- 1Key Laboratory of South China Sea Fishery Resources Exploitation and Utilization, Ministry of Agriculture and Rural Affairs, South China Sea Fisheries Research Institute, Chinese Academy of Fishery Sciences, Guangzhou, China
- 2College of Fisheries, Dalian Ocean University, Dalian, China
- 3Sanya Tropical Fisheries Research Institute, Sanya, China
- 4Guangdong Provincial Engineer Technology Research Center of Marine Biological Seed Industry, Guangzhou, China
As the precursor of taurine, cysteine serves physiological functions, such as anti-oxidative stress and immune improvement. Investigation of cysteine and its derivatives has made positive progress in avian and mammalian species, yet the study and application of cysteine in aquatic animals are relatively rare. Therefore, we evaluated the effects of supplementing a low-fishmeal diet with various levels of cysteine on the growth, antioxidant capacity, intestine immunity, and resistance against Streptococcus agalactiae of the juvenile golden pompano (Trachinotus ovatus). According to our study, exogenous supplementation with 0.6-1.2% cysteine greatly increased the final body weight (FBW) and specific growth rate (SGR) of golden pompano compared to the control group. Under the present conditions, the optimum dietary cysteine supplementation level for golden pompano was 0.91% based on the polynomial regression analysis of SGR. Meanwhile, we found that the Nrf2/Keap1/HO-1 signaling pathway was notably upregulated with the increase of exogenous cysteine, which increased antioxidant enzyme activity in serum and gene expression in the intestine and reduced the level of reactive oxygen species (ROS) in the serum of golden pompano. In addition, morphological analysis of the midgut demonstrated that exogenous cysteine improved muscle thickness and villi length, which suggested that the physical barrier of the intestine was greatly strengthened by cysteine. Moreover, cysteine increased the diversity and relative abundance of the intestinal flora of golden pompano. Cysteine suppressed intestinal NF-κB/IKK/IκB signaling and pro-inflammatory cytokine mRNA levels. Conversely, intestinal anti-inflammatory cytokine gene expression and serum immune parameters were upregulated with the supplementary volume of cysteine and improved intestine immunity. Further, exogenous cysteine supplementation greatly reduced the mortality rate of golden pompano challenged with S. agalactiae. In general, our findings provide more valuable information and new insights into the rational use of cysteine in the culture of healthy aquatic animals.
Introduction
As a major concern of the global aquaculture industry, the price of fishmeal has markedly increased over the past years, making the identification of fishmeal substitutes an urgent matter (1–3). However, previous studies indicate that neither plant protein, such as soybean meal (1, 2), rapeseed protein (3), and corn gluten meal (1, 4), nor animal proteins, such as insect meal (5), poultry by-product meal (1), and chicken meal (6), which are closer to fish meal in terms of nutrient composition, can completely replace fish meal in the diet of aquatic animals. As good sources of protein, these alternatives may have advantages in terms of their price, and small quantities required, however, the role of fishmeal as a bottleneck in aquafeeds seems impenetrable (7–9). Studies have shown that if the proportion of fishmeal is markedly reduced, it can cause a change in the intestinal microbiota of aquatic animals (10, 11), leading to a decline in growth performance (11, 12). The presence of abundant anti-nutritional factors in their diet can elicit oxidative stress in aquatic animals (9, 11). This typically results in structural damage to the intestinal tract (12) and ultimately leads to a decline in intestine immunity (8, 12–15), reducing the ability of aquatic animals to cope with external pathogen infection and, thus, leading to death. Therefore, considering fishmeal scarcity and the lack of an adequate substitution, it is important to explore novel ways to reduce the proportion of fishmeal in aquatic animal diets while maintaining growth, reducing oxidative stress, and enhancing intestine immunity.
Previous studies have shown that appropriate inclusion of functional supplements in aquatic diets can effectively mitigate the adverse effects of low-fishmeal diets on fish (11, 16). Taurine is a functional amino acid that promotes fish growth and immunity, and its levels are correlated with fish health (17–19). The primary source of dietary taurine is fishmeal, thus reducing the proportion of fishmeal in the diet of fish will inevitably decrease dietary taurine content and reduce growth performance and intestine immunity (16, 19).
Cysteine, the precursor of taurine in living organisms (20, 21), has important biological functions, including antioxidative (22) and immunity (23) enhancing effects. Research on the functions of cysteine and its derivatives in mammals, such as rat (22, 24), pig (25–27), and sheep (28, 29), has produced considerable advances; however, respective research and applications in aquatic animals are relatively scarce. Studies in rats have indicated that cysteine as a dietary additive improves resistance to oxidative stress associated with high sucrose levels (22). Moreover, the potential of cysteine to enhance intestine immunity may be related to its resistance to oxidative stress and defense against inflammatory responses (24). For example, cysteine may protect the physical barrier of piglet intestines through the Nrf2 and NF-κB pathways, increasing the length of intestinal villi, and protecting intestinal structure and functioning (25).
The intestine immunity system, consisting of physical barriers, intestinal flora, and immune barriers, is essential for organismic health (30), and cysteine has been shown to improve intestine immunity. For example, dietary supplementation with 0.4% cysteine can increase the quantity and diversity of intestinal microorganisms in gestating sows (31). In addition, cysteine can revive the intestine immunity system in pigs by downregulating the mRNA levels of pro-inflammatory cytokines and improving the local inflammatory response in the intestine (27). Moreover, cysteine supplementation may, to some extent, protect broiler chickens infected with Eimeria from intestinal lesions (32).
The golden pompano (Trachinotus ovatus) is one of the predominant aquaculture fish in China, however, the effects and mechanisms of cysteine on the intestine immunity of golden pompano are not entirely clear. Theoretically, satisfying the 42% dietary protein level of golden pompano with Peruvian fishmeal, containing 67% protein, would require a diet with a minimum of 60% fishmeal, which would inevitably increase the cost of farming given the current shortage of fishmeal resources (33). Nevertheless, diets with lower fishmeal content tend to reduce the immune capacity of fish (8, 9). Infectious diseases caused by Streptococcus agalactiae frequently broke out in recent years in large-scale cultures of marine fish due to excessive farming density and increasing environmental pollution (34). Therefore, we explored the effects of cysteine-supplemented low-fishmeal diets (containing 20% fishmeal) on growth performance, intestine immunity, and resistance against S. agalactiae in juvenile golden pompano in order to provide further insights into the mechanism of cysteine regulation of the intestine immunity of fish and to identify a solution for future usage of low-fishmeal diets.
Materials and methods
Ethical statement
All experiments in this study were conducted in accordance with the regulations and guidelines established and approved by the Animal Care and Use Committee of the South China Sea Fisheries Research Institute of the Chinese Academy of Fishery Sciences (No. SCSFRI96-253).
Experimental diets
Experimental diets of golden pompano were supplemented with food-grade cysteine (99.99% purity, Zhejiang Yi Nuo Biotechnology Co. Ltd., Wenzhou, China). The remaining ingredients were supplied by Guangzhou Nutriera Biotechnology Co. Ltd. (Guangzhou, China); Table 1 shows dietary formulations and nutritional levels. We produced five diets with equal nitrogen and lipid, based on the nutritional requirement of 42% protein for golden pompano (35). We mixed protein sources to avoid one of them reducing palatability; animal protein (fish meal and chicken meal) and plant protein (soybean protein concentrate, fermented soybean meal, and corn protein meal) were used as the base protein sources for golden pompano diets. Fish oil and soybean oil were used as sources of lipids. High gluten flour provided relatively little protein and fat and was used as a gamete to achieve the same quality in all diets (33). We set the amount of cysteine added to the diet at 0.00%, 0.30%, 0.60%, 0.90%, and 1.20% for groups C0 (control), C1, C2, C3, and C4 respectively, with reference to the amount of cysteine added to the Paralichthys olivaceus diet (36). In brief, the first step was to grind all solid ingredients separately until they could pass through a 40-mesh screen. Secondly, the ground ingredients were mixed at the proportions shown in Table 1; oil and water were added in batches during mixing, and each batch of diets was mixed for 30 min to achieve homogenization. The mixture was then placed in an extruder (Valva Machinery Co., Ltd., Guangzhou, China), and the diets were transformed into three sizes of spherical pellets (1, 2, and 3 mm diameter) to suit the growth state of the fish (37). Finally, the diets were placed in a drying oven at 45°C until the moisture content was reduced to approximately 10% (38); thereafter, the pellets were stored 4°C. Table 2 shows the amino acid composition of each diet.
Experimental procedure
To reproduce the large-scale culture environment of golden pompano, we used offshore cages (1.00 × 1.00 × 1.50 m) in Longgang District, Shenzhen, China. S. agalactiae was extracted from golden pompano and tested for its pathogenicity. The fish used for this experiment were selected from juvenile golden pompano bred in our laboratory throughout the year. Before the experiment, we separated 1,050 fish (10.05 ± 0.05 g) into 15 cages (five diets, three cages per diet), with 70 fish per cage. Since fish mortality is inevitable during the feeding process, we set the number of fish per cage to 70 to ensure that there would also be enough fish for subsequent challenge experiments. The fish were then fed the C0 diet without exogenous cysteine for one week to allow the fish to adjust to the experimental conditions. During the experiment, we fed the fish their respective diet at 8:00, 10:00, 14:00, and 16:00 each day, until the fish stopped eating. During the eight-week feeding period, feed intake status and hydrographic information at sea were observed and recorded daily. During the test period, the water conditions were maintained as follows: temperature at 28–32°C, pH at 7.4–8.3, salinity at 34–36 ‰ and dissolved oxygen > 6.0 mg/L.
Sample collection
At the end of the feeding experiment, the fish were fasted for 24 h, after which the fish were weighed and counted in each cage. Nine fish per cage were randomly chosen, and anesthetized with eugenol (100–200 mg/L; Shanghai Medical Devices Co., Ltd., Shanghai, China). Three fish per cage were transferred to -20°C after rapid freezing with liquid nitrogen for organism composition analysis. From three other individuals per cage, we collected blood and centrifuged it to obtain serum for measuring anti-oxidative stress and immunological parameters. After serum collection, the fish were dissected, and the intestines were collected for RNA extraction and gene expression analysis. The intestinal contents were removed and stored at -80°C for intestinal flora analysis. The midguts of the remaining three fish per cage were collected and preserved in 4% paraformaldehyde solution for histological analysis.
Growth performance
Growth performance-related parameters were calculated according to the following equations:
Serum biochemical and immunological parameter
To investigate the effects of exogenous dietary cysteine on the anti-oxidative stress capacity and immunity in juvenile golden pompano, we examined the relevant parameters in serum. For antioxidant indices, we determined the levels of MDA, ROS, and the activities of antioxidant enzymes, such as T-AOC, CAT, GSH-PX, and SOD. Serum immunological indicators included LZM, complement 3, complement 4, and immunoglobulins (IgA, IgG, and IgM).
Midgut histological examination
In accordance with a previous study (39), we stained the fish midgut with hematoxylin and eosin to observe effects of exogenous cysteine on the intestinal physical barrier. Briefly, the midguts were stored in a 4% paraformaldehyde solution for 24 h. We used ethanol to gradually eliminate the moisture. The midguts were then transferred to paraffin. When the paraffin was solidified, it was cut into 5-μm-thick slices using a microtome. Next, the slices were placed on slides and were stained with hematoxylin and eosin for nucleus and cytoplasm staining. Finally, we completely scanned the midgut sections using a light microscope (Leica, Wetzlar, Germany) with 200-fold magnification, divided the scans into eight equal parts, and randomly measured the length of intact intestinal villi, muscle thickness, and the number of goblet cells per villus in each part using Image-Pro Plus 6.0 software (National Institutes of Health, Bethesda, USA). The data were imported into GraphPad Prism 8 software (San Diego, California, USA) to examine differences and to draw graphs.
