- 1Guangdong Provincial Key Laboratory of Tumor Interventional Diagnosis and Treatment, Zhuhai People’s Hospital (Zhuhai Hospital Affiliated with Jinan University), Zhuhai, China
- 2Department of Urology, Zhuhai People’s Hospital (Zhuhai Hospital Affiliated with Jinan University), Zhuhai, China
- 3Faculty of Health Sciences, University of Macau, Macau, Macau SAR, China
- 4Department of R&D, OriCell Therapeutics Co. Ltd, Pudong, Shanghai, China
- 5Health Management Center, Zhuhai People’s Hospital (Zhuhai Hospital Affiliated with Jinan University), Zhuhai, China
Epstein–Barr virus (EBV) was the first tumor virus in humans. Nasopharyngeal carcinoma (NPC) accounts for approximately 60% of the 200,000 new tumor cases caused by EBV infection worldwide each year. NPC has an insidious onset and is highly malignant, with more than 70% of patients having intermediate to advanced disease at the time of initial diagnosis, and is strongly implicated in epithelial cancers as well as malignant lymphoid and natural killer/T cell lymphomas. Over 90% of patients with confirmed undifferentiated NPC are infected with EBV. In recent decades, much progress has been made in understanding the molecular mechanisms of NPC and developing therapeutic approaches. Radiotherapy and chemotherapy are the main treatment options for NPC; however, they have a limited efficacy in patients with locally advanced or distant metastatic tumors. Tumor immunotherapy, including vaccination, adoptive cell therapy, and immune checkpoint blockade, represents a promising therapeutic approach for NPC. Significant breakthroughs have recently been made in the application of immunotherapy for patients with recurrent or metastatic NPC (RM-NPC), indicating a broad prospect for NPC immunotherapy. Here, we review important research findings regarding immunotherapy for NPC patients and provide insights for future research.
1. Introduction
Approximately 95% of the global population are EBV asymptomatic carriers (1, 2). EBV primarily infects epithelial and B cells. Infection with EBV may cause many human cancers, including malignant lymphoid and epithelial cancers such as NPC, primary pulmonary lymphoepithelioma-like carcinoma (PLELC), EBV-associated intrahepatic cholangiocarcinoma, and EBV-associated gastric carcinoma (EBVaGC) [ (3–7), Figure 1]. NPC and EBVaGC are the two most common EBV-associated epithelial malignancies that account for 80% of these tumors. Over 90% of patients with confirmed undifferentiated NPC are infected with EBV (2, 13, 14). According to the last two global cancer statistical surveys from the International Agency for Research on Cancer, in 2018 and 2020, there were 129,079 and 133,354 new NPC cases in the world, and 72,987 and 80,008 NPC-associated deaths worldwide, respectively (15, 16). NPC is relatively rare compared to other cancers, accounting for only 0.7% of total cancers diagnosed each year. The geographical distribution of NPC is uneven, as approximately 80% of patients are from China and Southeast Asia (17, 18). Although both gene and lifestyle affect NPC incidence, EBV infection is particularly closely related to NPC, making EBV a unique target for tumor immunotherapy.
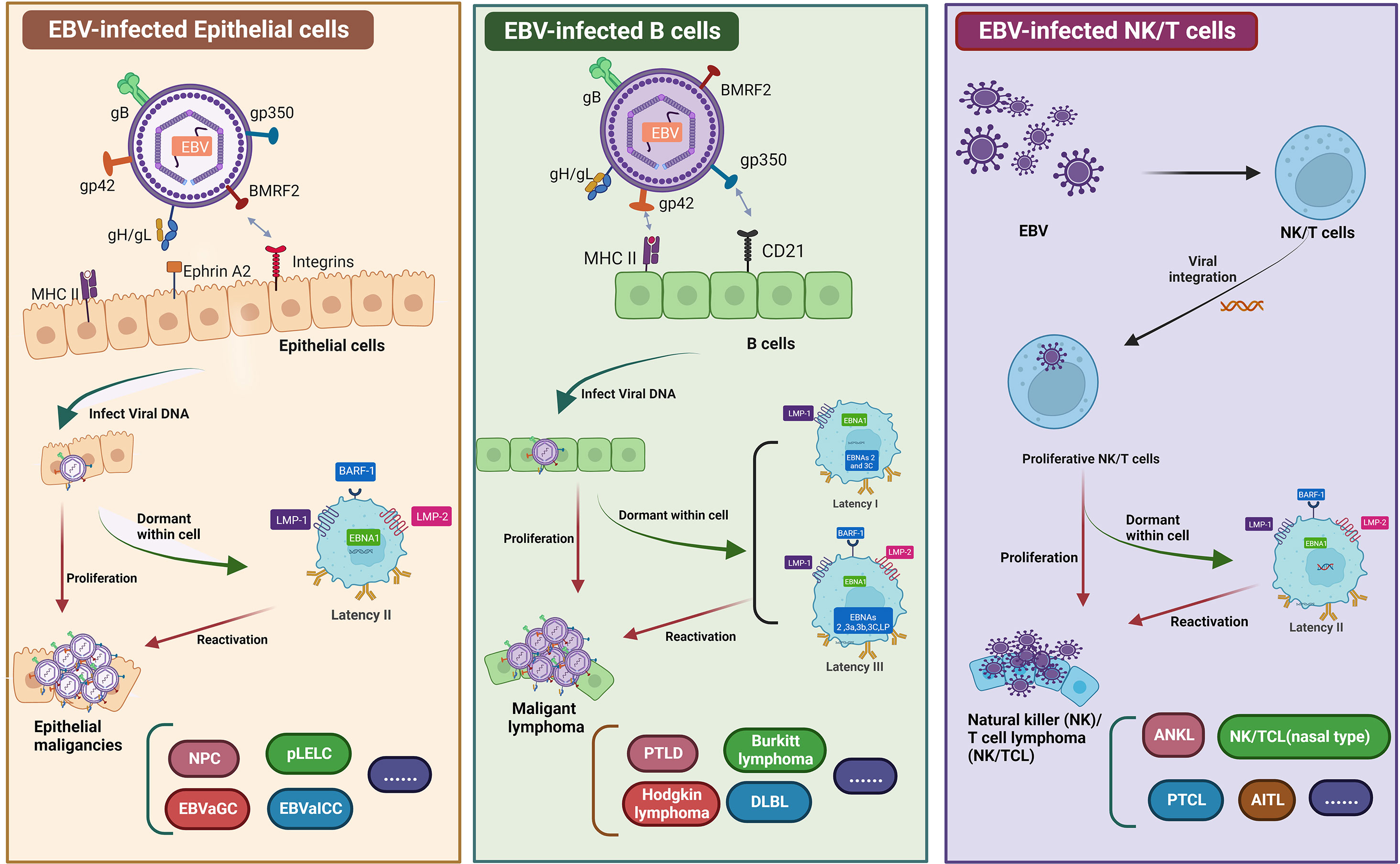
Figure 1 Graphical summary of EBV infected epithelial cells, B cells, and NK/T cell. EBV primarily infects epithelial and B cells. Infection with EBV may cause many human cancers, including epithelial cancers such as EBVaGC, NPC, EBVaICC, and pLELC. EBV-infected B cells can lead to malignant lymphomas, such as DLBL, PTLD, and BL. Similarly, EBV infects NK/T cells to form NK/T cell lymphomas. EBV establishes a persistent latent infection in malignant epithelial cells (known as latency I, latency II, and latency III). All EBV-associated epithelial cancers express a latency II program (8–12). Two therapeutic vaccines have been investigated for NPC, namely, peptide-based vaccines and DC vaccines. EBV requires a variety of envelope proteins to enter cells. The membrane proteins gp350, gH/gL, gB, and gp42 are required for B cell infection, whereas BMFR2, gH/gL, and gB are needed for epithelial cell infection. Another vaccine under study mainly uses LMP1, LMP2, EBNA1 and EBNA3 as target antigens to construct viral vaccines by single or multiple protein combinations. Figure was created with BioRender.
In accordance with the International Union Against Cancer staging system, NPC are graded according to several stages (I–IVB) (19–21). NPC treatment options vary depending on the stage. Conventional therapy for NPC includes surgery, radiotherapy, and chemotherapy (Figure 2). Owing to the deep tumor localization and complex anatomical structure of the tumor site, surgical options are limited. However, NPC is a highly radiosensitive and chemosensitive tumor; therefore, radiotherapy and chemotherapy alone or in combination are the primary treatments for patients with stage I/II NPC (22, 23). Although combined chemoradiotherapy has good prognosis (85–90% survival over 5 years), its efficacy is nonetheless limited, and approximately 8–10% of patients experience recurrence or metastasis (24–26). Platinum plus multidrug therapy is the preferred treatment for RM-NPC patients, however, the eventually developing resistance is a major barrier to successful treatment (27). Concurrent chemoradiotherapy is of great importance for improving treatment outcomes in locally advanced NPC but often leads to complications, such as xerostomia, trismus, and secondary tumors, which seriously impact patients’ quality of life (28–32). Concurrent chemoradiotherapy based on cisplatin is the standard treatment for patients with locally advanced NPC (33), and more than 50% of NPC patients are initially confirmed as advanced stage (34, 35). Therefore, the development of new strategies that not only prolong the disease-free survival of patients but also reduce treatment-related complications and adverse events is of critical clinical importance.
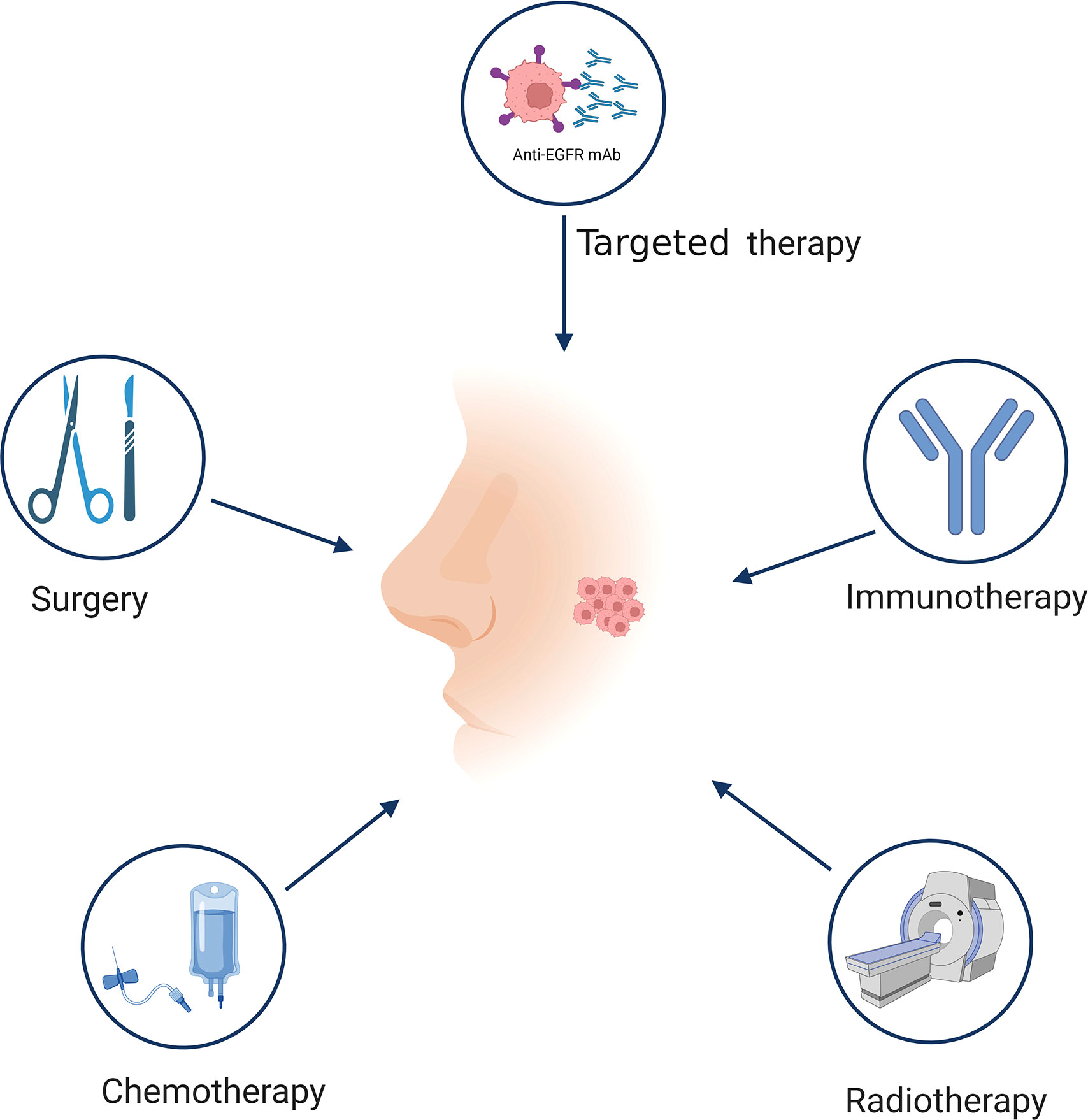
Figure 2 Treatment of NPC. Different stages of NPC have different treatment methods. The traditional treatment of NPC includes surgery, radiotherapy, chemotherapy, and targeted therapy. Immunotherapy is a promising therapeutic approach for NPC treatment. Figure was created with BioRender.
