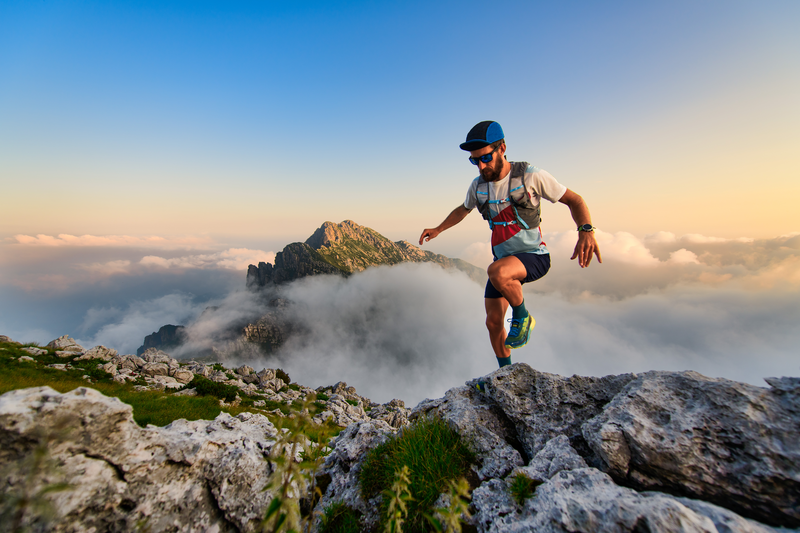
94% of researchers rate our articles as excellent or good
Learn more about the work of our research integrity team to safeguard the quality of each article we publish.
Find out more
ORIGINAL RESEARCH article
Front. Immunol. , 03 November 2022
Sec. Inflammation
Volume 13 - 2022 | https://doi.org/10.3389/fimmu.2022.1057746
This article is part of the Research Topic New Insights of Immune Cells in Cardiovascular and Metabolic Disorders View all 36 articles
Inflammatory macrophages play a pivotal role in the progression of inflammatory cystitis. Formation of NOD-, LRR- and PYD domains-containing protein 3 (NLRP3) inflammasome triggers the activation of caspase-1/IL-1β signaling cascades to mediate inflammatory response. However, it is not known whether NLRP3 activation in macrophages during cystitis may differ in normal or diabetic setting as well as the importance of it. In this study, we found that NLRP3 levels significantly increased in bladder macrophages in diabetic mice that underwent cystitis. Moreover, bladder macrophages from diabetic mice appeared to have increased their potential of growth, migration and phagocytosis. Furthermore, specific depletion of NLRP3 in macrophages alleviated the severity of cystitis in diabetic mice, but not in non-diabetic mice. Together, our data suggest that NLRP3 depletion in macrophages may be a promising strategy for treating diabetic cystitis.
Interstitial cystitis (IC) and bladder pain syndrome (BPS) are clinical syndromes manifested with frequent urgency, urination, accompanied with discomfort in the suprapubic bladder area (1). The majority of IC/PBS patients are women, with a male-to-female ratio of about 1:10 (2). In 2011, Berry et al. found that the prevalence of IC/BPS was about 2.7% to 6.5% based on large epidemiological data of the adult female population in the United States (3). IC/BPS seriously affects the quality of life of patients. Chronic pain, avoidance of sex, and poor sleep quality directly affect work and life (4). The etiology of IC/PBS is unknown, although interstitial cystitis has been regarded as a chronic inflammatory disorder.
To improve our current understanding of the pathophysiology of IC/BPS, many animal models have been used (5). So far, cyclophosphamide (CYP)-induced urinary bladder disorder is one of the best experimental models for IC/BPS (6). Of note, CYP-induced cystitis model causes a local inflammation associated with most symptoms detected in IC/BPS in human patients (7). Therefore, the CYP-induced cystitis model appears to be widely used in rodents to study the mechanisms underlying the pathological changes in cystitis (8).
Diabetic patients are prone to getting IC/BPS, and they are often suffered from other bladder issues such as frequent urinary tract infection (UTI) and neurogenic bladder, for which the patients lose the control of their bladder due to issues from the brain, spinal cord, or nerves (9). However, the mechanism remains largely unknown. Very recently, it is recognized that inflammatory macrophages play a pivotal role in the pathological progression of cystitis (10). This finding brought a breakthrough for understanding the effects of diabetes on IC/BPS, since diabetes is well-known metabolic disease associated with dysfunction in immunological regulation of the body (11).
