- 1Department of Reproductive Endocrinology, Women’s Hospital, Zhejiang University School of Medicine, Hangzhou, China
- 2Key Laboratory of reproductive Genetics, Ministry of Education, Zhejiang University, Hangzhou, China
- 3Department of Obstetrics, Hangzhou Fuyang Women And Children Hospital, Fuyang, China
- 4Department of Obstetrics and Gynecology, First Affiliated Hospital, Zhejiang University College of Medicine, Hangzhou, China
- 5Department of Reproduction, People’s Hospital of Xinjiang Uygur Autonomous Region, Urumqi, Xinjiang, China
Trophoblast immune cell interactions are central events in the immune microenvironment at the maternal-fetal interface. Their abnormalities are potential causes of various pregnancy complications, including pre-eclampsia and recurrent spontaneous abortion. Matrix metalloproteinase (MMP) is highly homologous, zinc(II)-containing metalloproteinase involved in altered uterine hemodynamics, closely associated with uterine vascular remodeling. However, the interactions between MMP and the immune microenvironment remain unclear. Here we discuss the key roles and potential interplay of MMP with the immune microenvironment in the embryo implantation process and pregnancy-related diseases, which may contribute to understanding the establishment and maintenance of normal pregnancy and providing new therapeutic strategies. Recent studies have shown that several tissue inhibitors of metalloproteinases (TIMPs) effectively prevent invasive vascular disease by modulating the activity of MMP. We summarize the main findings of these studies and suggest the possibility of TIMPs as emerging biomarkers and potential therapeutic targets for a range of complications induced by abnormalities in the immune microenvironment at the maternal-fetal interface. MMP and TIMPs are promising targets for developing new immunotherapies to treat pregnancy-related diseases caused by immune imbalance.
1 Introduction
Matrix metalloproteinase (MMP) belongs to a family of zinc(II)-dependent endopeptidases, which cleave extracellular matrix (ECM) proteins and participate in processes such as tissue remodeling and angiogenesis. From the immunological point of view, a successful pregnancy is one in which the maternal immune system can accept an embryo containing paternal antigens. Alterations in the immune environment at the maternal-fetal interface can induce immune imbalance, which can be accompanied by varying degrees of inflammatory responses, thus triggering pathological pregnancy outcomes. Tissue inhibitor of matrix metalloproteinase (TIMP), the predominant endogenous inhibitor of MMP, acts by binding MMP in a 1:1 ratio. In this review, we will provide a comprehensive overview of the immune role of MMP in the embryo implantation process and pregnancy-related diseases using data reported in PubMed and other scientific databases. Additionally, we present the possibility and potential benefits of MMP and its inhibitor as a biomarker and potential therapeutic target for pregnancy-related diseases.
2 Structure, functions, and regulations of MMP
2.1 Structure of MMP
MMP is a family of zinc(II)-dependent protein hydrolases with a common core structure that degrade the ECM, which is essential for vascular remodeling (1). Notably, zinc(II) of MMP-3 maintains its protease activity even when replaced by other ions (2). Twenty-eight types of MMP have been identified in vertebrates, and twenty-three of them are expressed in human tissues (3). MMP could be secreted by connective tissue, pro-inflammatory cells, and uterine placental cells, including fibroblasts, vascular smooth muscle (VSM), leukocytes, trophoblasts, etc.; A typical MMP consists of a pre-peptide sequence (80 amino acids), a catalytic metalloproteinase structural domain (170 amino acids), a variable length linker peptide or a hinge region, and a heme-binding protein structural domain (except for MMP-7, MMP-23, and MMP-26) (4, 5). In vivo, MMP generally exists as the inactive form of proMMP precursor, which is cleaved by various protein hydrolases (e.g., serine proteases, fibrinolytic enzymes, etc.), eventually producing active MMP to perform its functions (6).
2.2 Functions of MMP
Based on substrate specificity, MMP can be classified into gelatinase, collagenase, matrilysin, stromelysin, membrane-type MMP(MT-MMP), and others (4, 5). Because of the basic function of degrading ECM proteins, MMP is involved in a wide range of physiological and pathological processes in the human body. Physiologically, MMP plays a critical role in cell proliferation, migration and differentiation, tissue repair and remodulation, embryogenesis, and wound healing (7). Pathologically, MMP disorders are connected with tumor invasion and metastasis because MMP can degrade almost all protein components in ECM (8).
The degradation of ECM by MMP (mainly collagen and elastin) is the basis for the involvement of MMP in tissue damage repair. The degradation process of MMP requires the coordinated action of a Zn2+ active center and a water molecule (including three histidines and one glutamate), with methionine as a hydrophobic base to play a supporting role (5). During the MMP-substrate interaction, the Zn2+-bound water molecule launches a nucleophilic attack on the substrate, which eventually leads to its decomposition and release of water (9).
The process of tissue injury is inevitably accompanied by the development of inflammation, during which multiple inflammatory cells and mediators are included in the alteration. Recent research suggests that the ability to regenerate tissue may be independent of the inflammatory response, and thus the correlation between immunity and tissue regenerative capacity is gradually gaining widespread attention (10). In general, there may also be an underlying immune inflammatory response during tissue remodeling in MMP. Inflammatory cytokines (Interleukin(IL)1-α, IL1-β, IL-2, IL-17, C-reactive protein, Tumor necrosis factor-α (TNF-α), etc.) can be found in healing phases of chronic venous ulcers, which are believed to stimulate the production of neutrophil gelatinase-associated lipoprotein (NGAL),thus activating MMP-9 and form MMP-9/NGAL complexes to help to heal (11, 12). The endometrial remodeling process is precisely regulated in which MMP is essential (13). In all types of the endometrium, MMP-26 was found to have cyclical changes in its expression that may be associated with the endometrial tissue remodeling process (14, 15).
During the tumor growth and invasion process, MMP may be involved in key processes that disrupt the balance of growth and anti-growth factor signaling in the tumor microenvironment and tumor neovascularization (16). Non-catalytic functions targeting MMP are now an emerging researchhotspot. For example, the cytoplasmic tail of MT1-MMP canbind to Factor inhibiting hypoxia-inducible factor-1 (FIH-1) and promoting stable FIH-1-Munc18-1-interacting protein three interaction, which enhances hypoxia-inducible factor (HIF) target gene expression, thereby promoting Warburg effect and angiogenesis in a non-proteolytic manner (17).
This review focuses on the role of MMP in early pregnancy. The primary reproductive events include endometrial decidualization, uterine spiral artery remodeling, trophoblast cell invasion and differentiation, and placenta formation. MMP may be involved in uterine placental and vascular remodeling during normal pregnancy, as MMP is significant in tissue regulation remodeling (18).
2.3 MMP and ovarian sex hormone regulation
Apart from being expressed by cells, the expression of MMP can also be induced by various exogenous signals, such as cytokines, growth factors, hormones, and changes in cell-matrix and cell-cell interplay (7). Noticeably, during the implantation window period in early pregnancy, a large number of factors such as cytokines, adhesion molecules, and proteolytic enzymes are secreted by the endometrium under the mediation of estrogen and progesterone, which play critical roles in the identification and adhesion of the embryo and endometrium, and further regulate the process of embryo implantation. The interactions between steroid hormones and MMP need to be fully understood.
Affected by steroid hormones secreted by the ovary, the endometrium undergoes continuous cyclic exfoliation and remodeling throughout the female reproductive phase: estradiol stimulates endometrial proliferation during the proliferative phase of the menstrual cycle, while progesterone further acts on the estrogen-affected endometrium to induce its glandular secretion and stromal cell differentiation into metaphase cells (19). Endometrial MMP and TIMP expression regulations are essential for endometrial growth, rupture of circulating tissue, and pregnancy establishment, yet the mechanisms regulating the expression patterns of MMP and TIMP during the menstrual cycle have not been fully elucidated (20). Ovarian sex hormones (e.g., estrogen and progesterone) affect the expression of MMP, which in turn can coordinate with ovarian sex hormones to co-involve in the endometrial tissue remodeling and shedding process (21). Steroid hormone regulation of the MMP system includes direct or indirect regulation of gene transcription, specific changes in the expression, and action of local cytokines (20).
The relationship between estrogen and the specific expression of MMP family members remains unclear. Activator protein-1 (AP-1) transcription consists of c-Jun and c-Fosproteins, proven to be a significant regulator of multiple MMP transcription under multiple conditions (22). The promoters of most MMP genes contain AP-1 elements, and upon increased estrogen exposure, the ligand-bound estrogen receptor complex can increase the expression of the AP-1-bound transcription factors Fos and Jun (23).
Vitro research has shown that the addition of progesterone to endometrial explants or isolated stromal cells downregulates MMP expressions (24). Specifically,10-8m-1 estrogen and 10-7m-1 medroxyprogesterone acetate inhibited the pro-MMP-1 secreted by cultured human endometrial stromal cells (25). MMP-3 mRNA was remarkably curbed by estrogen and progestin medroxyprogesterone acetate (10-8mol/L-10-6mol/L) (26). An animal experiment using an estrogen-progestin subcutaneous implantation device to mimic the proliferative and secretory phases of the menstrual cycle in de-ovulatory rhesus monkeys found that all the MMP expressions were upregulated after progesterone withdrawal and spontaneously downregulated after menstruation in the absence of progesterone effects (27). The pattern of regulations of MMP by progesterone differs from that of estrogen. First, progesterone receptors can induce AP-1 activation in the absence of ligands, and the effect of progesterone receptors on AP-1 activity was shown to be cell type-specific (28). Second, progesterone can reverse AP-1 activation, and animal experiments suggest that progesterone can inhibit estrogen-induced c-fos mRNA expression (29). Finally, progesterone also indirectly affects the expressions of MMP by regulating cytokines (19), and IL-1α released from epithelial cells induces its expression in surrounding stromal cells through paracrine and autocrine amplification loops, thereby increasing the total amount of endometrial IL-1α and triggering MMP-1 expression (30). In contrast, progesterone at the stromal cells at the mRNA level inhibits IL-1α (19).
Human chorionic gonadotropin (HCG) has both local and systemic functions in early pregnancy (31). HCG can affect embryo attachment, embryo formation, trophoblast infiltration, and other pregnancy-related processes by up-regulating leukemia inhibitory factor (LIF), vascular endothelial growth factor (VEGF), MMP-9 and other factors, and it was reported that 500 IU/ml of HCG administration inhibited intrauterine insulin-like growth factor-binding protein-1 and macrophage-stimulating factor while elevating the level of LIF, VEGF and MMP-9 (32).The rapid increase of serum progesterone due to the rise of systemic HCG level in early pregnancy also has effects on MMP; as mentioned above, the inhibitory effect on MMP of progesterone may help limit trophoblast invasion in the proper range.
3 MMP and maternal-fetal interface events
3.1 Trophoblast invasion
Trophoblast invasion is a critical process in human placental formation, involving the regulation of cell adhesion and the degradation process of the ECM, which underlies the conversion of the uterine spiral arteries (33). To dilate the vasculature and provide adequate nutrition for embryonic growth, the extravillous trophoblast invades the maternal endometrium and remodels the spiral arteries, which allows sufficient uteroplacental perfusion (34, 35). Increased expression levels/activity of MMP-2 and MMP-9 can be found in the aorta of normal pregnant rats (36). It is suggested that these altered MMP-2 and MMP-9 may be associated with a series of cellular events at the maternal-fetal interface. EGF-mediated trophoblast invasion may be related to altered MMP-2 expression/activity (37). High levels of MMP-9 facilitate the degradation of the endometrial ECM and loosen intercellular junctions, thus favoring the invasion of extravillous trophoblast cells.With the highest levels of mRNA and protein during pregnancy at week 6-7, MMP-26 decreases gradually before reaching a minimum level at mid-gestation, and this is inconsistent with the spatiotemporal regulation of trophoblast invasive capacity (38, 39). Mishra (40) suggested that the extracellular matrix metalloproteinase inducer (EMMPRIN) may also affect embryonic adhesion and fusion with the tubular epithelium by influencing the expression of MMP-2 and MMP-14. EMMPRIN, serving as an MMP inducer, expresses membrane protein in the immunoglobulin superfamily with two heavily glycosylated extracellular structural domains (41, 42).