At present, the clinical efficacy of radiotherapy or chemotherapy alone for the patients with RM-NPC is suboptimal, and it may be improved by the use of immunotherapy. The dense matrix infiltration by immune cells and EBV antigen expression in NPC patients are the main research targets for immunotherapy (36, 37). The primary strategies for immunotherapy are vaccination, adoptive cell therapy (ACT), and immune checkpoint blockade. Here, we review important research advances in the field of NPC immunotherapy, hoping to provide insights for future studies.
2. EBV-directed vaccination
Infection with EBV primarily leads to epithelial and B cell malignancies, including NPC, infectious mononucleosis (IM), and Burkitt’s lymphoma (38–42). Concurrent chemoradiotherapy, induction chemotherapy, and adjuvant chemotherapy are the three main therapies for NPC patients that have good curative effects. However, the emergence of drug resistance and adverse events limit the application of chemotherapy for NPC (29). Sources of EBV infection are widespread, and transmission routes are difficult to cut off, similar to those of human papillomavirus and hepatitis B virus (43, 44). Vaccination is the most effective treatment to prevent infection with EBV and the most cost-effective way to treat IM and EBV-associated diseases, such as multiple sclerosis [(45), Figure 3A]. An effective EBV vaccine will make a great impact on public health and the economy. The use of EBV preventive vaccines is intended to prevent EBV infection of target cells by eliciting neutralizing antibodies (43–45). Two types of vaccines have been investigated for NPC treatment, peptide and viral vaccines. Peptide vaccines primarily focus on gp350, which stimulates the body to produce neutralizing antibodies to block the virus infection pathway after inoculation. Other antiviral vaccines currently being studied use LMP1, LMP2, EBNA1, and EBNA3 as target antigens or their combinations (46–50).
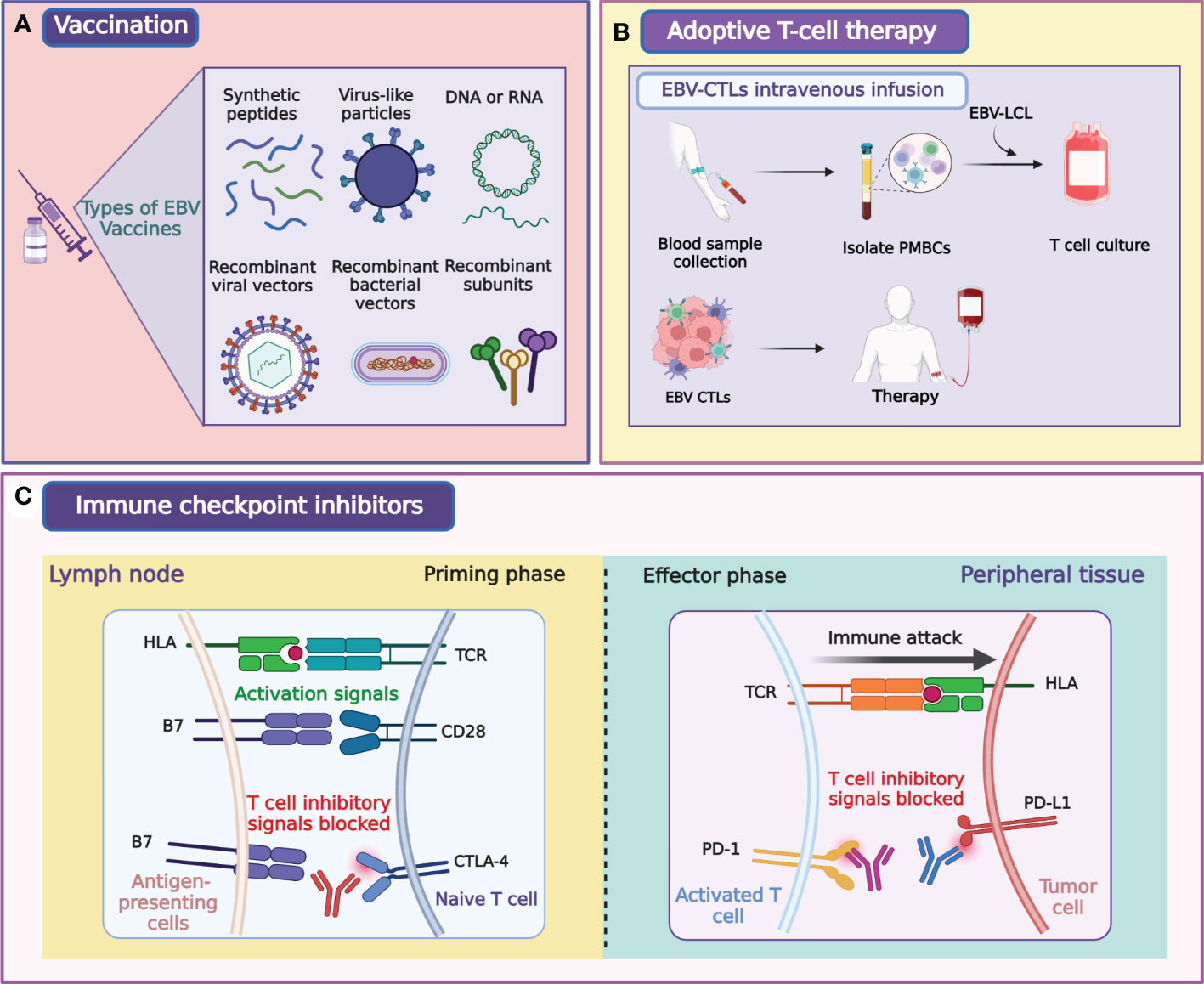
Figure 3 Illustration of major modalities of tumor immunotherapy for NPC. Primary strategies of tumor immunotherapy for NPC include EBV-directed vaccination (A), EBV-CTLs intravenous infusion (B), and immune checkpoint blockade (C). Figure was created with BioRender.
EBV needs to pass through a variety of membrane proteins to enter the cytoplasm of the epithelial, B cells, and NK/T cells. The infection of B cells by EBV requires five membrane proteins (gp350, gH, gL, gB, and gp42), whereas the infection of epithelial cells by EBV needs four membrane proteins (BMFR2, gH, gL, and gB). These proteins are expressed on EBV and could be good targets for EBV-preventive vaccine (35, 51–56). Studies on vaccines for EBV-related diseases were began in the 1980s, with approximately half the studies focusing on the EBV protein gp350 (also known as gp340) that binds to CD21/CD35 of B cells [ (57, 58), Table 1]. Early studies demonstrated that the gp350-containing vaccine impacts the infection process. Non-recombinant gp340 vaccine was first shown to work against EBV-induced lymphoma in an animal trial in the 1980s (70). In the early 1990s, the first clinical trial of a vaccine containing gp350 were conducted in China. Subjects developed corresponding antibodies after receiving the vaccine; however, this vaccine was not further developed (59). Two clinical trials of the vaccine containing recombinant gp350 showed considerable efficacy (mean response rate, 78.0%) in preventing IM caused by EBV infection but not the asymptomatic EBV infection (60, 61). This was most likely because gp350-induced antibodies did not protect epithelial cells from EBV infection. Therefore, it is important to identify new target antigens to develop an effective EBV-preventive vaccine. EBV glycoproteins gH/gL and gB co-mediate the fusion step of EBV into B cells or epithelium. Inoculation with vaccines containing these proteins induced the development of antibodies that could broadly protect against EBV infection (71, 72). The immunogens of proteins expressed or polymerized by multimers are relatively stronger than those of single protein polymers (73–75). Cui et al. compared the vaccines based on the tetrameric and monomeric isoforms of the gp350 protein in ovarian cells and found that the tetrameric gp350 had markedly higher immunogenicity than its monomeric counterpart. These data showed that the application of the tetrameric gp3501–470 and EBV protein multimerization, in general, may facilitate the development of a potent prophylactic EBV vaccine (76). According to two reports published in 2016 and 2021, serum neutralization titers of antibodies raised against EBV gp350 monomer were lower than those induced by three dimer and trimer gH/gL, trimer gB, or four gp3501–470 polymers (77, 78). This may be attributed to the key role of gH/gL and gB in the fusion and entry of EBV into B cells or epithelium. Therefore, compared with gp350 alone, EBV gH/gL and gB may be the better targets for EBV-preventive vaccine, and gp350 binding with gH/gL, gB may yield a stronger EBV-preventive vaccine. In 2021, the gH/gL-specific antibody 1D8 was isolated from EBV-infected individuals to target EBV-vulnerable sites (79). 1D8 binds to the critical fragile interfaces of viral gH/gL proteins, disrupting the gH/gL-mediated fusion of EBV with the membrane of target cells. The discovery of antibodies against new EBV targets is therefore highly warranted. Our team comprehensively described the response between T cells and full-length gB. In vitro, gB-specific CD8+ T cells were found to inhibit the transformation of B cells; available gB epitopes, including gB D-II and D-IV, were identified; and two specific gB antibodies (3A3 and 3A5) were isolated. The two antibodies were identified to target and neutralize EBV-infected B cells and epithelial cells. These studies indicate that accurate localization of gB T cell epitopes is beneficial to the development of gB subunit vaccines and immune surveillance and that gB D-II and D-IV are promising targets for the development of EBV vaccines (58, 80).
Despite numerous attempts to produce vaccines against EBV, none has been licensed to date. Virus-like particles (VLPs) may be promising vaccine candidates. The VLP-based vaccine against hepatitis B and HPV have been widely used in clinical practice, suggesting that a similar approach may be fruitful for an effective vaccine against EBV (79, 81–83). The first complete EBV VLPs had knockouts of the potential cancer-causing genes and repetitive sequences in the EBV terminal, such as LMP1 and BZLF1. These EBV VLPs elicited EBV-specific responses in mice after immunization (45, 84). A study constructed an EBV VLP-based vaccine on the basis of a novel Newcastle disease virus (NDV). The platform, named EBVgp350/220-F, was formed by the fusion of EBVgp350/220 with the NDV fusion (F) protein (85). In vivo experiments, EBVgp350/220-F VLPs induced a high and persistent neutralizing antibody response in mice (85). At present, the platform of NDV VLP is applied to developing gH/gL-EBNA1 and gB/LMP2 VLPs. LMP1, LMP2, EBNA1, and EBNA3, alone or in combinations, are primarily used as target antigens for anti-EBV vaccines because of their important roles in mediating EBV infection and subsequent malignant transformation of infected cells (50, 86–88). A clinical trial performed in 2002 showed that immunization with EBV peptide-pulsed DCs induces tumor disappearance in patients with EBV-induced NPC (89). Epitope-specific CD8+ T cell responses were induced or enhanced in 9 out of 16 subjects (56%) in that trial. In another study, 16 patients with HLA-A2-positive stage II/III NPC were administered autologous DCs containing the HLA-A2-restricted LMP2A peptide, and in nine of them, the responses of circulating LMP2-specific T cells were improved and serum EBV DNA levels were modestly reduced (66). There was a study assessed the efficacy of Ad-ΔLMP1-LMP2 transduced DCs vaccine in total 12 patients with NPC, this vaccine increased the activity of LMP1/2-specific T cells in vitro (64). Furthermore, in nine out of these 12 patients, this vaccine caused late-onset hypersensitivity reactions (64). Thus, although the clinical efficacy of DC-based vaccines in these clinical trials was mixed, the safety and tolerability of EBV vaccines in NPC patients were consistently demonstrated.
Recently, recombinant viral vector vaccines, such as the one based on modified vaccinia Ankara (MVA), have been created (90, 91). One study showed that an MVA fusion protein could effectively reactivate CD4+ memory T cell responses in vitro, and the MVA fusion protein comprised both the carboxyl terminus of EBNA1 and full-length LMP2(MVA-EL) (92). The first human MVA-EL vaccine trial involving 18 NPC patients was conducted in Hong Kong (67). Responses of T cells to vaccine antigens were enhanced in fifteen out of the 18 subjects in that study; therefore, MVA-El was safe and immunogenic. A separate phase I study of the MVA-EL vaccine in the patients with NPC was conducted in the United Kingdom, which demonstrated increased CD4+ and CD8+ T cell responses to antigens in eight out of 14 patients (68). Another two clinical trials with this vaccine, NCT01800071 and NCT01094405, are in progress.
