med (Berlin Germany) (2017) 95(11):1143–52. doi: 10.1007/s00109-017-1573-x
126. Kierdorf K, et al. Macrophages at CNS Interfaces: Ontogeny and Function in Health and Disease. Nat Rev Neurosci (2019) 20(9):547–62. doi: 10.1038/s41583-019-0201-x
127. Williams KC, Corey S, Westmoreland SV, Pauley D, Knight H, deBakker C, et al. Perivascular Macrophages Are the Primary Cell Type Productively Infected by Simian Immunodeficiency Virus in the Brains of Macaques: Implications for the Neuropathogenesis of AIDS. J Exp Med (2001) 193(8):905–15. doi: 10.1084/jem.193.8.905
128. Matias I, Morgado J, Gomes FCA. Astrocyte Heterogeneity: Impact to Brain Aging and Disease. Front Aging Neurosci (2019) 11(59). doi: 10.3389/fnagi.2019.00059
129. Liddelow SA, et al. Neurotoxic Reactive Astrocytes Are Induced by Activated Microglia. Nature (2017) 541(7638):481–7. doi: 10.1038/nature21029
130. Shinozaki Y, Shibata K, Yoshida K, Ikenaka K, Tanaka KF, Koizumi S. Transformation of Astrocytes to a Neuroprotective Phenotype by Microglia via P2Y(1) Receptor Downregulation. Cell Rep (2017) 19(6):1151–64. doi: 10.1016/j.celrep.2017.04.047
131. Yu X, Nagai J, Khakh BS. Improved Tools to Study Astrocytes. Nat Rev Neurosci (2020) 21(3):121–38. doi: 10.1038/s41583-020-0264-8
132. Yuanjie Z, Jianghan C, Nan X, Xiaojun W, Hai W, Wanqing L. Cryptococcal Meningitis in Immunocompetent Children. Mycoses (2012) 55(2):168–71. doi: 10.1016/j.celrep.2017.04.047
133. Olave MC, et al. Infective Capacity of Cryptococcus Neoformans and Cryptococcus Gattii in a Human Astrocytoma Cell Line. Mycoses (2017) 60(7):447–53. doi: 10.1111/myc.12619
134. Lee SC, et al. Human Astrocytes Inhibit Cryptococcus Neoformans Growth by a Nitric Oxide-Mediated Mechanism. J Exp Med (1994) 180(1):365–9. doi: 10.1084/jem.180.1.365
135. Ton H, Xiong H. Astrocyte Dysfunctions and HIV-1 Neurotoxicity. J AIDS Clin Res (2013) 4(11):255–5. doi: 10.1111/myc.12619
136. Woo YH, Martinez LR. Cryptococcus Neoformans-Astrocyte Interactions: Effect on Fungal Blood Brain Barrier Disruption, Brain Invasion, and Meningitis Progression. Crit Rev Microbiol (2021) 47(2):206–23. doi: 10.1080/1040841X.2020.1869178
137. Smolders J, et al. Characteristics of Differentiated CD8+ and CD4+ T Cells Present in the Human Brain. Acta Neuropathol (2013) 126(4):525–35. doi: 10.1007/s00401-013-1155-0
138. Drummond R. Neuro-Immune Mechanisms of Anti-Cryptococcal Protection. J Fungi (2017) 4(1):4. doi: 10.3390/jof4010004
139. Engelhardt B, Ransohoff RM. The Ins and Outs of T-Lymphocyte Trafficking to the CNS: Anatomical Sites and Molecular Mechanisms. Trends Immunol (2005) 26(9):485–95. doi: 10.1016/j.it.2005.07.004
140. Korin B, et al. High-Dimensional, Single-Cell Characterization of the Brain’s Immune Compartment. Nat Neurosci (2017) 20(9):1300–9. doi: 10.1038/nn.4610
141. Derecki NC, et al. Regulation of Learning and Memory by Meningeal Immunity: A Key Role for IL-4. J Exp Med (2010) 207(5):1067–80. doi: 10.1084/jem.20091419
142. Filiano AJ, Gadani SP, Kipnis J. Interactions of Innate and Adaptive Immunity in Brain Development and Function. Brain Res (2015) 1617:18–27. doi: 10.1016/j.brainres.2014.07.050
143. Kipnis J, Gadani S, Derecki NC. Pro-Cognitive Properties of T Cells. Nat Rev Immunol (2012) 12(9):663–9. doi: 10.1038/nri3280
144. Kipnis J, Cohen H, Cardon M, Ziv Y, Schwartz M. T Cell Deficiency Leads to Cognitive Dysfunction: Implications for Therapeutic Vaccination for Schizophrenia and Other Psychiatric Conditions. Proc Natl Acad Sci USA (2004) 101(21):8180–5. doi: 10.1073/pnas.0402268101
145. Brynskikh A, Warren T, Zhu J, Kipnis J. Adaptive Immunity Affects Learning Behavior in Mice. Brain Behav Immun (2008) 22(6):861–9. doi: 10.1016/j.bbi.2007.12.008
146. Williams GP, Schonhoff AM, Jurkuvenaite A, Gallups NJ, Standaert DG, Harms AS. CD4 T Cells Mediate Brain Inflammation and Neurodegeneration in a Mouse Model of Parkinson’s Disease. Brain (2021) 144(7):2047–59. doi: 10.1093/brain/awab103
147. Ito M, et al. Brain Regulatory T Cells Suppress Astrogliosis and Potentiate Neurological Recovery. Nature (2019) 565(7738):246–50. doi: 10.1038/s41586-018-0824-5
