- 1Bond Life Sciences Center, University of Missouri, Columbia, MO, United States
- 2Department of Otolaryngology-Head and Neck Surgery, School of Medicine, University of Missouri, Columbia, MO, United States
- 3Department of Surgery, School of Medicine, University of Missouri, Columbia, MO, United States
- 4Department of Molecular Microbiology and Immunology, School of Medicine, University of Missouri, Columbia, MO, United States
- 5Department of Biological and Biomedical Engineering, College of Engineering, University of Missouri, Columbia, MO, United States
- 6Division of Clinical Pharmacology, Department of Pediatrics, School of Medicine, University of Utah, Salt Lake City, UT, United States
- 7Department of Molecular Pharmaceutics, College of Pharmacy, University of Utah, Salt Lake City, UT, United States
- 8Department of Biochemistry, University of Missouri, Columbia, MO, United States
Sjögren’s syndrome is a chronic inflammatory autoimmune disease characterized by diminished secretory function of the exocrine glands. Although extensive investigation has been done to understand Sjögren’s syndrome, the causes of the disease are as yet unknown and treatments remain largely ineffective, with established therapeutic interventions being limited to use of saliva substitutes with modest effectiveness. A primary feature of Sjögren’s syndrome is uncontrolled inflammation of exocrine tissues and previous studies have demonstrated that lipid-based specialized pro-resolving mediators reduce inflammation and restores tissue integrity in salivary glands. However, these studies are limited to a single specialized pro-resolving lipid mediator’s family member resolvin D1 or RvD1 and its aspirin-triggered epimer, AT-RvD1. Consequently, additional studies are needed to explore the potential benefits of other members of the specialized pro-resolving lipid mediator’s family and related molecules (e.g., additional resolvin subtypes as well as lipoxins, maresins and protectins). In support of this goal, the current review aims to briefly describe the range of current experimental methods to investigate the impact of specialized pro-resolving lipid mediators on Sjögren’s syndrome, including both strengths and weaknesses of each approach where this information is known. With this article, the possibilities presented by specialized pro-resolving lipid mediators will be introduced to a wider audience in immunology and practical advice is given to researchers who may wish to take up this work.
1 Introduction
Sjögren’s syndrome (SS) is a chronic inflammatory autoimmune disease characterized by diminished secretory function of the exocrine glands (1–9). It affects approximately 1% of the general population and up to 3% of people above the age of fifty, with women accounting for more than 90% of diagnosed cases (10, 11). Although extensive investigation has been done to understand the disease (12–14), the causes of SS are as yet unknown and treatments remain largely ineffective, with established therapeutic interventions being limited to use of saliva substitutes with modest effectiveness (15–17) and medications that provide only temporary relief (16). As such, development of alternative treatments to restore salivary gland (SG) functioning is critical.
A primary feature of SS is a systemic uncontrolled inflammation of exocrine tissues (18, 19) thought to be initiated by viral and bacterial infection together with the activation of susceptibility genes (20–24). This leads to an initial tissue damage followed by cytokines and chemokine release (25–28) as well as exaggerated antibody production by hyperactivated B cells (7, 18, 19), all of which can be detected on exocrine tissues and serum (29). When resolution mechanisms are working properly, neutrophils and M2 macrophages are able to clear the injury/infection site (30–33). However, when these mechanisms are absent, dead cells cannot be removed from the injury/infection site leading to production of autoantigens, elevations in chemokine and cytokine levels (34) that stimulate peripheral lymphocytes to bind to and infiltrate across the vascular endothelium into the SG (35–37), which leads to chronic immune responses, SG damage and dysfunction (38–41). To fully investigate the cell-specific mechanisms involved in the initiation of the inflammatory response, proven mouse models having SS-like features are required (42–45) to allow for identification of novel targets and approaches for inhibiting a futile systemic immune response and averting chronic inflammation (46–49).
Resolution of inflammation is an actively regulated process mediated in part by a family of specialized pro-resolving lipid mediators (SPM) (50–54) which include resolvins, maresins, lipoxins and protectins as well as their aspirin-triggered (AT) forms, which are comparable in their properties to naturally occurring SPM (reviewed in (55–62), but have a longer half-life (58, 63). The SPM receptors belong to the G-protein coupled receptor (GPCR) family as detailed in Figure 1A (64–66). SPM and their AT forms present an intriguing alternative for treating inflammatory diseases by limiting uncontrolled inflammation in response to environmental challenges (67–73) while at the same time promoting its termination and likewise leading to tissue regeneration (74–81). They have been detected in human fluids (e.g., saliva) as well as in animal models of infection and inflammation (82–87). Studies of SPM and AT forms within the SG have been largely confined to a single resolvin (AT-RvD1, one of many in the resolvin family) that has shown promise for treating hyposalivation. Specifically, previous studies demonstrated that AT-RvD1 reduces inflammation and restores tissue integrity in SG cells (88–91), AT-RvD1 biosynthetic and signaling pathways are active in mice and human SG (89–93), the progression of the SS-like features in NOD/ShiLtJ mice is halted using AT-RvD1, with mice treated systemically prior to disease onset displaying downregulation of pro-inflammatory cytokines, upregulation of anti-inflammatory molecules and intact saliva production (44, 94), similar results are obtained with AT-RvD1 treatment at disease onset and lack of the RvD1 receptor ALX/FPR2 leads to impaired innate (42) and adaptive immunity (33) in mouse submandibular glands (SMG), with such results derived within the SG being consistent with studies obtained using the full range of SPM in other organs (95–105). Despite the promise shown by SPM in general and AT-RvD1 in particular for treating SS, there are several obstacles that must be overcome for this treatment to be implemented in oral medicine. First, we only know the effects of RvD1 and AT-RvD1 within the SG (43, 44, 88, 91, 94), while other SPM that may contribute to resolution of inflammation in the SG are as yet unexplored. Next, there is a limited knowledge regarding how specific resolution mechanisms work in SG in vivo (106, 107) and still less is known in humans. Finally, researchers need to know the available options to study SPM in relation to autoimmunity; however, information as to the relative benefits and limitations of common experimental methods is not readily available in a single source. Together, an overarching review of the investigation models used to better understand the impact of SPM on SS is needed and for the purpose of this compilation we have divided the methods into three broad categories: in vitro studies, in vivo studies and mathematical models.
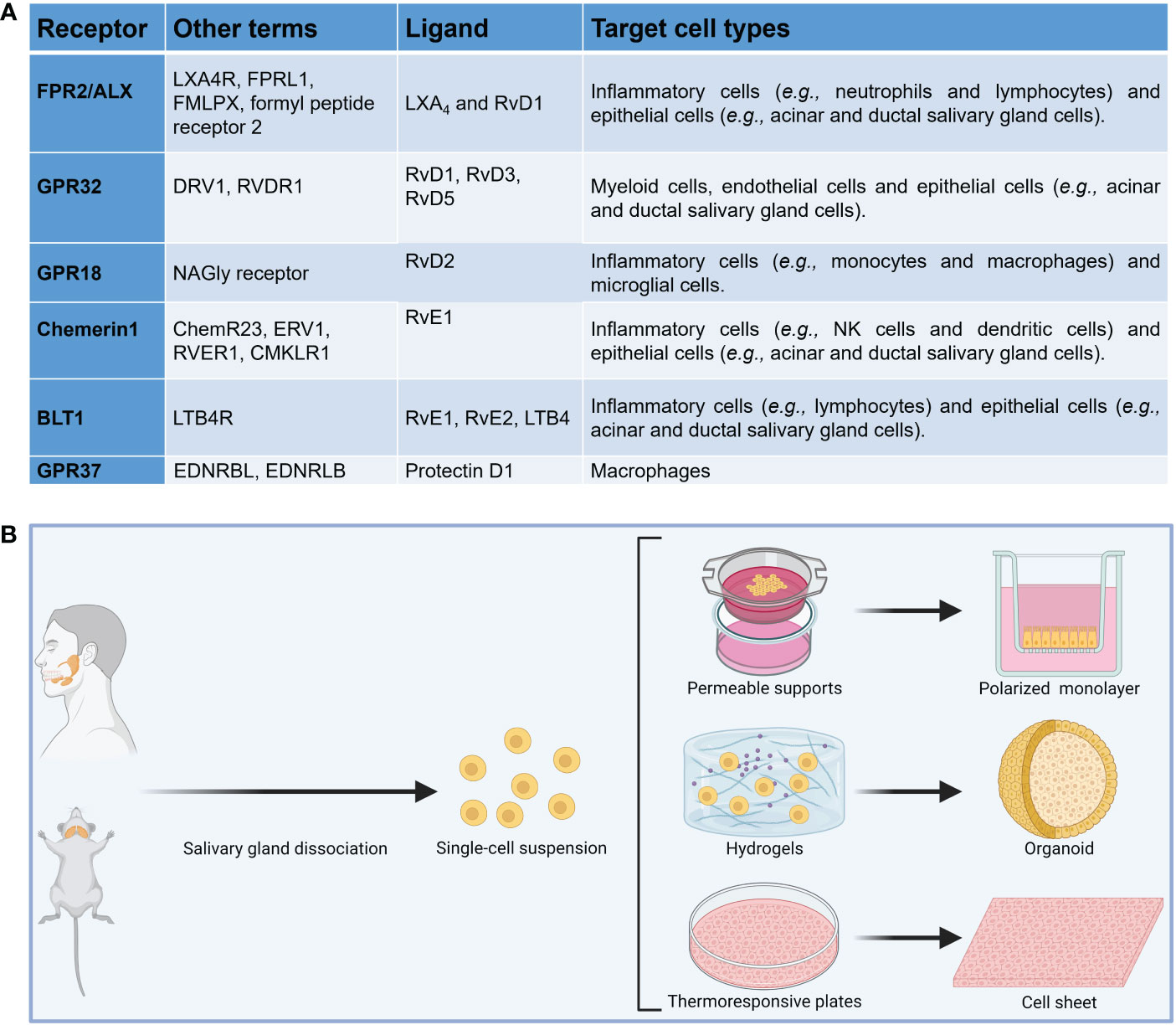
Figure 1 (A) Summary of SPM receptors, ligands and target cell types implicated in the resolution of inflammation. (B) Diagram showing common in vitro models to investigate the impact of specialized pro-resolving lipid mediators in salivary glands.
