- 1Laboratory of Cellular and Molecular Immunology, Universidade Federal de Ciências da Saúde de Porto Alegre (UFCSPA), Porto Alegre, RS, Brazil
- 2Graduate Program in Health Sciences, Universidade Federal de Ciências da Saúde de Porto Alegre (UFCSPA), Porto Alegre, RS, Brazil
- 3Laboratory of Technological Processes and Catalysis, Institute of Chemistry, Universidade Federal do Rio Grande do Sul (UFRGS), Porto Alegre, RS, Brazil
- 4Graduate Program in Biosciences, Universidade Federal de Ciências da Saúde de Porto Alegre (UFCSPA), Porto Alegre, RS, Brazil
- 5Graduate Program in Pharmaceutical Science, Graduate Program in Neuroscience and Cellular Biology, Faculty of Pharmacy, Universidade Federal do Pará, Belém, PA, Brazil
In this study we explored the previously established leishmanicidal activity of a complementary set of 24 imidazolium salts (IS), 1-hexadecylimidazole (C16Im) and 1-hexadecylpyridinium chloride (C16PyrCl) against Leishmania (Leishmania) amazonensis and Leishmania (Leishmania) infantum chagasi. Promastigotes of L. amazonensis and L. infantum chagasi were incubated with 0.1 to 100 μM of the compounds and eight of them demonstrated leishmanicidal activity after 48 h – C10MImMeS (IC50 L. amazonensis = 11.6), C16MImPF6(IC50 L. amazonensis = 6.9), C16MImBr (IC50 L. amazonensis = 6), C16M2ImCl (IC50 L. amazonensis = 4.1), C16M4ImCl (IC50 L. amazonensis = 1.8), (C10)2MImCl (IC50 L. amazonensis = 1.9), C16Im (IC50 L. amazonensis = 14.6), and C16PyrCl (IC50 L. amazonensis = 4).The effect of IS on reactive oxygen species production, mitochondrial membrane potential, membrane integrity and morphological alterations of promastigotes was determined, as well as on L. amazonensis-infected macrophages. Their cytotoxicity against macrophages and human erythrocytes was also evaluated. The IS C10MImMeS, C16MImPF6, C16MImBr, C16M2ImCl, C16M4ImCl and (C10)2MImCl, and the compounds C16Im and C16PyrCl killed and inhibited the growth of promastigote forms of L. amazonensis and L. infantum chagasi in a concentration-dependent manner, contributing to a better understanding of the structure-activity relationship of IS against Leishmania. These IS induced ROS production, mitochondrial dysfunction, membrane disruption and morphological alterations in infective forms of L. amazonensis and killed intracellular amastigote forms in very low concentrations (IC50 amastigotes ≤ 0.3), being potential drug candidates against L. amazonensis.
1 Introduction
Leishmaniasis are endemic in 102 countries or territories with around 1.3 million new cases and 20.000 to 30.000 deaths yearly (1). Brazil is one of the countries with the highest incidence of leishmaniasis (2). Leishmania (Leishmania) amazonensis is an important etiological agent of cutaneous, anergic diffuse cutaneous and less commonly mucocutaneous leishmaniasis, especially in South America (3, 4), and Leishmania (Leishmania) infantum chagasi causes visceral leishmaniasis, mainly in the Mediterranean Basin, Middle East, Central Asia and South America (4).
Currently, there is no vaccine for leishmaniasis, and their treatment is based on very toxic and poorly tolerated drugs, such as pentavalent antimonials and amphotericin B (5). Azole antifungals presented leishmanicidal activity against some Leishmania species (6). Moreover, various biological activities have been described for the cationic equivalent of imidazoles, imidazolium salts (IS), including antimicrobial (7), antifungal (8, 9), larvicidal (10), antitumoral, antioxidant and others (11). The IS present high structural variability and tunable interactions with biomolecules like phospholipids and proteins, especially because their amphiphilic nature (11), becoming potential candidates in medicinal chemistry (12). Recently, our group described for the first time the leishmanicidal activity of IS (13). Within the tested IS, 1-methyl-3-octadecylimidazolium chloride (C18MImCl), 1-hexadecyl-3-methylimidazolium methanesulfonate (C16MImMeS), 1-hexadecyl-3-methylimidazolium chloride (C16MImCl), 1-decyl-3-methylimidazolium chloride (C10MImCl) and 1-hexadecyl-3-methylimidazolium bis(trifluoromethylsulfonyl)imide (C16MImNTf2) were identified as potent agents against both promastigote and amastigote forms of L. amazonensis. Here, we further explored this leishmanicidal action with a structurally complementary set of 24 IS, 1-hexadecylimidazole (C16Im) and 1-hexadecylpyridinium chloride (C16PyrCl) (Figure 1) to (i) investigate the structure-activity relationship of these molecules on promastigote and amastigote forms of L. amazonensis and promastigote forms of L. infantum chagasi, (ii) identify mortality pathways, and (iii) evaluate cytotoxicity.
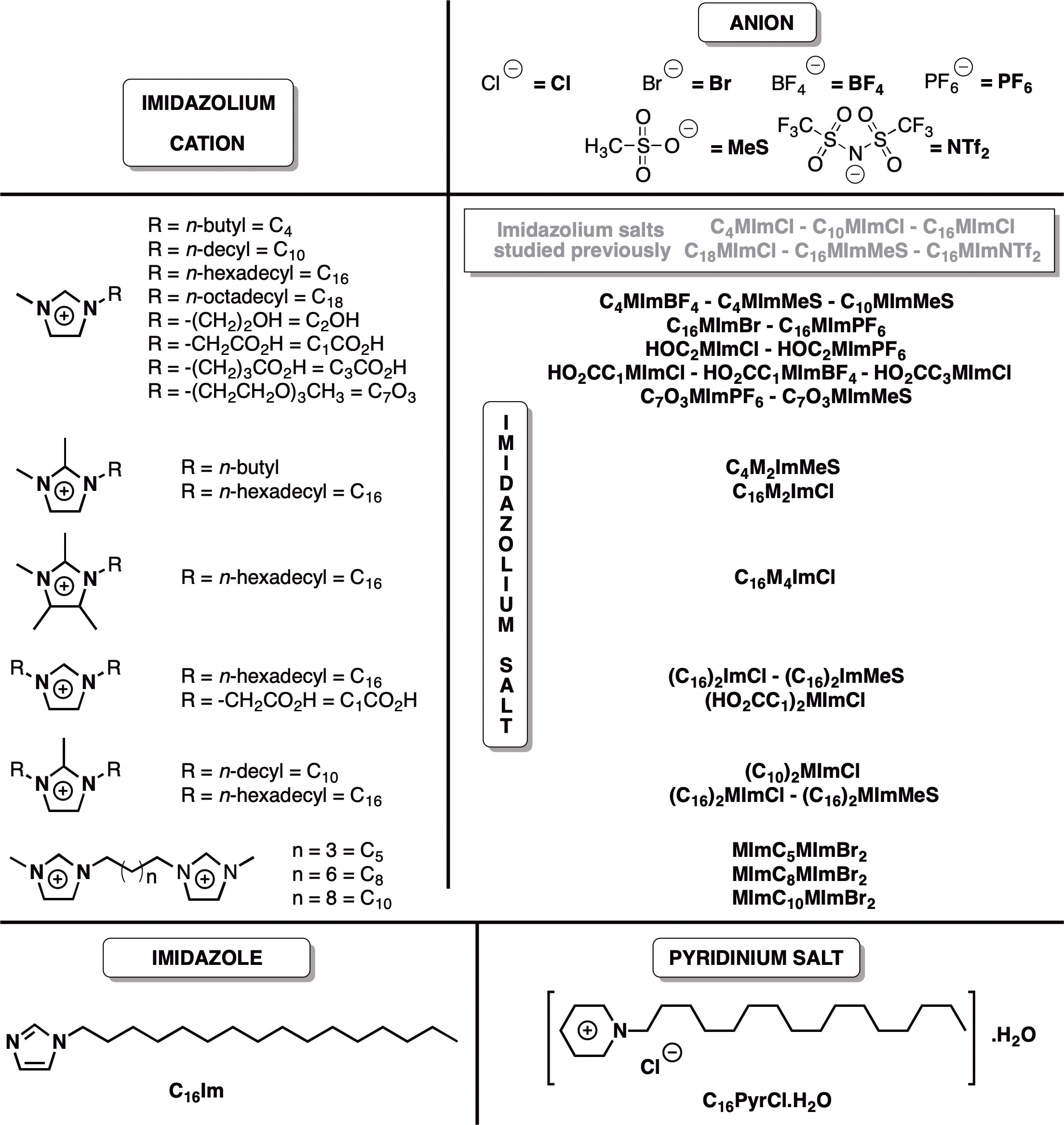
Figure 1 Chemical structures of the imidazolium salts, C16Im and C16PyrCl screened for anti-Leishmania activity tested in this work, and imidazolium salts studied previously for anti-Leishmania activity (ref 13). The compounds were given sample codes that contain information on their chemical structures. For instance, for C18MImCl: (1) C18 = N-octadecyl substituent; (2) M = N-methyl substituent; (3) Im = imidazolium cation; and (4) Cl = chloride anion.
2 Materials and methods
2.1 Imidazolium salts and reference compounds
1,3-Didecyl-2-methylimidazolium chloride ((C10)2MImCl) (CJC China JIE Chemical), 1-hexadecylpyridinium chloride monohydrate (C16PyrCl.H2O) (Sigma-Aldrich), and 1-(2-hydroxyethyl)-3-methylimidazolium chloride (HOC2MImCl) (IOLITEC) were purchased and used as received.