Recently, an mRNA vaccine has been successfully used against SARS-CoV-2, and a synthetic mRNA vaccine against EBV is currently in a clinical trial (phase I, NCT05164094). Although mRNA vaccines have many advantages than conventional vaccines, there are also potential challenges.
Thus, clinical trials demonstrated that vaccines against EBV have substantial clinical value. Nonetheless, they also have some limitations: DC-based vaccines have few targets and high preparation cost, whereas although recombinant virus vectors have broad epitopes, their immune function may be reduced after repeated immunizations. It should be noted that different administration routes should be systematically examined to facilitate EBV vaccine delivery. Future vaccines containing EBNA1, LMP1/2, gp350, gH/gL, and gB are promising directions for EBV vaccine research.
3. Adoptive cell therapy
In recent years, ACT has become a powerful strategy in the treatment of human cancers, especially some blood malignancies. However, extending these therapies to solid cancers such as NPC remains problematic. Here, we discuss recent developments in several ACT approaches.
3.1. EBV-specific cytotoxic T lymphocyte therapy
EBV-associated posttransplant lymphoproliferative disorders (PTLDs) are common complications in solid organ and bone marrow transplant recipients (93–96). PTLDs are related to the massive proliferation of EBV-B cells, cytotoxic T lymphocytes (CTLs) control its expansion in immunologically active individuals (97). Many clinical trials showed that ACT with EBV-specific CTLs prevented and treated PTLDs in patients with hematopoietic stem cell transplantation (98–102). EBV-specific CTLs also effectively treated PTLDs in patients with solid organ transplants that were on high immunosuppression regimens (93–95, 103, 104). These results have broadened the application of ACT in EBV-associated cancers, and both EBNA1 and LMP1 were the targets for the amplification of EBV-specific CTLs in the majority of studies (65, 105–111). The clinical ACT approach used for NPC treatment involves lymphoblastoid cell line-generated EBV-specific T cells (Figure 3B; Table 2). A clinical trial using EBV-specific CTLs to treat NPC patients showed that the content of EBV DNA in plasma was decreased to an undetectable level in all subjects (112). A clinical study published in 2004 showed that EBV-specific CTLs can be effectively used to treat NPC patients, and proved that EBV-specific CTLs with anticancer properties detected in vitro could increase the LMP2-specific immune response (113). Another study that used EBV-specific CTLs to treat NPC showed that disease progression was controlled in 6 of 10 patients. This trial also demonstrated that EBV-targeted autologous CTL therapy was safe and induced LMP2-specific immune activity that controlled the disease progression in stage IV NPC resistant to conventional therapy (107). Two clinical trials by Straathof et al. further proved that this treatment was safe and performed a activity for anti-tumors (108, 114).
Notably, the reaction rate varied in different clinical studies, primarily because these trials utilized different techniques to produce EBV-specific CTLs. In addition, these trials involved patients at different NPC stages, which could also have distinct previous treatments, comorbidities, and genetic susceptibility. To raise the immunogenicity and enhance antigen specificity, a new adenovirus vector, AdE1-LMPpoly was generated that encoded EBNA1, which covalently binds to multiple epitopes in CD8+ T cell (119). A clinical study showed that AdE1-LMPpoly vector stimulated CD8+ T cells in Hodgkin lymphoma donors, stimulating the rapid expansion of EBNA1 and LMP1/2-specific CD8+ T cells (120). Two studies using AdE1-LMPpoly confirmed that MVA vaccines harboring the EBNA1 sequence prevented and treated lymphoma (90, 121). A clinical trial to treat NPC by EBV-specific T cells induced by the new AdE1-LMPpoly vector showed that sixteen of 24 patients with NPC had expanded EBV-specific T cells, whereas no effect was noted in 27.3% of NPC cases. Furthermore, compared with the control group, the median overall survival increased from 220 days to 523 days (65). In subsequent studies, the same team demonstrated that T cell therapy combined with AdE1-LMPpoly was well tolerated in high-risk cases without residual diseases and cases with recurrent/metastatic diseases (111).
A phase 2 clinical trial was carried out by Chia et al. to assess the efficacy of chemotherapy combined with EBV-specific CTLs in 35 patients. The effective rate of combined therapy was 71.4%, with five cases not needing further chemotherapy over 34 months after the start of treatment with CTLs. Compared with all similar studies, this trial achieved the best results in patients with advanced NPC, which shows that chemotherapy combined with EBV-specific CTL therapy is a very promising approach (116).
3.2. EBV-specific chimeric antigen receptor T cell therapy
Chimeric antigen receptor T cell immunotherapy (CAR-T) has been tested for many years but was only recently approved for humans. CAR-T has shown a good efficacy in treating to acute leukemia and non-Hodgkin lymphoma and is considered to be one of the most promising tumor treatment modalities (Figure 4). Currently, there are eight approved CAR-T products: six targeting CD19 and two targeting BCMA (B cell maturation antigen). CAR-T is applied primarily in hematological malignancies, such as diffuse large B cell lymphoma (DLBCL), B cell acute lymphoblastic leukemia (B-ALL) as well as recurrent or refractory forms of multiple myeloma, follicular lymphoma, and mantle cell lymphoma. Although the innovative immune therapy with CAR-T cells shows considerable efficacy in hematological malignancies, its application in solid tumors is difficult (122). Despite the efforts of scientists, clinical doctors, and pharmaceutical companies worldwide, CAR-T cells have not been clinically approved to treat any solid tumor. The lack of good target antigens is the greatest obstacles facing in the CAR-T therapies (123, 124). Encouragingly, there are more than 1,100 registered clinical trials evaluating the treatment of cancers using CAR-T cells (59 have been completed), of which approximately 200 relate to solid tumors.
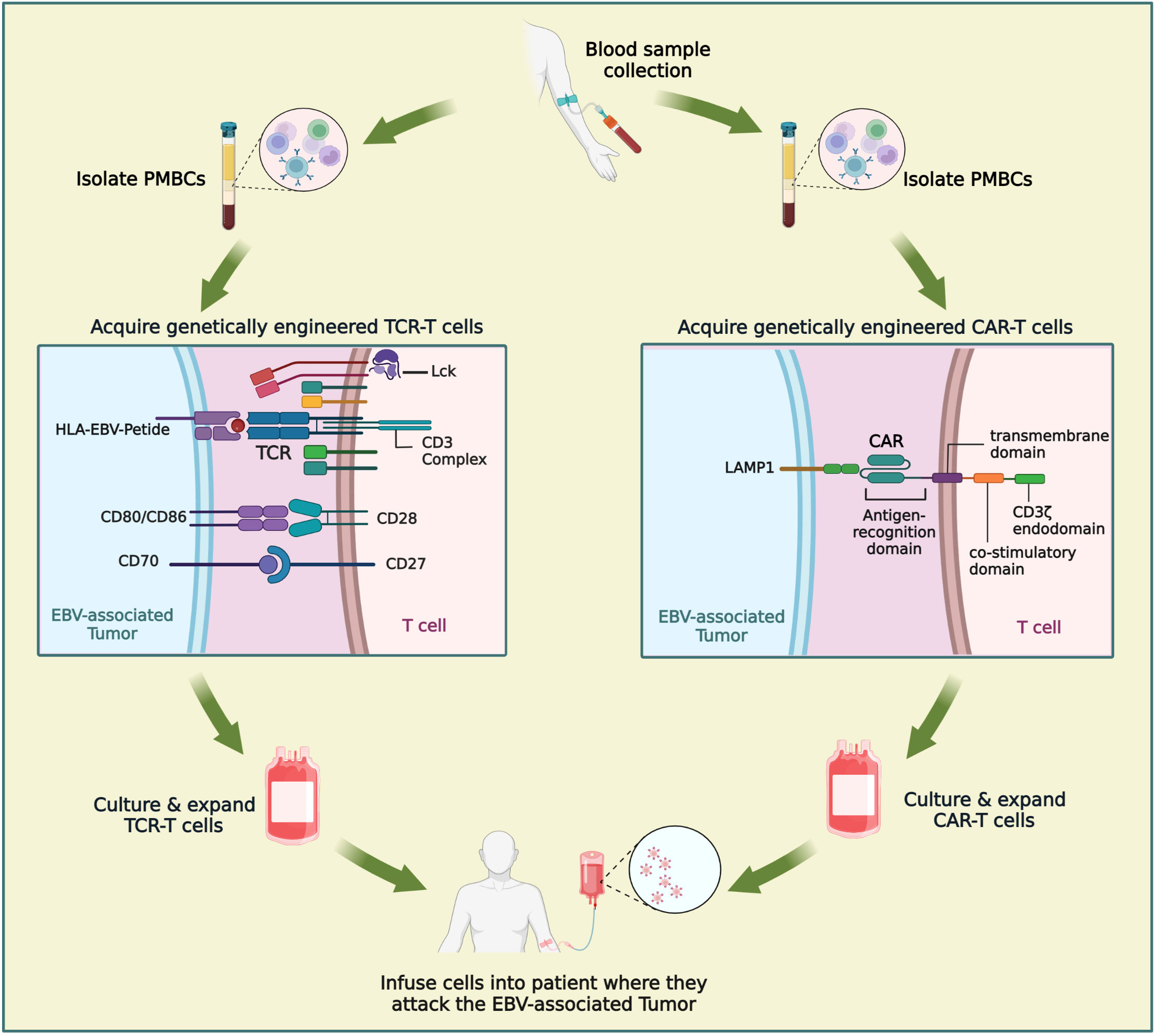
Figure 4 Comparison of CAR-T and TCR-T cells in the treatment of NPC. Engineered immune cell therapy works by modifying immune cells so that they can recognize disease states and respond appropriately. When engineered immune cells are transferred into patient, they are a “living drug”. TCR-T is similar to CAR-T in that it involves engineering a patient’s own T lymphocytes and then injecting back into the patient. The procedure mainly includes: extraction of patients’ peripheral blood, isolation of PBMC, engineering of immune cells, amplification of immune cells and cell transfusions into patients. Figure was created with BioRender.
As of today, six registered clinical studies are evaluating the efficacy of NPC by CAR-T therapies (Table 3). A phase 1 trial (NCT02915445) conducted on patients with NPC or breast cancer aimed to evaluate the therapy with CAR-T cells specifically targeting epithelial cell adhesion molecule (EpCAM). EpCAM-specific CAR-T cells were administered to different cohorts of patients (NCT02915445). In vitro assays demonstrated that LMP1-specific CAR-T cells kill 70% of NPC cells overexpressing LMP1. Furthermore, injecting LMP1-specific CAR-T cells into mice with tumors markedly reduced the number of LMP1high NPC cells. Although it is doubtful that LMP1-specific CAR-T cells can target cancer cells in NPC with much lower LMP1 expression, these results are encouraging (125, 126), and a clinical trial for LMP1-specific CAR-T cells to treat EBV-associated malignant tumors is underway (NCT02980315). Furthermore, a phase I study designed to treat patients with relapsed or refractory NPC is ongoing (NCT04107142).
3.3. T cell receptor-engineered T cell therapy
T cell receptor-engineered T cell therapy (TCR-T) has quietly arrived on the cancer therapy scene (Figure 4). TCR-T has shown unprecedented potential in the therapy of solid tumors and has gradually attracted increasing attention because of its ability to target a variety of antigens within tumors. TCR-T is similar to CAR-T in that it involves engineering a patient’s own T lymphocytes and then injecting back into the patient. However, the mechanisms by which TCR-T and CAR-T recognize antigens differ. As an antigen recognition element in T cell therapy, TCR can recognize a wider range of potential tumor-specific antigens, especially the ultra-sensitive recognition of low-level variation of intracellular antigens, while CAR recognizes only tumor cell surface antigens. The immunosuppressive tumor microenvironment and tumor-associated antigen expression rate on the cell surface of solid tumors are low, and most of the cell proteins are intracellular. These factors limit the clinical application of CAR-T in solid tumors, while TCR-T may have a more effective role against solid tumors. In nearly two years, clinical studies of TCR-T cell therapy in HBV-related HCC and HPV-related cervical cancer have been reported, and good results have been achieved (127–129). There are more and more studies on the treatment of nasopharyngeal carcinoma by TCR-T cells. LMP2 antigens are a potential target of TCR-T cells; targeted clearance of cells containing LMP2 may have robust antitumor properties and limited toxicity to normal cells. Tumor progression in LMP2-expressing NPC cell lines implanted in mice was inhibited by LMP2-specific TCR-T cells (130). Similar results were observed for the LMP1-specific TCR-T cells (131). At present, there are four ongoing clinical trials evaluating TCR-T for NPC (NCT04509726, NCT03925896, NCT03648697, NCT05587543) (Table 3). The first clinical study of TCR-T therapy for NPC was launched in 2018, in which NPC patients with high expression of LMP1, LMP2, and EBNA1 were selected for study. The TCR targets were screened to explore the role of EBV antigen-specific T cells (YT-E001) in RM-NPC patients (NCT03648697). The latest clinical trial, first posted in October 2022, aims to compare the efficacy of CAR-T and TCR-T cells in the treatment of NPC (NCT05587543). TCR-T therapy for cancer is an exciting and rapidly developing field. The application of TCR-T therapy has pioneered an innovation h to treating cancer, viral infections, and other immunomodulatory diseases. It is hoped that more appropriate immune targets will be selected, and TCR-T transfection methods will be optimized in the near future through the unremitting efforts of researchers. TCR-T therapy is bound to play an significant role in cancer, infectious diseases, or autoimmune diseases.