2 Methods to investigate SPM using in vitro models
The main eicosapentaenoic acid (EPA), docosahexaenoic acid (DHA) and arachidonic acid (AA)-based metabolomes operating during resolution of inflammation have been discovered and can provide insight into the mechanisms of inflammation resolution in multiple organs (65, 108). Applying these findings to SS, previous studies found that these metabolomes are present in mouse and human SG as well as saliva (109). Given the above, it is necessary to study spatio-temporal SPM biosynthesis and the upstream signaling mechanisms for EPA, DHA and AA-based metabolomes during resolution of inflammation in SS. Generally speaking, however, understanding of SPM upstream signaling mechanisms in SG lags behind that of downstream mechanisms in other organs (58–60, 103, 110–113) and, as earlier stated, the only SPM to have been studied extensively to date is RvD1 and its aspirin-triggered epimer (88–91, 94).
To investigate whether SPM biosynthesis is dysregulated in vitro, several models using freshly isolated cells from SS-like mouse models and SS human specimens can be compared to their healthy counterparts. These cells may be plated on permeable supports to allow monolayer formation (91, 114), scaffolds (e.g., hydrogels) to form SG organoids (94, 115) or thermoresponsive plates to form cell sheets (116) (Figure 1B). Permeable supports will permit us to study apico-basal polarized SPM biosynthesis while scaffolds will help us to understand polarized SPM biosynthesis in a three-dimensional environment. Nevertheless, permeable supports assays have limitations, including staining steps that may wash off attached cells and unevenly distributed membrane pores; however, protocol optimization can minimize this limitation (117). Moreover, cell sheets will further our understanding of how SPM are produced in a more natural environment (i.e., in the presence of extracellular matrix proteins and secretory granules), which are absent in the prior two models. A limitation of cell sheets is that the exact cell composition is still under investigation and future studies using RNA seq might be needed (118). Finally, the use of fresh human minor SG can verify results obtained with mouse models in humans; yet cell culture optimization is critical to ensure cells viability under in vitro conditions. Measuring SPM and their metabolites at various times points in each of these models using both ELISA (109) and targeted metabololipidomics (119), with results from each in vitro model analyzed and SPM biosynthesis among these complementary models, can provide an approximation of how SPM biosynthesis occurs in vivo. These experiments can use EPA, DHA and AA as substrates for SPM biosynthesis in vitro while also using ELISA and metabololipidomics in response to these lipids (109, 119).
SPM are generated in resolvin exudates in vivo and are a product of transcellular biosynthesis with human leukocytes and endothelial or epithelial cells (63, 65, 120), with sequential oxygenations by human 15-lipoxygenase (LOX) in humans or mouse ortholog 12/15-LOX and 5-LOX (121) involved in their biosynthesis. The first step in SPM biosynthesis involves release of ω-3 fatty acids from membrane phospholipids by PLA2, which is responsible for lipid release in mammalian cells (122, 123). The second step is transformation of ω-3 fatty acids to 17S-Hp forms by the enzymatic conversion through 15-LOX or 12/15-LOX forms (61, 63, 124), with the final step in SPM biosynthesis being release of 17S-Hp forms from epithelial or endothelial cells to the extracellular medium. 17S-Hp forms are then captured by adjacent cells and transformed into SPM by the action of the enzyme 5-LOX (61, 63, 124). In the presence of aspirin, the 15-LOX or 12/15-LOX activities in epithelial cells are transformed into cyclooxygenases by COX-2 activation (63). This leads to the formation of 17R-Hp forms, which is transformed to AT-forms by 5-LOX (63). In light of the above, it is important to measure expression of biosynthetic enzymes, determine their activities and quantify metabolite production in response to ω-3 fatty acids. Finally, for these studies it is critical to measure the impact of enzymes involved in SPM biosynthesis using mouse lines specific to each particular enzyme (e.g., 5LOX-/-).
3 Methods to investigate SPM using in vivo models
Having expanded SPM mechanistic studies to additional morphological factors using the various in vitro models listed above, it is necessary to further isolate SPM mechanisms by studying their metabolomes in vivo, thereby tracking mechanisms originating in the SG and operating in conjunction with other organs throughout the body, as detailed below. Specifically, SPM biosynthesis and signaling pathways may be activated in response to a wide variety of internal and/or environmental stressors (31, 125–128), such that the use of multiple mouse models covering these various conditions can be utilized as follows: NOD/ShiLtJ mouse model for autoimmune disease (129–134), NOD.H-2h4 mouse expressing the major histocompatibility complex haplotype H-2K on the NOD mouse background, rendering the NOD.H-2h4 mouse susceptible to autoimmune-like features, including spontaneous thyroiditis and SS-like features, but not diabetes (135–137), surgically wounded mouse SMG for tissue injury (138–140) and LPS-challenged mouse model (42). Additionally, although the NOD mouse lines are accepted models for SS, use of the wounded and LPS mice facilitate comparisons between inflammation due to physical injury and infection, respectively. Together, these models allow for measurement of SPM production in plasma, saliva and SMG during both acute and chronic inflammation.
To further identify SPM downstream mechanisms, receptor signaling studies are necessary. For instance, the G protein-coupled ALX/FPR2 receptor for AT-RvD1 is effective in restoring secretory function in SS mouse SG (43, 44, 94), indicating that activation of this receptor diminishes the systemic immune response. Given the remarkable effects with AT-RvD1, it is important to investigate the downstream mechanisms that resolve chronic inflammation via ALX/FPR2 or through activation of additional SPM receptors using SS mouse models and test the effect of targeting SPM in SS mouse models to diminish systemic immune response. One such model is the NOD/ShiLtJ mouse that can be studied at five time points representing the pre-disease stage (4 wk), the early pre-clinical stage (8 wk), the early clinical phase of autoimmunity (12-16 wk) and the onset of clinical SS-like features characterized by secretory dysfunction (16-20 wk). At 8-16 wk, lymphocytic infiltration and upregulation of SS-associated pro-inflammatory cytokines are observed, while at 20 wk, anti-nuclear antibodies and anti-M3 autoantibodies are detected in sera of NOD/ShiLtJ mice together with decreased amylase activity (141–144). Together, the progressive changes in SS-like phenotype suggest that immune cell subpopulations mediate distinct aspects of the NOD mouse model’s autoimmune features and mimics SS clinical presentation by exhibiting hypergammaglobulinemia and elevated levels of immunoglobulin-secreting B lymphocytes (7, 18, 19). Previous studies demonstrated that systemic treatment with AT-RvD1 partially decreases lymphocytic infiltration of SMG and restores saliva secretion (43, 44, 88, 94); however, to completely eliminate lymphocytic infiltration in the SS mouse models, it would be necessary to determine whether a combination of SPM completely abolishes lymphocytic infiltration and diminished saliva secretion. Should such a response be noted, it would be beneficial to elucidate their downstream signaling mechanisms. Therefore, the investigator should identify the temporal changes in SS-like pathological endpoints following systemic treatment with a range of SPM, quantify alternations in inflammatory cells and their SPM receptor expression and identify changes in the formation of hematopoietic stem cells and lymphoid progenitor cells. In SS, epithelial cells adjacent to sites of inflammation display high levels of immunoactive proteins (145, 146), cell adhesion molecules (147–150) and proinflammatory mediators (25, 28, 151, 152), thus playing a role in regulation of immune cells. Given that SPM have been shown to reduce these events in a range of other tissues (153–157), contributions of SPM receptors to the autoimmune phenotype of SS mouse models should be investigated.