16S rDNA high-throughput sequencing of the intestinal flora
According to previous studies (39, 40), total DNA of intestinal bacteria was obtained using a DNA extraction kit (TIANGEN BIOTECH Co., Ltd., Beijing, China), DNA integrity was assessed using 1% agarose gel electrophoresis, and concentration was measured using a Nanodrop 2000 device (Thermo Fisher Scientific, Waltham, MA, USA). The DNA samples were diluted to 1 ng·μL-1 using sterile water, and PCR amplification of the bacterial 16S rDNA V3–V4 variable region was performed using specific primers. The primers 341F (5′-CCTAYGGGRBGCASCAG-3′) and 806R (5′-GGACTACNNGGGTATCTAAT-3′) were used to amplify the V3–V4 variable region. The reaction system and procedure for PCR amplification were used as described previously (39). PCR products were recovered using an AxyPrepDNA Gel Recovery Kit (AXYGEN Inc., California, USA) for gel cutting, Tris HCl elution, and 2% agarose electrophoresis for detection. The PCR products were quantified using a QuantiFluor™-ST Blue Fluorescence Quantification System (Promega Corporation, Madison, Wisconsin, USA), based on the preliminary quantification results of electrophoresis. Paired-end Illumina libraries were then constructed by mixing the corresponding ratios according to the sequencing volume requirement of each sample. Paired-end reads obtained using an Illumina sequencing platform (Illumina, San Diego, CA, USA) were first spliced according to the overlapping relationship, the sequences were quality-controlled and filtered, and the samples were differentiated and then subjected to operational taxonomic unit (OTU) clustering and clustering-based taxonomy analyses. Various diversity indices were produced based on the OTU clustering analysis. Abundance, alpha diversity (Chao1 index, Shannon index, and Simpson index), beta diversity (principal component analysis [PCA], UniFrac-based principal coordinate analysis [Pcoa], and UniFrac-based non-metric multi-dimensional scaling [NMDS] analysis), and linear discriminatory analysis were performed on OTUs to obtain information on species richness and evenness within the samples. Dilution curves were plotted using R software (V3.6.3, University of Auckland, New Zealand.) (36).
Quantitative real-time PCR
To further examine the modulatory effects of dietary cysteine on the growth, anti-oxidative stress, and intestine immunity of golden pompano, we assayed the expression of several genes. For the antioxidant stress capacity, we measured mRNA levels of antioxidant enzymes (CAT, GSH-PX, and SOD), critical factors of the Nrf2 pathway (Nrf2 and Keap-1), and HO-1 in the intestine of golden pompano. EF-1α was used as the internal reference gene (41). All primer sources are listed in Table 3.
The RNA extraction, cDNA production, and qPCR methods were based on a previous study (34). In brief, total RNA was extracted from the intestine of golden pompano using a HiPure Universal RNA Mini kit (Magen Biotech Co., Ltd., Guangzhou, China) according to the manufacturer’s instructions. The integrity of RNA was assessed using 1% agarose gel electrophoresis, and the concentration was assayed using a Nanodrop 2000 (Thermo Fisher Scientific, MA, USA). We used a PrimeScript™ RT kit (Accurate Biotechnology Co., Ltd., Hunan, China) for reverse-transcription. The gDNA Eraser in the kit eliminated adverse effects. The SYBR® Green Premix Pro Taq HS qPCR Kit (Accurate Biotechnology Co., Ltd., Hunan, China) was used to perform qPCR. qPCR parameters were used as described previously (35, 43). To minimize the impact of incidental factors, each gene was repeated four times and three of the results were selected to calculate target gene mRNA levels using the 2-ΔΔCT method (45).
Streptococcus agalactiae challenge
To complement the impact of cysteine on golden pompano immunity, we conducted an S. agalactiae challenge experiment following the feeding trial. The S. agalactiae concentration of 2.0 × 107 CFU/fish was the LD50 of golden pompano challenged by S. agalactiae for 120 h, as determined previously by our lab (34). After sample collection, we stochastically selected 20 fish per cage with similar size and healthy condition, injected 200 μL of the bacterial suspension at a concentration of 2.0 × 107 CFU/fish into the peritoneal cavity of each fish using a sterile syringe and returned the fish to their respective cages for continued feeding for 120 h. The remaining number of fish in each group was recorded every 12 h, and dead fish were removed. At the end of the challenge trial, all fish were rendered harmless using the alcohol. The same hydrological conditions as those used in the feeding experiment were maintained throughout the challenge. After the challenge experiment, we imported the data into GraphPad Prism 8 software and used the Kaplan-Meier algorithm to calculate the survival curves. A log-rank test was used to compare variances among groups.
Statistical analyses
Gene expression and serum parameters were analyzed by one-way analysis of variance (ANOVA) using GraphPad Prism 8 and Origin Pro 2021 (OriginLab Corporation, Northampton, MA, USA), respectively. The results of the analysis are presented as mean ± standard deviation (mean ± SD). Tukey’s test was used for multiple comparisons when there was a significant difference (P< 0.05).
Results
Growth performance
As shown in Table 4, the levels of SR, FI, and CF in C0 fish were not significantly different from those in the other groups (P > 0.05). However, growth performance was proportional to cysteine content in the diets. Cysteine markedly increased the levels of FBW, WGR, and SGR in C2, C3, and C4 fish compared to C0 (P< 0.05). In addition, dietary cysteine addition of 0.6% was responsible for a significant downregulation of FCR, HSI, and VSI in fish compared with the C0 group (P< 0.05). Under these experimental conditions, the optimal cysteine supplementation level in golden pompano diet was 0.91%, according to the polynomial regression results of SGR (Figure 1).
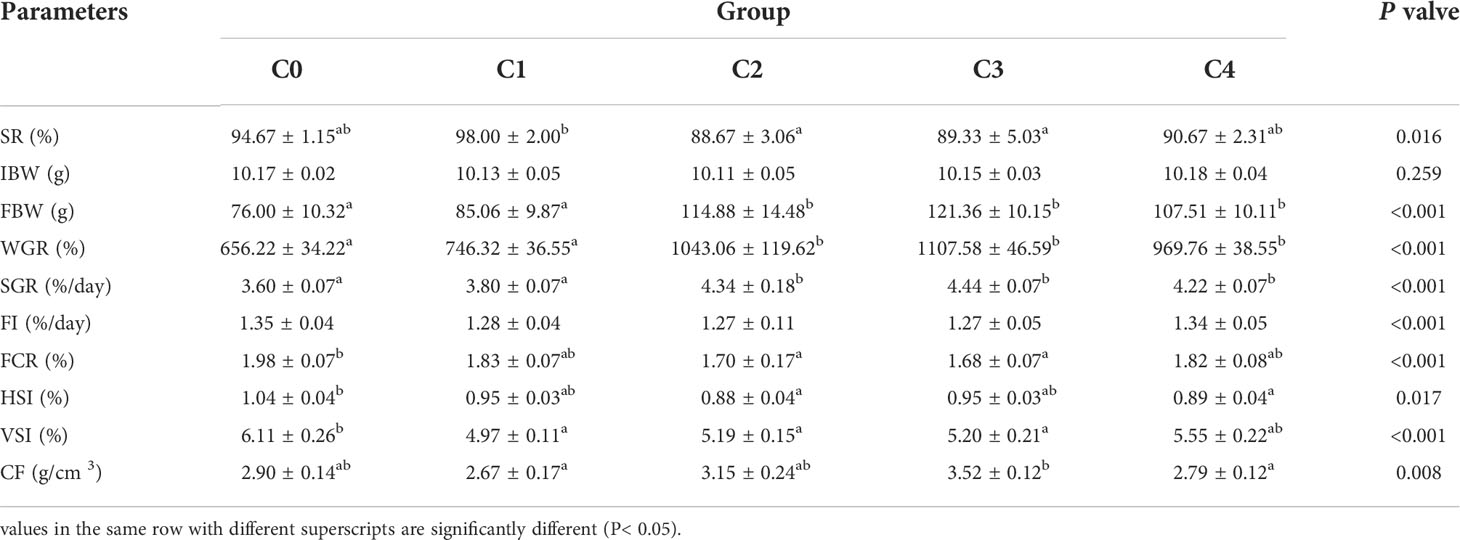
Table 4 Growth performance of T. ovatus fed diets with different dose cysteine supplementation after 8 weeks.
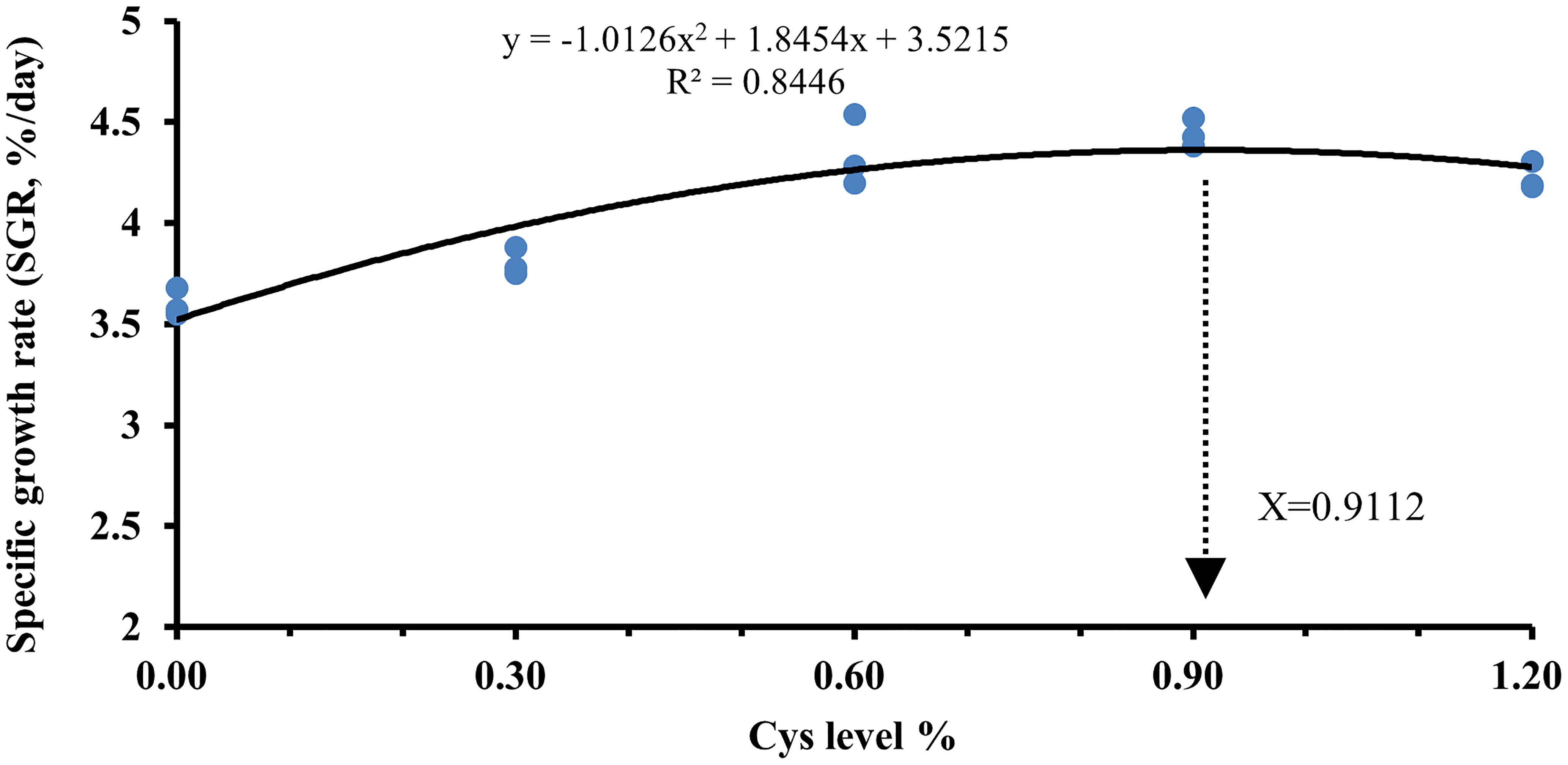
Figure 1 Estimation of the optimal dietary cysteine supplementation level for T. ovatus by means of polynomial regression analysis using the SGR.
