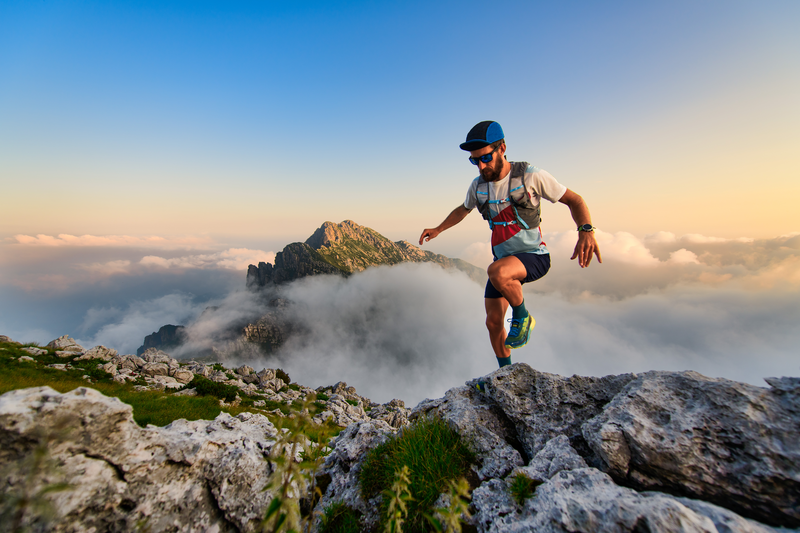
94% of researchers rate our articles as excellent or good
Learn more about the work of our research integrity team to safeguard the quality of each article we publish.
Find out more
MINI REVIEW article
Front. Immunol. , 18 January 2023
Sec. T Cell Biology
Volume 13 - 2022 | https://doi.org/10.3389/fimmu.2022.1109347
This article is part of the Research Topic T cells in age-related diseases View all 5 articles
CD8+ T cells belonging to the adaptive immune system play key roles in defending against viral infections and cancers. The current CD8+ T cell-based immunotherapy has emerged as a superior therapeutic avenue for the eradication of tumor cells and long-term prevention of their recurrence in hematologic malignancies. It is believed that an effective adaptive immune response critically relies on the help of the innate compartment. Invariant natural killer T (iNKT) cells are innate-like T lymphocytes that have been considered some of the first cells to respond to infections and can secrete a large amount of diverse cytokines and chemokines to widely modulate the innate and adaptive immune responders. Like CD8+ T cells, iNKT cells also play an important role in defense against intracellular pathogenic infections and cancers. In this review, we will discuss the CD8+ T-cell immunity contributed by iNKT cells, including iNKT cell-mediated cross-priming and memory formation, and discuss recent advances in our understanding of the mechanisms underlying memory CD8+ T-cell differentiation, as well as aging-induced impairment of T-cell immunity.
CD8+ T lymphocytes function as a key component of the adaptive immune system and are critical for combating intracellular pathogens and cancer cells (1, 2). Following acute infection, CD8+ T cells can be typically divided into three phases (3). During the first phase (priming phase), CD8+ T cells differentiated into heterogenic effector cells with proliferation, cytokine production, and cytotoxic functions (4, 5). Regarding the potential of memory cell differentiation, the effector compartments are roughly divided into memory precursor effector cells (MPECs) and short-lived effector cells (SLECs), which can be distinguished by the surface markers KLRG1 and CD127 (6, 7). With the pathogens cleared, the second phase (contraction phase) ensues, and most effector cells during robust contraction undergo activation-induced cell death. Only 5%–10% of CD8+ T cells survive and enter the memory phase, with long-term maintenance and self-renewal abilities (8, 9).
Natural killer T (NKT) cells are an innate-like T-cell subset that shares both physical and functional characteristics with NK cells and T cells (10). Unlike conventional αβT lymphocytes that recognize protein peptides presented by MHC class I or class II, NKT cells utilize their T-cell receptors (TCRs) to recognize self or foreign lipid antigens presented by a non-classical MHC class I-like molecule, CD1d (11, 12). According to their TCR repertoire, NKT cells are classified into type I and type II NKT cells. Type I NKT cells, also termed invariant NKT (iNKT) cells, express invariant TCR α chain (Vα14-Jα18 chain in mice; Vα24-Jα18 chain in humans) pairing with a limited spectrum of β chains (Vβ8.2, Vβ2, and Vβ7 in mice; Vβ11 in humans) (10, 13). iNKT cells are the most broadly studied subset of NKT cells that can be activated by α-galactosyl ceramide (α-Galcer), a marine sponge Agelas mauritiana-derived lipid (14), whereas type II NKT cells are α-Galcer non-reactive cells displaying more heterogeneous αβ chains of TCR. So far, the knowledge of type II NKT cells is less identified because they lack specific markers. The well-known role of Type II NKT cells is their suppressive immune response, especially in tumor immunity (15). In this review, we will focus on iNKT cells.
The population of iNKT cells is much smaller than that of conventional T cells. In mice, iNKT cells are 0.5% of lymphocytes in peripheral blood, 1%–2% in the spleen, and 20%–30% in the liver (16, 17). However, the number of iNKT cells in humans is significantly less and is discrepant among individuals. In humans, NKT cells are only 0.05%–1% of lymphocytes in the liver and 0.01%–0.1% of lymphocytes in peripheral blood generally, while it is up to 3% of peripheral blood mononuclear cells in some individuals (18–22). Even though iNKT cells are such a small sub-population compared with conventional peptide reactive T cells, several unique properties are positioned as a crucial regulatory population to influence diverse immune responses. First, their TCR repertoire is very limited, which contributes to the number of iNKT cell precursors being reactive to a certain lipid antigen being much higher than that of certain peptide-reactive T-cell precursors. Second, iNKT cells are autoreactive by responding to self-lipids (23). iNKT cells develop and differentiate into distinct subsets that are analogous to T helper 1 (Th1), Th2, and Th17 subsets in the thymus and move to peripheral lymphoid and non-lymphoid organs where they are further activated (24). These unique properties stimulate iNKT cells to respond to infection and inflammation through TCR engagement and/or cytokine signals within hours, with the production of a large amount of diverse cytokines to regulate innate and adaptive immune responders, such as neutrophils, NK cells, dendritic cells, macrophages, B cells, and T cells (25–30).
A large body of work has attempted to elucidate the regulatory roles of iNKT cells in affecting the response of CD8+ T cells including their effector functions and fate decisions. Among them, CD4+ T cell-mediated help is largely studied (31, 32). Like CD4+ T cells, iNKT cells also play an important role in the impact of CD8+ T-cell immunity. In this review, we will summarize current knowledge about the roles of iNKT cells in regulating CD8+ T-cell response, including cross-priming, effector function, and memory differentiation.
