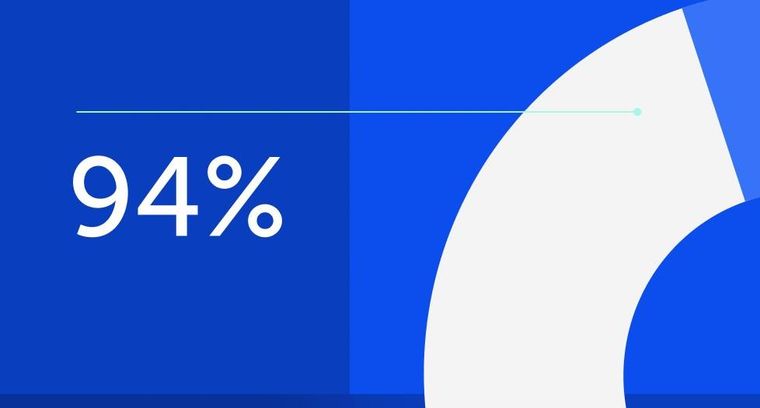
94% of researchers rate our articles as excellent or good
Learn more about the work of our research integrity team to safeguard the quality of each article we publish.
Find out more
ORIGINAL RESEARCH article
Front. Immunol., 20 December 2022
Sec. Cancer Immunity and Immunotherapy
Volume 13 - 2022 | https://doi.org/10.3389/fimmu.2022.1050876
Background: Exploring the cancer risks of rheumatoid arthritis (RA) patients with disease-modifying anti-rheumatic drugs (DMARDs) can help detect, evaluate, and treat malignancies at an early stage for these patients. Thus, a comprehensive analysis was conducted to determine the cancer risk of RA patients using different types of DMARDs and analyze their relationship with tumor mutational burdens (TMBs) reflecting immunogenicity.
Methods: A thorough search of PubMed, EMBASE, Web of Science, and Medline was conducted up to 20 August 2022. Standardized incidence ratios (SIRs) were constructed with a random-effect model to determine risks for different types of malignancies in comparison with the general population. We also analyzed the correlation between SIRs and TMBs using linear regression (LR).
Results: From a total of 22 studies, data on 371,311 RA patients receiving different types of DMARDs, 36 kinds of malignancies, and four regions were available. Overall cancer risks were 1.15 (SIR 1.15; 1.09–1.22; p < 0.001) and 0.91 (SIR 0.91; 0.72–1.14; p = 0.402) in RA populations using conventional synthetic DMARDs (csDMARDs) and biologic DMARDs (bDMARDs), respectively. RA patients taking csDMARDs displayed a 1.77-fold lung cancer risk (SIR 1.77; 1.50–2.09; p < 0.001), a 2.15-fold lymphoma risk (SIR 2.15; 1.78–2.59; p < 0.001), and a 1.72-fold melanoma risk (SIR 1.72; 1.26–2.36; p = 0.001). Correlation coefficients between TMBs and SIRs were 0.22 and 0.29 from those taking csDMARDs and bDMARDs, respectively.
Conclusion: We demonstrated a cancer risk spectrum of RA populations using DMARDs. Additionally, TMBs were not associated with elevated cancer risks in RA patients following immunosuppressive therapy, which confirmed that iatrogenic immunosuppression might not increase cancer risks in patients with RA.
Interpretation: Changes were similar in cancer risk after different immunosuppressive treatments, and there was a lack of correlation between SIRs and TMBs. These suggest that we should look for causes of increased risks from the RA disease itself, rather than using different types of DMARDs.
RA is a chronic persistent autoimmune illness with an uncertain etiology (1). It is characterized by progressive joint injury and extra-articular manifestations, resulting in various clinical symptoms and injuries, even causing permanent disability (2). The global prevalence of RA is 0.27% according to the 2017 Global Burden of Disease Study. The age-standardized prevalence of RA in North America and Western Europe reaches 0.38 and 0.35%, respectively. The prevalence is also higher in India and South American countries (3). In addition, its impact on the female population is particularly severe (4). Over the past few decades, the improvement of the standard of RA treatment has improved the response rate and the quality of life for RA patients. However, RA populations’ rising rates of comorbidity and related mortality from cardiovascular disease, infections, and cancers have gained the attention of the public (5–9).
Patients with RA were frequently treated with DMARDs, including csDMARDs and bDMARDs, to relieve the condition (10). These drugs have a slow onset but provide sustained remission of disease activity in patients, fundamentally inhibiting progressive damage to tissues and joints and delaying or halting disease progression (4, 11). DMARDs usually have an immunosuppressive effect and may cause immunosuppression in the human body. However, because immunological disorders are a common symptom of RA, it is unclear how the immune system will respond to csDMARDs and bDMARDs. This has raised concerns about the immune status of RA patients who received immunosuppressive therapies, including infectious burden, vaccination, and malignancy burden (9, 12, 13).