The display of macrophage phenotype to exhibit either a pro-inflammatory phenotype, which is called “M1”, or an anti-inflammatory phenotype, which is called “M2”, is known as “polarization of a macrophage” (12). The newly generated macrophages with any polarization are naïve macrophages (M0) (13). Interleukin 1β (IL-1β) is a key cytokine that regulate inflammation (14). The effects of IL-1β on inflammation are complex and context-sensitive. Inflammasomes are known regulators for IL-1β activation (15). The most important inflammasome is NOD-, LRR- and PYD domains-containing protein 3 (NLRP3), which promotes the dimerization of caspase-1 to catalyze the pro-IL-1β into IL-1β to be active (16). However, it is not known whether NLRP3 activation in macrophages during IC may differ in normal or a diabetic setting as well as the importance of it. This question was hence addressed in the current study.
All the experiments including animal work have been approved by and performed according to the guideline by institutional Animal Research Ethics Council from Shanghai Jiao Tong University School of Medicine. This study did not involve human specimens.
Mice with macrophage-depletion of NLRP3 were generated using Lysosome2 (Lys2)-Cre (Strain #:018956; The Jackson Laboratory, Bar Harbor, ME, USA) and NLRP3(fx/fx) (Strain #:017970; The Jackson Laboratory). The NLRP3(fx/fx) mice without Lys-Cre were used as controls. C57/Bl6 mice were used as wildtype mice. In all experiments, both male and female mice at age of 10 weeks were used and evenly distributed in the experimental groups. After an overnight fasting that exceeds 16 hours (D0), one intraperitoneal (i.p) injection of 110 mg/kg streptozotocin (STZ) in 150µl normal saline was performed in mice to induce diabetes. An equal volume of normal saline was given i.p. to control mice. An overnight fasting was required for both fasting blood glucose measurement and intraperitoneal glucose tolerance testing (IPGTT). For IPGTT, 2mg glucose was challenged to the mice, after which glucose levels were determined at different time points. Cystitis was induced by i.p. injection of CYP (70 mg/kg, Fisher-Scientific, Pittsburgh, PA, USA) 4 times on D6, 8, 10 and 12. Control animals received saline of equal volume. Von Frey test was done at D13 according to published protocols. Briefly, mice were put on a raised wire mesh floor to allow at least 30 minutes’ acclimatization before starting the test. Nociceptive response was evaluated after the mouse abdomen close to urinary bladder were challenged with several von Frey filaments of increasing forces. The scoring of nociceptive response was as 0 for no response, as 1 for detection of some reaction, as 2 for detection of mouse reaction and position changing, and as 3 for detection of licking or vocalizing of stimulated area in addition to those for 2. Nociceptive score was presented as a relative value. Beta-cell mass was assessed at sacrifice (D16) by calculating the product of the percentage of the area positive for insulin staining (Abcam, San Jose, CA, USA) to the total pancreatic area with the pancreatic weight. For the first experiment, 4 groups of C57/Bl6 mice of 6 of each were used. Group 1: mice received i.p. saline (control for STZ) at D0 and i.p. saline (control for CYP) at D6, 8, 10 and 12 (saline+saline); Group 2: mice received i.p. STZ at D0 and i.p. saline (control for CYP) at D6, 8, 10 and 12 (STZ+saline); Group 3: mice received i.p. saline (control for STZ) at D0 and i.p. CYP at D6, 8, 10 and 12 (saline+CYP); Group 4: mice received i.p. STZ at D0 and i.p. CYP at D6, 8, 10 and 12 (STZ+CYP). For the second experiment, 4 groups of Lys2-Cre; NLRP3(fx/fx) or NLRP3(fx/fx) mice of 6 of each were used. Group 1: NLRP3(fx/fx) mice received i.p. saline (control for STZ) at D0 and i.p. CYP at D6, 8, 10 and 12 (saline+CYP/N); Group 2: Lys2-Cre; NLRP3(fx/fx) mice received i.p. saline (control for STZ) at D0 and i.p. CYP at D6, 8, 10 and 12 (saline+CYP/LN); Group 3: NLRP3(fx/fx) mice received i.p. STZ at D0 and i.p. CYP at D6, 8, 10 and 12 (STZ+CYP/N); Group 4: Lys2-Cre; NLRP3(fx/fx) mice received i.p. STZ at D0 and i.p. CYP at D6, 8, 10 and 12 (STZ+CYP/LN).
At sacrifice (D16), mouse bladders were immediately collected and measured for bladder weight, edema and vascular permeability Inflammation. Edema score was obtained as 0 for no edema, 1 for mild edema, 2 for moderate edema and 3 for severe edema. Vesical vascular permeability was evaluated by the Evans blue extravasation technique on the sectioned bladder slides. Briefly, Evans blue (20 mg/kg) was injected from tail vein 30 minutes before post-perfusion sacrifice and then measured by tissue absorbance at 620 nm.