There are different ways to activate iNKT cells. Like conventional T cells, iNKT cells can be activated via the engagement of TCR with a glycolipid–CD1d complex. The first lipid antigen, α-Galcer, was discovered by Kawano and colleagues 25 years ago, which can potently activate both mouse and human iNKT cells (14). Thereafter, most knowledge on the function of iNKT cells came from it or its synthetic analog KRN7000. Upon activation, iNKT cells can rapidly produce a large amount of cytokines, such as IFN-γ, TNF-α, interleukin (IL)-2, IL-4, IL-5, IL-3, IL-13, IL-10, IL-9, IL-17, IL-21, and IL-22 (33, 34). Stimulated iNKT cells can also secrete diverse chemokines, including monocyte chemoattractant protein (MCP)-1, RANTES (regulated on activation, normal T cell expressed and secreted), macrophage inflammatory protein (MIP)-1α and MIP-1β, and eotaxins (35–38). Additionally, microbial lipid antigens also have been extensively identified, such as α-glucosyl diacylglycerols (αGlc-DAGs) from Streptococcus pneumoniae and group B Streptococcus, α-glucuronosylceramides and α-galacturonosylceramides from Sphingomonas spp., α-galactosyldiacylglycerols (α-GalDAGs) from Borrelia burgdorferi, and phosphatidylinositol mannosides (PIMs) from Mycobacterium tuberculosis (39–43). These microbe-derived glycolipids sustained iNKT cell activation through engagement of their invariant TCRs (Figure 1). The high-affinity lipids such as α-glucuronosylceramides elicit iNKT cell production of IFN-γ and IL-4 similar to α-Galcer (39). In the absence of pathogen-associated lipid antigens, or lipid antigens with low affinity, cytokine-driven activation of iNKT cells is dominant (44, 45). iNKT cells can be activated by antigen-presenting cells (APCs) that have been stimulated by Toll-like receptor agonists in the absence of infection, and this activation requires a lipid–CD1d complex, which suggests that self-lipids can contribute to iNKT cell activation (44, 46, 47). This activation requires iNKT cells to receive the signals from the complex of TCR–self-lipid–CD1d and pro-inflammatory cytokines, such as IL-12, IL-18, IL-23, and IL-25, released from APCs. Even in the absence of TCR engagement, iNKT cells can also be activated by responding to pro-inflammatory cytokines due to their high expression of the cytokine receptors in the steady state (44). IL-12 is the best-described cytokine mediator of iNKT cell activation. In some bacterial and viral infections, the stimulated APCs produce a large amount of IL-12, which is sufficient to activate iNKT cells, whereas without IL-12 production, APCs cannot activate iNKT cells, even in the presence of identified microorganism-derived lipid antigens, which indicates that cytokine signaling plays a crucial role in iNKT cell activation (48–50). In addition, cytokine-induced activation results in iNKT cell production of IFN-γ but not IL-4 (44, 51), which suggests that IL-12 may induce the activation of a subset of iNKT cells, or it only provides a weak stimulatory signal due to failure of proliferation. Furthermore, it is also found that IL-18 signaling is essential for splenic iNKT cells rather than liver iNKT cells during cytomegalovirus (CMV) infection (50), which implies that cytokine signaling may affect not only iNKT cell response but also localization.
Figure 1 TCR and/or cytokine-driven iNKT cell activation. There are two pathways involved in the physiological activation of iNKT cells: 1) for pathogens expressing affinity lipid antigens, iNKT cell activation is dominantly driven by TCR signals provided by the complex of high-affinity microbial lipid antigen presented by CD1d of an APC. It is less dependent on additional cytokine signals, which are provided by APCs after stimulation of pattern recognition receptors (PRRs). 2) For pathogens lacking lipid antigens or with low-affinity lipid antigens, iNKT cell activation is dominantly driven by cytokine signals. The engagement of microbial products with PRRs leads to APC production of pro-inflammatory cytokines such as IL-12. In this case, TCR signals are commonly still required, which is provided by self-lipid or low-affinity lipid antigens. TCR, T-cell receptor; iNKT, invariant natural killer T; APC, antigen-presenting cell.
To date, mouse iNKT cells have been classified into five effector subsets on the basis of their specific markers referring to cytokine production and transcriptional factor signatures. Analogous to Th cell subsets, they include NKT1, NKT2, NKT17, iNKT10, and follicular help NKT (iNKTFH). NKT1 cells are enriched in the spleen and liver and express Th1 cell-associated transcription factor T-bet. However, unlike Th1 cells, they express both type 1 and type 2 cytokines (52, 53). NKT2 cells are primarily located in the medullary area of the thymus and T-cell zone of the spleen and mesenteric lymph nodes as well as the lungs. NKT2 cells can produce IL-4 and IL-13 and therefore resemble Th2 cells (53). Similar to Th17 cells, IL-17A-producing iNKT cells are termed NKT17, which are primarily located in the lymph nodes, skin, and lungs (53). NKTFH cells are similar to TFH cells that are primarily located in the spleen and act in germinal centers to promote affinity maturation of antibodies. iNKT10 cells are IL-10-producing cells with a regulatory phenotype but without foxp3 expression (54). Therefore, the regulation role of iNKT cells in the modulation of other immune cells may be partly associated with diverse cytokine production by functionally distinct subsets of these cells, and the different tissue-homing preferences of these iNKT cell subsets may also ultimately influence local immune responses. Mitchell Kronenberg and colleagues have recently provided an excellent in-depth review of these subsets of iNKT cells (24).
Priming of naïve T cells requires three key signals: TCR engagements, costimulatory signals provided by CD28 and TNF receptor family, and cytokine signals. Dendritic cells (DCs) are APCs responsible for providing the three signals of cross-priming of CD8+ T cells. Cross-priming is a process that permits specialized DCs to cross-presentation of extracellular antigens to CD8+ T cells conferring their effector functions to defend against tumors and intracellular pathogenic infections. This immunogenic cross-presentation requires the presence of pathogen-derived molecule patterns and/or CD4+ T help cells, a process termed as “licensing” of DCs, which prevents unwanted immune responses for the innocuous antigens or autoantigens (31, 55). Classically, the licensing is mediated through CD40–CD40L interactions of CD4+ T help cells with DCs, which is the primary mechanism that supports CD8+ T-cell response by CD4+ T cells (56, 57). However, a growing number of studies have suggested that iNKT cells are another subset for DC licensing (30, 58). Consistent with CD4+ T-cell licensing, iNKT cell-mediated DC licensing also allows DC functional maturation in a CD40L-dependent manner (59). After iNKT cells recognize their cognate self or foreign lipid antigens displayed by CD1d of APCs, immediate activation of iNKT cells induces transient upregulation of CD40L on iNKT cells and subsequently activates DCs via interaction of CD40L with CD40. The cross-talk between iNKT cells and DCs via CD40–CD40L interaction triggers DC functional maturation resulting in the upregulation of costimulatory proteins and IL-12 production, which further activate iNKT cells (60, 61). Additionally, TNF-α and IFN-γ released by DCs and iNKT cells, respectively, assist in CD40–CD40L-mediated DC licensing. As a consequence, CD8+ T-cell response is promoted by the licensed DCs through interaction with the costimulatory proteins and inflammatory signals (61, 62). CD27 is a member of TNFR family, with stimulation of CD27 signals via interaction with CD70. Distinct from CD28, CD27 costimulation signal not only promotes CD8+ T-cell effector differentiation but also enhances their survival (63, 64). This survival relies completely on IL-2R signaling and autocrine IL-2 production (63). Additionally, CD4+ T cell-mediated help via CD27 costimulation is also involved in memory CD8+ T-cell development (65, 66), as well as in preventing CD8+ T cells from tolerance by reducing PD-1 expression (67). Consistent with CD4+ T cell-mediated help, the interaction of CD70 and CD27 is also crucial for iNKT cell-mediated promotion of CD8+ T-cell response including CD8+ T cell-mediated antitumor immunity (62).
When iNKT cells are primed, they will accumulate in the marginal zone of the spleen, where they co-interact with CD8α+ conventional DCs (CD8α+cDCs) with a unique expression of chemokine receptor XCR1, a major type of APCs responsible for cross-priming (68, 69). After initial activation, CD8+ T cells will recruit other types of cells to the site of initial antigen recognition to create their own optimal priming microenvironment. Plasmacytoid DCs (pDCs) are another subset of DCs playing a critical role in anti-viral infections via the production of type I interferon (IFN) (70). Shin-ichiro Fujii and colleagues suggested that iNKT cell-mediated DC (XCR1+DC) licensing for cross-priming of CD8+ T cells is through induction of the cross-talk between pDCs and XCR1+ DCs (71). This cross-talk is important for the cross-presentation of XCR1+ DCs, which is further supported by later studies (72–74). Cell-mediated licensing requires the same DC to physically interact with the help cells and CD8+ T cells. Such interactions are usually governed by chemokines and their receptors. For CD4+ T cell-mediated help, the DCs licensed by CD4+ T cells express a high level of CCR5 ligands and recruit CCR5-expressing CD8+ T cells (naïve and effector) and process cross-priming. Unlike CD4+ T cell-mediated help, iNKT cell-licensed DCs produce CCL17 to attract CCR4 (CCL17 receptor) expressing naïve CD8+ T cells (75, 76). Nine years later, they further extend this notion that iNKT cell-mediated induction of CXCR3 and CCR4 expression on CD8+ T cells could affect the fate of CD8+ T cells (77). Consistently, a recent study shows that iNKT cells can promote the generation of functional CXCR3+CCR4+CD8+ T cells, which mediate rapid rejection of allogeneic hepatocytes engrafted in the liver (78). Moreover, on DCs, CD1d molecules are expressed in all hematopoietic cells, including monocytes, B cells, and T cells (11, 79). Our study showed that there is a direct interaction between TCRs (iNKT cells) and CD1d molecules (CD8+ T cells), which promotes CD8+ T-cell activation in a DC-independent manner (80).
