- 1Shenzhen Institutes of Advanced Technology, Chinese Academy of Sciences (CAS), Shenzhen, China
- 2Department of Otolaryngology, Children’s Hospital of Chongqing Medical University, National Clinical Research Center for Child Health and Disorders, Ministry of Education Key Laboratory of Child Development and Disorders, Chongqing Key Laboratory of Translational Medical Research in Cognitive Development and Learning and Memory Disorders, Chongqing, China
The aryl hydrocarbon receptor (AhR) is a widely studied ligand-activated cytosolic transcriptional factor that has been associated with the initiation and progression of various diseases, including autoimmune diseases, cancers, metabolic syndromes, and allergies. Generally, AhR responds and binds to environmental toxins/ligands, dietary ligands, and allergens to regulate toxicological, biological, cellular responses. In a canonical signaling manner, activation of AhR is responsible for the increase in cytochrome P450 enzymes which help individuals to degrade and metabolize these environmental toxins and ligands. However, canonical signaling cannot be applied to all the effects mediated by AhR. Recent findings indicate that activation of AhR signaling also interacts with some non-canonical factors like Kruppel-like-factor-6 (KLF6) or estrogen-receptor-alpha (Erα) to affect the expression of downstream genes. Meanwhile, enormous research has been conducted to evaluate the effect of AhR signaling on innate and adaptive immunity. It has been shown that AhR exerts numerous effects on mast cells, B cells, macrophages, antigen-presenting cells (APCs), Th1/Th2 cell balance, Th17, and regulatory T cells, thus, playing a significant role in allergens-induced diseases. This review discussed how AhR mediates immune responses in allergic diseases. Meanwhile, we believe that understanding the role of AhR in immune responses will enhance our knowledge of AhR-mediated immune regulation in allergic diseases. Also, it will help researchers to understand the role of AhR in regulating immune responses in autoimmune diseases, cancers, metabolic syndromes, and infectious diseases.
Introduction
The aryl hydrocarbon receptor (AhR) belongs to the PAS (Per-ARNT-Sim), a superfamily of transcriptional factors widely found in numerous tissues throughout the body (1, 2). Genes belonging to PAS domains, typically AhR, enhance the environmental adaptations by sensing and detecting the environmental signals, including the redox potential or oxygen tension (HIF-1α, -2α, and -3α) and circadian rhythm (BMAL-1 and -2) (3). Besides, numerous chemicals and toxins, such as polycyclic aromatic hydrocarbons (PAHs) and Polyhalogenated aromatic hydrocarbons (PHAHs), affect the AhR-dependent responses. Among these, 2,3,7,8- tetrachlorodibenzo-[p]-dioxin (TCDD) is a PHAH toxin that is the most potent ligand for AhR (4). Upon activation, AhR alters the immunotoxicological outcomes and the transcription of AhR-targeted genes cytochrome P450 1A1 and cytochrome P450 1B1 (5, 6).
It was discussed that, upon activation, AhR regulates the transcription of multiple genes by translocating from the cytoplasm to the nucleus. However, recent studies imply that numerous physiologic ligands, e.g., intestinal microbiota, metabolites, and diet, interact with the host to influence AhR-dependent transcription. Identification and characterization of these natural ligands in AhR transgenic mice have demonstrated their pivotal role in AhR signaling (7).
As a transcriptional factor, AhR cooperates with various environmental factors, which act as agonists for AhR, and participates in the disease progression (7–9). AhR sensing and responding to environmental stimuli can play fundamental roles in cellular developmental processes and immune regulation (10). It has been well established that AhR plays a modulatory role in mediating the innate and adaptive immune response to combat various infectious diseases (11, 12), metabolic diseases (13–15), cancer (16, 17), and allergic diseases (18, 19). In the existing manuscript, we will review the role of AhR on the immune cells in response to environmental allergens.
Mode of action of AhR
AhR, a well-conserved protein, is ubiquitously expressed in mammalian organs with flexible expression levels amongst different tissues at different stages of life (20–22). Briefly, in an inactive state, AhR is present within the cell cytosol in the form of a protein complex. During activation after binding to its ligands, such as an allergen or environmental toxin, AhR undergoes conformational changes and translocates to the nucleus. After translocation, AhR triggers the transcription of numerous target genes through dioxin-responsive elements (DREs) (23, 24). Conversely, AhR prevents self-activation in a negative feedback loop by degrading AhR through AhR repressor (AhRR), which is a primary downstream target of AhR (23, 24). In addition to this canonical process, AhR also influences biological processes in a non-canonical way. In a non-canonical pathway, the cytoplasmic AhR complex is dissociated from its ligand-receptor complex. It results in the release of many biologically active molecules, including the c-SRC kinase, which leads to the phosphorylation of numerous genes (25). Remarkably, ligand-AhR acts as a facilitating protein during the formation of the ubiquitin ligase complex, which eases the degradation of steroid receptors, e.g., the central regulator of adipogenesis peroxisome proliferator-activated receptor γ (PPARγ) (26) or estrogen receptor (Erα) (7, 27), by enhancing the substrate specificity. The AhR-canonical and non-canonical signaling pathway is illustrated in Figure 1.
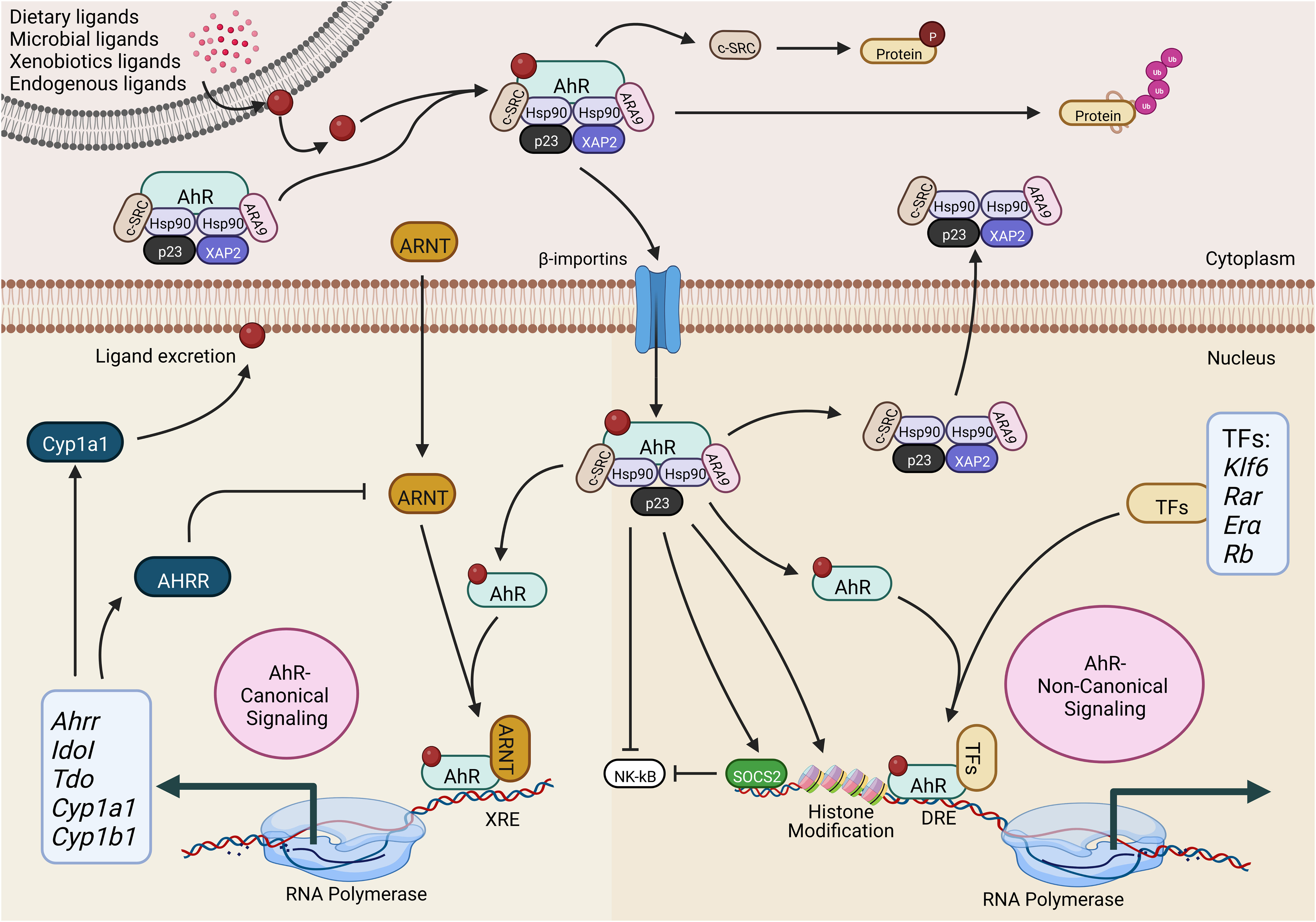
Figure 1 Activation of the aryl hydrocarbon receptor (AhR) canonical and non-canonical signaling pathway. AhR is present in all cell types in the form of a complex with Hsp90, ARA9, XAP2, p23 and c-SRC. Upon interaction with exogenous or endogenous ligands, AhR-complex undergoes conformational changes and uses importin β for the nucleocytoplasmic shuttling. In the AhR-canonical signaling pathway, AhR interacts with ARNT and binds to the XRE to regulate gene expression. In the AhR-non-canonical signaling pathway, AhR binds to various other transcription factors to regulate gene expression. AhR-aryl hydrocarbon receptor; ARNT-AhR nuclear translocator; AhRR-AhR repressor; CYP1A1, cytochrome P450 1A1; CYP1B1-cytochrome P450 1B1; c-SRC-proto-oncogene tyrosine-protein kinase Src; ARA9-immunophilin homolog ARA9; HSP90-heat shock protein of 90 kDa; p23-prostaglandin E synthase 3; XAP2-hepatitis B virus X-associated protein 2; IDOI-indoleamine 2,3-dioxygenase 1; TDO-tryptophan 2,3-dioxygenase; TF-transcription factor; KLF6-Krüppel-like factor 6; RAR-retinoic acid receptor; ERα-oestrogen receptor-alpha; Rb-retinoblastoma protein; SOCS2-suppressor of cytokine signaling 2; XRE-xenobiotic response element; DRE- Ub-ubiquitylation; P-phosphorylation. The illustration is drawn by BioRender (biorender.com).
Before exposure to the ligand in the cytoplasm, inactive AhR is present in the form of a protein complex that is comprised of the hepatitis B virus X-associated protein (XAP2), the chaperone heat-shock protein 90 (HSP-90), the co-chaperone p23 (p23), and the c-SRC protein kinase (21, 25, 28–31). Hsp90 protein keeps the right conformation of AhR for receptor bindings but also averts the AhR translocation to the nucleus (32). Conversely, the co-chaperone p23 is highly involved in stabilizing the interaction and complex of AhR-Hsp90, while the ARA9 elevates the AhR activation by properly folding AHR in the cytoplasm (33, 34).
Furthermore, HSP90 adjusts the AhR in the correct position to provide a high affinity for its ligands (32), while AhR steady-state cellular levels are maintained by AIP, which prevents AhR ubiquitination and degradation (35). The release of AIP from the complex exposes the nuclear signals of AhR. This leads to pivotal conformational changes in AhR; thus, it may lead to the nuclear translocation of AhR (36). On the other side, the nuclear translocation of AhR is also dependent on the phosphorylation of protein kinase C (37), establishing the fact that AhR is being controlled through several mechanisms. Additionally, regulating the nuclear translocation of AhR can be a potential therapeutic target for the precise reorientation of non-canonical AhR signaling.
During the activation of the canonical AhR signaling pathway in the presence of agonists, the ARA9-AhR-HSP90-p23 complex undergoes a conformational change which helps the translocation of this complex to the nucleus via β-importins (21, 25, 28–31). Several investigations suggest that XAP2 anchors the AhR complex in the cytoplasm. Other inquiries contend that XAP2 restricts the interaction of β-importins with nuclear localization signal. Importantly, prior to the nucleocytoplasmic shuttling, the release of XAP2 from the AhR complex is necessary (36, 38–40).
Further studies conducted in HeLa cells indicated that translocation of AhR to the nucleus could happen without being dissociated from HSP90 (38). Though, it’s unclear whether this finding can be generalized to various cell types or other AhR agonists (41, 42). Technically, AhR within the nucleus undergoes conformational changes by heterodimerizing with the AhR nuclear translocator (ARNT). Meanwhile, it also binds with particular DNA sequences, known as xenobiotics- or dioxin-response elements (XRE or DRE), to modulate the level of specific genes (29, 43–46). These genes contain numerous enzymes involved in xenobiotic metabolization, e.g., NAD(P)H-quinone oxidoreductase, CYP1A1, CYP1A2, and CYP1B1 (47).
In addition, AhR can control the expression of various genes directly by interacting with specific DNA sequences or indirectly by interacting with regulatory RNAs and transcription factors. These AhR-mediated regulations of gene expressions are generally achieved in an AhR-non-canonical signaling pathway manner. Practically, 5’-TNGCGTG-3’ is a DNA consensus motif located in the AhR target gene responsible for the interaction of AhR in the genomic regulatory regions. These DNA consensus motifs are recognized as DRE or XRE (48). Besides interacting with DREs or XREs, the AhR/ARNT complex also modulates the expression of various genes by interacting with various transcriptional factors. AhR exerts a significant effect during the chromatin remodeling during the interaction of AhR with chromatin-remodeling complexes, i.e., the steroid receptor coactivator-1 (SRC-1) (49), SWI/SNF (50), and by relocating the histone deacetylase (HDAC) complexes (51).
AhR has also been categorized to regulate the expression of a variety of downstream target genes by its interaction with their respective transcription factors, e.g., retinoblastoma protein (Rb), the estrogen receptor and E2F, the retinoic acid receptor, c-Maf, and NF-κB (52). AhR-dependent activity of these transcription factors is involved in mediating the numerous feedback loops which are extremely related to immune regulation. For instance, NF-κB elevates the AhR expression; however, AhR can alternatively modulate the NF-κB signaling (53–55).
Similarly, an alternative to canonical DREs, AhR can also be associated with numerous other transcription factors, which can help AhR to be directly recruited to the target DNA sequences. For instance, dimerization of AhR with RelA and RelB can direct the recruitment of AhR at the responding sites of NF-κB sites (56, 57) and with KLF6 (58). It suggests that non-canonical AhR signaling leads to the AhR recruitment to various non-consensus DNA elements. Strikingly, various ligands have been associated with enhancing the interaction of AhR with multiple genes (59). The recruitment of AhR at different target sites can subsequently alter the biological and cellular processes, including the immune responses in allergic diseases, cancers, and autoimmune diseases.