Notably, Nissi (43) et al. monitored serum concentrations of MMP-9, TIMP-1, TIMP-2, and MMP-2/TIMP-2 complexes in normal pregnant women at different gestational weeks, finding no statistically significant changes in levels during normal pregnancy. Few studies have been conducted on maternal serum MMP and TIMP during normal pregnancy, and further experimental studies with expanded sample sizes are needed in the future.
3.2 Angiogenesis and remodeling
Angiogenesis and remodeling are complex series of processes including recruitment, migration, proliferation, and apoptosis of vascular cells consisting of stem/progenitor cells, endothelial cells (ECs), vascular smooth muscle cells (VSMC), etc., while the ECM plays its essential role in vascular development and morphogenesis by providing matrix scaffolds, interacting with matrix receptors or providing growth factors (44). MMP regulates VSMC growth, proliferation, and migration processes critical in vascular remodeling. MMP-2 secretion is closely associated with the migration of VSMC from rat thoracic aorta cultured in vitro, involving the breakdown of the basement membrane and pericellular ECM, while in bovine studies, it was found that MMP-2 induces the migration of VSMC by triggering oxidized low-density lipoprotein (OxLDL)-induced activation of the sphingomyelin/ceramide pathway, which ultimately leads to smooth muscle cell (SMC) proliferation and migration, and that this MMP-2 activation process is mediated by MT1-MMP (45, 46). Additionally, animal studies demonstrate that MMP-2 mRNA is increased in the uterine artery at day 7 and day 21 of gestation in rats, which suggests its pregnancy-associated vascular remodeling role (47).
4 MMP and immune microenvironment at the maternal-fetal interface
The maternal-fetal interface is a critical site for the establishment and maintenance of normal pregnancy, where immune cell populations such as macrophages, T cells, natural killer cells, and Dendritic Cells (DCs) accumulate (48).
4.1 Macrophage
Macrophages are primary monocyte-derived intrinsic immune cells with remarkable heterogeneity and plasticity that are essential for homeostasis and host defense (49, 50). As the second most abundant population of leukocytes in the decidual cells, macrophages are actively involved in coordinating the apoptotic process during tissue remodeling, thereby preventing the release of potentially pro-inflammatory and pro-immunogenic cellular contents during secondary necrosis, current studies suggest that CD14 or CD68 can be used as immune markers to identify decidual macrophages (51, 52). The percentage of macrophages in leukocytes showed no significant change in early and mid-pregnancy, while the percentage of CD14+ macrophages tended to decrease significantly by late pregnancy (53).
It is currently believed that the phenotypic characteristics of macrophages reflect the local microenvironment response, including various cytokines and other mediators secreted by adventitial cells, and that during the embryonic implantation window, macrophages establish a pro-inflammatory microenvironment for embryonic implantation (54). In vitro, macrophages are usually classified into M1 and M2 phenotypes: pro-inflammatory M1 macrophages based on classical activation (LPS+ Interferon-γ (IFN-γ)), NFκB pathway, JAK/STAT signaling or IFN regulatory factor (IRF) induction and IL-4 or IL-4/IL-3-induced anti-inflammatory M2-type macrophages (55–57). Induced by IL-4, IL-10 and IL-13, M2-type macrophages have the function of anti-inflammatory by producing a large amount of IL-10 and TGF-β; Besides, its immune regulatory function is critical for early pregnancy maintenance (58).
It has been proven that decidual macrophages involved in ECM degrading in vascular remodeling are mediated by MMP-3 (59). Macrophages are an important source of MMP, and inflammatory factors can regulate the expression of macrophage proteases, including MMP (16, 60). For example, the expression of MMP-9 can be induced by TNF-α at the transcriptional level (61). Also, the MMP-9 promoter is subject to IL-18-mediated AP-1 and NF-kappaß-dependent activation (62). It was shown that MT1-MMP can control macrophage invasion by ECM components and cell surface molecular signaling (63, 64). Recent studies revealed that the transcriptional target phosphoinositide 3-kinase δ (PI3Kδ)-expressing MT1-MMP can dependently trigger Akt/GSK3 cascade signaling and ultimately restrict the expression of macrophage-derived pro-inflammatory mediators (44). The potential link between MMP and macrophages and their expression products before may be one of the directions for further work in the future.
4.2 Dendritic cell
Maternal immune cells and fetus-derived trophoblasts have bidirectional regulation interaction in early pregnancy, and polarization toward Th2 in the immune response is considered to be the key to successful pregnancy (65). Generally, DCs play a pivotal role in this process. Even though only approximately 1% of early pregnancy DCs are present in the decidual cells, they have a dual critical role as potent antigen-presenting cells mediating immune activation and immune tolerance, which is particularly important during pregnancy (48, 66–68). These paradoxical dual functions of DCs seem to depend on their different stages of differentiation: immature dendritic cells (iDC), characterized by DC-SIGN, promote T-cell tolerance, whereas CD83+ mature DCs function as an inducer of T-cell immunity (69–72). It should be noted that there also existed DEC205+ DCs in the metaphase stromal cells that were activated but still showed an immature state (73).
DCs are critical effectors of TGF-β activity, promoting both Peripheral T-regulatory cell production during TGF-β activation and inducing differentiation of pathogenic Th17 cells for T-cell tolerance (74). MMP may provide an alternative pathway for the proteolytic activation of potential TGF-β in vivo. An in vitro experiment demonstrates that MMP-9 depends on CD44 for cell surface localization in TA3 mouse breast cancer cells, activating TGF-β2 and TGF-β3, which promote cell invasion and angiogenesis (75).
4.3 Natural killer cell
Natural killer cells (NK cells), which account for more than 70% of metaphase leukocytes in early pregnancy, are represented by the CD56+CD16- phenotype (48). According to the type of cytokines secreted, NK cells can be divided into NK1 (mainly secreting IFN-γ, TNF-α) and NK2 (mainly secreting IL-4, IL-5, IL-10, IL-13). However, the number of uterine NK (uNK) cells in the placenta decreases in late pregnancy (76). The complete spectrum of MMP expressed by NK cells and macrophages has not been determined, while in vitro, experimental studies have identified NK cells and macrophages expressing MMP-2, MMP-7, MMP-9, MMP-11, MMP-16, MMP-19, and TIMP (77). Current clinical studies suggest that protein array studies of CD56+uNK cells collected at 8-10 weeks of gestation indicate that uNK cells are the primary producers of angiogenic growth factors, but uNK cells collected at 12-14 weeks of pregnancy are the primary producers of cytokines (78).
With strong secretion of pro-inflammatory factors, angiogenic factors, etc., previous studies have reported a regulatory interaction of decidual NK cells (dNK cells) in trophoblast invasiveness through the secretion of IL-8 and interferon-inducible protein-10 (IP-10), which further stimulate CXCR1 and CXCR3-mediated pathways and thus exert regulatory effects (79). It has been suggested that IL-6 and IL-8 secreted by CD56+uNK cells and CD14+ macrophages are involved in uterine spiral artery remodeling either by secreting IFN-γ (80, 81).
Exploration of the functions of immune cells at the maternal-fetal interface throughout pregnancy is almost impossible due to the limited access to specimens, and the speculated role of immune cells at the maternal-fetal interface in human early pregnancy is revealed in Figure 1. Existing studies have adequately demonstrated their essential part during pregnancy, and an in-depth understanding of their interactions can assist in identifying potential immune risks in pathological pregnancies and thus responding to them.
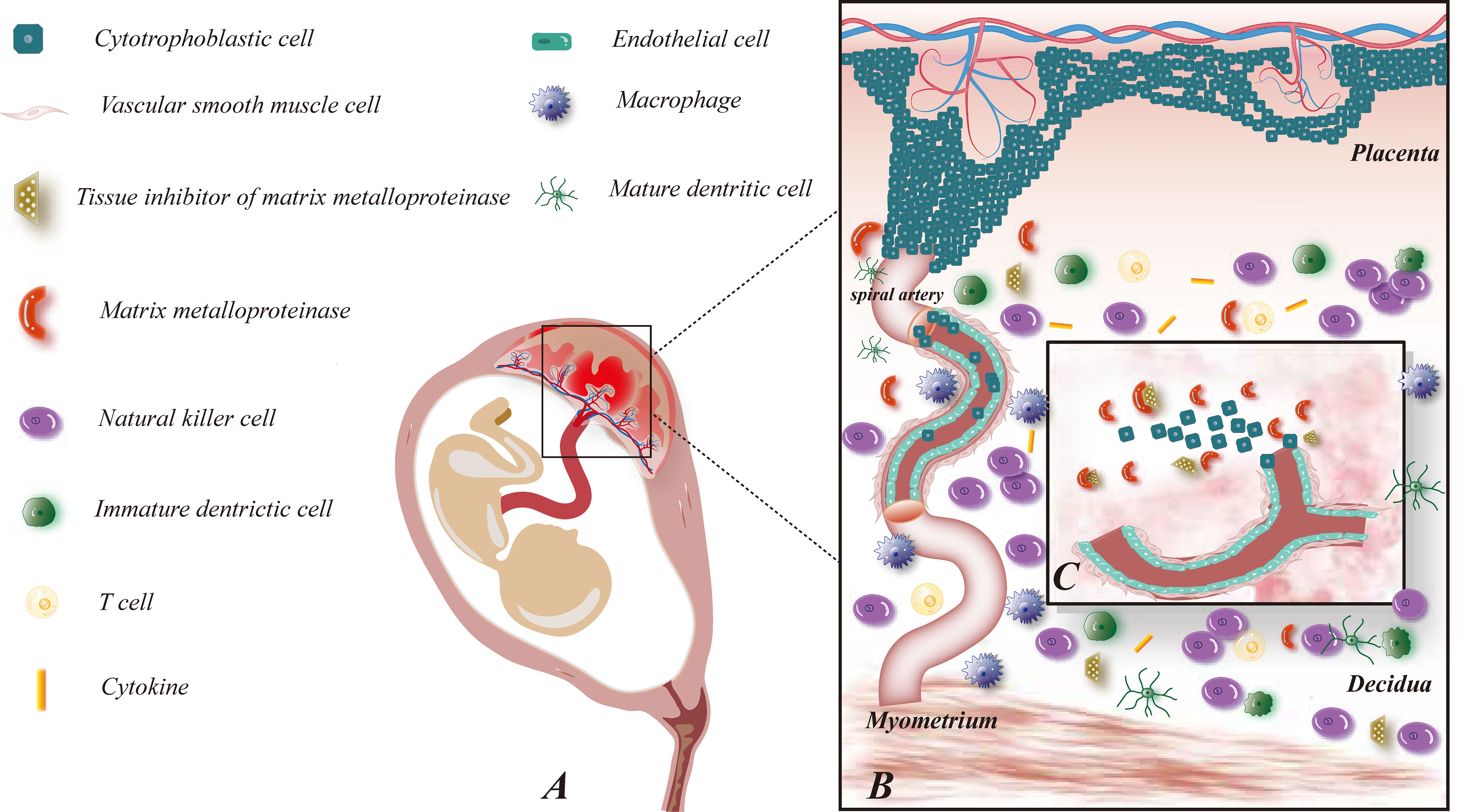
Figure 1 Maternal-fetal interface immune microenvironment on early pregnancy and role of MMP on embryo implantation. (A) This figure demonstrates the relative location of the fetus and its appendages on the maternal uterus; (B) This figure shows the composition of the maternal-fetal interface immune microenvironment, including fetal-originated cytotrophoblast cell, immune cell subsets: NK cells, macrophages, T cells, DC cells, cytokines, etc., maternal uterine spiral artery: vascular smooth muscle cells and vascular endothelial cells, MMP and TIMP families; (C) This figure illustrates the enlarged view of the functions of MMP on early pregnancy. MMP and TIMP assist the process of trophoblast invasion and uterine spiral artery remodeling by degrading ECM.