In the past decades, a growing number of studies have focused on the incidence or prevalence of cancer in RA populations. A comprehensive analysis conducted by Simon et al. demonstrated that elevated SIRs of overall cancer in RA patients and SIRs of site-specific cancers were different (14). In solid tumors, lung cancer showed an elevated risk (15), whereas breast cancer demonstrated a decreased risk (16). Increased lymphoma risk was a common finding in hematologic neoplasms (17). All of these suggested that cancer risks were organ specific. However, this organ specificity of cancer was not well interpreted by available studies.
TMB is defined as the utilization of sequencing technology to detect the total number of somatic cell gene coding errors, base replacements, gene insertions, or deletion errors per million bases (18). TMB and cancer type diversity indicates differences in immunogenicity, which is directly tied to the immune system’s capacity to identify tumor cells. TMB may be associated with site-specific cancer risks in RA patients after using immunosuppressants, while the underlying cause is uncertain.
Here, we performed a comprehensive analysis to determine the overall cancer risk of RA patients using various immunosuppressant therapies, as well as the risk of particular site-specific cancers. In addition, we utilized LR to investigate the correlation between corresponding SIRs and TMBs on participants with distinct baseline characteristics. The aims of this study were to explore whether immunosuppressive therapy increased cancer risks in RA populations and to compare characteristics of association through a synthetic analysis of the relationship between different patients and thus to understand how immune system functions better.
We conducted a search of PubMed, EMBASE, Web of Science, and Medline databases up to 1 August 2022. Studies that involved RA patients receiving immunosuppressive therapy were qualified. References from pertinent papers were also checked. When crucial information was lacking, we asked the authors for further information. All search results were assessed in accordance with the PRISMA statement (19). The protocol was registered in the Prospective Register of Systematic Reviews (PROSPERO ID CRD42021232432).
Our analysis comprised studies that satisfied the following criteria: (1) only case–control studies and cohort studies were included, (2) documented at least one site-specific cancer risk in RA patients receiving immunosuppressive therapies, and (3) published or accepted English-language studies can be retrieved from the web database described above, as of August 2022. Studies were excluded for the following reasons: (1) studies not published in English or not retrievable via the aforementioned network databases, (2) SIRs and 95% CIs could not be determined or calculated from the paper, and (3) a deficiency of statistics based on available data.
Three authors (YZ, JL, and PH) independently extracted relevant data meeting the inclusion criteria and addressed any disputes through discussion. The following demographic and clinical characteristics of patients were extracted as outcome data: first author, publication year, region, age characteristic, type of immunosuppressive therapy drugs, number of RA patients who received immunosuppressive therapies, number of studies on various cancers, and SIRs in various cancers after immunosuppressive treatment was recorded.
Using CGP tests, Chalmers et al. (18) found the distribution of TMB in 100,000 cancer cases in a heterogeneous cohort study and confirmed that TMB was associated with somatic alterations in more than 100 different types of tumors. Chalmers et al. (18) also established pertinent median TMB values for tumors in different organs (Supplementary Table S1). If the median TMB for a particular tumor was not available, it was calculated by averaging TMB values of subtypes that were included in the study (NHL, pancreas, ovary, small intestine, brain and central nervous system, colorectum, soft tissue, urethra, skin, and lung). The detected median TMB and their natural logarithms are displayed in Supplementary Tables S1, S2.
The Newcastle–Ottawa Scale (NOS) criteria (20) were used to assess the methodological quality of selected trials, including selection (four items), comparability (one item), and outcome (three items), presented in Supplementary Table S3. Any disputes were settled through consultation.
We extracted SIRs published in each study and their 95% CIs and pooled cancer risks of RA patients who received immunosuppressive therapy (csDMARDs or bDMARDs). A randomized effect model was employed to synthesize SIRs and 95% CIs in RA patients receiving immunosuppressive therapy (21, 22). The synthesized SIRs were divided into six modules by anatomical site or histology: respiratory system cancers, hematological malignancies, cutaneous system cancers, reproductive and urinary organ system cancers, digestive system cancers, and other malignancies. Cochran’s Q test and the I (2) statistic were adopted to assess the heterogeneity across studies (23). When the I (2) statistic >50%, statistical heterogeneity was deemed significant (23). In addition, subgroup analyses by geographic region (North America, Europe, Oceania, and Asia) and age (55 and 60 years served as cutoff points) were undertaken to assess possible correlations between included studies’ varied features. To determine if any study had a substantial impact on the outcomes, pooled-effect estimates were put through sensitivity analyses by removing each study. Publication biases were evaluated statistically by running Funnel plot tests and Egger’s test (24).