1-Butyl-3-methylimidazolium methanesulfonate (C4MImMeS) (14), 1-decyl-3-methylimidazolium methanesulfonate (C10MImMeS) (15), 1-hexadecyl-3-methylimidazolium bromide (C16MImBr) (16), 1-butyl-3-methylimidazolium tetrafluoroborate (C4MImBF4) (14), 1,10-bis(methylimidazolium-1-yl) decane bromide (MImC10MImBr2) (16), 1-triethylene glycol monomethyl ether-3-methylimidazolium hexafluorophosphate (C7O3MImPF6) (15), 1-triethylene glycol monomethyl ether-3-methylimidazolium methanesulfonate (C7O3MImMeS) (15), 1-butyl-2,3-dimethylimidazolium methanesulfonate (C4M2ImMeS) (17), 1-hexadecylimidazole (C16Im) (18), 1,3-dihexadecylimidazolium chloride ((C16)2ImCl) (19), 1-(3-hydroxypropyl)-3-methylimidazolium hexafluorophosphate (HOC3MImPF6) (20), 1-carboxymethyl-3-methylimidazolium chloride (HO2CC1MImCl) (21), 1,3-bis(carboxymethyl)imidazolium chloride ((HO2CC1)2ImCl) (21), 1-carboxymethyl-3-methylimidazolium tetrafluoroborate (HO2CC1MImBF4) (22), 1-carboxypropyl-3-methylimidazolium chloride (HO2CC3MImCl) (23), 1-hexadecyl-3-methylimidazolium hexafluorophosphate (C16MImPF6) (24), 1-hexadecyl-2,3,4,5-tetramethylimidazolium chloride (C16M4ImCl) (25), decyl methanesulfonate (C10MeS) (15), hexadecyl methanesulfonate (C16MeS) (26), butyl methanesulfonate (C4MeS) (14), 1-hexadecyl-2-methylimidazole (C16MIm) (27), and triethylene glycol monomethyl ether methanesulfonate (C7O3MeS) (15) were synthesized according to previously reported procedures. C4MeS, C10MeS, C16MeS, C16Im, C16MIm, and C7O3MeS were used as source for the syntheses of C4MImMeS, C4M2ImMeS, C10MImMeS, (C16)2ImCl, C7O3MImMeS, 1,3-dihexadecylimidazolium methanesulfonate (C16)2ImMeS, 1,3-dihexadecyl-2-methylimidazolium chloride ((C16)2MImCl), and 1,3-dihexadecyl-2-methylimidazolium methanesulfonate ((C16)2MImMeS). Literature procedures were used as basis for the synthesis of 1-hexadecyl-2,3-dimethylimidazolium chloride (C16M2ImCl) (15), 1,5-bis(methylimidazolium-1-yl) pentane bromide (MImC5MImBr2) (16), 1,8-bis(methylimidazolium-1-yl) octane bromide (MImC8MImBr2) (16), (C16)2ImMeS (15), (C16)2MImCl (15) and (C16)2MImMeS (15), which are yet unpublished IS. See the Supporting Information for further details. Before use, the IS were dried under vacuum at 60°C for 5 h to remove residual water.
2.2 Parasite culture
Leishmania (L.) amazonensis (MHOM/BR/73/M2269 strain) and Leishmania (L.) infantum chagasi (MHOM/BR/74/PP75 strain) were cultured at 26 °C as previously described elsewhere (28). To avoid the loss of in vitro infectivity of promastigotes, samples of parasites were kept cryopreserved in liquid nitrogen soon after isolation of infected animals (BALB/c or hamsters, respectively). All experiments were performed using parasites with few passages of in vitro growth.
2.3 Anti-promastigote activity
The effect of IS, C16Im and C16PyrCl on L. amazonensis and L. infantum chagasi was evaluated as previously described (28). Promastigotes of L. amazonensis in the stationary phase were distributed in 96-well microplates (3 x 106 cells/well) and incubated with M199 medium or 100 μM (screening concentration) of tested compound (IS, C16Im or C16PyrCl) for 44 h at 26 °C. Subsequently, 0.5 mg/mL of 3-(4,5-dimethylthiazol-2-yl)-2,5-diphenyltetrazolium bromide (MTT, Sigma-Aldrich®, St. Louis, MO, USA) was added and the incubations continued for an additional 4 h at 26 °C. The purple formazan product that was formed by the action of mitochondrial enzymes in living cells was solubilized by the addition of acidic isopropanol, and the absorbance at 570 nm was measured using a microplate reader spectrophotometer EZ Read 400 from Biochrom. The compounds that caused 100% of L. amazonensis mortality in the screening test (C10MImMeS, C16MImPF6, C16MImBr, C16M2ImCl, C16M4ImCl, (C10)2MImCl, C16Im and C16PyrCl) were selected for additional tests against L. amazonensis and L. infantum chagasi at concentrations of 0.1 to 100 μM. The IS C10MImCl, C16MImCl and C16MImMeS, tested against L. amazonensis in our previous study (13), were tested here against promastigotes of L. infantum chagasi. The 50% inhibitory concentration (IC50) value for each compound was determined. The survival rate was calculated according to the formula: OD in treated group/OD of untreated group x 100. The 50% inhibitory concentration (IC50) value for each compound was determined by nonlinear regression analysis using GraphPad Prism 5.03 software. The activity of each compound was compared with control samples incubated with culture medium (M199 medium for L. amazonensis and Schneider’s medium for L. infantum chagasi) containing less than 0.05% of polysorbate 80 (Sigma-Aldrich) or amphotericin B at 5 µM (100% mortality: Sigma-Aldrich) as reference antileishmanial drug.
2.4 Evaluation of leishmania growth
Promastigote forms of L. amazonensis or L. infantum chagasi in the stationary growth phase were distributed in 12-well microplates at a density of 1 x 105 mL-1 in medium (control) (M199 medium for L. amazonensis and Schneider’s medium for L. infantum chagasi) or medium plus C10MImMeS, C16MImPF6, C16MImBr, C16M2ImCl, C16M4ImCl, (C10)2MImCl, C16Im or C16PyrCl at the IC50 found after 48 h of incubation, and the parasite growth at 26 °C was evaluated daily by motility and cell density using a hemocytometer (28).
2.5 Field emission scanning electron microscopy
To evaluate parasite ultrastructural alterations by scanning electron microscopy (SEM), promastigotes of L. amazonensis in the stationary phase were distributed in 96-well microplates (3 x 106 cells/well) and incubated at 26 °C with M199, M199 containing IS at the IC50 or amphotericin B at 0.4 µM (IC50) for 6 h. The parasites were washed with phosphate buffered saline (PBS) and fixed with 25% glutaraldehyde in 0.2 M phosphate buffer pH 7.4. After being fixed three times with the same buffer, the parasites were adhered to glass slides previously coated with 0.1% aqueous poly-L-lysine for 30 min at room temperature. Subsequently, the parasites were washed three times with 0.2 M phosphate buffer pH 7.4 and post-fixed in a solution of 2% OsO4 with 0.2 M phosphate buffer (1:1) for 45 min at room temperature. All samples were dehydrated in a graded series of acetone in water from 30% to 100%, critical point dried using CO2, mounted on metal stubs, and coated with gold for observation in a field emission scanning electron microscope (Inspect F50, FEI) (29). In addition, the IS C16MImMes and C16MImCl, tested in our previous study (13), were also analyzed.
2.6 Evaluation of cell membrane integrity
L. amazonensis promastigotes (2 x 105) in the logarithmic growth phase were distributed in 96-well microplates and either untreated, treated with IS (2-fold IC50 for 48 h), hydrogen peroxide (H2O2) 2 mM or amphotericin B (0.4 μM) as positive control. After 48 h at 26°C, the parasites were harvested and washed twice with PBS, resuspended and incubated with 100 μL of propidium iodide (PI – Sigma–Aldrich) 50 μg/mL at 26°C for 15 min. Following, each sample was completed with 400 μL of PBS and a total of 20,000 events were acquired and analyzed on BD FACScalibur flow cytometer and CellQuest Pro software. PI fluorescence intensity was quantified as the percentage of the fluorescence compared with untreated promastigotes (30). Results were obtained from two independent experiments performed in triplicate.
2.7 Determination of the mitochondrial membrane potential (ΔΨm)
Mitochondrial membrane potential (ΔΨm) was quantified using the fluorescent dye rhodamine 123 (Rh 123, Sigma-Aldrich) (29). Briefly, promastigote forms of L. amazonensis (2 x 105) in the logarithmic growth phase were incubated with M199 medium (6 h), medium plus IS (2-fold IC50 – 6 h), amphotericin B (0.4 μM - 6 h) or H2O2 (2 mM - 1 h) in 96-well microplates, at 26°C. These tested concentrations and time were chosen based on previous results with other IS (13). Then, cells were washed in PBS and incubated with 500 μL of Rh 123 (1 µg/mL) for 10 min at 37 °C. After being washed, they were resuspended in 0.5 mL of PBS and the analysis was performed using a BD FACSCalibur (Becton–Dickinson®, Rutherford, NJ, USA) flow cytometer and CellQuest® Pro software (Joseph Trotter, Scripps Research Institute, La Jolla, CA, USA), using the blue argon-ion 488 nm laser with the FL1 filter channel. A total of 30,000 events were acquired in the region that corresponded to the parasites. Histograms were build using the CellQuest Pro software (Joseph Trotter, Scripps Research Institute, La Jolla, CA, USA).
2.8 Detection of reactive oxygen species production
ROS generation was quantified using the dye 2′,7′-dichlorofluorescein diacetate (DCF-DA, Sigma-Aldrich) and flow cytometer analysis (13, 30, 31). Briefly, promastigote forms of L. amazonensis (2 x 105/well) in the logarithmic growth phase were incubated with M199 medium (6 h), M199 plus IS (2-fold IC50 - 6 h), amphotericin B (0.4 μM - 6 h) or H2O2 (2 mM – 1 h) in 96-well microplates, at 26°C. Then, cells were washed with PBS and incubated with 0.5 mL of 10 µM DCF-DA for 30 min at room temperature. After being washed with PBS, cells were resuspended in 0.5 mL PBS and analysed using a BD FACSCalibur (Becton–Dickinson, Rutherford, NJ, USA) flow cytometer and CellQuest Pro software (Joseph Trotter, Scripps Research Institute, La Jolla, CA, USA) using the blue argon-ion 488 nm laser with the FL1 filter channel. A total of 30,000 events were acquired in the region that corresponded to living parasite.