Immunological memory is established after successful priming, which is a fundamental feature of the acquired immune response. Clearly, the generation of long-lived memory CD8+ T cells is one of the primary goals in the development of therapeutic vaccines. The role of iNKT cells in the promotion of CD8+ T-cell response as well as memory formation in immunization, pathogenic infection, and tumor immunity in mice or humans has largely been addressed (81, 82). Considering that α-Galcer provokes strong iNKT cell activity in both mice and humans, it has long been used as a prototypical immune adjuvant co-administrated with antigens of interest in the arrangement of experimental and clinical settings. Some of the most extensive studies are using inactivated influenza virus as the source of antigen with co-administration of α-Galcer for iNKT cell activation, which significantly enhances both cellular and humoral responses (83). Injection of soluble α-Galcer limited the immediate CD8+ T-cell response but promoted the survival of long-lived memory populations, which are capable of protection from the influenza A virus challenge (84). In another study, it was suggested that α-Galcer conjugated with the peptides of influenza A virus-associated protein induces not only robust effector CD8+ T-cell response but also memory CD8+ T-cell generation (85). The difference in CD8+ T-cell priming/effector function may be attributed to the α-Galcer and antigen peptides accessing the same APCs to enable licensing events to occur. A similar protective effect was also shown in a mouse cytomegalovirus (MCMV) infection model. Injection of soluble α-Galcer increased central memory CD8+ T-cell differentiation (86). In tumor models, studies have shown that α-Galcer-loaded tumor cells induce a strong antitumor immunity along with memory cytotoxic T-lymphocyte (CTL) generation in a DC-dependent manner (87) as well as a recent popular therapeutic avenue, CAR-iNKT cells, which present superior antitumor effects along with the generation of antitumor central memory CD8+ T cells (81). Additionally, some studies also show that activation of autologous iNKT cells induces strong antitumor effects of CD8+ T cells including prevention of CD8+ T-cell exhaustion (88–91). Resident memory T cells are crucial for local immunity and recall response, which is considered more potential subsets in vaccine designation (92). A recent study shows that induction of iNKT cell activation can promote the generation of liver-resident memory CD8+ T cells, which prevents malaria (93). In naïve mice raised in specific pathogen-free and germ-free conditions, a small CD8+ T-cell population displaying memorial phenotypes is named “virtual memory” CD8+ T cells (CD8+ TVM). Some studies have shown that IL-4-producing iNKT cells increase the abundance of CD8+ TVM (94–96). The increased CD8+ TVM cells could provide broad protection (94). In conclusion, activation of iNKT cells not only can promote CD8+ T-cell response but also has a high potential in helping memory T-cell generation.
The knowledge of mechanisms of iNKT cells in help for memory CD8+ T-cell differentiation is critical for the development of new vaccination strategies. Unlike a large body of investigations in CD4+ T cell help for memory CD8+ T-cell formation, the studies about iNKT cell-mediated help are still limited. There are several studies that have tried to explore the potential mechanisms of iNKT cells in affecting memory CD8+ T-cell memory differentiation, which is concluded in Figure 2. pDCs are well known as an additional subset of DCs supporting conventional DC cross-priming of CD8+ T cells in a type I IFN-dependent manner (72, 74). An early study shows that the cross-talk between pDC and cDC, which is mediated by activated iNKT cells, is important for memory CD8+ T-cell formation (71). A recent study provides evidence that there is a cross-talk among iNKT cell, DC, and CD8+ T cells, which is involved in CD8+ T-cell memory programming (77). In line with our findings, the live imaging showed the presence of direct interaction of iNKT cells and CD8+ T cells at the early stage (80). A consistent cross-talk among iNKT cell, CD8+ T cell, and DC is formed at a later stage, and the fate of CD8+ T-cell memory differentiation is regulated by CXCR3/IFN-γ and IL-4/CCL17/CCR4 axes, which drive CD8+ T-cell differentiation into MPECs and SLECs, respectively. However, in contrast to this notion, the CXCR3 has been believed to drive CTL toward effector fate rather than memory fate (97, 98), whereas CCR4 is associated with memory T help cell development and phenotypes of memory CD8+ T cells (99–101). In addition to the above studies, an early study suggests that activation of iNKT cells enhances memory CD8+ T-cell homeostatic proliferation depending on IL-4-STAT6 signaling (95); similar to this study, IL-4-producing Kruppel-like factor (KLF) 13-positive iNKT cells is critical for thymic memory like CD8+ T-cell formation (96).
Figure 2 iNKT cell-mediated CD8+ T-cell immunity. I) iNKT cell-mediated cross-priming of CD8+ T cells after responding to α-Galcer: 1) iNKT cells are activated by α-Galcer without any costimulatory signals. 2) Immature DCs capture antigens and interact with activated iNKT cells to undergo iNKT cell-induced maturation via CD40–CD40L and inflammatory cytokine IFN-γ and TNF-α. Activated iNKT cells can also activate plasmacytoid DC (pDC) via OX40L–OX40 signaling; thereafter, pDC assists DC maturation and cross-priming of CD8+ T cells in a type I IFN-dependent manner. iNKT cell-mediated DC licensing promotes DC cross-presentation of antigens to CD8+ T cells via upregulation of costimulatory signals such as CD80/86–CD28, CD70–CD27, and cytokines IL-12, IFN-γ, and TNF-α. II) Without exogenous lipid antigen: iNKT cells can be activated by CD8+ T cells through CD1d–self lipid–TCR engagement. Activated iNKT cells enhance CD8+ T-cell effector function in an IFN-γ dependent manner. III) iNKT cell-mediated CD8+ T-cell fate decision: 1) iNKT cell-mediated cross-talk of pDC–cDC–CD8+ T cells promotes memory CD8+ T-cell development. 2) iNKT-mediated cDC licensing SLEC development via CCL17/CCR4 or MPEC development via CXCR3/CXCL9 and IFN-γ. iNKT, invariant natural killer T; DCs, dendritic cells; TCR, T-cell receptor; SLEC, short-lived effector cell; MPEC, memory precursor effector cell.
In conclusion, since iNKT cells rapidly produce diverse cytokines and chemokines and also can interact with APCs and T cells directly, the roles of iNKT cells in CD8+ T-cell fate regulation are intricate, which still remains largely unknown, as well as the mechanism.
A significant characteristic of the aging process is age-associated immune dysfunction, which results in an increase in susceptibility to infection, cancer, and autoimmune diseases. Reduction of the TCR repertoire, imbalance of naïve and memory compartments, T-cell senescence, and reduced proliferation and cytokine production are considered to be the hallmarks of T-cell aging (102, 103). The most notable changes are in CD8 rather than in CD4 T cells since the latter maintain their populations through homeostatic proliferation (104). Clearly, aging-induced alteration in either numbers or functions of iNKT cells could affect the quality of T-cell response. Several studies have reported that the number/frequency of NKT cells is increased in older mice and human beings (105–107). This increase exhibits an unusual effect of aging, as other types of immune cells decrease or are constant in number. Additionally, it is also found that the increased NKT cells originate from newly made cells. In contrast, it has been reported that Vα14 and Vα24 NKT cells are decreased in aged mice and humans, particularly in the liver (108, 109). A recent study also shows that aging changes the subset composition of iNKT cells, as well as decreases their proliferative capacity (110). Collectively, the mechanisms responsible for the increase or decrease of NKT cells in aged mice remain to be elucidated. In addition to changes in the number of NKT cells, the cytokine production of NKT cells is also changed in aged mice. Several data support the notion that NKT cells tend to differentiate to an immunosuppressive phenotype by an increase in IL-4 and IL-10 production in older mice and humans (105, 107, 111). The effects of NKT cells to modulate several aspects of T-cell function are different in aged mice. The suppressive functions of NKT cells on the effector phase of T-cell immunity including proliferation and cytokine expression were observed in aged mice (105, 112). As previously described, virtual memory T cells (TVM) are antigen naïve T cells, which are generated by homeostatic proliferation. Studies have reported that IL-4 is involved in the generation of TVM, and the thymic NKT pool is critical for enlarging frequencies of IL-4-producing cells (94, 95, 113, 114). Over the course of aging, TVM is accumulated (115). This age-related accumulation of TVM decreases the primary response of CD8+ T cells due to the development of senescence in TVM (116). Therefore, regardless that NKT cells affect TVM generation, the senescence of TVM in the context of aging should also be elucidated. Although relatively meager, the current set of studies supports the notion that aging affects the number and function of NKT cells, which will provoke a cascade of decreased innate and adaptive immune responses including CD8+ T-cell response, with the subsequent appearance of age-related diseases.