Moreover, we utilized the LR method to determine correlation coefficients and to investigate connections between TMBs and comprehensive SIRs. Since neither TMBs nor SIRs were normally distributed, we used the natural logarithm of each variable to compare them. For statistical and LR analysis, STATA 15.0 software (STATA Corp, College Station, TX, USA) was applied. Every p-value had a two-tailed distribution, and a p-value of 0.05 or lower was regarded as statistically significant.
Funders were not involved in decisions regarding study design, data collection, data synthesis and analysis, manuscript writing, or submission of articles for publication.
We identified 2,091 studies through database search, in addition to identifying two additional studies by other sources. After removing duplicates, 583 studies remained. Ultimately, 22 studies (15, 25–39) met the requirements for inclusion, which involved a total of 371,311 RA patients receiving immunosuppressive therapy and covered 36 types of site-specific cancers. Supplementary Table S3 provides more information on the included studies. Among these studies, 17 studies (15, 17, 25, 26, 28, 29, 31–34, 39–42) provided SIRs of multiple cancers in RA patients receiving immunosuppressive therapies [7 for multiple DMARDs (15, 25, 29, 31, 39, 41, 43), 5 for csDMARDs (17, 28, 33, 38, 42), and 5 for bDMARDs (26, 32, 34, 37, 40)]. The remaining five studies (27, 30, 35, 36, 44) provided SIRs for several or individual cancer risks of RA patients who received immunosuppressive therapies (Figure 1).
Figure 1 PRISMA diagram of study selection (available at https://prisma-statement.org/prismastatement/flowdiagram.aspx).
Firstly, in comparison with the general population, RA patients treated with csDMARDs had a 1.15-fold increased cancer risk (SIR 1.15; 1.09–1.22; p < 0.001). In addition, some elevated significant site-specific cancer risks were shown, such as lung cancer (SIR 1.77; 1.50–2.09; p < 0.001), lymphoma (SIR 2.15; 1.78–2.59; p < 0.001), melanoma (SIR 1.72; 1.26–2.36; p = 0.001), and NMSC (SIR 1.50; 1.10-2.06; p = 0.011). Secondly, RA patients taking bDMARDs did not have obvious increased cancer risks than the general population. However, among these patients, lymphoma (SIR 4.19; 2.51-7.00; p < 0.001) and NMSC (SIR 1.61; 1.34-1.96; p < 0.001) demonstrated raised cancer risks. SIRs of each site-specific cancer for csDMARDs or bDMARDs are listed in Table 1.
Table 1 SIRs of all-cancer and cancer types by anatomical site or histology among RA patients with different DMARDs.
We found no evident correlation between TMBs and SIRs in RA patients who received csDMARDs (p < 0.001) or bDMARDs (p < 0.001). Correlation coefficients between SIRs and TMBs were 0.22 and 0.29, respectively, in RA patients using csDMARDs or bDMARDs (Figure 2).
Figure 2 Correlation between Tumor Mutational Burdens and Standardized Incidence Ratios in RA patients taking CSDMARDs or bDMARDs. Data on the x and y axis are shown on a logarithmic scale.
Results of sensitivity analyses are displayed in Supplementary Figures S2, S3. Our findings demonstrated that pooled outcomes for RA patients utilizing csDMARDs were not greatly changed by excluding any one trial. However, there were some differences in results for RA patients using bDMARDs, which is possibly caused by the limited quantity of included studies.
We conducted exploratory subgroup analyses by region and age. The results of the subgroup analyses revealed that I² values decreased when stratified by region in included studies, indicating that region was the source of apparent heterogeneity in our study. However, we found no considerable reduction in heterogeneity in each group in exploratory subgroup analyses using the cutoff ages of 55 and 60 years. Supplementary Figure S4 presented forest plots of subgroup analyses.
Owing to the limited characteristics collected in RA patients, we could not detect sources other than region and age that contributed to manifest heterogeneity. Our findings of Egger’s test showed no publication bias for all cancers examined in RA patients taking csDMARDs. However, publication bias existed in several cancers for RA patients using bDMARDs, which is possibly induced by the small number of included studies (Supplementary Figure S5). Furthermore, we created funnel plots to evaluate bias, and the outcomes are displayed in Supplementary Figure S6.