5 MMP and pregnancy-related diseases
5.1 Preeclampsia
Insufficient remodeling of the uterine spiral arteries is one of the necessary pathological changes in Preeclampsia (PE). The association with an acute atherosclerotic response may be one of the interactions by which inadequate spiral arterial remodeling induces placental malperfusion, in which arteries undergo an atherosclerotic process, form intimal plaques, and enter a vicious cycle of ischemia and reperfusion with an extensive intravascular inflammatory response (82, 83). Dysregulated endogenous immune responses may lead to excessive inflammatory responses, such as a significant increase in pro-inflammatory cytokines in patients with PE;At the same time, a significantly increased risk of PE in pregnant women who are in a state of inflammatory overreaction early in pregnancy (84). Abnormal immune factors inducing trophoblast under invasion and endothelial cell dysfunction are considered significant causes of PE, including innate and adaptive immune factors (85, 86). Studies have shown that pregnant women with human immunodeficiency virus infection have a lower incidence ofPEor hypertensive pregnancy (HTN-Preg), providing evidence for a strong association between organismal immunity and PE (87).
The level of MMP-2 expression in patients with PE remains controversial: some studies have found high levels of MMP-2 in the plasma or amniotic fluid of patients with PE or subsequent development of PE, while others have suggested that there is no statistical difference in the level of MMP-2 expression (88–91). However, the results of most experimental studies showed low expression or low activity of MMP-9 in serum and placental tissues of patients with PE (92). Moreover, the decrease in MMP-9 is found to be more pronounced in patients with early-onset PE (93). These findings suggest that abnormal expression of MMP-2 and MMP-9 may be involved in the pathogenesis of PE. Since MMP has a significant proteolytic effect on ECM, downregulation of MMP expression in patients with PE may lead to impaired growth, proliferation, and migration of SMC, thus interfering with the process of uterine spiral artery remodeling, which is consistent with the findings of Li (94) et al. on increased collagen content in the uterus, placenta, and aorta in mice with a model of PE. Another study showed a decrease in MMP-2 expression levels in the placenta of patients with severe PE under hypoxic conditions, mediated by the Nodal/ALK7 signaling pathway, acting in coordination with taurine upregulated protein 1 (TUG1) to achieve an invasive impaired trophoblast outcome (91).
There is extensive evidence that placental ischemia and hypoxia promote the release of a variety of active growth factors, including pro-angiogenic factors such as VEGF and placental growth factor (PlGF), as well as anti-angiogenic factors such as soluble fms-like tyrosine kinase-1 (sFlt-1), anti-inflammatory cytokines TNF-α as reactive oxygen radicals(ROS), IL-6, and HIF (18, 95). VEGF is a supergene family derived from a platelet growth factor, while sFlt-1 is a soluble antagonist of VEGF, both of which function importantly in the regulation of angiogenic homeostasis (96). An elevated sFlt-1/PIGF ratio is observed in patients with late-onset PE, and several authors have proposed sFlt-1/PIGF as an early predictor of PE (97–100). Studies support sFlt-1 as a potential upstream mechanism linking placental ischemia and reduced MMP-2 and MMP-9 content in HTN-Preg, of which VEGF can reverse this reduction in MMP content induced by sFlt-1 (94). Removal of circulating sFlt-1 in patients with early PE by a plasma-specific dextran sulfate column may reduce urinary protein and prolong pregnancy with no significant adverse fetal effects (101). sFlt-1/PIGF offers new ideas for optimizing the management of PE.
In addition to the abnormal remodeling process of spiral arteries, PE is also closely associated with inflammatory immune hyperactivation. Previous studies have found an excess of macrophages in placental biopsy specimens from patients with PE and that these excess macrophages tend to be located in and around the spiral arteries, separating them from trophoblast cells rather than in the stroma surrounding the spiral arteries and extravillous trophoblast (52). Apoptosis of extravillous trophoblast cells induced by macrophages through inflammatory mediators such as TNF-α is associated with damaged intravascular trophoblast invasion in PE (102). Altered MMP-9 expression in the serum of patients with PE is associated with type I TNFR, suggesting an underlying inflammatory process, especially in early PE (103). The immune mechanisms underlying the association of MMP with the development of PE are not yet clear. Here we propose the following hypothesis based on the available evidence: differential expression levels or activity of MMP (especially MMP-9) are induced by mutual promotion with various inflammatory factors (e.g., TNF-α, IL-6), causing damage to vascular ECs, and the release of ROS in hypoxic conditions to stimulate oxidative stress-inducing vascular endothelialand smooth muscle cell dysfunction (Figure 2).
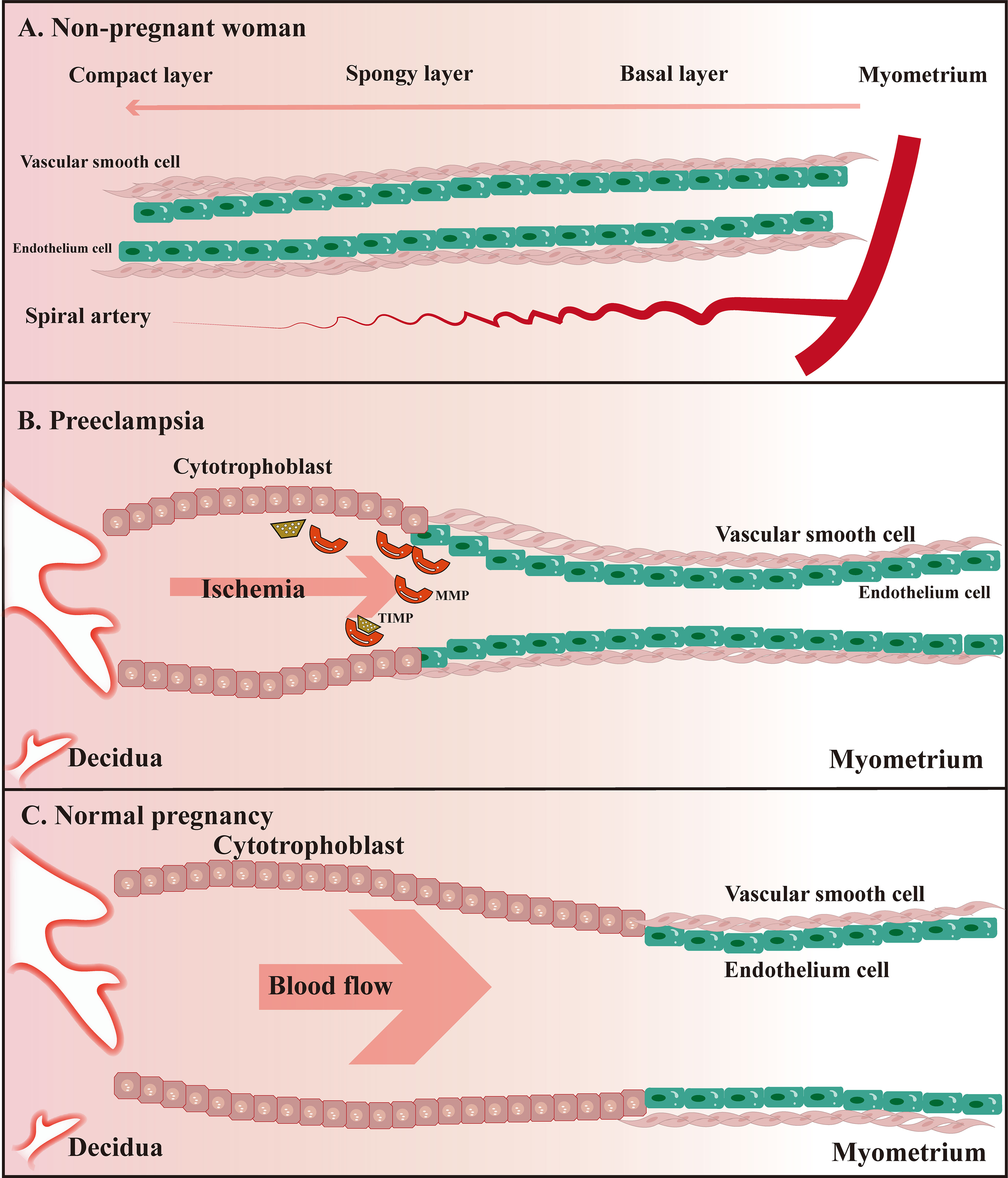
Figure 2 Schematic diagram of uterine spiral artery remodeling in non-pregnant women, PE, and normal pregnant women. (A) The uterine spiral arteries extend into the functional layer of the endometrium, of which the lumen diameter changes periodically under the influence of ovarian hormone levels; (B) Remodeling of the uterine spiral arteries is insufficient compared to normal pregnant women; (C) Physiological remodeling of the uterine spiral arteries is witnessed with the thickened spiral arteriole, enlarged lumen, and increased blood flow speed.
It is also worth mentioning that MMP is involved in atherogenesis by taking an essential role in the immune response and vascular inflammation (5). The balance between synthesis and degradation of ECM components is crucial for plaque stability, and MMP, in addition to its role in degrading the ECM of patients with atherosclerosis, reflects a systemic inflammatory response whose imbalance with TIMP may be the result of changes in the environment of pro- and anti-inflammatory factors in advanced clinical stages of coronary artery disease (104). However, most of these studies were oriented towards the assessment of the role of carotid or coronary arteries, and whether MMP exerts the same effect during atherosclerosis of the uterine spiral arteries at the maternal-fetal interface in patients with PE is not yet supported by precise experimental results, which may be one of the directions worthy of future research.
5.2 Recurrent spontaneous abortion
Recurrent spontaneous abortion (RSA) is a severe reproductive disorder of pregnancy that remains an incomplete problem in obstetrics and gynecology (105). In addition to the removal of the N-terminal structural domain by hydrolases and regulation by endogenous inhibitors, the regulation of MMP at the gene transcription level is influenced by various cytokines and growth factors, which may closely related to abortion.
VEGF plays a vital role in embryo implantation by participating in placental development and improving endometrial tolerance. It acts mainly through binding to tyrosine kinase receptors, including Flt-1, kinase insert domain receptor (KDR, also termed VEFGR-2), Flt-4 (also termed VEGFR-3), with Flt-1 also being expressed in macrophage cell lineage cells (106–108). Lash (109) explored changes in the expression of VEGF and its receptors in the endometrium of women possessing a history of recurrent miscarriage and proposed that the expression level of VEGF-A was decreased. In another study,He and Chen (110) detected the amount of VEGF protein in the early chorionic villous tissue of patients with recurrent miscarriage by western blot and found that the amount of VEGF protein was downregulated in the early chorionic villous tissue of patients with recurrent miscarriage (0.79 ± 0.40) compared to the control group (1.01 ± 0.37). The association of the ERK-VEGF/MMP-9 signaling pathway with the epithelial-mesenchymal transitionprocess can be observed in primary hepatocellular carcinoma cells (111, 112). Indeed, the underlying cause of VEGF dysregulation in recurrent miscarriage remains unknown, and genetic variation may be one of the potential causes (113). Yan, Fang (33) showed that the MMP2-735T allele and the MMP9-1562T allele might be associated with RSA risk. Among them, the MMP9-1562T allele was also associated with preterm birth (114). Due to the critical role of VEGF and MMP in the placental implantation process, in an analysis of blood and follicular fluid from women with multiple implantation failures,Benkhalifa (115) suggested that circulating MMP-7 and VEGF could serve as potential predictive biomarkers for recurrent implantation failure.