Allergic diseases
It is estimated that nearly 22% of the global population suffers from allergic disorders. These include eczema, allergic asthma, allergic rhinitis, drug or food allergic reactions, anaphylaxis, and allergic conjunctivitis (60, 61). Globally, allergic diseases are touching epidemic proportions with a particular increase among westerners. Since allergic diseases are highly associated with inflammation, various studies suggest a close association of allergic diseases with the onset of numerous complicated diseases, particularly metabolic diseases (62), cancer (63), mental disorders (64), stroke (65), and cardiovascular diseases (66). Similarly, allergic rhinitis and asthma are related to the high risk of obesity, insulin resistance, and blood sugar (67–70). These studies suggest that the pathogenesis of allergic diseases also can serve as an etiological factor for various other diseases. Therefore, we must improve our knowledge about allergic diseases and the impact of these allergic diseases on other associated diseases (71).
The current description of allergic diseases as abnormal conditions principally initiated in an immunoglobulin E (IgE)-dependent mechanisms is not well-applicable to all allergy patients (72). Typically, in allergic individuals, binding of IgE with primary allergens triggers allergic responses in ~50% of patients. In comparison, other non-primary allergens trigger an allergic reaction and activate various immune cells (73, 74). Therefore, it is necessary to determine the appropriate functions of immune cells in allergic inflammatory responses, so we can develop innovative, beneficial strategies to constrain allergic diseases.
Molecular mechanism of allergic diseases
Significant development has been made in understanding the intrinsic and structural biology of potential allergens and environmental agents (75). Allergic inflammatory reactions are specified through the assortment of the Th2-cell-dependent signaling pathway. This Th2-cell pathway begins upon the exposure of allergens and their uptake by the first line of defense antigen-presenting cells (APCs). APCs display the particular peptides/antigens to naïve T cells via MHC class II receptor. Thereby. Naïve T cells are directed towards the Th2-cell phenotype, which generally mediates the expression of secretory cytokine via transcription factor GATA3 (GATA-binding protein 3) (76). Meanwhile, Th1 phenotypic responses are generalized by the secretion of cytokines, i.e., interferon-γ (IFNγ) through the T-bet transcriptional factor. Overall, T cells play a leading role during the development and advancement of autoimmune, allergic, and cancerous diseases (Figure 2) (77).
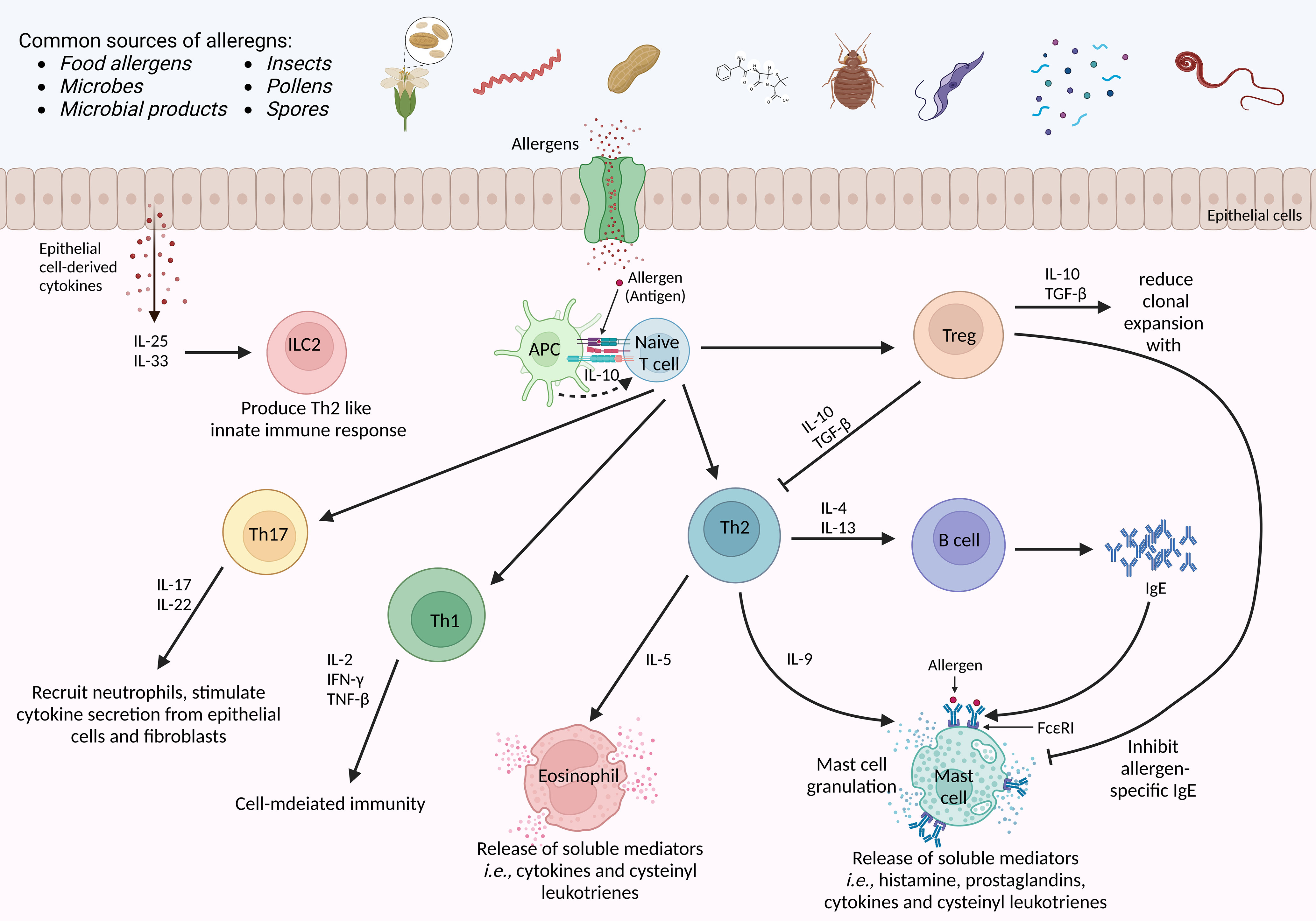
Figure 2 Molecular mechanism of an allergens-induced immune response. The host is exposed to multiple sources of allergens daily. Allergens-induced immune response begins when an allergen is presented to naïve T cells via antigen-presenting cells. Upon activation in the presence of first allergen exposure, naïve T cell is differentiated into Th2 cells, and the production of IgE by B cells. The IL-10 from APC favors the selective clonal expansion of Th2 cells, particularly the Th2 memory cells. in case of allergen re-exposure, crosslinking of antibody-loaded high-affinity IgE receptors is triggered on the surface of mast cells to provoke an acute-phase immune response. Subsequently, several pro-inflammatory mediators are secreted by Th2 cells, eosinophils, and mast cells in a late-phase reaction. Besides, Tregs suppress Th2-cell responses by secreting IL-10 and TGF-β. Meanwhile, Th1 secretes various cytokines to achieve cell-mediated immune responses, while Th17 recruits neutrophils and triggers epithelial cells and fibroblasts to secrete cytokines. IFNγ-interferon-γ, FceRI, the high-affinity receptor for IgE; TGF-β-transforming growth factor-beta; APC-antigen presenting cell; IL-interleukin. The illustration is drawn by BioRender (biorender.com).
Besides, it has been characterized that IgE binds to mast cells to activate mast cell degranulation and contributes to presenting the antigen to other immune cells. On the contrary, eosinophils yield some pro-inflammatory cytokines that can activate bronchial hyperreactivity and allergic inflammation (78, 79). Meanwhile, when APCs present potential antigens to naïve T cells via MHC-II, T cells are activated. Activated T cells coordinately elevate the secretion of a bunch of cytokines encoded by human chromosome 5q31–33. Briefly, activation of T cells encodes granulocyte/macrophage colony-stimulating factor (GM-CSF) and interleukin-3 (IL-3), IL-4, IL-5, IL-9, and IL-13 (80). These cytokines play crucial roles during allergic inflammation.
The Th2/Th1 imbalance during allergic responses has been established for more than two decades (81). However, recent investigations have discovered regulatory T (Tregs) cells as an additional crucial subset of CD4+ T cells, which is essential in mediating allergic disease (82). Increasing evidence from in-vivo allergic mice models sturdily associate Tregs with the suppression of allergen-specific responses and allergic inflammation (83). Moreover, numerous studies also stated that Tregs regulate the Th2-cell-mediated allergic responses by secreting various suppressive cytokines, including transforming growth factor-β (TGFβ) and IL-10, and urged that allergic reactions are consequent of Th2/Treg imbalance (84).
As the prevalence and morbidity rate of allergies is growing worldwide (85, 86), it is necessary to develop and design new treatment strategies that mask the symptoms of allergies and reduce the basic allergic cascade during allergic inflammation. As mentioned earlier, immune cell-mediated allergic responses are gaining importance in the scientific community. However, how immune cells mediate the immune responses to counter environmental nonpathogenic antigens, which progress to allergic reactions, is not well understood.
AhR in regulating immune responses in allergic diseases
Striking evidence indicates the extensive role of IgE and eosinophils in the pathogenesis of allergic inflammation and the development of therapeutic strategies targeting IgE and eosinophils (87). Nevertheless, the role of other immune cells in allergic reactions hasn’t been investigated well. Meanwhile, the role of AhR in regulating the inflammatory effects of environmental pollutants and allergens makes it an attractive target for achieving therapy against allergic diseases (88). In response to potential allergens and AhR ligands, the expression of AhR and the downstream signaling pathway exert various roles in different immune cells (Table 1). Therefore, it exhibits pleiotropic properties by participating and integrating with other signaling pathways, i.e., the Wnt-β catenin network and sex hormones receptors (101). The preliminary development of AhR-/- mice showed that AhR deficiency considerably reduced the lifespan, as indicated by frequent death after birth. Moreover, in the first few weeks of birth, AhR-/- mice also represented a compromised growth rate (102, 103). In addition, AhR-/- mice developed various hepatic abnormalities, such as abnormal retinoic acid metabolism, transient hepatic steatosis, and bile duct fibrosis (102–104). Similarly, an in-vivo model of imiquimod-induced psoriasis exhibits that, instead of hematopoietic cells, AHR in keratinocytes limits inflammation (105). Besides, an indole derivative IAId of microbiota-derived tryptophan metabolite exerts inverse effects on skin inflammation in atopic dermatitis patients by targeting AhR (106). It is known that AhR-deficient animals display high resistance to TCDD, which is an environmental pollutant. However, an in-depth examination of the immune responses was lacking (107). AhR regulates allergic diseases in both canonical and non-canonical pathways. It has been found that Di(2-ethylhexyl) phthalate (DEHP), a plasticizer, boosts the ovalbumin-induced allergic rhinitis by activating the AhR canonical pathway (108). However, a detailed understanding of the impact of the AhR-canonical signaling pathway on the AhR-non-canonical signaling pathway hasn’t been studied.
Emerging investigations have specified the profound influence of AhR signaling on host-pathogen and host-commensal interactions and in shaping the immune responses from various cell types. AhR is a critical player in mucosal interfaces where an individual interacts with environmental pollutants and other living entities (109, 110). As stated, the AhR might serve a considerably efficient role in mediating allergic immune responses by acting as an essential factor in regulating the functions of various immune system cells, together with B, T, and dendritic cells (18, 23, 111, 112). Briefly, AhR mediates mast cell differentiation and growth (90, 113), dendritic cell function (114), differentiation of type 1 regulatory T cell (Tr1) (115) and Th9 (116), intraepithelial lymphocytes functions (117), Treg intestinal homing (118) and impacts on γδ+ T cells homeostasis (119), affects the balance of Tregs and Th17 cells (120, 121), and lymphoid follicles (122). A brief overview of the role of AhR in immune cells has been shown in Figure 3. These findings suggest that AhR is critical for the progression of allergic inflammatory diseases and the development of allergic and inflammatory responses. However, the detailed mechanism of the impact of AhR in allergen-induced allergic inflammation and underlying mechanisms persist indefinably.
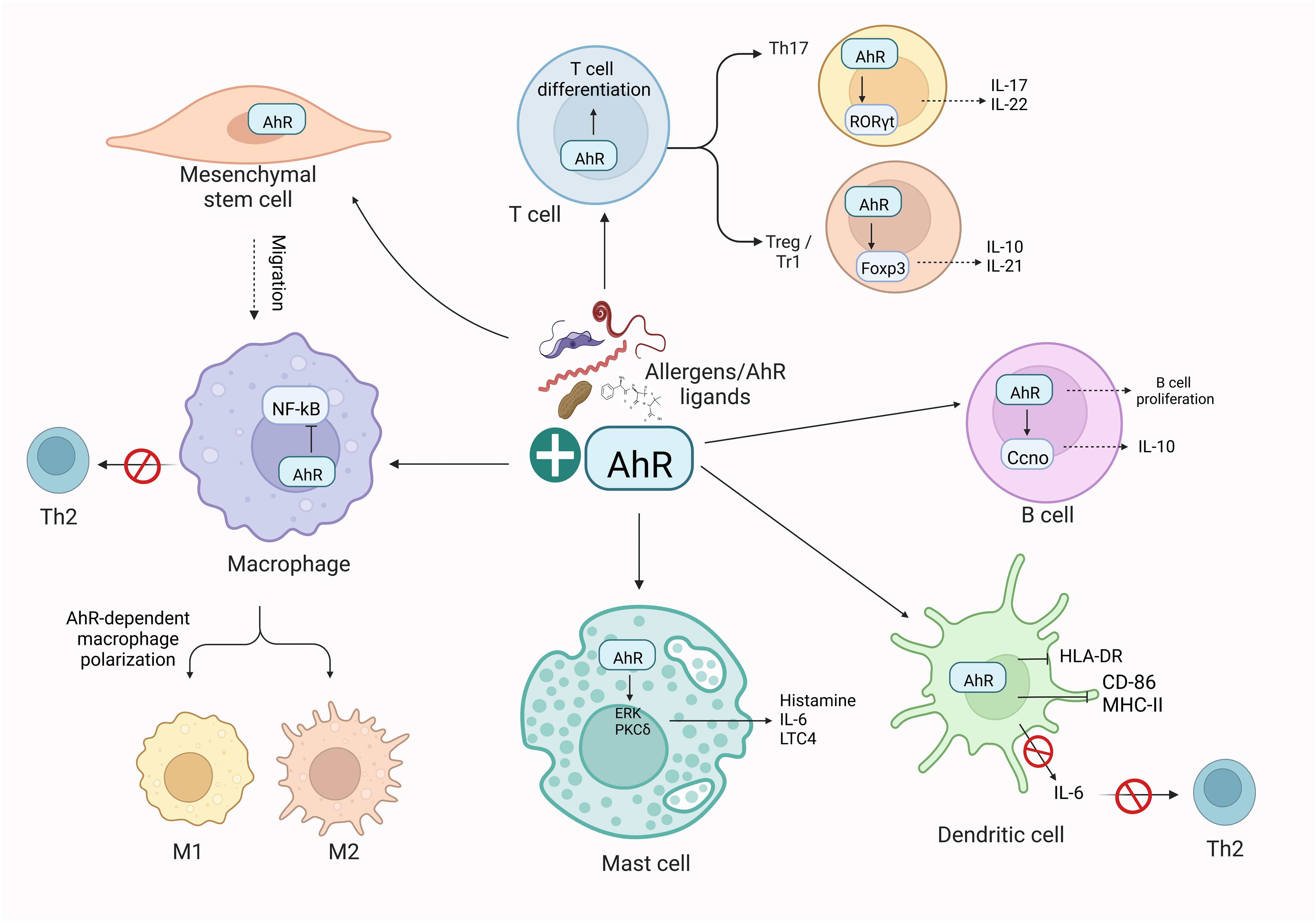
Figure 3 AhR-dependent regulation of immune cells. Activation of AhR in response to exogenous ligands or allergens influences the activity of adaptive and innate immune responses. Briefly, AhR affects the polarization of macrophages, the function of Th2 cells, T and B cell differentiation, and DC functions. These effects of AhR ultimately imbalance the M1/M2 polarization and Th17/Treg balance. The illustration is drawn by BioRender (biorender.com).