Our study presented the profile of overall and site-specific cancer risks in RA patients using immunosuppressive therapy in contrast to the general population. Subgroup analysis revealed an obvious reduction in overall cancer risk after immunosuppressive therapy when divided by geographic region. However, the difference in overall cancer risk after immunosuppressive therapy was not reduced when stratified by age (55 and 60 years, respectively), suggesting that age of onset of RA patients may not be the main source of heterogeneity in our study. For the relationship between SIRs and TMBs, the correlation coefficient was 0.22 (csDMARDs) and 0.29 (bDMARDs), indicating that varied cancer incidence in RA patients was extremely weakly related to the use of immunosuppressants, and the two could be considered uncorrelated.
Overall cancer risk was increased in RA patients using csDMARDs, while those using bDMARDs did not indicate the same result. Furthermore, lung cancer risk was increased in RA patients using csDMARDs. It is more interesting to note that those receiving csDMARDs or bDMARDs both had high risks of having hematological and integumentary system cancer.
According to estimates, smoking caused up to 85% of lung cancer cases (45). Tobacco use also had been found to enhance RA risk by 40% (46). Therefore, smoking might be a common risk factor for lung cancer and RA in certain populations (47). Previous meta-analyses (14, 48) have reported that RA may be one of the risk factors for lung cancer. A pooled analysis and Mendelian randomization study (49) found that RA was associated with a 44% increased risk of lung cancer (RR 1.44; 1.31–1.57). Some recently published studies were included in our study, which has a larger sample size and greater statistical power. Our findings suggested that RA patients using DMARDS also had a significantly higher risk of having lung cancer. In addition, pulmonary involvement in RA is the most common extra-articular manifestation, occurring in up to 60% (50–53). RA-related interstitial lung disease is the most challenging complication of lung manifestation (54). It leads to pathological epithelial damage, repair abnormalities, and epithelial–mesenchymal transformation, which may eventually lead to cancerous transformation (55).
Although the exact mechanism by which RA altered lung cancer risk is still uncertain, there have been several molecular pathways underlying RA and lung cancer. The cyclooxygenase (COX)-2/thromboxane A2 (TxA2) pathway has been demonstrated to play a potential role in lung cancer development through an auto-regulatory feedback loop (56). MAP4K3 (also known as GLK) is a serine/threonine kinase that directly interacts with and activates PKCu, resulting in the activation of IKK/NF-kB in human T cells (57). Overexpression of GLK in T cells is a key causative factor in the development of autoimmune diseases (58). Meanwhile, in cancer cells, GLK directly phosphorylates and activates IQGAP1, resulting in the induction of Cdc42-mediated cell migration and cancer metastasis (59). In summary, the bridge from RA to lung cancer still needs to be studied further.
In previous studies on RA (60–62) versus cancer, incidence of blood malignancies showed high-risk results, which is consistent with our findings. In 2005, Zintzaras et al. explored the correlation between RA and NHL through a pooled analysis of observational studies (63). They described that the SIR of RA with lymphoma was 3.9 (95% CI: 2.5–5.9). Our study confirmed the results of Zintzaras and colleagues. Moreover, we further concluded that RA patients treated with csDMARDs or bDMARDs had a 2.15-fold and 4.19-fold risk of lymphoma, respectively. Notably, RA itself, a representative chronic inflammatory disease, was one of the triggers for the generation of lymphoma. Conversion of lymphocytes into malignant clones was a multi-step process involving chronic stimulation with various antigens and/or viruses, such as Epstein–Barr virus, local inflammation, and immunosuppression. These could weaken immune protection and increase the risk of lymphoma (64, 65). As for integumentary system cancers, previous studies (15, 17, 27, 29, 31, 66–68) have indicated that RA patients using csDMARDs and bDMARDs had a 20%–80% increased risk of NMSC in contrast to the general population. In our study, non-melanoma risk increased in RA patients using csDMARDs and bDMARDs, as were melanoma risk in RA patients using csDMARDs. However, the number of studies using bDMARDs for RA alone was insufficient, and its relation with melanoma risk remains to be confirmed.