IFN-γ is a soluble dimeric cytokine,which are higher in the peripheral blood of non-pregnant women with recurrent miscarriage than in the healthy population, suggesting IFN-γ as a potential risk factor for patients with RSA (116, 117). This is consistent with the finding that IFN-γ reduces MMP-2 secretion and trophoblast invasiveness (118). Recognizing the relationship between maternal Th1/Th2 cytokines and unexplained recurrent spontaneous abortion (URSA) helps in the early diagnosis of URSA as well as treatment monitoring, and IFN-γ/IL-4 in the early diagnosis of URSA reduces the rate of missed diagnoses (119, 120). In addition to IL-4, IL-6, IL-1, and IL-12 can be found at altered levels in patients with RSA (121–123) (Table 1).
5.3 Trophoblastic disease of pregnancy
Gestational trophoblastic disease (GTD) is caused by allogeneic embryo transfer and includes a series of interrelated disorders, staphyloma, invasive staphyloma, choriocarcinoma, placental site trophoblastic tumors, and epithelioid trophoblastic tumors (136). Gestational trophoblastic diseases are characterized by vascular abnormalities in the trophectoderm with an imbalance in the expression of MMP and its inhibitors (137).
Several clinical studies have shown that the imbalance between activation and inhibition of MMP-2 plays an important role in the pathogenesis, progression, and metastasis of GTD and that MMP-2 is predominantly expressed in the syncytial trophoblast of gravida (138), as well as a higher positive rate for MMP-2 and TIMP-2 in gestational trophoblastic tumors compared to normal villi (139). In addition, those with malignant potential had higher MMP-9/TIMP-1 ratios than those without malignant transformation and normal villous tissues, illustrating its potential as a predictor (140). In an in vitro study using 0,5,10,25,50,100,200 μg/L IL-12 to treat human choriocarcinoma cell line JEG-3, it was observed that the overall expression level of MMP-9 was reduced by IL-12 treatment compared to the control, but increased with increasing IL-12 concentration, while application of 5 μg/L IL-12 observed that MMP-9 expression was downregulated with time (0,24,36,48,72 hours) (141). IL-12 coordinates the involvement of MMP-9 in cell invasion in a dose- and time-dependent manner and is one of the possible mechanisms of choriocarcinoma development.
MMP may be involved in the invasive and metastatic potential of choriocarcinoma, which has high expression of MMPand low expression of its inhibitors. Compared to choriocarcinoma, placental site trophoblastic tumor has low expression of MMP and increased expression of inhibitors of MMP, which explains the lower invasiveness of placental site trophoblastic tumor compared to choriocarcinoma (142).
6 Therapeutic potential of MMP and inhibitor in pregnancy-related diseases
The role of MMP in the maternal-fetal interface makes it a promising target for immunotherapy. Overall, MMP is inhibited by both endogenous and exogenous inhibitors, with TIMP acting as the predominant endogenous inhibitor of MMP (143).
TIMP N-terminus folds as a single unit with TIMP attached to the active sites of MMP to inhibit its functions, and TIMP-1, TIMP-2, TIMP-3, and TIMP-4 homologous TIMP have been identified, of which TIMP-1 and TIMP-3 are glycoproteins (144). Intriguingly, in general, a single TIMP can inhibit multiple MMP with different effects, e.g., TIMP-1 can act simultaneously on MT1-MMP, MT3-MMP, MT5-MMP, and MMP-19 (145). Furthermore, TIMP-3 can even inhibit metalloproteinases other than MMP (146). The inability to specifically target specific MMP may be one of the reasons for the multiple side effects seen in clinical trial studies related to TIMP. This problem seems to be solved by monoclonal antibodies with high specificity and affinity for specific MMP, such as monoclonal antibodies REGA-3G12 and REGA-2D9 that react specifically with MMP-9 without cross-reacting with MMP-2 (5).
Currently, there are extensive mechanistic studies and preliminary clinical attempts regarding MMP and its inhibitor in intestinal inflammatory diseases, vascular diseases, fibrotic lesions, and tumor-related diseases. Serum MMP-3 and MMP-9 levels have been considered good markers of ulcerative colitis (UC) and inflammatory bowel disease (IBD) associated with some clinical stages (147–149). The selective MMP inhibitor ND-322 in a melanoma orthotopic mouse model can inhibit tumor growth and metastatic processes by targeting MMP-2 and MT1-MMP, providing a new avenue for adjuvant treatment options for aggressive melanoma (150). In addition, EMMPRIN is an attractive target in the treatment of oncological diseases due to its pro-angiogenic and pro-metastatic properties. Walter, Simanovich (42) designed a novel epitope-specific antibody against EMMPRIN that inhibits the secretion of MMP-9 and VEGF, shifting the tumor microenvironment of macrophages from an anti-inflammatory microenvironment dominated by TGF-β to one that is less immunosuppressive, thus allowing stimulated macrophages to perform antibody-dependent cytotoxic effects (ADCC) and kill tumor cells. There are clinical applications for EMMPRIN antibodies, such as Licartin, which has been approved by the Chinese Food and Drug Administration as a therapeutic anti-hepatocellular carcinoma radioimmune agent that is effective in reducing recurrence of hepatocellular carcinoma and prolonging survival in patients with advanced hepatocellular carcinoma after in situ liver transplantation (OLT) (151). Application of 50 μM concentration of docosahexaenoic acid (DHA) in human breast cancer cell line MDA-MB-231 resulted in 80% cell growth inhibition observed, while DHA inhibited breast cancer proliferation in vitro mainly by blocking the Cox-2-PGE2-NF-κB cascade to achieve inhibition of MMP-2 and MMP-9 transcription (152). DHA, a typical ω3-polyunsaturated fatty acid (ω3-PUFA), is one of the important unsaturated fatty acids in the body, generally from fat-rich fish, and is now widely recommended in clinical applications for nutrient supplementation and preventing preterm birth in pregnant women (153, 154), for which the preventive function is supported by in vitro experiment, animal experiment and clinical study (155–157). These findings provide crucial preclinical evidence for using DHA in chemoprevention to overcome potential therapeutic options for the corresponding cancers.
In the field of female pregnancy, MMP and its inhibitors have also shown surprising promise for application. Currently, the most effective treatment for PE remains the termination of pregnancy, while the incidence of preterm births will inevitably increase. Measuring the expression levels of MMP-2 or MMP-9 in serum or amniotic fluid during pregnancy may serve as a new biomarker for predicting or monitoring PE while quantifying changes in the activity of MMP by, for example, measuring protein hydrolysis products in serum may also help in the diagnosis, condition monitoring and treatment evaluation of patients with PE. Immunotherapeutic approaches play an active role in RSA (158). Since the underlying cause of RSA is still unknown, several new therapeutic approaches have been proposed to treat RSA, including low-molecular heparin, corticosteroids, intravenous immunoglobulin, or leukapheresis, but none of them have proven their effectiveness with large-scale data to date (116). Immunotherapy regimens based on MMP may be able to give a new direction to RSA. Interestingly, patients with RSA may be associated with metabolic dysregulation, such as hyperglycemia, which can affect MMP/TIMP regulation, which may provide new evidence to support clinical glycemic regulation in RSA patients (159, 160). Exploring the expression studies of MMP and TIMP members in different gestational trophoblastic diseases can help screen potential molecular biological markers for GTD diagnosis, determine the degree of disease malignancy and prognosis, and also provide possible therapeutic targets. Selective inhibitory antibodies related to MMP could be used for future treatment of gestational trophoblastic diseases. Particular inhibitory antibodies to MMP-9 and MMP-14 have been developed and shown to be effective in inhibiting tumor growth and metastasis (161), but their clinical efficacy is currently uncertain.
Despite the significant progress now acquired in MMP inhibitor research, doxycycline is the only MMP inhibitor approved by the FDA (44). Currently, MMP inhibitor therapies have not been applied in clinical practice in obstetrics and gynecology. Until we fully understand their potential mechanisms and corresponding pharmacokinetic profiles in embryo implantation and pregnancy-related diseases, the related clinical applications of MMP inhibitor immunotherapy should be cautious.
7 Conclusions
MMP can be secreted by various cells and is involved in processes such as tissue remodeling and angiogenesis. This paper covers the immunomodulatory mechanisms of MMP and its inhibitors at the maternal-fetal interface. However, due to the difficulty of obtaining specimens at all stages of gestational age, most existing studies have focused on maternal-fetal interface studies at early gestation or delivery, and how MMP plays a role in mid-and late pregnancy has not been elucidated, which may be one of the future research directions. This paper offers the possibility of using MMP and TIMP as targets or clinical protocols for immunotherapy in pregnancy-related diseases. However, future challenges, such as preparation of specific targeting agents and clinical side effects beyond expectations, still need to be addressed.
Author contributions
MJ conducted the literature search, drew the images, and completed the first draft manuscript in collaboration with XC. HQ, WH, YZ, and DL helped prepare the manuscript. DW, YJ, and AL revised and edited the final version of the manuscript. All authors contributed to the article and approved the submitted version.
Funding
This work was supported by grants from Key R&D Program of Zhejiang province(2021C03100), and National Natural Science Foundation of China (82101771 and 81974224).
Conflict of interest
The authors declare that the research was conducted in the absence of any commercial or financial relationships that could be construed as a potential conflict of interest.
Publisher’s note
All claims expressed in this article are solely those of the authors and do not necessarily represent those of their affiliated organizations, or those of the publisher, the editors and the reviewers. Any product that may be evaluated in this article, or claim that may be made by its manufacturer, is not guaranteed or endorsed by the publisher.