AhR in mast cells to regulate allergic diseases
As discussed earlier, mast cells are critical to exert adjuvant functions during exposure to allergens and immune sensitization in response to these allergens (123, 124). Generally, mast cell produces a broad variety of inflammatory factors to respond to environmental allergens and initiate immune responses (125). Activation of rat RBL2H3 mast cell line with AhR endogenous ligands, kynurenine and kynurenic acid, elevates the level of IL-6, a well-known AhR downstream target, in a calcium-dependent manner (126). Later, the expression of AhR was reported in the rat RBL2H3 mast cell line (127) and the mouse and human mast cells (90, 91). Afterward, several lines of evidence indicate the role of AhR in the immune regulation of mast cells. Ligation of AhR with its ligand 6-formylindolo-[3,2-b]-carbazole (FICZ) potentiate the classical IgE/Ag-dependent response by elevating the secretion of pro-inflammatory cytokines IL-6 and IL-13, LTC4 and histamine, through the activation of PKCδ and ERK (90, 91). On the other hand, AhR activation also induces the production of reactive oxygen species (ROS) in response to AhR activation through mast cells to impact mast cell-dependent inflammatory responses (128). In association with this, chronic rhinosinusitis with nasal polyps (CRSwNP) was found to be associated with mast cell-dependent oxidant stress and inflammation. Mast cell-specific absence of AhR, predominantly found in the mast cells of nasal polyps, reduced ROS production and the expression of oxidized calmodulin-dependent protein kinase II (ox-CaMKII). This suggests the role of AhR in regulating the activation of mast cell by altering the levels of ox-CaMKII and ROS in CRSwNP patients (129). Meanwhile, genetic ablation of AhR in mast cells impairs the production of IL-13 and elevates mitochondrial damage; thus, it participates in the homeostasis and maturation of mast cells (90).
Interestingly, the response of mast cells is highly dependent on the duration of FICZ exposure. It was noted that a single dose of FICZ improved the activation characteristics of mast cells in terms of secretion of pro-inflammatory IL-6 and histamine. In contrast, extended exposure to FICZ boosted IL-17 production and decreased degranulation (91). These IL17+ mast cells were also found in the lung parenchyma of patients with chronic obstructive pulmonary disease (COPD) (91). ORM1 (yeast)-like protein 3 (ORMDL3), localized in the endoplasmic reticulum (ER), is pivotal in allergic asthma (130). A recent study illustrates that AhR ligand, PAH, activates sphingosine-1-phosphate S1P production, and decreases the activity of S1P lyase (S1PL) in resting immune cells, IgE/antigen-activated mast cells. This decreased activity of S1PL is reversed by silencing ORMDL3 or adding an antioxidant, thereby suggesting that the AhR-ligand axis regulates the time-dependent upregulation of ORMDL3/S1PL complex, particularly in allergic diseases (131). Meanwhile, cow/goat milk containing a protein family of lipocalins or secretoglobins acts as potential respiratory allergens (132). In another research, beta-lactoglobulin (BLG), a bovine lipocalin in milk, acts as an allergen and primes human mast cells for degranulation by facilitating the quercetin-dependent AhR activation and influencing the expression of AhR-downstream Cyp1A1 in the lung (133). Conclusively, increasing data reveal the fundamental involvement of mast cells in normal and allergic disease conditions. Mast cells affect the host in allergic diseases and participate in the deregulatory immune response.
AhR in dendritic cells to regulate allergic diseases
In response to allergens, DCs are crucial in inducing allergic sensitization or tolerance (134). As DCs are present and dispersed across the body, their localization is firmly expressed by their functions as APCs. Immature DCs exist in the place where the entry of the first antigen is projected, such as the urogenital system, upper and lower airways, and the epithelium of skin and gut mucosa. In the same way as other antigens, allergens cross cellular barriers to interact with DCs (134). These cells move to lymphoid organs and transmit antigens to immature T cells. Either the stimulation of Foxp3+ regulatory T (Treg) cells or the induction of T helper 2 (Th2) cells would cause allergic sensitization (135).
Skin-resident specialized DCs can swiftly detect and sample antigens to determine whether they constitute a health risk. Langerhans cells (LCs) are dendritic cells found in the skin. Epidermal LCs were identified as DCs when C3, Fc, and MHC Class II receptors were found on them, and they have been the subject of in-depth research ever since (136, 137). In the mammalian dermis, however, new DC subpopulations have also been found (138).
The level of many co-stimulatory molecules, including CD54, CD80, and CD86, indicates that epidermal LCs interact with allergens as soon as they penetrate the skin barrier and get activated. The secretion of pro-inflammatory cytokines, such as IL-1, IL-12, and TNF-α is also stimulated concurrently (139). According to animal studies, this inflammatory process is followed by the infiltration and buildup of moDC in tissues. The murine DC markers (CD11c, CD80, CD86, MHC II, and DEC205) as well as the macrophage-associated markers (Mac-3, F4/80), and monocyte (CD11b, Ly6C) are expressed by these cells (140).
CD1a+CD11b+CD1c+ plasmacytoid and myeloid DCs have been seen in the skin of people with atopic dermatitis (141). FcR1, a highly affinitive IgE receptor, is expressed by both groups (142–144). Because oral sensitivity to peanuts is associated with an elevated inflammatory CD11b+ DCs and a reduction in gut resident CD103+ DCs, it is crucial for patients with food allergy to maintain a balance between tolerogenic CD103+ DCs and inflammatory CD11b+ DCs (145). Mucosal CD103+ DCs have also been found to stimulate Foxp3+ regulatory T (Treg) cells through TGF-β1 and retinoic acid, which makes them crucial in the development of oral tolerance (146–148). Tregs control the strength of immunological responses and are crucial in controlling allergic sensitization (149).
Lung APCs, which get exposure to exogenous and endogenous AhR ligands during the asymptomatic sensitization phase of the asthmatic response, take up and process the allergen. The subsequent synthesis of Th2 cytokines and activation of B cells that develop into allergen-specific IgE-production is triggered by DCs-presentation of allergen to naïve T cells. These IgE antibodies attach to basophils and mast cells that express high IgE affinity receptors in anticipation of future allergen exposure. Both macrophages and DCs exhibit AhR expression, and new research indicate that AhR functions upstream of DC by steering the destiny of human monocytes toward DC rather than macrophages. This suggests that AhR activation may favor DC-dependent processes (150). Interestingly, mesenteric lymph node (MLN) CD103+ DCs elevate AhRR upon TCDD-dependent in vivo activation of AhR (151). However, limited information related to the AhR gene is available in association with DCs subsets (152).
AhR is extensively expressed in cDCs (101) and is crucial to control these cells. Multiple tolerogenic processes are activated in DCs by genomic and non-genomic AhR signaling, which modifies the production of cytokines essential for effector and Treg development (153). It has also been demonstrated that AhR signaling in DCs regulates the expression of metabolites with significant immunoregulatory roles. For instance, IDO, an enzyme that catalyzes the formation of the Trp-derived metabolite kynurenine, is driven by AhR and IL-6, IFN-γ, TNF-α, and IL-1β (154). It’s interesting to note that kynurenine is an AHR AhR agonist, promoting FoxP3+ Treg differentiation and reducing inflammation in various situations (114, 155). Interestingly, plant-derived indole-3-carbinol enhances CD11c+ and CD103+MHC-II+ cells in the lamina propria of mice induced with peanut allergy (100).
In vitro maturation of AhR-deficient LCs did not raise the expression of co-stimulatory markers CD40, CD80, and CD24a, and had a greater phagocytic capability. It’s interesting to note that AhR-deficient LC had much lower levels of tolerogenic Ido mRNA expression, and bone marrow-derived dendritic cells could not be activated by AhR (156). The generation of tolerogenic splenic CD103+ DCs and the ultimate activation of Treg cells are linked to in vivo AhR upregulation. Increase in Treg and the inhibition of the inflammatory response are thought to be caused by an upsurge in retinoic acid synthesis by DCs and/or an elevation in the IDO caused by AhR activation (153, 155, 157). Altogether, these studies demonstrate how AhR signaling may activate transcriptional pathways relevant to DC, making it an attractive option for medical approaches.
AhR in macrophages to regulate allergic diseases
Macrophages are crucial in establishing adaptive immunity. These cells respond to various environmental signals and aid the development of various allergic, autoimmune, infectious, and non-infectious inflammatory diseases (158). Upon exposure to allergens, macrophages attain two primary phenotypes: the classical inflammatory M1 phenotype and the alternative anti-inflammatory M2 phenotype (159). M1 phenotype is typically activated by IFN-γ and lipopolysaccharide (LPS), which ultimately elevate the expression of inflammatory genes helpful for the clearance of intracellular pathogens. Alternatively, the M2 phenotype is activated by IL-4 and IL-13, which enhance the expression of anti-inflammatory genes involved in wound healing (160). Elevated polarization of M2 macrophage was determined in various allergic diseases, including skin allergies and allergic asthma (161–163).
Activation of M1 macrophages through LPS elevates the expression of AhR in macrophages through the NF-κB pathway (164). The loss of AhR in macrophages diminishes the IL-10 secretion in LPS-activated macrophages. Mechanistically, AhR was found to be an essential component for the secretion of IL-10 and the phosphorylation of STAT3 through Src during inflammatory macrophage-dependent immune regulation (164). Besides, both exogenous (BaP) and endogenous (FICZ) AhR ligands can enhance the Rac1 ubiquitination, which takes part in the activation of macrophages through PI3K/AKT-pathway, ensuing in a pro-inflammatory macrophage phenotype (165). Meanwhile, AhR has been known to impact the M1/M2 macrophage polarization by influencing arginine and nitric oxide production. It was determined that macrophage-specific depletion of AhR accelerates the secretion of pro-inflammatory cytokines upon stimulation with LPS, affecting the balance between M1 and M2 macrophages (166). Similarly, ablation of AhR in tumor-associated macrophages led macrophages towards a pro-inflammatory phenotype which ultimately raised the population of intra-tumoral IFNγ secreting CD8+ T cells (167). Another study illustrated that AhR exerts a negative role in the differentiation of monocytes and bone marrow-derived macrophages (150, 168). It was established that the activation of AhR downregulates the differentiation of macrophages by influencing the AhR-miR-142a-IRF1/HIF-1α axis to mitigate M1 macrophage polarization and expand M2 macrophage polarization (169).
In systemic lupus erythematosus, the expression of AhR is increased on the surface of macrophages susceptible to apoptotic cells, which eventually promotes the secretion of immunosuppressive cytokine IL-10; thus, limits the pathogenesis of SLE in-vivo (170). Meanwhile, in the cockroach extract (CRE)-induced asthma mouse model, it was estimated that macrophages polarized from mesenchymal stem cells shift pro-inflammatory M1 toward an anti-inflammatory M2 macrophage which was highly dependent on the AhR signaling. However, the AhR antagonist (CH223191) decreased the M2 polarization and boosted M1 polarization in mice challenged with cockroach allergens (96). Overall, it can be recommended that AhR is decisive in the polarization of M1 and M2 macrophages.
AhR in B cells to regulate allergic diseases
Antigen-specific B-cell clones are activated and differentiated in response to the particular antigen when interacting with a mature naive B cell or with T cells. This happens in phases, with each stage corresponding to a modification in the genome level at the antibody loci, eventually leading to the development of memory B cells, effector cells (plasma cells), and antibody production (humoral immunity) (171). The AhR level in the various cells entangled in the pathogenesis of allergy disorders has been previously demonstrated. The IL-4 and IL-13 secretion stimulates B cells to transform them into plasma cells, producing allergen-specific IgE that binds to high-affinity IgE receptors on the mast cells. The allergen representation does the Th2 activation to naïve T cells through APCs (19). For sensitization and the emergence of the instant bronchospastic response by IgE production, the humoral response’s development in asthma is crucial.
B cells express AhR, and a prior investigation found that the AhR agonist [4-(3-chloro-phenyl)-pyrimidin-2-yl]-[4-trifluoromethyl-phenyl]-amine (VAF347)] suppresses the production of IgE by B cells (172). The AhR functions in B cells have recently been reexamined. It has been discovered to control the destiny decisions of B cells by favoring memory B cells which suppress the terminally developed antibody-secreting plasma cells (173). Additionally, the activation of the Ahr is activated by microbiota-derived butyrate, which helps in the accumulation of Il-10-secreting regulatory B cells (Bregs) (174, 175).
By inhibiting IgG and IgM production and reducing B cell development into Ig-secreting cells, TCDD inhibits humoral immune responses (176). The direct impacts of TCDD on the growth of B cells have recently been evaluated by Sulentic et al. (177). In a nutshell, TCDD interferes with B cell-dependent antibody production and their proliferation at various phases of B cell maturation and differentiation. B cells are particularly vulnerable to AhR-ligand TCDD, particularly during their activation phase, which will ultimately be low vulnerable as cells move closer to terminal differentiation. B cell activation produces a significant level of AhR.
Additionally, it’s likely that TCDD influences the transcription of various genes in B cells AhR-dependent manner and causes various biochemical modifications which can be unrelated to AhR-mediated gene transcription (177). Aside from these direct impacts on B cells, AhR stimulation can adversely impact the function and the activation activity of B cells on the Th cells and Th-secreted cytokines. As a result, we urge that AhR is highly engaged in controlling B cells, and targeting AhR can promote memory responses.