A notion that should be considered is that the knowledge of iNKT cell-mediated help for CD8+ T-cell response or memory formation is largely concluded by α-Galcer, a potent synthetic glycosphingolipid antigen. It may not represent all functions of iNKT cells. Examples presented in models of dextran sulfate sodium (DSS)-induced colitis show that iNKT cells suppress pathogenic NK1.1+CD8+ T cells via expansion Treg cells using CD1d or Jα18 knockout mice (117). Foreign lipid antigens are not always present. Indeed, most microorganisms lack cognate lipid antigens, as well as cancer and autoimmune diseases (118). The critical roles of iNKT cells in these contexts have been largely addressed without using the exogenous α-Galcer treatment. As discussed above, iNKT cells are activated by self-lipids and/or pro-inflammatory cytokines. A small number of self-lipid antigens have been explored, which are generally divided into glycosphingolipids and phospholipids, including isoglobotrihexosylceramide (iGb3) (119), β-glucosylceramide (β-GlcCer) (120), α-glycosylceramides (121), lysophosphatidylcholine (lysoPC) (122), plasmalogen lysophosphatidylethanolamine (pLysoPE), and lysophosphatidic acid (eLPA) (123). Although these self-lipids have been identified, their potential functions including whether they regulate CD8+ T-cell response are still largely unknown. Recent studies showed that self-lipids presented endoplasmic reticulum (ER)-stressed APCs can potently activate iNKT cells as α-Galcer dose (124), while some studies suggested that self-lipid and/or cytokine-driven stimulation-induced iNKT cell production of IFN-γ but not IL-4 (44, 51, 80). For regulation of CD8+ T-cell response, Albert Bendelac and colleagues suggested that the response of iGb3 enhances the cross-priming of CD8+ T cell-like α-Galcer dose (75). Se-Ho Park and colleagues also supported the notion that self-lipid-activated iNKT cells strengthen the primary response and secondary response of CTLs, while the potential self-lipid is not explored (80, 125). The optimal roles of iNKT cells in strengthening the antitumor response of CTLs were also determined (80). Further study to explore the physiological role of iNKT cells in regulating CD8+ T-cell immunity in the context of viral infection and cancers is necessary for the improvement of immunotherapy in chronic viral infectious diseases and cancers.
iNKT cells are a manipulated subset of T lymphocytes, with diverse regulatory functions that affect the effector function and fate of CD8+ T cells, which are attributed to their ability to release a rapid burst of cytokines and chemokines without the need for clonal expansion and differentiation, as well as providing indispensable costimulatory signals for licensing of DCs. Compared with CD4+ Th cells, iNKT cells play non-redundant roles in supporting CD8+ T-cell optimal priming and secondary response or regulating CD8+ T-cell fate decision (death or memory development) at their interaction timing, site, and subsets of iNKT cells. Additionally, significant changes in numbers and functions are determined in iNKT cells with age, and limited research has found that iNKT cells impair T-cell immunity. Further studies involving iNKT cell-mediated CD8+ T-cell immunity, especially in the modulation of antigen-experienced memory T-cell development, and antigen naïve TVM formation in both young and aged conditions need to be widely explored. Meanwhile, the physiological role of iNKT cells responding to natural lipid and/or inflammatory cytokines in critical diseases, such as chronic viral infectious disease and cancers, in which both CD8+ T and iNKT cells are laying critical roles, still needs to be elucidated.
YQ prepared the manuscript. XB critically reviewed the manuscript. MZ edited and finalized the manuscript. All authors contributed to the article and approved the submitted version.
This work was supported by the National Natural Science Foundation of China (82171717 and 32100712) and the 2021 High-level Personnel Project Funding of Jiangsu Province (JSSCBS20210104). This work was also supported by the Fundamental Research Funds for the Central Universities (2242022K4007).
The authors declare that the research was conducted in the absence of any commercial or financial relationships that could be construed as a potential conflict of interest.
All claims expressed in this article are solely those of the authors and do not necessarily represent those of their affiliated organizations, or those of the publisher, the editors and the reviewers. Any product that may be evaluated in this article, or claim that may be made by its manufacturer, is not guaranteed or endorsed by the publisher.
1. Wong P, G Pamer E. CD8 T cell responses to infectious pathogens. Annu Rev Immunol (2003) 21:29–70. doi: 10.1146/annurev.immunol.21.120601.141114
2. Raskov H, Orhan A, Christensen JP, Gögenur I. Cytotoxic CD8+ T cells in cancer and cancer immunotherapy. Br J Cancer (2021) 124:395–67. doi: 10.1146/annurev.immunol.21.120601.141114
3. Williams MA, Bevan MJ. Effector and memory CTL differentiation. Annu Rev Immunol (2007) 25:171–92. doi: 10.1146/annurev.immunol.25.022106.141548
4. Gerlach C, Heijst JWJv, Swart E, Sie D, Armstrong N, Kerkhoven RM, et al. One naive T cell, multiple fates in CD8+ T cell differentiation. J Exp Med (2010) 207:1235–46. doi: 10.1084/jem.20091175
5. Stemberger C, Huster KM, Koffler M, Anderl F, Schiemann M, Wagner H, et al. A single naive CD8+ T cell precursor can develop into diverse effector and memory subsets. Immunity (2007) 27:985–97. doi: 10.1016/j.immuni.2007.10.012
6. Mescher MF, Curtsinger JM, Agarwal P, Casey KA, Gerner M, Hammerbeck CD, et al. Signals required for programming effector and memory development by CD8+ T cells. Immunol Rev (2006) 211:81–92. doi: 10.1111/j.0105-2896.2006.00382.x
7. Parish IA, Kaech SM. Diversity in CD8(+) T cell differentiation. Curr Opin Immunol (2009) 21:291–7. doi: 10.1016/j.coi.2009.05.008
8. Martin MD, Badovinac VP. Defining memory CD8 T cell. Front Immunol (2018) 20:02692. doi: 10.3389/fimmu.2018.02692
9. Kaech SM, Cui W. Transcriptional control of effector and memory CD8+ T cell differentiation. Nat Rev Immunol (2012) 12:749–61. doi: 10.1038/nri3307
10. Godfrey DI, MacDonald HR, Kronenberg M, Smyth MJ, Kaer LV. NKT cells: what's in a name? Nat Rev Immunol (2004) 4:231–7. doi: 10.1038/nri1309
11. Bendelac A, Rivera MN, Park SH, Roark JH. Mouse CD1-specific NK1 T cells: development, specificity, and function. Annu Rev Immunol (1997) 15:535–62. doi: 10.1146/annurev.immunol.15.1.535