For flow cytometry analysis, mouse bladder was dissected out and dissociated into a single cell population with 40 minutes’ treatment with 0.25% Trypsin (Invitrogen) at 37°C. The cells were then labeled with PE-cy5-conjugated F4/80 and FITC-conjugated CD163 antibodies (Becton-Dickinson Biosciences, Shanghai, China). Flow cytometry data were analyzed and presented by FlowJo software (Flowjo LLC, Ashland, OR, USA).
Total protein was extracted from FACS-isolated cells to be used for ELISA analysis with specific kits for mouse NLRP3 (ab279417; Abcam, Cambridge, MA, USA), nitric oxide synthase (iNOS, ab253219; Abcam), tumor necrosis factor alpha (TNFα, ab208348; Abcam), interferon gamma (IFNγ, ab282874; Abcam), CD163 (ab272204; Abcam) and proliferating cell nuclear antigen (PCNA, ab196270; Abcam).
Cell growth was determined by viable cell number with a Cell Counting Kit-8 assay (CCK-8, Sigma-Aldrich). Cell migration was determined by a transwell cell migration assay, as reported (17). Briefly, cells were plated at 5X103 cells/cm2 in the corresponding culture vessel using RPMI1640 culture medium (Invitrogen), after which the cell culture inserts were put into the wells of the 24-well plate with 750μL medium with 10% FBS to the outer compartment. The transmigration was performed in a humidified incubator (37°C, 5% CO2) for 4 hours, followed by cell fixation and staining of the migrated cells with Crystal Violet (V5265, Sigma-Aldrich) for 20 minutes at room temperature.
Phagocytosis was assessed based on 30 minutes’ zymosan intake by macrophages using a zymosan-based phagocytotic kit (ab211156, Abcam), or based on intake of GFP+ bacteria with a flow cytometry-based method as described (18).
A GEO database GSE183698 was used to analyzed gene profile from mouse macrophages with or without NLRP3 depletion. R software was used to analyze differentiated genes, while String online tools were used to analyze gene interaction network. All data were statistically analyzed with GraphPad Prism 7 (GraphPad, Chicago, IL, USA). One-way ANOVA method was applied for determining the significance (p<0.05) or no significance (ns, p<0.05). Individual values, the mean and standard deviation (SD) were all shown in the figures.
In order to study the molecular mechanisms underlying the diabetic cystitis, we applied a most commonly used rodent model for cystitis. STZ was used at D0 to develop diabetes in mice. After 6 days when diabetes was confirmed, experimental cystitis was induced by 4 i.p. injection of CYP on D6, 8, 10 and 12. Von Frey test was done at D13, and mice were sacrificed at D16 for end assessments (Figure 1A). Four groups of mice were used. Group 1: mice received i.p. saline (control for STZ) at D0 and i.p. saline (control for CYP) at D6, 8, 10 and 12 (saline+saline); Group 2: mice received i.p. STZ at D0 and i.p. saline (control for CYP) at D6, 8, 10 and 12 (STZ+saline); Group 3: mice received i.p. saline (control for STZ) at D0 and i.p. CYP at D6, 8, 10 and 12 (saline+CYP); Group 4: mice received i.p. STZ at D0 and i.p. CYP at D6, 8, 10 and 12 (STZ+CYP). In saline-treated group (control of CYP), diabetes did not alter the nociceptive score of the mice at Von Frey test, while in CYP-treated group, diabetes significantly worsened the nociceptive score of the mice at Von Frey test (Figure 1B). Moreover, in saline-treated group (control of CYP), diabetes did not cause the changes in body weight, while in CYP-treated group, diabetes caused significantly more loss of the body weight (Figure 1C). At sacrifice, we found that in saline-treated group (control of CYP), diabetes did not alter the bladder weight (Figure 1D), edema score of the bladder (Figure 1E) or the vesical vascular permeability in bladder (Figure 1F), while in CYP-treated group, diabetes significantly increased bladder weight (Figure 1D), edema score of the bladder (Figure 1E) or the vesical vascular permeability in bladder (Figure 1F). Together, these data suggest that diabetes aggravates CYP-induced cystitis.
Figure 1 Diabetes aggravates CYP-induced cystitis. (A) Schematic of experiment: STZ was used at D0 to develop diabetes in mice. After 6 days when diabetes was confirmed, experimental cystitis was induced by 4 i.p. injection of CYP on D6, 8, 10 and 12. Von Frey test was done at D13, and mice were sacrificed at D16 for end assessments. (B-F) Four groups of mice were used. Group 1: mice received i.p. saline (control for STZ) at D0 and i.p. saline (control for CYP) at D6, 8, 10 and 12 (saline+saline); Group 2: mice received i.p. STZ at D0 and i.p. saline (control for CYP) at D6, 8, 10 and 12 (STZ+saline); Group 3: mice received i.p. saline (control for STZ) at D0 and i.p. CYP at D6, 8, 10 and 12 (saline+CYP); Group 4: mice received i.p. STZ at D0 and i.p. CYP at D6, 8, 10 and 12 (STZ+CYP). (B) Nociceptive score of the mice at Von Frey test at D13. (C) Changes in body weight. (D) Bladder weight at D16. (E) Edema score of the bladder at D16. (F) Vesical vascular permeability in bladder at D16. *p<0.05 (STZ+CYP vs saline+CYP). #p<0.05 (saline+CYP vs saline+saline or STZ+saline).
