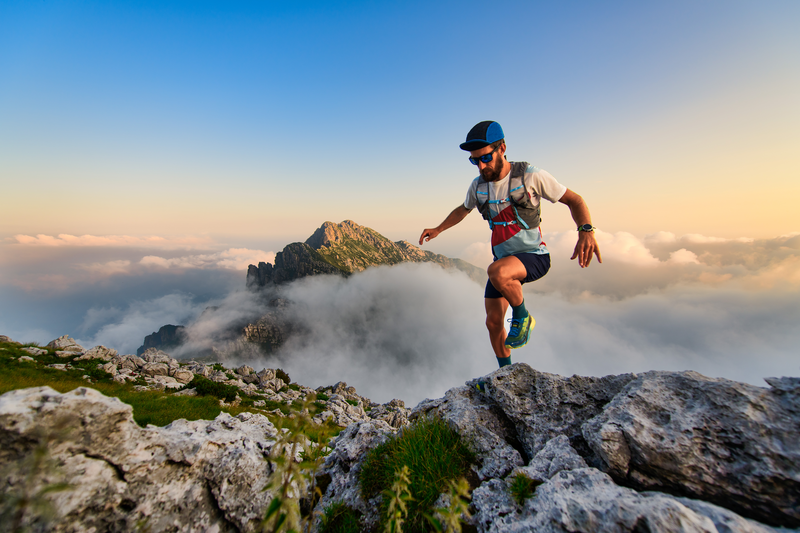
95% of researchers rate our articles as excellent or good
Learn more about the work of our research integrity team to safeguard the quality of each article we publish.
Find out more
ORIGINAL RESEARCH article
Front. Immunol. , 24 November 2022
Sec. Comparative Immunology
Volume 13 - 2022 | https://doi.org/10.3389/fimmu.2022.1074198
This article is part of the Research Topic Aquatic Nutrition and Intestine Immunity View all 18 articles
Tea polysaccharides plays a role in lipid metabolism, antioxidant capacity and immunity of mammals. To investigate the functions of tea polysaccharides on fish, the common carp (Cyprinus carpio L.) was selected as the animal model in this study. In our study, the common carp (45±0.71g) were randomly divided into four groups and were fed fodder with 50% carbohydrate. The common carp were orally administrated with 0 mg/kg BW (control group), 200 mg/kg BW (low-dose group), 400 mg/kg BW (medium-dose group) and 800 mg/kg BW (high-dose group) tea polysaccharide for two week. At the end of experiment, the serum glucose, TG, MDA contents and antioxidase activities were measured by commercial kits. The serum immune factors levels were tested by ELISA. The genes expression levels related to antioxidant capacity, metabolism and immunity were measured by real-time PCR. The results showed that the glucose, TG and MDA contents in serum were significantly decreased by tea polysaccharides treatment. The serum activities of SOD were significantly increased by low-dose tea polysaccharides treatment. The serum activities of GPX were significantly increased by medium-dose tea polysaccharides treatment. The serum levels of IL-1β and TNFα were significantly decreased in the tea polysaccharides treatment group. In the high-dose treatment group, the serum level of TGFβ was significantly increased, and the serum level of IL-12 was markedly decreased. In the hepatopancreas, the expression of acc1, fas, srebp1c, lpl, gys and pparγ were significantly reduced, and the expression of pygl, cat, mnsod, ho-1 and gr were significantly up-regulated in the tea polysaccharides group. In the intestine, the expression of zo-1, occ and gip was significantly up-regulated in the high-dose treatment group. Moreover, the expression of glut2 and sglt1 were significantly down regulated. In the spleen, the expression of il-12, tnfα and il-6 were significantly decreased, and the expression of il-10 and tgfβ was significantly increased by the tea polysaccharides. In the spleen cells, the tea polysaccharides could relieve the LPS-induced immune damage. In conclusion, tea polysaccharides can improve antioxidant capacity, lipid metabolism and immunity of common carp.
Aquaculture provides an essential source of edible protein for humans. With the rapid development of aquaculture, the demand of fishmeal is increasing, which induces the escalating price of fishmeal (1). The study showed that dietary carbohydrate and lipid inclusion at optimal levels could promote protein utilization, prevent lipid oxidation, and save fishmeal protein in the aquatic animal feed (2). However, overloaded dietary carbohydrate induced low feed intake, poor growth performance (3), metabolism dysfunction, impairment of antioxidant capacity and sub-health status of fish (4, 5). Moreover, overloaded dietary carbohydrate will reduce the immunity, and increase the infection with disease of cultured fish (6). In addition, with the expansion of farming scale and environmental degradation, diseases in farming occur frequently, which causes a number of deaths of cultured fish (7). Although the antibiotic can relieve the morbidity of fish, antibiotic resistance has become a severe problem worldwide (8). The frequent occurrence of antibiotic-resistant bacteria in the aquaculture sites is due to the abuse of antibiotics in aquaculture (9). Therefore, the antibiotics were prohibited in aquaculture by the Chinese government because of the various negative effects after July 2020. For these reasons, it is an urgent problem that the new antibiotic substitute has been exploited for use in aquaculture. As the environmental friendly substance, plant extracts are low toxicity, safety, and minimal environmental impacts (10). In aquatic animals, plant extracts play important role in enhancing the immune function, and promote antibacterial, antiviral, antiparasitic activities of the immune system (10). In addition, plant extracts have been as immunostimulant to prevent diseases of aquatic animals in recent years (11).