AhR regulating T cells in allergic diseases
Airway hyperresponsiveness, eosinophil recruitment, mucus hypersecretion, and the secretion of the abovementioned mediators contribute to the initiation and progression of the allergen-specific inflammatory reaction (178, 179). The unique asthmatic reaction player from the innate lymphoid cell family (ILC) has more recently been identified as critical for allergic reactions (180). ILCs are mostly found in mucosal regions, lack antigen receptor expression, and react to cytokines in their surroundings. Innate equivalents of Th1, Th2, and Th17/Th22 cells are type 1, 2 and 3 ILCs, respectively, and their cytokine pattern is similar to that of conventional T helper cell populations. ILC2 participates in both experimental and clinical allergies by generating airway hyperresponsiveness, mucus hyperproduction and lung eosinophilia (180). ILC2 also generates IL-5 and -13 after stimulation by TSLP, IL-25, or -33. Lung ILC3 has only previously been discovered in a few experimental asthma types, particularly those that are linked to obesity (181, 182). When AhR activity is increased, the ILC2 function is suppressed, whereas ILC3 function is promoted (111, 183, 184). This suggests that AhR favors the generation of Th17-type cytokines by subtly counter-regulating ILC subsets, with the best in the gut. Besides the fact that AhR is abundantly found in ILC2 and ILC3 of the gut, no research has yet looked at how AhR ligands with allergens cause Th17-type responses, even though several studies have demonstrated this.
While the induction of Treg is considered advantageous, some kinds of asthma have a high Th17 profile that may be a factor in their severity. Th17 cells are a well-known immune regulator subgroup of CD4+ T cells that secrete the cytokines interleukin (IL)-17 (also known as IL-17A), IL-17F, and IL-22. AhR activation promotes T helper (Th)17 cell development, which worsens in vivo Th17 cell-mediated autoimmunity (such as EAE) (120). In a model of the ovalbumin-induced allergic disease model, it was studied that AhR regulates the differentiation of T cells by dendritic cells (93). The levels of AhR expression vary among the T cell subsets, with Th17 and Treg cells expressing the most AhR and Th1 and Th2 cells the least (185). It was undermined that indoxyl 3-sulfate (I3S) acts as AhR ligand and regulates the differentiation of Th2 cells in the ovalbumin-sensitized allergic asthma model (92). The AhR also modulates the production of cytokines by Th2 cells. 4-nonylphenol Indeno[1,2,3-cd]pyrene is a prominent air pollutant that increases the secretion of cytokines from Th2 cells in an ovalbumin-induced allergic asthma model (94, 95). AhR became a major player in the control of T cell biology in the 2000s. It has been demonstrated that AhR is increased during the maturation of Th17 cells, which increases the synthesis of IL-17 and IL-22 in mice (120, 121, 186) and IL-22 in humans (187, 188). As a result, Th17 cell production of IL-22 is reduced in AhR-deficient animals (120). However, AhR ligands may exert different effects on the Th17-mediated allergic response outcomes through FICZ as favoring and TCDD as inhibiting this profile (120, 121). During Th17 development, signal transducer and transcription 3 are activated, which causes AhR expression to increase (115). AhR also inhibits Stat5 and Stat1 signaling, which would otherwise prevent Th17 formation and create a positive feedback loop (186). It was also found that urban dust particle (SRM1649b) enhances Th17 polarization (97). Thus, AhR could contribute to the early phases of Th17 differentiation, but additional elements are necessary for the growth of fully pathogenic effector Th17 cells (189).
AhR activation has also been connected to type 1 T regulatory cells (Tr1) cells (secreting IL-10) and Foxp3 expressing Tregs (190, 191). AhR ligands like TCDD or kynurenine boost Foxp3 expression by a variety of methods, including epigenetic changes in T cells and regulation of DC, in contrast to FICZ, which encourages differentiation of Th17 cells in both non-eosinophilic asthma model and cockroach-sensitized mouse model (89, 98, 99, 155, 192). These epigenetic T cell changes, particularly DNA methylation, promote plasticity and flexibility among CD4+ T cells and enable differentiation of various subpopulations (193). Increased FOXP3 methylations in peripheral Treg cells in asthma patients have been more linked to their impaired functions in a high ambient air pollution environment than asthmatics in an environment with low air pollution (194). It is noteworthy that, other than TCDD, the non-dioxin-like AhR ligands, such as FICZ, β-NF, and 6-MCDF, exert no significant effect on the Treg in the peanut-induced allergy model (99).
Alternatively, Il-27, known as a cytokine with pleiotropic activity while performing various immune regulatory actions, has been shown to increase Tr1 cells’ AhR expression through a process mediated by Stat3 (195). The immunosuppressive cytokine IL-10 is secreted by Tr1 cells because of this activation-dependent effect on their transcriptional program. It is notable that activin-A, one of the members of the TGF-family, can stimulate the level of AhR and IRF4 transcription factors in human CD4+ T cells, resulting in the development of Tr1-like cells capable of suppressing the reactions to allergens in a mouse model (196). It is interesting to note that AhR can change mature Th17 cells into Tr1 cells that produce IL-10 (197), a mechanism evident in allergic rhinitis (198). Therefore, it is worth associating the AhR with the plasticity of Th17 cells that need stimulus to differentiate into regulatory Tr1 cells or pathogenic Th17 cells.
AhR ligands encourage the production of Foxp3 Tr1-like cells on human CD4+ T cells, which inhibit through granzyme B and produce IL-10. Additionally, AhR ligands, in the presence of TGF-β1, activate the Foxp3+ Treg, which suppresses by activating CD39, an enzyme that hydrolyzes ATP to AMP. SMAD1 is induced by TGF-β1 and AhR ligands in a mechanism that encourages Foxp3 expression by binding to the Foxp3 enhancer. Aiolos, which binds to Foxp3 and inhibits IL-2 production, is also produced to counter the AhR ligands and TGF-β1 (191, 199). AhR is also implicated in the trans-differentiation of Th17 to Tr1 since AhR agonists facilitate this process (197). AhR modulates Tr1 cell metabolism in the late phases when Hif-1 is no longer expressed (115). Interestingly, AhR and TGF-β1 form a functional axis that advances the allergic airway inflammation induced by cockroach allergens (200).
Of note, AhR is indispensable for developing Th17 and Tr1 cells along with the IL-6-dependent Th22 cells, which secrete IL-22 and function as a defense against enteropathogenic bacteria (201, 202). Meanwhile, besides the role of AhR in the differentiation of CD4+ cells, it has been evident that AhR also alters the CD8+ T cell responses in an antigen-specific manner. AhR deficiency disrupts the primary CD8+ cell responses in response to influenza virus-related infections in a cell-extrinsic way (203). Meanwhile, it has been noted that during the silencing of AhR, CD8+ T cells with antigen-specificity can display altered patterns of DNA methylation and an aberrant transcriptional profile suggesting the presence of exhausted CD8 T cells (204). The retention and/or survival of tissue-resident memory CD8 T lymphocytes in the skin is also regulated by AhR (205).
The role of AhR in γδT cell biology has also been well established. It was illustrated that IL-17-secreting innate-like γδ T cells (206, 207) increase the level of AhR, which ultimately play a pivotal role in the production of IL-22 by γδ T cells (208). Similarly, AhR signaling also plays a crucial role in maintaining the population of skin-resident Vγ3+ γδ T cells, also recognized as Dendritic Epidermal γδ T cells (DECT), which originate from thymic precursors and move to the skin during early stages of life (117, 119). Moreover, for the survival and maintenance of both CD8αα+ TCRαβ+ cells and Vγ5+ γδ T cells, intraepithelial lymphocytes in small intestinal LP, the presence of AhR ligands in the food is an essential factor (117). Together, these investigations support the notion that AhR in T cell subsets continuously interacts with the environmental cues at the mucosal level and responds to the microbial products.
Conclusion
In conclusion, AhR controls the innate and adaptive response at a variety of levels, with consequences that are expected to vary depending on ancestry and the results of the asthmatic reaction. These results demonstrate positive and negative impacts on the allergens-specific response when using gene silencing, knockout mice, or AhR antagonists. Even though AhR was cloned more than 20 years ago, there is still much we don’t know about how it works in health and diseases. Recent research amply supports the notion that AhR plays a critical function in immune responses far beyond the mere identification of contaminants. However, the AhR crystal structure is still a mystery, and nothing is known about how nutritional, environmental, bacterial, and endogenous ligands interact with one another to influence AhR signaling.
The molecular dissection for the deep understanding of AhR signaling has focused mainly on AhR-canonical signaling, which generally represents the association of exposure to environmental pollutants with the diverse pathophysiological effects in an AhR-dependent manner. This canonical signaling is associated with the AhR heterodimerization with ARNT and XREs. It has been demonstrated that the Th1/Th2 cell balance, regulatory T cells, and dendritic cells are among the immune system cells that are impacted by the activation of the AhR in response to dioxins. Due to their involvement in the development of either tolerance or allergic sensitization, these cells, as previously mentioned, play a significant part in numerous allergies. Activating the AhR by dioxin-like substances has recently been demonstrated to decrease allergy sensitization by reducing the absolute number of precursor and effector T cells, maintaining CD4+CD25+Foxp3+ Treg cells, and altering DCs and their interaction with effector T cells in peanut allergy model (18, 209). However, in several diseases, AhR interacts with and influences numerous other genes, including Erα and NF-κB, in a non-canonical pathway. Our unpublished data shows CCl4, which is not a ligand for AhR and induces hepatic inflammation, also influences the accumulation of immune cells in the inflamed liver and decreases the hepatic disease severity after the knockout AhR in Tregs. Additionally, a previous study also suggests that binding of AhR with Erα exhibits higher affinity compared to ARNT, and alterations of AhR signaling significantly influence the functions of Tregs in autoimmune hepatitis (210). Thus, blocking the AhRR and Erα can potentially reestablish immune homeostasis in autoimmune diseases and other immune-mediated diseases.
Several lines of research suggest that the AhR pathway may be a valuable target for diseases, e.g., multiple sclerosis, inflammatory bowel disorders, psoriasis, cancer, and stem cell transplantation, although caution is still advised. Most likely, to get the intended outcomes, AhR activation has to be strictly regulated. For instance, proper wound healing and defense against bacterial infections are controlled by AhR-dependent IL-22 secretion from innate and adaptive cells. However, IL-22 production that is persistent and dysregulated develops into a pathogen and causes colitis and cancer (211, 212).
We have discussed that AhR is pivotal in modulating the effects on the immune response. As AhR interacts with various exogenous ligands, it serves as a potential target for numerous small molecules for the therapeutic intervention. It was reported that tapinarof and laquinimod could significantly target the AhR to treat multiple sclerosis, atopic dermatitis, and psoriasis (213–215). Similarly, metformin, which acts as an anti-diabetic drug, can also influence the activity of AhR in mast cells to treat allergic diseases (216). Similar tight regulation is probably crucial in many biological processes that AhR regulates. However, the presence of AhR in numerous tissues and cell types is challenging to treat AhR signaling pathway. Therefore, cell-specific delivery should also be considered to target this pathway. For instance, targeted delivery of chemically modified Foxp3 mRNA to sites of inflammation in the house dust mite-induced allergic asthma served as a safe and efficient therapeutic tool by regulating T cell immune responses (217). Overall, it appears that AhR pathway is activated through numerous signals from several sources to ensure that the host responds accurately and adapts to ongoing environmental changes and allergens, a step crucial to adaptation.
Author contributions
FR, FP, WP outlined the manuscript,FR drafted it. FP and PW reviewed and improved the rigorousness of the manuscript. FP and PW supervised the study. All authors contributed to the article and approved the submitted version.
Funding
FP is supported by the National Key R&D Program of China (2021YFC2400500), the Shenzhen Science and Technology Program (KQTD20210811090115019), the National Natural Science Foundation of China (Grant 32170925), and the start-up fund of SIAT, CAS. PW is supported by Natural Science Foundation of Chongqing Grant CSTB2022NSCQ-MSX1069, Entrepreneurship and Innovation Support Program of Chongqing for overseas Scholars Grant CX2022118.
Conflict of interest
The authors declare that the research was conducted in the absence of any commercial or financial relationships that could be construed as a potential conflict of interest.
Publisher’s note
All claims expressed in this article are solely those of the authors and do not necessarily represent those of their affiliated organizations, or those of the publisher, the editors and the reviewers. Any product that may be evaluated in this article, or claim that may be made by its manufacturer, is not guaranteed or endorsed by the publisher.
References
1. Gu YZ, Hogenesch JB, Bradfield CA. The PAS superfamily: sensors of environmental and developmental signals. Annu Rev Pharmacol Toxicol (2000) 40:519–61. doi: 10.1146/annurev.pharmtox.40.1.519
2. Frericks M, Meissner M, Esser C. Microarray analysis of the AHR system: tissue-specific flexibility in signal and target genes. Toxicol Appl Pharmacol (2007) 220(3):320–32. doi: 10.1016/j.taap.2007.01.014
3. Kewley RJ, Whitelaw ML, Chapman-Smith A. The mammalian basic helix-loop-helix/PAS family of transcriptional regulators. Int J Biochem Cell Biol (2004) 36(2):189–204. doi: 10.1016/S1357-2725(03)00211-5
4. Denison MS, Nagy SR. Activation of the aryl hydrocarbon receptor by structurally diverse exogenous and endogenous chemicals. Annu Rev Pharmacol Toxicol (2003) 43:309–34. doi: 10.1146/annurev.pharmtox.43.100901.135828
5. Manners S, Alam R, Schwartz DA, Gorska MM. A mouse model links asthma susceptibility to prenatal exposure to diesel exhaust. J Allergy Clin Immunol (2014) 134(1):63–72. doi: 10.1016/j.jaci.2013.10.047
6. Stevens EA, Mezrich JD, Bradfield CA. The aryl hydrocarbon receptor: a perspective on potential roles in the immune system. Immunology (2009) 127(3):299–311. doi: 10.1111/j.1365-2567.2009.03054.x
7. Gutiérrez-Vázquez C, Quintana FJ. Regulation of the immune response by the aryl hydrocarbon receptor. Immunity (2018) 48(1):19–33. doi: 10.1016/j.immuni.2017.12.012
8. Nguyen LP, Bradfield CA. The search for endogenous activators of the aryl hydrocarbon receptor. Chem Res Toxicol (2008) 21(1):102–16. doi: 10.1021/tx7001965
9. Rothhammer V, Quintana FJ. The aryl hydrocarbon receptor: an environmental sensor integrating immune responses in health and disease. Nat Rev Immunol (2019) 19(3):184–97. doi: 10.1038/s41577-019-0125-8
10. Quintana FJ, Sherr DH. Aryl hydrocarbon receptor control of adaptive immunity. Pharmacol Rev (2013) 65(4):1148–61. doi: 10.1124/pr.113.007823
11. Torti MF, Giovannoni F, Quintana FJ, García CC. The aryl hydrocarbon receptor as a modulator of anti-viral immunity. Front Immunol (2021) 12:624293. doi: 10.3389/fimmu.2021.624293
12. Lawrence BP, Vorderstrasse BA. New insights into the aryl hydrocarbon receptor as a modulator of host responses to infection. Semin Immunopathol (2013) 35(6):615–26. doi: 10.1007/s00281-013-0395-3
13. Girer NG, Tomlinson CR, Elferink CJ. The aryl hydrocarbon receptor in energy balance: The road from dioxin-induced wasting syndrome to combating obesity with ahr ligands. Int J Mol Sci (2020) 22(1):49. doi: 10.3390/ijms22010049
14. Lin YH, Luck H, Khan S, Schneeberger PHH, Tsai S, Clemente-Casares X, et al. Aryl hydrocarbon receptor agonist indigo protects against obesity-related insulin resistance through modulation of intestinal and metabolic tissue immunity. Int J Obes (Lond) (2019) 43(12):2407–21. doi: 10.1038/s41366-019-0340-1
15. Wang B, Zhou Z, Li L. Gut microbiota regulation of AHR signaling in liver disease. Biomolecules (2022) 12(9):1244. doi: 10.3390/biom12091244
16. Perepechaeva ML, Grishanova AY. The role of aryl hydrocarbon receptor (AhR) in brain tumors. Int J Mol Sci (2020) 21(8):2863. doi: 10.3390/ijms21082863
17. Xue P, Fu J, Zhou Y. The aryl hydrocarbon receptor and tumor immunity. Front Immunol (2018) 9:286. doi: 10.3389/fimmu.2018.00286
18. Schulz VJ, Smit JJ, Pieters RH. The aryl hydrocarbon receptor and food allergy. Vet Q (2013) 33(2):94–107. doi: 10.1080/01652176.2013.804229
19. Poulain-Godefroy O, Bouté M, Carrard J, Alvarez-Simon D, Tsicopoulos A, de Nadai P. The aryl hydrocarbon receptor in asthma: Friend or foe? Int J Mol Sci (2020) 21(22):8797. doi: 10.3390/ijms21228797
20. Yi T, Wang J, Zhu K, Tang Y, Huang S, Shui X, et al. Aryl hydrocarbon receptor: A new player of pathogenesis and therapy in cardiovascular diseases. BioMed Res Int 2018 (2018) p:6058784.