To exclude a possibility that the CYP treatment may affect the diabetic status in mice, we monitored the changes in fasting blood glucose, and examined the end point glucose response of mice as well as beta cell mass. We found that CYP-treatment did not alter fasting blood glucose (Figure 2A), the end point glucose response of mice (Figure 2B), as well as beta cell mass (Figures 2C, D). These data suggest that CYP-induced cystitis does not affect diabetic status in mice.
Figure 2 CYP-induced cystitis does not affect diabetic status in mice. (A) Fasting blood glucose. (B) IPGTT at D16. (C) Beta cell mass at D16. (D) Representative insulin staining in mouse pancreas. *p<0.05 (STZ+CYP vs saline+CYP or STZ+saline vs saline+saline). Scale bars are 100µm.
Next, we aimed to figure out whether bladder macrophages could be affected by diabetes and then play a role in the aggravation of the cystitis. Thus, we digested mouse bladders and analyzed and sorted bladder macrophages based on positivity for F4/80, and further distinguished M1 (CD163-) and M2 (CD163+) subtypes among all F4/80+ macrophages. We did not detect significant changes in the total macrophage numbers or M1/M2 distributions in all 4 groups (Figures 3A, B). However, when the sorted bladder macrophages were analyzed for some critical genes for macrophage phenotype and functionality, we found that diabetic status of the mice caused increases in iNOS, TNFα and IFNγ, 3 factors associated with proinflammatory function and phagocytosis of macrophage, increases in PCNA, a cell proliferation marker, and a dramatic increase in NLRP3, without changing CD163, a M2 macrophage marker (Figures 3C). These data suggest that although diabetes did not cause a major shift of macrophage polarity, it appeared to significantly alter the function of macrophages.
Figure 3 NLRP3 significantly increases in bladder macrophages in diabetic mice that undergo cystitis. (A, B) Mouse bladders were sorted for bladder macrophages based on positivity for F4/80, and further for M1 (CD163-) and M2 (CD163+) subtypes among all F4/80+ macrophages, shown by representative flow charts (A) and by quantification (B). (C) ELISA for some critical factors for macrophage phenotype and functionality. *p<0.05. ns: no significance.
In order to determine whether NLRP3 may be responsible for the phenotypic changes in macrophages under diabetes, a GEO database GSE183698 was used to analyzed gene profile from mouse macrophages with or without NLRP3 depletion (NLRP3KO, WT). In this database, in all significantly upregulated and significantly downregulated genes (p<0.05; logFC>1 or logFC<-1), there were 120 genes associated with fine determination of macrophage phenotype (Figure 4A) analyzed by String online tool. However, although NLRP3 depletion (Figure 4B) resulted in alteration in 120 genes associated with macrophage polarization, none of the key M1/M2 markers [iNOS, TNFα, IFNγ, arginase 1 (ARG1), CD163 and IL-10] were significantly altered (Figure 4C), suggesting that depletion of NLRP3 may result in some delicate changes in the characteristics of macrophages, but not result in a major M1/M2 shift.
Figure 4 NLRP3 depletion in macrophages alters genes associated with macrophage phenotype. (A-C) A GEO database GSE183698 was used to analyzed gene profile from mouse macrophages with or without NLRP3 depletion (NLRP3KO, WT). (A) Genes associated with fine determination of macrophage phenotype analyzed by String online tool. (B, C) Gene reads from NLRP3 and some key factors associated with macrophage phenotype and functionality. *p<0.05.
Next, bladder macrophages from 4 groups of the mice were subjected to analysis on cell growth and migration. We found that diabetes increased the growth of bladder macrophages from CYP-treated mice, but not in bladder macrophages from mice without cystitis (Figures 5A, B). Similarly, diabetes increased the migration of bladder macrophages from CYP-treated mice, but not in bladder macrophages from mice without cystitis (Figures 5C, D). These data were consistent with analysis on the key factors in macrophages (Figures 3C), suggesting that diabetes increases growth and migration potential of macrophages from inflammatory bladder.