As the important economic agricultural product, tea possesses multiple beneficial effects, including antioxidant capacity, reduction of cholesterol, protect against cardiovascular disease, anti-microbial, and anti-cancer (12–14). The beneficial effects of tea attribute to its variety of bioactive compounds, including polysaccharides, polyphenols, alkaloids, volatile oils, amino acids, etc (14–18). The tea polysaccharides attracted attention for its bioactivities, such as antioxidant, anti-cancer, anti-radiation, hypoglycemic activities and anti-HIV (14, 15, 19, 20). Tea polysaccharides were mostly heteropolysaccharides, in which a protein via N- or O- covalently linkages carries one or more carbohydrate chains attached to a polypeptide backbone (14, 15). The bioactivity study indicated that the tea polysaccharides suppressed the formation and accumulation of fat, and promoted its decomposition to prevent obesity of rats (Rattus norvegicus) (21). For example, a report of polysaccharides from green tea of Huangshan Maofeng (HMTP) showed that HMTP could protect against liver injury by CCl4-induced, and inhibit lipid peroxidation and the increase antioxidant activity in mice (Mus musculus) (22). Furthermore, the tea polysaccharides could significantly reduce blood glucose levels, and increase the capacity of glucose tolerance in mice (23, 24).
Common carp is one of the most widely cultivated freshwater fish species all over the world, which is the fourth most cultured fish species in aquaculture (25). The production of common carp accounts for 7.7% of the total fish production in 2018 in the world (26). In addition, the production of common carp reaches 4,411,900 metric tons in 2019 (25). To meet the needs of human for fish, the intensive high-density and high nutrition farming model was rapidly developed. With the farming model and environmental degradation, diseases in fish farming occur frequently, which causes huge losses to the aquaculture industry. As a safe and environmental friendly plant extracts, tea polysaccharides have antioxidant capacity, anti-microbial actions and increases immunity in mammals. But the beneficial effects of tea polysaccharides in fish have never been reported. To assess the biological functions of tea polysaccharides on fish, the common carp was employed as a model in this study. The effects of tea polysaccharides on the immunity, metabolism and antioxidant capacity were evaluated in common carp in our study.
Tea polysaccharides was produced in meilunbio (Dalian, China). Glucose test kit was purchased from Rsbio (Shanghai, China). Triglyceride (TG) test kit was purchased from Dongou (Zhejiang, China). Superoxide dismutase (SOD), glutathione oxidase (GPX), total antioxidant capacity (T-AOC) and methane dicarboxylic aldehyde (MDA) test kits were obtained from Nanjing Jiancheng Bioengineering Institute (Nanjing, China).
The experiment process was referred to the previous studies (27, 28). The common carp with an initial body weight (45 ± 0.71g) were obtained from Yanjin Fishery (Yanjin, Henan). Approximately 120 healthy individuals were randomly divided into four groups (30 fish in each group). Before the experiment, fish were acclimated to indoor tanks (diameter: 52 cm, water high: 62cm) at room temperature with recirculating water under a cyclical light-dark photoperiod (12 h: 12 h) for two weeks. Then, the fish were fed with a high carbohydrate diet or the same diet with 200 mg/kg BW/day (low-dose group), 400 mg/kg BW/day (medium-dose group) and 800 mg/kg BW/day (high-dose group) tea polysaccharides by gavage for two weeks. The formulations and compositions of each diet were listed in Table 1. All ingredients were weighted individually before mixed thoroughly in a feed drum mixer for 30 min. Then dissolved water was added and mixed to form a loosely shaped dough. The mixture was transformed into pellets using a single screw extruder (Fishery Machinery and Instrument Research Institute, China Academy of Fishery Science, Shanghai, China); the pellets were then air-dried at room temperature and stored at − 20°C.
At the end of the experiment, all fish were anesthetized by MS222 (Sigma, USA), and the blood samples were collected from the caudal vein. After still standing at 4°C at least 30 min, the serum was isolated by centrifugation at 7500 g for 10 min. The serum was stored at −80°C for the detection of immune factors and biochemical analysis. And then, the fish were decapitated. The hepatopancreas, foregut and spleen samples were immediately collected, and snap-frozen in liquid nitrogen and stored at −80°C until RNA extraction. Parts of the hepatopancreas tissue was removed for glycogen contents measure via the commercial kit (Jiancheng, China). All animal experiments were approved by the Animal Care Committee of Henan Normal University.
In the serum, the content of glucose, TG and MDA of all groups was determined by commercial kits (Jiancheng, China). The enzyme activities of SOD, GPX and T-AOC were determined by commercial kits (Jiancheng, China). The experiments were performed according to the manufacturer’s protocol. The levels of IL-1β, IL-6, IL-10, IL-12, tumor necrosis factor α (TNFα) and transforming growth factor β (TGFβ) were measured by ELISA assay referred to pervious study (28).
The common carp spleen cells were isolated by collagenase IV/DNase II digestion method. The experimental method of isolation was referred to previous study (29). The isolated spleen cells were cultured in the 24 wells plate with 1 mL DMEM/F12 medium contained 10% fetal bovine serum (FBS) with the density of 1×106 cells/well. After overnight cultured, the cell medium was replaced to fresh DMEM/F12 without FBS. Before treatment, the cells were cultured for 1 h in the DMEM/F12 without FBS. Then, the cells were treated with LPS (25 μg/mL), tea polysaccharides (400 μg/mL), LPS (25 μg/mL) + tea polysaccharides (400 μg/mL) for 12 h. By the end of the study, the cells were lysed by RNAiso Plus for RNA extraction.