2.9 Intramacrophage anti-amastigote activity
The anti-amastigote assay was performed as previously described (13). Macrophages RAW 264.7 cell line (ATCC) were cultured in DMEM plus 10% fetal bovine serum (FBS), penicillin (100 U/mL) and streptomycin (100 µg/mL) until reaching 90% confluence, when were distributed into 96-well microplates at a concentration of 2 x 105 cells/well in the presence of DMEM. After 12-16 h at 37°C in a humidified atmosphere of 5% CO2, non-adherent cells were removed, and adherent macrophages were infected with promastigotes of L. amazonensis (10 parasites/cell) in the stationary phase, and 4 h later were washed with PBS to remove parasites and incubated with DMEM (control), lipopolysaccharide (LPS) (10 ng/mL) plus interferon-γ (IFN-γ (1 ng/mL) as positive control, or IS, C16Im or C16PyrCl at concentrations of 0.1, 1 μM or 5 μM for 48 h. After, cells were incubated with 100 µL of 0.01% (w/v) SDS solution in serum-free M199 medium at 37°C for 20 min. Then, cells were supplemented with M199 30% FBS and cultured at 26°C for 7 days to determine the number of promastigotes recovered, once only viable amastigotes are capable to differentiate to motile promastigotes. The leishmanicidal activity of macrophages was assessed by determining the number of viable parasites (4 replicates) using a hemocytometer.
2.10 Macrophage viability and hemolytic assay
RAW cells were incubated or not with IS, C16Im or C16PyrCl (5 to 200 μM) for 48 h at 37 °C and in vitro cytotoxicity determined by MTT assay (30). Triton X-100 1% was used as positive control. The hemolytic assay was performed according to the procedure used by (13). Briefly, red blood cells solution 1% (v/v) obtained from healthy voluntary donors (n=3) (project approved by the research ethical committee from the Universidade Federal de Ciências da Saúde de Porto Alegre - CAAE 63282416.6.0000.5345) were distributed into 96-well microplate and incubated with different IS, C16Im or C16PyrCl (1 to 100 μM). The microplate was incubated at 37°C under agitation (90 rpm) for 60 min. Then, the suspension was centrifuged at 3,000 rpm for 5 min, and the absorbance of the supernatant was measured at 540 nm using a spectrophotometer EZ Read 400 from Biochrom. Triton X-100 1% and PBS were used as positive and negative control, respectively. The 50% cytotoxicity concentration (CC50) and 50% of red blood cell hemolysis (RBC50) were determined by nonlinear regression analysis. The selectivity index (SI) (CC50 or RBC50/IC50 against L. amazonensis) were determined for each compound.
2.11 Statistical analysis
The results are expressed as mean ± standard error of the mean (SEM) from five replicates in each three independent experiments. Kolmogorov-Smirnov test was applied to verify normality. Comparisons were performed by the One-way ANOVA test followed by Bonferroni’s post-test, Two-way ANOVA repeated measures test followed by Bonferroni’s post-test. Differences were considered statistically significant when p < 0.05. All statistical tests were performed using GraphPad Prism 6.01 software.
3 Results
3.1 Imidazolium salts
The results regarding the syntheses of IS are reported in the Supplementary materials, together with 1H NMR spectra of the synthesized compounds, and 13C NMR and FTIR spectra for the novel IS.
3.2 Leishmanicidal activity of the compounds on promastigotes of L. amazonensis and L. infantum chagasi
Six of the 24 IS tested (Figure 1) were able to kill promastigotes of L. amazonensis in a concentration-dependent manner (Figure S38A: Leishmanicidal activity of imidazolium salts, C16Im and C16PyrCl (0.1 to 100 μM) on promastigote forms of L. amazonensis, determined at 48 h incubation using MTT assay). C10MImMeS, C16MImPF6, C16MImBr, C16M2ImCl, C16M4ImCl and (C10)2MImCl at 5 µM caused a significant decrease in the viability after 48 h, resulting in 60, 37, 42, 85, 95 and 97% of mortality, respectively. The neutral imidazole C16Im and the pyridinium salt C16PyrCl at concentrations ≥ 10 µM and ≥ 5 µM caused significant parasite mortality, respectively (Figure S38A). The IC50 values of C10MImMeS, C16MImPF6, C16MImBr, C16M2ImCl, C16M4ImCl and (C10)2MImCl on promastigotes of L. amazonensis were calculated to be 11.6, 6.9, 6.0, 4.1, 1.8 and 1.9 µM, respectively (Table 1). Although C10MImMeS, C16MImPF6, C16MImBr, C16M2ImCl, C16M4ImCl and (C10)2MImCl also presented leishmanicidal activity against L. infantum chagasi (Figure S38B: Leishmanicidal activity of imidazolium salts, C16Im and C16PyrCl (0.1 to 100 μM) on promastigote forms of L. infantum chagasi, determined at 48 h incubation using MTT assay), the IC50 values were in general higher than against L. amazonensis (Table 1). In contrast, the IC50 values for C16Im and C16PyrCl were lower in the case of L. infantum chagasi. To reinforce the higher activity of IS towards L. amazonensis we tested the effect of IS with the best activity against L. amazonensis in our previous study (13) in promastigotes of L. infantum chagasi. The IS C10MImCl, C16MImCl and C16MImMeS presented lower IC50 values for L. amazonensis (2.31, 1.23 and 0.75 µM, respectively) (13) when compared to those values found for L. infantum chagasi (IC50 = 114.9 µM, 9.9 µM and 10.8 µM, respectively; data not shown). As expected, amphotericin B at 5 µM caused 100% mortality of L. amazonensis and L. infantum chagasi.
3.3 Imidazolium salts, C16Im and C16PyrCl inhibit the growth of promastigotes of L. amazonensis and L. infantum chagasi
When promastigotes of L. amazonensis or L. infantum chagasi were treated with IS, C16Im or C16PyrCl at IC50 concentrations, a complete inhibition of the parasites growth was observed (Figures 2A, B).
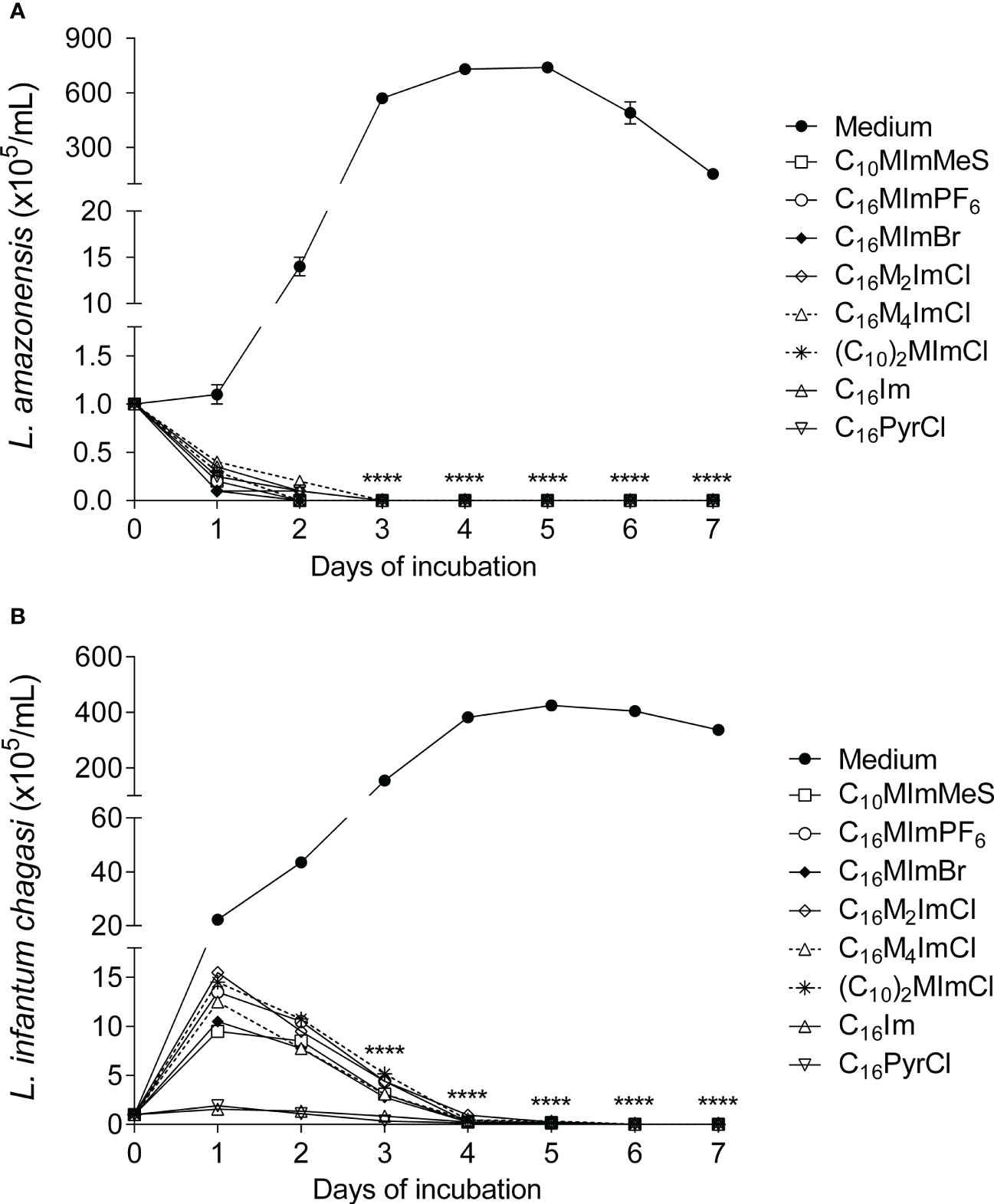
Figure 2 Growth kinetics of L. amazonensis and L. infantum chagasi promastigotes treated with the different compounds. L. amazonensis (A) or L. infantum chagasi (B) promastigotes in the stationary phase (1 × 105 cells/mL) were incubated with medium as control (M199 to L. amazonensis and Schneider’s medium to L. infantum chagasi) or medium plus compounds at IC50 values (for 48 h incubation), at 26°C, and the growth was determined daily using a hemocytometer. Data are expressed as means ± SEM (n = 5) and are representative of three independent experiments. The Two-way ANOVA repeated measures test followed by the Bonferroni’s test were applied. ****p < 0.0001.