4 Methods to investigate SPM metabolism using mathematical models
Mathematical models such as those presented below may be used as supplementary methods to inform study design using the principal methods already presented (i.e., in vivo and in vitro studies), and in so doing the investigator can better focus their traditional lab work to better use resources (i.e., time and lab materials) and also reduce the suffering of animals (e.g., in vitro studies). These mathematical models using differential equations, virtual models of the systems to be studied, both in terms of the minimum effective treatment dose of a given drug as well as its likely path and interactions within the body. The payoff on such methods should be apparent – they provide an educated guess as to a starting point for dose-response studies, thereby resulting in a better chance of getting a desired result with fewer trials as compared to a literature review and/or trial and error alone. Likewise, the results derived from in vitro and in vivo studies subsequent to mathematical modeling can then be fed back into a revised and verified version of that model, based on these real-world inputs, to better inform future iterations of the investigative process. Recently, various types of mathematical models have been applied to the drug development process (158–162). Principal among them is pharmacokinetic (PK) modeling, which combines investigator-determined pharmacological parameters with the biological and physiological parameters derived from general experience and/or literature review to achieve a well-informed virtual mechanistic representation of that drug, thereby allowing for a priori simulation of expected concentration-time profiles (158–160). Furthermore, PK modeling enables the estimation of drug exposure, not only in plasma but also at the site of action, which may be difficult or impossible to measure experimentally (158–160). Given the utility of a well-validated PK model for estimating SPM concentrations in vivo, it is likewise necessary to produce a whole-body mouse PK model of SPM to be coupled with an earlier derived pharmacodynamics (PD) model. Such a PK/PD tandem allows the PD effects of SPM (e.g., salivary flow as well as local and systemic inflammation binding to simulated plasma and SG SPM concentrations) to be quantified. The PK/PD model can then be validated by administering the target dose of SPM to a healthy mouse, sacrificing it and measuring its concentration within specified organs using liquid chromatography-tandem mass spectrometry (LC-MS/MS) as well as ELISA analyses (109, 119), thus establishing baseline values for future SPM studies (Figure 2). The target administration amount can then be introduced systemically at multiple time points, after which the degree of inflammatory resolution in the SG and the mechanisms by which this change has occurred should be determined. The validated mouse PK/PD effects obtained in mice can be extrapolated to humans by replacing the model input parameters for the mouse species with those of humans on the basis of a previously developed whole-body PK model of AT-RvD1 and extrapolation protocol for humans (163, 164), a process of model development that will need to be replicated for exploration of future SPM to be identified and explored in this context. However, the PK model has some limitations. First, the physicochemical and biological parameters used to build the mouse models are obtained either from the literature or estimated using algorithms and need to be confirmed experimentally. Second, in PK human models for RvD1 and AT-RvD1 data must be verified using results from prospective clinical studies. Finally, these models do not consider endogenous RvD1 which may influence the PK of exogenous administrated RvD1 and AT-RvD1.
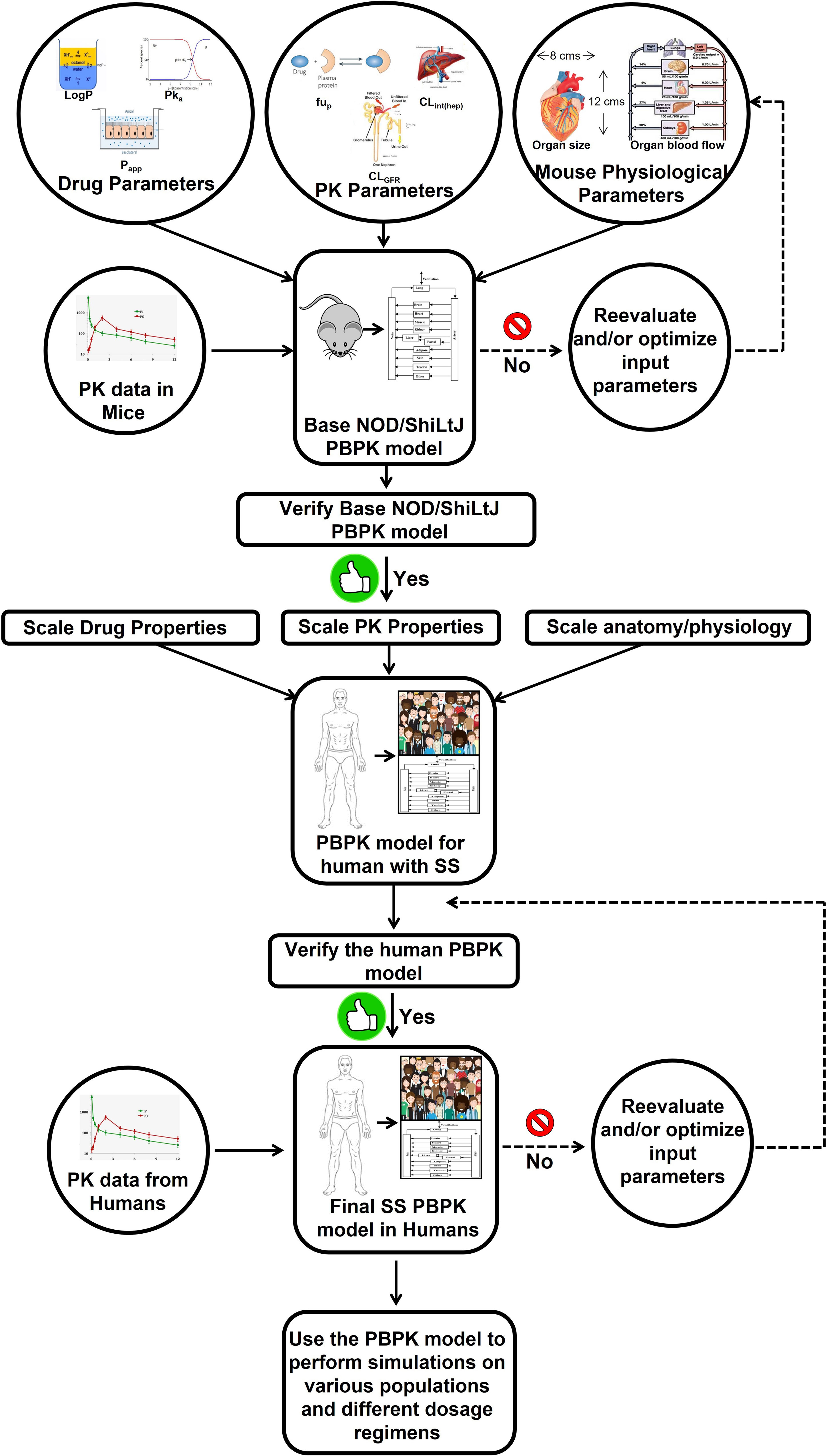
Figure 2 Workflow of PBPK model development for the specialized pro-resolving lipid mediator AT-RvD1. The PBPK model for human with Sjögren’s syndrome refers to the virtual population of the subjects whose anatomical physiological parameters are consistent with disease features. If the model-predicted data does not agree with the observed experimental data, a parameter identification and optimization will be performed using the default tools provided by the PK-Sim® software to identify the parameter responsible for the lack of accurate predicts and then its value will be optimized using the observed data until a goodness of fit is achieved between predicted versus observed data. Although AT-RvD1 is the subject of the figure, the same model can be applied to other specialized pro-resolving lipid mediators. This image was adapted from (163). AT-RvD1: aspirin-triggered resolvin D1; PBPK, physiologically based pharmacokinetic; PK, pharmacokinetics; SS, Sjögren’s syndrome.
5 Discussion
This review has been presented with the aim of promoting both the rationale and means to study SPM as a potential treatment for SS. The need to do so is based on the current lack of effective treatments and the methods for doing so, as detailed above, are numerous and ever expanding. Regarding in vitro studies, results should be interpreted with caution as SPM production may be different depending on growth and differentiation stages within the SG as well as the various culture conditions. Moreover, if the researcher finds SPM dysregulation to occur in human tissue cultures, studies should be confirmed multiple times and correlated with clinical data. As for in vivo models, previous studies indicated that endotoxin challenge temporally regulates lipid mediator production in human serum (50), where pro-inflammatory eicosanoids concentrations peak after 8 h and similar results could occur in SG. Also, SPM such as resolvins and lipoxins initially decreased after 2 h but are then elevated at 24 h. Therefore, a similar timing of SPM production may occur in in vivo models of inflammatory resolution (i.e., wound healing (138–140) and innate immunity (165) models); however, dysregulation of SPM production in SS mouse model may occur during disease progression, as these mice do not resolve inflammation (1, 44, 46, 48, 166). With respect to virtual mathematical models (158–162), such techniques can provide a reasonable starting point for required total dosage to be administered while also eliminating the need for excessive animal usage, as would be the case should this estimate be derived by trial and error alone; however, a limitation of mathematical models is that the physicochemical and biological parameters used to build the RvD1 and AT-RvD1 PK/PD models are obtained either from data on from data, from the literature or estimated using algorithms (163, 164). Therefore, it is critical to keep in mind that all such estimates must be confirmed and thus validated using models in vivo. To expand on this work, future investigations may involve determining if activation of SPM receptors in SG epithelium and vascular SG endothelium diminishes SS autoimmune phenotype, identifying mechanisms by which SPM receptors diminish SG lymphocytic infiltration in SS mouse models and testing whether SPM activation in B and T cells diminishes SS phenotype. Finally, it is worth emphasizing yet again that the SPM family is vast and work has only been performed in relation to the SG with a small fraction of the potential candidates. It would therefore appear warranted to extend the range of SPM to be explored by repeating already performed studies with new SPM candidates and related molecules while also stretching this work to make use of emerging experimental techniques and technologies.
Author contributions
HS, KN, DG, and VY wrote the manuscript and prepared figures. OB planned, wrote and overall supervised the preparation of the manuscript. All authors contributed to the article and approved the submitted version.
Funding
This study is supported by the National Institutes of Health–National Institute of Dental and Craniofacial Research (grant R01DE022971and R01DE027884 to OJB).