Figure 5 Diabetes increases growth and migration potential of macrophages from inflammatory bladder. (A, B) Bladder macrophages from 4 groups of the mice were analyzed for cell growth in a CCK-8 assay (A) and in assay to determine DNA (B). (C, D) Bladder macrophages from 4 groups of the mice were analyzed for migration, shown by quantification (C) and by representative images (D). *p<0.05. ns: no significance. Scale bars are 100µm.
Phagocytosis is a major function of macrophages and is related to levels of inflammatory responses and resolution. We thus analyzed the phagocytosis of bladder macrophages from 4 groups of the mice. First, we used a phagocytosis assay that analyzes 30 minutes’ zymosan intake, which showed that diabetes significantly increased the phagocytosis of bladder macrophages from CYP-treated mice, but not in bladder macrophages from mice without cystitis (Figures 6A, B). Next, we analyzed the intake of GFP+ bacteria by the bladder macrophages from 4 groups, showing that diabetes significantly increased the mean cellular GFP density from CYP-treated mice, but not in bladder macrophages from mice without cystitis (Figure 6C), suggesting that diabetes increases phagocytosis of macrophages from inflammatory bladder, also consistent with analysis on the key factors in macrophages (Figure 3C).
Figure 6 Diabetes increases phagocytosis of macrophages from inflammatory bladder. (A) A phagocytosis assay that analyzes 30 minutes’ zymosan intake. (B, C) Analysis of the intake of GFP+ bacteria, shown by representative images (B), by the mean cellular GFP density of macrophages (C). *p<0.05. Scale bars are 15µm. ns, no significance.
Finally, we wanted to understand whether the over-activation of NLRP3 in macrophages during diabetic cystitis may be important to the increased severity of the disease. For this reason, we generated mice with macrophage-depletion of NLRP3, Lys2-Cre; NLRP3(fx/fx) and control NLRP3(fx/fx). Macrophages were isolated with the bladders of these mice (Figure 7A) and confirmed for specific depletion of NLRP3 in macrophages from Lys2-Cre; NLRP3(fx/fx) mice (Figure 7B). Next, we applied the CYP model to these mice. Again, STZ was used at D0 to develop diabetes in mice. After 6 days when diabetes was confirmed, experimental cystitis was induced by 4 i.p. injection of CYP on D6, 8, 10 and 12. Von Frey test was done at D13, and mice were sacrificed at D16 for end assessments. Group 1: NLRP3(fx/fx) mice received i.p. saline (control for STZ) at D0 and i.p. CYP at D6, 8, 10 and 12 (saline+CYP/N); Group 2: Lys2-Cre; NLRP3(fx/fx) mice received i.p. saline (control for STZ) at D0 and i.p. CYP at D6, 8, 10 and 12 (saline+CYP/LN); Group 3: NLRP3(fx/fx) mice received i.p. STZ at D0 and i.p. CYP at D6, 8, 10 and 12 (STZ+CYP/N); Group 4: Lys2-Cre; NLRP3(fx/fx) mice received i.p. STZ at D0 and i.p. CYP at D6, 8, 10 and 12 (STZ+CYP/LN). We found that macrophage-depletion of NLRP3 did not alter the nociceptive score of the mice at Von Frey test in non-diabetic mice, but significantly increased the nociceptive score of the mice at Von Frey test in non-diabetic mice (Figure 7C). Moreover, macrophage-depletion of NLRP3 did not cause the changes in body weight of non-diabetic mice, but significantly decreased the body weight of diabetic mice (Figure 7D). At sacrifice, we found that macrophage-depletion of NLRP3 did not alter the bladder weight (Figure 7E), edema score of the bladder (Figure 7F) or the vesical vascular permeability in bladder (Figure 7G) of non-diabetic mice, but significantly increased bladder weight (Figure 7E), edema score of the bladder (Figure 7F) or the vesical vascular permeability in bladder (Figure 7G) of diabetic mice. Together, these data suggest that specific depletion of NLRP3 in macrophages alleviates the severity of cystitis in diabetic mice.
Figure 7 Specific depletion of NLRP3 in macrophages alleviates the severity of cystitis in diabetic mice. We generated mice with macrophage-depletion of NLRP3, Lys2-Cre; NLRP3(fx/fx) and control NLRP3(fx/fx). (A) Flow cytometry showing isolation of macrophages from the bladders of these mice. (B) ELISA for NLRP3 in macrophages nor non-macrophages from the mouse bladder. (C) STZ was used at D0 to develop diabetes in mice. After 6 days when diabetes was confirmed, experimental cystitis was induced by 4 i.p. injection of CYP on D6, 8, 10 and 12. Von Frey test was done at D13, and mice were sacrificed at D16 for end assessments. Group 1: NLRP3(fx/fx) mice received i.p. saline (control for STZ) at D0 and i.p. CYP at D6, 8, 10 and 12 (saline+CYP/N); Group 2: Lys2-Cre; NLRP3(fx/fx) mice received i.p. saline (control for STZ) at D0 and i.p. CYP at D6, 8, 10 and 12 (saline+CYP/LN); Group 3: NLRP3(fx/fx) mice received i.p. STZ at D0 and i.p. CYP at D6, 8, 10 and 12 (STZ+CYP/N); Group 4: Lys2-Cre; NLRP3(fx/fx) mice received i.p. STZ at D0 and i.p. CYP at D6, 8, 10 and 12 (STZ+CYP/LN). (C) Nociceptive score of the mice at Von Frey test at D13. (D) Changes in body weight. (E) Bladder weight at D16. (F) Edema score of the bladder at D16. (G) Vesical vascular permeability in bladder at D16. *p<0.05. ns: no significance.