12. Exley M, Garcia J, Balk SP, Porcelli S. Requirements for CD1d recognition by human invariant Valpha24+ CD4-CD8- T cells. J Exp Med (1997) 186:109–20. doi: 10.1084/jem.186.1.109
13. Dellabona P, Padovan E, Casorati G, Brockhaus M. A lanzavecchia. An invariant V alpha 24-J alpha Q/V beta 11 T cell receptor is expressed in all individuals by clonally expanded CD4-8- T cells. J Exp Med (1994) 180:1171–6. doi: 10.1084/jem.180.3.1171
14. Kawano T, Cui J, Koezuka Y, Toura I, Kaneko Y, Motoki K, et al. CD1d-restricted and TCR-mediated activation of valpha14 NKT cells by glycosylceramides. Scinece (1997) 278:1626–9. doi: 10.1126/science.278.5343.1626
15. Singh AK, Tripathi P, Cardell SL. Type II NKT cells: an elusive population with immunoregulatory properties. Front Immunol (2018) 9:1969. doi: 10.3389/fimmu.2018.01969
16. Matsuda 1 JL, Naidenko OV, Gapin L, Nakayama T, Taniguchi M, Wang CR, et al. Tracking the response of natural killer T cells to a glycolipid antigen using Cd1d tetramers. J Exp Med (2000) 192:741–54. doi: 10.1084/jem.192.5.741
17. Benlagha 1 K, Weiss A, Beavis A, Teyton L, Bendelac A. In vivo identification of glycolipid antigen–specific T cells using fluorescent Cd1d tetramers. J Exp Med (1999) 191:1895–904. doi: 10.1084/jem.191.11.1895
18. Gumperz JE, Miyake S, Yamamura T, Brenner MB. Functionally distinct subsets of CD1d-restricted natural killer T cells revealed by CD1d tetramer staining. J Exp Med (2002) 195:625–36. doi: 10.1084/jem.20011786
19. Lee PT, Benlagha K, Teyton L, Bendelac A. Distinct functional lineages of human V(alpha)24 natural killer T cells. J Exp Med (2002) 195:637–41. doi: 10.1084/jem.20011908
20. Berzins 1 SP, Cochrane AD, Pellicci DG, Smyth MJ, Godfrey DI. Limited correlation between human thymus and blood NKT cell content revealed by an ontogeny study of paired tissue samples. Eur J Immunol (2005) 35:1399–407. doi: 10.1002/eji.200425958
21. Chan 1 AC, Serwecinska L, Cochrane A, Harrison LC, Godfrey DI, Berzins SP. Immune characterization of an individual with an exceptionally high natural killer T cell frequency and her immediate family. Clin Exp Immunol (2009) 156:238–45. doi: 10.1111/j.1365-2249.2009.03888.x
22. Lee PT, Putnam A, Benlagha K, Teyton L, Gottlieb PA, Bendelac A. Testing the NKT cell hypothesis of human IDDM pathogenesis. J Clin Invest (2002) 110:793–800. doi: 10.1172/JCI15832
23. Gapin L. iNKT cell autoreactivity: what is “self” and how is it recognized? Nat Rev Immunol (2010) 10:272–7. doi: 10.1038/nri2743
24. Crosby CM, Kronenberg M. Tissue-specific functions of invariant natural killer T cells. Nat Rev Immunol (2018) 18:559–74. doi: 10.1038/s41577-018-0034-2
25. Brennan PJ, Brigl M, Brenner MB. Invariant nature killer T cells: an innate activation scheme linked to diverse effector functions. Nat Rev Immunol (2013) 13:101–17. doi: 10.1038/nri3369
26. Goto T, Ito Y, Satoh M, Nakamoto S, Nishizawa N, Hosono K, et al. Activation of iNKT cells facilitates liver repair after hepatic ischemia reperfusion injury through acceleration of macrophage polarization. Front Immunol (2021) 12:754106. doi: 10.3389/fimmu.2021.754106
27. Kim EY, Ner-Gaon H, Varon J, Cullen AM, Guo J, Choi J, et al. Post-sepsis immunosuppression depends on NKT cell regulation of mTOR/IFN-γ in NK cells. J Clin Invest. (2020) 130:3238–52. doi: 10.1172/JCI128075
28. Cruz MS, Loureiro JP, Oliveira MJ, Macedo MF. The iNKT cell-macrophage axis in homeostasis and disease. Int J Mol Sci (2022) 23:1640. doi: 10.3390/ijms23031640
29. Leadbetter EA, Karlsson MCI. Invariant natural killer T cells balance b cell immunity. Immunol Rev (2021) 299:93–107. doi: 10.1111/imr.12938
30. Gottschalk C, Mettke E, Kurts C. The role of invariant natural killer T cells in dendritic cell licensing,cross-priming, and memory CD8+ T cell generation. Front Immunol (2015) 6:379. doi: 10.3389/fimmu.2015.00379
31. Bevan MJ. Helping the CD8+T cell response. Nat Rev Immunol (2004) 4:595–602. doi: 10.1038/nri1413
32. Laidlaw BJ, Craft JE, Kaech SM. The multifaceted role of CD4+ T cells in the regulation of CD8+ T cell memory maturation. Nat Rev Immunol (2016) 16:102–11. doi: 10.1038/nri.2015.10
33. Coquet JM, Chakravarti S, Kyparissoudis K, McNab FW, Pitt LA, McKenzie BS, et al. Diverse cytokine production by NKT cell subsets and identification of an IL-17-producing CD4-NK1.1- NKT cell population. Proc Natl Acad Sci U.S.A. (2008) 105:11287–92. doi: 10.1073/pnas.0801631105
34. Salio SM, Silk JD, Jones EY, Cerundolo V. Biology of CD1- and MR1-restricted T cells. Annu Rev Immunol (2014) 32:323–66. doi: 10.1146/annurev-immunol-032713-120243
35. Chang Y-J, Huang J-R, Tsai Y-C, Hung J-T, Wu D, Fujio M, et al. Potent immune-modulating and anticancer effects of NKT cell stimulatory glycolipids. Proc Natl Acad Sci U.S.A. (2007) 104:10299–304. doi: 10.1073/pnas.0703824104
36. Kim JH, Chung DH. CD1d-restricted IFN-γ-secreting NKT cells promote immune complex-induced acute lung injury by regulating macrophage-inflammatory protein-1α production and activation of macrophages and dendritic cells. J Immunol (2011) 186:1432–41. doi: 10.4049/jimmunol.1003140
37. Bilenki L, Yang J, Fan Y, Wang S, Yang X. Natural killer T cells contribute to airway eosinophilic inflammation induced by ragweed through enhanced IL-4 and eotaxin production. Eur J Immunol (2004) 34:345–54. doi: 10.1002/eji.200324303
38. Faunce DE, Stein-Streilein J. NKT cell-derived RANTES recruits APCs and CD8+ T cells to the spleen during the generation of regulatory T cells in tolerance. J Immunol (2002) 169:31–8. doi: 10.4049/jimmunol.169.1.31
39. Kinjo Y, Illarionov P, Vela JL, Pei B, Girardi E, Li X, et al. Invariant natural killer T cells recognize glycolipids from pathogenic gram-positive bacteria. Nat Immunol (2011) 12:966–74. doi: 10.1038/ni.2096
40. Chang Y-J, Kim HY, Albacker LA, Lee HH, Baumgarth N, Akira S, et al. Influenza infection insuckling mice expands an NKT cell subset that protects against airway hyper reactivity. J Cli Invest (2011) 121:57–69. doi: 10.1172/JCI44845
41. Kinjo Y, Wu D, Kim G, Xing G-W, Poles MA, Ho DD, et al. Recognition of bacterial glycosphingolipids by natural killer T cells. Nature (2005) 434:520–5. doi: 10.1038/nature03407
42. Kinjo Y, Tupin E, Wu D, Fujio M, Garcia-Navarro R, Rafii-El-Idrissi Benhnia M, et al. Natural killer T cells recognize diacylglycerol antigens from pathogenic bacteria. Nat Immunol (2006) 7:978–86. doi: 10.1038/ni1380
43. Brown LCW, Penaranda C, Kashyap PC, Williams BB, Clardy J, Kronenberg M, et al. Production of alpha-galactosylceramide by a prominent member of the human gut microbiota. PloS Biol (2013) 11:e1001610. doi: 10.1371/journal.pbio.1001610