Conflict of interest
The authors declare that the research was conducted in the absence of any commercial or financial relationships that could be construed as a potential conflict of interest.
Publisher’s note
All claims expressed in this article are solely those of the authors and do not necessarily represent those of their affiliated organizations, or those of the publisher, the editors and the reviewers. Any product that may be evaluated in this article, or claim that may be made by its manufacturer, is not guaranteed or endorsed by the publisher.
References
1. Luciano N, Valentini V, Calabro A, Elefante E, Vitale A, Baldini C, et al. One year in review 2015: Sjogren’s syndrome. Clin Exp Rheumatol (2015) 33(2):259–71.
2. Bredberg A, Henriksson G, Larsson A, Manthorpe R, Sallmyr A. Sjogren’s syndrome and the danger model. Rheumatol (Oxford) (2005) 44(8):965–70. doi: 10.1093/rheumatology/keh647
3. Delaleu N, Jonsson MV, Appel S, Jonsson R. New concepts in the pathogenesis of sjogren’s syndrome. Rheumatic Dis Clinics North America (2008) 34(4):833–45. doi: 10.1016/j.rdc.2008.08.004
4. Delaleu N, Jonsson R, Koller MM. Sjogren’s syndrome. Eur J Oral Sci (2005) 113(2):101–13. doi: 10.1111/j.1600-0722.2004.00183.x
5. Fox RI, Stern M. Sjogren’s syndrome: Mechanisms of pathogenesis involve interaction of immune and neurosecretory systems. Scand J Rheumatol Suppl (2002) 116):3–13. doi: 10.1080/030097402317474874
6. Jonsson R, Moen K, Vestrheim D, Szodoray P. Current issues in sjogren’s syndrome. Oral Dis (2002) 8(3):130–40. doi: 10.1034/j.1601-0825.2002.02846.x
7. Mavragani CP, Moutsopoulos HM. Sjogren’s syndrome. Annu Rev Pathol (2014) 9:273–85. doi: 10.1146/annurev-pathol-012513-104728
8. Tan Z, Wang L, Li X. Composition and regulation of the immune microenvironment of salivary gland in sjögren’s syndrome. Front Immunol (2022) 13:967304. doi: 10.3389/fimmu.2022.967304
9. Choudhry HS, Hosseini S, Choudhry HS, Fatahzadeh M, Khianey R, Dastjerdi MH. Updates in diagnostics, treatments, and correlations between oral and ocular manifestations of sjogren’s syndrome. Ocul Surf (2022) 26:75–87. doi: 10.1016/j.jtos.2022.08.001
10. Gaubitz M. Epidemiology of connective tissue disorders. Rheumatology (2006) 45 Suppl 3:iii3–4. doi: 10.1093/rheumatology/kel282
11. Baldini C, Talarico R, Tzioufas AG, Bombardieri S. Classification criteria for sjogren’s syndrome: A critical review. J Autoimmun (2012) 39(1-2):9–14. doi: 10.1016/j.jaut.2011.12.006
12. Nocturne G, Mariette X. Advances in understanding the pathogenesis of primary sjogren’s syndrome. Nat Rev Rheumatol (2013) 9(9):544–56. doi: 10.1038/nrrheum.2013.110
13. Ferro F, Vagelli R, Bruni C, Cafaro G, Marcucci E, Bartoloni E, et al. One year in review 2016: Sjogren’s syndrome. Clin Exp Rheumatol (2016) 34(2):161–71.
14. Cha S, Brayer J, Gao J, Brown V, Killedar S, Yasunari U, et al. A dual role for interferon-gamma in the pathogenesis of sjogren’s syndrome-like autoimmune exocrinopathy in the nonobese diabetic mouse. Scand J Immunol (2004) 60(6):552–65. doi: 10.1111/j.0300-9475.2004.01508.x
15. Dost F, Farah CS. Stimulating the discussion on saliva substitutes: A clinical perspective. Aust Dent J (2013) 58(1):11–7. doi: 10.1111/adj.12023
16. Porter SR, Scully C, Hegarty AM. An update of the etiology and management of xerostomia. Oral Surg Oral Med Oral Pathol Oral Radiol Endod (2004) 97(1):28–46. doi: 10.1016/S1079210403005572
17. Silvestre FJ, Minguez MP, Sune-Negre JM. Clinical evaluation of a new artificial saliva in spray form for patients with dry mouth. Med Oral Patol Oral Cir Bucal (2009) 14(1):E8–E11.
18. Pringle S, Verstappen GM, van Ginkel MS, Nakshbandi U, Girigoria Z, Bootsma H, et al. Lymphoepithelial lesions in the salivary glands of primary sjögren’s syndrome patients: The perfect storm? Clin Exp Rheumatol (2022) 40(12):2434–42. doi: 10.55563/clinexprheumatol/06an99
19. Verstappen GM, Pringle S, Bootsma H, Kroese FGM. Epithelial-immune cell interplay in primary sjögren syndrome salivary gland pathogenesis. Nat Rev Rheumatol (2021) 17(6):333–48. doi: 10.1038/s41584-021-00605-2
20. Safonova TN, Zaitseva GV, Loginov VI, Burdenniy AM, Lukina SS. [Association of polymorphisms of the Trim21 gene with the severity of dry keratoconjunctivitis in rheumatoid arthritis and sjogren’s disease]. Vestn Oftalmol (2019) 135(5. Vyp. 2):192–8. doi: 10.17116/oftalma2019135052192
21. Katsiougiannis S, Tenta R, Skopouli FN. Autoimmune epithelitis (Sjogren’s syndrome); the impact of metabolic status of glandular epithelial cells on auto-immunogenicity. J Autoimmun (2019) 104:102335. doi: 10.1016/j.jaut.2019.102335
22. Karagianni P, Tzioufas AG. Epigenetic perspectives on systemic autoimmune disease. J Autoimmun (2019) 104:102315. doi: 10.1016/j.jaut.2019.102315
23. Liu X, Xing H, Gao W, Yu D, Zhao Y, Shi X, et al. A functional variant in the Oas1 gene is associated with sjogren’s syndrome complicated with hbv infection. Sci Rep (2017) 7(1):17571. doi: 10.1038/s41598-017-17931-9
24. Teos LY, Alevizos I. Genetics of sjogren’s syndrome. Clin Immunol (2017) 182:41–7. doi: 10.1016/j.clim.2017.04.018
25. Szodoray P, Alex P, Brun JG, Centola M, Jonsson R. Circulating cytokines in primary sjogren’s syndrome determined by a multiplex cytokine array system. Scand J Immunol (2004) 59(6):592–9. doi: 10.1111/j.0300-9475.2004.01432.x
26. Garcic-Carrasco M, Font J, Filella X, Cervera R, Ramos-Casals M, Siso A, et al. Circulating levels of Th1/Th2 cytokines in patients with primary sjogren’s syndrome: Correlation with clinical and immunological features. Clin Exp Rheumatol (2001) 19(4):411–5.
27. Oxholm P, Daniels TE, Bendtzen K. Cytokine expression in labial salivary glands from patients with primary sjogren’s syndrome. Autoimmunity (1992) 12(3):185–91. doi: 10.3109/08916939209148458
28. Fox RI, Kang HI, Ando D, Abrams J, Pisa E. Cytokine mrna expression in salivary gland biopsies of sjogren’s syndrome. J Immunol (1994) 152(11):5532–9.
29. Padern G, Duflos C, Ferreira R, Assou S, Guilpain P, Maria ATJ, et al. Identification of a novel serum proteomic signature for primary sjögren’s syndrome. Front Immunol (2021) 12:631539. doi: 10.3389/fimmu.2021.631539
30. Park GT, Kwon YW, Lee TW, Kwon SG, Ko HC, Kim MB, et al. Formyl peptide receptor 2 activation ameliorates dermal fibrosis and inflammation in bleomycin-induced scleroderma. Front Immunol (2019) 10:2095. doi: 10.3389/fimmu.2019.02095
31. Norris PC, Libreros S, Serhan CN. Resolution metabolomes activated by hypoxic environment. Sci Adv (2019) 5(10):eaax4895. doi: 10.1126/sciadv.aax4895
32. Atri C, Guerfali FZ, Laouini D. Role of human macrophage polarization in inflammation during infectious diseases. Int J Mol Sci (2018) 19(6):1801. doi: 10.3390/ijms19061801
33. Wang CS, Baker OJ. The G-Protein-Coupled receptor Alx/Fpr2 regulates adaptive immune responses in mouse submandibular glands. Am J Pathol (2018) 188(7):1555–62. doi: 10.1016/j.ajpath.2018.04.003
34. Munoz LE, Janko C, Schulze C, Schorn C, Sarter K, Schett G, et al. Autoimmunity and chronic inflammation - two clearance-related steps in the etiopathogenesis of sle. Autoimmun Rev (2010) 10(1):38–42. doi: 10.1016/j.autrev.2010.08.015
35. Saito I, Terauchi K, Shimuta M, Nishiimura S, Yoshino K, Takeuchi T, et al. Expression of cell adhesion molecules in the salivary and lacrimal glands of sjogren’s syndrome. J Clin Lab Anal (1993) 7(3):180–7. doi: 10.1002/jcla.1860070309
36. Aziz KE, McCluskey PJ, Montanaro A, Wakefield D. Vascular endothelium and lymphocyte adhesion molecules in minor salivary glands of patients with sjogren’s syndrome. J Clin Lab Immunol (1992) 37(1):39–49.