148. Brachman RA, et al. Lymphocytes From Chronically Stressed Mice Confer Antidepressant-Like Effects to Naive Mice. J Neurosci (2015) 35(4):1530–8. doi: 10.1523/JNEUROSCI.2278-14.2015
149. Olszewski MA, Zhang Y, Huffnagle GB. Mechanisms of Cryptococcal Virulence and Persistence. Future Microbiol (2010) 5(8):1269–88. doi: 10.2217/fmb.10.93
150. Wozniak KL, Young ML, Wormley FL Jr. Protective Immunity Against Experimental Pulmonary Cryptococcosis in T Cell-Depleted Mice. Clin Vaccine Immunol (2011) 18(5):717–23. doi: 10.1128/CVI.00036-11
151. Rohatgi S, Pirofski L-A. Host Immunity to Cryptococcus Neoformans. Future Microbiol (2015) 10(4):565–81. doi: 10.2217/fmb.14.132
152. Xu J, Neal LM, Ganguly A, Kolbe JL, Hargarten JC, Elsegeiny W, et al. Chemokine Receptor CXCR3 Is Required for Lethal Brain Pathology But Not Pathogen Clearance During Cryptococcal Meningoencephalitis. Sci Adv (2020) 6(25):eaba2502. doi: 10.1126/sciadv.aba2502
153. Xu J, Ganguly A, Zhao J, Ivey M, Lopez R, Osterholzer JJ. CCR2 Signaling Promotes Brain Infiltration of Inflammatory Monocytes and Contributes to Neuropathology During Cryptococcal Meningoencephalitis. mBio (2015) 12(4):e01076–21. doi: 10.1128/mBio.01076-21
154. Dufaud C, Rivera J, Rohatgi S, Pirofski L-A. Naïve B Cells Reduce Fungal Dissemination in Cryptococcus Neoformans Infected Rag1(-/-) Mice. Virulence (2018) 9(1):173–84. doi: 10.1080/21505594.2017.1370529
155. Gupta S, et al. Disseminated Cryptococcal Infection in a Patient With Hypogammaglobulinemia and Normal T Cell Functions. Am J Med (1987) 82(1):129–31. doi: 10.1016/0002-9343(87)90388-3
156. Subramaniam K, Metzger B, Hanau LH, Guh A, Rucker L, Badri S, et al. Igm(+) Memory B Cell Expression Predicts HIV-Associated Cryptococcosis Status. J Infect Dis (2009) 200(2):244–51. doi: 10.1086/599318
157. Chamilos G, Lionakis MS, Kontoyiannis DP. Call for Action: Invasive Fungal Infections Associated With Ibrutinib and Other Small Molecule Kinase Inhibitors Targeting Immune Signaling Pathways. Clin Infect Dis (2018) 66(1):140–8. doi: 10.1093/cid/cix687
158. Szymczak WA, Davis MJ, Lundy SK, Dufaud C, Olszewski M, Pirofski L-a. X-Linked Immunodeficient Mice Exhibit Enhanced Susceptibility to Cryptococcus Neoformans Infection. mBio (2013) 4(4):244–51. doi: 10.1128/mBio.00265-13
159. Brioschi S, Wang W-L, Peng V, Wang M, Shchukina I, Greenberg ZJ. Heterogeneity of Meningeal B Cells Reveals a Lymphopoietic Niche at the CNS Borders. Science (2021) 26(9):1105–13. doi: 10.1126/science.abf9277
160. Herisson F, Frodermann V, Courties G, Rohde D, Sun Y, Vandoorne K. Direct Vascular Channels Connect Skull Bone Marrow and the Brain Surface Enabling Myeloid Cell Migration. Nat Neurosci (2018) 21(9):1209–17. doi: 10.1038/s41593-018-0213-2
161. Cai R, Pan C, Ghasemigharagoz A, Todorov MI, Förstera B, Zhao S. Panoptic Imaging of Transparent Mice Reveals Whole-Body Neuronal Projections and Skull-Meninges Connections. Nat Neurosci (2019) 22(2):317–27. doi: 10.1038/s41593-018-0301-3
162. Yao H, et al. Leukaemia Hijacks a Neural Mechanism to Invade the Central Nervous System. Nature (2018) 560(7716):55–60. doi: 10.1038/s41586-018-0342-5
Keywords: microglia, cryptococcal meningitis, fungal infection, astrocyte, HAART
Citation: Mohamed SH, Nyazika TK, Ssebambulidde K, Lionakis MS, Meya DB and Drummond RA (2022) Fungal CNS Infections in Africa: The Neuroimmunology of Cryptococcal Meningitis. Front. Immunol. 13:804674. doi: 10.3389/fimmu.2022.804674
Received: 29 October 2021; Accepted: 03 March 2022;
Published: 01 April 2022.
Edited by:
Willias Masocha, Kuwait University, KuwaitReviewed by:
Meiqing Shi, University of Maryland, United StatesRachael Dangarembizi, University of Cape Town, South Africa
Copyright © 2022 Mohamed, Nyazika, Ssebambulidde, Lionakis, Meya and Drummond. This is an open-access article distributed under the terms of the Creative Commons Attribution License (CC BY). The use, distribution or reproduction in other forums is permitted, provided the original author(s) and the copyright owner(s) are credited and that the original publication in this journal is cited, in accordance with accepted academic practice. No use, distribution or reproduction is permitted which does not comply with these terms.
*Correspondence: Rebecca A. Drummond, ci5kcnVtbW9uZEBiaGFtLmFjLnVr