21. Harper PA, Riddick DS, Okey AB. Regulating the regulator: factors that control levels and activity of the aryl hydrocarbon receptor. Biochem Pharmacol (2006) 72(3):267–79. doi: 10.1016/j.bcp.2006.01.007
22. Yamamoto J, Ihara K, Nakayama H, Hikino S, Satoh K, Kubo N, et al. Characteristic expression of aryl hydrocarbon receptor repressor gene in human tissues: organ-specific distribution and variable induction patterns in mononuclear cells. Life Sci (2004) 74(8):1039–49. doi: 10.1016/j.lfs.2003.07.022
23. Shinde R, McGaha TL. The aryl hydrocarbon receptor: Connecting immunity to the microenvironment. Trends Immunol (2018) 39(12):1005–20. doi: 10.1016/j.it.2018.10.010
24. Cella M, Colonna M. Aryl hydrocarbon receptor: Linking environment to immunity. Semin Immunol (2015) 27(5):310–4. doi: 10.1016/j.smim.2015.10.002
25. Dong B, Cheng W, Li W, Zheng J, Wu D, Matsumura F, et al. FRET analysis of protein tyrosine kinase c-src activation mediated via aryl hydrocarbon receptor. Biochim Biophys Acta (2011) 1810(4):427–31. doi: 10.1016/j.bbagen.2010.11.007
26. Dou H, Duan Y, Zhang X, Yu Q, Di Q, Song Y, et al. Aryl hydrocarbon receptor (AhR) regulates adipocyte differentiation by assembling CRL4B ubiquitin ligase to target PPARγ for proteasomal degradation. J Biol Chem (2019) 294(48):18504–15. doi: 10.1074/jbc.RA119.009282
27. Ohtake F, Baba A, Takada I, Okada M, Iwasaki K, Miki H, et al. Dioxin receptor is a ligand-dependent E3 ubiquitin ligase. Nature (2007) 446(7135):562–6. doi: 10.1038/nature05683
28. Cox MB, Miller 3CA. The p23 co-chaperone facilitates dioxin receptor signaling in a yeast model system. Toxicol Lett (2002) 129(1-2):13–21. doi: 10.1016/S0378-4274(01)00465-9
29. Uemura S, Nakajima Y, Yoshida Y, Furuya M, Matsutani S, Kawate S, et al. Biochemical properties of human full-length aryl hydrocarbon receptor (AhR). J Biochem (2020) 168(3):285–94. doi: 10.1093/jb/mvaa047
30. Meyer BK, Perdew GH. Characterization of the AhR-hsp90-XAP2 core complex and the role of the immunophilin-related protein XAP2 in AhR stabilization. Biochemistry (1999) 38(28):8907–17. doi: 10.1021/bi982223w
31. Perdew GH. Association of the ah receptor with the 90-kDa heat shock protein. J Biol Chem (1988) 263(27):13802–5. doi: 10.1016/S0021-9258(18)68314-0
32. Pongratz I, Mason GG, Poellinger L. Dual roles of the 90-kDa heat shock protein hsp90 in modulating functional activities of the dioxin receptor. evidence that the dioxin receptor functionally belongs to a subclass of nuclear receptors which require hsp90 both for ligand binding activity and repression of intrinsic DNA binding activity. J Biol Chem (1992) 267(19):13728–34. doi: 10.1016/S0021-9258(18)42274-0
33. Carver LA, LaPres JJ, Jain S, Dunham EE, Bradfield CA. Characterization of the ah receptor-associated protein, ARA9. J Biol Chem (1998) 273(50):33580–7. doi: 10.1074/jbc.273.50.33580
34. Kazlauskas A, Poellinger L, Pongratz I. The immunophilin-like protein XAP2 regulates ubiquitination and subcellular localization of the dioxin receptor. J Biol Chem (2000) 275(52):41317–24. doi: 10.1074/jbc.M007765200
35. Lees MJ, Peet DJ, Whitelaw ML. Defining the role for XAP2 in stabilization of the dioxin receptor. J Biol Chem (2003) 278(38):35878–88. doi: 10.1074/jbc.M302430200
36. Ikuta T, Eguchi H, Tachibana T, Yoneda Y, Kawajiri K. Nuclear localization and export signals of the human aryl hydrocarbon receptor. J Biol Chem (1998) 273(5):2895–904. doi: 10.1074/jbc.273.5.2895
37. Ikuta T, Kobayashi Y, Kawajiri K. Phosphorylation of nuclear localization signal inhibits the ligand-dependent nuclear import of aryl hydrocarbon receptor. Biochem Biophys Res Commun (2004) 317(2):545–50. doi: 10.1016/j.bbrc.2004.03.076
38. Tsuji N, Fukuda K, Nagata Y, Okada H, Haga A, Hatakeyama S, et al. The activation mechanism of the aryl hydrocarbon receptor (AhR) by molecular chaperone HSP90. FEBS Open Bio (2014) 4:796–803. doi: 10.1016/j.fob.2014.09.003
39. Pappas B, Yang Y, Wang Y, Kim K, Chung HJ, Cheung M. p23 protects the human aryl hydrocarbon receptor from degradation via a heat shock protein 90-independent mechanism. Biochem Pharmacol (2018) 152:34–44. doi: 10.1016/j.bcp.2018.03.015
40. Kazlauskas A, Sundström S, Poellinger L, Pongratz I. The hsp90 chaperone complex regulates intracellular localization of the dioxin receptor. Mol Cell Biol (2001) 21(7):2594–607. doi: 10.1128/MCB.21.7.2594-2607.2001
41. Davarinos NA, Pollenz RS. Aryl hydrocarbon receptor imported into the nucleus following ligand binding is rapidly degraded via the cytosplasmic proteasome following nuclear export. J Biol Chem (1999) 274(40):28708–15. doi: 10.1074/jbc.274.40.28708
42. Reyes H, Reisz-Porszasz S, Hankinson O. Identification of the ah receptor nuclear translocator protein (Arnt) as a component of the DNA binding form of the ah receptor. Science (1992) 256(5060):1193–5. doi: 10.1126/science.256.5060.1193
43. Seok SH, Lee W, Jiang L, Molugu K, Zheng A, Li Y, et al. Structural hierarchy controlling dimerization and target DNA recognition in the AHR transcriptional complex. Proc Natl Acad Sci U.S.A. (2017) 114(21):5431–6. doi: 10.1073/pnas.1617035114
44. Larigot L, Juricek L, Dairou J, Coumoul X. AhR signaling pathways and regulatory functions. Biochim Open (2018) 7:1–9. doi: 10.1016/j.biopen.2018.05.001
45. Henry EC, Gasiewicz TA. Transformation of the aryl hydrocarbon receptor to a DNA-binding form is accompanied by release of the 90 kDa heat-shock protein and increased affinity for 2,3,7,8-tetrachlorodibenzo-p-dioxin. Biochem J (1993) 294(Pt 1):95–101. doi: 10.1042/bj2940095
46. Wright EJ, De Castro KP, Joshi AD, Elferink CJ. Canonical and non-canonical aryl hydrocarbon receptor signaling pathways. Curr Opin Toxicol (2017) 2:87–92. doi: 10.1016/j.cotox.2017.01.001
47. Furman DP, Oshchepkova EA, Oshchepkov DY, Shamanina MY, Mordvinov VA. Promoters of the genes encoding the transcription factors regulating the cytokine gene expression in macrophages contain putative binding sites for aryl hydrocarbon receptor. Comput Biol Chem (2009) 33(6):465–8. doi: 10.1016/j.compbiolchem.2009.10.004
48. Durrin LK, Jones PB, Fisher JM, Galeazzi DR, Whitlock JP Jr. 2,3,7,8-tetrachlorodibenzo-p-dioxin receptors regulate transcription of the cytochrome P1-450 gene. J Cell Biochem (1987) 35(2):153–60. doi: 10.1002/jcb.240350208
49. Beischlag TV, Wang S, Rose DW, Torchia J, Reisz-Porszasz S, Muhammad K, et al. Recruitment of the NCoA/SRC-1/p160 family of transcriptional coactivators by the aryl hydrocarbon receptor/aryl hydrocarbon receptor nuclear translocator complex. Mol Cell Biol (2002) 22(12):4319–33. doi: 10.1128/MCB.22.12.4319-4333.2002
50. Wang S, Hankinson O. Functional involvement of the Brahma/SWI2-related gene 1 protein in cytochrome P4501A1 transcription mediated by the aryl hydrocarbon receptor complex. J Biol Chem (2002) 277(14):11821–7. doi: 10.1074/jbc.M110122200
51. Schnekenburger M, Peng L, Puga A. HDAC1 bound to the Cyp1a1 promoter blocks histone acetylation associated with ah receptor-mediated trans-activation. Biochim Biophys Acta (2007) 1769(9-10):569–78. doi: 10.1016/j.bbaexp.2007.07.002
52. Hankinson O. Role of coactivators in transcriptional activation by the aryl hydrocarbon receptor. Arch Biochem Biophys (2005) 433(2):379–86. doi: 10.1016/j.abb.2004.09.031
53. Kimura A, Naka T, Nakahama T, Chinen I, Masuda K, Nohara K, et al. Aryl hydrocarbon receptor in combination with Stat1 regulates LPS-induced inflammatory responses. J Exp Med (2009) 206(9):2027–35. doi: 10.1084/jem.20090560
54. Yeste A, Takenaka MC, Mascanfroni ID, Nadeau M, Kenison JE, Patel B, et al. Tolerogenic nanoparticles inhibit T cell-mediated autoimmunity through SOCS2. Sci Signal (2016) 9(433):ra61. doi: 10.1126/scisignal.aad0612
55. Vogel CF, Matsumura F. A new cross-talk between the aryl hydrocarbon receptor and RelB, a member of the NF-kappaB family. Biochem Pharmacol (2009) 77(4):734–45. doi: 10.1016/j.bcp.2008.09.036
56. Kim DW, Gazourian L, Quadri SA, Romieu-Mourez R, Sherr DH, Sonenshein GE, et al. The RelA NF-kappaB subunit and the aryl hydrocarbon receptor (AhR) cooperate to transactivate the c-myc promoter in mammary cells. Oncogene (2000) 19(48):5498–506. doi: 10.1038/sj.onc.1203945
57. Vogel CF, Sciullo E, Li W, Wong P, Lazennec G, Matsumura F, et al. RelB, a new partner of aryl hydrocarbon receptor-mediated transcription. Mol Endocrinol (2007) 21(12):2941–55. doi: 10.1210/me.2007-0211
58. Wilson SR, Joshi AD, Elferink CJ. The tumor suppressor kruppel-like factor 6 is a novel aryl hydrocarbon receptor DNA binding partner. J Pharmacol Exp Ther (2013) 345(3):419–29. doi: 10.1124/jpet.113.203786
59. Murray IA, Morales JL, Flaveny CA, Dinatale BC, Chiaro C, Gowdahalli K, et al. Evidence for ligand-mediated selective modulation of aryl hydrocarbon receptor activity. Mol Pharmacol (2010) 77(2):247–54. doi: 10.1124/mol.109.061788
60. Warner JO, Kaliner MA, Crisci CD, Del Giacco S, Frew AJ, Liu GH, et al. Allergy practice worldwide: a report by the world allergy organization specialty and training council. Int Arch Allergy Immunol (2006) 139(2):166–74. doi: 10.1159/000090502
61. Kay AB. Allergy and allergic diseases. first of two parts. N Engl J Med (2001) 344(1):30–7. doi: 10.1056/NEJM200101043440106
62. Lee TK, Jeon YJ, Jung SJ. Bi-directional association between allergic rhinitis and diabetes mellitus from the national representative data of south Korea. Sci Rep (2021) 11(1):4344. doi: 10.1038/s41598-021-83787-9
63. Hwang CY, Chen YJ, Lin MW, Chen TJ, Chu SY, Chen CC, et al. Cancer risk in patients with allergic rhinitis, asthma and atopic dermatitis: a nationwide cohort study in Taiwan. Int J Cancer (2012) 130(5):1160–7. doi: 10.1002/ijc.26105
64. Amritwar AU, Lowry CA, Brenner LA, Hoisington AJ, Hamilton R, Stiller JW, et al. Mental health in allergic rhinitis: Depression and suicidal behavior. Curr Treat Options Allergy (2017) 4(1):71–97. doi: 10.1007/s40521-017-0110-z
65. Tseng CH, Chen JH, Lin CL, Kao CH. Risk relation between rhinitis and acute ischemic stroke. Allergy Asthma Proc (2015) 36(6):e106–12. doi: 10.2500/aap.2015.36.3891
66. Crans Yoon AM, Chiu V, Rana JS, Sheikh J. Association of allergic rhinitis, coronary heart disease, cerebrovascular disease, and all-cause mortality. Ann Allergy Asthma Immunol (2016) 117(4):359–364.e1. doi: 10.1016/j.anai.2016.08.021
67. Raj D, Kabra SK, Lodha R. Childhood obesity and risk of allergy or asthma. Immunol Allergy Clin North Am (2014) 34(4):753–65. doi: 10.1016/j.iac.2014.07.001
68. Ma J, Xiao L, Knowles SB. Obesity, insulin resistance and the prevalence of atopy and asthma in US adults. Allergy (2010) 65(11):1455–63. doi: 10.1111/j.1398-9995.2010.02402.x
69. Thuesen BH, Husemoen LL, Hersoug LG, Pisinger C, Linneberg A. Insulin resistance as a predictor of incident asthma-like symptoms in adults. Clin Exp Allergy (2009) 39(5):700–7. doi: 10.1111/j.1365-2222.2008.03197.x
70. Hashimoto Y, Futamura A. Prevalence of allergic rhinitis is lower in subjects with higher levels of fasting plasma glucose. Diabetes Care (2010) 33(11):e143. doi: 10.2337/dc10-1338
71. Sicherer SH, Sampson HA. Peanut allergy: emerging concepts and approaches for an apparent epidemic. J Allergy Clin Immunol (2007) 120(3):491–503. doi: 10.1016/j.jaci.2007.07.015
72. Keet CA, Wood RA, Matsui EC. Limitations of reliance on specific IgE for epidemiologic surveillance of food allergy. J Allergy Clin Immunol (2012) 130(5):1207–1209.e10. doi: 10.1016/j.jaci.2012.07.020