Serum antioxidant capacity and non-specific immune parameters
To explore the resistance of fish supplied with exogenous cysteine to oxidative stress, we determined the activity of several antioxidant enzymes in the serum of golden pompano (Figures 2A–D). The activity of T-AOC in serum was remarkably increased in C1, C3, and C4 fish compared to C0 fish (P< 0.05), but there was no remarkable difference between C2 and C0 fish (P >0.05, Figure 2A). With higher dietary cysteine levels, CAT activity was significantly higher in all treatment fish than in the C0 fish (P< 0.05, Figure 2B). Exogenous supplementation with 0.6-1.2% cysteine remarkably upregulated the activities of GSH-PX compared to the C0 fish (P< 0.05, Figure 2D). Even though 0.3%–0.6% dietary cysteine supplementation decreased the activity of SOD, with higher cysteine supplementation of 0.9%–1.2%, the SOD activity of C3 and C4 fish was considerably higher than in the C0 group (P< 0.05, Figure 2C). This suggests that the antioxidant effect can only be achieved at a specific dietary cysteine level. This phenomenon was also observed for the levels of MDA and ROS (Figures 2E, F). The MDA and ROS levels in the serum of golden pompano decreased considerably when the diet was supplemented with 0.9%–1.2% cysteine, and they were markedly lower than in the C0 group.
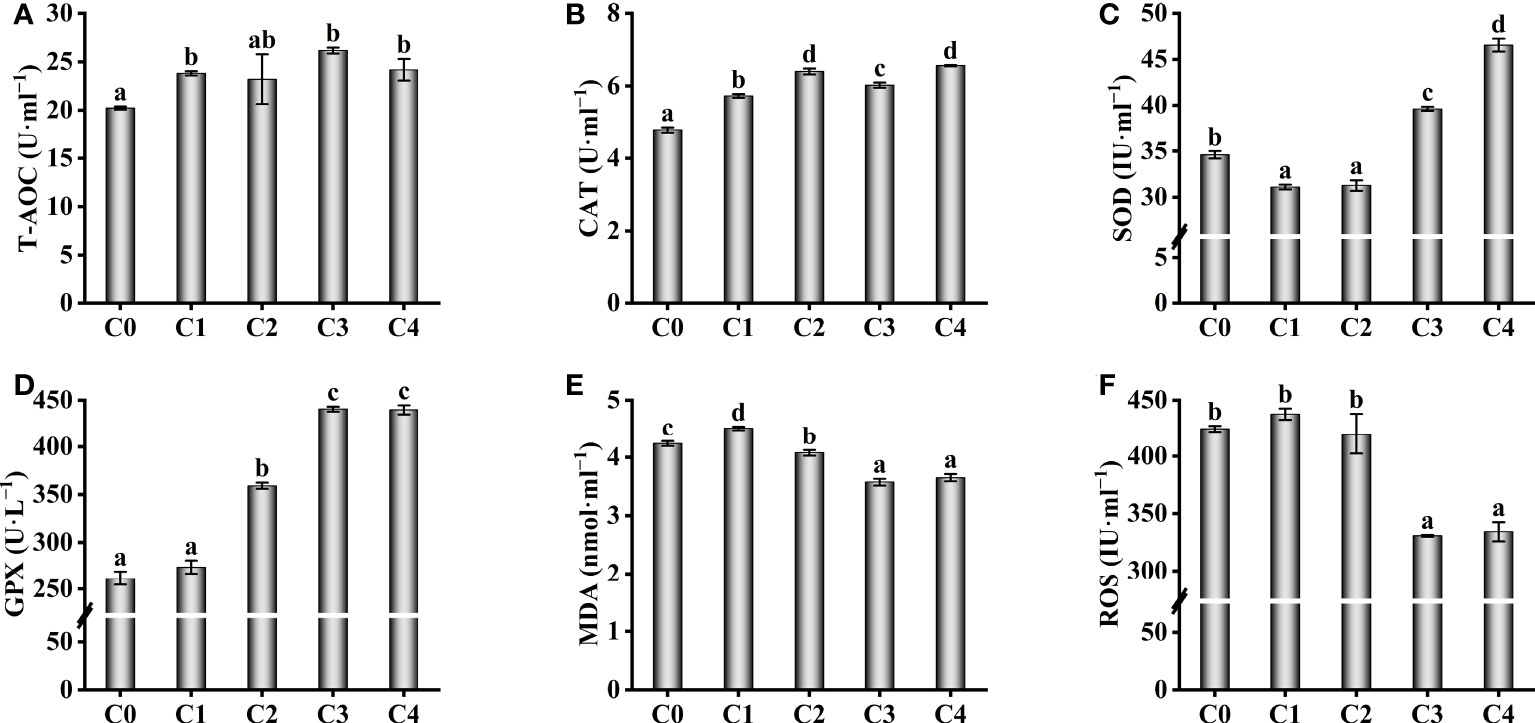
Figure 2 Effect of antioxidant capability such as T-AOC (A), CAT (B), SOD (C), GSH-PX (D), MDA (E) and ROS (F) in the serum of T. ovatus fed diets with different dose cysteine supplementation after 8 weeks. Mean values (n = 9) within values in the picture above with different superscripts are significantly different (P< 0.05).
The serum immunological parameters were also affected by exogenous cysteine (Figure 3). Exogenous supplementation with 0.3% cysteine did not increase the immunoglobulin (IgM, IgA, and IgG) content and LZM activity in serum of golden pompano, compared to the C0 group (P > 0.05, Figures 3A–D); however, at 0.6%–1.2% cysteine, the immunoglobulin content and LZM activity were markedly higher than those in the C0 group (P< 0.05). The levels of complement 3 and complement 4, important parameters of serum immunology increased significantly with increasing cysteine supplementation (P< 0.05), reaching a maximum at 0.9%–1.2% cysteine supplementation (Figures 3E, F).
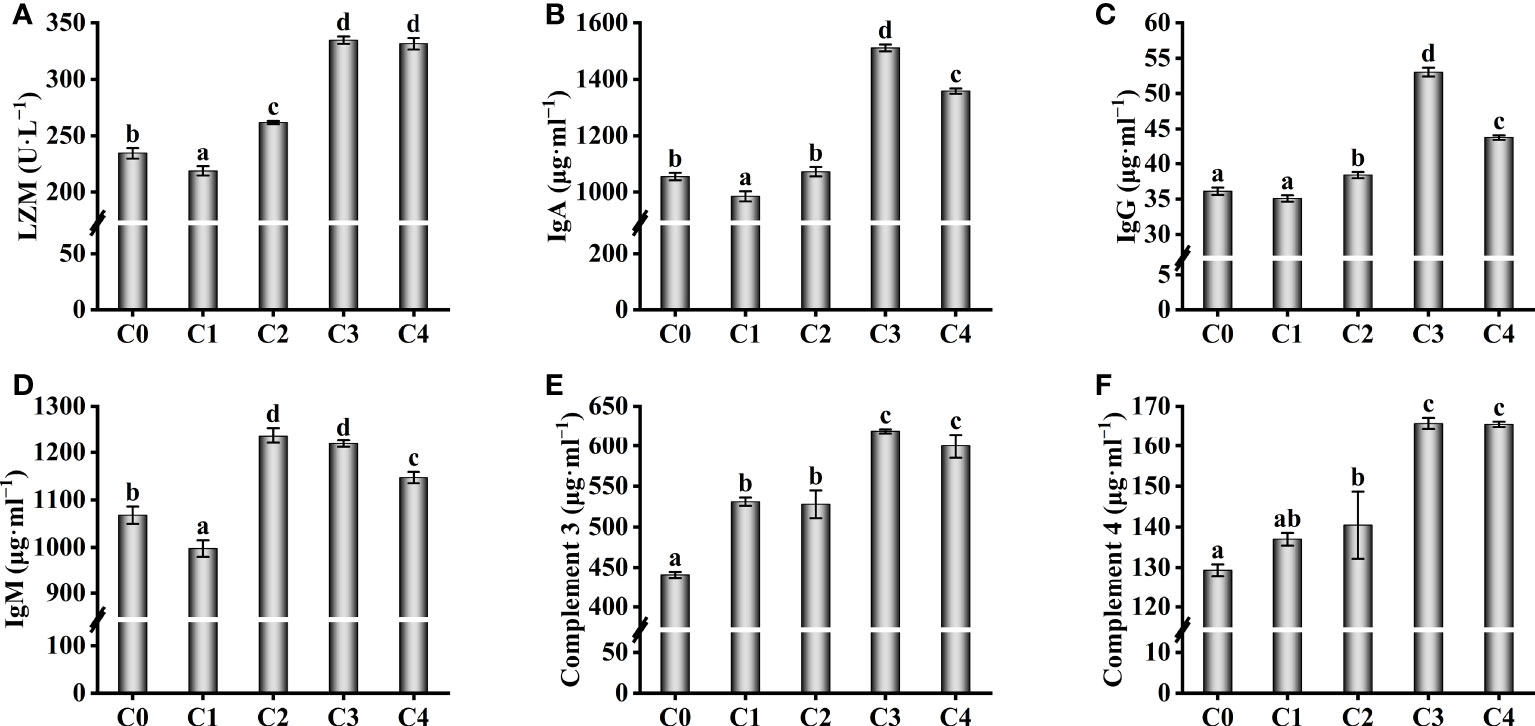
Figure 3 Effect of immunological parameters such as LZM (A), IgA (B), IgG (C), IgM (D), C3 (E), C4 (F) in the serum of T. ovatus fed diets with different dose cysteine supplementation after 8 weeks. Mean values (n = 9) within values in the picture above with different superscripts are significantly different (P< 0.05).
Midgut histological observation
The histological parameters of the golden pompano intestine are shown in Figure 4. With higher cysteine supplementation, intestinal villus length and muscular thickness increased markedly (Figure 4F). Villus length was significantly higher in C2, C3, and C4 fish than in C0 and C1 fish (P< 0.05). In addition, the statistical results indicated that exogenous supplementation with 0.3% and 1.2% cysteine substantially increased muscular thickness, compared to C0 fish (P< 0.05, Figure 4G). Nevertheless, cysteine supplementation decreased the number of goblet cells per intestinal villus (Figure 4H). The abundance of goblet cells was considerably lower in C3 and C4 fish compared to that in the controls (P< 0.05).