The total RNA of the hepatopancreas, gut and spleen were extracted by RNAiso Plus (Takara, Janpa). The total RNA concentration was measured by UV spectrophotometer (Nanodrop 2000, Thermo). 1 μg of total RNA was digested with gDNA Eraser at 42°C for 2 min to eliminate genomic DNA. Then, the first-strand cDNA was synthesized using PrimeScript RT reagent kit (PrimeScript RT reagent kit with gDNA Eraser, Takara). The synthesized first-strand cDNA was used as template for real-time PCR and the primers were shown in Table 2.
The genes expression levels were evaluated by real-time PCR. Real-time PCR was performed using SYBR green qPCR mix (Bimake, China) on the LightCycler 480 II Sequence Detection System (Roche, Switzerland) according to the manufacturer’s instructions. The real-time PCR reaction was in a total volume of 10 μl and the following conditions were used: 95°C for 5 min; and 40 cycles of 95°C for 15 s, 56°C for 15 s, and 72°C for 30 s. 18S rRNA are used as the internal reference, and remained stable in various treatments throughout the study. The genes relative expression levels were normalized to 18S rRNA. The results were calculated by the comparative Ct method (30).
All data are shown as mean ± standard error of the mean (S.E.M). Statistical analysis was performed with SPSS version 18.0 (SPSS Inc., Chicago, IL, USA). One-way ANOVA followed by Fisher’s Least Significance Difference (LSD) test was used to identify the significant difference. A probability value of P < 0.05 was considered significant.
In our present study, the result showed that the content of glucose and TG in serum was significantly decreased in the tea polysaccharides treatment groups compared to that of control group (Table 3). Moreover, the activity of SOD enzyme significantly increase by low-dose tea polysaccharides treatment (Table 3). The activity of GPX enzyme significantly increase by medium-dose tea polysaccharides treatment (Table 3). However, the contents of MDA were dramatically reduced in the tea polysaccharides groups compared to that of control group (Table 3). As shown in the Figure 1A, the contents of glycogen in hepatopancreas were markedly decreased in the high-dose group compared to that in the control group.
Figure 1 Effects of tea polysaccharides on hepatopancreas glycogen content and genes expression related to lipid metabolism in hepatopancreas. (A) Hepatopancreas glycogen content. At end of the experiment, fish were killed and the hepatopancreas was collected for glycogen content detected. (B–H) genes expression related to lipid metabolism in hepatopancreas. (B) acc1; (C) fas; (D) pparγ; (E) srebp1c; (F) lpl; (G) pygl; (H) gys. At end of the experiment, fish were killed and the hepatopancreas was collected for RNA extraction and real-time PCR. All data are shown as mean ± S.E.M. (n = 10-12). Significant differences were indicated by asterisks, *,P < 0.05; **,P < 0.01; ***,P < 0.001.
In Table 4, the results showed that the contents of IL-1β and TNFα in serum were significantly reduced in the tea polysaccharides treatment groups compared to that of the control group. The content of IL-12 in serum was markedly decreased in the high-dose tea polysaccharides treatment group. However, the content of TGFβ in serum was dramatically elevated in the high-dose tea polysaccharides treatment group compared to that of the control group.
The results showed that the expression of fatty acid synthesis gene acc1, fas and pparγ in hepatopancreas was significantly inhibited in the tea polysaccharides treatment groups compared to that of control group (Figures 1B, C). The srebp1c expression level was markedly decreased by the low- and medium-dose tea polysaccharides treatment (Figure 1D). The lpl expression level was markedly decreased by the medium- and high-dose tea polysaccharides treatment (Figure 1F). Moreover, the pygl expression level was significantly increased in the high-dose tea polysaccharides treatment group (Figure 1G). However, the gys expression level was significantly decreased in the high-dose tea polysaccharides treatment group (Figure 1H).
In the foregut, the expression of sglt1 and glut2 was dramatically inhibited by the tea polysaccharides treatment (Figures 2A, B). Moreover, the gip expression level was significantly promoted by the high-dose tea polysaccharides treatment (Figure 2C). In the foregut, the mRNA levels of occ1 and zo-1 were dramatically increased in the medium- and high-dose tea polysaccharides treatment groups (Figures 2D, E).
Figure 2 Effects of tea polysaccharides on genes expression related to glucose intake and gut barrier in foregut. (A) sglt1; (B) glut2; (C) gip; (D) occ1; (E) zo-1. At end of the experiment, fish were killed and the tissue was collected for RNA extraction and real-time PCR. All data are shown as mean ± S.E.M. (n = 10-12). Significant differences were indicated by asterisks, *,P < 0.05; **,P < 0.01; ***,P < 0.001.
In the Figure 3, the results showed that the ho-1 expression level in hepatopancreas was significantly increased in the high-dose tea polysaccharides treatment group compared to that of control group (Figure 3A). In the low and medium-dose tea polysaccharides treatment groups, the expression of gr and mnsod were markedly promoted compared to that of control group (Figures 3B, C). In addition, the cat expression level was significantly up-regulated in hepatopancreas by the medium-dose tea polysaccharides treatment (Figure 3D).
Figure 3 Effects of tea polysaccharides on genes expression related to antioxidant capacity in hepatopancreas. (A) ho-1; (B) gr; (C) mnsod; (D) cat. At end of the experiment, fish were killed and the hepatopancreas was collected for RNA extraction and real-time PCR. All data are shown as mean ± S.E.M. (n = 10-12). Significant differences were indicated by asterisks, *,P < 0.05; **,P < 0.01; ***,P < 0.001.
The effect of tea polysaccharides on expression of immune-related genes was detected in the spleen tissue of common carp. The results showed that the mRNA levels of tnfα and il-12 were significantly inhibited in the medium- and high-dose tea polysaccharides treatment groups (Figures 4A, B). The il-6 expression level was significantly decreased in the tea polysaccharides treatment groups (Figure 4C). Moreover, the expression of il-10 was markedly promoted in the low- and medium-dose tea polysaccharides treatment groups (Figure 4E). The expression of anti-inflammatory factor tgfβ was significantly increased by the high-dose tea polysaccharides treatment (Figure 4F).