3.4. NK cell therapy
Our team has been actively developing new approaches and targets for the treatment of EBV-NPC. We found that after EBV infection, LMP2A induced the upregulation of F3 expression and was associated with the dysfunction of NK cells in NPC (2). We used a combination of F3 inhibitor and NK cell adoptive therapy and achieved a considerable therapeutic effect in a mouse model of NPC. Another study by our group was performed to elucidate the mechanisms involved in EBV-induced NK cell dysfunction. We found that deletion of B7-H3 on tumor cells, in combination with anti-PD-L1(programmed death-ligand 1) treatment, restored NK cell-mediated antitumor activities and showed synergistic therapeutic efficacy. This study, which is currently under submission, aims to provide a rationale for NK cell-based immunotherapies in combination with PD-L1 blockade for overcoming the immunosuppression of B7-H3 to treat EBV-associated NPC. Therefore, targeting EBV-related signaling pathways, combined with NK cell adoptive therapy, may be a new direction to explore for the treatment of NPC in the future.
4. Immune checkpoint inhibitors
Patients with RM-NPC have limited treatment options, making chemotherapy the main treatment; however, its curative effect is unsatisfactory (34, 132–137). The emergence of immunotherapy has turned around the therapy for RM-NPC. Cisplatin combined with gemcitabine is the first-line therapy for RM-NPC; however, the second-line treatment is still lacking (138–140). Programmed death-1 (PD-1) and PD-L1 are associated with tumor immune escape and immunotherapy, which are critical for the tumor survival. NPC induced by EBV often presents with high levels of PD-L1 and substantial lymphocyte infiltration, thus, application of PD-1 blockade immunotherapy may be beneficial (141). Indeed, preclinical studies have shown that EBV proteins LMP1 and EBNA1/2 regulate PD-L1 levels, thereby modulating the extent of immune escape (142–144).
Treatment of NPC by immune checkpoint inhibitors (ICIs) is currently a hot research topic. Effective ICIs may break the immune defense and revitalize endogenous antitumor immunity (Figure 3C). Primary targets of ICIs include PD-1/L1 and cytotoxic T lymphocyte-associated protein 4 (CTLA-4). Three clinical trials of anti-PD-1 in patients with RM-NPC reported the objective response rates in the range of 20.5–34.1% (145–148). To date, a considerable number of ICIs have been licensed for use by the FDA.
Pembrolizumab has been demonstrated to have a good antitumor activity and safety profile in previously treated patients with RM-NPC (147, 149–151). Another ongoing phase II trial (NCT03544099) aims to evaluate the efficacy of pembrolizumab for NPC patients with plasma EBV DNA after radio-chemotherapy. A study of nivolumab in patients with RM-NPC reported promising antitumor activity and favorable 1-year overall survival rates (145). A case of NPC was reported in which the patient was given nivacizumab, a PD-L1 inhibitor, because of the high PD-L1 expression in his tumor. Following this treatment, it was found that the tumor rapidly and completely subsided, and there was no recurrence after 22 months of treatment. This study sets a foundation for future trials, involving many NPC patients (152). Fan et al. showed that camrelizumab, another anti-PD-1 inhibitor also had strong antitumor activity and was well tolerated in patients with RM-NPC. Based on the above results in these two papers, the authors will start a phase III trial to treat the patients with RM-NPC by using chemotherapy combined with PD-1 inhibitors (148). A phase 3 trial aimed to study the effect of RM-NPC treated by chemotherapy combined with camrelizumab in the treatment of RM-NPC. The results showed considerable prolongation of progression-free survival in patients that underwent combination therapy; therefore, the combination of camrelizumab and chemotherapy may become a new method to treat NPC (153).
On February 19, 2021, an anti-PD-1 monoclonal antibody named toripalimab was licensed by the National Medical Products Administration in China to treat the patients with RM-NPC whom had previously failed second-line or systemic therapy. Thus, toripalimab has become the first anti-PD-1 monoclonal antibody approved for NPC treatment in the world as a breakthrough in immunotherapy in this field (154). Wan et al. reported key clinical results of treatment with toripalizumab (POLARIS-02), which showed that the drug is safe, controllable, and induces a lasting clinical response in patients with refractory NPC that poorly respond to chemotherapy (155). More than 80 clinical studies aiming to evaluate anti-PD-1 treatments for NPC have been or are currently being conducted, with 20 of them testing the efficacy of toripalimab.
CTLA-4, belongs to the CD28 immunoglobulin subfamily and functions as a receptor that suppresses T cell activation (156). CTLA-4 and PD-1 are two of the earliest targets for which ICIs have been developed. The immune response rate to an ICI treatment is limited because it is a single-drug therapy, which, in turn, restricts the clinical application of ICIs (157). After years of clinical experience, the direction of CTLA-4 inhibitor development has become increasingly clear: the combination therapy of CTLA-4 inhibitor and PD-1 or PD-L1 may have the broadest application. CTLA-4 and PD-L1 work within different parts of the immune system and exert distinct effects. CTLA-4 acts primarily in the priming phase and is the main factor affecting antigen presentation. PD-L1 primarily acts in the effector phase, blocking immune checkpoints and inducing tumor death. Therefore, blocking both CTLA-4 and PD-L1 may enhance antitumor effects. Ipilimumab is the only CTLA-4 drugs authorized worldwide, furthermore, many anti-CTLA-4-targeting inhibitors in development and more than 20 in clinical trials. Ipilimumab is combined with pembrolizumab and nivolumab for immunotherapy of highly metastatic colorectal cancer, gastroesophageal, and other cancers (158–162). Combination therapy is more effective than conventional monoclonal antibody therapy and provides an opportunity for the development and application of bi-specific antibodies. The first PD-1/CTLA-4 bi-specific antibody product approved worldwide was developed by Akeso, Inc. The range of this antibody is applicable to a series of solid tumors, such as cervical cancer, lung cancer, gastric cancer, and NPC (163). Continuous monitoring of adverse events is required during treatment with bi-specific blockers, as inhibiting multiple targets simultaneously may increase the frequency of side effects.
Our team have found that anti-human CD39 antibody increased the expression of human CD8+T cells and inhibited human B-cell lymphoma after autologous EBV-specific T cell metastasis (164, 165). Based on our study, we conducted the first human trial of anti-CD39 in patients with advanced cancer (NCT03884556) (164, 165). These studies have provided new targets and ideas for the immunotherapy of EBV-NPC.
5. Conclusion
Tumor immunotherapy is a new technological approach that can overcome the disadvantages of the three traditional methods in cancer therapy: surgery, radiotherapy, and chemotherapy. In particular, tumor immunotherapy may provide a better outcome in cases of incompletely surgically removed, metastatic, and easy-to-relapse tumors. The specificity of immunotherapy effectively kills tumor cells in the absence of serious side effects and prolongs patient survival. Immunotherapy controls the proliferation of tumor cells by irritating and enhancing immune functions in endogenous organisms, and its current main approaches are based on the use of vaccination, ACT, and ICIs. At present, there are several therapeutic strategies to generate EBV vaccines, including the prevention of EBV infection, modulation of the incubation period, and inhibition of the occurrence of EBV-associated tumors. Development of the polymeric EBV vaccine containing gH/gL, gB, and gp350 is a particularly promising direction. Therapeutic vaccines against EBV are likely to be more efficient than preventive vaccines because it takes a long time for EBV infection to induce diseases; therefore, developing preventive vaccines against EBV-related diseases such as NPC may not be possible. Most therapeutic vaccine preparations currently use EBNA1, LMP1, and LMP2 as targets. These proteins play an important role in the malignant transformation of EBV-infected cells. During the last few years, many studies have achieved remarkable successes in developing ACT against EBV antigens. EBV vaccine together with T cell infusion may be a good way to treat NPC and this combination can enhance the specificity of T cells. To enhance the clinical efficacy of ACT therapy in NPC, CAR-T cells raised against an EBV target and a surface receptor may be utilized. To expand the efficiency of CAR-T cells, new antigen targets for EBV-induced NPC should be urgently identified. In recent years, many cellular immunotherapies have emerged and are developing rapidly, undoubtedly setting off an upsurge in cancer treatment. T cell immunotherapy in general is a hot field in cellular immunotherapy. Both CAR-T cells and TCR-T cells are T cells modified by genetic engineering. While TCR-T therapy may not be as well known to the general public, it may offer advantages over CAR-T therapy in the treatment of solid tumors. Another possible effective strategy to treat EBV-NPC is to combine ACT with ICIs against PD-1/L1, or CTLA-4. The optimization of these methods is expected to render immunotherapy the first-line therapy for various EBV-related diseases. Tumor immunotherapy also decreases the incidence of adverse reactions and complications arising from chemotherapy. It can kill two birds with one stone, thus protecting NPC patients. Therefore, chemotherapy combined with immunotherapy may increase the efficacy of the treatment and reduce its toxicity for NPC patients. Integrative therapy that combines NPC immunotherapy with conventional therapeutic approach is not simply combination of existing methods but, rather, a combination of treatments that achieves the highest synergy.
Although most immunotherapy strategies for EBV-related NPC are still at the clinical trial stage, the published and emerging research results indicate that immunotherapy for NPC may soon be used in clinical settings. Ongoing research hopes to achieve a selection of practical immune targets and optimized TCR-T transfection methods in the near future. We believe that TCR-T cell therapy may currently be the most promising approach to treatment of EBV.
Author contributions
WL, XBD and XC performed literature review and wrote the manuscript. MZ and HP revised the figures and tables. YM and XL revised the manuscript. X-YL, GP, and XHD developed the review concept. All authors contributed to the article and approved the submitted version.
Funding
This study was supported by research grants from the National Natural Science Foundation of China (No. 82230067, 81902936, 82003450, 82103433), Guangdong Provincial Key Laboratory of Tumor Interventional Diagnosis and Treatment (2021B1212040004), Guangdong Basic and Applied Basic Research Foundation (No. 2020B1515020010, 2020A1515110013, 2022B1515020010), and Zhuhai Science and Technology Planning Medical and Health Project (No. ZH22036201210108PWC).
Acknowledgments
The authors thank Professor Li-gong Lu from Zhuhai People’s Hospital affiliated with Jinan University for providing the necessary support for our article.
Conflict of interest
X-YL is an employee of OriCell Therapeutics.
The remaining authors declare that the research was conducted in the absence of any commercial or financial relationships that could be constructed as a potential conflict of interest.
Publisher’s note
All claims expressed in this article are solely those of the authors and do not necessarily represent those of their affiliated organizations, or those of the publisher, the editors and the reviewers. Any product that may be evaluated in this article, or claim that may be made by its manufacturer, is not guaranteed or endorsed by the publisher.