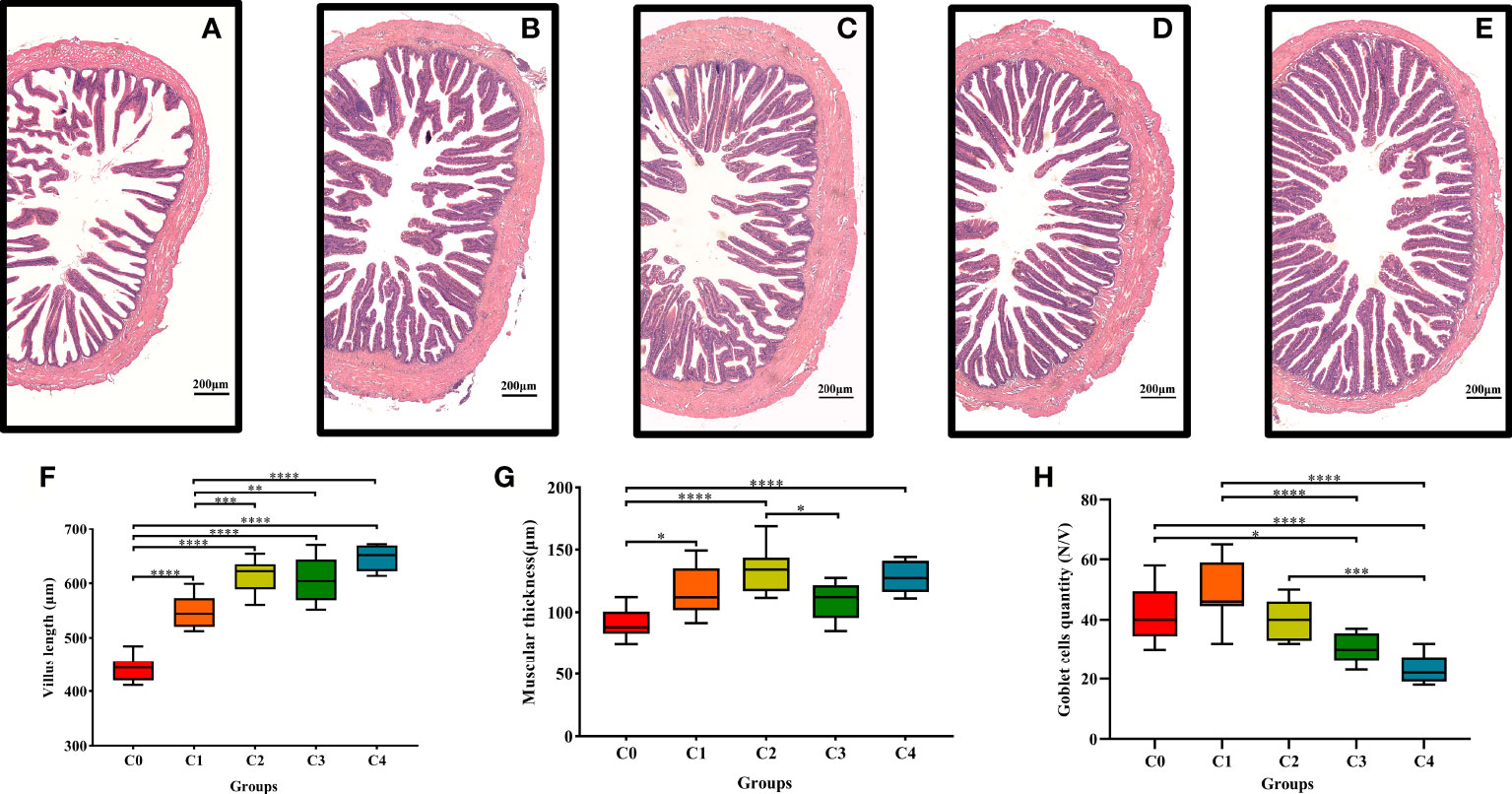
Figure 4 Effects of dietary cysteine on mid-gut morphology of T. ovatus. (A) 0% cysteine; (B): 0.40% cysteine; (C): 0.80% cysteine; (D): 1.20% cysteine; (E): 1.60% cysteine. Scale bar: 200 μm. The villus length (F), muscular thickness (G), and goblet cells quantity (H) of mid-gut in T. ovatus. data are presented as mean ± SD (n = 9). Asterisks *, **, ***, and **** indicate statistically significant difference between treated group and control group at P< 0.05, P< 0.01 P< 0.001, and P< 0.0001, respectively.
16S rDNA high-throughput sequencing of the intestinal flora
We obtained 678,797 clean reads and 2,807 OTUs by high-throughput sequencing of the intestinal microbes of the golden pompano. A Venn diagram was produced to visualize that 15 OTUs were identical in all groups, and dietary cysteine increased the amount of specific OTUs in the gut flora (Figure 5A). Rarefaction curves (Figure 5B), OTU rank-abundance curves (Figure 5C), and species accumulation curves (Figure 5D) converged to saturation, allowing assessment of sequencing depth, species evenness, and species richness. The dominant phyla included Proteobacteria, Firmicutes, and Bacteroidetes (Figure 5E). Firmicutes showed a decreasing trend (0%–0.9%) and then increased (0.9%–1.2%) with increasing dietary cysteine content. At the genus level, Ralstonia, Saccharibacterianorank, and [Ruminococcus] gnavus were dominant (Figure 5F). A sample clustering tree (Figure 5G) and abundance similarity clustering (Figure 5H) of intestinal flora among the groups showed that cysteine supplementation enriched the composition of the intestinal flora of golden pompano. With increasing dietary cysteine content, the abundance of the intestinal flora of golden pompano gradually increased.
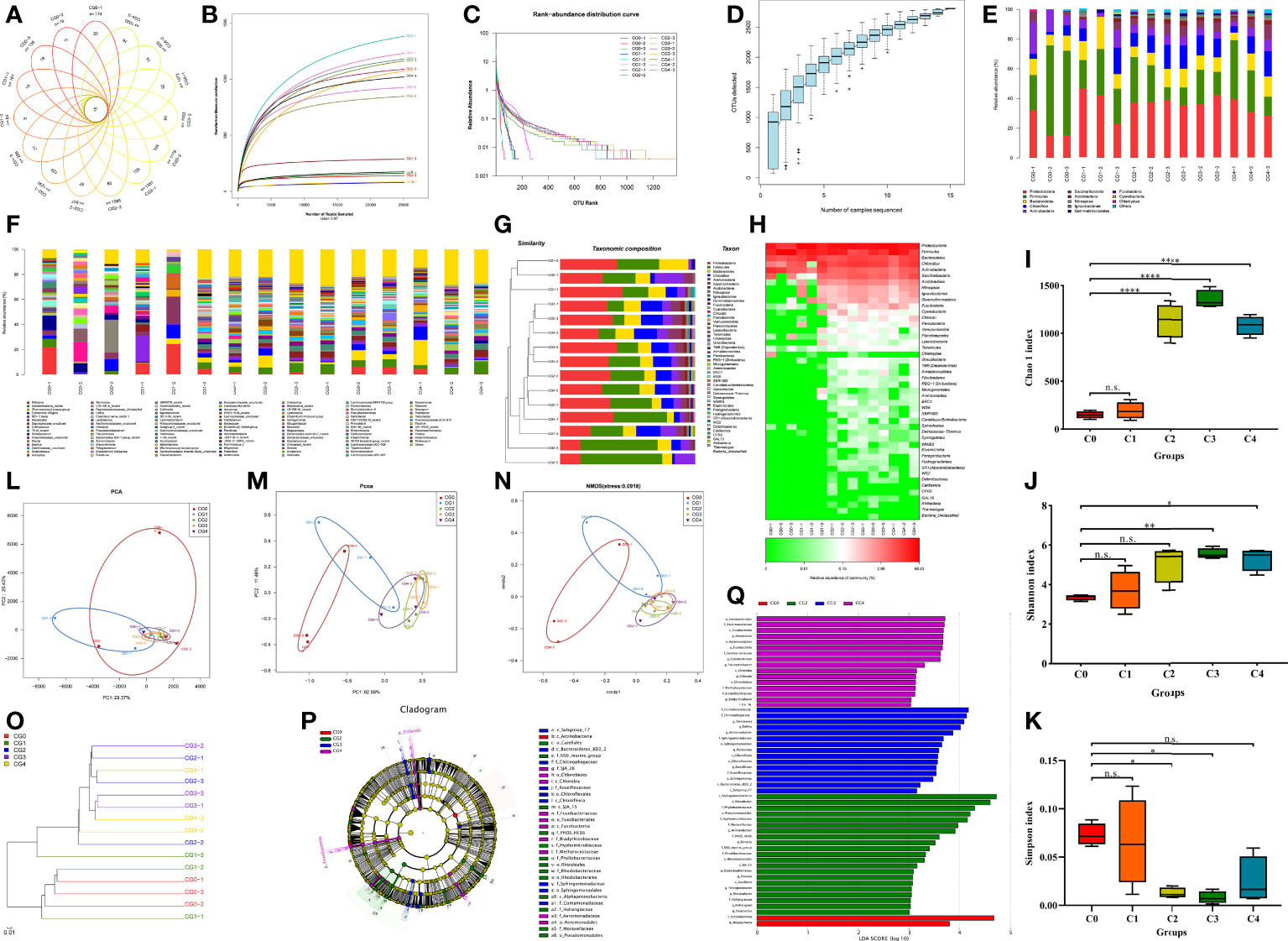
Figure 5 Cysteine supplementation regulated gut microbiota composition and abundance. Profile of the intestinal microbiota (n = 3). The basic structure of the gut microbiota was determined by Venn diagram (A), Rarefaction curves (B), Rank-Abundance curves (C), species accumulation curves (D), relative abundance histograms of phylum (E) and genus (F), Mcrobial community barplot with cluster tree (G), and heat map comparison (H) were depicted. The diversity and richness of the communities were compared by alpha diversity indices, including Chao 1 index (I), Shannon index (J), and Simpson index (K). The community diversity and richness were compared by Principal Component Analysis (L), UniFrac-based principal co-ordinates analysis (M), and UniFrac-based non-metric multi-dimensional scaling analysis (N) were performed for β-diversity analysis. Communities or species with significantly different effects on sample delineation were identified by UniFrac-based cluster tree (O), Evolutionary branching plots (P), and Linear discriminant analysis (Q). "n.s."indicate No significant difference. Asterisks *, **, and **** indicate statistically significant difference between treated group and control group at P < 0.05, P < 0.01, and P < 0.0001, respectively.
To further investigate the effect of cysteine on the abundance and diversity of intestinal microbial populations, we used alpha diversity index analysis, which showed that supplementation with 0.6%–1.2% cysteine significantly increased the Chao1 index (Figure 5I) and reduced the Simpson index (Figure 5K), compared to the controls, except for the C0 and C4 fish in which the Simpson index did not differ significantly. Similarly, the Shannon index showed (Figure 5J) that the exogenous supplementation with 0.9%–1.2% cysteine increased the abundance and diversity of the flora.
We used PCA, UniFrac-based PCOA, and UniFrac-based NMDS for comparative analysis of the similarity between groups and samples (β diversity analysis). Exogenous supplementation with 0.6%–1.2% cysteine caused a similar gut microbial composition across experimental fish, which differed from that of the C0 fish (Figures 5L, N). The UniFrac-based PCOA weighted results showed that differences in the PC1 axis and PC2 axis explained 62.56% and 11.46% of the variation, respectively (Figure 5M). UniFrac-based cluster tree analysis using the unweighted pair group method with arithmetic mean was used to visualize the evolutionary similarities and differences of microorganisms in different samples, and the results showed that the gut flora of C0 and C1 fish were more similar and clustered in one branch. In contrast, C2, C3, and C4 fish were similar and clustered on a different branch (Figure 5O).