73. Steering Committee Authors; Review Panel Members. A WAO - ARIA - GA(2)LEN consensus document on molecular-based allergy diagnosis (PAMD@): Update 2020. World Allergy Organ J (2020) 13(2):100091 doi: 10.1016/j.waojou.2019.100091
74. Matricardi PM, Kleine-Tebbe J, Hoffmann HJ, Valenta R, Hilger C, Hofmaier S, et al. EAACI molecular allergology user's guide. Pediatr Allergy Immunol (2016) 27 Suppl 23:1–250. doi: 10.1111/pai.12563
75. Eder W, Ege MJ, von Mutius E. The asthma epidemic. N Engl J Med (2006) 355(21):2226–35. doi: 10.1056/NEJMra054308
76. Hammad H, Lambrecht BN. Recent progress in the biology of airway dendritic cells and implications for understanding the regulation of asthmatic inflammation. J Allergy Clin Immunol (2006) 118(2):331–6. doi: 10.1016/j.jaci.2006.03.041
77. Glimcher LH. Trawling for treasure: tales of T-bet. Nat Immunol (2007) 8(5):448–50. doi: 10.1038/ni0507-448
78. Broide DH. Molecular and cellular mechanisms of allergic disease. J Allergy Clin Immunol (2001) 108(2 Suppl):S65–71. doi: 10.1067/mai.2001.116436
79. von Garnier C, Wikstrom ME, Zosky G, Turner DJ, Sly PD, Smith M, et al. Allergic airways disease develops after an increase in allergen capture and processing in the airway mucosa. J Immunol (2007) 179(9):5748–59. doi: 10.4049/jimmunol.179.9.5748
80. Cousins DJ, Lee TH, Staynov DZ. Cytokine coexpression during human Th1/Th2 cell differentiation: direct evidence for coordinated expression of Th2 cytokines. J Immunol (2002) 169(5):2498–506. doi: 10.4049/jimmunol.169.5.2498
81. Romagnani S. Regulation of the T cell response. Clin Exp Allergy (2006) 36(11):1357–66. doi: 10.1111/j.1365-2222.2006.02606.x
82. Bacher P, Heinrich F, Stervbo U, Nienen M, Vahldieck M, Iwert C, et al. Regulatory T cell specificity directs tolerance versus allergy against aeroantigens in humans. Cell (2016) 167(4):1067–1078.e16. doi: 10.1016/j.cell.2016.09.050
83. Bacchetta R, Gambineri E, Roncarolo MG. Role of regulatory T cells and FOXP3 in human diseases. J Allergy Clin Immunol (2007) 120(2):227–35. doi: 10.1016/j.jaci.2007.06.023
84. Larché M. Regulatory T cells in allergy and asthma. Chest (2007) 132(3):1007–14. doi: 10.1378/chest.06-2434
85. Schäfer T, Ring J. Epidemiology of allergic diseases. Allergy (1997) 52(38 Suppl):14–22. doi: 10.1111/j.1398-9995.1997.tb04864.x
86. Hartert TV, Peebles Jr. RS. Epidemiology of asthma: the year in review. Curr Opin Pulm Med (2000) 6(1):4–9. doi: 10.1097/00063198-200001000-00002
87. Matucci A, Vultaggio A, Maggi E, Kasujee I. Is IgE or eosinophils the key player in allergic asthma pathogenesis? are we asking the right question? Respir Res (2018) 19(1):113. doi: 10.1186/s12931-018-0813-0
88. Vogel CFA, Van Winkle LS, Esser C, Haarmann-Stemmann T. The aryl hydrocarbon receptor as a target of environmental stressors - implications for pollution mediated stress and inflammatory responses. Redox Biol (2020) 34:101530. doi: 10.1016/j.redox.2020.101530
89. Li XM, Peng J, Gu W, Guo XJ. TCDD-induced activation of aryl hydrocarbon receptor inhibits Th17 polarization and regulates non-eosinophilic airway inflammation in asthma. PloS One (2016) 11(3):e0150551. doi: 10.1371/journal.pone.0150551
90. Zhou Y, Tung HY, Tsai YM, Hsu SC, Chang HW, Kawasaki H, et al. Aryl hydrocarbon receptor controls murine mast cell homeostasis. Blood (2013) 121(16):3195–204. doi: 10.1182/blood-2012-08-453597
91. Sibilano R, Frossi B, Calvaruso M, Danelli L, Betto E, Dall'Agnese A, et al. The aryl hydrocarbon receptor modulates acute and late mast cell responses. J Immunol (2012) 189(1):120–7. doi: 10.4049/jimmunol.1200009
92. Hwang YJ, Yun MO, Jeong KT, Park JH. Uremic toxin indoxyl 3-sulfate regulates the differentiation of Th2 but not of Th1 cells to lessen allergic asthma. Toxicol Lett (2014) 225(1):130–8. doi: 10.1016/j.toxlet.2013.11.027
93. Thatcher TH, Williams MA, Pollock SJ, McCarthy CE, Lacy SH, Phipps RP, et al. Endogenous ligands of the aryl hydrocarbon receptor regulate lung dendritic cell function. Immunology (2016) 147(1):41–54. doi: 10.1111/imm.12540
94. Wong TH, Lee CL, Su HH, Lee CL, Wu CC, Wang CC, et al. A prominent air pollutant, Indeno[1,2,3-cd]pyrene, enhances allergic lung inflammation via aryl hydrocarbon receptor. Sci Rep (2018) 8(1):5198. doi: 10.1038/s41598-018-23542-9
95. Suen JL, Hsu SH, Hung CH, Chao YS, Lee CL, Lin CY, et al. A common environmental pollutant, 4-nonylphenol, promotes allergic lung inflammation in a murine model of asthma. Allergy (2013) 68(6):780–7. doi: 10.1111/all.12156
96. Cui Z, Feng Y, Li D, Li T, Gao P, Xu T, et al. Activation of aryl hydrocarbon receptor (AhR) in mesenchymal stem cells modulates macrophage polarization in asthma. J Immunotoxicol (2020) 17(1):21–30. doi: 10.1080/1547691X.2019.1706671
97. van Voorhis M, Knopp S, Julliard W, Fechner JH, Zhang X, Schauer JJ, et al. Exposure to atmospheric particulate matter enhances Th17 polarization through the aryl hydrocarbon receptor. PloS One (2013) 8(12):e82545. doi: 10.1371/journal.pone.0082545
98. Sun L, Fu J, Lin SH, Sun JL, Xia L, Lin CH, et al. Particulate matter of 2.5 μm or less in diameter disturbs the balance of T(H)17/regulatory T cells by targeting glutamate oxaloacetate transaminase 1 and hypoxia-inducible factor 1α in an asthma model. J Allergy Clin Immunol (2020) 145(1):402–14. doi: 10.1016/j.jaci.2019.10.008
99. Schulz VJ, Smit JJ, Huijgen V, Bol-Schoenmakers M, van Roest M, Kruijssen LJ, et al. Non-dioxin-like AhR ligands in a mouse peanut allergy model. Toxicol Sci (2012) 128(1):92–102. doi: 10.1093/toxsci/kfs131
100. Hammerschmidt-Kamper C, Biljes D, Merches K, Steiner I, Daldrup T, Bol-Schoenmakers M, et al. Indole-3-carbinol, a plant nutrient and AhR-ligand precursor, supports oral tolerance against OVA and improves peanut allergy symptoms in mice. PloS One (2017) 12(6):e0180321. doi: 10.1371/journal.pone.0180321
101. Esser C, Rannug A. The aryl hydrocarbon receptor in barrier organ physiology, immunology, and toxicology. Pharmacol Rev (2015) 67(2):259–79. doi: 10.1124/pr.114.009001
102. Fernandez-Salguero P, Pineau T, Hilbert DM, McPhail T, Lee SS, Kimura S, et al. Immune system impairment and hepatic fibrosis in mice lacking the dioxin-binding ah receptor. Science (1995) 268(5211):722–6. doi: 10.1126/science.7732381
103. Schmidt JV, Su GH, Reddy JK, Simon MC, Bradfield CA, et al. Characterization of a murine ahr null allele: involvement of the ah receptor in hepatic growth and development. Proc Natl Acad Sci U.S.A. (1996) 93(13):6731–6. doi: 10.1073/pnas.93.13.6731
104. Andreola F, Fernandez-Salguero PM, Chiantore MV, Petkovich MP, Gonzalez FJ, De Luca LM, et al. Aryl hydrocarbon receptor knockout mice (AHR-/-) exhibit liver retinoid accumulation and reduced retinoic acid metabolism. Cancer Res (1997) 57(14):2835–8.
105. Di Meglio P, Duarte JH, Ahlfors H, Owens ND, Li Y, Villanova F, et al. Activation of the aryl hydrocarbon receptor dampens the severity of inflammatory skin conditions. Immunity (2014) 40(6):989–1001. doi: 10.1016/j.immuni.2014.04.019
106. Yu J, Luo Y, Zhu Z, Zhou Y, Sun L, Gao J, Sun J, et al, et al. A tryptophan metabolite of the skin microbiota attenuates inflammation in patients with atopic dermatitis through the aryl hydrocarbon receptor. J Allergy Clin Immunol (2019) 143(6):2108–2119.e12. doi: 10.1016/j.jaci.2018.11.036
107. Fernandez-Salguero PM, Hilbert DM, Rudikoff S, Ward JM, Gonzalez FJ, et al. Aryl-hydrocarbon receptor-deficient mice are resistant to 2,3,7,8-tetrachlorodibenzo-p-dioxin-induced toxicity. Toxicol Appl Pharmacol (1996) 140(1):173–9. doi: 10.1006/taap.1996.0210
108. Zou QY, Hong SL, Kang HY, Ke X, Wang XQ, Li J, et al. Effect of di-(2-ethylhexyl) phthalate (DEHP) on allergic rhinitis. Sci Rep (2020) 10(1):14625. doi: 10.1038/s41598-020-71517-6
109. Grishanova AY, Perepechaeva ML. Aryl hydrocarbon receptor in oxidative stress as a double agent and its biological and therapeutic significance. Int J Mol Sci (2022) 23(12):6719. doi: 10.3390/ijms23126719
110. Lee WJ, Liu SH, Chiang CK, Lin SY, Liang KW, Chen CH, et al. Aryl hydrocarbon receptor deficiency attenuates oxidative stress-related mesangial cell activation and macrophage infiltration and extracellular matrix accumulation in diabetic nephropathy. Antioxid Redox Signal (2016) 24(4):217–31. doi: 10.1089/ars.2015.6310
111. Qiu J, Heller JJ, Guo X, Chen ZM, Fish K, Fu YX, et al. The aryl hydrocarbon receptor regulates gut immunity through modulation of innate lymphoid cells. Immunity (2012) 36(1):92–104. doi: 10.1016/j.immuni.2011.11.011
112. Beamer CA, Shepherd DM. Role of the aryl hydrocarbon receptor (AhR) in lung inflammation. Semin Immunopathol (2013) 35(6):693–704. doi: 10.1007/s00281-013-0391-7
113. Kawasaki H, Chang HW, Tseng HC, Hsu SC, Yang SJ, Hung CH, et al. A tryptophan metabolite, kynurenine, promotes mast cell activation through aryl hydrocarbon receptor. Allergy (2014) 69(4):445–52. doi: 10.1111/all.12346
114. Nguyen NT, Kimura A, Nakahama T, Chinen I, Masuda K, Nohara K, et al. Aryl hydrocarbon receptor negatively regulates dendritic cell immunogenicity via a kynurenine-dependent mechanism. Proc Natl Acad Sci U.S.A. (2010) 107(46):19961–6. doi: 10.1073/pnas.1014465107
115. Mascanfroni ID, Takenaka MC, Yeste A, Patel B, Wu Y, Kenison JE, et al. Metabolic control of type 1 regulatory T cell differentiation by AHR and HIF1-α. Nat Med (2015) 21(6):638–46. doi: 10.1038/nm.3868
116. Takami M, Fujimaki K, Nishimura MI, Iwashima M. Cutting edge: AhR is a molecular target of calcitriol in human T cells. J Immunol (2015) 195(6):2520–3. doi: 10.4049/jimmunol.1500344
117. Li Y, Innocentin S, Withers DR, Roberts NA, Gallagher AR, Grigorieva EF, et al. Exogenous stimuli maintain intraepithelial lymphocytes via aryl hydrocarbon receptor activation. Cell (2011) 147(3):629–40. doi: 10.1016/j.cell.2011.09.025
118. Xiong L, Dean JW, Fu Z, Oliff KN, Bostick JW, Ye J, et al. Ahr-Foxp3-RORγt axis controls gut homing of CD4(+) T cells by regulating GPR15. Sci Immunol (2020) 5(48):eaaz7277. doi: 10.1126/sciimmunol.aaz7277
119. Kadow S, Jux B, Zahner SP, Wingerath B, Chmill S, Clausen BE, et al. Aryl hydrocarbon receptor is critical for homeostasis of invariant gammadelta T cells in the murine epidermis. J Immunol (2011) 187(6):3104–10. doi: 10.4049/jimmunol.1100912
120. Veldhoen M, Hirota K, Westendorf AM, Buer J, Dumoutier L, Renauld JC, et al. The aryl hydrocarbon receptor links TH17-cell-mediated autoimmunity to environmental toxins. Nature (2008) 453(7191):106–9. doi: 10.1038/nature06881
121. Quintana FJ, Basso AS, Iglesias AH, Korn T, Farez MF, Bettelli E, et al. Control of t(reg) and T(H)17 cell differentiation by the aryl hydrocarbon receptor. Nature (2008) 453(7191):65–71. doi: 10.1038/nature06880
122. Kiss EA, Vonarbourg C, Kopfmann S, Hobeika E, Finke D, Esser C, et al. Natural aryl hydrocarbon receptor ligands control organogenesis of intestinal lymphoid follicles. Science (2011) 334(6062):1561–5. doi: 10.1126/science.1214914
123. Kanagaratham C, El Ansari YS, Lewis OL, Oettgen HC. IgE and IgG antibodies as regulators of mast cell and basophil functions in food allergy. Front Immunol (2020) 11:603050. doi: 10.3389/fimmu.2020.603050
124. Méndez-Enríquez E, Hallgren J. Mast cells and their progenitors in allergic asthma. Front Immunol (2019) 10:821. doi: 10.3389/fimmu.2019.00821