References
1. Duellman T, Warren CL, Peissig P, Wynn M, Yang J. Matrix metalloproteinase-9 genotype as a potential genetic marker for abdominal aortic aneurysm. Circ Cardiovasc Genet (2012) 5(5):529–37. doi: 10.1161/CIRCGENETICS.112.963082
2. Verma RP, Hansch C. Matrix metalloproteinases (MMPs): chemical-biological functions and (Q)SARs. Bioorg Med Chem (2007) 15(6):2223–68. doi: 10.1016/j.bmc.2007.01.011
3. Visse R, Nagase H. Matrix metalloproteinases and tissue inhibitors of metalloproteinases: structure, function, and biochemistry. Circ Res (2003) 92(8):827–39. doi: 10.1161/01.RES.0000070112.80711.3D
4. Cauwe B, Van den Steen PE, Opdenakker G. The biochemical, biological, and pathological kaleidoscope of cell surface substrates processed by matrix metalloproteinases. Crit Rev Biochem Mol Biol (2007) 42(3):113–85. doi: 10.1080/10409230701340019
5. Wang X, Khalil RA. Matrix metalloproteinases, vascular remodeling, and vascular disease. Adv Pharmacol (2018) 81:241–330. doi: 10.1016/bs.apha.2017.08.002
6. Nagase H, Visse R, Murphy G. Structure and function of matrix metalloproteinases and TIMPs. Cardiovasc Res (2006) 69(3):562–73. doi: 10.1016/j.cardiores.2005.12.002
7. Laronha H, Caldeira J. Structure and function of human matrix metalloproteinases. Cells (2020) 9(5):1076. doi: 10.3390/cells9051076
8. Najafi M, Farhood B, Mortezaee K. Extracellular matrix (ECM) stiffness and degradation as cancer drivers. J Cell Biochem (2019) 120(3):2782–90. doi: 10.1002/jcb.27681
9. Jacobsen JA, Major Jourden JL, Miller MT, Cohen SM. To bind zinc or not to bind zinc: an examination of innovative approaches to improved metalloproteinase inhibition. Biochim Biophys Acta (2010) 1803(1):72–94. doi: 10.1016/j.bbamcr.2009.08.006
10. Mathew LK, Sengupta S, Kawakami A, Andreasen EA, Löhr CV, Loynes CA, et al. Unraveling tissue regeneration pathways using chemical genetics. J Biol Chem (2007) 282(48):35202–10. doi: 10.1074/jbc.M706640200
11. Ayuk SM, Abrahamse H, Houreld NN. The role of matrix metalloproteinases in diabetic wound healing in relation to photobiomodulation. J Diabetes Res (2016) 2016:2897656. doi: 10.1155/2016/2897656
12. Serra R, Buffone G, Falcone D, Molinari V, Scaramuzzino M, Gallelli L, et al. Chronic venous leg ulcers are associated with high levels of metalloproteinases-9 and neutrophil gelatinase-associated lipocalin. Wound Repair Regen (2013) 21(3):395–401. doi: 10.1111/wrr.12035
13. Roy SC, Ghosh J. Dynamic in vivo changes in the activities of gelatinases, matrix metalloproteinases (MMPs), and tissue inhibitor of metalloproteinases (TIMPs) in buffalo (Bubalus bubalis) uterine luminal fluid during estrous cycle and early pregnancy. Mol Reprod Dev (2010) 77(11):944–53. doi: 10.1002/mrd.21240
14. Chegini N, Rhoton-Vlasak A, Williams RS. Expression of matrix metalloproteinase-26 and tissue inhibitor of matrix metalloproteinase-3 and -4 in endometrium throughout the normal menstrual cycle and alteration in users of levonorgestrel implants who experience irregular uterine bleeding. Fertil Steril (2003) 80(3):564–70. doi: 10.1016/S0015-0282(03)00797-0
15. Pilka R, Whatling C, Domanski H, Hansson S, Eriksson P, Casslén B. Epithelial expression of matrix metalloproteinase-26 is elevated at mid-cycle in the human endometrium. Mol Hum Reprod (2003) 9(5):271–7. doi: 10.1093/molehr/gag039
16. Kessenbrock K, Plaks V, Werb Z. Matrix metalloproteinases: regulators of the tumor microenvironment. Cell (2010) 141(1):52–67. doi: 10.1016/j.cell.2010.03.015
17. Sakamoto T, Seiki M. Integrated functions of membrane-type 1 matrix metalloproteinase in regulating cancer malignancy: Beyond a proteinase. Cancer Sci (2017) 108(6):1095–100. doi: 10.1111/cas.13231
18. Chen J, Khalil RA. Matrix metalloproteinases in normal pregnancy and preeclampsia. Prog Mol Biol Transl Sci (2017) 148:87–165. doi: 10.1016/bs.pmbts.2017.04.001
19. Gaide Chevronnay HP, Selvais C, Emonard H, Galant C, Marbaix E, Henriet P. Regulation of matrix metalloproteinases activity studied in human endometrium as a paradigm of cyclic tissue breakdown and regeneration. Biochim Biophys Acta (2012) 1824(1):146–56. doi: 10.1016/j.bbapap.2011.09.003
20. Curry TE Jr., Osteen KG. The matrix metalloproteinase system: changes, regulation, and impact throughout the ovarian and uterine reproductive cycle. Endocr Rev (2003) 24(4):428–65. doi: 10.1210/er.2002-0005
21. Zhang J, Salamonsen LA. In vivo evidence for active matrix metalloproteinases in human endometrium supports their role in tissue breakdown at menstruation. J Clin Endocrinol Metab (2002) 87(5):2346–51. doi: 10.1210/jcem.87.5.8487
22. Tombulturk FK, Soydas T, Sarac EY, Tuncdemir M, Coskunpinar E, Polat E, et al. Regulation of MMP 2 and MMP 9 expressions modulated by AP-1 (c-jun) in wound healing: improving role of lucilia sericata in diabetic rats. Acta Diabetol (2019) 56(2):177–86. doi: 10.1007/s00592-018-1237-5
23. Teyssier C, Belguise K, Galtier F, Chalbos D. Characterization of the physical interaction between estrogen receptor alpha and JUN proteins. J Biol Chem (2001) 276(39):36361–9. doi: 10.1074/jbc.M101806200
24. Henriet P, Cornet PB, Lemoine P, Galant C, Singer CF, Courtoy PJ, et al. Circulating ovarian steroids and endometrial matrix metalloproteinases (MMPs). Ann N Y Acad Sci (2002) 955:119–38. doi: 10.1111/j.1749-6632.2002.tb02773.x
25. Lockwood CJ, Krikun G, Hausknecht VA, Papp C, Schatz F. Matrix metalloproteinase and matrix metalloproteinase inhibitor expression in endometrial stromal cells during progestin-initiated decidualization and menstruation-related progestin withdrawal. Endocrinol (1998) 139(11):4607–13. doi: 10.1210/endo.139.11.6304
26. Schatz F, Papp C, Toth-Pal E, Lockwood CJ. Ovarian steroid-modulated stromelysin-1 expression in human endometrial stromal and decidual cells. J Clin Endocrinol Metab (1994) 78(6):1467–72. doi: 10.1210/jcem.78.6.8200951
27. Rudolph-Owen LA, Slayden OD, Matrisian LM, Brenner RM. Matrix metalloproteinase expression in macaca mulatta endometrium: evidence for zone-specific regulatory tissue gradients. Biol Reprod (1998) 59(6):1349–59. doi: 10.1095/biolreprod59.6.1349
28. Bamberger AM, Bamberger CM, Gellersen B, Schulte HM. Modulation of AP-1 activity by the human progesterone receptor in endometrial adenocarcinoma cells. Proc Natl Acad Sci U S A (1996) 93(12):6169–74. doi: 10.1073/pnas.93.12.6169
29. Kirkland JL, Murthy L, Stancel GM. Progesterone inhibits the estrogen-induced expression of c-fos messenger ribonucleic acid in the uterus. Endocrinol (1992) 130(6):3223–30. doi: 10.1210/endo.130.6.1375896
30. Pretto CM, Gaide Chevronnay HP, Cornet PB, Galant C, Delvaux D, Courtoy PJ, et al. Production of interleukin-1alpha by human endometrial stromal cells is triggered during menses and dysfunctional bleeding and is induced in culture by epithelial interleukin-1alpha released upon ovarian steroids withdrawal. J Clin Endocrinol Metab (2008) 93(10):4126–34. doi: 10.1210/jc.2007-2636
31. Licht P, Russu V, Wildt L. On the role of human chorionic gonadotropin (hCG) in the embryo-endometrial microenvironment: implications for differentiation and implantation. Semin Reprod Med (2001) 19(1):37–47. doi: 10.1055/s-2001-13909
32. Licht P, Fluhr H, Neuwinger J, Wallwiener D, Wildt L. Is human chorionic gonadotropin directly involved in the regulation of human implantation? Mol Cell Endocrinol (2007) 269(1-2):85–92. doi: 10.1016/j.mce.2006.09.016
33. Yan Y, Fang L, Li Y, Yu Y, Li Y, Cheng JC, et al. Association of MMP2 and MMP9 gene polymorphisms with the recurrent spontaneous abortion: A meta-analysis. Gene (2021) 767:145173. doi: 10.1016/j.gene.2020.145173
34. van den Brûle F, Berndt S, Simon N, Coulon C, Le Goarant J, Munaut C, et al. Trophoblast invasion and placentation: molecular mechanisms and regulation. Chem Immunol Allergy (2005) 88:163–80. doi: 10.1159/000087833
35. Cohen M, Wuillemin C, Irion O, Bischof P. Role of decidua in trophoblastic invasion. Neuro Endocrinol Lett (2010) 31(2):193–7. https://www.nel.edu/userfiles/articlesnew/NEL310210A03.pdf.
36. Dang Y, Li W, Tran V, Khalil RA. EMMPRIN-mediated induction of uterine and vascular matrix metalloproteinases during pregnancy and in response to estrogen and progesterone. Biochem Pharmacol (2013) 86(6):734–47. doi: 10.1016/j.bcp.2013.06.030
37. Biadasiewicz K, Sonderegger S, Haslinger P, Haider S, Saleh L, Fiala C, et al. Transcription factor AP-2α promotes EGF-dependent invasion of human trophoblast. Endocrinol (2011) 152(4):1458–69. doi: 10.1210/en.2010-0936
38. Qiu W, Bai SX, Zhao MR, Wu XQ, Zhao YG, Sang QX, et al. Spatio-temporal expression of matrix metalloproteinase-26 in human placental trophoblasts and fetal red cells during normal placentation. Biol Reprod (2005) 72(4):954–9. doi: 10.1095/biolreprod.104.036772
39. Liu J, Cao B, Li YX, Wu XQ, Wang YL. GnRH I and II up-regulate MMP-26 expression through the JNK pathway in human cytotrophoblasts. Reprod Biol Endocrinol (2010) 8:5. doi: 10.1186/1477-7827-8-5
40. Mishra B, Kizaki K, Koshi K, Ushizawa K, Takahashi T, Hosoe M, et al. Expression of extracellular matrix metalloproteinase inducer (EMMPRIN) and its related extracellular matrix degrading enzymes in the endometrium during estrous cycle and early gestation in cattle. Reprod Biol Endocrinol (2010) 8:60. doi: 10.1186/1477-7827-8-60
41. Biswas C, Zhang Y, DeCastro R, Guo H, Nakamura T, Kataoka H, et al. The human tumor cell-derived collagenase stimulatory factor (renamed EMMPRIN) is a member of the immunoglobulin superfamily. Cancer Res (1995) 55(2):434–9. https://aacrjournals.org/cancerres/article/55/2/434/501497/The-Human-Tumor-Cell-derived-Collagenase.