3.4 Imidazolium salts increase reactive oxygen species and induce mitochondrial membrane potential (ΔΨm) alterations in L. amazonensis
The treatment of promastigotes of L. amazonensis with IS at 2-fold IC50 values led to a significant increase in ROS production at 6 h of incubation (Figures 3A, B). C16MImPF6, C16MImBr, C16M2ImCl, C16M4ImCl and (C10)2MImCl caused significant ΔΨm depolarization (loss of Rh 123 fluorescence), while C10MImMeS induced ΔΨm hyperpolarization (increase in Rh 123 fluorescence) (Figures 3C, D). Hydrogen peroxide at 2 mM or amphotericin B at 0.4 µM also induced significant elevation in ROS production and mitochondrial depolarization.
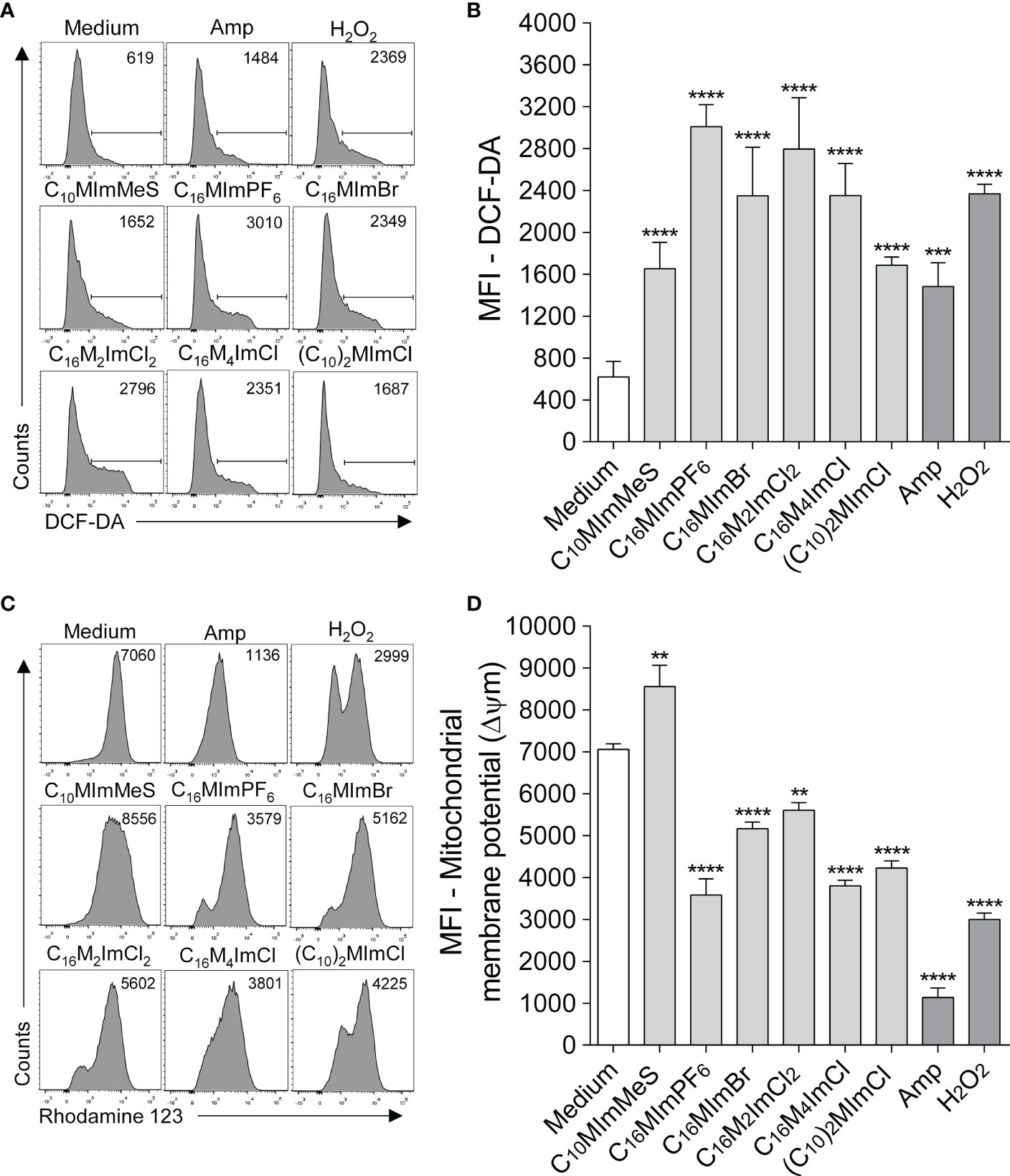
Figure 3 Determination of intracellular ROS (A, B) and mitochondrial membrane potential (ΔΨm) (C, D) of L. amazonensis promastigotes incubated with imidazolium salts. Promastigote forms in the logarithmic phase (2 x 105 cells/well) were incubated or not with 2-fold IC50 value of IS at 26°C for 6 h. M199 was used as negative control, and amphotericin B (0.4 µM) and H2O2 (2 mM) as positive controls. Data are expressed as means ± SEM and are representative of three independent experiments. The One-way ANOVA test followed by the Bonferroni’s test were applied. **p < 0.01, ***p < 0.001 and ****p < 0.0001 when compare with the medium group.
3.5 Imidazolium salts induce loss of plasma membrane integrity in L. amazonensis
When parasites were treated with IS at 2-fold IC50 values for 48 h, a significant increase in propidium iodide labeling was observed for all IS tested, indicating that the IS interfere with membrane integrity causing cell death (Figures 4A, B). Besides, as illustrated in Figure 4C, incubation with C16M4ImCl at 1.8 µM (IC50) for 48 h led to total lysis of the parasites.
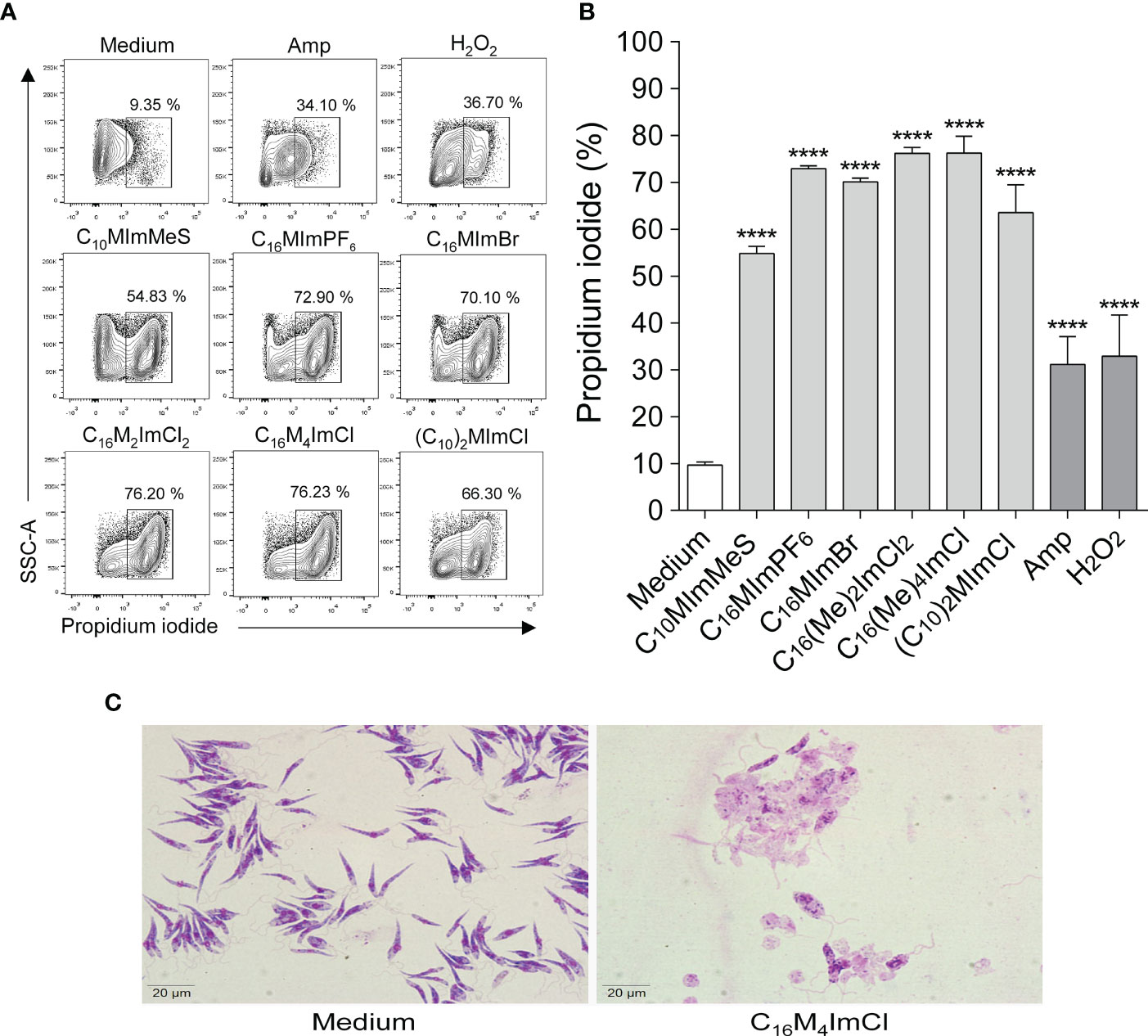
Figure 4 Determination of membrane integrity of L. amazonensis promastigotes treated with imidazolium salts (A, B). Promastigote forms in the logarithmic phase (2 x 105 cells/well) were incubated or not with 2-fold IC50 value of IS for 48 h at 26°C. M199 was used as negative control, and amphotericin B (0.4 µM) or H2O2 (2 mM) as positive controls. Data are expressed as means ± SEM (n = 5) and are representative of three independent experiments. The One-way ANOVA test followed by the Bonferroni’s test were applied. ****p < 0.0001 when compare with the medium group. (C) L. amazonensis integrity after 48 h of exposure to C16M4ImCl at 1.8 μM or M199 (panoptic stain – 1000 × magnification).