Diabetes increases the incidence and severity of IC/BPS (19). The recognition of the importance of local inflammation to the development of cystitis highlights the importance of studies of the major player for innate immunity, bladder macrophages (20). A recent study has demonstrated a 6-fold increase in bladder monocytes/macrophages in IC/BPS patients compared to healthy controls (21). However, the exact molecular signaling that underlies the effects of innate immunity on cystitis is complex and not fully determined. It is known that the phenotypic adaptations of macrophages during the interaction are dynamic and involve not only changes in major functionality (M1 versus M2), but also some delicate changes in certain function such as phagocytotic, regenerative or immune resolution (22).
Here in this study, we found that diabetes likely affected the phenotype of macrophages in such a delicate manner, since diabetes seemed to alter the levels of markers for macrophage functionality, iNOS, TNFα and IFNγ, and the levels of PCNA, a cell proliferation marker (23–25). Interestingly, the effects of diabetes on cystitis are at least partially contributable to the increases in NLRP3, a regulator for IL-1β. It was supported by our in vivo experiment clearly showing a loss of aggravated cystitis in diabetes by macrophage-depletion of NLRP3.
The NLRP3 inflammasome has been found activated by many different stimuli, such as the increased extracellular glucose level in a diabetic setting (26). However, the exact molecular regulation of NLRP3 activation appeared to be complex and controlled by multiple factors (27, 28). It has been reported that NLRP3 is activated in different types of diabetes, while NLRP3 depletion seemed to have a protective effect against diabetes (29, 30).
Based on our findings in the current study, the increased proliferation, migration and the phagocytosis of bladder macrophages in a diabetic status appeared to be harmful to individuals baring cystitis (31, 32). It is believed that exaggerated inflammation could have an adverse effect on the normal immune reaction and its resolution (33). Apparently, diabetes can be such a condition that causes hyperreaction of the immune cells and the worsening of the disease.
To summarize, our data suggest that macrophage-depletion of NLRP3 attenuates the severity of cystitis in a diabetic status. Further exploration of the molecular mechanisms may improve our knowledge on the pathological and molecular events happening during cystitis to allow development of novel therapies.
The original contributions presented in the study are included in the article/supplementary material. Further inquiries can be directed to the corresponding author.
The animal study was reviewed and approved by Shanghai Jiao Tong University School of Medicine.
YP and YG are responsible for data acquisition and analysis. YP are responsible for study conception and design, data acquisition and analysis. YP wrote the manuscript and all authors have read the manuscript and agreed with the publication. YP are responsible for funding and are the guarantee of the study. All authors contributed to the article and approved the submitted version.
This work was supported by internal funding from RenJi Hospital.
The authors declare that the research was conducted in the absence of any commercial or financial relationships that could be construed as a potential conflict of interest.
All claims expressed in this article are solely those of the authors and do not necessarily represent those of their affiliated organizations, or those of the publisher, the editors and the reviewers. Any product that may be evaluated in this article, or claim that may be made by its manufacturer, is not guaranteed or endorsed by the publisher.