125. Mukai K, Tsai M, Saito H, Galli SJ. Mast cells as sources of cytokines, chemokines, and growth factors. Immunol Rev (2018) 282(1):121–50. doi: 10.1111/imr.12634
126. DiNatale BC, Schroeder JC, Francey LJ, Kusnadi A, Perdew GH. Mechanistic insights into the events that lead to synergistic induction of interleukin 6 transcription upon activation of the aryl hydrocarbon receptor and inflammatory signaling. J Biol Chem (2010) 285(32):24388–97. doi: 10.1074/jbc.M110.118570
127. Maaetoft-Udsen K, Shimoda LM, Frøkiær H, Turner H. Aryl hydrocarbon receptor ligand effects in RBL2H3 cells. J Immunotoxicol (2012) 9(3):327–37. doi: 10.3109/1547691X.2012.661802
128. Swindle EJ, Metcalfe DD. The role of reactive oxygen species and nitric oxide in mast cell-dependent inflammatory processes. Immunol Rev (2007) 217:186–205. doi: 10.1111/j.1600-065X.2007.00513.x
129. Wang H, Do DC, Liu J, Wang B, Qu J, Ke X, et al. Functional role of kynurenine and aryl hydrocarbon receptor axis in chronic rhinosinusitis with nasal polyps. J Allergy Clin Immunol (2018) 141(2):586–600.e6. doi: 10.1016/j.jaci.2017.06.013
130. James B, Milstien S, Spiegel S. ORMDL3 and allergic asthma: From physiology to pathology. J Allergy Clin Immunol (2019) 144(3):634–40. doi: 10.1016/j.jaci.2019.07.023
131. Wang HC, Wong TH, Wang LT, Su HH, Yu HY, Wu AH, et al. Aryl hydrocarbon receptor signaling promotes ORMDL3-dependent generation of sphingosine-1-phosphate by inhibiting sphingosine-1-phosphate lyase. Cell Mol Immunol (2019) 16(10):783–90. doi: 10.1038/s41423-018-0022-2
132. Janssen-Weets B, Kerff F, Swiontek K, Kler S, Czolk R, Revets D, et al. Mammalian derived lipocalin and secretoglobin respiratory allergens strongly bind ligands with potentially immune modulating properties. Front Allergy (2022) 3:958711. doi: 10.3389/falgy.2022.958711
133. Roth-Walter F, Afify SM, Pacios LF, Blokhuis BR, Redegeld F, Regner A, et al. Cow's milk protein β-lactoglobulin confers resilience against allergy by targeting complexed iron into immune cells. J Allergy Clin Immunol (2021) 147(1):321–334.e4. doi: 10.1016/j.jaci.2020.05.023
134. Humeniuk P, Dubiela P, Hoffmann-Sommergruber K. Dendritic cells and their role in allergy: Uptake, proteolytic processing and presentation of allergens. Int J Mol Sci (2017) 18(7):1491. doi: 10.3390/ijms18071491
135. Ruiter B, Shreffler WG. The role of dendritic cells in food allergy. J Allergy Clin Immunol (2012) 129(4):921–8. doi: 10.1016/j.jaci.2012.01.080
136. Rowden G, Lewis MG, Sullivan AK. Ia antigen expression on human epidermal langerhans cells. Nature (1977) 268(5617):247–8. doi: 10.1038/268247a0
137. Stingl G, Wolff-Schreiner EC, Pichler WJ, Gschnait F, Knapp W, Wolff K. Epidermal langerhans cells bear fc and C3 receptors. Nature (1977) 268(5617):245–6. doi: 10.1038/268245a0
138. Merad M, Ginhoux F, Collin M. Origin, homeostasis and function of langerhans cells and other langerin-expressing dendritic cells. Nat Rev Immunol (2008) 8(12):935–47. doi: 10.1038/nri2455
139. Aiba S, Tagami H. Dendritic cells play a crucial role in innate immunity to simple chemicals. J Investig Dermatol Symp Proc (1999) 4(2):158–63. doi: 10.1038/sj.jidsp.5640201
140. Serbina NV, Salazar-Mather TP, Biron CA, Kuziel WA, Pamer EG. TNF/iNOS-producing dendritic cells mediate innate immune defense against bacterial infection. Immunity (2003) 19(1):59–70. doi: 10.1016/S1074-7613(03)00171-7
141. Wollenberg A, Wagner M, Günther S, Towarowski A, Tuma E, Moderer M, et al. Plasmacytoid dendritic cells: a new cutaneous dendritic cell subset with distinct role in inflammatory skin diseases. J Invest Dermatol (2002) 119(5):1096–102. doi: 10.1046/j.1523-1747.2002.19515.x
142. Stary G, Bangert C, Stingl G, Kopp T. Dendritic cells in atopic dermatitis: expression of FcepsilonRI on two distinct inflammation-associated subsets. Int Arch Allergy Immunol (2005) 138(4):278–90. doi: 10.1159/000088865
143. Wollenberg A, Mommaas M, Oppel T, Schottdorf EM, Günther S, Moderer M, et al. Expression and function of the mannose receptor CD206 on epidermal dendritic cells in inflammatory skin diseases. J Invest Dermatol (2002) 118(2):327–34. doi: 10.1046/j.0022-202x.2001.01665.x
144. Bieber T, Kraft S, Geiger E, Wollenberg A, Koch S, Novak N, et al. Fc [correction of ec] epsilon RI expressing dendritic cells: the missing link in the pathophysiology of atopic dermatitis? J Dermatol (2000) 27(11):698–9. doi: 10.1111/j.1346-8138.2000.tb02261.x
145. Smit JJ, Bol-Schoenmakers M, Hassing I, Fiechter D, Boon L, Bleumink R, et al. The role of intestinal dendritic cells subsets in the establishment of food allergy. Clin Exp Allergy (2011) 41(6):890–8. doi: 10.1111/j.1365-2222.2011.03738.x
146. Coombes JL, Siddiqui KR, Arancibia-Cárcamo CV, Hall J, Sun CM, Belkaid Y, et al. A functionally specialized population of mucosal CD103+ DCs induces Foxp3+ regulatory T cells via a TGF-beta and retinoic acid-dependent mechanism. J Exp Med (2007) 204(8):1757–64. doi: 10.1084/jem.20070590
147. Sun CM, Hall JA, Blank RB, Bouladoux N, Oukka M, Mora JR, et al. Small intestine lamina propria dendritic cells promote de novo generation of Foxp3 T reg cells via retinoic acid. J Exp Med (2007) 204(8):1775–85. doi: 10.1084/jem.20070602
148. Coombes JL, Powrie F. Dendritic cells in intestinal immune regulation. Nat Rev Immunol (2008) 8(6):435–46. doi: 10.1038/nri2335
149. van Wijk F, Wehrens EJ, Nierkens S, Boon L, Kasran A, Pieters R, et al. CD4+CD25+ T cells regulate the intensity of hypersensitivity responses to peanut, but are not decisive in the induction of oral sensitization. Clin Exp Allergy (2007) 37(4):572–81. doi: 10.1111/j.1365-2222.2007.02681.x
150. Goudot C, Coillard A, Villani AC, Gueguen P, Cros A, Sarkizova S, et al. Aryl hydrocarbon receptor controls monocyte differentiation into dendritic cells versus macrophages. Immunity (2017) 47(3):582–596.e6. doi: 10.1016/j.immuni.2017.08.016
151. Chmill S, Kadow S, Winter M, Weighardt H, Esser C. 2,3,7,8-tetrachlorodibenzo-p-dioxin impairs stable establishment of oral tolerance in mice. Toxicol Sci (2010) 118(1):98–107. doi: 10.1093/toxsci/kfq232
152. Bankoti J, Burnett A, Navarro S, Miller AK, Rase B, Shepherd DM, et al. Effects of TCDD on the fate of naive dendritic cells. Toxicol Sci (2010) 115(2):422–34. doi: 10.1093/toxsci/kfq063
153. Quintana FJ, Murugaiyan G, Farez MF, Mitsdoerffer M, Tukpah AM, Burns EJ, et al. An endogenous aryl hydrocarbon receptor ligand acts on dendritic cells and T cells to suppress experimental autoimmune encephalomyelitis. Proc Natl Acad Sci U.S.A. (2010) 107(48):20768–73. doi: 10.1073/pnas.1009201107
154. Jaronen M, Quintana FJ. Immunological relevance of the coevolution of IDO1 and AHR. Front Immunol (2014) 5:521. doi: 10.3389/fimmu.2014.00521
155. Mezrich JD, Fechner JH, Zhang X, Johnson BP, Burlingham WJ, Bradfield CA, et al. An interaction between kynurenine and the aryl hydrocarbon receptor can generate regulatory T cells. J Immunol (2010) 185(6):3190–8. doi: 10.4049/jimmunol.0903670
156. Jux B, Kadow S, Esser C. Langerhans cell maturation and contact hypersensitivity are impaired in aryl hydrocarbon receptor-null mice. J Immunol (2009) 182(11):6709–17. doi: 10.4049/jimmunol.0713344
157. Vogel CF, Goth SR, Dong B, Pessah IN, Matsumura F. Aryl hydrocarbon receptor signaling mediates expression of indoleamine 2,3-dioxygenase. Biochem Biophys Res Commun (2008) 375(3):331–5. doi: 10.1016/j.bbrc.2008.07.156
158. Ross EA, Devitt A, Johnson JR. Macrophages: The good, the bad, and the gluttony. Front Immunol (2021) 12:708186. doi: 10.3389/fimmu.2021.708186
159. Saradna A, Do DC, Kumar S, Fu QL, Gao P. Macrophage polarization and allergic asthma. Transl Res (2018) 191:1–14. doi: 10.1016/j.trsl.2017.09.002
160. Mills CD. M1 and M2 macrophages: Oracles of health and disease. Crit Rev Immunol (2012) 32(6):463–88. doi: 10.1615/CritRevImmunol.v32.i6.10
161. Suzuki K, Meguro K, Nakagomi D, Nakajima H. Roles of alternatively activated M2 macrophages in allergic contact dermatitis. Allergol Int (2017) 66(3):392–7. doi: 10.1016/j.alit.2017.02.015
162. Iwasaki N, Terawaki S, Shimizu K, Oikawa D, Sakamoto H, Sunami K, et al. Th2 cells and macrophages cooperatively induce allergic inflammation through histamine signaling. PloS One (2021) 16(3):e0248158. doi: 10.1371/journal.pone.0248158
163. Girodet PO, Nguyen D, Mancini JD, Hundal M, Zhou X, Israel E, et al. Alternative macrophage activation is increased in asthma. Am J Respir Cell Mol Biol (2016) 55(4):467–75. doi: 10.1165/rcmb.2015-0295OC
164. Zhu J, Luo L, Tian L, Yin S, Ma X, Cheng S, et al. Aryl hydrocarbon receptor promotes IL-10 expression in inflammatory macrophages through src-STAT3 signaling pathway. Front Immunol (2018) 9. doi: 10.3389/fimmu.2018.02033
165. Großkopf H, Walter K, Karkossa I, von Bergen M, Schubert K. Non-genomic AhR-signaling modulates the immune response in endotoxin-activated macrophages after activation by the environmental stressor BaP. Front Immunol (2021) 12. doi: 10.3389/fimmu.2021.620270
166. Climaco-Arvizu S, Domínguez-Acosta O, Cabañas-Cortés MA, Rodríguez-Sosa M, Gonzalez FJ, Vega L, et al. Aryl hydrocarbon receptor influences nitric oxide and arginine production and alters M1/M2 macrophage polarization. Life Sci (2016) 155:76–84. doi: 10.1016/j.lfs.2016.05.001
167. Hezaveh K, Shinde RS, Klötgen A, Halaby MJ, Lamorte S, Ciudad MT, et al. Tryptophan-derived microbial metabolites activate the aryl hydrocarbon receptor in tumor-associated macrophages to suppress anti-tumor immunity. Immunity (2022) 55(2):324–340.e8. doi: 10.1016/j.immuni.2022.01.006
168. Riemschneider S, Kohlschmidt J, Fueldner C, Esser C, Hauschildt S, Lehmann J, et al. Aryl hydrocarbon receptor activation by benzo(a)pyrene inhibits proliferation of myeloid precursor cells and alters the differentiation state as well as the functional phenotype of murine bone marrow-derived macrophages. Toxicol Lett (2018) 296:106–13. doi: 10.1016/j.toxlet.2018.07.050
169. Yang X, Liu H, Ye T, Duan C, Lv P, Wu X, et al. AhR activation attenuates calcium oxalate nephrocalcinosis by diminishing M1 macrophage polarization and promoting M2 macrophage polarization. Theranostics (2020) 10(26):12011–25. doi: 10.7150/thno.51144
170. Shinde R, Hezaveh K, Halaby MJ, Kloetgen A, Chakravarthy A, da Silva Medina T, et al. Apoptotic cell-induced AhR activity is required for immunological tolerance and suppression of systemic lupus erythematosus in mice and humans. Nat Immunol (2018) 19(6):571–82. doi: 10.1038/s41590-018-0107-1
171. Cancro MP, Tomayko MM. Memory b cells and plasma cells: The differentiative continuum of humoral immunity. Immunol Rev (2021) 303(1):72–82. doi: 10.1111/imr.13016
172. Ettmayer P, Mayer P, Kalthoff F, Neruda W, Harrer N, Hartmann G, et al. A novel low molecular weight inhibitor of dendritic cells and b cells blocks allergic inflammation. Am J Respir Crit Care Med (2006) 173(6):599–606. doi: 10.1164/rccm.200503-468OC
173. Vaidyanathan B, Chaudhry A, Yewdell WT, Angeletti D, Yen WF, Wheatley AK, et al. The aryl hydrocarbon receptor controls cell-fate decisions in b cells. J Exp Med (2017) 214(1):197–208. doi: 10.1084/jem.20160789
174. Piper CJM, Rosser EC, Oleinika K, Nistala K, Krausgruber T, Rendeiro AF, et al. Aryl hydrocarbon receptor contributes to the transcriptional program of IL-10-Producing regulatory b cells. Cell Rep (2019) 29(7):1878–1892.e7. doi: 10.1016/j.celrep.2019.10.018