44. Brigl M, Bry L, Kent SC, Gumperz JE, Brenner MB. Mechanism of CD1d-restricted natural killer T cell activation during microbial infection. Nat Immunol (2003) 4:1230–7. doi: 10.1038/ni1002
45. Brigl M, Tatituri RVV, Watts GFM, Bhowruth V, Leadbetter EA, Barton N, et al. Innate and cytokine-driven signals, rather than microbial antigens, dominate in natural killer T cell activation during microbial infection. J Exp Med (2011) 208:1163–77. doi: 10.1084/jem.20102555
46. Paget C, Mallevaey T, Speak AO, Torres D, Fontaine J, Sheehan KCF, et al. Activation of invariant NKT cells by toll-like receptor 9−stimulated dendritic cells requires type I interferon and charged glycosphingolipids. Immunity (2007) 27:597–609. doi: 10.1016/j.immuni.2007.08.017
47. Darmoise A, Teneberg S, Bouzonville L, Brady RO, Beck M, Kaufmann SHE, et al. Lysosomal α-galactosidase controls the generation of self lipid antigens for natural killer T cells. Immunity (2010) 33:216–28. doi: 10.1016/j.immuni.2010.08.003
48. Wesley JD, Tessmer MS, Chaukos D, Brossay L. NK cell–like behavior of Vα14i NK T cells during MCMV infection. PloS Pathog (2008) 4:e1000106. doi: 10.1371/journal.ppat.1000106
49. Tyznik AJ, Tupin E, Nagarajan NA, Her MJ, Benedict CA, Kronenberg M. The mechanism of invariant NKT cell responses to viral danger signals. J Immunol (2008) 181:4452–6. doi: 10.4049/jimmunol.181.7.4452
50. Anderson CK, Reilly SP, Brossay L. The invariant NKT cell response has differential signaling requirements during antigen-dependent and antigen-independent activation. J Immunol (2022) 206:132–40. doi: 10.4049/jimmunol.2000870
51. A Nagarajan N, Kronenberg M. Invariant NKT cells amplify the innate immune response to lipopolysaccharide. J Immunol (2007) 178:2706–13. doi: 10.4049/jimmunol.178.5.2706
52. Barral P, Sánchez-Niño MD, Rooijen Nv, Cerundolo V, Batista FD. The location of splenic NKT cells favours their rapid activation by blood-borne antigen. EMBO J (2012) 31:2378–90. doi: 10.1038/emboj.2012.87
53. Lee YJ, Wang H, Starrett GJ, Phuong V, Jameson SC, Hogquist KA. Tissue specific distribution of iNKT cells impacts their cytokine response. Immunity (2015) 43:566–78. doi: 10.1016/j.immuni.2015.06.025
54. Sag D, Krause P, Hedrick CC, Kronenberg M, Wingender G. IL-10–producing NKT10 cells are a distinct regulatory invariant NKT cell subset. J Clin Invest (2014) 124:3725–40. doi: 10.1172/JCI72308
55. Smith CM, Wilson NS, Waithman J, Villadangos JA, Carbone FR, Heath WR, et al. Cognate CD4(+) T cell licensing of dendritic cells in CD8(+) T cell immunity. Nat Immunol (2004) 5:1143–8. doi: 10.1038/ni1129
56. Bennett SR, Carbone FR, Karamalis F, Flavell RA, Miller JF, Heath WR. Help for cytotoxic-t-cell responses is mediated by CD40 signalling. Nature (1998) 393:478–80. doi: 10.1038/30996
57. Bedoui S, Heath WR, Mueller SN. CD4(+) T-cell help amplifies innate signals for primary CD8(+) T-cell immunity. Immunol Rev (2016) 272:52–64. doi: 10.1111/imr.12426
58. Bousso P, Albert ML. Signal 0 for guided priming of CTLs: NKT cells do it too. Nat Immunol (2010) 11:284–6. doi: 10.1038/ni0410-284
59. Fujii S-I, Liu K, Smith C, Bonito AJ, Steinman RM. The linkage of innate to adaptive immunity via maturing dendritic cells in vivo requires CD40 ligation in addition to antigen presentation and CD80/86 costimulation. J Exp Med (2004) 199:1607–18. doi: 10.1084/jem.20040317
60. Kitamura H, Iwakabe K, Yahata T, Nishimura S, Ohta A, Ohmi Y, et al. The natural killer T (NKT) cell ligand α-galactosylceramide demonstrates its immunopotentiating effect by inducing interleukin (IL)-12 production by dendritic cells and IL-12 receptor expression on NKT cells. J Exp Med (1999) 189:1121–8. doi: 10.1084/jem.189.7.1121
61. Fujii S-I, Shimizu K, Smith C, Bonifaz L, M Steinman R. Steinman. activation of natural killer T cells by α-galactosylceramide rapidly induces the full maturation of dendritic cells in vivo and thereby acts as an adjuvant for combined CD4 and CD8 T cell immunity to a coadministered protein. J.Exp.Med (2003) 198:267–9. doi: 10.1084/jem.20030324
62. Taraban VY, Martin S, Attfield KE, Glennie MJ, Elliott T, Elewaut D, et al. Invariant NKT cells promote CD8+cytotoxic T cell responses by inducing CD70 expression on dendritic cells. J Immunol (2008) 180:4615–20. doi: 10.4049/jimmunol.180.7.4615
63. Peperzak V, Xiao Y, Veraar EAM, Borst J. CD27 sustains survival of CTLs in virus-infected nonlymphoid tissue in mice by inducing autocrine IL-2 production. J Clin Invest (2010) 120:168–78. doi: 10.1172/JCI40178
64. Feau S, Garcia Z, Arens R, Yagita H, Borst J, Schoenberger SP. The CD4 + T-cell help signal is transmitted from APC to CD8 + T-cells via CD27–CD70 interactions. Nat Commun (2012) 3:948. doi: 10.1038/ncomms1948
65. Taraban VY, Rowley TF, Al-Shamkhani A. Cutting edge: a critical role for CD70 in CD8 T cell priming by CD40-licensed APCs. J Immunol (2004) 173:6542–6. doi: 10.4049/jimmunol.173.11.6542
66. Ochsenbein AF, Riddell SR, Brown M, Corey L, Baerlocher GM, Lansdorp PM, et al. CD27 expression promotes long-term survival of functional effector–memory CD8 + cytotoxic T lymphocytes in HIV-infected patients. J Exp Med (2004) 200:1407–17. doi: 10.1084/jem.20040717
67. Ahrends T, Spanjaard A, Pilzecker B, Bąbała N, Bovens A, Xiao Y, et al. CD4+ T cell help confers a cytotoxic T cell effector program including coinhibitory receptor downregulation and increased tissue invasiveness. Immunity (2017) 47:858–61. doi: 10.1016/j.immuni.2017.10.009
68. Bachem A, Hartung E, Güttler S, Mora A, Zhou X, Hegemann A, et al. Expression of XCR1 characterizes the batf3-dependent lineage of dendritic cells capable of antigen cross-presentation. Front Immunol (2012) 3:214. doi: 10.3389/fimmu.2012.00214
69. Dorner BG, Dorner MB, Zhou X, Opitz C, Mora A, Güttler S, et al. Selective expression of the chemokine receptor XCR1 on cross-presenting dendritic cells determines cooperation with CD8+ T cells. Immunity (2009) 31:823–33. doi: 10.1016/j.immuni.2009.08.027
70. Fitzgerald-Bocarsly P, Dai J, Singh S. Plasmacytoid dendritic cells and type I IFN: 50 years of convergent history. Cytokine Growth Factor Rev (2008) 19:3–19. doi: 10.1016/j.cytogfr.2007.10.006
71. Shimizu K, Asakura M, Shinga J, Sato Y, Kitahara S, Hoshino K, et al. Invariant NKT cells induce plasmacytoid dendritic cell(DC) cross-talk with conventional DCs for efficient memory CD8+T cell induction. J Immunol (2013) 190:5609–19. doi: 10.4049/jimmunol.1300033
72. Shirley JL, Keeler GD, Sherman A, Zolotukhin I, Markusic DM, Hoffman BE, et al. Type I IFN sensing by cDCs and CD4+ T cell help are both requisite for cross-priming of AAV capsid-specific CD8+ T cells. Mol Ther (2020) 28:758–70. doi: 10.1016/j.ymthe.2019.11.011