Figure 4 Effects of tea polysaccharides on genes expression related to immunity in spleen. (A) tnfα; (B) il-12; (C) il-6; (D) il-1β; (E) il-10; (F) tgfβ. At end of the experiment, fish were killed and the hepatopancreas was collected for RNA extraction and real-time PCR. All data are shown as mean ± S.E.M. (n = 10-12). Significant differences were indicated by asterisks, *,P < 0.05; **,P < 0.01; ***,P < 0.001.
The genes expression of tnfα, il-1β, il-6 and il-12 were significantly increased in common carp spleen cells by treatment with LPS compared to those in control group (Figures 5A–D). By tea polysaccharides treatment, the mRNA levels of tnfα, il-1β, il-6 and il-12 were inhibited in spleen cells. Moreover, the promoted mRNA levels of tnfα, il-1β, il-6 and il-12 in spleen cells were alleviated in the LPS and tea polysaccharides group (Figures 5A–D). The il-10 and tgfβ expression were markedly decreased in common carp spleen cells by treatment with LPS (Figures 5E, F). The il-10 expression was significantly increased by treatment with tea polysaccharides (Figure 5E). Furthermore, the inhibited mRNA levels of il-10 and tgfβ were alleviated in the LPS and tea polysaccharides treatment group (Figures 5E, F).
Figure 5 Effects of LPS and tea polysaccharides on genes expression related to immunity in common carp spleen cells. (A) tnfα; (B) il-12; (C) il-6; (D) il-1β; (E) il-10; (F) tgfβ. The cells were seeded in 24-well plates at 1×106 per well in 1mL DMEM/F12 with 10% FBS. The next day, cells were placed in DMEM/F12 without FBS for 1 h. Then, the cells were treated with LPS (25 μg/mL), tea polysaccharides (400 μg/mL), LPS (25 μg/mL) + tea polysaccharides (400 μg/mL) for 12 h. All data are shown as mean ± S.E.M. (n = 5-6). Significant differences (P<0.05) were indicated by different letters.
As a group of heteropolysaccharides extracted from tea, tea polysaccharides reveals multiple beneficial bioactivity in previous studies (14, 15). In our study, the serum glucose levels were significantly decreased in the tea polysaccharides treatment groups. The result was similar to that in previous studies. In mice, the blood glucose level was significantly decreased after injection with tea polysaccharides (31). Furthermore, the blood glucose content of alloxan-induced diabetic mice was markedly reduced after four-week oral administration of puerh tea polysaccharides (PTPS) (32), and was suppressed increase after six days oral administration of green tea polysaccharides (GTPS) (33). In addition, the serum glucose levels were decreased by daily oral administration of tea polysaccharides in diabetic and non-diabetic mice (23, 34, 35). These results indicate tea polysaccharides can reduce blood glucose level in mammals and fish. Previous study suggested that the reduction in intestinal glucose transport by tea polysaccharides was mainly mediated by the biochemical inhibition of transport activity (36). In our study, the results also showed that the tea polysaccharides can decrease the sglt1 and gult2 genes expression in the foregut. We speculate that tea polysaccharides reduce the serum glucose by lowing the glucose transport in gut of common carp.
The antioxidant activity of tea polysaccharides was reported in previous studies (14, 15). In our study, the enzyme activities of serum SOD and GPX were promoted in the tea polysaccharides groups. However, the contents of MDA were significantly reduced in the tea polysaccharides groups. A report showed that the serum level of T-SOD was significantly promoted in rats, and the elevated serum content of MDA was attenuated in the green tea polysaccharides treated group (37). In addition, pretreatment with Keemun black tea polysaccharides (KBTP), the hepatic T-SOD and GSH levels were reduced, and the MDA content was decreased in CCl4-intoxicated mice (38). Moreover, the study showed that the contents of MDA were reduced, and the SOD, catalase and GPX activities were increased in the plasma, liver and heart of mice after treatment with crude tea polysaccharides for 30 days (39). Furthermore, compared to MC (HFD without additional treatment) group, the content of MDA was significantly decreased and the GPX and CAT activities were increased in the Chinese Liupao tea polysaccharides (CLTPS) treatment groups (40). It is indicated that the antioxidant activity of tea polysaccharides can implement in the carbohydrate-, lipid-, toxicant-induced or normal physiological status. Furthermore, tea polysaccharides can increase the genes expression related to antioxidant capacity (ho-1, gr, cat and mnsod) in the liver of common carp in our study. Based on the above results, we speculate that tea polysaccharides increase the antioxidant capacity by lowing the levels of MDA and increasing activities and gene expression of antioxidase of common carp.