To identify communities or species that had a significant differential effect on sample delineation, we used the non-parametric factorial Kruskal-Wallis sum-rank test method to detect characteristics with significant abundance differences and to identify taxa that differed significantly in abundance. Linear discriminant analysis was applied using LEfSe software to estimate the magnitude of the effect of abundance on the different effects for each component (species). Evolutionary branching plots (Figure 5P) and linear discriminant analysis (Figure 5Q) showed that only the class Actinobacteria and the genus Megasphaera played a significant role in C0 fish. In contrast, C2, C3, and C4 fish had more diverse gut flora. Six phyla (Proteobacteria, Bacteroidetes, Actinobacteria, Firmicutes, Chloroflexi, and Ignavibacteriae), nine orders, eleven families, and eight genera were present more frequently in C2 fish. Four phyla (Proteobacteria, Acidobacteria, Chloroflexi, and Bacteroidetes), six orders, four families, and six genera were more abundant in the C3 fish. Four phyla (Fusobacteria, Proteobacteria, Firmicutes, and Chlorobi), five orders, five phyla, six families, and four genera were more abundant in C4 fish.
Antioxidant enzyme expression in the intestine
We examined the expression of antioxidant enzyme genes in the intestine of golden pompano to examine the effects of exogenous cysteine on antioxidant stress capacity (Figure 6A). Compared to the control group, CAT and GSH-PX expression were substantially upregulated in C2, C3, and C4 fish (P< 0.05), and the GSH-PX expression was highest in the C3 group. The CAT expression levels between the C3 and C4 groups were not markedly different (P > 0.05), with both significantly higher than the other groups (P< 0.05). The effect of exogenous cysteine on SOD expression was considered significant. The expression of these three genes was upregulated owing to exogenous cysteine supplementation, compared to that in the control group, and it was considerably higher than that in the C0 group (P< 0.05). In addition, dietary cysteine reduced intestinal Keap-1 mRNA levels and led to upregulation of HO-1 and Nrf2 (Figure 6B).
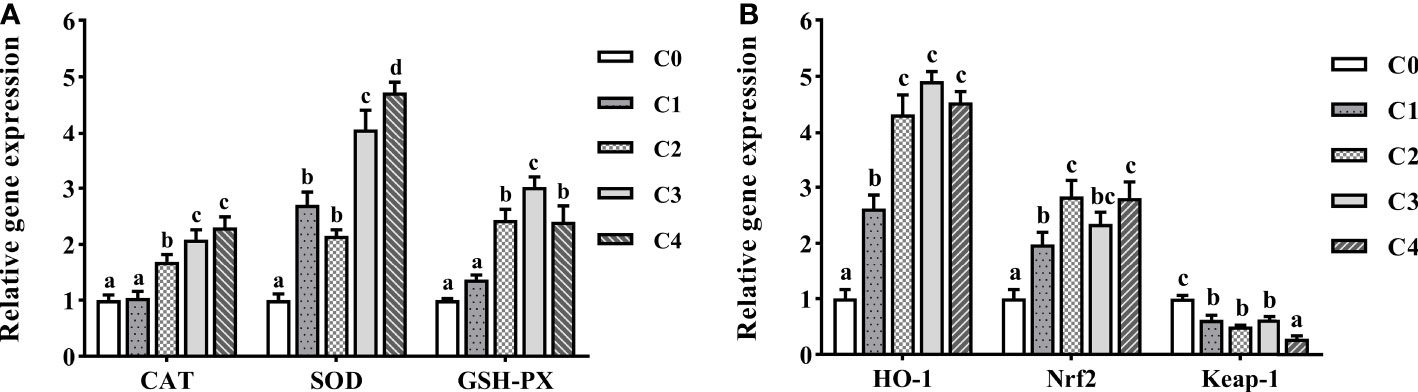
Figure 6 The expression profiles of antioxidant genes (A) and signaling pathway (B) in the intestine of T. ovatus fed diets with different dose cysteine supplementation after 8 weeks. Mean values (n = 9) within values in the picture above with different superscripts are significantly different (P< 0.05).
Intestine immunity-related gene expression analysis
To gain further information on the effect of exogenous cysteine on intestine immunity of golden pompano, we selected the essential genes of inflammatory response-related cytokines and the NF-κB pathway for qPCR (Figure 7). Exogenous supplementation with cysteine suppressed the expression of TNF-α, IL-1β, IL-8 (Figure 7A), NF-κB, and IKK (Figure 7B) in the intestine of golden pompano. Dietary cysteine supplementation of 0.6%–1.2% led to lower expression levels of TNF-α, IL-1β, NF-κB, IKK, and IL-8, compared to C0 fish (P< 0.05). Meanwhile, expression of IκB (Figure 7B) and IL-10 (Figure 7A) was significantly upregulated by exogenous cysteine, compared with that in the controls (P< 0.05). This demonstrated that cysteine could promote intestine immunity in golden pompano.
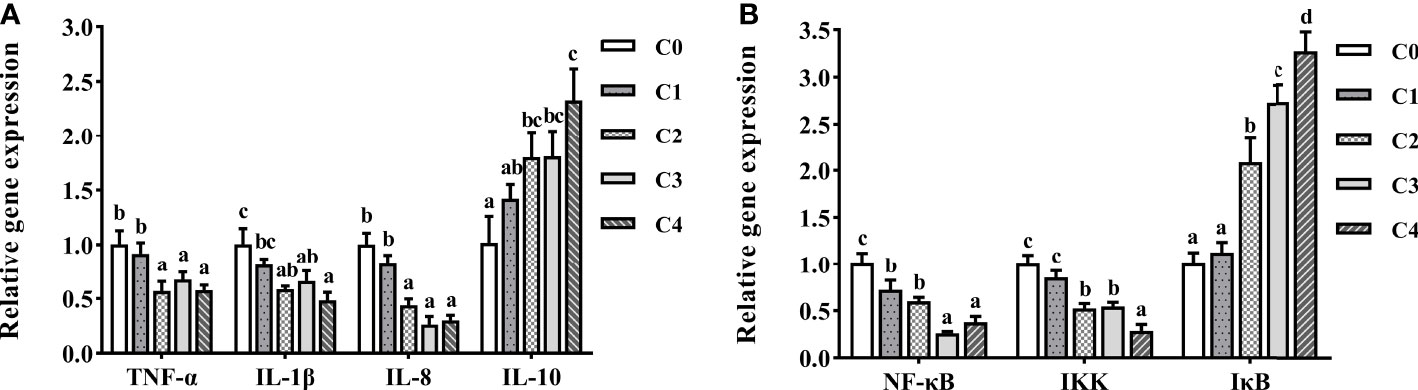
Figure 7 The expression profiles of inflammatory genes (A) and signaling pathway (B) in the intestine of T. ovatus fed diets with different dose cysteine supplementation after 8 weeks. Mean values (n = 9) within values in the picture above with different superscripts are significantly different (P< 0.05).
Streptococcus agalactiae challenge
The survival rates of juvenile golden pompano after S. agalactiae challenge is presented in Figure 8. The survival rates of C0 (0), C1 (0.3%), C2 (0.6%), C3 (0.9%), and C4 (1.2%) fish after the challenge for 120 h were 41.67%, 50.00%, 63.33%, 73.33%, and 63.33%, respectively. The survival of juvenile golden pompano gradually increased with an increase in dietary cysteine supplementation from 0% to 0.9%. Exogenous supplementation with 0.6%–1.2% cysteine markedly improved the resistance of golden pompano to S. agalactiae, compared to the C0 group (P< 0.05). Thus, appropriate cysteine supplementation can greatly promote immunity in golden pompano.
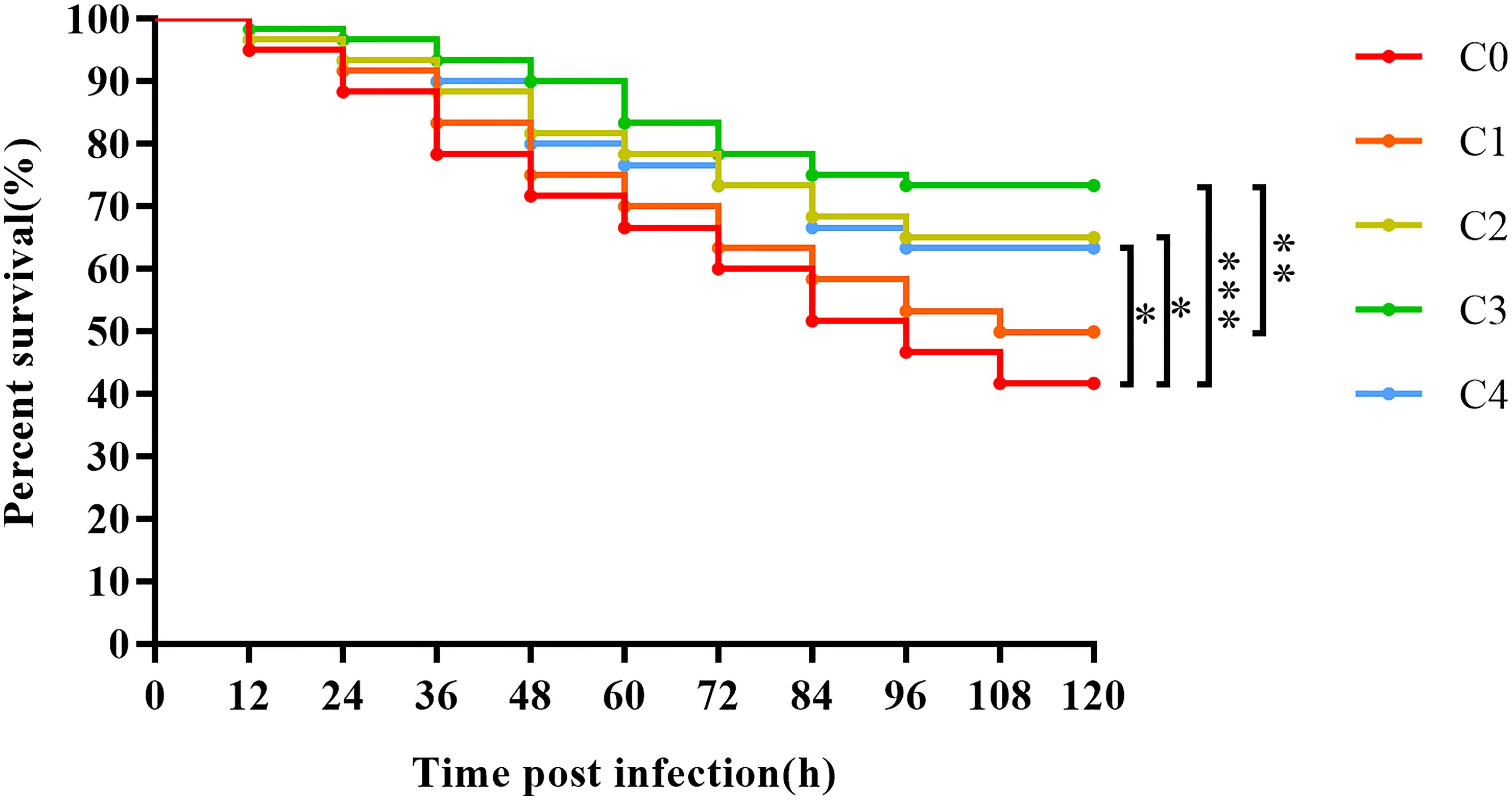
Figure 8 The Kaplan-Meier survival analysis of T. ovatus after S. agalactiae infection. Asterisks *, **, and *** indicate statistically significant difference between the two groups at P < 0.05, P < 0.01, and P < 0.001, respectively.