175. Rosser EC, Piper CJM, Matei DE, Blair PA, Rendeiro AF, Orford M, et al. Microbiota-derived metabolites suppress arthritis by amplifying aryl-hydrocarbon receptor activation in regulatory b cells. Cell Metab (2020) 31(4):837–851.e10. doi: 10.1016/j.cmet.2020.03.003
176. Kerkvliet NI. Recent advances in understanding the mechanisms of TCDD immunotoxicity. Int Immunopharmacol (2002) 2(2-3):277–91. doi: 10.1016/S1567-5769(01)00179-5
177. Sulentic CE, Kaminski NE. The long winding road toward understanding the molecular mechanisms for b-cell suppression by 2,3,7,8-tetrachlorodibenzo-p-dioxin. Toxicol Sci (2011) 120 Suppl 1(Suppl 1):S171–91. doi: 10.1093/toxsci/kfq324
178. Lambrecht BN, Hammad H. The immunology of the allergy epidemic and the hygiene hypothesis. Nat Immunol (2017) 18(10):1076–83. doi: 10.1038/ni.3829
179. Robinson DS, Hamid Q, Ying S, Tsicopoulos A, Barkans J, Bentley AM, et al. Predominant TH2-like bronchoalveolar T-lymphocyte population in atopic asthma. N Engl J Med (1992) 326(5):298–304. doi: 10.1056/NEJM199201303260504
180. McKenzie AN. Type-2 innate lymphoid cells in asthma and allergy. Ann Am Thorac Soc (2014) 11 Suppl 5(Suppl 5):S263–70. doi: 10.1513/AnnalsATS.201403-097AW
181. Everaere L, Ait-Yahia S, Molendi-Coste O, Vorng H, Quemener S, LeVu P, et al. Innate lymphoid cells contribute to allergic airway disease exacerbation by obesity. J Allergy Clin Immunol (2016) 138(5):1309–1318.e11. doi: 10.1016/j.jaci.2016.03.019
182. Kim HY, Lee HJ, Chang YJ, Pichavant M, Shore SA, Fitzgerald KA, et al. Interleukin-17-producing innate lymphoid cells and the NLRP3 inflammasome facilitate obesity-associated airway hyperreactivity. Nat Med (2014) 20(1):54–61. doi: 10.1038/nm.3423
183. Lee JS, Lee HJ, Chang YJ, Pichavant M, Shore SA, Fitzgerald KA, et al. AHR drives the development of gut ILC22 cells and postnatal lymphoid tissues via pathways dependent on and independent of notch. Nat Immunol (2011) 13(2):144–51. doi: 10.1038/ni.2187
184. Li S, Cella M, McDonald KG, Garlanda C, Kennedy GD, Nukaya M, et al. Aryl hydrocarbon receptor signaling cell intrinsically inhibits intestinal group 2 innate lymphoid cell function. Immunity (2018) 49(5):915–928.e5. doi: 10.1016/j.immuni.2018.09.015
185. Stockinger B, Di Meglio P, Gialitakis M, Duarte JH. The aryl hydrocarbon receptor: multitasking in the immune system. Annu Rev Immunol (2014) 32:403–32. doi: 10.1146/annurev-immunol-032713-120245
186. Kimura A, Naka T, Nohara K, Fujii-Kuriyama Y, Kishimoto T, et al. Aryl hydrocarbon receptor regulates Stat1 activation and participates in the development of Th17 cells. Proc Natl Acad Sci U.S.A. (2008) 105(28):9721–6. doi: 10.1073/pnas.0804231105
187. Plé C, Fan Y, Ait Yahia S, Vorng H, Everaere L, Chenivesse C, et al. Polycyclic aromatic hydrocarbons reciprocally regulate IL-22 and IL-17 cytokines in peripheral blood mononuclear cells from both healthy and asthmatic subjects. PloS One (2015) 10(4):e0122372. doi: 10.1371/journal.pone.0122372
188. Trifari S, Kaplan CD, Tran EH, Crellin NK, Spits H. Identification of a human helper T cell population that has abundant production of interleukin 22 and is distinct from T(H)-17, T(H)1 and T(H)2 cells. Nat Immunol (2009) 10(8):864–71. doi: 10.1038/ni.1770
189. Lee Y, Awasthi A, Yosef N, Quintana FJ, Xiao S, Peters A, et al. Induction and molecular signature of pathogenic TH17 cells. Nat Immunol (2012) 13(10):991–9. doi: 10.1038/ni.2416
190. Funatake CJ, Marshall NB, Steppan LB, Mourich DV, Kerkvliet NI. Cutting edge: activation of the aryl hydrocarbon receptor by 2,3,7,8-tetrachlorodibenzo-p-dioxin generates a population of CD4+ CD25+ cells with characteristics of regulatory T cells. J Immunol (2005) 175(7):4184–8. doi: 10.4049/jimmunol.175.7.4184
191. Gandhi R, Kumar D, Burns EJ, Nadeau M, Dake B, Laroni A, et al. Activation of the aryl hydrocarbon receptor induces human type 1 regulatory T cell-like and Foxp3(+) regulatory T cells. Nat Immunol (2010) 11(9):846–53. doi: 10.1038/ni.1915
192. Singh NP, Singh UP, Singh B, Price RL, Nagarkatti M, Nagarkatti PS, et al. Activation of aryl hydrocarbon receptor (AhR) leads to reciprocal epigenetic regulation of FoxP3 and IL-17 expression and amelioration of experimental colitis. PloS One (2011) 6(8):e23522. doi: 10.1371/journal.pone.0023522
193. Suarez-Alvarez B, Rodriguez RM, Fraga MF, López-Larrea C. DNA Methylation: a promising landscape for immune system-related diseases. Trends Genet (2012) 28(10):506–14. doi: 10.1016/j.tig.2012.06.005
194. Nadeau K, McDonald-Hyman C, Noth EM, Pratt B, Hammond SK, Balmes J, et al. Ambient air pollution impairs regulatory T-cell function in asthma. J Allergy Clin Immunol (2010) 126(4):845–852.e10. doi: 10.1016/j.jaci.2010.08.008
195. Apetoh L, Quintana FJ, Pot C, Joller N, Xiao S, Kumar D, et al. The aryl hydrocarbon receptor interacts with c-maf to promote the differentiation of type 1 regulatory T cells induced by IL-27. Nat Immunol (2010) 11(9):854–61. doi: 10.1038/ni.1912
196. Tousa S, Semitekolou M, Morianos I, Banos A, Trochoutsou AI, Brodie TM, et al. Activin-a co-opts IRF4 and AhR signaling to induce human regulatory T cells that restrain asthmatic responses. Proc Natl Acad Sci U.S.A. (2017) 114(14):E2891–e2900. doi: 10.1073/pnas.1616942114
197. Gagliani N, Amezcua Vesely MC, Iseppon A, Brockmann L, Xu H, Palm NW, et al. Th17 cells transdifferentiate into regulatory T cells during resolution of inflammation. Nature (2015) 523(7559):221–5. doi: 10.1038/nature14452
198. Wei P, Hu GH, Kang HY, Yao HB, Kou W, Liu H, et al. An aryl hydrocarbon receptor ligand acts on dendritic cells and T cells to suppress the Th17 response in allergic rhinitis patients. Lab Invest (2014) 94(5):528–35. doi: 10.1038/labinvest.2014.8
199. Quintana FJ, Jin H, Burns EJ, Nadeau M, Yeste A, Kumar D, et al. Aiolos promotes TH17 differentiation by directly silencing Il2 expression. Nat Immunol (2012) 13(8):770–7. doi: 10.1038/ni.2363
200. Wang J, Zhao Y, Zhang X, Tu W, Wan R, Shen Y, et al. Type II alveolar epithelial cell aryl hydrocarbon receptor protects against allergic airway inflammation through controlling cell autophagy. Front Immunol (2022) 13:964575. doi: 10.3389/fimmu.2022.964575
201. Basu R, O'Quinn DB, Silberger DJ, Schoeb TR, Fouser L, Ouyang W, et al. Th22 cells are an important source of IL-22 for host protection against enteropathogenic bacteria. Immunity (2012) 37(6):1061–75. doi: 10.1016/j.immuni.2012.08.024
202. Ramirez JM, Brembilla NC, Sorg O, Chicheportiche R, Matthes T, Dayer JM, et al. Activation of the aryl hydrocarbon receptor reveals distinct requirements for IL-22 and IL-17 production by human T helper cells. Eur J Immunol (2010) 40(9):2450–9. doi: 10.1002/eji.201040461
203. Lawrence BP, Roberts AD, Neumiller JJ, Cundiff JA, Woodland DL, et al. Aryl hydrocarbon receptor activation impairs the priming but not the recall of influenza virus-specific CD8+ T cells in the lung. J Immunol (2006) 177(9):5819–28. doi: 10.4049/jimmunol.177.9.5819
204. Winans B, Nagari A, Chae M, Post CM, Ko CI, Puga A, et al. Linking the aryl hydrocarbon receptor with altered DNA methylation patterns and developmentally induced aberrant antiviral CD8+ T cell responses. J Immunol (2015) 194(9):4446–57. doi: 10.4049/jimmunol.1402044
205. Zaid A, Mackay LK, Rahimpour A, Braun A, Veldhoen M, Carbone FR, et al. Persistence of skin-resident memory T cells within an epidermal niche. Proc Natl Acad Sci U.S.A. (2014) 111(14):5307–12. doi: 10.1073/pnas.1322292111
206. Lockhart E, Green AM, Flynn JL. IL-17 production is dominated by gammadelta T cells rather than CD4 T cells during mycobacterium tuberculosis infection. J Immunol (2006) 177(7):4662–9. doi: 10.4049/jimmunol.177.7.4662
207. Duan J, Chung H, Troy E, Kasper DL. Microbial colonization drives expansion of IL-1 receptor 1-expressing and IL-17-producing gamma/delta T cells. Cell Host Microbe (2010) 7(2):140–50. doi: 10.1016/j.chom.2010.01.005
208. Martin B, Hirota K, Cua DJ, Stockinger B, Veldhoen M. Interleukin-17-producing gammadelta T cells selectively expand in response to pathogen products and environmental signals. Immunity (2009) 31(2):321–30. doi: 10.1016/j.immuni.2009.06.020
209. Schulz VJ, Smit JJ, Bol-Schoenmakers M, van Duursen MB, van den Berg M, Pieters RH, et al. Activation of the aryl hydrocarbon receptor reduces the number of precursor and effector T cells, but preserves thymic CD4+CD25+Foxp3+ regulatory T cells. Toxicol Lett (2012) 215(2):100–9. doi: 10.1016/j.toxlet.2012.09.024
210. Vuerich M, Harshe R, Frank LA, Mukherjee S, Gromova B, Csizmadia E, et al. Altered aryl-hydrocarbon-receptor signalling affects regulatory and effector cell immunity in autoimmune hepatitis. J Hepatol (2021) 74(1):48–57. doi: 10.1016/j.jhep.2020.06.044
211. Kamanaka M, Huber S, Zenewicz LA, Gagliani N, Rathinam C, O'Connor Jr W, et al. Memory/effector (CD45RB(lo)) CD4 T cells are controlled directly by IL-10 and cause IL-22-dependent intestinal pathology. J Exp Med (2011) 208(5):1027–40. doi: 10.1084/jem.20102149
212. Kirchberger S, Royston DJ, Boulard O, Thornton E, Franchini F, Szabady RL, et al. Innate lymphoid cells sustain colon cancer through production of interleukin-22 in a mouse model. J Exp Med (2013) 210(5):917–31. doi: 10.1084/jem.20122308
213. Robbins K, Bissonnette R, Maeda-Chubachi T, Ye L, Peppers J, Gallagher K, et al. Phase 2, randomized dose-finding study of tapinarof (GSK2894512 cream) for the treatment of plaque psoriasis. J Am Acad Dermatol (2019) 80(3):714–21. doi: 10.1016/j.jaad.2018.10.037
214. Kaye J, Piryatinsky V, Birnberg T, Hingaly T, Raymond E, Kashi R, et al. Laquinimod arrests experimental autoimmune encephalomyelitis by activating the aryl hydrocarbon receptor. Proc Natl Acad Sci U.S.A. (2016) 113(41):E6145–e6152. doi: 10.1073/pnas.1607843113
215. Smith SH, Jayawickreme C, Rickard DJ, Nicodeme E, Bui T, Simmons C, et al. Tapinarof is a natural AhR agonist that resolves skin inflammation in mice and humans. J Invest Dermatol (2017) 137(10):2110–9. doi: 10.1016/j.jid.2017.05.004
216. Wang HC, Huang SK. Metformin inhibits IgE- and aryl hydrocarbon receptor-mediated mast cell activation in vitro and in vivo. Eur J Immunol (2018) 48(12):1989–96. doi: 10.1002/eji.201847706
Keywords: aryl hydrocarbon receptor (AhR), allergy, innate immunity, adaptive immunity, T cell, autoimmune disease
Citation: Riaz F, Pan F and Wei P (2022) Aryl hydrocarbon receptor: The master regulator of immune responses in allergic diseases. Front. Immunol. 13:1057555. doi: 10.3389/fimmu.2022.1057555
Received: 29 September 2022; Accepted: 02 December 2022;
Published: 19 December 2022.
Edited by:
Bo Zhang, Huazhong University of Science and Technology, ChinaReviewed by:
Huaqi Guo, Shanghai Jiao Tong University, ChinaXue-Feng Bai, The Ohio State University, United States
Guangyong Peng, Saint Louis University, United States
Copyright © 2022 Riaz, Pan and Wei. This is an open-access article distributed under the terms of the Creative Commons Attribution License (CC BY). The use, distribution or reproduction in other forums is permitted, provided the original author(s) and the copyright owner(s) are credited and that the original publication in this journal is cited, in accordance with accepted academic practice. No use, distribution or reproduction is permitted which does not comply with these terms.
*Correspondence: Ping Wei, d2Vpd2VpZG9jdG9yQHNpbmEuY24=; Fan Pan, ZmFuLnBhbkBzaWF0LmFjLmNu