It is a crucial activity that tea polysaccharides promote immunity (14, 15, 36, 41). Tea polysaccharides activate immune cells to secrete various biological reaction mediators, such as cytokines, free radicals, and lyases (36). In the present study, the contents of IL-1β, TNFα and IL-12 were significantly decreased, and the level of TGFβ was markedly promoted in the serum of common carp after tea polysaccharides treatment. Furthermore, the contents of IL-2, IL-6, IL-10 and IFN-γ were significantly reduced in the colitis-associated cancer (CAC) mice by treatment with tea polysaccharides, and the levels of IL-10 were markedly increased in tea polysaccharides groups compared to that in Azoxymethane/Dextran sulfate sodium (AOM/DSS) group (42). Moreover, the serum IL-6 and TNFα levels were decreased, and the serum IL-2, IL-4 and IL-10 levels were increased in gastric cancer mice after tea polysaccharides treatment (43). In addition, the study of Yuan and his colleagues showed that IL-6 and TNFα levels were significantly decreased in mouse splenocytes treated with tea polysaccharides compared with that in native control (NC) group (44). Based on those results, it is indicated that improving the activity of antioxidant enzymes of tea polysaccharides was not only in mammals but also in the fish. In addition, the expression of il-1β, tnfα, il-6 and il-12 were significantly inhibited, and the expression of il-10 and tgfβ were dramatically increased in the spleen of common carp in the tea polysaccharides groups. In the common carp spleen cells, the tea polysaccharides could relieved LPS-induced immune related genes expression. The previous study also showed that the expression levels of il-6 and tnfα were reduced in white adipose tissue of rats in the tea polysaccharides treatment groups (37). Based on the above study, it is manifested that tea polysaccharides plays immunocompetence by regulating the activity of antioxidant enzymes and expression of immune factors.
Tea polysaccharides also have a fat-lowing effect (36). In our study, the serum TG contents were also significantly reduced in the tea polysaccharides groups. The activity of reducing TG levels of tea polysaccharides was reported in previous studies. For instance, the black tea polysaccharides significantly reduced the TG content in serum and liver of rats compared to that in model control (Wu et al., 2016). The HMTP can decrease the CCl4-elevated level of serum TG (22), and administration of GTPS or black tea polysaccharides (BTPS) in mice before the CCl4 injection can resist the CCl4-induced increases in the level of TG (15). In addition, the contents of serum TG were markedly reduced in mice after Ilex Kuding tea polysaccharides (IKTP) treated with 200, 400 and 800 mg/kg BW (45). Those results indicate that the activity of reducing TG of tea polysaccharides is ubiquitous. In addition, the expression of lpl, acc1, fas, srebp1c and pparγ were significantly decreased in the tea polysaccharides treatment groups. Our results were similar to that in previous study, in which the tea polysaccharides effected the gene expression related to fat metabolic pathways of rats, and suppressed the accumulation and formation of fat and promoted lipolysis to prevent obesity (Wu et al., 2016). Moreover, a study reported that green tea polyphenols prevent HFD-induced obesity by increasing adiponectin levels, and alleviation of PPARγ phosphorylation (46). Another study reported that tea-supplemented reduced body fat mass of rats and down-regulated the expression of pparγ, c/ebpβ and lpl (47). Furthermore, the results revealed that the lipogenic-related genes expression was affected by kuding tea treatment, and that the expression of pparγ and lipogenic genes were inhibited in the liver of mice (48). From above results, it is indicate that the fat-lowing activity of tea polysaccharides is exerted by regulating the expression of lipid metabolism related genes in mammals and fish.
In the present study, the expression of occ and zo-1 were significantly increased in the foregut of common carp in the tea polysaccharides groups. The expression of gip was increased, and sglt1 and glut2 expression were decreased in the foregut in the tea polysaccharides groups. SGLT1 and GLUT2 are the important glucose transporter in the intestines. In a previous study, the intestinal glucose transport was significantly decreased by treatment with green tea extract (GTE), water soluble polysaccharides derived from green tea (WSP), and GTE+WSP. The expression of sglt1 was markedly decreased in the Caco-2 cells by treatment with wheat starch + GTE + WSP (49). Moreover, the protein expression of PI3Kp85, p-Akt and GLUT4 in diabetic mice were increased in the liver by orally gavage with tea polysaccharides, and the serum glucose level was accompanied decreased (34). In addition, the expression of gys was inhibited and the hepatopancreas glycogen content was decreased in the tea polysaccharides treatment group. The expression of pygl was significantly increased in the tea polysaccharides treatment group. The decreased hepatopancreas glycogen content was caused by the decreased glycogen synthesis (gys) and increased glycogenolysis (pygl) in hepatopancreas and decreased glucose absorption (sglt1 and glut2) in foregut. It is indicated that the absorption of glucose and glycogenesis were affected by tea polysaccharides via regulating the related-genes expression. Intestinal barrier function is an important aspect for intestinal health. The study of cyclophosphamide (Cy)-induced BALB/c mice showed that the colonic TLR4/MyD88/NF-κB p65 and JAK2/STAT3 pathway was activated by pectic heteropolysaccharides and the expression of claudin1, claudin5 and occludin1 were significantly increased (50). In addition, the expression levels of claudin1 and claudin5 were significantly promoted in the colonic tissues of mice in the polysaccharides from the tea flower (TFPS) treatment group (51). Those results indicated that the tea polysaccharides play beneficial role in intestinal health by regulating intestinal barrier related genes expression. The regulatory mechanism underlying the impact of tea polysaccharides on intestinal barrier related genes expression of fish should be investigated in future studies.
In conclusion, our present results suggested that tea polysaccharides promoted immunity, antioxidant capacity and intestinal barrier function and reduced lipogenesis and glucose transporter of common carp. The results of this study will provide a theoretical foundation of tea polysaccharides application in aquaculture.
The datasets presented in this study can be found in online repositories. The names of the repository/repositories and accession number(s) can be found in the article/supplementary material.
The animal study was reviewed and approved by Animal Care and Use Ethics Committee of the Henan Normal University.
GY, XL, JH: conceived and performed the experiments, formal analysis, writing, and original draft. XM, GY: Reviewing and editing. WH, KL, XC, YZ: Supervision, reviewing, and editing. CL, YS, XZ: analyzed the data. All authors contributed to the article and approved the submitted version.
This work was supported by the National Natural Science Foundation of China (U1904118, 32273149), the Natural Science Foundation of Henan Province (212300410174).