Discussion
Supplementation of low-fishmeal diets with cysteine promotes growth of juvenile golden pompano
In the present study, supplementation with 0.6%–1.2% cysteine remarkably increased the FBW, WGR, and SGR levels in golden pompano. Moreover, adding 0.6% cysteine to the diet decreased the FCR, HSI, and VSI significantly, compared to the C0 group. These indices indicated that dietary supplementation with cysteine greatly enhanced the growth performance of golden pompano. Similar findings were made in a previous study on Psetta maxima, which showed that a moderate amount of dietary cysteine (0.3%) enhanced the SGR levels and the mean final weight of P. maxima and improved its growth performance (20). Oxidative stress and immune capacity reduction caused by low fishmeal content are the main reasons for reduced growth performance (8, 9, 46). In the present study, there were many reasons that cysteine supplementation improved the growth performance of golden pompano in low fishmeal conditions, such as the increased abundance and diversity of gut microbes (31), increased antioxidant capacity (47, 48), and improved immune ability (27). Our results showed that dietary supplementation with 0.6%–1.2% cysteine could increase the activity of serum antioxidant enzymes (T-AOC, CAT, and GSH-PX) and decrease the serum MDA and ROS levels in juvenile golden pompano. Meanwhile, non-specific serum immune parameters were improved, and the levels of immunoglobulins (IgM, IgA, and IgG), complement 3, complement 4, and LZM activity were increased, which may help the fish cope with oxidative stress and immunity reduction caused by low-fishmeal diets, thus improving growth performance. Our findings are similar to those found in Seriola lalandi (47) and Scophthalmus maximus L (48), where cysteine enhanced growth performance by improving resistance to oxidative stress and intestine immunity.
Supplementation of low-fishmeal diets with cysteine improves the intestinal physical barrier in juvenile golden pompano
The intestinal physical barrier is a critical component of intestine immunity with the function of taking in nutrients and defending against harmful substances (49, 50). Low-fishmeal diets damage the intestinal physical barrier; for example, replacing fishmeal with soybean meal reduces the villi length of Epinephelus coioides (12), Litopenaeus vannamei (49), and Penaeus monodon (50) and damages the intestinal mucosal folds, leading to intestinal inflammation. Cysteine effectively protects the physical barrier of the intestine. For instance, cysteine protects the intestine of broiler chicks by increasing the length of the intestinal villi (51). In addition, cysteine has been found to improve intestinal integrity and permeability and reduce the intestinal inflammatory response in pigs (25, 27). Similarly, our results showed that exogenous cysteine supplementation at 0.6% and 1.2% greatly improved intestinal villus length and muscular thickness, thus effectively alleviating the weakening of the intestinal physical barrier associated with low-fishmeal diets. Alterations in the intestinal physical barrier typically accompany changes in the abundance of intestinal flora (52, 53) and expression of intestinal immune-related genes (54). Therefore, we propose that the protective effect of cysteine on the intestinal physical barrier of golden pompano may be correlated with increased abundance of intestinal flora and high expression of intestinal antioxidant stress- and immune-related genes.
Supplementation of low-fishmeal diets with cysteine improves the diversity of intestinal flora in juvenile golden pompano
Maintaining the diversity and abundance of intestinal flora is essential for the intestinal health of fish, and disturbances to the intestinal flora tend to damage the physical barrier of the intestine and elicit inflammatory responses (53, 55). In our study, alpha and beta diversity analysis of the intestinal flora showed that supplementation of the diet with cysteine regulated the abundance and diversity and improved the structure of intestinal flora in golden pompano. Alpha diversity results demonstrated that exogenous supplementation with 0.6%–1.2% cysteine remarkably increased the Chao 1 index and decreased the Simpson index, thus the diversity of the intestinal flora was markedly improved, and similar results were found regarding the Shannon index (C2 and C3 fish). At the phylum level, the dominant taxa included Proteobacteria, Firmicutes, Bacteroidetes, Fusobacteria, Chloroflexi, and Actinobacteria. Appropriate addition of cysteine to the diet increased the relative abundance of Proteobacteria and Bacteroidetes and decreased the relative abundance of Firmicutes. Bacteroidetes are essential players in polysaccharide (56–58) and cholesterol metabolism (59), and it is possible that altering the abundance of Bacteroidetes is one of the ways cysteine regulates nutrient metabolism. At the genus level, Ralstonia, Saccharibacterianorank, and [Ruminococcus] gnavus group were predominant in the intestinal tract of golden pompano. Adequate cysteine addition can thus increase the relative abundance of the [Ruminococcus] gnavus group. Similarly, previous studies have shown that dietary cysteine has a positive effect on intestinal flora richness and diversity in gestating sows (31) and S. maximus L (48).
Supplementation of low-fishmeal diets with cysteine enhances the expression of intestinal antioxidant and immune-related genes in juvenile golden pompano
The protective effect of cysteine on intestinal integrity may be related to signaling pathways associated with antioxidant and inflammatory responses. Numerous studies confirmed that excessive substitution of fishmeal in diets elicits oxidative stress in the fish intestine owing to excessive anti-nutrients, increasing ROS levels, and leading to structural damage in the intestine (8, 9). For instance, the addition of plant proteins to diets causes oxidative stress and structural damage in the intestine of S. maximus L (9)., Monopterus albus (11), and Amurenser schrenckii (60). ROS levels are predominantly regulated by the Nrf2/Keap-1 signaling pathway (43, 61). Nrf2 can reduce ROS levels and increase the expression of antioxidant enzyme genes in golden pompano by enabling the high expression of HO-1 (42). Our results indicate that cysteine supplementation activated the Nrf2/Keap-1 signaling pathway in the intestine of golden pompano. Downregulation of Keap-1 mRNA levels led to the upregulation of HO-1 and Nrf2 mRNA levels, increasing the mRNA levels of intestinal antioxidant enzymes (CAT, SOD, and GSH-PX) and the corresponding enzyme activities in serum.
Oxidative stress not only damages tissue integrity but may also elicit inflammatory responses and reduce the immune capacity of the body (41). Inflammatory responses are regulated by a combination of anti-inflammatory cytokines (IL-10, TGF-β, etc.) (30, 62) and pro-inflammatory cytokines (IL-1β, IL-8, TNF-α, etc.) (30, 48, 63), which in turn are typically modulated through the NF-κB/IκB/IKK signaling pathway (64, 65). A study showed that cysteine inhibited NF-κB expression and downregulated pro-inflammatory cytokines (IL-6, TNF-α, and IL-8) in the intestine of piglets, which improved the physical barrier of the intestine and promoted intestinal health (25). Similarly, we found that NF-κB, IKK (Figure 7B), IL-1β, IL-8, and TNF-α (Figure 7A) in the intestine of golden pompano were negatively correlated with dietary cysteine content, whereas the expression levels of IκB and IL-10 showed contrasting patterns. These results suggest that dietary supplementation with 0.6%–1.2% cysteine (C2, C3, and C4 fish) decreases the expression level of pro-inflammatory cytokines by inhibiting the NF-κB signaling pathway. Thus, the inflammatory response was suppressed, and the intestine immunity of golden pompano was improved.
Supplementation of low-fishmeal diets with cysteine enhances the resilience of juvenile golden pompano to Streptococcus agalactiae
Infectious diseases caused by S. agalactiae have frequently erupted in recent years in large-scale aquacultures of marine fish because of high culture densities (34). Therefore, we also examined the protective effect of cysteine during an S. agalactiae challenge in golden pompano. Survival curves showed that cysteine had a positive effect on the survival rate of golden pompano. The survival rate was remarkably higher in C2, C3, and C4 (0.6%–1.2% cysteine) than in C0 fish. Bacterial challenge can reduce the diversity of intestinal flora in fish (66, 67), whereas our results showed that cysteine substantially increased the diversity of the intestinal flora. Therefore, considering the high agreement between the survival rate of the present challenge test and intestinal immune parameters, such as histological results of the intestine, results of microbial diversity analysis, and intestinal immune gene expression, we infer that cysteine may have enhanced the resistance of golden pompano to S. agalactiae by improving its intestine immunity.
Conclusions
Overall, a moderate level of cysteine (0.6%–1.2%) improved the growth performance of golden pompano, and the optimum dietary cysteine supplementation level for juvenile golden pompano was 0.91%, based on polynomial regression analysis of SGR. Moderate dietary cysteine also improved the abundance and diversity of intestinal flora and enhanced the structural integrity of the intestine to maintain the stability of the intestinal physical barrier. Furthermore, dietary cysteine activated the Nrf2/Keap1/HO-1 signaling pathway and inhibited NF-κB signaling, increased intestinal antioxidant enzyme genes (CAT, SOD, and GSH-PX) and anti-inflammatory cytokine mRNA levels, increased serum antioxidant enzyme activity, and substantially improved intestine immunity, resulting in higher survival rates of golden pompano exposed to S. agalactiae. These findings provide new insights into the development and use of cysteine as a dietary supplement.
Data availability statement
The data presented in the study are deposited in the NCBI repository, accession number: PRJNA889580. The data can be found below: https://www.ncbi.nlm.nih.gov/search/all/?term=PRJNA889580.
Ethics statement
The animal study was reviewed and approved by Animal Care and Use Committee of the South China Sea Fisheries Research Institute of the Chinese Academy of Fishery Sciences.
Author contributions
D-CZ designed the experiments and wrote the manuscript. J-XL conducted the experiments and wrote the manuscript. K-CZ: Methodology, Software. H-YG: Data curation, Methodology. B-SL: Supervision, Software. NZ: Visualization, Investigation. All authors contributed to the article and approved the submitted version.
Funding
This research was financially supported by Hainan Yazhou Bay Seed Lab (B21HJ0702), China Agriculture Research System of MOF and MARA (CARS-47), Central Public-Interest Scientific Institution Basal Research Fund of South China Sea Fisheries Research Institute CAFS (2021SD12), Central Public-interest Scientific Institution Basal Research Fund, CAFS (NO.2020TD29), Key Projects of Joint Fund for Regional Innovation and Development of NSFC (U20A2064), National Marine Genetic Resource Center, Guangdong Provincial Special Fund for Modern Agriculture Industry Technology Innovation Teams (2019KJ143).
Conflict of interest
The authors declare that the research was conducted in the absence of any commercial or financial relationships that could be construed as a potential conflict of interest.
Publisher’s note
All claims expressed in this article are solely those of the authors and do not necessarily represent those of their affiliated organizations, or those of the publisher, the editors and the reviewers. Any product that may be evaluated in this article, or claim that may be made by its manufacturer, is not guaranteed or endorsed by the publisher.
Abbreviations
T-AOC, total antioxidant capacity; CAT, catalase; GSH-PX, glutathione peroxidase; SOD, superoxide dismutase; MDA, malondialdehyde; ROS, reactive oxygen species; LZM, lysozyme; IgA, immunoglobulins A; IgG, immunoglobulins G; IgM, immunoglobulins M; Nrf2, nf-e2-related nuclear factor 2; Keap-1, Kelch-like ECH-associated protein-1; HO-1, heme oxygenase-1; NF-κB, nuclear factor kappa B; IκB, inhibitor protein-κB; IKK, IκB kinase; TNF-α, tumor necrosis factor-α; IL-1β, interleukin 1β; IL-8, interleukin 8; IL-10, interleukin 10; WGR, weight gain rate; SGR, specific growth rate; FCR, feed conversion ratio; CF, condition factor; SR, survival rate; HSI, hepatosomatic index; VSI, viscerasomatic index; FI, feed intake; IBW, initial body weight; FBW, final body weight; FBL, final body length; DDI, dry diet intake; NOD, number of days; VW, viscera weight; LW, liver weight; NWG, net weight gain; FFN, final fish numbers.