37. Chinthamani S, Odusanwo O, Mondal N, Nelson J, Neelamegham S, Baker OJ. Lipoxin A4 inhibits immune cell binding to salivary epithelium and vascular endothelium. Am J Physiol Cell Physiol (2012) 302(7):C968–78. doi: 10.1152/ajpcell.00259.2011
38. Mueller CG, Nayar S, Gardner D, Barone F. Cellular and vascular components of tertiary lymphoid structures. Methods Mol Biol (2018) 1845:17–30. doi: 10.1007/978-1-4939-8709-2_2
39. Reksten TR, Lessard CJ, Sivils KL. Genetics in sjogren syndrome. Rheumatic Dis Clinics North America (2016) 42(3):435–47. doi: 10.1016/j.rdc.2016.03.003
40. Hosoda C, Hosaka Y, Ryu K, Kinoshita A, Saito K, Kuwano K. Pleuritis associated with primary sjogren syndrome. Respirol Case Rep (2018) 6(2):e00285. doi: 10.1002/rcr2.285
41. Scofield RH. Vasculitis in sjogren’s syndrome. Curr Rheumatol Rep (2011) 13(6):482–8. doi: 10.1007/s11926-011-0207-5
42. Wang CS, Wee Y, Yang CH, Melvin JE, Baker OJ. Alx/Fpr2 modulates anti-inflammatory responses in mouse submandibular gland. Sci Rep (2016) 6:24244. doi: 10.1038/srep24244
43. Dean S, Wang CS, Nam K, Maruyama CL, Trump BG, Baker OJ. Aspirin triggered resolvin D1 reduces inflammation and restores saliva secretion in a sjogren’s syndrome mouse model. Rheumatol (Oxford) (2019) 58(7):1285–92. doi: 10.1093/rheumatology/kez072
44. Wang CS, Maruyama CL, Easley JT, Trump BG, Baker OJ. At-Rvd1 promotes resolution of inflammation in Nod/Shiltj mice. Sci Rep (2017) 7:45525. doi: 10.1038/srep45525
45. Kaklamanos A, Goules AV, Tzioufas AG. Experimental models of sjögren’s syndrome: Differences and similarities with human disease. Clin Exp Rheumatol (2022). doi: 10.55563/clinexprheumatol/d4cx78
46. Garcia-Carrasco M, Fuentes-Alexandro S, Escarcega RO, Salgado G, Riebeling C, Cervera R. Pathophysiology of sjogren’s syndrome. Arch Med Res (2006) 37(8):921–32. doi: 10.1016/j.arcmed.2006.08.002
47. Ramos-Casals M, Maria A, Suarez-Almazor ME, Lambotte O, Fisher BA, Hernandez-Molina G, et al. Sicca/Sjogren’s syndrome triggered by pd-1/Pd-L1 checkpoint inhibitors. data from the international immunocancer registry (Icir). Clin Exp Rheumatol (2019) 37 Suppl 118(3):114–22.
49. Mavragani CP, Moutsopoulos HM. Sjogren’s syndrome: Old and new therapeutic targets. J Autoimmun (2019) 110:102364. doi: 10.1016/j.jaut.2019.102364
50. Norris PC, Skulas-Ray AC, Riley I, Richter CK, Kris-Etherton PM, Jensen GL, et al. Identification of specialized pro-resolving mediator clusters from healthy adults after intravenous low-dose endotoxin and omega-3 supplementation: A methodological validation. Sci Rep (2018) 8(1):18050. doi: 10.1038/s41598-018-36679-4
51. Motwani MP, Colas RA, George MJ, Flint JD, Dalli J, Richard-Loendt A, et al. Pro-resolving mediators promote resolution in a human skin model of uv-killed escherichia coli-driven acute inflammation. JCI Insight (2018) 3(6):e94463. doi: 10.1172/jci.insight.94463
52. Hansen TV, Vik A, Serhan CN. The protectin family of specialized pro-resolving mediators: Potent immunoresolvents enabling innovative approaches to target obesity and diabetes. Front Pharmacol (2018) 9:1582. doi: 10.3389/fphar.2018.01582
53. Serhan CN, Levy BD. Resolvins in inflammation: Emergence of the pro-resolving superfamily of mediators. J Clin Invest (2018) 128(7):2657–69. doi: 10.1172/JCI97943
54. Kooij G, Derada Troletti C, Leuti A, Norris PC, Riley I, Albanese M, et al. Specialized pro-resolving lipid mediators are differentially altered in peripheral blood of patients with multiple sclerosis and attenuate monocyte and blood-brain barrier dysfunction. Haematologica (2019) 105(8):2056–70. doi: 10.3324/haematol.2019.219519
55. Chiang N, Serhan CN. Structural elucidation and physiologic functions of specialized pro-resolving mediators and their receptors. Mol Aspects Med (2017) 58:114–29. doi: 10.1016/j.mam.2017.03.005
56. Recchiuti A, Codagnone M, Pierdomenico AM, Rossi C, Mari VC, Cianci E, et al. Immunoresolving actions of oral resolvin D1 include selective regulation of the transcription machinery in resolution-phase mouse macrophages. FASEB J (2014) 28(7):3090–102. doi: 10.1096/fj.13-248393
57. Serhan CN. Systems approach with inflammatory exudates uncovers novel anti-inflammatory and pro-resolving mediators. Prostaglandins Leukot Essent Fatty Acids (2008) 79(3-5):157–63. doi: 10.1016/j.plefa.2008.09.012
58. Serhan CN. Pro-resolving lipid mediators are leads for resolution physiology. Nature (2014) 510(7503):92–101. doi: 10.1038/nature13479
59. Serhan CN. Discovery of specialized pro-resolving mediators marks the dawn of resolution physiology and pharmacology. Mol Aspects Med (2017) 58:1–11. doi: 10.1016/j.mam.2017.03.001
60. Serhan CN. Treating inflammation and infection in the 21st century: New hints from decoding resolution mediators and mechanisms. FASEB J (2017) 31(4):1273–88. doi: 10.1096/fj.201601222R
61. Serhan CN, Chiang N. Endogenous pro-resolving and anti-inflammatory lipid mediators: A new pharmacologic genus. Br J Pharmacol (2008) 153 Suppl 1:S200–15. doi: 10.1038/sj.bjp.0707489
62. Julliard WA, Myo YPA, Perelas A, Jackson PD, Thatcher TH, Sime PJ. Specialized pro-resolving mediators as modulators of immune responses. Semin Immunol (2022) 59:101605. doi: 10.1016/j.smim.2022.101605
63. Sun YP, Oh SF, Uddin J, Yang R, Gotlinger K, Campbell E, et al. Resolvin D1 and its aspirin-triggered 17r epimer. stereochemical assignments, anti-inflammatory properties, and enzymatic inactivation. J Biol Chem (2007) 282(13):9323–34. doi: 10.1074/jbc.M609212200
64. Pirault J, Back M. Lipoxin and resolvin receptors transducing the resolution of inflammation in cardiovascular disease. Front Pharmacol (2018) 9:1273. doi: 10.3389/fphar.2018.01273
65. Dyall SC, Balas L, Bazan NG, Brenna JT, Chiang N, da Costa Souza F, et al. Polyunsaturated fatty acids and fatty acid-derived lipid mediators: Recent advances in the understanding of their biosynthesis, structures, and functions. Prog Lipid Res (2022) 86:101165. doi: 10.1016/j.plipres.2022.101165
66. Park J, Langmead CJ, Riddy DM. New advances in targeting the resolution of inflammation: Implications for specialized pro-resolving mediator gpcr drug discovery. ACS Pharmacol Trans Sci (2020) 3(1):88–106. doi: 10.1021/acsptsci.9b00075
67. Keinan D, Leigh NJ, Nelson JW, De Oleo L, Baker OJ. Understanding resolvin signaling pathways to improve oral health. Int J Mol Sci (2013) 14(3):5501–18. doi: 10.3390/ijms14035501
68. Chen Y, Wang J, Nie R, Zhou S. Endogenous pro-resolving and anti-inflammatory lipid mediators: The new hope of atherosclerotic diseases. Med Hypotheses (2008) 71(2):237–40. doi: 10.1016/j.mehy.2008.03.026
69. Fredman G, Serhan CN. Specialized pro-resolving mediators: Wiring the circuitry of effector immune and tissue homeostasis. Endodontic Topics (2012) 24(1):39–58. doi: 10.1111/etp.12010
70. Haas-Stapleton EJ, Lu Y, Hong S, Arita M, Favoreto S, Nigam S, et al. Candida albicans modulates host defense by biosynthesizing the pro-resolving mediator resolvin E1. PloS One (2007) 2(12):e1316. doi: 10.1371/journal.pone.0001316
71. Jin Y, Arita M, Zhang Q, Saban DR, Chauhan SK, Chiang N, et al. Anti-angiogenesis effect of the novel anti-inflammatory and pro-resolving lipid mediators. Invest Ophthalmol Vis Sci (2009) 50(10):4743–52. doi: 10.1167/iovs.08-2462
72. Norris PC, Arnardottir H, Sanger JM, Fichtner D, Keyes GS, Serhan CN. Resolvin D3 multi-level proresolving actions are host protective during infection. Prostaglandins Leukotrienes Essential Fatty Acids (PLEFA) (2016) 138:81–9. doi: 10.1016/j.plefa.2016.01.001