Authors WH and KL were employed by Henan JinBaiHe Biotechnology Co., Ltd.
The remaining authors declare that the research was conducted in the absence of any commercial or financial relationships that could be construed as a potential conflict of interest.
All claims expressed in this article are solely those of the authors and do not necessarily represent those of their affiliated organizations, or those of the publisher, the editors and the reviewers. Any product that may be evaluated in this article, or claim that may be made by its manufacturer, is not guaranteed or endorsed by the publisher.
1. Barbosa V, Maulvault AL, Anacleto P, Santos M, Mai M, Oliveira H, et al. Effects of steaming on health-valuable nutrients from fortified farmed fish: Gilthead seabream (Sparus aurata) and common carp (Cyprinus carpio) as case studies. Food Chem Toxicol an Int J published Br Ind Biol Res Assoc (2021) 152:112218. doi: 10.1016/j.fct.2021.112218
2. Tian J, Wu F, Yu LJ, Lu X, Jiang M, Liu W, et al. The effects of high-macronutrient (protein, fat and carbohydrate) diets on growth performance and muscular metabolic responses in grass carp. Aquacult Nutr (2020) 26:2135–46. doi: 10.1111/anu.13152
3. Wu CL, Ye JY, Gao JE, Chen L, Lu ZB. The effects of dietary carbohydrate on the growth, antioxidant capacities, innate immune responses and pathogen resistance of juvenile black carp mylopharyngodon piceus. Fish Shellfish Immunol (2016) 49:132–42. doi: 10.1016/j.fsi.2015.12.030
4. Lin SM, Shi CM, Mu MM, Chen YJ, Luo L. Effect of high dietary starch levels on growth, hepatic glucose metabolism, oxidative status and immune response of juvenile largemouth bass, micropterus salmoides. Fish Shellfish Immunol (2018) 78:121–6. doi: 10.1016/j.fsi.2018.04.046
5. Zhou CP, Ge XP, Lin HZ, Niu J. Effect of dietary carbohydrate on non-specific immune response, hepatic antioxidative abilities and disease resistance of juvenile golden pompano (Trachinotus ovatus). Fish Shellfish Immunol (2014) 41:183–90. doi: 10.1016/j.fsi.2014.08.024
6. Zhao H, Luo YE, Wu ZX, Zhou Y, Guo DY, Wang H, et al. Hepatic lipid metabolism and oxidative stress responses of grass carp (Ctenopharyngodon idella) fed diets of two different lipid levels against aeromonas hydrophila infection. Aquaculture (2019) 509:149–58. doi: 10.1016/j.aquaculture.2019.05.029
7. Meng XL, Wu SK, Hu WP, Zhu ZX, Yang GK, Zhang YM, et al. Clostridium butyricum improves immune responses and remodels the intestinal microbiota of common carp (Cyprinus carpio l.). Aquaculture (2021) 530:735753. doi: 10.1016/j.aquaculture.2020.735753
8. Church NA, McKillip JL. Antibiotic resistance crisis: challenges and imperatives. Biologia (2021) 76:1535–50. doi: 10.1007/s11756-021-00697-x
9. Choi WM, Mo WY, Wu SC, Mak NK, Bian ZX, Nie XP, et al. Effects of traditional Chinese medicines (TCM) on the immune response of grass carp (Ctenopharyngodon idellus). Aquacult Int (2014) 22:361–77. doi: 10.1007/s10499-013-9644-7
10. Zhu F. A review on the application of herbal medicines in the disease control of aquatic animals. Aquaculture (2020) 526:735422. doi: 10.1016/j.aquaculture.2020.735422
11. Pu HY, Li XY, Du QB, Cui H, Xu YP. Research progress in the application of Chinese herbal medicines in aquaculture: A review. Engineering-Prc (2017) 3:731–7. doi: 10.1016/J.Eng.2017.03.017
12. Beliveau R, Gingras D. Green tea: prevention and treatment of cancer by nutraceuticals. Lancet (2004) 364:1021–2. doi: 10.1016/S0140-6736(04)17076-1
13. Koo MW, Cho CH. Pharmacological effects of green tea on the gastrointestinal system. Eur J Pharmacol (2004) 500:177–85. doi: 10.1016/j.ejphar.2004.07.023
14. Nie SP, Xie MY. A review on the isolation and structure of tea polysaccharides and their bioactivities. Food Hydrocoll (2011) 25:144–9. doi: 10.1016/j.foodhyd.2010.04.010
15. Du LL, Fu QY, Xiang LP, Zheng XQ, Lu JL, Ye JH, et al. Tea polysaccharides and their bioactivities. Molecules (2016) 21:1449. doi: 10.3390/Molecules21111449
16. Guo L, Liang Q, Du XF. Effects of molecular characteristics of tea polysaccharide in green tea on glass transitions of potato amylose, amylopectin and their mixtures. Food Hydrocoll (2011) 25:486–94. doi: 10.1016/j.foodhyd.2010.07.027
17. Liang YR, Liu C, Xiang LP, Zheng XQ. Health benefits of theanine in green tea: A review. Trop J Pharm Res (2015) 14:1943–9. doi: 10.4314/tjpr.v14i10.29
18. Xiang LP, Wang A, Ye JH, Zheng XQ, Polito CA, Lu JL, et al. Suppressive effects of tea catechins on breast cancer. Nutrients (2016) 8:458. doi: 10.3390/nu8080458
19. Ren DY, Hu YY, Luo YY, Yang XB. Selenium-containing polysaccharides from ziyang green tea ameliorate high-fructose diet induced insulin resistance and hepatic oxidative stress in mice. Food Funct (2015) 6:3342–50. doi: 10.1039/c5fo00557d