References
1. Young LS, Yap LF, Murray PG. Epstein-Barr Virus: more than 50 years old and still providing surprises. Nat Rev Cancer (2016) 16(12):789–802. doi: 10.1038/nrc.2016.92
2. Duan X, Chen H, Zhou X, Liu P, Zhang X, Zhu Q, et al. EBV infection in epithelial malignancies induces resistance to antitumor natural killer cells via F3-mediated platelet aggregation. Cancer Res (2022) 82(6):1070–83. doi: 10.1158/0008-5472.CAN-21-2292
3. Huang YH, Zhang CZ, Huang QS, Yeong J, Wang F, Yang X, et al. Clinicopathologic features, tumor immune microenvironment and genomic landscape of Epstein-Barr virus-associated intrahepatic cholangiocarcinoma. J Hepatol (2021) 74(4):838–49. doi: 10.1016/j.jhep.2020.10.037
5. Zheng X, Wang R, Zhang X, Sun Y, Zhang H, Zhao Z, et al. A deep learning model and human-machine fusion for prediction of EBV-associated gastric cancer from histopathology. Nat Commun (2022) 13(1):2790. doi: 10.1038/s41467-022-30459-5
6. Kanda T, Yajima M, Ikuta K. Epstein-Barr Virus strain variation and cancer. Cancer Sci (2019) 110(4):1132–9. doi: 10.1111/cas.13954
7. Jha HC, Pei Y, Robertson ES. Epstein-Barr Virus: Diseases linked to infection and transformation. Front Microbiol (2016) 7:1602. doi: 10.3389/fmicb.2016.01602
8. De Leo A, Arena G, Stecca C, Raciti M, Mattia E. Resveratrol inhibits proliferation and survival of Epstein Barr virus-infected burkitt's lymphoma cells depending on viral latency program. Mol Cancer Res MCR (2011) 9(10):1346–55. doi: 10.1158/1541-7786.MCR-11-0145
9. Kozireva S, Rudevica Z, Baryshev M, Leonciks A, Kashuba E, Kholodnyuk I. Upregulation of the chemokine receptor CCR2B in EpsteinBarr virus-positive burkitt lymphoma cell lines with the latency III program. Viruses (2018) 10(5):239. doi: 10.3390/v10050239
10. Tsao SW, Tsang CM, To KF, Lo KW. The role of Epstein-Barr virus in epithelial malignancies. J Pathol (2015) 235(2):323–33. doi: 10.1002/path.4448
11. Vrzalikova K, Ibrahim M, Nagy E, Vockerodt M, Perry T, Wei W, et al. Co-Expression of the Epstein-Barr virus-encoded latent membrane proteins and the pathogenesis of classic Hodgkin lymphoma. Cancers (Basel) (2018) 10(9):285. doi: 10.3390/cancers10090285
12. Rosales-Perez S, Cano-Valdez AM, Flores-Balcazar CH, Guedea-Edo F, Lino-Silva LS, Lozano-Borbalas A, et al. Expression of Epstein-Barr virus-encoded latent membrane protein (LMP-1), p16 and p53 proteins in nonendemic nasopharyngeal carcinoma (NPC): a clinicopathological study. Arch Med Res (2014) 45(3):229–36. doi: 10.1016/j.arcmed.2014.02.002
13. Xiang T, Lin YX, Ma W, Zhang HJ, Chen KM, He GP, et al. Vasculogenic mimicry formation in EBV-associated epithelial malignancies. Nat Commun (2018) 9(1):5009. doi: 10.1038/s41467-018-07308-5
14. Leong MML, Lung ML. The impact of Epstein-Barr virus infection on epigenetic regulation of host cell gene expression in epithelial and lymphocytic malignancies. Front Oncol (2021) 11:629780. doi: 10.3389/fonc.2021.629780
15. Bray F, Ferlay J, Soerjomataram I, Siegel RL, Torre LA, Jemal A. Global cancer statistics 2018: GLOBOCAN estimates of incidence and mortality worldwide for 36 cancers in 185 countries. CA: Cancer J Clin (2018) 68(6):394–424. doi: 10.3322/caac.21492
16. Sung H, Ferlay J, Siegel RL, Laversanne M, Soerjomataram I, Jemal A, et al. Global cancer statistics 2020: GLOBOCAN estimates of incidence and mortality worldwide for 36 cancers in 185 countries. CA: Cancer J Clin (2021) 71(3):209–49. doi: 10.3322/caac.21660
17. Tang LL, Chen WQ, Xue WQ, He YQ, Zheng RS, Zeng YX, et al. Global trends in incidence and mortality of nasopharyngeal carcinoma. Cancer Lett (2016) 374(1):22–30. doi: 10.1016/j.canlet.2016.01.040
18. Ji MF, Sheng W, Cheng WM, Ng MH, Wu BH, Yu X, et al. Incidence and mortality of nasopharyngeal carcinoma: interim analysis of a cluster randomized controlled screening trial (PRO-NPC-001) in southern China. Ann Oncol (2019) 30(10):1630–7. doi: 10.1093/annonc/mdz231
19. Doescher J, Veit JA, Hoffmann TK. [The 8th edition of the AJCC cancer staging manual : Updates in otorhinolaryngology, head and neck surgery]. HNO (2017) 65(12):956–61. doi: 10.1007/s00106-017-0391-3
20. Matos LL, Dedivitis RA, Kulcsar MAV, de Mello ES, Alves VAF, Cernea CR. External validation of the AJCC cancer staging manual, 8th edition, in an independent cohort of oral cancer patients. Oral Oncol (2017) 71:47–53. doi: 10.1016/j.oraloncology.2017.05.020
21. Amin MB, Greene FL, Edge SB, Compton CC, Gershenwald JE, Brookland RK, et al. The eighth edition AJCC cancer staging manual: Continuing to build a bridge from a population-based to a more "personalized" approach to cancer staging. CA: Cancer J Clin (2017) 67(2):93–9. doi: 10.3322/caac.21388
22. Spano JP, Busson P, Atlan D, Bourhis J, Pignon JP, Esteban C, et al. Nasopharyngeal carcinomas: an update. Eur J Cancer (2003) 39(15):2121–35. doi: 10.1016/s0959-8049(03)00367-8
23. Xie Z, Li W, Ai J, Xie J, Zhang X. C2orf40 inhibits metastasis and regulates chemo-resistance and radio-resistance of nasopharyngeal carcinoma cells by influencing cell cycle and activating the PI3K/AKT/mTOR signaling pathway. J Transl Med (2022) 20(1):264. doi: 10.1186/s12967-022-03446-z
24. Jiromaru R, Nakagawa T, Yasumatsu R. Advanced nasopharyngeal carcinoma: Current and emerging treatment options. Cancer Manag Res (2022) 14:2681–9. doi: 10.2147/CMAR.S341472
25. Zhang L, Chen QY, Liu H, Tang LQ, Mai HQ. Emerging treatment options for nasopharyngeal carcinoma. Drug Des Devel Ther (2013) 7:37–52. doi: 10.2147/DDDT.S30753
26. Spratt DE, Lee N. Current and emerging treatment options for nasopharyngeal carcinoma. Onco Targets Ther (2012) 5:297–308. doi: 10.2147/OTT.S28032
27. Guan S, Wei J, Huang L, Wu L. Chemotherapy and chemo-resistance in nasopharyngeal carcinoma. Eur J Med Chem (2020) 207:112758. doi: 10.1016/j.ejmech.2020.112758
28. Sidaway P. Chemoradiotherapy improves NPC outcomes. Nat Rev Clin Oncol (2020) 17(10):592. doi: 10.1038/s41571-020-0424-9
29. Chen YP, Ismaila N, Chua MLK, Colevas AD, Haddad R, Huang SH, et al. Chemotherapy in combination with radiotherapy for definitive-intent treatment of stage II-IVA nasopharyngeal carcinoma: CSCO and ASCO guideline. J Clin Oncol (2021) 39(7):840–59. doi: 10.1200/JCO.20.03237
30. Li WF, Chen NY, Zhang N, Hu GQ, Xie FY, Sun Y, et al. Concurrent chemoradiotherapy with/without induction chemotherapy in locoregionally advanced nasopharyngeal carcinoma: Long-term results of phase 3 randomized controlled trial. Int J Cancer (2019) 145(1):295–305. doi: 10.1002/ijc.32099
31. Tang QN, Liu LT, Qi B, Guo SS, Luo DH, Sun R, et al. Effect of concurrent chemoradiotherapy with nedaplatin vs cisplatin on the long-term outcomes of survival and toxic effects among patients with stage II to IVB nasopharyngeal carcinoma: A 5-year follow-up secondary analysis of a randomized clinical trial. JAMA Netw Open (2021) 4(12):e2138470. doi: 10.1001/jamanetworkopen.2021.38470
32. Yang Q, Cao SM, Guo L, Hua YJ, Huang PY, Zhang XL, et al. Induction chemotherapy followed by concurrent chemoradiotherapy versus concurrent chemoradiotherapy alone in locoregionally advanced nasopharyngeal carcinoma: long-term results of a phase III multicentre randomised controlled trial. Eur J Cancer (2019) 119:87–96. doi: 10.1016/j.ejca.2019.07.007
33. Chitapanarux I, Lorvidhaya V, Kamnerdsupaphon P, Sumitsawan Y, Tharavichitkul E, Sukthomya V, et al. Chemoradiation comparing cisplatin versus carboplatin in locally advanced nasopharyngeal cancer: randomised, non-inferiority, open trial. Eur J Cancer (2007) 43(9):1399–406. doi: 10.1016/j.ejca.2007.03.022
34. Chen YP, Chan ATC, Le QT, Blanchard P, Sun Y, Ma J. Nasopharyngeal carcinoma. Lancet (2019) 394(10192):64–80. doi: 10.1016/S0140-6736(19)30956-0
35. Bossi P, Chan AT, Licitra L, Trama A, Orlandi E, Hui EP, et al. Nasopharyngeal carcinoma: ESMO-EURACAN clinical practice guidelines for diagnosis, treatment and follow-up(dagger). Ann Oncol (2021) 32(4):452–65. doi: 10.1016/j.annonc.2020.12.007
36. Le QT, Colevas AD, O'Sullivan B, Lee AWM, Lee N, Ma B, et al. Current treatment landscape of nasopharyngeal carcinoma and potential trials evaluating the value of immunotherapy. J Natl Cancer Inst (2019) 111(7):655–63. doi: 10.1093/jnci/djz044
37. Chen YP, Lv JW, Mao YP, Li XM, Li JY, Wang YQ, et al. Unraveling tumour microenvironment heterogeneity in nasopharyngeal carcinoma identifies biologically distinct immune subtypes predicting prognosis and immunotherapy responses. Mol Cancer (2021) 20(1):14. doi: 10.1186/s12943-020-01292-5
38. Toner K, Bollard CM. EBV+ lymphoproliferative diseases: opportunities for leveraging EBV as a therapeutic target. Blood (2022) 139(7):983–94. doi: 10.1182/blood.2020005466
39. Taylor GS, Long HM, Brooks JM, Rickinson AB, Hislop AD. The immunology of Epstein-Barr virus-induced disease. Annu Rev Immunol (2015) 33:787–821. doi: 10.1146/annurev-immunol-032414-112326
40. Akiyama M, Suzuki K, Yoshimoto K, Yasuoka H, Kaneko Y, Takeuchi T. Peripheral TIGIT+ T follicular helper cells that produce high levels of interleukin-21 via OX40 represent disease activity in IgG4-related disease. Front Immunol (2021) 12:651357. doi: 10.3389/fimmu.2021.651357
41. Rowe M, Kelly GL, Bell AI, Rickinson AB. Burkitt's lymphoma: the Rosetta stone deciphering Epstein-Barr virus biology. Semin Cancer Biol (2009) 19(6):377–88. doi: 10.1016/j.semcancer.2009.07.004
42. Agergaard J, Larsen CS. Acute acalculous cholecystitis in a patient with primary Epstein-Barr virus infection: a case report and literature review. Int J Infect Dis (2015) 35:67–72. doi: 10.1016/j.ijid.2015.04.004
43. Dasari V, Sinha D, Neller MA, Smith C, Khanna R. Prophylactic and therapeutic strategies for Epstein-Barr virus-associated diseases: emerging strategies for clinical development. Expert Rev Vaccines (2019) 18(5):457–74. doi: 10.1080/14760584.2019.1605906
44. Okano M, Gross TG. Advanced therapeutic and prophylactic strategies for Epstein-Barr virus infection in immunocompromised patients. Expert Rev Anti Infect Ther (2007) 5(3):403–13. doi: 10.1586/14787210.5.3.403
45. Cui X, Snapper CM. Epstein Barr Virus: Development of vaccines and immune cell therapy for EBV-associated diseases. Front Immunol (2021) 12:734471. doi: 10.3389/fimmu.2021.734471
46. Munz C, Bickham KL, Subklewe M, Tsang ML, Chahroudi A, Kurilla MG, et al. Human CD4(+) T lymphocytes consistently respond to the latent Epstein-Barr virus nuclear antigen EBNA1. J Exp Med (2000) 191(10):1649–60. doi: 10.1084/jem.191.10.1649
47. Fu T, Voo KS, Wang RF. Critical role of EBNA1-specific CD4+ T cells in the control of mouse burkitt lymphoma in vivo. J Clin Invest (2004) 114(4):542–50. doi: 10.1172/JCI22053
48. Tellam J, Connolly G, Green KJ, Miles JJ, Moss DJ, Burrows SR, et al. Endogenous presentation of CD8+ T cell epitopes from Epstein-Barr virus-encoded nuclear antigen 1. J Exp Med (2004) 199(10):1421–31. doi: 10.1084/jem.20040191
49. Brooks L, Yao QY, Rickinson AB, Young LS. Epstein-Barr Virus latent gene transcription in nasopharyngeal carcinoma cells: coexpression of EBNA1, LMP1, and LMP2 transcripts. J Virol (1992) 66(5):2689–97. doi: 10.1128/JVI.66.5.2689-2697.1992
50. Straathof KC, Leen AM, Buza EL, Taylor G, Huls MH, Heslop HE, et al. Characterization of latent membrane protein 2 specificity in CTL lines from patients with EBV-positive nasopharyngeal carcinoma and lymphoma. J Immunol (2005) 175(6):4137–47. doi: 10.4049/jimmunol.175.6.4137
51. Jean-Pierre V, Lupo J, Buisson M, Morand P, Germi R. Main targets of interest for the development of a prophylactic or therapeutic Epstein-Barr virus vaccine. Front Microbiol (2021) 12:701611. doi: 10.3389/fmicb.2021.701611
52. Han D, Xu Y, Zhao X, Mao Y, Kang Q, Wen W, et al. A novel human anti-TIGIT monoclonal antibody with excellent function in eliciting NK cell-mediated antitumor immunity. Biochem Biophys Res Commun (2021) 534:134–40. doi: 10.1016/j.bbrc.2020.12.013
53. Cohen JI. Vaccine development for Epstein-Barr virus. Adv Exp Med Biol (2018) 1045:477–93. doi: 10.1007/978-981-10-7230-7_22
54. Zuo LL, Zhu MJ, Du SJ, Lu JH, Li GY. [The entry of Epstein-Barr virus into b lymphocytes and epithelial cells during infection]. Bing Du Xue Bao (2014) 30(4):476–82.