3.6 Imidazolium salts cause morphological alterations on L. amazonensis promastigotes
As illustrated in Figure 5, when promastigotes were treated with IS at IC50 values for 6 h, the parasites showed striking morphological alterations. While untreated parasites exhibited elongated shape, integral flagellum and membrane integrity (Figures 5A, B), promastigotes treated with amphotericin B at 0.4 µM (Figures 5C, D), as well as with C10MImMeS, C16MImPF6, C16MImBr, C16M2ImCl, C16M4ImCl or (C10)2MImCl (Figures 5E–J) evidenced rounded shape, membrane damage, flagellum disruption or twisted, and cell aggregation. In addition, C16MImMeS and C16MImCl that killed L. amazonensis in our previous study (13) also caused similar morphological alterations in promastigotes of L. amazonensis (Figures 5K, L).
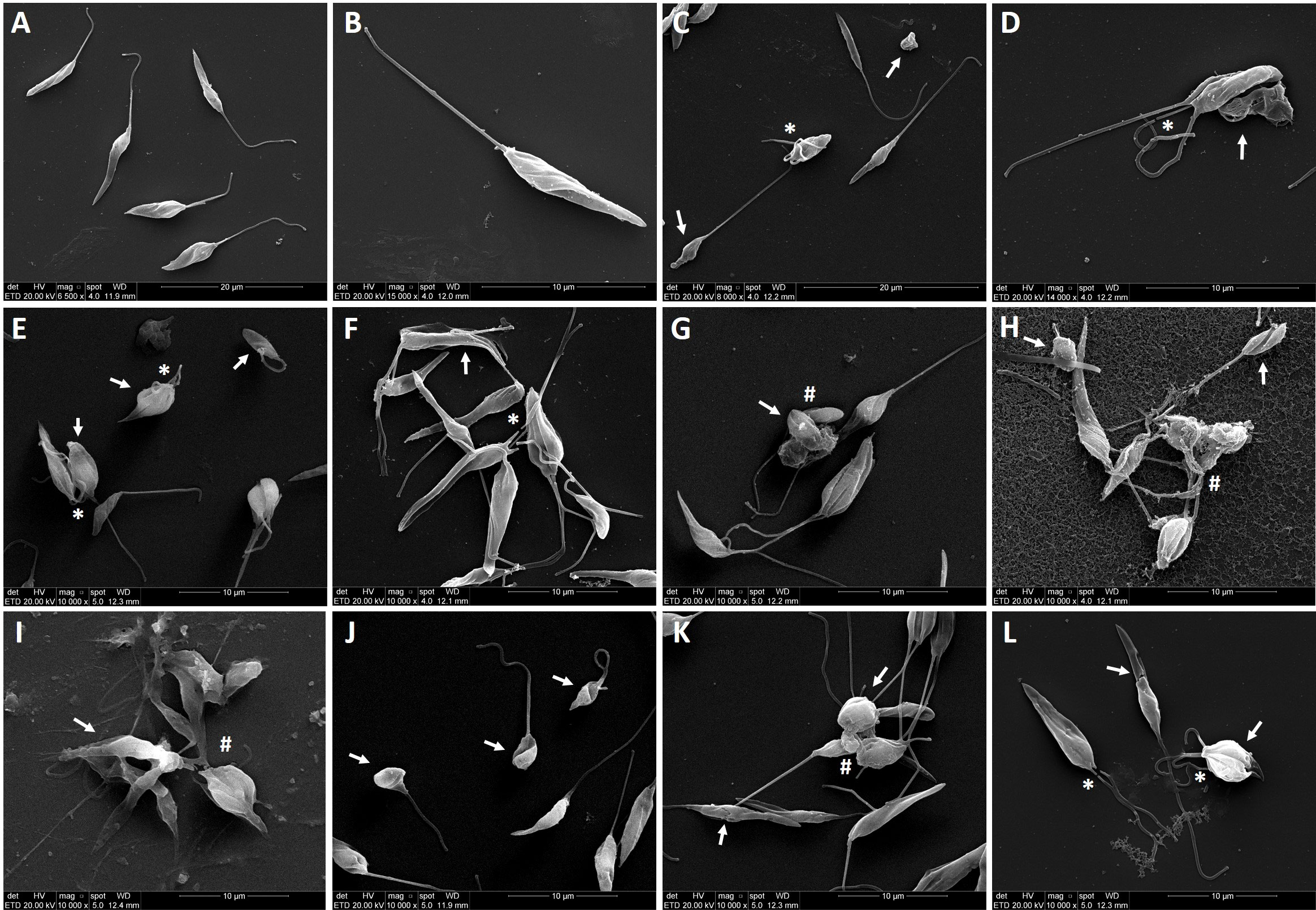
Figure 5 Scanning electron micrographs of L. amazonensis promastigotes treated or not with imidazolium salts at IC50 values for 6 h. Untreated parasites showing the typical elongated shape (parasite body and anterior flagella) (A, B). Parasites treatment with amphotericin B at 0.4 µM (C, D), C10MImMeS at 11.6 µM (E), C16MImPF6 at 6.9 µM (F), C16MImBr at 6 µM (G), C16M2ImCl at 4.1 µM (H), C16M4ImCl at 1.8 µM (I), (C10)2MImCl at 1.9 µM (J), C16MImMeS at 0.75 µM (K) and C16MImCl at 1.23 µM (L). The images show alterations in promastigote body shape or disruption of plasma membrane (arrows), flagellum loss (asterisks), and aggregation of cells (hashes). Bars: (A, C) represent 20 µm, (B) and (D–L) represent 10 µm.
3.7 Cytotoxicity of imidazolium salts on mammalian cells
In vitro cytotoxicity of imidazolium salts, C16Im and C16PyrCl (5 to 200 μM) towards macrophages, determined by MTT assay
In relation to the effects of IS on macrophages, a concentration-dependent cytotoxicity was found (Figure S39A: In vitro cytotoxicity of imidazolium salts, C16Im and C16PyrCl [5 to 200 μM] towards macrophages, determined by MTT assay). All IS at 10 µM kept more than 90% of the macrophages alive after 48 h of incubation, and C10MImMeS maintained this condition up to 50 µM. Besides that, C16M4ImCl, (C10)2MImCl and C10MImMeS at concentrations of 5.6-, 5.3- and 4.3-fold IC50 for L. amazonensis kept 90, 94 and 89% of macrophages viability, respectively. C16M4ImCl and (C10)2MImCl presented the best selectivity indexes (10 and 8.6, respectively) (Table 1). Moreover, human erythrocytes incubated with IS at 10 µM demonstrated hemolysis up to 8% and, as well as for macrophages, C10MImMeS was the less toxic IS, without significant hemolysis up to 40 µM (Figure S39B: In vitro cytotoxicity of imidazolium salts, C16Im and C16PyrCl (1 to 100 μM) towards human red blood cells, determined by hemolysis rates at 540 nm). C16M4ImCl and (C10)2MImCl also presented the best SI when considering hemolysis – 8.7 and 11, respectively (Table 1). The neutral imidazole C16Im presented lower cytotoxicity against both macrophages and erythrocytes, however it presented low SI (Table 1). The pyridinium salt C16PyrCl presented the higher toxicity and lowest SI among the tested compounds (Table 1).
3.8 Imidazolium salts reduce the intracellular survival of L. amazonensis on macrophages
As Leishmania can survive inside macrophages, we evaluate whether IS, C16Im and C16PyrCl stimulate the leishmanicidal activity of macrophages. Our data showed that all compounds at 1 µM were able to reduce amastigotes survival in 86% to 93% (Figure 6).