42. Walter M, Simanovich E, Brod V, Lahat N, Bitterman H, Rahat MA. An epitope-specific novel anti-EMMPRIN polyclonal antibody inhibits tumor progression. Oncoimmunology (2016) 5(2):e1078056. doi: 10.1080/2162402X.2015.1078056
43. Nissi R, Santala M, Talvensaari-Mattila A. The serum levels of circulating matrix metalloproteinase MMP-9, MMP-2/TIMP-2 complex and TIMP-1 do not change significantly during normal pregnancy: a pilot study. BMC Res Notes (2021) 14(1):31. doi: 10.1186/s13104-021-05442-w
44. Chen Q, Jin M, Yang F, Zhu J, Xiao Q, Zhang L. Matrix metalloproteinases: inflammatory regulators of cell behaviors in vascular formation and remodeling. Mediators Inflamm (2013) 2013:928315. doi: 10.1155/2013/928315
45. Uzui H, Lee JD, Shimizu H, Tsutani H, Ueda T. The role of protein-tyrosine phosphorylation and gelatinase production in the migration and proliferation of smooth muscle cells. Atherosclerosis (2000) 149(1):51–9. doi: 10.1016/S0021-9150(99)00295-6
46. Augé N, Maupas-Schwalm F, Elbaz M, Thiers JC, Waysbort A, Itohara S, et al. Role for matrix metalloproteinase-2 in oxidized low-density lipoprotein-induced activation of the sphingomyelin/ceramide pathway and smooth muscle cell proliferation. Circulation (2004) 110(5):571–8. doi: 10.1161/01.CIR.0000136995.83451.1D
47. Kelly BA, Bond BC, Poston L. Gestational profile of matrix metalloproteinases in rat uterine artery. Mol Hum Reprod (2003) 9(6):351–8. doi: 10.1093/molehr/gag043
48. Yang F, Zheng Q, Jin L. Dynamic function and composition changes of immune cells during normal and pathological pregnancy at the maternal-fetal interface. Front Immunol (2019) 10:2317. doi: 10.3389/fimmu.2019.02317
49. He X, Tan S, Shao Z, Wang X. Latitudinal and longitudinal regulation of tissue macrophages in inflammatory diseases. Genes Dis (2022) 9(5):1194–207. doi: 10.1016/j.gendis.2021.06.007
50. Liao AH, Liu H. The epigenetic regulation of the immune system during pregnancy. Reprod Immunol (2021), 365–85. doi: 10.1016/B978-0-12-818508-7.00005-1
51. Bulmer JN, Williams PJ, Lash GE. Immune cells in the placental bed. Int J Dev Biol (2010) 54(2-3):281–94. doi: 10.1387/ijdb.082763jb
52. Abrahams VM, Kim YM, Straszewski SL, Romero R, Mor G. Macrophages and apoptotic cell clearance during pregnancy. Am J Reprod Immunol (2004) 51(4):275–82. doi: 10.1111/j.1600-0897.2004.00156.x
53. Williams PJ, Searle RF, Robson SC, Innes BA, Bulmer JN. Decidual leucocyte populations in early to late gestation normal human pregnancy. J Reprod Immunol (2009) 82(1):24–31. doi: 10.1016/j.jri.2009.08.001
54. Repnik U, Tilburgs T, Roelen DL, van der Mast BJ, Kanhai HH, Scherjon S, et al. Comparison of macrophage phenotype between decidua basalis and decidua parietalis by flow cytometry. Placenta (2008) 29(5):405–12. doi: 10.1016/j.placenta.2008.02.004
55. Zhao X, Chen J, Sun H, Zhang Y, Zou D. New insights into fibrosis from the ECM degradation perspective: the macrophage-MMP-ECM interaction. Cell Biosci (2022) 12(1):117. doi: 10.1186/s13578-022-00856-w
56. Wei J, Tang D, Lu C, Yang J, Lu Y, Wang Y, et al. Irf5 deficiency in myeloid cells prevents necrotizing enterocolitis by inhibiting M1 macrophage polarization. Mucosal Immunol (2019) 12(4):888–96. doi: 10.1038/s41385-019-0169-x
57. Kang K, Park SH, Chen J, Qiao Y, Giannopoulou E, Berg K, et al. Interferon-γ represses M2 gene expression in human macrophages by disassembling enhancers bound by the transcription factor MAF. Immunity (2017) 47(2):235–50.e4. doi: 10.1016/j.immuni.2017.07.017
58. Shapouri-Moghaddam A, Mohammadian S, Vazini H, Taghadosi M, Esmaeili SA, Mardani F, et al. Macrophage plasticity, polarization, and function in health and disease. J Cell Physiol (2018) 233(9):6425–40. doi: 10.1002/jcp.26429
59. Pan Y, Yang L, Chen D, Hou H, Zhang M, Chen M, et al. Decidual macrophage derived MMP3 contributes to extracellular matrix breakdown in spiral artery remodeling in early human pregnancy. J Reprod Immunol (2022) 150:103494. doi: 10.1016/j.jri.2022.103494
60. Siasos G, Tousoulis D, Kioufis S, Oikonomou E, Siasou Z, Limperi M, et al. Inflammatory mechanisms in atherosclerosis: the impact of matrix metalloproteinases. Curr Top Med Chem (2012) 12(10):1132–48. doi: 10.2174/1568026611208011132
61. Li H, Liang J, Castrillon DH, DePinho RA, Olson EN, Liu ZP. FoxO4 regulates tumor necrosis factor alpha-directed smooth muscle cell migration by activating matrix metalloproteinase 9 gene transcription. Mol Cell Biol (2007) 27(7):2676–86. doi: 10.1128/MCB.01748-06
62. Chandrasekar B, Mummidi S, Mahimainathan L, Patel DN, Bailey SR, Imam SZ, et al. Interleukin-18-induced human coronary artery smooth muscle cell migration is dependent on NF-kappaB- and AP-1-mediated matrix metalloproteinase-9 expression and is inhibited by atorvastatin. J Biol Chem (2006) 281(22):15099–109. doi: 10.1074/jbc.M600200200
63. Gonzalo P, Guadamillas MC, Hernández-Riquer MV, Pollán A, Grande-García A, Bartolomé RA, et al. MT1-MMP is required for myeloid cell fusion via regulation of Rac1 signaling. Dev Cell (2010) 18(1):77–89. doi: 10.1016/j.devcel.2009.11.012
64. Cougoule C, Le Cabec V, Poincloux R, Al Saati T, Mège JL, Tabouret G, et al. Three-dimensional migration of macrophages requires hck for podosome organization and extracellular matrix proteolysis. Blood (2010) 115(7):1444–52. doi: 10.1182/blood-2009-04-218735
65. Wang S, Qian J, Sun F, Li M, Ye J, Li M, et al. Bidirectional regulation between 1st trimester HTR8/SVneo trophoblast cells and in vitro differentiated Th17/Treg cells suggest a fetal-maternal regulatory loop in human pregnancy. Am J Reprod Immunol (2019) 81(5):e13106. doi: 10.1111/aji.13106
66. Gardner L, Moffett A. Dendritic cells in the human decidua. Biol Reprod (2003) 69(4):1438–46. doi: 10.1095/biolreprod.103.017574
67. Audiger C, Rahman MJ, Yun TJ, Tarbell KV, Lesage S. The importance of dendritic cells in maintaining immune tolerance. J Immunol (2017) 198(6):2223–31. doi: 10.4049/jimmunol.1601629
68. Wei R, Lai N, Zhao L, Zhang Z, Zhu X, Guo Q, et al. Dendritic cells in pregnancy and pregnancy-associated diseases. BioMed Pharmacother (2021) 133:110921. doi: 10.1016/j.biopha.2020.110921
69. Laskarin G, Kämmerer U, Rukavina D, Thomson AW, Fernandez N, Blois SM. Antigen-presenting cells and materno-fetal tolerance: an emerging role for dendritic cells. Am J Reprod Immunol (2007) 58(3):255–67. doi: 10.1111/j.1600-0897.2007.00511.x
70. Geijtenbeek TB, Kwon DS, Torensma R, van Vliet SJ, van Duijnhoven GC, Middel J, et al. DC-SIGN, a dendritic cell-specific HIV-1-binding protein that enhances trans-infection of T cells. Cell (2000) 100(5):587–97. doi: 10.1016/S0092-8674(00)80694-7
71. Kato M, Neil TK, Fearnley DB, McLellan AD, Vuckovic S, Hart DN. Expression of multilectin receptors and comparative FITC-dextran uptake by human dendritic cells. Int Immunol (2000) 12(11):1511–9. doi: 10.1093/intimm/12.11.1511
72. Kim MK, Kim J. Properties of immature and mature dendritic cells: phenotype, morphology, phagocytosis, and migration. RSC Adv (2019) 9(20):11230–8. doi: 10.1039/C9RA00818G
73. Kemp B, Schmitz S, Krusche CA, Rath W, von Rango U. Dendritic cells are equally distributed in intrauterine and tubal ectopic pregnancies. Fertil Steril (2011) 95(1):28–32. doi: 10.1016/j.fertnstert.2010.05.045
74. Sanjabi S, Oh SA, Li MO. Regulation of the immune response by TGF-β: From conception to autoimmunity and infection. Cold Spring Harb Perspect Biol (2017) 9(6):a022236. doi: 10.1101/cshperspect.a022236
75. Yu Q, Stamenkovic I. Cell surface-localized matrix metalloproteinase-9 proteolytically activates TGF-beta and promotes tumor invasion and angiogenesis. Genes Dev (2000) 14(2):163–76. doi: 10.1101/gad.14.2.163
76. Faas MM, de Vos P. Uterine NK cells and macrophages in pregnancy. Placenta (2017) 56:44–52. doi: 10.1016/j.placenta.2017.03.001
77. Choudhury RH, Dunk CE, Lye SJ, Harris LK, Aplin JD, Jones RL. Decidual leucocytes infiltrating human spiral arterioles are rich source of matrix metalloproteinases and degrade extracellular matrix in vitro and in situ. Am J Reprod Immunol (2019) 81(1):e13054. doi: 10.1111/aji.13054
78. Lash GE, Robson SC, Bulmer JN. Review: Functional role of uterine natural killer (uNK) cells in human early pregnancy decidua. Placenta (2010) 31 Suppl:S87–92. doi: 10.1016/j.placenta.2009.12.022
79. Hanna J, Goldman-Wohl D, Hamani Y, Avraham I, Greenfield C, Natanson-Yaron S, et al. Decidual NK cells regulate key developmental processes at the human fetal-maternal interface. Nat Med (2006) 12(9):1065–74. doi: 10.1038/nm1452
80. Zhang J, Chen Z, Smith GN, Croy BA. Natural killer cell-triggered vascular transformation: maternal care before birth? Cell Mol Immunol (2011) 8(1):1–11. doi: 10.1038/cmi.2010.38
81. Pitman H, Innes BA, Robson SC, Bulmer JN, Lash GE. Altered expression of interleukin-6, interleukin-8 and their receptors in decidua of women with sporadic miscarriage. Hum Reprod (2013) 28(8):2075–86. doi: 10.1093/humrep/det233
82. Burton GJ, Yung HW, Cindrova-Davies T, Charnock-Jones DS. Placental endoplasmic reticulum stress and oxidative stress in the pathophysiology of unexplained intrauterine growth restriction and early onset preeclampsia. Placenta (2009) 30 Suppl A(Suppl):S43–8. doi: 10.1016/j.placenta.2008.11.003
83. Mayrink J, Costa ML, Cecatti JG. Preeclampsia in 2018: Revisiting concepts, physiopathology, and prediction. ScientificWorldJournal (2018) 2018:6268276. doi: 10.1155/2018/6268276
84. Raguema N, Gannoun MBA, Zitouni H, Meddeb S, Benletaifa D, Lavoie JL, et al. Interleukin-10 rs1800871 (-819C/T) and ATA haplotype are associated with preeclampsia in a Tunisian population. Pregnancy Hypertens (2018) 11:105–10. doi: 10.1016/j.preghy.2018.01.011
85. Lu HQ, Hu R. The role of immunity in the pathogenesis and development of pre-eclampsia. Scand J Immunol (2019) 90(5):e12756. doi: 10.1111/sji.12756
86. Perez-Sepulveda A, Torres MJ, Khoury M, Illanes SE. Innate immune system and preeclampsia. Front Immunol (2014) 5:244. doi: 10.3389/fimmu.2014.00244
87. Hall D, Gebhardt S, Theron G, Grové D. Pre-eclampsia and gestational hypertension are less common in HIV infected women. Pregnancy Hypertens (2014) 4(1):91–6. doi: 10.1016/j.preghy.2013.11.008
88. Palei AC, Sandrim VC, Cavalli RC, Tanus-Santos JE. Comparative assessment of matrix metalloproteinase (MMP)-2 and MMP-9, and their inhibitors, tissue inhibitors of metalloproteinase (TIMP)-1 and TIMP-2 in preeclampsia and gestational hypertension. Clin Biochem (2008) 41(10-11):875–80. doi: 10.1016/j.clinbiochem.2008.04.015
89. Shokry M, Omran OM, Hassan HI, Elsedfy GO, Hussein MR. Expression of matrix metalloproteinases 2 and 9 in human trophoblasts of normal and preeclamptic placentas: preliminary findings. Exp Mol Pathol (2009) 87(3):219–25. doi: 10.1016/j.yexmp.2009.08.001