20. Xu P, Wu J, Zhang Y, Chen H, Wang YF. Physicochemical characterization of puerh tea polysaccharides and their antioxidant and alpha-glycosidase inhibition. J Funct Foods (2014) 6:545–54. doi: 10.1016/j.jff.2013.11.021
21. Wu T, Guo Y, Liu R, Wang K, Zhang M. Black tea polyphenols and polysaccharides improve body composition, increase fecal fatty acid, and regulate fat metabolism in high-fat diet-induced obese rats. Food Funct (2016) 7:2469–78. doi: 10.1039/c6fo00401f
22. Lu XS, Zhao Y, Sun YF, Yang S, Yang XB. Characterisation of polysaccharides from green tea of huangshan maofeng with antioxidant and hepatoprotective effects. Food Chem (2013) 141:3415–23. doi: 10.1016/j.foodchem.2013.06.058
23. Chen DJ, Sun JY, Dong WX, Shen YX, Xu ZM. Effects of polysaccharides and polyphenolics fractions of zijuan tea (Camellia sinensis var. kitamura) on -glucosidase activity and blood glucose level and glucose tolerance of hyperglycaemic mice. Int J Food Sci Tech (2018) 53:2335–41. doi: 10.1111/ijfs.13825
24. Chen XQ, Fang YP, Nishinari K, We H, Sun CC, Li JR, et al. Physicochemical characteristics of polysaccharide conjugates prepared from fresh tea leaves and their improving impaired glucose tolerance. Carbohydr Polymers (2014) 112:77–84. doi: 10.1016/j.carbpol.2014.05.030
25. Xie X, Franěk R, Pšenička M, Chen F, Kašpar V. Optimization of in vitro culture conditions of common carp germ cells for purpose of surrogate production. Front Vet Sci (2022) 9:1036495. doi: 10.3389/fvets.2022.1036495
26. Sotnikov A, Rodina M, Stechkina T, Benevente CF, Gela D, Boryshpolets S, et al. High sperm concentration during cryopreservation decreases post-thaw motility percentage without compromising in vitro fertilization outcomes in common carp. Aquaculture (2023) 562:738746. doi: 10.1016/j.aquaculture.2022.738746
27. Feng J, Chang X, Zhang Y, Lu R, Meng X, Song D, et al. Characterization of a polysaccharide HP-02 from honeysuckle flowers and its immunoregulatory and anti-aeromonas hydrophila effects in Cyprinus carpio l. Int J Biol Macromol (2019) 140:477–83. doi: 10.1016/j.ijbiomac.2019.08.041
28. Feng JC, Cai ZL, Zhang XP, Chen YY, Chang XL, Wang XF, et al. The effects of oral rehmannia glutinosa polysaccharide administration on immune responses, antioxidant activity and resistance against aeromonas hydrophila in the common carp, cyprinus carpio l. Front Immunol (2020) 11:904. doi: 10.3389/fimmu.2020.00904
29. Yang G, Zhao W, Qin C, Yang L, Meng X, Lu R, et al. Igfbp3 in grass carp (Ctenopharyngodon idellus): Molecular identification and mRNA expression under glucose, insulin and glucagon. Comp Biochem Physiol B Biochem Mol Biol (2020) 242:110394. doi: 10.1016/j.cbpb.2019.110394
30. Livak KJ, Schmittgen TD. Analysis of relative gene expression data using real-time quantitative PCR and the 2(-delta delta C(T)) method. Methods (San Diego Calif.) (2001) 25:402–8. doi: 10.1006/meth.2001.1262
31. Wang Dongfeng W, Wang Chenghong W, Li Jun L, Zhao Guiwen Z. Components and activity of polysaccharides from coarse tea. J Agric Food Chem (2001) 49:507–10. doi: 10.1021/jf000029m
32. Xu P, Chen H, Wang YQ, Hochstetter D, Zhou T, Wang YF. Oral administration of puerh tea polysaccharides lowers blood glucose levels and enhances antioxidant status in alloxan-induced diabetic mice. J Food Sci (2012) 77:H246–52. doi: 10.1111/j.1750-3841.2012.02950.x
33. Chen HX, Zhang M, Xie BJ. Components and antioxidant activity of polysaccharide conjugate from green tea. Food Chem (2005) 90:17–21. doi: 10.1016/j.foodchem.2004.03.001
34. Li SQ, Chen HX, Wang J, Wang XM, Hu B, Lv FN. Involvement of the PI3K/Akt signal pathway in the hypoglycemic effects of tea polysaccharides on diabetic mice. Int J Biol Macromol (2015) 81:967–74. doi: 10.1016/j.ijbiomac.2015.09.037
35. Chen XQ, Lin Z, Ye Y, Zhang R, Yin JF, Jiang YW, et al. Suppression of diabetes in non-obese diabetic (NOD) mice by oral administration of water-soluble and alkali-soluble polysaccharide conjugates prepared from green tea. Carbohydr Polymers (2010) 82:28–33. doi: 10.1016/j.carbpol.2010.04.017
36. Xu AN, Lai WY, Chen P, Awasthi MK, Chen XQ, Wang YF, et al. A comprehensive review on polysaccharide conjugates derived from tea leaves: Composition, structure, function and application. Trends Food Sci Tech (2021) 114:83–99. doi: 10.1016/j.tifs.2021.05.020
37. Xu Y, Zhang M, Wu T, Dai S, Xu J, Zhou Z. The anti-obesity effect of green tea polysaccharides, polyphenols and caffeine in rats fed with a high-fat diet. Food Funct (2015) 6:297–304. doi: 10.1039/c4fo00970c