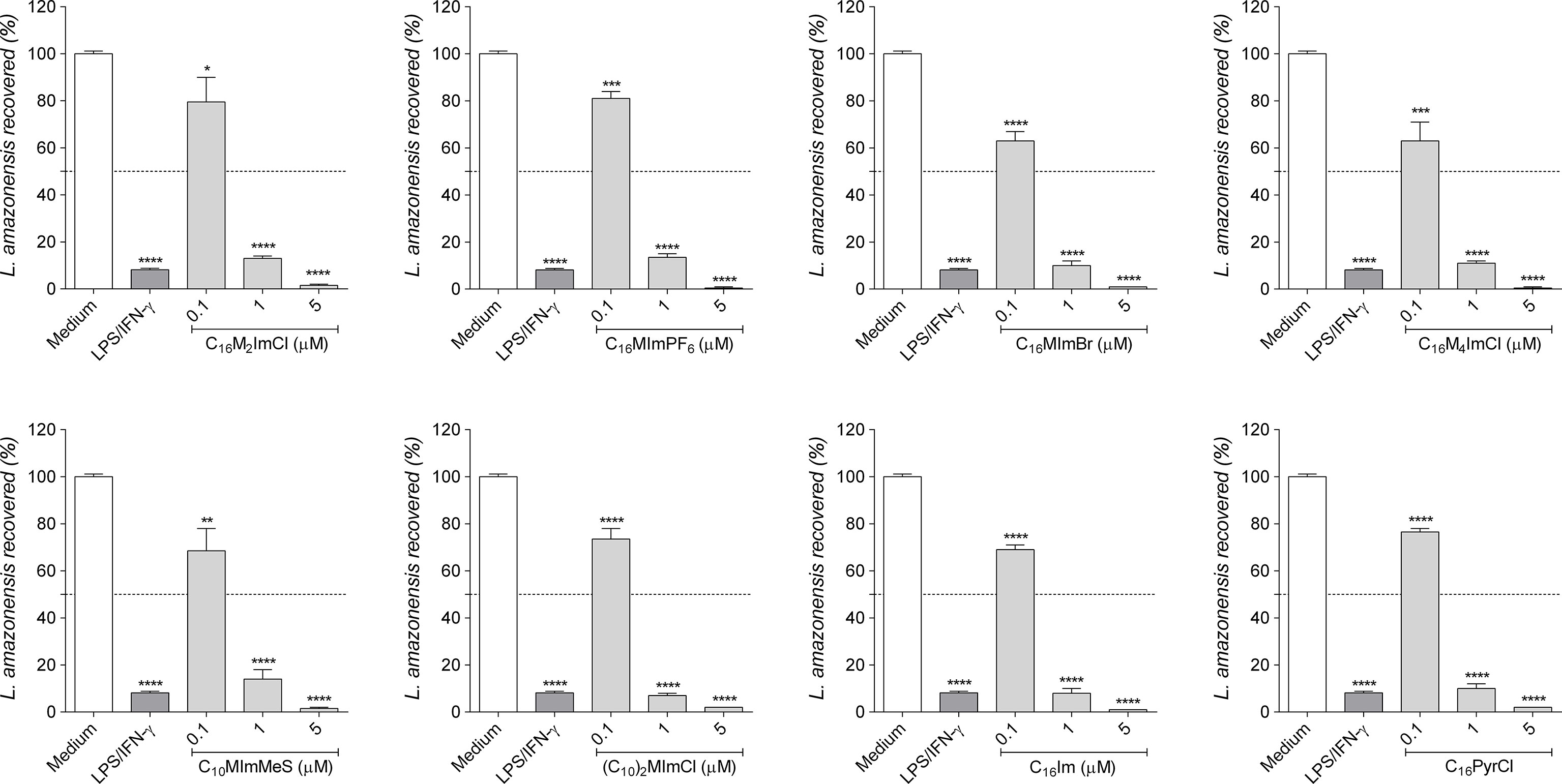
Figure 6 Intramacrophage anti-amastigote activity of compounds. RAW cells (2 × 105 cells/well) were infected with promastigotes of L. amazonensis (10 parasites/cell) in the stationary phase (day 5 of culture) and incubated with DMEM (control), lipopolysaccharide (LPS; 10 ng/mL) plus interferon-γ (IFN-γ; 1 ng/mL) as positive control, or IS, C16Im or C16PyrCl at concentrations of 0.1 μM, 1 μM or 5 μM for 48 h. After, cells were lysed, supplemented with M199 30% FBS and cultured at 26°C for 7 days to determine the number of promastigotes recovered using a hemocytometer. Data are expressed as means ± SEM (n = 5). The One-way ANOVA test followed by the Bonferroni’s test were applied. *p < 0.05, ** p < 0.01, ***p < 0.001 and ****p < 0.0001 when compared with the medium group (100% parasite recovered).
4 Discussion
C4MImMeS, C10MImMeS, C16MImBr, C4MImBF4, MImC10MImBr2, C7O3MImPF6, C7O3MImMeS, C4M2ImMeS, (C16)2ImCl, HOC3MImPF6, HO2CC1MImCl, (HO2CC1)2ImCl, HO2CC1MImBF4, HO2CC3MImCl, C16MImPF6, C16M4ImCl, C16M2ImCl, MImC5MImBr2, MImC8MImBr2, (C16)2ImMeS, (C16)2MmCl, and (C16)2MImMeS were synthesized, which along with the commercially available (C10)2MImCl, and HOC2MImCl constituted the group of 24 IS tested (Figure 1). The spectral data of C4MImMeS (14), C10MImMeS (15), C16MImBr (16), C4MImBF4 (14), MImC10MImBr2 (16), C7O3MImPF6 (15), C7O3MImMeS (15), C4M2ImMeS (17), C16Im (18), (C16)2ImCl (19), HOC3MImPF6 (20), HO2CC1MImCl (21), (HO2CC1)2ImCl (21), HO2CC1MImBF4 (22), HO2CC3MImCl (23), C16MImPF6 (24), C16M4ImCl (25), C10MeS (15), C16MeS (26), C4MeS (14), C16MIm (27), and C7O3MeS (15) are in agreement with those previously reported. For the new IS C16M2ImCl, MImC5MImBr2, MImC8MImBr2, (C16)2ImMeS, (C16)2MmCl, and (C16)2MImMeS, the NMR, FTIR and MS data support their structures and purity.
To the best of our knowledge, this is the first study reporting the leishmanicidal and immunomodulatory activities for C10MImMeS, C16MImPF6, C16MImBr, C16M2ImCl, C16M4ImCl and (C10)2MImCl (Figure 1) on two Leishmania species. Here, it was demonstrated that depending on the concentration, IS were able to kill infective forms of L. amazonensis and L. infantum chagasi (Figure S38).
In a previous study, we demonstrated that IS are able to kill both promastigote and amastigote forms of L. amazonensis (13). Here, we investigated a structurally complementary set of mono- (N-substituent = methyl; N’-substituent = n-butyl, n-decyl, n-hexadecyl, 2-hydroxyethyl, methylcarboxylic acid, propylcarboxylic acid, methoxycarbonylmethyl, triethylene glycol monomethyl ether; C2-substituent = H, methyl; C4-substituent = H, methyl; C5-substituent = H, methyl – N,N’-substituents = n-decyl, n-hexadecyl, methylcarboxylic acid; C2-substituent = H, methyl) and dicationic (N-substituent = methyl; alkane bridge = pentane, octane, decane) IS with different anions, as well as the neutral C16Im and the pyridinium salt C16PyrCl (Figure 1) against L. amazonensis and L. infantum chagasi. This provided the perspective to compare the chemical structures and extend the comprehension about activity of this class of compounds against Leishmania. Significant differences in leishmancidal activity of IS against L. amazonensis were observed (Table 1). (C10)2MImCl and C16M4ImCl presented the highest activity (lowest IC50 values): (C10)2MImCl = C16M4ImCl > C16M2ImCl > C16MImBr > C16MImPF6 > C10MImMes. Corroborating with scientific literature (10, 11, 13), the monocationic methylimidazolium salts with longer N-alkyl chain-lengths are closely related to higher leishmanicidal activities. In this sense, we point out that C16MImMeS showed the higher leishmanicidal activity (IC50 = 0.75 µM) (13) compared to C10MImMeS (IC50 = 11.6 µM) and C4MImMeS (without activity at 100 µM) tested here. On the other hand, shorter N-alkyl chain-lengths afford lower lipophilicity to the molecule and consequently less cytotoxic effects (32), as demonstrated by C10MImMeS which showed lower toxicity towards macrophages (CC50 = 76.9 µM) and erythrocytes (RBC50 > 100 µM) when compared to C16MImMeS (CC50 = 11.5 µM and RBC50 = 36.9 µM) (13).
The N,N’dialkyl substituted IS with n-decyl and n-hexadecyl groups (Figure 1) showed that, independent of the anions present in the n-hexadecyl-based IS (MeS, Cl or Br), only (C10)2MImCl presented leishmanicidal activity (IC50 = 1.9 μM), evidencing a higher leishmanicidal effect than C10MImCl – tested in the previous study of our group (IC50 = 2.31 µM) (13). Another study demonstrated that (C10)2MImCl also inhibits bacterial (Escherichia coli and Staphylococcus aureus) and fungal (Candida albicans and Candida tropicalis) growth in vitro (33), and the addition of two hydrophobic alkyl chains to the imidazolium ring also contributed greatly to improve the activity of IS against tumor-derived cell lines (34). This reaffirms the potential balance between hydrophilicity and lipophilicity, which is essential for the antimicrobial activity of IS (7).
The introduction of methyl groups on the C2-, C4- and C5-positions of the imidazolium ring influences the L. amazonensis mortality (Table 1). When comparing the activities of C16M4ImCl (IC50 = 1.8 µM) and C16M2ImCl (IC50 = 4.1 µM), the additional two methyl groups reduced 2.3-fold the IC50 value for L. amazonensis mortality. However, C16MImCl presented the lowest IC50 value among these three compounds (IC50 = 1.23 µM) (13). This H-methyl substituent alteration in the imidazolium ring does not seem to interfere to the same extend on L. infantum chagasi mortality, according to the IS C16M4ImCl (IC50 = 14.9 µM) and C16M2ImCl (IC50 = 15.4 µM).
From results of this study and our previous work (13), it is possible to compare the effect of different IS anions on L. amazonensis mortality. The IS C16MImMeS (IC50 = 0.75 µM), C16MImCl (IC50 = 1.23 µM) and C16MImNTf2 (IC50 = 1.64 µM) demonstrated higher capacities to kill L. amazonensis than C16MImBr (IC50 = 6.0 µM) and C16MImPF6 (IC50 = 6.9 µM), reaffirming the hypothesis that the IS anion also has an important but secondary effect on activity against Leishmania (13). For the C16MIm-based IS, the established order of anion-dependent inhibition was MeS > Cl > NTf2 > Br > PF6. As observed for L. amazonensis mortality with C16MImBr and C16MImPF6, Br and PF6 anions did not trigger differences in L. infantum chagasi mortality – C16MImPF6 (IC50 = 26.3 µM) and C16MImBr (IC50 = 29.7 µM). A decrease in anion volume has been related to an increasing fungal mortality, evidencing the IS with MeS and chloride anions as the most promising antifungals (9). This was also observed for anti-leishmanial activity of the IS.