References
1. Guimarães IG, Pezzato LE, Barros MM. Amino acid availability and protein digestibility of several protein sources for Nile tilapia, oreochromis niloticus. Aquac Nutr (2008) 14(5):396–404. doi: 10.1111/j.1365-2095.2007.00540.x
2. Walsh S, Davis R, Weldon A, Reis J, Stites W, Rhodes M, et al. Effects of fishmeal replacement, attractants, and taurine removal on juvenile and sub-adult red snapper (Lutjanus campechanus). Aquaculture (2021) 544:737054. doi: 10.1016/j.aquaculture.2021.737054
3. Kaiser F, Harbach H, Schulz C. Rapeseed proteins as fishmeal alternatives: A review. Rev Aquac (2022) 14(4):1887–1911. doi: 10.1111/raq.12678
4. Regost C, Arzel J, Kaushik SJ. Partial or total replacement of fish meal by corn gluten meal in diet for turbot (Psetta maxima). Aquaculture (1999) 180(1):99–117. doi: 10.1016/s0044-8486(99)00026-5
5. Rapatsa M, Moyo N. A review and meta-analysis of the effects of replacing fishmeal with insect meals on growth of tilapias and sharptooth catfish. Aquac Nutr (2022) 2022:9367587. doi: 10.1155/2022/9367587
6. Subhadra B, Lochmann R, Rawles S, Chen R. Effect of fish-meal replacement with poultry by-product meal on the growth, tissue composition and hematological parameters of largemouth bass (Micropterus salmoides) fed diets containing different lipids. Aquaculture (2006) 260(1):221–31. doi: 10.1016/j.aquaculture.2006.06.029
7. Chou RL, Her BY, Su MS, Hwang G, Wu YH, Chen HY. Substituting fish meal with soybean meal in diets of juvenile cobia Rachycentron canadum. Aquaculture (2004)229(1)325–333. doi: 10.1016/s0044-8486(03)00395-8
8. Zhang CX, Rahimnejad S, Wang YR, Lu KL, Song K, Wang L, et al. Substituting fish meal with soybean meal in diets for Japanese seabass (Lateolabrax japonicus): Effects on growth, digestive enzymes activity, gut histology, and expression of gut inflammatory and transporter genes. Aquaculture (2018) 483:173–82. doi: 10.1016/j.aquaculture.2017.10.029
9. Tan C, Zhou HH, Wang X, Mai KS, He G. Resveratrol attenuates oxidative stress and inflammatory response in turbot fed with soybean meal based diet. Fish Shellfish Immunol (2019) 91:130–5. doi: 10.1016/j.fsi.2019.05.030
10. Yang H, Bian YH, Huang LL, Lan Q, Ma LZ, Li XQ, et al. Effects of replacing fish meal with fermented soybean meal on the growth performance, intestinal microbiota, morphology and disease resistance of largemouth bass (Micropterus salmoides). Aquac Rep (2022) 22:100954. doi: 10.1016/j.aqrep.2021.100954
11. Shi Y, Zhong L, Zhong H, Zhang JZ, Liu X, Peng M, et al. Taurine supplements in high-carbohydrate diets increase growth performance of monopterus albus by improving carbohydrate and lipid metabolism, reducing liver damage, and regulating intestinal microbiota. Aquaculture (2022) 554:738150. doi: 10.1016/j.aquaculture.2022.738150
12. Wang YR, Wang L, Zhang CX, Song K. Effects of substituting fishmeal with soybean meal on growth performance and intestinal morphology in orange-spotted grouper (Epinephelus coioides). Aquac Rep (2017) 5:52–7. doi: 10.1016/j.aqrep.2016.12.005
13. Zhao W, Xie JJ, Fang HH, Liu YJ, Tian LX, Niu J. Effects of corn starch level on growth performance, antioxidant capacity, gut morphology and intestinal microflora of juvenile golden pompano, trachinotus ovatus. Aquaculture (2020) 524:735197. doi: 10.1016/j.aquaculture.2020.735197
14. Zhou CP, Lin HZ, Huang Z, Wang J, Wang Y, Yu W. Effects of dietary leucine levels on intestinal antioxidant status and immune response for juvenile golden pompano (Trachinotus ovatus) involved in Nrf2 and NF-κB signaling pathway. Fish Shellfish Immunol (2020) 107(Pt A):336–45. doi: 10.1016/j.fsi.2020.10.012
15. Dong J, Cheng RJ, Yang YH, Zhao YY, Wu GF, Zhang RY, et al. Effects of dietary taurine on growth, non-specific immunity, anti-oxidative properties and gut immunity in the Chinese mitten crab eriocheir sinensis. Fish Shellfish Immunol (2018) 82:212–9. doi: 10.1016/j.fsi.2018.08.029
16. Shi Y, Zhong L, Zhong H, Zhang JZ, Che CB, Fu GH, et al. Taurine supplements in high-fat diets improve survival of juvenile monopterus albus by reducing lipid deposition and intestinal damage. Aquaculture (2022) 547:737431. doi: 10.1016/j.aquaculture.2021.737431
17. Shi Y, Hu Y, Wang ZQ, Zhou JC, Zhang JZ, Zhong H, et al. The protective effect of taurine on oxidized fish-Oil-Induced liver oxidative stress and intestinal barrier-function impairment in juvenile ictalurus punctatus. Antioxidants (2021) 10(11):1690. doi: 10.3390/antiox10111690
18. Matsunari H, Furuita H, Yamamoto T, Kim SK, Sakakura Y, Takeuchi T. Effect of dietary taurine and cystine on growth performance of juvenile red sea bream pagrus major. Aquaculture (2008) 274(1):142–7. doi: 10.1016/j.aquaculture.2007.11.002
19. Kotzamanis Y, Tsironi T, Brezas A, Grigorakis K, Ilia V, Vatsos I, et al. High taurine supplementation in plant protein-based diets improves growth and organoleptic characteristics of European seabass (Dicentrarchus labrax). Sci Rep (2020) 10(1):12294. doi: 10.1038/s41598-020-69014-x
20. Wang QC, He G, Wang X, Mai KS, Xu W, Zhou HH. Dietary sulfur amino acid modulations of taurine biosynthesis in juvenile turbot (Psetta maxima). Aquaculture (2014) 422-423:141–5. doi: 10.1016/j.aquaculture.2013.12.014
21. Bin P, Huang R, Zhou X. Oxidation resistance of the sulfur amino acids: Methionine and cysteine. BioMed Res Int (2017) 2017:9584932. doi: 10.1155/2017/9584932
22. Blouet C, Mariotti F, Azzout-Marniche D, Mathé V, Mikogami T, Tomé D, et al. Dietary cysteine alleviates sucrose-induced oxidative stress and insulin resistance. (2007) Free Radical Biology and Medicine42(7):1089–97. doi: 10.1016/j.freeradbiomed.2007.01.006
23. Li P, Wu GY. Important roles of amino acids in immune responses. Br J Nutr (2022) 127(3):398–402. doi: 10.1017/s0007114521004566
24. Stojanović M, Šćepanović L, Todorović D, Mitrović D, Šćepanović V, Šćepanović R, et al. Suppression of methionine-induced colon injury of young rats by cysteine and n-acetyl-l-cysteine. Mol Cell Biochem (2018) 440(1):53–64. doi: 10.1007/s11010-017-3155-1
25. Song ZH, Tong G, Xiao K, Jiao LF, Ke YL, Hu CH. L-cysteine protects intestinal integrity, attenuates intestinal inflammation and oxidant stress, and modulates NF-κB and Nrf2 pathways in weaned piglets after LPS challenge. Innate Immun (2016) 22(3):152–61. doi: 10.1177/1753425916632303
26. Ni HJ, Long LN, Bin P, Azad MAK, Xu K, Zhou XH, et al. Maternal cysteine intake influenced oxidative status and lipid-related gut microbiota and plasma metabolomics in male suckling piglets. Anim Feed Sci Technol (2021) 276:114947. doi: 10.1016/j.anifeedsci.2021.114947
27. Kim CJ, Kovacs-Nolan J, Yang C, Archbold T, Fan MZ, Mine Y. L-cysteine supplementation attenuates local inflammation and restores gut homeostasis in a porcine model of colitis. Biochim Biophys Acta (BBA) - Gen Subj (2009) 1790(10):1161–9. doi: 10.1016/j.bbagen.2009.05.018
28. Lee J, Harris PM, Sinclair BR, Treloar BP. Whole body metabolism of cysteine and glutathione and their utilization in the skin of Romney sheep: consequences for wool growth. J Agric Sci (1993) 121(1):111–24. doi: 10.1017/s0021859600076863
29. Dryden GM, Wickham GA, Cockrem F. Intravenous infusion of cysteine and wool growth of romney sheep. New Z J Agric Res (1969) 12(3):580–7. doi: 10.1080/00288233.1969.10421242
30. Chang XL, Liu P, Feng JC, Su X, Huang MY, Chen YY, et al. Impact of chronic exposure to the ionic liquid ([C8mim][PF6]) on intestinal physical barrier, immunological barrier and gut microbiota in common carp (Cyprinus carpio l.). Environ Res (2020) 189109919. doi: 10.1016/j.envres.2020.109919
31. Ding SJ, Fang J, Liu G, Veeramuthu D, Naif Abdullah AD, Yin YL. The impact of different levels of cysteine on the plasma metabolomics and intestinal microflora of sows from late pregnancy to lactation. Food Funct (2019) 10(2):691–702. doi: 10.1039/c8fo01838c
32. Ren ZZ, Yan JK, Whelan R, Liao XJ, Bütz DE, Arendt MK, et al. Dietary supplementation of sulfur amino acids improves intestinal immunity to eimeria in broilers treated with anti-interleukin-10 antibody. Anim Nutr (2022) 10:382–9. doi: 10.1016/j.aninu.2022.06.008
33. Niu J, Figueiredo-Silva C, Dong Y, Yue YR, Lin HZ, Wang J, et al. Effect of replacing fish meal with soybean meal and of DL-methionine or lysine supplementation in pelleted diets on growth and nutrient utilization of juvenile golden pompano (Trachinotus ovatus). Aquac Nutr (2016) 22(3):606–14. doi: 10.1111/anu.12284
34. Gao J, Liu MJ, Guo HY, Zhu KC, BS L, et al. ROS induced by streptococcus agalactiae activate inflammatory responses via the TNF-α/NF-κB signaling pathway in golden pompano Trachinotus ovatus (Linnaeus, 1758). Antioxidants (2022) 11(9):1809. doi: 10.3390/antiox11091809
35. Ma QW, Guo HY, Zhu KC, Guo L, Liu BS, Zhang N, et al. Dietary taurine intake affects growth and taurine synthesis regulation in golden pompano, Trachinotus ovatus (Linnaeus 1758). Aquaculture (2021) 530:735918. doi: 10.1016/j.aquaculture.2020.735918
36. Nakamura K, Gonzales-Plasus MM, Ushigusa-Ito T, Masuda R, Kabeya N, Kondo H, et al. Taurine synthesis via the cysteic acid pathway: effect of dietary cysteic acid on growth, body taurine content, and gene expression of taurine-synthesizing enzymes, growth hormone, and insulin-like growth factor 1 in Japanese flounder Paralichthys olivaceus. Fisheries Sci (2021) 87(3):353–63. doi: 10.1007/s12562-021-01500-1