73. Ramon S, Gao F, Serhan CN, Phipps RP. Specialized proresolving mediators enhance human b cell differentiation to antibody-secreting cells. J Immunol (2012) 189(2):1036–42. doi: 10.4049/jimmunol.1103483
74. Benabdoune H, Rondon EP, Shi Q, Fernandes J, Ranger P, Fahmi H, et al. The role of resolvin D1 in the regulation of inflammatory and catabolic mediators in osteoarthritis. Inflammation Res (2016) 65(8):635–45. doi: 10.1007/s00011-016-0946-x
75. Eickmeier O, Seki H, Haworth O, Hilberath JN, Gao F, Uddin M, et al. Aspirin-triggered resolvin D1 reduces mucosal inflammation and promotes resolution in a murine model of acute lung injury. Mucosal Immunol (2013) 6(2):256–66. doi: 10.1038/mi.2012.66
76. Xie W, Wang H, Wang L, Yao C, Yuan R, Wu Q. Resolvin D1 reduces deterioration of tight junction proteins by upregulating ho-1 in lps-induced mice. Lab Invest (2013) 93(9):991–1000. doi: 10.1038/labinvest.2013.80
77. Zhang X, Wang T, Gui P, Yao C, Sun W, Wang L, et al. Resolvin D1 reverts lipopolysaccharide-induced tj proteins disruption and the increase of cellular permeability by regulating ikappabalpha signaling in human vascular endothelial cells. Oxid Med Cell Longev (2013) 2013:185715. doi: 10.1155/2013/185715
78. Sok MCP, Tria MC, Olingy CE, San Emeterio CL, Botchwey EA. Aspirin-triggered resolvin D1-modified materials promote the accumulation of pro-regenerative immune cell subsets and enhance vascular remodeling. Acta Biomater (2017) 53:109–22. doi: 10.1016/j.actbio.2017.02.020
79. Chiang N, Riley IR, Dalli J, Rodriguez AR, Spur BW, Serhan CN. New maresin conjugates in tissue regeneration pathway counters leukotriene D4-stimulated vascular responses. FASEB J (2018) 32(7):4043–52. doi: 10.1096/fj.201701493R
80. Zheng S, Wang Q, D’Souza V, Bartis D, Dancer R, Parekh D, et al. Resolvind1 stimulates epithelial wound repair and inhibits tgf-Beta-Induced emt whilst reducing fibroproliferation and collagen production. Lab Invest (2018) 98(1):130–40. doi: 10.1038/labinvest.2017.114
81. Osorio Parra MM, Elangovan S, Lee CT. Specialized pro-resolving lipid mediators in experimental periodontitis: A systematic review. Oral Dis (2019) 25(5):1265–76. doi: 10.1111/odi.12979
82. Duvall MG, Levy BD. Dha- and epa-derived resolvins, protectins, and maresins in airway inflammation. Eur J Pharmacol (2015) 785:144–55. doi: 10.1016/j.ejphar.2015.11.001
83. Elajami TK, Colas RA, Dalli J, Chiang N, Serhan CN, Welty FK. Specialized proresolving lipid mediators in patients with coronary artery disease and their potential for clot remodeling. FASEB J (2016) 30(8):2792–801. doi: 10.1096/fj.201500155R
84. Hansen TV, Dalli J, Serhan CN. The novel lipid mediator Pd1n-3 dpa: An overview of the structural elucidation, synthesis, biosynthesis and bioactions. Prostaglandins Other Lipid Mediat (2017) 133:103–10. doi: 10.1016/j.prostaglandins.2017.06.003
85. Ji RR, Xu ZZ, Strichartz G, Serhan CN. Emerging roles of resolvins in the resolution of inflammation and pain. Trends Neurosci (2011) 34(11):599–609. doi: 10.1016/j.tins.2011.08.005
86. Orr SK, Butler KL, Hayden D, Tompkins RG, Serhan CN, Irimia D. Gene expression of proresolving lipid mediator pathways is associated with clinical outcomes in trauma patients. Crit Care Med (2015) 43(12):2642–50. doi: 10.1097/CCM.0000000000001312
87. Serhan CN. Controlling the resolution of acute inflammation: A new genus of dual anti-inflammatory and proresolving mediators. J Periodontol (2008) 79(8 Suppl):1520–6. doi: 10.1902/jop.2008.080231
88. Easley JT, Nelson JW, Mellas RE, Sommakia S, Wu C, Trump B, et al. Aspirin-triggered resolvin D1 versus dexamethasone in the treatment of sjogren’s syndrome-like Nod/Shiltj mice - a pilot study. J Rheum Dis Treat (2015) 1(4):027. doi: 10.23937/2469-5726/1510027
89. Leigh NJ, Nelson JW, Mellas RE, Aguirre A, Baker OJ. Expression of resolvin D1 biosynthetic pathways in salivary epithelium. J Dent Res (2014) 93(3):300–5. doi: 10.1177/0022034513519108
90. Nelson JW, Leigh NJ, Mellas RE, McCall AD, Aguirre A, Baker OJ. Alx/Fpr2 receptor for Rvd1 is expressed and functional in salivary glands. Am J Physiol Cell Physiol (2014) 306(2):C178–85. doi: 10.1152/ajpcell.00284.2013
91. Odusanwo O, Chinthamani S, McCall A, Duffey ME, Baker OJ. Resolvin D1 prevents tnf-Alpha-Mediated disruption of salivary epithelial formation. Am J Physiol Cell Physiol (2012) 302(9):C1331–45. doi: 10.1152/ajpcell.00207.2011
92. Dos Santos HT, Nam K, Maslow F, Trump B, Baker OJ. Specialized pro-resolving receptors are expressed in salivary glands with sjögren’s syndrome. Ann Diagn Pathol (2022) 56:151865. doi: 10.1016/j.anndiagpath.2021.151865
93. Dos Santos HT, Nam K, Hunt JP, Buchmann LO, Monroe MM, Baker OJ. Spm receptor expression and localization in irradiated salivary glands. J Histochem Cytochem (2021) 69(8):523–34. doi: 10.1369/00221554211031678
94. Easley JT, Maruyama CL, Wang CS, Baker OJ. At-Rvd1 combined with dex is highly effective in treating tnf-Alpha-Mediated disruption of the salivary gland epithelium. Physiol Rep (2016) 4(19):e12990. doi: 10.14814/phy2.12990
95. Ariel A, Serhan CN. Resolvins and protectins in the termination program of acute inflammation. Trends Immunol (2007) 28(4):176–83. doi: 10.1016/j.it.2007.02.007
96. Arita M, Oh SF, Chonan T, Hong S, Elangovan S, Sun YP, et al. Metabolic inactivation of resolvin E1 and stabilization of its anti-inflammatory actions. J Biol Chem (2006) 281(32):22847–54. doi: 10.1074/jbc.M603766200
97. Bannenberg GL, Chiang N, Ariel A, Arita M, Tjonahen E, Gotlinger KH, et al. Molecular circuits of resolution: Formation and actions of resolvins and protectins. J Immunol (2005) 174(7):4345–55. doi: 10.4049/jimmunol.174.7.4345
98. Chiurchiu V, Leuti A, Dalli J, Jacobsson A, Battistini L, Maccarrone M, et al. Proresolving lipid mediators resolvin D1, resolvin D2, and maresin 1 are critical in modulating T cell responses. Sci Transl Med (2016) 8(353):353ra111. doi: 10.1126/scitranslmed.aaf7483
99. Dalli J, Chiang N, Serhan CN. Elucidation of novel 13-series resolvins that increase with atorvastatin and clear infections. Nat Med (2015) 21(9):1071–5. doi: 10.1038/nm.3911
100. Dalli J, Winkler Jeremy W, Colas Romain A, Arnardottir H, Cheng C-Yee C, Chiang N, et al. Resolvin D3 and aspirin-triggered resolvin D3 are potent immunoresolvents. Chem Biol (2013) 20(2):188–201. doi: 10.1016/j.chembiol.2012.11.010
101. Duffield JS, Hong S, Vaidya VS, Lu Y, Fredman G, Serhan CN, et al. Resolvin d series and protectin D1 mitigate acute kidney injury. J Immunol (2006) 177(9):5902–11. doi: 10.4049/jimmunol.177.9.5902
102. Masoodi M, Mir AA, Petasis NA, Serhan CN, Nicolaou A. Simultaneous lipidomic analysis of three families of bioactive lipid mediators leukotrienes, resolvins, protectins and related hydroxy-fatty acids by liquid Chromatography/Electrospray ionisation tandem mass spectrometry. Rapid Commun Mass Spectrom (2008) 22(2):75–83. doi: 10.1002/rcm.3331
103. Spite M, Claria J, Serhan CN. Resolvins, specialized proresolving lipid mediators, and their potential roles in metabolic diseases. Cell Metab (2014) 19(1):21–36. doi: 10.1016/j.cmet.2013.10.006
104. Sulciner ML, Serhan CN, Gilligan MM, Mudge DK, Chang J, Gartung A, et al. Resolvins suppress tumor growth and enhance cancer therapy. J Exp Med (2018) 215(1):115–40. doi: 10.1084/jem.20170681
105. Yacoubian S, Serhan CN. New endogenous anti-inflammatory and proresolving lipid mediators: Implications for rheumatic diseases. Nat Clin Pract Rheumatol (2007) 3(10):570–9. doi: 10.1038/ncprheum0616
106. Arita M, Serhan CN. Novel chemical mediators in the resolution of inflammation. Tanpakushitsu Kakusan Koso (2007) 52(4):348–54.