38. Sun Y, Yang X, Lu X, Wang D, Zhao Y. Protective effects of keemun black tea polysaccharides on acute carbon tetrachloride-caused oxidative hepatotoxicity in mice. Food Chem Toxicol an Int J published Br Ind Biol Res Assoc (2013) 58:184–92. doi: 10.1016/j.fct.2013.04.034
39. Cao H. Polysaccharides from Chinese tea: recent advance on bioactivity and function. Int J Biol Macromol (2013) 62:76–9. doi: 10.1016/j.ijbiomac.2013.08.033
40. Mao Y, Wei BY, Teng JW, Xia N, Zhao MM, Huang L, et al. Polysaccharides from Chinese liupao dark tea and their protective effect against hyperlipidemia. Int J Food Sci Tech (2018) 53:599–607. doi: 10.1111/ijfs.13633
41. Chen GJ, Yuan QX, Saeeduddin M, Ou SY, Zeng XX, Ye H. Recent advances in tea polysaccharides: Extraction, purification, physicochemical characterization and bioactivities. Carbohydr Polymers (2016) 153:663–78. doi: 10.1016/j.carbpol.2016.08.022
42. Liu LQ, Nie SP, Shen MY, Hu JL, Yu Q, Gong DM, et al. Tea polysaccharides inhibit colitis-associated colorectal cancer via interleukin-6/STAT3 pathway. J Agric Food Chem (2018) 66:4384–93. doi: 10.1021/acs.jafc.8b00710
43. Yang JJ, Chen B, Gu Y. Pharmacological evaluation of tea polysaccharides with antioxidant activity in gastric cancer mice. Carbohydr Polymers (2012) 90:943–7. doi: 10.1016/j.carbpol.2012.06.024
44. Yuan C, Li Z, Peng F, Xiao F, Ren D, Xue H, et al. Combination of selenium-enriched green tea polysaccharides and huo-ji polysaccharides synergistically enhances antioxidant and immune activity in mice. J Sci Food Agric (2015) 95:3211–7. doi: 10.1002/jsfa.7287
45. Zhai X, Ren D, Luo Y, Hu Y, Yang X. Chemical characteristics of an ilex kuding tea polysaccharide and its protective effects against high fructose-induced liver injury and vascular endothelial dysfunction in mice. Food Funct (2017) 8:2536–47. doi: 10.1039/c7fo00490g
46. Tian C, Ye X, Zhang R, Long J, Ren W, Ding S, et al. Green tea polyphenols reduced fat deposits in high fat-fed rats via erk1/2-PPARgamma-adiponectin pathway. PloS One (2013) 8:e53796. doi: 10.1371/journal.pone.0053796
47. Chen N, Bezzina R, Hinch E, Lewandowski PA, Cameron-Smith D, Mathai ML, et al. Green tea, black tea, and epigallocatechin modify body composition, improve glucose tolerance, and differentially alter metabolic gene expression in rats fed a high-fat diet. Nutr Res (2009) 29:784–93. doi: 10.1016/j.nutres.2009.10.003
48. Fan S, Zhang Y, Hu N, Sun Q, Ding X, Li G, et al. Extract of kuding tea prevents high-fat diet-induced metabolic disorders in C57BL/6 mice via liver X receptor (LXR) beta antagonism. PloS One (2012) 7:e51007. doi: 10.1371/journal.pone.0051007
49. Lee YE, Yoo SH, Chung JO, Park MY, Hong YD, Park SH, et al. Hypoglycemic effect of soluble polysaccharide and catechins from green tea on inhibiting intestinal transport of glucose. J Sci Food Agric (2020) 100:3979–86. doi: 10.1002/jsfa.10442
50. Chen D, Chen GJ, Ding Y, Wan P, Peng YJ, Chen CX, et al. Polysaccharides from the flowers of tea (Camellia sinensis l.) modulate gut health and ameliorate cyclophosphamide-induced immunosuppression. J Funct Foods (2019) 61:103470. doi: 10.1016/J.Jff.2019.103470
Keywords: tea polysaccharide, metabolism, antioxidant, immunity, common carp
Citation: Yang G, Liang X, Hu J, Li C, Hu W, Li K, Chang X, Zhang Y, Zhang X, Shen Y and Meng X (2022) Feeding tea polysaccharides affects lipid metabolism, antioxidant capacity and immunity of common carp (Cyprinus carpio L.). Front. Immunol. 13:1074198. doi: 10.3389/fimmu.2022.1074198
Received: 19 October 2022; Accepted: 11 November 2022;
Published: 24 November 2022.
Edited by:
Changle Qi, Huzhou University, ChinaReviewed by:
Xianyong Bu, Ocean University of China, ChinaCopyright © 2022 Yang, Liang, Hu, Li, Hu, Li, Chang, Zhang, Zhang, Shen and Meng. This is an open-access article distributed under the terms of the Creative Commons Attribution License (CC BY). The use, distribution or reproduction in other forums is permitted, provided the original author(s) and the copyright owner(s) are credited and that the original publication in this journal is cited, in accordance with accepted academic practice. No use, distribution or reproduction is permitted which does not comply with these terms.
*Correspondence: Xiaolin Meng, bWVuZ3hpYW9saW5xZGlvQDEyNi5jb20=
†These authors have contributed equally to this work
Disclaimer: All claims expressed in this article are solely those of the authors and do not necessarily represent those of their affiliated organizations, or those of the publisher, the editors and the reviewers. Any product that may be evaluated in this article or claim that may be made by its manufacturer is not guaranteed or endorsed by the publisher.
Research integrity at Frontiers
Learn more about the work of our research integrity team to safeguard the quality of each article we publish.