1. Sant GR, Saban R. Interstitial cystitis/bladder pain syndrome (IC/BPS) 2015: Part 1. Transl Androl Urol (2015) 4:484–5. doi: 10.3978/j.issn.2223-4683.2015.10.06
2. Sant GR, Saban R. Focused issue on interstitial cystitis/bladder pain syndrome (IC/BPS) 2015: Part 2. Transl Androl Urol (2015) 4:592–3. doi: 10.3978/j.issn.2223-4683.2015.11.07
3. Berry SH, Elliott MN, Suttorp M, Bogart LM, Stoto MA, Eggers P, et al. Prevalence of symptoms of bladder pain syndrome/interstitial cystitis among adult females in the united states. J Urol (2011) 186:540–4. doi: 10.1016/j.juro.2011.03.132
4. Konkle KS, Berry SH, Elliott MN, Hilton L, Suttorp MJ, Clauw DJ, et al. Comparison of an interstitial cystitis/bladder pain syndrome clinical cohort with symptomatic community women from the RAND interstitial cystitis epidemiology study. J Urol (2012) 187:508–12. doi: 10.1016/j.juro.2011.10.040
5. Akiyama Y, Luo Y, Hanno PM, Maeda D, Homma Y. Interstitial cystitis/bladder pain syndrome: The evolving landscape, animal models and future perspectives. Int J Urol (2020) 27:491–503. doi: 10.1111/iju.14229
6. Song PH, Chun SY, Chung JW, Kim YY, Lee HJ, Lee JN, et al. Comparison of 5 different rat models to establish a standard animal model for research into interstitial cystitis. Int Neurourol J (2017) 21:163–70. doi: 10.5213/inj.1734898.449
7. Matz EL, Hsieh MH. Review of advances in uroprotective agents for cyclophosphamide- and ifosfamide-induced hemorrhagic cystitis. Urology (2017) 100:16–9. doi: 10.1016/j.urology.2016.07.030
8. Birder L, Andersson KE. Animal modelling of interstitial Cystitis/Bladder pain syndrome. Int Neurourol J (2018) 22:S3–9. doi: 10.5213/inj.1835062.531
9. Laden BF, Bresee C, De Hoedt A, Dallas KB, Scharfenberg A, Saxena R, et al. Comorbidities in a nationwide, heterogenous population of veterans with interstitial Cystitis/Bladder pain syndrome. Urology (2021) 156:37–43. doi: 10.1016/j.urology.2021.04.015
10. Vera PL, Preston DM, Moldwin RM, Erickson DR, Mowlazadeh B, Ma F, et al. Elevated urine levels of macrophage migration inhibitory factor in inflammatory bladder conditions: A potential biomarker for a subgroup of interstitial Cystitis/Bladder pain syndrome patients. Urology (2018) 116:55–62. doi: 10.1016/j.urology.2018.02.039
11. Golden TN, Simmons RA. Immune dysfunction in developmental programming of type 2 diabetes mellitus. Nat Rev Endocrinol (2021) 17:235–45. doi: 10.1038/s41574-020-00464-z
12. Taylor PR, Martinez-Pomares L, Stacey M, Lin HH, Brown GD, Gordon S. Macrophage receptors and immune recognition. Annu Rev Immunol (2005) 23:901–44. doi: 10.1146/annurev.immunol.23.021704.115816
13. Gao J, Liang Y, Wang L. Shaping polarization of tumor-associated macrophages in cancer immunotherapy. Front Immunol (2022) 13:888713. doi: 10.3389/fimmu.2022.888713
14. Lin TY, Tsai MC, Tu W, Yeh HC, Wang SC, Huang SP, et al. Role of the NLRP3 inflammasome: Insights into cancer hallmarks. Front Immunol (2020) 11:610492. doi: 10.3389/fimmu.2020.610492
15. Zhivaki D, Kagan JC. NLRP3 inflammasomes that induce antitumor immunity. Trends Immunol (2021) 42:575–89. doi: 10.1016/j.it.2021.05.001
16. Chen MY, Ye XJ, He XH, Ouyang DY. The signaling pathways regulating NLRP3 inflammasome activation. Inflammation (2021) 44:1229–45. doi: 10.1007/s10753-021-01439-6
17. Decaillot FM, Kazmi MA, Lin Y, Ray-Saha S, Sakmar TP, Sachdev P. CXCR7/CXCR4 heterodimer constitutively recruits {beta}-arrestin to enhance cell migration. J Biol Chem (2011) 286:32188–7. doi: 10.1074/jbc.M111.277038
18. Boero E, Brinkman I, Juliet T, van Yperen E, van Strijp JAG, Rooijakkers SHM, et al. Use of flow cytometry to evaluate phagocytosis of staphylococcus aureus by human neutrophils. Front Immunol (2021) 12:635825. doi: 10.3389/fimmu.2021.635825
19. Peng L, Di XP, He SX, Zeng X, Shen H, Zhu HL, et al. Metabolic syndrome in women with and without interstitial cystitis/bladder pain syndrome. Int Urogynecol J (2021) 32:1299–306. doi: 10.1007/s00192-020-04605-w
20. Tsubota M, Miyamoto T, Hiruma S, Saeki H, Miyazaki T, Sekiguchi F, et al. Repeated cold stress reduces cyclophosphamide-induced Cystitis/Bladder pain and macrophage activity in mice. Pharmacology (2017) 99:286–90. doi: 10.1159/000461588