37. Busti S, Bonaldo A, Diana A, Perfetti S, Viroli C, Fontanillas R, et al. The incidence of different pellet size on growth, gut evacuation, feed digestibility and feed waste in gilthead sea bream (Sparus aurata). Aquaculture (2022) 555:738204. doi: 10.1016/j.aquaculture.2022.738204
38. Fan JQ, Lu KC, Chen GL, Li BB, Song F, Chen YH. Transcriptome analysis of the influence of high plant protein based diet on trachinotus ovatus liver. Fish Shellfish Immunol 2021119:339–46. doi: 10.1016/j.fsi.2021.10.013
39. Ding ZJ, Wang X, Liu YD, Zheng YC, Li HP, Zhang MY, et al. Dietary mannan oligosaccharides enhance the non-specific immunity, intestinal health, and resistance capacity of juvenile blunt snout bream (Megalobrama amblycephala) against aeromonas hydrophila. Front Immunol (2022) 13:863657. doi: 10.3389/fimmu.2022.863657
40. Zhang Z, Tang YH, Fang W, Cui K, Xu D, Liu GB, et al. Octanoate alleviates dietary soybean oil-induced intestinal physical barrier damage, oxidative stress, inflammatory response and microbial dysbiosis in Large yellow croaker (Larimichthys crocea). Front Immunol (2022) 13:892901. doi: 10.3389/fimmu.2022.892901
41. Liu MJ, Guo HY, Liu B, Zhu KC, Guo L, Liu BS, et al. Gill oxidative damage caused by acute ammonia stress was reduced through the HIF-1α/NF-κb signaling pathway in golden pompano (Trachinotus ovatus). Ecotoxicol Environ Saf (2021) 222:112504. doi: 10.1016/j.ecoenv.2021.112504
42. Xie JJ, He XS, Fang HH, Liao SY, Liu YJ, Tian LX, et al. Identification of heme oxygenase-1 from golden pompano (Trachinotus ovatus) and response of Nrf2/HO-1 signaling pathway to copper-induced oxidative stress. Chemosphere (2020) 253:126654. doi: 10.1016/j.chemosphere.2020.126654
43. Liu MJ, Guo HY, Zhu KC, Liu BS, Liu B, Guo L, et al. Effects of acute ammonia exposure and recovery on the antioxidant response and expression of genes in the Nrf2-Keap1 signaling pathway in the juvenile golden pompano (Trachinotus ovatus). Aquat Toxicol (2021) 240:105969. doi: 10.1016/j.aquatox.2021.105969
44. Zhang ZS, Hu XC, Diao QY, Zhang PP, Wu Y, Cao ZJ, et al. Macrophage migration inhibitory factor (MIF) of golden pompano (Trachinotus ovatus) is involved in the antibacterial immune response. Dev Comp Immunol (2022) 133:104445. doi: 10.1016/j.dci.2022.104445
45. Livak KJ, Schmittgen TD. Analysis of relative gene expression data using real-time quantitative PCR and the 2–ΔΔCT method. Methods (2001) 25(4):402–8. doi: 10.1006/meth.2001.1262
46. Torrecillas S, Terova G, Makol A, Serradell A, Valdenegro-Vega V, Izquierdo M, et al. Dietary phytogenics and galactomannan oligosaccharides in low fish meal and fish oil-based diets for European Sea bass (Dicentrarchus labrax) juveniles: Effects on gill structure and health and implications on oxidative stress status. Front Immunol (2021) 12:663106. doi: 10.3389/fimmu.2021.663106
47. Candebat CL, Stephens F, Booth MA, Fernando F, Lopata A, Pirozzi I. Adequate levels of dietary sulphur amino acids impart improved liver and gut health in juvenile yellowtail kingfish (Seriola lalandi). Br J Nutr (2022) pp:1–58. doi: 10.1017/s0007114522002458
48. Li SH, Luo X, Liao ZB, Xu HG, Liang MQ, Mai K, et al. Additional supplementation of sulfur-containing amino acids in the diets improves the intestinal health of turbot fed high-lipid diets. Fish Shellfish Immunol (2022) 130:368–79. doi: 10.1016/j.fsi.2022.09.015
49. Li XY, Yao XZ, Zhang XC, Dong XH, Chi SY, Tan BP, et al. Effects of dietary chenodeoxycholic acid supplementation in a low fishmeal diet on growth performance, lipid metabolism, autophagy and intestinal health of pacific white shrimp, litopenaeus vannamei. Fish Shellfish Immunol (2022) 127:1088–99. doi: 10.1016/j.fsi.2022.07.045
50. Xie SW, Wei D, Liu YJ, Tian LX, Niu J. Dietary fish oil levels modulated lipid metabolism, immune response, intestinal health and salinity stress resistance of juvenile penaeus monodon fed a low fish-meal diet. Anim Feed Sci Technol (2022) 289:115321. doi: 10.1016/j.anifeedsci.2022.115321
51. Ajayi OI, Smith OF, Oso AO, Oke OE. Evaluation of in ovo feeding of low or high mixtures of cysteine and lysine on performance, intestinal morphology and physiological responses of thermal-challenged broiler embryos. Front Physiol (2022) 13:972041. doi: 10.3389/fphys.2022.972041
52. Martel J, Chang SH, Ko YF, Hwang TL, Young JD, Ojcius DM. Gut barrier disruption and chronic disease. Trends Endocrinol Metab (2022) 33(4):247–65. doi: 10.1016/j.tem.2022.01.002
53. Zhou Q, Zhu X, Li YZ, Yang PS, Wang SP, Ning K, et al. Intestinal microbiome-mediated resistance against vibriosis for cynoglossus semilaevis. Microbiome (2022) 10(1):153. doi: 10.1186/s40168-022-01346-4
54. An J, Liu YQ, Wang YQ, Fan R, Hu XR, Zhang F, et al. The role of intestinal mucosal barrier in autoimmune disease: A potential target. Front Immunol (2022) 13:871713. doi: 10.3389/fimmu.2022.871713
55. Chopyk DM, Grakoui A. Contribution of the intestinal microbiome and gut barrier to hepatic disorders. Gastroenterology (2020) 159(3):849–63. doi: 10.1053/j.gastro.2020.04.077
56. Edwards JL, Smith DL, Connolly J, McDonald JE, Cox MJ, Joint I, et al. Identification of carbohydrate metabolism genes in the metagenome of a marine biofilm community shown to be dominated by gammaproteobacteria and bacteroidetes. Genes (2010) 1(3):371–84. doi: 10.3390/genes1030371
57. Kabisch A, Otto A, König S, Becher D, Albrecht D, Schüler M, et al. Functional characterization of polysaccharide utilization loci in the marine Bacteroidetes ‘Gramella forsetii’ KT0803. ISME J (2014) 8(7):1492–502. doi: 10.1038/ismej.2014.4
58. Tang K, Lin YF, Han Y, Jiao NZ. Characterization of potential polysaccharide utilization systems in the marine Bacteroidetes gramella flava JLT2011 using a multi-omics approach. Front Microbiol (2017) 8:220. doi: 10.3389/fmicb.2017.00220
59. Wu T, Gao YF, Hao JY, Geng JT, Zhang JJ, Yin JJ, et al. Capsanthin extract prevents obesity, reduces serum TMAO levels and modulates the gut microbiota composition in high-fat-diet induced obese C57BL/6J mice. Food Res Int (2020) 128:108774. doi: 10.1016/j.foodres.2019.108774
60. Wei HC, Chen P, Liang XF, Yu HH, Wu XF, Han J, et al. Plant protein diet suppressed immune function by inhibiting spiral valve intestinal mucosal barrier integrity, anti-oxidation, apoptosis, autophagy and proliferation responses in amur sturgeon (Acipenser schrenckii). Fish Shellfish Immunol (2019) 94:711–22. doi: 10.1016/j.fsi.2019.09.061
61. Itoh K, Wakabayashi N, Katoh Y, Ishii T, Engel JD, Yamamoto M. Keap1 represses nuclear activation of antioxidant responsive elements by Nrf2 through binding to the amino-terminal Neh2 domain. Genes Dev (1999) 13(1):76–86. doi: 10.1101/gad.13.1.76
62. Li Y, Liang SS, Shao YR, Li YY, Chen CY, You CH, et al. Impacts of dietary konjac glucomannan supplementation on growth, antioxidant capacity, hepatic lipid metabolism and inflammatory response in golden pompano (Trachinotus ovatus) fed a high fat diet. Aquaculture (2021) 545:737113. doi: 10.1016/j.aquaculture.2021.737113
63. Yu GJ, Liu Y, Ou WH, Dai JH, Ai QH, Zhang WB, et al. The protective role of daidzein in intestinal health of turbot (Scophthalmus maximus l.) fed soybean meal-based diets. Sci Rep (2021) 11(1):3352. doi: 10.1038/s41598-021-82866-1
64. Jain SK, Velusamy T, Croad JL, Rains JL, Bull R. L-cysteine supplementation lowers blood glucose, glycated hemoglobin, CRP, MCP-1, and oxidative stress and inhibits NF-κB activation in the livers of zucker diabetic rats. Free Radical Biol Med (2009) 46(12):1633–8. doi: 10.1016/j.freeradbiomed.2009.03.014
65. Liu N, Zhang GX, Niu YT, Wang Q, Zheng J, Yang JM, et al. Anti-inflammatory and analgesic activities of indigo through regulating the IKKβ/IκB/NF-κB pathway in mice. Food Funct (2020) 11(10):8537–46. doi: 10.1039/c9fo02574j
66. Rungrassamee W, Klanchui A, Maibunkaew S, Karoonuthaisiri N. Bacterial dynamics in intestines of the black tiger shrimp and the pacific white shrimp during vibrio harveyi exposure. J Invertebrate Pathol (2016) 133:12–9. doi: 10.1016/j.jip.2015.11.004
Keywords: Trachinotus ovatus, cysteine, intestine immunity, growth, antioxidant capacity, Streptococcus agalactiae
Citation: Liu J-X, Zhu K-C, Guo H-Y, Liu B-S, Zhang N and Zhang D-C (2022) Effects of cysteine addition to low-fishmeal diets on the growth, anti-oxidative stress, intestine immunity, and Streptococcus agalactiae resistance in juvenile golden pompano (Trachinotus ovatus). Front. Immunol. 13:1066936. doi: 10.3389/fimmu.2022.1066936
Received: 11 October 2022; Accepted: 01 November 2022;
Published: 17 November 2022.
Edited by:
Qiyou Xu, Huzhou University, ChinaReviewed by:
Bo Liu, Freshwater Fisheries Research Center (CAFS), ChinaGuoxing Nie, Henan Normal University, China
Wu Xiaoyi, Hainan University, China
Copyright © 2022 Liu, Zhu, Guo, Liu, Zhang and Zhang. This is an open-access article distributed under the terms of the Creative Commons Attribution License (CC BY). The use, distribution or reproduction in other forums is permitted, provided the original author(s) and the copyright owner(s) are credited and that the original publication in this journal is cited, in accordance with accepted academic practice. No use, distribution or reproduction is permitted which does not comply with these terms.
*Correspondence: Dian-Chang Zhang, zhangdch@scsfri.ac.cn