55. Shannon-Lowe C, Rowe M. Epstein Barr Virus entry; kissing and conjugation. Curr Opin Virol (2014) 4:78–84. doi: 10.1016/j.coviro.2013.12.001
56. Bi J, Zheng X, Chen Y, Wei H, Sun R, Tian Z. TIGIT safeguards liver regeneration through regulating natural killer cell-hepatocyte crosstalk. Hepatology (2014) 60(4):1389–98. doi: 10.1002/hep.27245
57. Ruiss R, Jochum S, Mocikat R, Hammerschmidt W, Zeidler R. EBV-gp350 confers b-cell tropism to tailored exosomes and is a neo-antigen in normal and malignant b cells–a new option for the treatment of b-CLL. PloS One (2011) 6(10):e25294. doi: 10.1371/journal.pone.0025294
58. Chen H, Zhang X, Zhang S, Duan X, Xiang T, Zhou X, et al. T Cell epitope screening of Epstein-Barr virus fusion protein gB. J Virol (2021), 95(10):e00081–21. doi: 10.1128/JVI.00081-21
59. Gu SY, Huang TM, Ruan L, Miao YH, Lu H, Chu CM, et al. First EBV vaccine trial in humans using recombinant vaccinia virus expressing the major membrane antigen. Dev Biol Stand (1995) 84:171–7.
60. Moutschen M, Leonard P, Sokal EM, Smets F, Haumont M, Mazzu P, et al. Phase I/II studies to evaluate safety and immunogenicity of a recombinant gp350 Epstein-Barr virus vaccine in healthy adults. Vaccine (2007) 25(24):4697–705. doi: 10.1016/j.vaccine.2007.04.008
61. Sokal EM, Hoppenbrouwers K, Vandermeulen C, Moutschen M, Leonard P, Moreels A, et al. Recombinant gp350 vaccine for infectious mononucleosis: a phase 2, randomized, double-blind, placebo-controlled trial to evaluate the safety, immunogenicity, and efficacy of an Epstein-Barr virus vaccine in healthy young adults. J Infect Dis (2007) 196(12):1749–53. doi: 10.1086/523813
62. Elliott SL, Suhrbier A, Miles JJ, Lawrence G, Pye SJ, Le TT, et al. Phase I trial of a CD8+ T-cell peptide epitope-based vaccine for infectious mononucleosis. J Virol (2008) 82(3):1448–57. doi: 10.1128/JVI.01409-07
63. Rees L, Tizard EJ, Morgan AJ, Cubitt WD, Finerty S, Oyewole-Eletu TA, et al. A phase I trial of epstein-barr virus gp350 vaccine for children with chronic kidney disease awaiting transplantation. Transplantation (2009) 88(8):1025–9. doi: 10.1097/TP.0b013e3181b9d918
64. Chia WK, Wang WW, Teo M, Tai WM, Lim WT, Tan EH, et al. A phase II study evaluating the safety and efficacy of an adenovirus-DeltaLMP1-LMP2 transduced dendritic cell vaccine in patients with advanced metastatic nasopharyngeal carcinoma. Ann Oncol (2012) 23(4):997–1005. doi: 10.1093/annonc/mdr341
65. Smith C, Tsang J, Beagley L, Chua D, Lee V, Li V, et al. Effective treatment of metastatic forms of Epstein-Barr virus-associated nasopharyngeal carcinoma with a novel adenovirus-based adoptive immunotherapy. Cancer Res (2012) 72(5):1116–25. doi: 10.1158/0008-5472.CAN-11-3399
66. Li F, Song D, Lu Y, Zhu H, Chen Z, He X. Delayed-type hypersensitivity (DTH) immune response related with EBV-DNA in nasopharyngeal carcinoma treated with autologous dendritic cell vaccination after radiotherapy. J Immunother (2013) 36(3):208–14. doi: 10.1097/CJI.0b013e31828bd87b
67. Hui EP, Taylor GS, Jia H, Ma BB, Chan SL, Ho R, et al. Phase I trial of recombinant modified vaccinia ankara encoding Epstein-Barr viral tumor antigens in nasopharyngeal carcinoma patients. Cancer Res (2013) 73(6):1676–88. doi: 10.1158/0008-5472.CAN-12-2448
68. Taylor GS, Jia H, Harrington K, Lee LW, Turner J, Ladell K, et al. A recombinant modified vaccinia ankara vaccine encoding Epstein-Barr virus (EBV) target antigens: a phase I trial in UK patients with EBV-positive cancer. Clin Cancer Res (2014) 20(19):5009–22. doi: 10.1158/1078-0432.CCR-14-1122-T
69. Si Y, Deng Z, Lan G, Du H, Wang Y, Si J, et al. The safety and immunological effects of rAd5-EBV-LMP2 vaccine in nasopharyngeal carcinoma patients: A phase I clinical trial and two-year follow-up. Chem Pharm Bull (Tokyo) (2016) 64(8):1118–23. doi: 10.1248/cpb.c16-00114
70. Morgan AJ, Epstein MA, North JR. Comparative immunogenicity studies on Epstein-Barr virus membrane antigen (MA) gp340 with novel adjuvants in mice, rabbits, and cotton-top tamarins. J Med Virol (1984) 13(3):281–92. doi: 10.1002/jmv.1890130310
71. Perez EM, Foley J, Tison T, Silva R, Ogembo JG. Novel Epstein-Barr virus-like particles incorporating gH/gL-EBNA1 or gB-LMP2 induce high neutralizing antibody titers and EBV-specific T-cell responses in immunized mice. Oncotarget (2017) 8(12):19255–73. doi: 10.18632/oncotarget.13770
72. Plate AE, Reimer JJ, Jardetzky TS, Longnecker R. Mapping regions of Epstein-Barr virus (EBV) glycoprotein b (gB) important for fusion function with gH/gL. Virology (2011) 413(1):26–38. doi: 10.1016/j.virol.2010.12.006
73. Liu W, Chen YH. High epitope density in a single protein molecule significantly enhances antigenicity as well as immunogenicity: a novel strategy for modern vaccine development and a preliminary investigation about b cell discrimination of monomeric proteins. Eur J Immunol (2005) 35(2):505–14. doi: 10.1002/eji.200425749
74. Ilyinskii PO, Thoidis G, Sherman MY, Shneider A. Adjuvant potential of aggregate-forming polyglutamine domains. Vaccine (2008) 26(26):3223–6. doi: 10.1016/j.vaccine.2008.03.078
75. van Beers MM, Jiskoot W, Schellekens H. On the role of aggregates in the immunogenicity of recombinant human interferon beta in patients with multiple sclerosis. J Interferon Cytokine Res (2010) 30(10):767–75. doi: 10.1089/jir.2010.0086
76. Cui X, Cao Z, Sen G, Chattopadhyay G, Fuller DH, Fuller JT, et al. A novel tetrameric gp350 1-470 as a potential Epstein-Barr virus vaccine. Vaccine (2013) 31(30):3039–45. doi: 10.1016/j.vaccine.2013.04.071
77. Cui X, Cao Z, Chen Q, Arjunaraja S, Snow AL, Snapper CM. Rabbits immunized with Epstein-Barr virus gH/gL or gB recombinant proteins elicit higher serum virus neutralizing activity than gp350. Vaccine (2016) 34(34):4050–5. doi: 10.1016/j.vaccine.2016.06.021
78. Cui X, Cao Z, Ishikawa Y, Cui S, Imadome KI, Snapper CM. Immunization with Epstein-Barr virus core fusion machinery envelope proteins elicit high titers of neutralizing activities and protect humanized mice from lethal dose EBV challenge. Vaccines (Basel) (2021) 9(3):285. doi: 10.3390/vaccines9030285
79. Mohsen MO, Zha L, Cabral-Miranda G, Bachmann MF. Major findings and recent advances in virus-like particle (VLP)-based vaccines. Semin Immunol (2017) 34:123–32. doi: 10.1016/j.smim.2017.08.014
80. Zhang X, Hong J, Zhong L, Wu Q, Zhang S, Zhu Q, et al. Protective anti-gB neutralizing antibodies targeting two vulnerable sites for EBV-cell membrane fusion. Proc Natl Acad Sci U.S.A. (2022) 119(32):e2202371119. doi: 10.1073/pnas.2202371119
81. Petrosky E, Bocchini JA Jr., Hariri S, Chesson H, Curtis CR, Saraiya M, et al. Use of 9-valent human papillomavirus (HPV) vaccine: updated HPV vaccination recommendations of the advisory committee on immunization practices. MMWR Morb Mortal Wkly Rep (2015) 64(11):300–4.
82. Roden RBS, Stern PL. Opportunities and challenges for human papillomavirus vaccination in cancer. Nat Rev Cancer (2018) 18(4):240–54. doi: 10.1038/nrc.2018.13
83. Mobini S, Chizari M, Mafakher L, Rismani E, Rismani E. Computational design of a novel VLP-based vaccine for hepatitis b virus. Front Immunol (2020) 11:2074. doi: 10.3389/fimmu.2020.02074
84. Ruiss R, Jochum S, Wanner G, Reisbach G, Hammerschmidt W, Zeidler R. A virus-like particle-based Epstein-Barr virus vaccine. J Virol (2011) 85(24):13105–13. doi: 10.1128/JVI.05598-11
85. Ogembo JG, Muraswki MR, McGinnes LW, Parcharidou A, Sutiwisesak R, Tison T, et al. A chimeric EBV gp350/220-based VLP replicates the virion b-cell attachment mechanism and elicits long-lasting neutralizing antibodies in mice. J Transl Med (2015) 13:50. doi: 10.1186/s12967-015-0415-2
86. Hartlage AS, Liu T, Patton JT, Garman SL, Zhang X, Kurt H, et al. The Epstein-Barr virus lytic protein BZLF1 as a candidate target antigen for vaccine development. Cancer Immunol Res (2015) 3(7):787–94. doi: 10.1158/2326-6066.CIR-14-0242
87. Balfour HH Jr. Progress, prospects, and problems in Epstein-Barr virus vaccine development. Curr Opin Virol (2014) 6:1–5. doi: 10.1016/j.coviro.2014.02.005
88. Sun C, Chen XC, Kang YF, Zeng MS. The status and prospects of Epstein-Barr virus prophylactic vaccine development. Front Immunol (2021) 12:677027. doi: 10.3389/fimmu.2021.677027
89. Lin CL, Lo WF, Lee TH, Ren Y, Hwang SL, Cheng YF, et al. Immunization with Epstein-Barr virus (EBV) peptide-pulsed dendritic cells induces functional CD8+ T-cell immunity and may lead to tumor regression in patients with EBV-positive nasopharyngeal carcinoma. Cancer Res (2002) 62(23):6952–8.