90. Lavee M, Goldman S, Daniel-Spiegel E, Shalev E. Matrix metalloproteinase-2 is elevated in midtrimester amniotic fluid prior to the development of preeclampsia. Reprod Biol Endocrinol (2009) 7:85. doi: 10.1186/1477-7827-7-85
91. Hu M, Wang Y, Meng Y, Hu J, Qiao J, Zhen J, et al. Hypoxia induced-disruption of lncRNA TUG1/PRC2 interaction impairs human trophoblast invasion through epigenetically activating Nodal/ALK7 signalling. J Cell Mol Med (2022) 26(14):4087–100. doi: 10.1111/jcmm.17450
92. Plaks V, Rinkenberger J, Dai J, Flannery M, Sund M, Kanasaki K, et al. Matrix metalloproteinase-9 deficiency phenocopies features of preeclampsia and intrauterine growth restriction. Proc Natl Acad Sci U S A (2013) 110(27):11109–14. doi: 10.1073/pnas.1309561110
93. Timokhina E, Strizhakov A, Ibragimova S, Gitel E, Ignatko I, Belousova V, et al. Matrix metalloproteinases MMP-2 and MMP-9 occupy a new role in severe preeclampsia. J Pregnancy (2020) 2020:8369645. doi: 10.1155/2020/8369645
94. Li W, Mata KM, Mazzuca MQ, Khalil RA. Altered matrix metalloproteinase-2 and -9 expression/activity links placental ischemia and anti-angiogenic sFlt-1 to uteroplacental and vascular remodeling and collagen deposition in hypertensive pregnancy. Biochem Pharmacol (2014) 89(3):370–85. doi: 10.1016/j.bcp.2014.03.017
95. Ahmad S, Ahmed A. Elevated placental soluble vascular endothelial growth factor receptor-1 inhibits angiogenesis in preeclampsia. Circ Res (2004) 95(9):884–91. doi: 10.1161/01.RES.0000147365.86159.f5
96. Liao L, Zhao X, Zhou M, Deng Y, Li Y, Peng C. sFlt-1: A double regulator in angiogenesis-related diseases. Curr Pharm Des (2021) 27(40):4160–70. doi: 10.2174/1381612827666210902155015
97. March MI, Geahchan C, Wenger J, Raghuraman N, Berg A, Haddow H, et al. Circulating angiogenic factors and the risk of adverse outcomes among Haitian women with preeclampsia. PloS One (2015) 10(5):e0126815. doi: 10.1371/journal.pone.0126815
98. Bian X, Biswas A, Huang X, Lee KJ, Li TK, Masuyama H, et al. Short-term prediction of adverse outcomes using the sFlt-1 (Soluble fms-like tyrosine kinase 1)/PlGF (Placental growth factor) ratio in Asian women with suspected preeclampsia. Hypertension (2019) 74(1):164–72. doi: 10.1161/HYPERTENSIONAHA.119.12760
99. Jeon HR, Jeong DH, Lee JY, Woo EY, Shin GT, Kim SY. sFlt-1/PlGF ratio as a predictive and prognostic marker for preeclampsia. J Obstet Gynaecol Res (2021) 47(7):2318–23. doi: 10.1111/jog.14815
100. Zeisler H, Hund M, Verlohren S. The sFlt-1:PlGF ratio in women with suspected preeclampsia. New Engl J Med (2016) 374(18):1785–6. doi: 10.1056/NEJMc1602338
101. Thadhani R, Hagmann H, Schaarschmidt W, Roth B, Cingoez T, Karumanchi SA, et al. Removal of soluble fms-like tyrosine kinase-1 by dextran sulfate apheresis in preeclampsia. J Am Soc Nephrol (2016) 27(3):903–13. doi: 10.1681/ASN.2015020157
102. Reister F, Frank HG, Kingdom JC, Heyl W, Kaufmann P, Rath W, et al. Macrophage-induced apoptosis limits endovascular trophoblast invasion in the uterine wall of preeclamptic women. Lab Invest (2001) 81(8):1143–52. doi: 10.1038/labinvest.3780326
103. Poon LC, Nekrasova E, Anastassopoulos P, Livanos P, Nicolaides KH. First-trimester maternal serum matrix metalloproteinase-9 (MMP-9) and adverse pregnancy outcome. Prenat Diagn (2009) 29(6):553–9. doi: 10.1002/pd.2234
104. Brunner S, Kim JO, Methe H. Relation of matrix metalloproteinase-9/tissue inhibitor of metalloproteinase-1 ratio in peripheral circulating CD14+ monocytes to progression of coronary artery disease. Am J Cardiol (2010) 105(4):429–34. doi: 10.1016/j.amjcard.2009.10.013
105. Li D, Zheng L, Zhao D, Xu Y, Wang Y. The role of immune cells in recurrent spontaneous abortion. Reprod Sci (2021) 28(12):3303–15. doi: 10.1007/s43032-021-00599-y
106. Guo X, Yi H, Li TC, Wang Y, Wang H, Chen X. Role of vascular endothelial growth factor (VEGF) in human embryo implantation: Clinical implications. Biomolecules (2021) 11(2):253. doi: 10.3390/biom11020253
107. Shibuya M. Differential roles of vascular endothelial growth factor receptor-1 and receptor-2 in angiogenesis. J Biochem Mol Biol (2006) 39(5):469–78. doi: 10.5483/BMBRep.2006.39.5.469
108. Melincovici CS, Boşca AB, Şuşman S, Mărginean M, Mihu C, Istrate M, et al. Vascular endothelial growth factor (VEGF) - key factor in normal and pathological angiogenesis. Rom J Morphol Embryol (2018) 59(2):455–67. https://rjme.ro/RJME/resources/files/590218455467.pdf.
109. Lash GE, Innes BA, Drury JA, Robson SC, Quenby S, Bulmer JN. Localization of angiogenic growth factors and their receptors in the human endometrium throughout the menstrual cycle and in recurrent miscarriage. Hum Reprod (2012) 27(1):183–95. doi: 10.1093/humrep/der376
110. He X, Chen Q. Reduced expressions of connexin 43 and VEGF in the first-trimester tissues from women with recurrent pregnancy loss. Reprod Biol Endocrinol (2016) 14(1):46. doi: 10.1186/s12958-016-0179-4
111. Huang M, Huang B, Li G, Zeng S. Apatinib affect VEGF-mediated cell proliferation, migration, invasion via blocking VEGFR2/RAF/MEK/ERK and PI3K/AKT pathways in cholangiocarcinoma cell. BMC Gastroenterol (2018) 18(1):169. doi: 10.1186/s12876-018-0870-3
112. Chen Y, Chen X, Ding X, Wang Y. Afatinib, an EGFR inhibitor, decreases EMT and tumorigenesis of Huh−7 cells by regulating the ERK−VEGF/MMP9 signaling pathway. Mol Med Rep (2019) 20(4):3317–25. doi: 10.3892/mmr.2019.10562
113. Xu X, Du C, Li H, Du J, Yan X, Peng L, et al. Association of VEGF genetic polymorphisms with recurrent spontaneous abortion risk: a systematic review and meta-analysis. PloS One (2015) 10(4):e0123696. doi: 10.1371/journal.pone.0123696
114. Pandey M, Awasthi S. Role of MMP-1, MMP-8 and MMP-9 gene polymorphisms in preterm birth. J Genet (2020) 99:2. doi: 10.1007/s12041-019-1161-7
115. Benkhalifa M, Zidi W, Bahri H, Mahjoub S, Boudhraa K, Sanhaji H, et al. Circulating MMP-7 and VEGF as potential predictive biomarkers for recurrent implantation failures. Zygote (2021) 29(5):365–71. doi: 10.1017/S0967199421000113
116. Guerrero B, Hassouneh F, Delgado E, Casado JG, Tarazona R. Natural killer cells in recurrent miscarriage: An overview. J Reprod Immunol (2020) 142:103209. doi: 10.1016/j.jri.2020.103209
117. Azizieh FY, Raghupathy R. IL-10 and pregnancy complications. Clin Exp Obstet Gynecol (2017) 44(2):252–8. doi: 10.12891/ceog3456.2017
118. Lash GE, Otun HA, Innes BA, Kirkley M, De Oliveira L, Searle RF, et al. Interferon-gamma inhibits extravillous trophoblast cell invasion by a mechanism that involves both changes in apoptosis and protease levels. FASEB J (2006) 20(14):2512–8. doi: 10.1096/fj.06-6616com
119. Peng Y, Yin S, Wang M. Significance of the ratio interferon-γ/interleukin-4 in early diagnosis and immune mechanism of unexplained recurrent spontaneous abortion. Int J Gynaecol Obstet (2021) 154(1):39–43. doi: 10.1002/ijgo.13494
120. Raghupathy R. Pregnancy: success and failure within the Th1/Th2/Th3 paradigm. Semin Immunol (2001) 13(4):219–27. doi: 10.1006/smim.2001.0316
121. Wilson R, McInnes I, Leung B, McKillop JH, Walker JJ. Altered interleukin 12 and nitric oxide levels in recurrent miscarriage. Eur J Obstet Gynecol Reprod Biol (1997) 75(2):211–4. doi: 10.1016/S0301-2115(97)00124-3
122. Zhao X, Jiang Y, Ping Y, Guo H, He M, Feng X. Associations between tumor necrosis factor-α and interleukin-6 polymorphisms and unexplained recurrent spontaneous abortion risk: A meta-analysis. Med (Baltimore) (2019) 98(46):e17919. doi: 10.1097/MD.0000000000017919
123. Löb S, Amann N, Kuhn C, Schmoeckel E, Wöckel A, Zati Zehni A, et al. Interleukin-1 beta is significantly upregulated in the decidua of spontaneous and recurrent miscarriage placentas. J Reprod Immunol (2021) 144:103283. doi: 10.1016/j.jri.2021.103283
124. Hadinedoushan H, Mirahmadian M, Aflatounian A. Increased natural killer cell cytotoxicity and IL-2 production in recurrent spontaneous abortion. Am J Reprod Immunol (2007) 58(5):409–14. doi: 10.1111/j.1600-0897.2007.00524.x
125. Madduru D, Dirsipam K, Goli M, Ramana Devi V, Jahan P. Association of reduced maternal sHLA-G5 isoform levels and elevated TNF-α/IL-4 cytokine ratio with recurrent pregnancy loss: A study on south Indian women. Scand J Immunol (2021) 94(4):e13095. doi: 10.1111/sji.13095
126. Qian J, Zhang N, Lin J, Wang C, Pan X, Chen L, et al. Distinct pattern of Th17/Treg cells in pregnant women with a history of unexplained recurrent spontaneous abortion. Biosci Trends (2018) 12(2):157–67. doi: 10.5582/bst.2018.01012
127. Ozkan ZS, Deveci D, Simsek M, Ilhan F, Risvanli A, Sapmaz E. What is the impact of SOCS3, IL-35 and IL17 in immune pathogenesis of recurrent pregnancy loss? J Matern Fetal Neonatal Med (2015) 28(3):324–8. doi: 10.3109/14767058.2014.916676
128. Hua F, Li CH, Wang H, Xu HG. Relationship between expression of COX-2, TNF-α, IL-6 and autoimmune-type recurrent miscarriage. Asian Pac J Trop Med (2013) 6(12):990–4. doi: 10.1016/S1995-7645(13)60178-9
129. Danaii S, Ghorbani F, Ahmadi M, Abbaszadeh H, Koushaeian L, Soltani-Zangbar MS, et al. IL-10-producing b cells play important role in the pathogenesis of recurrent pregnancy loss. Int Immunopharmacol (2020) 87:106806. doi: 10.1016/j.intimp.2020.106806
130. Toth B, Haufe T, Scholz C, Kuhn C, Friese K, Karamouti M, et al. Placental interleukin-15 expression in recurrent miscarriage. Am J Reprod Immunol (2010) 64(6):402–10. doi: 10.1111/j.1600-0897.2010.00879.x
131. Löb S, Ochmann B, Ma Z, Vilsmaier T, Kuhn C, Schmoeckel E, et al. The role of interleukin-18 in recurrent early pregnancy loss. J Reprod Immunol (2021) 148:103432. doi: 10.1016/j.jri.2021.103432
132. O'Hern Perfetto C, Fan X, Dahl S, Krieg S, Westphal LM, Bunker Lathi R, et al. Expression of interleukin-22 in decidua of patients with early pregnancy and unexplained recurrent pregnancy loss. J Assist Reprod Genet (2015) 32(6):977–84. doi: 10.1007/s10815-015-0481-7
133. Giannubilo SR, Landi B, Pozzi V, Sartini D, Cecati M, Stortoni P, et al. The involvement of inflammatory cytokines in the pathogenesis of recurrent miscarriage. Cytokine (2012) 58(1):50–6. doi: 10.1016/j.cyto.2011.12.019
134. Wang WJ, Liu FJ, Qu HM, Hao CF, Qu QL, Xiong W, et al. Regulation of the expression of Th17 cells and regulatory T cells by IL-27 in patients with unexplained early recurrent miscarriage. J Reprod Immunol (2013) 99(1-2):39–45. doi: 10.1016/j.jri.2013.04.002
135. Hu WT, Li MQ, Liu W, Jin LP, Li DJ, Zhu XY. IL-33 enhances proliferation and invasiveness of decidual stromal cells by up-regulation of CCL2/CCR2 via NF-κB and ERK1/2 signaling. Mol Hum Reprod (2014) 20(4):358–72. doi: 10.1093/molehr/gat094
136. Bruce S, Sorosky J. Gestational trophoblastic disease. StatPearls. Treasure Island (FL: StatPearls PublishingCopyright © 2022, StatPearls Publishing LLC (2022).