107. Buckley CD, Gilroy DW, Serhan CN, Stockinger B, Tak PP. The resolution of inflammation. Nat Rev Immunol (2013) 13(1):59–66. doi: 10.1038/nri3362
108. Norling LV, Ly L, Dalli J. Resolving inflammation by using nutrition therapy: Roles for specialized proresolving mediators. Curr Opin Clin Nutr Metab Care (2017) 20(2):145–52. doi: 10.1097/MCO.0000000000000353
109. Sommakia S, Baker OJ. Regulation of inflammation by lipid mediators in oral diseases. Oral Dis (2016) 23(5):576–97. doi: 10.1111/odi.12544
110. Serhan CN, Chiang N, Dalli J. The resolution code of acute inflammation: Novel pro-resolving lipid mediators in resolution. Semin Immunol (2015) 27(3):200–15. doi: 10.1016/j.smim.2015.03.004
111. Zhu M, Wang X, Hjorth E, Colas RA, Schroeder L, Granholm AC, et al. Pro-resolving lipid mediators improve neuronal survival and increase Abeta42 phagocytosis. Mol Neurobiol (2016) 53(4):2733–49. doi: 10.1007/s12035-015-9544-0
112. Orr SK, Colas RA, Dalli J, Chiang N, Serhan CN. Proresolving actions of a new resolvin D1 analog mimetic qualifies as an immunoresolvent. Am J Physiol - Lung Cell Mol Physiol (2015) 308(9):L904–L11. doi: 10.1152/ajplung.00370.2014
113. Serhan CN, Chiang N, Dalli J. New pro-resolving n-3 mediators bridge resolution of infectious inflammation to tissue regeneration. Mol Aspects Med (2017) 64:1–17. doi: 10.1016/j.mam.2017.08.002
114. Baker OJ, Camden JM, Redman RS, Jones JE, Seye CI, Erb L, et al. Proinflammatory cytokines tumor necrosis factor-alpha and interferon-gamma alter tight junction structure and function in the rat parotid gland par-C10 cell line. Am J Physiol Cell Physiol (2008) 295(5):C1191–201. doi: 10.1152/ajpcell.00144.2008
115. Baker OJ, Schulz DJ, Camden JM, Liao Z, Peterson TS, Seye CI, et al. Rat parotid gland cell differentiation in three-dimensional culture. Tissue Eng Part C Methods (2010) 16(5):1135–44. doi: 10.1089/ten.TEC.2009.0438
116. Nam K, Kim K, Dean SM, Brown CT, Davis RS, Okano T, et al. Using cell sheets to regenerate mouse submandibular glands. NPJ Regener Med (2019) 4:16. doi: 10.1038/s41536-019-0078-3
117. Zhang C, Barrios MP, Alani RM, Cabodi M, Wong JY. A microfluidic transwell to study chemotaxis. Exp Cell Res (2016) 342(2):159–65. doi: 10.1016/j.yexcr.2016.03.010
118. Tamama K, Kawasaki H, Kerpedjieva SS, Guan J, Ganju RK, Sen CK. Differential roles of hypoxia inducible factor subunits in multipotential stromal cells under hypoxic condition. J Cell Biochem (2011) 112(3):804–17. doi: 10.1002/jcb.22961
119. Parashar KS, F Hardt M, Baker O. Sex mediated elevation of the specialized pro-resolving lipid mediator in a sjögren’s syndrome mouse model. FASEB J (2020) 34(6):7733–44. doi: 10.1096/fj.201902196R
120. Serhan CN, Hong S, Gronert K, Colgan SP, Devchand PR, Mirick G, et al. Resolvins: A family of bioactive products of omega-3 fatty acid transformation circuits initiated by aspirin treatment that counter proinflammation signals. J Exp Med (2002) 196(8):1025–37. doi: 10.1084/jem.20020760
121. Hong S, Gronert K, Devchand PR, Moussignac RL, Serhan CN. Novel docosatrienes and 17s-resolvins generated from docosahexaenoic acid in murine brain, human blood, and glial cells. autacoids in anti-inflammation. J Biol Chem (2003) 278(17):14677–87. doi: 10.1074/jbc.M300218200
122. Shikano M, Masuzawa Y, Yazawa K. Effect of docosahexaenoic acid on the generation of platelet-activating factor by eosinophilic leukemia cells, eol-1. J Immunol (1993) 150(8 Pt 1):3525–33.
123. Shikano M, Masuzawa Y, Yazawa K, Takayama K, Kudo I, Inoue K. Complete discrimination of docosahexaenoate from arachidonate by 85 kda cytosolic phospholipase A2 during the hydrolysis of diacyl- and alkenylacylglycerophosphoethanolamine. Biochim Biophys Acta (1994) 1212(2):211–6. doi: 10.1016/0005-2760(94)90255-0
124. Krishnamoorthy S, Recchiuti A, Chiang N, Yacoubian S, Lee CH, Yang R, et al. Resolvin D1 binds human phagocytes with evidence for proresolving receptors. Proc Natl Acad Sci U.S.A. (2010) 107(4):1660–5. doi: 10.1073/pnas.0907342107
125. Kwon Y. Immuno-resolving ability of resolvins, protectins, and maresins derived from omega-3 fatty acids in metabolic syndrome. Mol Nutr Food Res (2019) 64:e1900824. doi: 10.1002/mnfr.201900824
126. Kytikova O, Novgorodtseva T, Denisenko Y, Antonyuk M, Gvozdenko T. Pro-resolving lipid mediators in the pathophysiology of asthma. Medicina (Kaunas) (2019) 55(6):284. doi: 10.3390/medicina55060284
127. Satish M, Agrawal DK. Pro-resolving lipid mediators in the resolution of neointimal hyperplasia pathogenesis in atherosclerotic diseases. Expert Rev Cardiovasc Ther (2019) 17(3):177–84. doi: 10.1080/14779072.2019.1563483
128. Tungen JE, Gerstmann L, Vik A, De Matteis R, Colas RA, Dalli J, et al. Resolving inflammation: Synthesis, configurational assignment, and biological evaluations of Rvd1n-3 dpa. Chemistry (2019) 25(6):1476–80. doi: 10.1002/chem.201806029
129. Cha S, Nagashima H, Brown VB, Peck AB, Humphreys-Beher MG. Two nod idd-associated intervals contribute synergistically to the development of autoimmune exocrinopathy (Sjogren’s syndrome) on a healthy murine background. Arthritis Rheum (2002) 46(5):1390–8. doi: 10.1002/art.10258
130. Humphreys-Beher MG, Hu Y, Nakagawa Y, Wang P-L, Purushotham KR. Utilization of the nod mouse as an animal model for the study of secondary sjögren’s syndrome. Adv Exp Med Biol (1994) 350:631–6. doi: 10.1007/978-1-4615-2417-5_105
131. Humphreys-Beher MG, Yamachika S, Yamamoto H, Maeda N, Nakagawa Y, Peck AB, et al. Salivary gland changes in the nod mouse model for sjögren’s syndrome: Is there a non-immune genetic trigger? Eur J Morphol (1998) 36:247–51.