Considering the highest leishmanial activity achieved with C16MImMeS, we tested the effect of its neutral imidazole equivalent C16Im on promastigote forms of Leishmania. This study showed that C16Im was not so effective to kill L. amazonensis as C16MImMeS. Furthermore, this compound presented lower toxicity than all other tested compounds, except C10MImMeS (Table 1). In contrast, C16Im showed a higher activity than the IS tested in this work against L. infantum chagasi.
Compounds containing cationic pyridinium heterocycles have concerned great awareness as prospective chemotherapeutic agents, such as anticancer, analgesic, antimicrobial and antiviral (35). Based on this, the effect of C16PyrCl on Leishmania mortality was tested, and this compound showed being effective against L. amazonensis (IC50 = 4.0 µM) (Figure S38A and Table 1), but being more toxic toward macrophages than IS (Figure S39A and Table 1). In our previous study we tested the structurally related IS C16MImCl, which showed higher leishmanicidal activity and lower macrophage and red blood cell cytotoxicity than C16PyrCl (13). C16PyrCl also demonstrated high capacity to kill L. infantum chagasi (IC50 = 2.6 µM) (Figure S38B). Although this compound is commonly used as antiseptic (mouthwash), its high toxicity against macrophages turns it into a weak candidate for drug development.
To elucidate possible mechanisms of action of IS on Leishmania parasites, membrane and mitochondrial dysfunctions, ROS generation and morphological changes in response to IS were investigated. In Leishmania, mitochondria are a drug target (36), since this unique organelle is the main site of ROS production, and excessive ROS may lead to oxidative stress, which may be one of the major contributors to cell death by IS (37, 38). In addition, the maintenance of an appropriate mitochondrial membrane potential (ΔΨm) is essential for Leishmania survival (36, 37). Here, we demonstrate that IS with leishmanicidal activity caused an increase in ROS production (Figures 4A, B) and ΔΨm alterations (Figures 34C, D) in L. amazonensis. C10MImMeS triggered mitochondrial membrane hyperpolarization, while C16MImPF6, C16MImBr, C16M2ImCl, C16M4ImCl and (C10)2MImCl caused depolarization of mitochondrial membrane. Both hyperpolarization and depolarization can result in promastigotes death (29), and depolarization can be preceded by a transient hyperpolarization as the last attempt by cells to avoid death (39). Moreover, all six IS presenting leishmanicidal activity caused plasma membrane alterations (Figures 4A, B), indicating cell death involving necrosis (40).
In this regard, the scanning electron microscopy analyses revealed that the treatment with IS induced swelling and overall rounding, cell aggregation, membrane rupture, besides lower parasite length and flagellum damage (Figure 5), corroborating with evidences of the action of IS on L. amazonensis (13). In addition to the biochemical alterations, IS inhibited the in vitro growth of promastigotes forms of L. amazonensis (Figure 2A) and L. infantum chagasi (Figure 2B). This effect could be related to the ergosterol availability. Similarly to fungi, ergosterol in Leishmania is essential for the organization of membrane domains, cell growth and survival. In a previous study it was demonstrated that C16MImCl reduced the total ergosterol content of clinical isolates of yeasts, suggesting that IS inhibit the ergosterol biosynthesis (8). Although C16MImCl and other IS presented potent leishmanicidal activity, the activity of enzyme involved in ergosterol synthesis (lanosterol 14α-demethylase) or ergosterol content were not evaluated in this study.
IS, C16Im and C16PyrCl at 1 µM reduced the amastigote survival of L. amazonensis in about 90% (Figure 6), with an IC50 between 0.2 to 0.3 and higher SI (31.5 to 275) than those calculated for promastigotes, indicating a higher selectivity for amastigotes. These data are in agreement with our previous data showing the better selectivity of IS for amastigotes (13).
For the first time, the IS C10MImMeS, C16MImPF6, C16MImBr, C16M2ImCl, C16M4ImCl, and (C10)2MImCl have been identified as potent agents against L. amazonensis. These compounds induced ROS generation, membrane, and mitochondrial dysfunction in parasites. The results reaffirm the hypothesis that IS with longer N-alkyl chain-lengths are related with higher biological activities, and on a secondary basis IS with MeS and chloride anions are the most promising antileishmanial agents. As a limitation of this study, we mentioned that we did not test the effects of these IS on antimony-resistant Leishmania spp. lines, or their in vivo effects.
5 Conclusions
The IS C10MImMeS, C16MImPF6, C16MImBr, C16M2ImCl, C16M4ImCl and (C10)2MImCl, and the compounds C16Im and C16PyrCl killed and inhibited the growth of promastigote forms of L. amazonensis and L. infantum chagasi in a concentration-dependent manner. Moreover, induced ROS production, mitochondrial dysfunction, membrane disruption and morphological alterations in infective forms of L. amazonensis and were able to kill intracellular amastigote forms in a very low concentration, being worthy of further in vivo studies as potential antileishmanial drugs.
Data availability statement
The original contributions presented in the study are included in the article/Supplementary Material. Further inquiries can be directed to the corresponding authors.
Author contributions
Conceptualization PR, HS, FB. Methodology: FB, TF, MS, PF, VS, DP, JK, ES. Software: TF, FB, LJ. Validation: FB, TF. Formal analysis: FB, LJ. Investigation: FB, ED, TF. Resources: PR, HS, MM. Data curation: FB, PR. Writing—original draft preparation: KN-L, FB, PR, TF. Writing—review and editing: MM. PR, HS. Visualization: AP, LJ, MM, ED. Supervision: PR, HS, MM. Project administration: PR, HS. Funding acquisition: PR, HS, MM. All authors contributed to the article and approved the submitted version.
Funding
This project was funded, in part, with support from the Fundação de Amparo à Pesquisa do Estado de Rio Grande do Sul (FAPERGS), Coordenação de Aperfeiçoamento de Pessoal de Nível Superior – Brasil (CAPES) Finance Code 001, and Conselho Nacional de Desenvolvimento Científico e Tecnológico (CNPq). This project was also funded, in part, with support from the Federal University of Health Sciences of Porto Alegre. TF is recipient of a PNPD/CAPES fellowship. MM, AP, HS and PR are Research Career Awardees of the CNPq.
Acknowledgments
The authors were most grateful for the financial support by the Brazilian’s agencies: Conselho Nacional de Desenvolvimento Científico e Tecnológico (CNPq), Coordenação de Aperfeiçoamento de Pessoal de Nível Superior (CAPES), Fundação de Amparo à Pesquisa do Estado de Rio Grande do Sul (FAPERGS), Federal University of Pará and MM, AP, HS and PR thanks for the fellowship from CNPq.
Conflict of interest
The authors declare that the research was conducted in the absence of any commercial or financial relationships that could be construed as a potential conflict of interest.
Publisher’s note
All claims expressed in this article are solely those of the authors and do not necessarily represent those of their affiliated organizations, or those of the publisher, the editors and the reviewers. Any product that may be evaluated in this article, or claim that may be made by its manufacturer, is not guaranteed or endorsed by the publisher.
Supplementary material
The Supplementary Material for this article can be found online at: https://www.frontiersin.org/articles/10.3389/fimmu.2022.1096312/full#supplementary-material
References
1. OPAS. Manual de procedimientos para vigilancia y control de las leishmaniasis en las américas. (2019). doi: 10.37774/9789275320631.
2. WHO. Leishmaniasis in high-burden countries: An epidemiological update based on data reported in 2014. Wkly Epidemiol Rec (2016) 91:287–96.