137. Rahat B, Sharma R, Bagga R, Hamid A, Kaur J. Imbalance between matrix metalloproteinases and their tissue inhibitors in preeclampsia and gestational trophoblastic diseases. Reproduction (2016) 152(1):11–22. doi: 10.1530/REP-16-0060
138. Petignat P, Laurini R, Goffin F, Bruchim I, Bischof P. Expression of matrix metalloproteinase-2 and mutant p53 is increased in hydatidiform mole as compared with normal placenta. Int J Gynecol Cancer (2006) 16(4):1679–84. doi: 10.1111/j.1525-1438.2006.00643.x
139. Ding F, Zhang QS, Xing FQ. [MMP-2/TIMP-2 expression in the trophoblasts of patients with gestational trophoblastic disease]. Nan Fang Yi Ke Da Xue Xue Bao (2007) 27(2):150–2. https://kns.cnki.net/kcms/detail/detail.aspx?dbcode=CJFD&dbname=CJFD2007&filename=DYJD200702007&uniplatform=NZKPT&v=T0EFysgR7nGiaGsXy0vRLZfb85CXao_5rDs3nc9foKr8zNFehO5F2ST9eNsVos5w.
140. Li Z, Xiang Y, Dai L. [Prediction of malignant transformation of hydatidiform mole by mRNA determination of matrix metalloproteinases and tissue inhibitor of metalloproteinases]. Zhonghua Fu Chan Ke Za Zhi (2000) 35(9):547–50. https://kns.cnki.net/kcms/detail/detail.aspx?dbcode=CJFD&dbname=CJFD2000&filename=ZHFC200009015&uniplatform=NZKPT&v=0vYH4XnvPbADR1IQsq3J9SNkWHEwGUcboZDp5Q1bOgNG_Eq4SWR48ahkxl9n5_a3.
141. Nicolai E, Sinibaldi F, Sannino G, Laganà G, Basoli F, Licoccia S, et al. Omega-3 and Omega-6 Fatty Acids Act as Inhibitors of the Matrix Metalloproteinase-2 and Matrix Metalloproteinase-9 Activity. Protein J (2017) 36(4):278–85. doi: 10.3892/ijmm.2012.960
142. Singh M, Kindelberger D, Nagymanyoki Z, Ng SW, Quick CM, Elias KM, et al. Matrix metalloproteinases and their inhibitors and inducer in gestational trophoblastic diseases and normal placenta. Gynecol Oncol (2011) 122(1):178–82. doi: 10.1016/j.ygyno.2011.03.025
143. Quintero-Fabián S, Arreola R, Becerril-Villanueva E, Torres-Romero JC, Arana-Argáez V, Lara-Riegos J, et al. Role of matrix metalloproteinases in angiogenesis and cancer. Front Oncol (2019) 9:1370. doi: 10.3389/fonc.2019.01370
144. Harris MA, Hansen RA, Vidsudhiphan P, Koslo JL, Thomas JB, Watkins BA, et al. Effects of conjugated linoleic acids and docosahexaenoic acid on rat liver and reproductive tissue fatty acids, prostaglandins and matrix metalloproteinase production. Prostaglandins Leukot Essent Fatty Acids (2001) 65(1):23–9. doi: 10.4103/0971-6580.128818
145. Baker AH, Edwards DR, Murphy G. Metalloproteinase inhibitors: biological actions and therapeutic opportunities. J Cell Sci (2002) 115(Pt 19):3719–27. doi: 10.1242/jcs.00063
146. Jacobsen J, Visse R, Sørensen HP, Enghild JJ, Brew K, Wewer UM, et al. Catalytic properties of ADAM12 and its domain deletion mutants. Biochemistry (2008) 47(2):537–47. doi: 10.1021/bi701629c
147. Siloşi I, Boldeanu MV, Mogoantă S, Ghiluşi M, Cojocaru M, Biciuşcă V, et al. Matrix metalloproteinases (MMP-3 and MMP-9) implication in the pathogenesis of inflammatory bowel disease (IBD). Rom J Morphol Embryol (2014) 55(4):1317–24. https://rjme.ro/RJME/resources/files/55041413171324.pdf.
148. Kofla-Dłubacz A, Matusiewicz M, Krzesiek E, Noga L, Iwańczak B. Metalloproteinase-3 and -9 as novel markers in the evaluation of ulcerative colitis activity in children. Adv Clin Exp Med (2014) 23(1):103–10. doi: 10.17219/acem/37031
149. Goffin L, Fagagnini S, Vicari A, Mamie C, Melhem H, Weder B, et al. Anti-MMP-9 antibody: A promising therapeutic strategy for treatment of inflammatory bowel disease complications with fibrosis. Inflammation Bowel Dis (2016) 22(9):2041–57. doi: 10.1097/MIB.0000000000000863
150. Marusak C, Bayles I, Ma J, Gooyit M, Gao M, Chang M, et al. The thiirane-based selective MT1-MMP/MMP2 inhibitor ND-322 reduces melanoma tumor growth and delays metastatic dissemination. Pharmacol Res (2016) 113(Pt A):515–20. doi: 10.1016/j.phrs.2016.09.033
151. Xu J, Shen ZY, Chen XG, Zhang Q, Bian HJ, Zhu P, et al. A randomized controlled trial of licartin for preventing hepatoma recurrence after liver transplantation. Hepatology (2007) 45(2):269–76. doi: 10.1002/hep.21465
152. Yun EJ, Song KS, Shin S, Kim S, Heo JY, Kweon GR, et al. Docosahexaenoic acid suppresses breast cancer cell metastasis by targeting matrix-metalloproteinases. Oncotarget (2016) 7(31):49961–71. doi: 10.18632/oncotarget.10266
153. Nicolai E, Sinibaldi F, Sannino G, Laganà G, Basoli F, Licoccia S, et al. Omega-3 and omega-6 fatty acids act as inhibitors of the matrix metalloproteinase-2 and matrix metalloproteinase-9 activity. Protein J (2017) 36(4):278–85. doi: 10.1007/s10930-017-9727-9
154. Haast RA, Kiliaan AJ. Impact of fatty acids on brain circulation, structure and function. Prostaglandins Leukot Essent Fatty Acids (2015) 92:3–14. doi: 10.1016/j.plefa.2014.01.002
155. Frew L, Sugiarto NU, Rajagopal SP, He J, Leask R, Norman JE, et al. The effect of omega-3 polyunsaturated fatty acids on the inflammatory response of the amnion. Prostaglandins Leukot Essent Fatty Acids (2013) 89(4):221–5. doi: 10.1016/j.plefa.2013.07.001
156. Harris MA, Hansen RA, Vidsudhiphan P, Koslo JL, Thomas JB, Watkins BA, et al. Effects of conjugated linoleic acids and docosahexaenoic acid on rat liver and reproductive tissue fatty acids, prostaglandins and matrix metalloproteinase production. Prostaglandins Leukot Essent Fatty Acids (2001) 65(1):23–9. doi: 10.1054/plef.2001.0283
157. Sundrani DP, Chavan-Gautam PM, Pisal HR, Mehendale SS, Joshi SR. Matrix metalloproteinase-1 and -9 in human placenta during spontaneous vaginal delivery and caesarean sectioning in preterm pregnancy. PloS One (2012) 7(1):e29855. doi: 10.1371/journal.pone.0029855
158. Odendaal J, Quenby S, Sammaritano L, Macklon N, Branch DW, Rosenwaks Z. Immunologic and rheumatologic causes and treatment of recurrent pregnancy loss: what is the evidence? Fertil Steril (2019) 112(6):1002–12. doi: 10.1016/j.fertnstert.2019.10.002
159. Death AK, Fisher EJ, McGrath KC, Yue DK. High glucose alters matrix metalloproteinase expression in two key vascular cells: potential impact on atherosclerosis in diabetes. Atherosclerosis (2003) 168(2):263–9. doi: 10.1016/S0021-9150(03)00140-0
160. Chung AW, Hsiang YN, Matzke LA, McManus BM, van Breemen C, Okon EB. Reduced expression of vascular endothelial growth factor paralleled with the increased angiostatin expression resulting from the upregulated activities of matrix metalloproteinase-2 and -9 in human type 2 diabetic arterial vasculature. Circ Res (2006) 99(2):140–8. doi: 10.1161/01.RES.0000232352.90786.fa
Keywords: matrix metalloproteinase, maternal-fetal interface immune microenvironment, pregnancy-related diseases, matrix metalloproteinase inhibitor, immunotherapy
Citation: Jing M, Chen X, Qiu H, He W, Zhou Y, Li D, Wang D, Jiao Y and Liu A (2023) Insights into the immunomodulatory regulation of matrix metalloproteinase at the maternal-fetal interface during early pregnancy and pregnancy-related diseases. Front. Immunol. 13:1067661. doi: 10.3389/fimmu.2022.1067661
Received: 13 October 2022; Accepted: 19 December 2022;
Published: 09 January 2023.
Edited by:
Raj Raghupathy, Kuwait University, KuwaitReviewed by:
Andrea Balduit, Institute for Maternal and Child Health Burlo Garofolo (IRCCS), ItalyVladimir Stanislavovich Rogovskii, Independent Researcher, Switzerland
Copyright © 2023 Jing, Chen, Qiu, He, Zhou, Li, Wang, Jiao and Liu. This is an open-access article distributed under the terms of the Creative Commons Attribution License (CC BY). The use, distribution or reproduction in other forums is permitted, provided the original author(s) and the copyright owner(s) are credited and that the original publication in this journal is cited, in accordance with accepted academic practice. No use, distribution or reproduction is permitted which does not comply with these terms.
*Correspondence: Yonghui Jiao, SnloMzgxMEAxNjMuY29t; Dimin Wang, ZGltaW53YW5nQHpqdS5lZHUuY24=; Aixia Liu, bGl1YWl4aWFAemp1LmVkdS5jbg==
†These authors have contributed equally to this work