132. Nguyen C, Singson E, Kim JY, Cornelius JG, Attia R, Doyle ME, et al. Sjogren’s syndrome-like disease of C57bl/6.Nod-Aec1 Aec2 mice: Gender differences in keratoconjunctivitis sicca defined by a cross-over in the chromosome 3 Aec1 locus. Scand J Immunol (2006) 64(3):295–307. doi: 10.1111/j.1365-3083.2006.01828.x
133. Nguyen CQ, Kim H, Cornelius JG, Peck AB. Development of sjogren’s syndrome in nonobese diabetic-derived autoimmune-prone C57bl/6.Nod-Aec1aec2 mice is dependent on complement component-3. J Immunol (2007) 179(4):2318–29. doi: 10.4049/jimmunol.179.4.2318
134. Nguyen CQ, Sharma A, Lee BH, She JX, McIndoe RA, Peck AB. Differential gene expression in the salivary gland during development and onset of xerostomia in sjogren’s syndrome-like disease of the C57bl/6.Nod-Aec1aec2 mouse. Arthritis Res Ther (2009) 11(2):R56. doi: 10.1186/ar2676
135. Mahmoud TI, Wang J, Karnell JL, Wang Q, Wang S, Naiman B, et al. Autoimmune manifestations in aged mice arise from early-life immune dysregulation. Sci Transl Med (2016) 8(361):361ra137. doi: 10.1126/scitranslmed.aag0367
136. Kayes TD, Weisman GA, Camden JM, Woods LT, Bredehoeft C, Downey EF, et al. New murine model of early onset autoimmune thyroid Disease/Hypothyroidism and autoimmune exocrinopathy of the salivary gland. J Immunol (2016) 197(6):2119–30. doi: 10.4049/jimmunol.1600133
137. Voynova E, Mahmoud T, Woods LT, Weisman GA, Ettinger R, Braley-Mullen H. Requirement for Cd40/Cd40l interactions for development of autoimmunity differs depending on specific checkpoint and costimulatory pathways. Immunohorizons (2018) 2(1):54–66. doi: 10.4049/immunohorizons.1700069
138. Nam K, Maruyama CL, Wang CS, Trump BG, Lei P, Andreadis ST, et al. Laminin-111-Derived peptide conjugated fibrin hydrogel restores salivary gland function. PloS One (2017) 12(11):e0187069. doi: 10.1371/journal.pone.0187069
139. Nam K, Wang CS, Maruyama CLM, Lei P, Andreadis ST, Baker OJ. L1 peptide-conjugated fibrin hydrogels promote salivary gland regeneration. J Dent Res (2017) 96(7):798–806. doi: 10.1177/0022034517695496
140. Dos Santos HT, Nam K, Brown CT, Dean SM, Lewis S, Pfeifer CS, et al. Trimers conjugated to fibrin hydrogels promote salivary gland function. J Dent Res (2021) 100(3):268–75. doi: 10.1177/0022034520964784
141. Humphreys-Beher MG, Peck AB. New concepts for the development of autoimmune exocrinopathy derived from studies with the nod mouse model. Arch Oral Biol (1999) 44:S21–S5. doi: 10.1016/s0003-9969(99)00045-x
142. Robinson CP, Yamachika S, Bounous DI, Brayer J, Jonsson R, Holmdahl R, et al. A novel nod-derived murine model of primary sjögren’s syndrome. Arthritis Rheumatism (1998) 41(1):150–6. doi: 10.1002/1529-0131(199801)41:1<150::AID-ART18>3.0.CO;2-T
143. Yamano S, Atkinson JC, Baum BJ, Fox PC. Salivary gland cytokine expression in nod and normal Balb/C mice. Clin Immunol (1999) 92(3):265–75. doi: 10.1006/clim.1999.4759
144. Young EF, Hess PR, Arnold LW, Tisch R, Frelinger JA. Islet lymphocyte subsets in Male and female nod mice are qualitatively similar but quantitatively distinct. Autoimmunity (2009) 42(8):678–91. doi: 10.3109/08916930903213993
145. Gordon TP, Bolstad AI, Rischmueller M, Jonsson R, Waterman SA. Autoantibodies in primary sjogren’s syndrome: New insights into mechanisms of autoantibody diversification and disease pathogenesis. Autoimmunity (2001) 34(2):123–32. doi: 10.3109/08916930109001960
146. Halse A, Harley JB, Kroneld U, Jonsson R. Ro/Ss-a-Reactive b lymphocytes in salivary glands and peripheral blood of patients with sjogren’s syndrome. Clin Exp Immunol (1999) 115(1):203–7. doi: 10.1046/j.1365-2249.1999.00778.x
147. McMurray RW. Adhesion molecules in autoimmune disease. Semin Arthritis Rheum (1996) 25(4):215–33. doi: 10.1016/s0049-0172(96)80034-5
148. Nakabayashi T, Letterio JJ, Geiser AG, Kong L, Ogawa N, Zhao W, et al. Up-regulation of cytokine mrna, adhesion molecule proteins, and mhc class ii proteins in salivary glands of tgf-Beta1 knockout mice: Mhc class ii is a factor in the pathogenesis of tgf-Beta1 knockout mice. J Immunol (1997) 158(11):5527–35.
149. Sfikakis PP, Mavrikakis M. Adhesion and lymphocyte costimulatory molecules in systemic rheumatic diseases. Clin Rheumatol (1999) 18(4):317–27. doi: 10.1007/s100670050109
150. St Clair EW, Angellilo JC, Singer KH. Expression of cell-adhesion molecules in the salivary gland microenvironment of sjogren’s syndrome. Arthritis Rheum (1992) 35(1):62–6. doi: 10.1002/art.1780350110
151. Ohyama Y, Nakamura S, Matsuzaki G, Shinohara M, Hiroki A, Fujimura T, et al. Cytokine messenger rna expression in the labial salivary glands of patients with sjogren’s syndrome. Arthritis Rheum (1996) 39(8):1376–84. doi: 10.1002/art.1780390816
152. Tapinos NI, Polihronis M, Tzioufas AG, Moutsopoulos HM. Sjogren’s syndrome. autoimmune epithelitis. Adv Exp Med Biol (1999) 455:127–34.
153. Chatterjee A, Komshian S, Sansbury BE, Wu B, Mottola G, Chen M, et al. Biosynthesis of proresolving lipid mediators by vascular cells and tissues. FASEB J (2017) 31(8):3393–402. doi: 10.1096/fj.201700082R
154. Conte MS, Desai TA, Wu B, Schaller M, Werlin E. Pro-resolving lipid mediators in vascular disease. J Clin Invest (2018) 128(9):3727–35. doi: 10.1172/JCI97947
155. Fredman G, Spite M. Specialized pro-resolving mediators in cardiovascular diseases. Mol Aspects Med (2017) 58:65–71. doi: 10.1016/j.mam.2017.02.003
156. Wu B, Mottola G, Schaller M, Upchurch GR Jr., Conte MS. Resolution of vascular injury: Specialized lipid mediators and their evolving therapeutic implications. Mol Aspects Med (2017) 58:72–82. doi: 10.1016/j.mam.2017.07.005
157. Perez-Hernandez J, Chiurchiù V, Perruche S, You S. Regulation of T-cell immune responses by pro-resolving lipid mediators. Front Immunol (2021) 12:768133. doi: 10.3389/fimmu.2021.768133
158. Yellepeddi VK, Zhudeva MY, Movahedi F, Vo A, Phan J, Kirsh RD, et al. Biopharmaceutical characterization and oral efficacy of a new rapid acting antidepressant ro 25-6981. J Pharm Sci (2018) 107(9):2472–8. doi: 10.1016/j.xphs.2018.05.005
159. Constance JE, Campbell SC, Somani AA, Yellepeddi V, Owens KH, Sherwin CMT. Pharmacokinetics, pharmacodynamics and pharmacogenetics associated with nonsteroidal anti-inflammatory drugs and opioids in pediatric cancer patients. Expert Opin Drug Metab Toxicol (2017) 13(7):715–24. doi: 10.1080/17425255.2017.1329415
160. Yellepeddi V, Rower J, Liu X, Kumar S, Rashid J, Sherwin CMT. State-of-the-Art review on physiologically based pharmacokinetic modeling in pediatric drug development. Clin Pharmacokinet (2019) 58(1):1–13. doi: 10.1007/s40262-018-0677-y
161. Qiao Y, Wang Y, Chen Y, Luo K, Fan J. Mathematical modeling of shear-activated targeted nanoparticle drug delivery for the treatment of aortic diseases. Biomech Model Mechanobiol (2022) 21(1):221–30. doi: 10.1007/s10237-021-01530-9
162. Clarelli F, Liang J, Martinecz A, Heiland I, Abel Zur Wiesch P. Multi-scale modeling of drug binding kinetics to predict drug efficacy. Cell Mol Life Sci (2020) 77(3):381–94. doi: 10.1007/s00018-019-03376-y
163. Yellepeddi VK, Baker OJ. Predictive modeling of aspirin-triggered resolvin D1 pharmacokinetics for the study of sjögren’s syndrome. Clin Exp Dent Res (2020) 6(2):225–35. doi: 10.1002/cre2.260
164. Yellepeddi VK, Parashar K, Dean SM, Watt KM, Constance JE, Baker OJ. Predicting resolvin D1 pharmacokinetics in humans with physiologically-based pharmacokinetic modeling. Clin Transl Sci (2021) 14(2):683–91. doi: 10.1111/cts.12930
165. Wang YP. Aspirin-triggered lipoxin A4 attenuates lps-induced pro-inflammatory responses by inhibiting activation of nf-kappab and mapks in bv-2 microglial cells. J Neuroinflamm (2011) 8:95. doi: 10.1186/1742-2094-8-95
Keywords: resolvins, immunology, mathematical modeling, cultures, mice, saliva, salivary glands
Citation: dos Santos HT, Nam K, Gil D, Yellepeddi V and Baker OJ (2023) Current experimental methods to investigate the impact of specialized pro-resolving lipid mediators on Sjögren’s syndrome. Front. Immunol. 13:1094278. doi: 10.3389/fimmu.2022.1094278
Received: 09 November 2022; Accepted: 27 December 2022;
Published: 12 January 2023.
Edited by:
Hui Hua, Thorne HealthTech, United StatesReviewed by:
Harini Bagavant, Oklahoma Medical Research Foundation, United StatesChing-Shuen Wang, Taipei Medical University, Taiwan
Copyright © 2023 dos Santos, Nam, Gil, Yellepeddi and Baker. This is an open-access article distributed under the terms of the Creative Commons Attribution License (CC BY). The use, distribution or reproduction in other forums is permitted, provided the original author(s) and the copyright owner(s) are credited and that the original publication in this journal is cited, in accordance with accepted academic practice. No use, distribution or reproduction is permitted which does not comply with these terms.
*Correspondence: Olga J. Baker, bakero@health.missouri.edu