90. Ruhl J, Citterio C, Engelmann C, Haigh T, Dzionek A, Dreyer J, et al. Heterologous prime-boost vaccination protects against EBV antigen-expressing lymphomas. J Clin Invest (2019) 129(5):2071–87. doi: 10.1172/JCI125364
91. Sharma S, Rouce RH. Are we there yet? the never-ending quest for an Epstein-Barr virus vaccine. J Clin Invest (2019) 129(5):1836–8. doi: 10.1172/JCI128370
92. Taylor GS, Haigh TA, Gudgeon NH, Phelps RJ, Lee SP, Steven NM, et al. Dual stimulation of Epstein-Barr virus (EBV)-specific CD4+- and CD8+-t-cell responses by a chimeric antigen construct: potential therapeutic vaccine for EBV-positive nasopharyngeal carcinoma. J Virol (2004) 78(2):768–78. doi: 10.1128/jvi.78.2.768-778.2004
93. Savoldo B, Goss JA, Hammer MM, Zhang L, Lopez T, Gee AP, et al. Treatment of solid organ transplant recipients with autologous Epstein Barr virus-specific cytotoxic T lymphocytes (CTLs). Blood (2006) 108(9):2942–9. doi: 10.1182/blood-2006-05-021782
94. Comoli P, Labirio M, Basso S, Baldanti F, Grossi P, Furione M, et al. Infusion of autologous Epstein-Barr virus (EBV)-specific cytotoxic T cells for prevention of EBV-related lymphoproliferative disorder in solid organ transplant recipients with evidence of active virus replication. Blood (2002) 99(7):2592–8. doi: 10.1182/blood.v99.7.2592
95. Khanna R, Bell S, Sherritt M, Galbraith A, Burrows SR, Rafter L, et al. Activation and adoptive transfer of Epstein-Barr virus-specific cytotoxic T cells in solid organ transplant patients with posttransplant lymphoproliferative disease. Proc Natl Acad Sci U.S.A. (1999) 96(18):10391–6. doi: 10.1073/pnas.96.18.10391
96. Papadopoulos EB, Ladanyi M, Emanuel D, Mackinnon S, Boulad F, Carabasi MH, et al. Infusions of donor leukocytes to treat Epstein-Barr virus-associated lymphoproliferative disorders after allogeneic bone marrow transplantation. N Engl J Med (1994) 330(17):1185–91. doi: 10.1056/NEJM199404283301703
97. Rickinson AB, Moss DJ. Human cytotoxic T lymphocyte responses to Epstein-Barr virus infection. Annu Rev Immunol (1997) 15:405–31. doi: 10.1146/annurev.immunol.15.1.405
98. Bollard CM, Rooney CM, Heslop HE. T-Cell therapy in the treatment of post-transplant lymphoproliferative disease. Nat Rev Clin Oncol (2012) 9(9):510–9. doi: 10.1038/nrclinonc.2012.111
99. Smith C, Khanna R. Adoptive cellular immunotherapy for virus-associated cancers: a new paradigm in personalized medicine. Immunol Cell Biol (2017) 95(4):364–71. doi: 10.1038/icb.2016.127
100. Dasari V, Bhatt KH, Smith C, Khanna R. Designing an effective vaccine to prevent Epstein-Barr virus-associated diseases: challenges and opportunities. Expert Rev Vaccines (2017) 16(4):377–90. doi: 10.1080/14760584.2017.1293529
101. Heslop HE, Ng CY, Li C, Smith CA, Loftin SK, Krance RA, et al. Long-term restoration of immunity against Epstein-Barr virus infection by adoptive transfer of gene-modified virus-specific T lymphocytes. Nat Med (1996) 2(5):551–5. doi: 10.1038/nm0596-551
102. Rooney CM, Smith CA, Ng CY, Loftin SK, Sixbey JW, Gan Y, et al. Infusion of cytotoxic T cells for the prevention and treatment of Epstein-Barr virus-induced lymphoma in allogeneic transplant recipients. Blood (1998) 92(5):1549–55. doi: 10.1182/blood.V92.5.1549.417k32_1549_1555
103. Smith C, Beagley L, Rehan S, Neller MA, Crooks P, Solomon M, et al. Autologous adoptive T-cell therapy for recurrent or drug-resistant cytomegalovirus complications in solid organ transplant recipients: A single-arm open-label phase I clinical trial. Clin Infect Dis (2019) 68(4):632–40. doi: 10.1093/cid/ciy549
104. Garcia-Rios E, Nuevalos M, Mancebo FJ, Perez-Romero P. Is it feasible to use CMV-specific T-cell adoptive transfer as treatment against infection in SOT recipients? Front Immunol (2021) 12:657144. doi: 10.3389/fimmu.2021.657144
105. Gandhi MK, Lambley E, Duraiswamy J, Dua U, Smith C, Elliott S, et al. Expression of LAG-3 by tumor-infiltrating lymphocytes is coincident with the suppression of latent membrane antigen-specific CD8+ T-cell function in Hodgkin lymphoma patients. Blood (2006) 108(7):2280–9. doi: 10.1182/blood-2006-04-015164
106. Smith C, Beagley L, Khanna R. Acquisition of polyfunctionality by Epstein-Barr virus-specific CD8+ T cells correlates with increased resistance to galectin-1-mediated suppression. J Virol (2009) 83(12):6192–8. doi: 10.1128/JVI.00239-09
107. Comoli P, Pedrazzoli P, Maccario R, Basso S, Carminati O, Labirio M, et al. Cell therapy of stage IV nasopharyngeal carcinoma with autologous Epstein-Barr virus-targeted cytotoxic T lymphocytes. J Clin Oncol (2005) 23(35):8942–9. doi: 10.1200/JCO.2005.02.6195
108. Louis CU, Straathof K, Bollard CM, Ennamuri S, Gerken C, Lopez TT, et al. Adoptive transfer of EBV-specific T cells results in sustained clinical responses in patients with locoregional nasopharyngeal carcinoma. J Immunother (2010) 33(9):983–90. doi: 10.1097/CJI.0b013e3181f3cbf4
109. Louis CU, Straathof K, Bollard CM, Gerken C, Huls MH, Gresik MV, et al. Enhancing the in vivo expansion of adoptively transferred EBV-specific CTL with lymphodepleting CD45 monoclonal antibodies in NPC patients. Blood (2009) 113(11):2442–50. doi: 10.1182/blood-2008-05-157222
110. Cho HI, Hong YS, Lee MA, Kim EK, Yoon SH, Kim CC, et al. Adoptive transfer of Epstein-Barr virus-specific cytotoxic T-lymphocytes for the treatment of angiocentric lymphomas. Int J Hematol (2006) 83(1):66–73. doi: 10.1532/IJH97.A30505
111. Smith C, Lee V, Schuessler A, Beagley L, Rehan S, Tsang J, et al. Pre-emptive and therapeutic adoptive immunotherapy for nasopharyngeal carcinoma: Phenotype and effector function of T cells impact on clinical response. Oncoimmunology (2017) 6(2):e1273311. doi: 10.1080/2162402X.2016.1273311
112. Chua D, Huang J, Zheng B, Lau SY, Luk W, Kwong DL, et al. Adoptive transfer of autologous Epstein-Barr virus-specific cytotoxic T cells for nasopharyngeal carcinoma. Int J Cancer (2001) 94(1):73–80. doi: 10.1002/ijc.1430
113. Comoli P, De Palma R, Siena S, Nocera A, Basso S, Del Galdo F, et al. Adoptive transfer of allogeneic Epstein-Barr virus (EBV)-specific cytotoxic T cells with in vitro antitumor activity boosts LMP2-specific immune response in a patient with EBV-related nasopharyngeal carcinoma. Ann Oncol (2004) 15(1):113–7. doi: 10.1093/annonc/mdh027
114. Straathof KC, Bollard CM, Popat U, Huls MH, Lopez T, Morriss MC, et al. Treatment of nasopharyngeal carcinoma with Epstein-Barr virus–specific T lymphocytes. Blood (2005) 105(5):1898–904. doi: 10.1182/blood-2004-07-2975
115. Secondino S, Zecca M, Licitra L, Gurrado A, Schiavetto I, Bossi P, et al. T-Cell therapy for EBV-associated nasopharyngeal carcinoma: preparative lymphodepleting chemotherapy does not improve clinical results. Ann Oncol (2012) 23(2):435–41. doi: 10.1093/annonc/mdr134
116. Chia WK, Teo M, Wang WW, Lee B, Ang SF, Tai WM, et al. Adoptive T-cell transfer and chemotherapy in the first-line treatment of metastatic and/or locally recurrent nasopharyngeal carcinoma. Mol Ther (2014) 22(1):132–9. doi: 10.1038/mt.2013.242
117. Lutzky VP, Crooks P, Morrison L, Stevens N, Davis JE, Corban M, et al. Cytotoxic T cell adoptive immunotherapy as a treatment for nasopharyngeal carcinoma. Clin Vaccine Immunol (2014) 21(2):256–9. doi: 10.1128/CVI.00121-13
118. Huang J, Fogg M, Wirth LJ, Daley H, Ritz J, Posner MR, et al. Epstein-Barr Virus-specific adoptive immunotherapy for recurrent, metastatic nasopharyngeal carcinoma. Cancer (2017) 123(14):2642–50. doi: 10.1002/cncr.30541
119. Duraiswamy J, Bharadwaj M, Tellam J, Connolly G, Cooper L, Moss D, et al. Induction of therapeutic T-cell responses to subdominant tumor-associated viral oncogene after immunization with replication-incompetent polyepitope adenovirus vaccine. Cancer Res (2004) 64(4):1483–9. doi: 10.1158/0008-5472.can-03-2196
120. Smith C, Cooper L, Burgess M, Rist M, Webb N, Lambley E, et al. Functional reversion of antigen-specific CD8+ T cells from patients with Hodgkin lymphoma following in vitro stimulation with recombinant polyepitope. J Immunol (2006) 177(7):4897–906. doi: 10.4049/jimmunol.177.7.4897
121. Russi RC, Del Balzo D, Lujan A, Reidel IG, Garcia MI, Veaute C, et al. Heterologous prime-boost vaccination based on polymorphic protein d protects against intravaginal chlamydia trachomatis infection in mice. Sci Rep (2022) 12(1):6664. doi: 10.1038/s41598-022-10633-x
122. Zhang H, Ye ZL, Yuan ZG, Luo ZQ, Jin HJ, Qian QJ. New strategies for the treatment of solid tumors with CAR-T cells. Int J Biol Sci (2016) 12(6):718–29. doi: 10.7150/ijbs.14405
123. Yong CSM, Dardalhon V, Devaud C, Taylor N, Darcy PK, Kershaw MH. CAR T-cell therapy of solid tumors. Immunol Cell Biol (2017) 95(4):356–63. doi: 10.1038/icb.2016.128
124. Patel U, Abernathy J, Savani BN, Oluwole O, Sengsayadeth S, Dholaria B. CAR T cell therapy in solid tumors: A review of current clinical trials. EJHaem (2022) 3(Suppl 1):24–31. doi: 10.1002/jha2.356
125. Tang X, Tang Q, Mao Y, Huang X, Jia L, Zhu J, et al. CD137 Co-stimulation improves the antitumor effect of LMP1-specific chimeric antigen receptor T cells In vitro and in vivo. Onco Targets Ther (2019) 12:9341–50. doi: 10.2147/OTT.S221040
126. Tang X, Zhou Y, Li W, Tang Q, Chen R, Zhu J, et al. T Cells expressing a LMP1-specific chimeric antigen receptor mediate antitumor effects against LMP1-positive nasopharyngeal carcinoma cells in vitro and in vivo. J BioMed Res (2014) 28(6):468–75. doi: 10.7555/JBR.28.20140066
127. Hafezi M, Lin M, Chia A, Chua A, Ho ZZ, Fam R, et al. Immunosuppressive drug-resistant armored T-cell receptor T cells for immune therapy of HCC in liver transplant patients. Hepatology (2021) 74(1):200–13. doi: 10.1002/hep.31662
128. Tsimberidou AM, Van Morris K, Vo HH, Eck S, Lin YF, Rivas JM, et al. T-Cell receptor-based therapy: an innovative therapeutic approach for solid tumors. J Hematol Oncol (2021) 14(1):102. doi: 10.1186/s13045-021-01115-0
129. Nagarsheth NB, Norberg SM, Sinkoe AL, Adhikary S, Meyer TJ, Lack JB, et al. TCR-engineered T cells targeting E7 for patients with metastatic HPV-associated epithelial cancers. Nat Med (2021) 27(3):419–25. doi: 10.1038/s41591-020-01225-1