73. Fu C, Peng P, Loschko J, Feng L, Pham P, Cui W, et al. Plasmacytoid dendritic cells cross-prime naive CD8 T cells by transferring antigen to conventional dendritic cells through exosomes. Proc Natl Acad Sci U.S.A. (2020) 117:23730–41. doi: 10.1073/pnas.2002345117
74. Brewitz A, Eickhoff S, Dähling S, Quast T, Bedoui S, Kroczek RA, et al. CD8+ T cells orchestrate pDC-XCR1+ dendritic cell spatial and functional cooperativity to optimize priming. Immunity (2017) 208:205–19. doi: 10.1016/j.immuni.2017.01.003
75. Semmling V, Lukacs-Kornek V, Thaiss CA, Quast T, Hochheiser K, Panzer U. Alternative cross-priming through CCL17-CCR4-mediated attraction of CTLs toward NKT cell–licensed DCs. Nat Immunol (2010) 11:313–21. doi: 10.1038/ni.1848
76. Shimizu K, Yamasaki S, Shinga J, Sato Y, Watanabe T, Ohara O, et al. Systemic DC activation modulates the tumor microenvironment and shapes the longlived tumor-specific memory mediated by CD8+ T cells. Cancer Res (2016) 76:3756–66. doi: 10.1158/0008-5472.CAN-15-3219
77. Valente M, Dölen Y, van Dinther E, Vimeux L, Fallet M, Feuillet V, et al. Cross-talk between iNKT cells and CD8 T cells in the spleen requires the IL-4/CCL17 axis for the generation of short-lived effector cells. Proc Natl Acad Sci U.S.A. (2019) 116:25816–27. doi: 10.1073/pnas.1913491116
78. Zimmerer JM, Ringwald BA, Chaudhari SR, Han J, Peterson CM, Warren RT, et al. Invariant NKT cells promote the development of highly cytotoxic multipotent CXCR3 + CCR4 + CD8 + T cells that mediate rapid hepatocyte allograft rejection. J Immunol (2021) 207:3107–21. doi: 10.4049/jimmunol.2100334
79. Pereira CS, Macedo MF. CD1-restricted T cells at the crossroad of innate and adaptive immunity. J Immunol Res (2016) 2016:2876275. doi: 10.1155/2016/2876275
80. Yingyu Qin SO, Lim S, Shin JH, Yoon MS, Park S-H. Invariant NKT cells facilitate cytotoxic T-cell activation via direct recognition of CD1d on T cells. Exp Mol Med (2019) 51:1–9. doi: 10.1038/s12276-019-0329-9
81. Simonetta F, Lohmeyer JK, Hirai T, Maas-Bauer K, Alvarez M, Wenokur AS, et al. Allogeneic CAR invariant natural killer T cells exert potent antitumor effects through host CD8 T-cell cross-priming. Clin Cancer Res (2021) 27:6054–64. doi: 10.1158/1078-0432.CCR-21-1329
82. Zhao L, Yang X. Cross talk between natural killer T and dendritic cells and its impact on T cell responses in infections. Front Immunol (2022) 13:837767. doi: 10.3389/fimmu.2022.837767
83. Driver JP, de Carvalho Madrid DM, Gu W, Artiaga BL, Richt JA. Modulation of immune responses to influenza a virus vaccines by natural killer T cells. Front Immunol (2020) 11:2172. doi: 10.3389/fimmu.2020.02172
84. Guillonneau C, Mintern JD, Hubert F-X, Hurt AC, Besra GS, Porcelli S, et al. Combined NKT cell activation and influenza virus vaccination boosts memory CTL generation and protective immunity. Proc Natl Acad Sci U.S.A. (2009) 106:3330–5. doi: 10.1073/pnas.0813309106
85. Anderson RJ, Li J, Kedzierski L, Compton BJ, Hayman CM, Osmond TL, et al. Augmenting influenza-specific T cell memory generation with a natural killer T cell dependent glycolipid-peptide vaccine. ACS Chem Biol (2017) 12:2898–905. doi: 10.1021/acschembio.7b00845
86. Reilly EC, Thompson EA, Aspeslagh S, Wands JR, Elewaut D, Brossay L. Activated iNKT cells promote memory CD8+T cell differentiation during viral infection. PloS One (2012) 7:e37991. doi: 10.1371/journal.pone.0037991
87. Shimizu K, Kurosawa Y, Taniguchi M, Steinman RM, Fujii S-I. Cross-presentation of glycolipid from tumor cells loaded with α-galactosylceramide leads to potent and long-lived T cell–mediated immunity via dendritic cells. J Exp Med (2007) 204:2641–53. doi: 10.1084/jem.20070458
88. Compton BJ, Farrand KJ, Tang C-W, Osmond TL, Speir M, Authier-Hall A, et al. Enhancing T cell responses and tumour immunity by vaccination with peptides conjugated to a weak NKT cell agonist. Org Biomol Chem (2019) 17:1225–37. doi: 10.1039/c8ob02982b
89. Grasso C, Field CS, Tang C-W, Ferguson PM, Compton BJ, Anderson RJ, et al. Vaccines adjuvanted with an NKT cell agonist induce effective T-cell responses in models of CNS lymphoma. Immunotherapy (2020) 12:395–406. doi: 10.2217/imt-2019-0134
90. Burn OK, Farrand K, Pritchard T, Draper S, Tang C-W, Mooney AH, et al. Glycolipid-peptide conjugate vaccines elicit CD8 + T-cell responses and prevent breast cancer metastasis. Clin Transl Immunol (2022) 11:e1401. doi: 10.1002/cti2.1401
91. Bae E-A, Seo H, Kim B-S, Choi J, Jeon I, Shin K-S, et al. Activation of NKT cells in an anti-PD-1-resistant tumor model enhances antitumor immunity by reinvigorating exhausted CD8 T cells. Cancer Res (2018) 78:5315–26. doi: 10.1158/0008-5472.CAN-18-0734
92. Mueller SN, Mackay LK. Tissue-resident memory T cells: local specialists in immune defence. Nat Rev Immunol (2016) 6:79–89. doi: 10.1038/nri.2015.3
93. Holz LE, Chua YC, de Menezes MN, Anderson RJ, Draper SL, Compton BJ, et al. Glycolipid-peptide vaccination induces liver-resident memory CD8+ T cells that protect against rodent malaria. Sci Immunol (2020) 5:eaaz8035. doi: 10.1126/sciimmunol.aaz8035
94. Lin JS, Mohrs K, Szaba FM, Kummer LW, Leadbetter EA, Mohrs M. Virtual memory CD8 T cells expanded by helminth infection confer broad protection against bacterial infection. Mucosal Immunol (2019) 12:258–64. doi: 10.1038/s41385-018-0100-x
95. Ueda N, Kuki H, Kamimura D, Sawa S, Seino K, Tashiro T, et al. CD1d-restricted NKT cell activation enhanced homeostatic proliferation of CD8+ T cells in a manner dependent on IL-4. Int Immunol (2006) 18:1397–404. doi: 10.1093/intimm/dxl073
96. Lai D, Zhu J, Wang T, Hu-Li J, Terabe M, Berzofsky JA, et al. KLF13 sustains thymic memory-like CD8(+) T cells in BALB/c mice by regulating IL-4-generating invariant natural killer T cells. J Exp Med (2011) 208:1093–103. doi: 10.1084/jem.20101527
97. Kurachi M, Kurachi J, Suenaga F, Tsukui T, Abe J, Ueha S, et al. Chemokine receptor CXCR3 facilitates CD8+ T cell differentiation into short-lived effector cells leading to memory degeneration. J Exp Med (2011) 208:1605–20. doi: 10.1084/jem.20102101
98. Hu JK, Kagari T, Clingan JM, Matloubian M. Expression of chemokine receptor CXCR3 on T cells affects the balance between effector and memory CD8 T-cell generation. Proc Natl Acad Sci U.S.A. (2011) 108:118–27. doi: 10.1073/pnas.1101881108
99. Baekkevold ES, Wurbel M-A, Kivisäkk P, Wain CM, Power CA, Haraldsen G, et al. A role for CCR4 in development of mature circulating cutaneous T helper memory cell populations. J Exp Med (2005) 201:1045–51. doi: 10.1084/jem.20041059