3. Silveira FT, Lainson R, Corbett CEP. Clinical and immunopathological spectrum of american cutaneous leishmaniasis with special reference to the disease in Amazonian Brazil - a review. Mem Inst Oswaldo Cruz (2004) 99:239–51. doi: 10.1590/S0074-02762004000300001
4. Burza S, Croft SL, Boelaert M. Leishmaniasis. Lancet (2018) 392:951–70. doi: 10.1016/S0140-6736(18)31204-2
5. Tiuman TS, Santos AO, Ueda-Nakamura T, Filho BPD, Nakamura CV. Recent advances in leishmaniasis treatment. Int J Infect Dis (2011) 15:525–32. doi: 10.1016/j.ijid.2011.03.021
6. Emami S, Tavangar P, Keighobadi M. An overview of azoles targeting sterol 14α-demethylase for antileishmanial therapy. Eur J Med Chem (2017) 135:241–59. doi: 10.1016/j.ejmech.2017.04.044
7. Pendleton JN, Gilmore BF. The antimicrobial potential of ionic liquids: A source of chemical diversity for infection and biofilm control. Int J Antimicrob Agents (2015) 46:131–9. doi: 10.1016/j.ijantimicag.2015.02.016
8. Schrekker HS, Donato RK, Fuentefria AM, Bergamo V, Oliveira LF, Machado MM. Imidazolium salts as antifungal agents: Activity against emerging yeast pathogens, without human leukocyte toxicity. Medchemcomm (2013) 4:1–5. doi: 10.1039/c3md00222e
9. Dalla Lana DF, Donato RK, Bündchen C, Guez CM, Bergamo VZ, de Oliveira LFS, et al. Imidazolium salts with antifungal potential against multidrug-resistant dermatophytes. J Appl Microbiol (2015) 119:377–88. doi: 10.1111/jam.12862
10. Goellner E, Schmitt AT, Couto JL, Muller ND, Pilz Junior HL, Schrekker HS, et al. Larvicidal and residual activity of imidazolium salts against aedes aegypti (Diptera: Culicidae). Pest Manag Sci (2018) 74:1013–9. doi: 10.1002/ps.4803
11. Riduan SN, Zhang Y. Imidazolium salts and their polymeric materials for biological applications. Chem Soc Rev (2013) 42:9055–70. doi: 10.1039/c3cs60169b
12. Rakers L, Glorius F. Flexible design of ionic liquids for membrane interactions. Biophys Rev (2018) 10:747–50. doi: 10.1007/s12551-018-0412-9
13. Martins RC, Dorneles GP, Teixeira VON, Antonello AM, Couto JL, Rodrigues Júnior LC, et al. Imidazolium salts as innovative agents against leishmania amazonensis. Int Immunopharmacol (2018) 63:101–9. doi: 10.1016/j.intimp.2018.07.038
14. Cassol CC, Ebeling G, Ferrera B, Dupont J. A simple and practical method for the preparation and purity determination of halide-free imidazolium ionic liquids. Adv Synth Catal (2006) 348:243–8. doi: 10.1002/adsc.200505295
15. Schrekker HS, Silva DO, Gelesky MA, Stracke MP, Schrekker CML, Gonçalves RS, et al. Preparation, cation-anion interactions and physicochemical properties of ether-functionalized imidazolium ionic liquids. J Braz Chem Soc (2008) 19:426–33. doi: 10.1590/S0103-50532008000300009
16. Villetti MA, Ziembowicz FI, Bender CR, Frizzo CP, Martins MAP, De Souza TD, et al. Thermodynamic insights into the binding of mono- and dicationic imidazolium surfactant ionic liquids with methylcellulose in the diluted regime. J Phys Chem B (2017) 121:8385–98. doi: 10.1021/acs.jpcb.7b03525
17. Scott M, Deuss PJ, De Vries JG, Prechtl MHG, Barta K. New insights into the catalytic cleavage of the lignin β-O-4 linkage in multifunctional ionic liquid media. Catal Sci Technol (2016) 6:1882–91. doi: 10.1039/c5cy01554e
18. Chakraborty A, Debnath S, Ghosh T, Maiti DK, Majumdar S. An efficient strategy for n-alkylation of benzimidazoles/imidazoles in SDS-aqueous basic medium and n-alkylation induced ring opening of benzimidazoles. Tetrahedron (2018) 74:5932–41. doi: 10.1016/j.tet.2018.08.029
19. Rohini R, Lee CK, Lu JT, Lin IJB. Symmetrical 1, 3-dialkylimidazolium based ionic liquid crystals. J Chin Chem Soc (2013) 60:745–54. doi: 10.1002/jccs.201200598
20. Zhang S, Qi X, Ma X, Lu L, Deng Y. Hydroxyl ionic liquids: The differentiating effect of hydroxyl on polarity due to ionic hydrogen bonds between hydroxyl and anions. J Phys Chem B (2010) 114:3912–20. doi: 10.1021/jp911430t
21. Huang Z, Wang Y, Zhang N, Zhang L, Darensbourg DJ. One-pot synthesis of ion-containing CO2-based polycarbonates using protic ionic liquids as chain transfer agents. Macromolecules (2018) 51:9122–30. doi: 10.1021/acs.macromol.8b01834
22. Miao CX, He LN, Wang JQ, Wang JL. TEMPO and carboxylic acid functionalized imidazolium salts/sodium nitrite: An efficient, reusable, transition metal-free catalytic system for aerobic oxidation of alcohols. Adv Synth Catal (2009) 351:2209–16. doi: 10.1002/adsc.200900285
23. Xu T, Waehler T, Vecchietti J, Bonivardi A, Bauer T, Schwegler J, et al. Gluing ionic liquids to oxide surfaces: Chemical anchoring of functionalized ionic liquids by vapor deposition onto Cobalt(II) oxide. Angew Chem Int Ed (2017) 56:9072–6. doi: 10.1002/anie.201704107
24. Xu F, Matsumoto K, Hagiwara R. Effects of alkyl chain length and anion size on thermal and structural properties for 1-alkyl-3-methylimidazolium hexafluorocomplex salts (C xMImAF 6, x = 14, 16 and 18; a = p, as, Sb, Nb and Ta). Dalton Trans (2012) 41:3494–502. doi: 10.1039/c2dt11693f
25. Neves YF, Eloi ACL, de Freitas HMM, Soares EGO, Rivillo D, Demétrio da Silva V, et al. Imidazolium salts as alternative compounds to control diseases caused by plant pathogenic bacteria. J Appl Microbiol (2020) 128:1236–47. doi: 10.1111/jam.14575
26. Toppino A, Bova ME, Crich SG, Alberti D, Diana E, Barge A, et al. A carborane-derivative “click” reaction under heterogeneous conditions for the synthesis of a promising lipophilic MRI/GdBNCT agent. Chem Eur J (2013) 19:721–8. doi: 10.1002/chem.201201634
27. Langat J, Bellayer S, Hudrlik P, Hudrlik A, Maupin PH, Gilman JW, et al. Synthesis of imidazolium salts and their application in epoxy montmorillonite nanocomposites. Polymer (Guildf) (2006) 47:6698–709. doi: 10.1016/j.polymer.2006.06.067
28. Antonello AM, Sartori T, Folmer Correa AP, Brandelli A, Heermann R, Rodrigues Júnior LC, et al. Entomopathogenic bacteria photorhabdus luminescens as drug source against leishmania amazonensis. Parasitology (2018) 145:1065–74. doi: 10.1017/S0031182017002001
29. Dagnino APA, Mesquita CS, Dorneles GP, Teixeira VDON, De Barros FMC, Vidal Ccana-Ccapatinta G, et al. Phloroglucinol derivatives from hypericum species trigger mitochondrial dysfunction in leishmania amazonensis. Parasitology (2018) 145:1199–209. doi: 10.1017/S0031182018000203
30. Cezarotto CS, Dorneles A, Baldissera FG, da Silva MB, Markoski MM, Rodrigues-Júnior LC, et al. Leishmanicidal and antichemotactic activities of icetexanes from salvia uliginosa benth. Phytomedicine (2018), 58. doi: 10.1016/j.phymed.2018.11.009
31. Wu D, Yotnda P. Production and detection of reactive oxygen species (ROS) in cancers. J Vis Exp (2011) 57:2–5. doi: 10.3791/3357
32. Garcia MT, Gathergood N, Scammells PJ. Biodegradable ionic liquids part II. effect of the anion and toxicology. Green Chem (2005) 7:9–14. doi: 10.1039/b411922c
33. Raucci MG, Fasolino I, Pastore SG, Soriente A, Brentano L, Dessuy M, et al. Antimicrobial imidazolium ionic liquids for the development of minimal invasive calcium phosphate-based bio-nanocomposites. ACS Appl Mater Interfaces (2018) 10:42766–76. doi: 10.1021/acsami.8b12696
34. Wang D, Galla HJ, Drücker P. Membrane interactions of ionic liquids and imidazolium salts. Biophys Rev (2018) 10:735–46. doi: 10.1007/s12551-017-0388-x
35. Kumar S, Sharma PK, Dudhe R, Kumar N. Pyridine: Potential for biological activities. J Chron Drug Delivery (2011) 2:71–8.
36. Fidalgo LM, Gille L. Mitochondria and trypanosomatids: Targets and drugs. Pharm Res (2011) 28:2758–70. doi: 10.1007/s11095-011-0586-3
37. Granato JT, Santos JA, dos, Calixto SL, Prado da Silva N, da Silva Martins J, da Silva AD, et al. Novel steroid derivatives: Synthesis, antileishmanial activity, mechanism of action, and in silico physicochemical and pharmacokinetics studies. BioMed Pharmacother (2018) 106:1082–90. doi: 10.1016/j.biopha.2018.07.056
38. Martins TVF, Zeraik AE, Alves NO, de Oliveira LL, Mendes TA, de O, et al. Lipophosphoglycan 3 from leishmania infantum chagasi binds heparin with micromolar affinity. Bioinform Biol Insights (2018) 12. doi: 10.1177/1177932218763363
39. Jiménez-Ruiz A, Alzate JF, MacLeod ET, Lüder CGK, Fasel N, Hurd H. Apoptotic markers in protozoan parasites. Parasit Vectors (2010) 3:104. doi: 10.1186/1756-3305-3-104
Keywords: imidazolium salt, L. amazonensis, L. infantum chagasi, oxygen reactive species, leishmanicidal activity
Citation: Baldissera FG, Fazolo T, da Silva MB, de Santana Filho PC, da Silva VD, Rivillo Perez DM, Klitzke JS, de Oliveira Soares EG, Rodrigues Júnior LC, Peres A, Dallegrave E, Navegantes-Lima KC, Monteiro MC, Schrekker HS and Torres Romão PR (2023) Imidazolium salts as an alternative for anti-Leishmania drugs: Oxidative and immunomodulatory activities. Front. Immunol. 13:1096312. doi: 10.3389/fimmu.2022.1096312
Received: 14 November 2022; Accepted: 30 December 2022;
Published: 17 January 2023.
Edited by:
Nemat Ali, King Saud University, Saudi ArabiaReviewed by:
Danilo Ciccone Miguel, State University of Campinas, BrazilRohit Sharma, Banaras Hindu University, India
Mohammad Arish, University of Virginia, United States
Copyright © 2023 Baldissera, Fazolo, da Silva, de Santana Filho, da Silva, Rivillo Perez, Klitzke, de Oliveira Soares, Rodrigues Júnior, Peres, Dallegrave, Navegantes-Lima, Monteiro, Schrekker and Torres Romão. This is an open-access article distributed under the terms of the Creative Commons Attribution License (CC BY). The use, distribution or reproduction in other forums is permitted, provided the original author(s) and the copyright owner(s) are credited and that the original publication in this journal is cited, in accordance with accepted academic practice. No use, distribution or reproduction is permitted which does not comply with these terms.
*Correspondence: Henri Stephan Schrekker, aGVucmkuc2NocmVra2VyQHVmcmdzLmJy; Marta Chagas Monteiro, bWFydGFjaGFnYXMyQHlhaG9vLmNvbS5icg==