21. Moldwin RM, Nursey V, Yaskiv O, Dalvi S, Macdonald EJ, Funaro M, et al. Immune cell profiles of patients with interstitial cystitis/bladder pain syndrome. J Transl Med (2022) 20:97. doi: 10.1186/s12967-022-03236-7
22. Boutilier AJ, Elsawa SF. Macrophage polarization states in the tumor microenvironment. Int J Mol Sci (2021) 22:13. doi: 10.3390/ijms22136995
23. Vera PL, Iczkowski KA, Howard DJ, Jiang L, Meyer-Siegler KL. Antagonism of macrophage migration inhibitory factor decreases cyclophosphamide cystitis in mice. Neurourol Urodyn (2010) 29:1451–7. doi: 10.1002/nau.20878
24. Vera PL, Iczkowski KA, Wang X, Meyer-Siegler KL. Cyclophosphamide-induced cystitis increases bladder CXCR4 expression and CXCR4-macrophage migration inhibitory factor association. PloS One (2008) 3:e3898. doi: 10.1371/journal.pone.0003898
25. Lee KW, Kim WB, Lee SW, Kim JH, Kim JM, Kim YH, et al. Alterations of macrophage migration inhibitory factor expression in the nervous system of the rat cystitis model. Urol Int (2017) 98:228–35. doi: 10.1159/000456077
26. Zhou R, Tardivel A, Thorens B, Choi I, Tschopp J. Thioredoxin-interacting protein links oxidative stress to inflammasome activation. Nat Immunol (2010) 11:136–40. doi: 10.1038/ni.1831
27. Jose S, Groves NJ, Roper KE, Gordon R. Mechanisms of NLRP3 activation and pathology during neurodegeneration. Int J Biochem Cell Biol (2022) 151:106273. doi: 10.1016/j.biocel.2022.106273
28. Banerjee SK, Chatterjee A, Gupta S, Nagar A. Activation and regulation of NLRP3 by sterile and infectious insults. Front Immunol (2022) 13:896353. doi: 10.3389/fimmu.2022.896353
29. Chen X, Zhang D, Li Y, Wang W, Bei W, Guo J. NLRP3 inflammasome and IL-1beta pathway in type 2 diabetes and atherosclerosis: Friend or foe? Pharmacol Res (2021) 173:105885. doi: 10.1016/j.phrs.2021.105885
30. Sun X, Pang H, Li J, Luo S, Huang G, Li X, et al. The NLRP3 inflammasome and its role in T1DM. Front Immunol (2020) 11:1595. doi: 10.3389/fimmu.2020.01595
31. Yoshimura N, Chancellor MB. Interstitial cystitis and bladder research: Progress and future directions: Highlights of the national institute of diabetes and digestive and kidney diseases (NIDDK) and the interstitial cystitis association (ICA) international research symposium October 19-20, 2000, Minneapolis, MN. Rev Urol (2001) 3:146–51.
32. Bhadada S, Reddy KS, Bhansali A, Dutta P, Sridhar C, Khandelwal N. Co-Occurrence of emphysematous cystitis and emphysematous myositis in type 2 diabetes. J Assoc Phys India (2005) 53:821–3.
33. Erickson DR, Tomaszewski JE, Kunselman AR, Bentley CM, Peters KM, Rovner ES, et al. Do the national institute of diabetes and digestive and kidney diseases cystoscopic criteria associate with other clinical and objective features of interstitial cystitis? J Urol (2005) 173:93–7. doi: 10.1097/01.ju.0000146466.71311.ab
Keywords: inflammatory cystitis, diabetes, macrophages, inflammation, NOD-, LRR-and PYD domains-containing protein 3 (NLRP3)
Citation: Peng Y and Gao Y (2022) NLRP3+ macrophages aggravate inflammatory cystitis in diabetes. Front. Immunol. 13:1057746. doi: 10.3389/fimmu.2022.1057746
Received: 30 September 2022; Accepted: 18 October 2022;
Published: 03 November 2022.
Edited by:
Xiangwei Xiao, University of Pittsburgh, United StatesReviewed by:
Yinan Jiang, University of Pittsburgh, United StatesCopyright © 2022 Peng and Gao. This is an open-access article distributed under the terms of the Creative Commons Attribution License (CC BY). The use, distribution or reproduction in other forums is permitted, provided the original author(s) and the copyright owner(s) are credited and that the original publication in this journal is cited, in accordance with accepted academic practice. No use, distribution or reproduction is permitted which does not comply with these terms.
*Correspondence: Yubing Peng, cHlia3p0QDE2My5jb20=
Disclaimer: All claims expressed in this article are solely those of the authors and do not necessarily represent those of their affiliated organizations, or those of the publisher, the editors and the reviewers. Any product that may be evaluated in this article or claim that may be made by its manufacturer is not guaranteed or endorsed by the publisher.
Research integrity at Frontiers
Learn more about the work of our research integrity team to safeguard the quality of each article we publish.