100. Casciano F, Diani M, Altomare A, Granucci F, Secchiero P, Banfi G, et al. CCR4+ skin-tropic phenotype as a feature of central memory CD8+ T cells in healthy subjects and psoriasis patients. Front Immunol (2020) 11:529. doi: 10.3389/fimmu.2020.00529
101. Kondo T, Takiguchi M. Human memory CCR4+CD8+T cell subset has the ability to produce multiple cytokines. Int Immunol (2009) 21:523–32. doi: 10.1093/intimm/dxp019
102. Carrasco E, Heras MMG, Gabandé-Rodríguez E, Desdín-Micó G, Aranda JF, Mittelbrunn M. The role of T cells in age-related diseases. Nat Rev Immunol (2022) 22:97–111. doi: 10.1038/s41577-021-00557-4
103. Mittelbrunn M, Kroemer G. Hallmarks of T cell aging. Nat Immunol (2021) 22:687–98. doi: 10.1038/s41590-021-00927-z
104. Rodriguez IJ, Ruiz NL, León ML, Martínez Enríquez L, Del Pilar M, Velásquez M, et al. Immunosenescence study of T cells: A systematic review. Front Immunol (2021) 11:604591. doi: 10.3389/fimmu.2020.604591
105. Faunce DE, Palmer JL, Paskowicz KK, Witte PL, Kovacs EJ. CD1d-restricted NKT cells contribute to the age-associated decline of T cell immunity. J Immunol (2005) 175:3102–9. doi: 10.4049/jimmunol.175.5.3102
106. Inui T, Nakagawa R, Ohkura S, Habu Y, Koike Y, Motoki K, et al. Age-associated augmentation of the synthetic ligand- mediated function of mouse NK1.1 ag(+) T cells: their cytokine production and hepatotoxicity in vivo and in vitro. J Immunol (2002) 169:6127–32. doi: 10.4049/jimmunol.169.11.6127
107. Mocchegiani E, Malavolta M. NK and NKT cell functions in immunosenescence. Aging Cell (2004) 3:177–84. doi: 10.1111/j.1474-9728.2004.00107.x
108. Tsukahara A, Seki S, Iiai T, Moroda T, Watanabe H, Suzuki S, et al. Mouse liver T cells: their change with aging and in comparison with peripheral T cells. Hepatology (1997) 26:301–9. doi: 10.1002/hep.510260208
109. DelaRosa O, Tarazona R, Casado JG, Alonso C, Ostos B, Peña J, et al. Valpha24+NKT cells are decreased in elderly humans. Exp Gerontol (2002) 37:213–7. doi: 10.1016/s0531-5565(01)00186-3
110. Papadogianni G, Ravens I, Dittrich-Breiholz O, Bernhardt G, Georgiev H. Impact of aging on the phenotype of invariant natural killer T cells in mouse thymus. Front Immunol (2020) 20:575764. doi: 10.3389/fimmu.2020.575764
111. Poynter ME, Mu HH, Chen XP, Daynes RA. Activation of NK1.1+ T cells in vitro and their possible role in age-associated changes in inducible IL-4 production. Cell Immunol (1997) 179:22–9. doi: 10.1006/cimm.1997.1141
112. Plackett TP, Schilling EM, Faunce DE, Choudhry MA, Witte PL, Kovacs EJ. Aging enhances lymphocyte cytokine defects after injury. FASEB J (2003) 17:688–9. doi: 10.1096/fj.02-0452fje
113. Lee YJ, Jameson SC, Hogquist KA. Alternative memory in the CD8 lineage. Trends Immunol (2011) 32:50–6. doi: 10.1016/j.it.2010.12.004
114. Lee YJ, Holzapfel KL, Zhu J, Jameson SC, Hogquist KA. Steady-state production of IL-4 modulates immunity in mouse strains and is determined by lineage diversity of iNKT cells. Nat Immunol (2013) 14:1146–54. doi: 10.1038/ni.2731
115. Chiu B-C, Martin BE, Stolberg VR, Chensue SW. Cutting edge: Central memory CD8 T cells in aged mice are virtual memory cells. J Immunol (2013) 191:5793–6. doi: 10.4049/jimmunol.1302509
116. Quinn KM, Fox A, Harland KL, Russ BE, Li J, Nguyen THO, et al. Ge-related decline in primary CD8+ T cell responses is associated with the development of senescence in virtual memory CD8+ T cells. Cell Rep (2018) 23:3512–24. doi: 10.1016/j.celrep.2018.05.057
117. Lee SW, Park HJ, Cheon JH, Wu L, Kaer LV, Hong S. iNKT cells suppress pathogenic NK1.1+CD8+ T cells in DSS-induced colitis. Front Immunol (2018) 9:2168. doi: 10.3389/fimmu.2018.02168
118. Gapin L. iNKT cell autoreactivity: what is ‘self’ and how is it recognized? Nat Rev Immunol (2010) 10:272–7. doi: 10.1038/nri2743
119. Mattner J, Debord KL, Ismail N, Goff RD, Cantu C3, Zhou D, et al. Exogenous and endogenous glycolipid antigens activate NKT cells during microbial infections. Nature (2005) 434:525–9. doi: 10.1038/nature03408
120. Brennan PJ, Tatituri RVV, Brigl M, Kim EY, Tuli A, Sanderson JP, et al. Invariant natural killer T cells recognize lipid self antigen induced by microbial danger signals. Nat Immunol (2011) 12:1202–11. doi: 10.1038/ni.2143
121. Darmoise A, Teneberg S, Bouzonville L, Brady RO, Beck M, Kaufmann SHE, et al. Lysosomal alpha-galactosidase controls the generation of self lipid antigens for natural killer T cells. Immunity (2010) 33:216–28. doi: 10.1016/j.immuni.2010.08.003
122. Fox LM, Cox DG, Lockridge JL, Wang X, Chen X, Scharf L, et al. Recognition of lyso-phospholipids by human natural killer T lymphocytes. PloS Biol (2009) 7:e1000228. doi: 10.1371/journal.pbio.1000228
123. Facciotti F, Ramanjaneyulu GS, Lepore M, Sansano S, Cavallari M, Kistowska M, et al. Peroxisome-derived lipids are self antigens that stimulate invariant natural killer T cells in the thymus. Nat Immunol (2012) 13:474–80. doi: 10.1038/ni.2245
124. Govindarajan S, Verheugen E, Venken K, Gaublomme D, Maelegheer M, Cloots E. ER stress in antigen-presenting cells promotes NKT cell activation through endogenous neutral lipids. EMBO Rep (2020) 21:e48927. doi: 10.15252/embr.201948927
Keywords: invariant NKT (iNKT) cell, CD8+ T cell, cross-priming, memory, aging
Citation: Qin Y, Bao X and Zheng M (2023) CD8+ T-cell immunity orchestrated by iNKT cells. Front. Immunol. 13:1109347. doi: 10.3389/fimmu.2022.1109347
Received: 27 November 2022; Accepted: 30 December 2022;
Published: 18 January 2023.
Edited by:
Lifei Hou, Boston Children’s Hospital and Harvard Medical School, United StatesReviewed by:
Chengxian Xu, Boston Children’s Hospital and Harvard Medical School, United StatesCopyright © 2023 Qin, Bao and Zheng. This is an open-access article distributed under the terms of the Creative Commons Attribution License (CC BY). The use, distribution or reproduction in other forums is permitted, provided the original author(s) and the copyright owner(s) are credited and that the original publication in this journal is cited, in accordance with accepted academic practice. No use, distribution or reproduction is permitted which does not comply with these terms.
*Correspondence: Mingzhu Zheng, emhlbmdtekBzZXUuZWR1LmNu
Disclaimer: All claims expressed in this article are solely those of the authors and do not necessarily represent those of their affiliated organizations, or those of the publisher, the editors and the reviewers. Any product that may be evaluated in this article or claim that may be made by its manufacturer is not guaranteed or endorsed by the publisher.
Research integrity at Frontiers
Learn more about the work of our research integrity team to safeguard the quality of each article we publish.