130. Yang D, Shao Q, Sun H, Mu X, Gao Y, Jiang R, et al. Evaluation of Epstein-Barr virus latent membrane protein 2 specific T-cell receptors driven by T-cell specific promoters using lentiviral vector. Clin Dev Immunol (2011) 2011:716926. doi: 10.1155/2011/716926
131. Cho HI, Kim UH, Shin AR, Won JN, Lee HJ, Sohn HJ, et al. A novel Epstein-Barr virus-latent membrane protein-1-specific T-cell receptor for TCR gene therapy. Br J Cancer (2018) 118(4):534–45. doi: 10.1038/bjc.2017.475
132. Chang ET, Adami HO. The enigmatic epidemiology of nasopharyngeal carcinoma. Cancer Epidemiol Biomarkers Prev (2006) 15(10):1765–77. doi: 10.1158/1055-9965.EPI-06-0353
133. Feng RM, Zong YN, Cao SM, Xu RH. Current cancer situation in China: good or bad news from the 2018 global cancer statistics? Cancer Commun (Lond) (2019) 39(1):22. doi: 10.1186/s40880-019-0368-6
134. Ngan HL, Wang L, Lo KW, Lui VWY. Genomic landscapes of EBV-associated nasopharyngeal carcinoma vs. HPV-Associated Head Neck Cancer. Cancers (Basel) (2018) 10(7):210. doi: 10.3390/cancers10070210
135. Ou SI, Zell JA, Ziogas A, Anton-Culver H. Epidemiology of nasopharyngeal carcinoma in the united states: improved survival of Chinese patients within the keratinizing squamous cell carcinoma histology. Ann Oncol (2007) 18(1):29–35. doi: 10.1093/annonc/mdl320
136. Burt RD, Vaughan TL, McKnight B. Descriptive epidemiology and survival analysis of nasopharyngeal carcinoma in the united states. Int J Cancer (1992) 52(4):549–56. doi: 10.1002/ijc.2910520409
137. Prawira A, Oosting SF, Chen TW, Delos Santos KA, Saluja R, Wang L, et al. Systemic therapies for recurrent or metastatic nasopharyngeal carcinoma: a systematic review. Br J Cancer (2017) 117(12):1743–52. doi: 10.1038/bjc.2017.357
138. Hong S, Zhang Y, Yu G, Peng P, Peng J, Jia J, et al. Gemcitabine plus cisplatin versus fluorouracil plus cisplatin as first-line therapy for recurrent or metastatic nasopharyngeal carcinoma: Final overall survival analysis of GEM20110714 phase III study. J Clin Oncol (2021) 39(29):3273–82. doi: 10.1200/JCO.21.00396
139. Chen X, Liang W, Wan N, Zhang L, Yang Y, Jiang J, et al. Cost-effectiveness analysis of gemcitabine plus cisplatin versus fluorouracil plus cisplatin for first-line treatment of recurrent or metastatic nasopharyngeal carcinoma. Oral Oncol (2019) 94:80–5. doi: 10.1016/j.oraloncology.2019.04.022
140. Zhang L, Huang Y, Hong S, Yang Y, Yu G, Jia J, et al. Gemcitabine plus cisplatin versus fluorouracil plus cisplatin in recurrent or metastatic nasopharyngeal carcinoma: a multicentre, randomised, open-label, phase 3 trial. Lancet (2016) 388(10054):1883–92. doi: 10.1016/S0140-6736(16)31388-5
141. Zhang J, Fang W, Qin T, Yang Y, Hong S, Liang W, et al. Co-Expression of PD-1 and PD-L1 predicts poor outcome in nasopharyngeal carcinoma. Med Oncol (2015) 32(3):86. doi: 10.1007/s12032-015-0501-6
142. Bi XW, Wang H, Zhang WW, Wang JH, Liu WJ, Xia ZJ, et al. PD-L1 is upregulated by EBV-driven LMP1 through NF-kappaB pathway and correlates with poor prognosis in natural killer/T-cell lymphoma. J Hematol Oncol (2016) 9(1):109. doi: 10.1186/s13045-016-0341-7
143. Moon JW, Kong SK, Kim BS, Kim HJ, Lim H, Noh K, et al. IFNgamma induces PD-L1 overexpression by JAK2/STAT1/IRF-1 signaling in EBV-positive gastric carcinoma. Sci Rep (2017) 7(1):17810. doi: 10.1038/s41598-017-18132-0
144. Anastasiadou E, Stroopinsky D, Alimperti S, Jiao AL, Pyzer AR, Cippitelli C, et al. Epstein-Barr Virus-encoded EBNA2 alters immune checkpoint PD-L1 expression by downregulating miR-34a in b-cell lymphomas. Leukemia (2019) 33(1):132–47. doi: 10.1038/s41375-018-0178-x
145. Ma BBY, Lim WT, Goh BC, Hui EP, Lo KW, Pettinger A, et al. Antitumor activity of nivolumab in recurrent and metastatic nasopharyngeal carcinoma: An international, multicenter study of the Mayo clinic phase 2 consortium (NCI-9742). J Clin Oncol (2018) 36(14):1412–8. doi: 10.1200/JCO.2017.77.0388
146. Doi T, Piha-Paul SA, Jalal SI, Saraf S, Lunceford J, Koshiji M, et al. Safety and antitumor activity of the anti-programmed death-1 antibody pembrolizumab in patients with advanced esophageal carcinoma. J Clin Oncol (2018) 36(1):61–7. doi: 10.1200/JCO.2017.74.9846
147. Hsu C, Lee SH, Ejadi S, Even C, Cohen RB, Le Tourneau C, et al. Safety and antitumor activity of pembrolizumab in patients with programmed death-ligand 1-positive nasopharyngeal carcinoma: Results of the KEYNOTE-028 study. J Clin Oncol (2017) 35(36):4050–6. doi: 10.1200/JCO.2017.73.3675
148. Fang W, Yang Y, Ma Y, Hong S, Lin L, He X, et al. Camrelizumab (SHR-1210) alone or in combination with gemcitabine plus cisplatin for nasopharyngeal carcinoma: results from two single-arm, phase 1 trials. Lancet Oncol (2018) 19(10):1338–50. doi: 10.1016/S1470-2045(18)30495-9
149. Mansfield AS, Herbst RS, de Castro G Jr., Hui R, Peled N, Kim DW, et al. Outcomes with pembrolizumab monotherapy in patients with programmed death-ligand 1-positive NSCLC with brain metastases: Pooled analysis of KEYNOTE-001, 010, 024, and 042. JTO Clin Res Rep (2021) 2(8):100205. doi: 10.1016/j.jtocrr.2021.100205
150. Nishio M, Takahashi T, Yoshioka H, Nakagawa K, Fukuhara T, Yamada K, et al. KEYNOTE-025: Phase 1b study of pembrolizumab in Japanese patients with previously treated programmed death ligand 1-positive advanced non-small-cell lung cancer. Cancer Sci (2019) 110(3):1012–20. doi: 10.1111/cas.13932
151. Varga A, Piha-Paul S, Ott PA, Mehnert JM, Berton-Rigaud D, Morosky A, et al. Pembrolizumab in patients with programmed death ligand 1-positive advanced ovarian cancer: Analysis of KEYNOTE-028. Gynecol Oncol (2019) 152(2):243–50. doi: 10.1016/j.ygyno.2018.11.017
152. Smith C, McGrath M, Neller MA, Matthews KK, Crooks P, Le Texier L, et al. Complete response to PD-1 blockade following EBV-specific T-cell therapy in metastatic nasopharyngeal carcinoma. NPJ Precis Oncol (2021) 5(1):24. doi: 10.1038/s41698-021-00162-7
153. Yang Y, Qu S, Li J, Hu C, Xu M, Li W, et al. Camrelizumab versus placebo in combination with gemcitabine and cisplatin as first-line treatment for recurrent or metastatic nasopharyngeal carcinoma (CAPTAIN-1st): a multicentre, randomised, double-blind, phase 3 trial. Lancet Oncol (2021) 22(8):1162–74. doi: 10.1016/S1470-2045(21)00302-8
154. Wei XL, Ren C, Wang FH, Zhang Y, Zhao HY, Zou BY, et al. A phase I study of toripalimab, an anti-PD-1 antibody, in patients with refractory malignant solid tumors. Cancer Commun (Lond) (2020) 40(8):345–54. doi: 10.1002/cac2.12068
155. Wang FH, Wei XL, Feng J, Li Q, Xu N, Hu XC, et al. Efficacy, safety, and correlative biomarkers of toripalimab in previously treated recurrent or metastatic nasopharyngeal carcinoma: A phase II clinical trial (POLARIS-02). J Clin Oncol (2021) 39(7):704–12. doi: 10.1200/JCO.20.02712
156. Van Coillie S, Wiernicki B, Xu J. Molecular and cellular functions of CTLA-4. Adv Exp Med Biol (2020) 1248:7–32. doi: 10.1007/978-981-15-3266-5_2
157. Lingel H, Brunner-Weinzierl MC. CTLA-4 (CD152): A versatile receptor for immune-based therapy. Semin Immunol (2019) 42:101298. doi: 10.1016/j.smim.2019.101298
158. Olson DJ, Eroglu Z, Brockstein B, Poklepovic AS, Bajaj M, Babu S, et al. Pembrolizumab plus ipilimumab following anti-PD-1/L1 failure in melanoma. J Clin Oncol (2021) 39(24):2647–55. doi: 10.1200/JCO.21.00079
159. Robert C, Ribas A, Schachter J, Arance A, Grob JJ, Mortier L, et al. Pembrolizumab versus ipilimumab in advanced melanoma (KEYNOTE-006): post-hoc 5-year results from an open-label, multicentre, randomised, controlled, phase 3 study. Lancet Oncol (2019) 20(9):1239–51. doi: 10.1016/S1470-2045(19)30388-2
160. Janjigian YY, Bendell J, Calvo E, Kim JW, Ascierto PA, Sharma P, et al. CheckMate-032 study: Efficacy and safety of nivolumab and nivolumab plus ipilimumab in patients with metastatic esophagogastric cancer. J Clin Oncol (2018) 36(28):2836–44. doi: 10.1200/JCO.2017.76.6212
161. Shitara K, Ajani JA, Moehler M, Garrido M, Gallardo C, Shen L, et al. Nivolumab plus chemotherapy or ipilimumab in gastro-oesophageal cancer. Nature (2022) 603(7903):942–8. doi: 10.1038/s41586-022-04508-4
162. Overman MJ, Lonardi S, Wong KYM, Lenz HJ, Gelsomino F, Aglietta M, et al. Durable clinical benefit with nivolumab plus ipilimumab in DNA mismatch repair-Deficient/Microsatellite instability-high metastatic colorectal cancer. J Clin Oncol (2018) 36(8):773–9. doi: 10.1200/JCO.2017.76.9901
163. Keam SJ. Cadonilimab: First approval. Drugs (2022) 82(12):1333–9. doi: 10.1007/s40265-022-01761-9
164. Moesta AK, Li XY, Smyth MJ. Targeting CD39 in cancer. Nat Rev Immunol (2020) 20(12):739–55. doi: 10.1038/s41577-020-0376-4
Keywords: Epstein-Barr virus, EBV-associated cancer, EBV-directed vaccination, adoptive cell therapy, TCR-T therapy, immune checkpoint inhibitors
Citation: Li W, Duan X, Chen X, Zhan M, Peng H, Meng Y, Li X, Li X-Y, Pang G and Dou X (2023) Immunotherapeutic approaches in EBV-associated nasopharyngeal carcinoma. Front. Immunol. 13:1079515. doi: 10.3389/fimmu.2022.1079515
Received: 25 October 2022; Accepted: 23 December 2022;
Published: 11 January 2023.
Edited by:
Sha Wu, Southern Medical University, ChinaReviewed by:
Jiang Deng, Institute of Health Service and Transfusion Medicine, ChinaHussein Sultan, Washington University in St. Louis, United States
Copyright © 2023 Li, Duan, Chen, Zhan, Peng, Meng, Li, Li, Pang and Dou. This is an open-access article distributed under the terms of the Creative Commons Attribution License (CC BY). The use, distribution or reproduction in other forums is permitted, provided the original author(s) and the copyright owner(s) are credited and that the original publication in this journal is cited, in accordance with accepted academic practice. No use, distribution or reproduction is permitted which does not comply with these terms.
*Correspondence: Xiaohui Dou, MTM1MjcyNzY2NzFAMTYzLmNvbQ==; Guofu Pang, cGFuZ2d1b2Z1MDEwOTE5QDE2My5jb20=; Xian-Yang Li, bGl4aWFueWFuZ0BvcmljZWxsLmNvbQ==
†These authors have contributed equally to this work