TMB is a promising biomarker that can be used to predict the efficacy of tumors on ICIs (18). In 2017, Elizabeth Marion Jaffee et al. (69) plotted a linear plot between the median TMB of 27 tumors and the ORR of ICI treatment (analytical data came from multiple clinical studies), in which TMB was positively correlated to ORR (p < 0.001) and the correlation coefficient was 0.74. Additionally, to a certain degree, TMB reflects immunogenicity. TMB refers to the number of somatic mutations after the tumor genome removes the germline mutation; a large number of mutated somatic cells will produce a large number of altered peptides, some of which are successfully expressed and processed by the major histocompatibility complex, thereby producing new antigens recognized by the human immune system, which can cause anti-tumor responses to mutated somatic cells through specific killing. This makes mutated somatic cells more likely to be targeted by activated immune cells (18). Therefore, when the immune system is in a normal state, malignant tumor cells with high TMB values are more easily recognized and cleared by the body’s immune system during the embryonic stage. However, the use of immunosuppressants diminishes the role of the human immune system in immune surveillance. This results in an increased survival rate of malignant tumors with high TMB values, which may ultimately result in higher rates of overall and site-specific cancers in a segment of the population.
In 2020, Huo et al. (70) undertook a comprehensive study of cancer risks for recipients of solid organ transplantation and then plotted a linear plot between TMBs of site-specific malignancies and cancer combined SIRs of solid organ transplant recipients. They found a remarkable positive relationship between TMBs and SIRs (p < 0.001) with a correlation coefficient of 0.68, indicating that an increase in cancer incidence was related to immunosuppression. Our study also used LR to explore the correlation between TMB and the corresponding cancer incidence. However, our study found that both RA patients treated with csDMARDs and bDMARDs had low coefficients (csDMARDs r = 0.22, bDMARDs r = 0.29) of correlation between TMB and the corresponding cancer incidence line. This partly demonstrated that the risk of organ-specific cancer in RA patients was not related to DMARDs use, which reminded us to explore the potential mechanism of cancer risks from the RA disease itself. Additionally, some immunomodulatory agents used in the clinical treatment of different autoimmune disorders have been shown to increase Treg frequency and susceptibility to Treg suppression and to restore the balance between immunosuppression and immune activation, so as to achieve the purpose of treatment (71–76). Our pooled analysis and correlation analysis of TMBs and SIRs preliminarily indicated that changes of cancer risks in RA patients were not primarily dependent on immunosuppressive effects after DMARDs. However, exact factors contributing to changes in cancer risks are still uncertain.
The three main strengths of our study should be emphasized. First and foremost, to the best of our knowledge, this study is the most pooled study in the world to evaluate cancer risks in RA patients using different kinds of immunosuppressants. Secondly, the study explored the relationship between SIRs of corresponding malignant tumors and their TMBs, investigated the cancer risk of RA patients from a specific immunological perspective through fitting analysis, and clarified that changes in cancer risks were not due to the use of DMARDs. Lastly, previously published meta-analyses were restricted to specific organs, specific malignancies, individual countries, individual regions, or small sample sizes. The study gathered a large sample of data from around the world and assessed cancer risks in RA patients treated with DMARDs. These global data, along with our findings, can provide a strong reference for clinicians and researchers on the risks of immunosuppressive therapy in patients with RA, as well as reliable evidence-based medical evidence for primary prevention of certain cancers in RA patients.
Despite the above advantages of our study, there are some limitations to this comprehensive analysis. First, there is a strong heterogeneity between involved studies, which may be due to the following reasons: (1) differences in tumor characteristics of malignancies were incorporated; (2) few studies provided detailed information on BMI (77), smoking status (78), alcohol consumption (79), and immunosuppressant drug dosages to adjust for these potential confounding factors; and (3) reference materials of included studies are all general populations, but countries and regions of studies are different, resulting in different final matching criteria. Therefore, we conducted subgroup analyses based on the populations’ age included in the study as well as regional distribution to improve stability of results, but the study is still only a preliminary observation of cancer risks in RA patients with immunosuppressant therapy. Second, the involved studies have used different age and sex ratios for the standardization of SIR, which may have some effect on fitting results. Third, due to the limited number of studies using bDMARDs, there was a publication bias in sensitivity analysis and Egger’s test for certain site-specific cancers. Fourth, the TMB algorithm used in our study was relatively basic, and findings should be interpreted with caution. Because we cannot directly use TMB in the study of Chalmers et al. (18), we replaced TMB by averaging cancer subtypes mentioned in that study. Therefore, in order to increase the reliability of analysis associations, more precise TMBs should be determined according to the proportion of tumor pathological typing. Last but not least, most studies did not specify the dosage of DMARDs used, limiting our ability to explain whether potential adverse effects caused by patient medications impacted cancer risks in RA patients through subgroup analysis.
The number of studies on the risk of cancers after DMARDs is far from adequate. Thus, future iterations of the evaluation for cancer risks require more relevant clinical observations. In addition, in subsequent clinical evidence, we also hope that more studies would record the timing of cancer onset, specific drug names and doses, duration of drug treatment, and final efficacy in detail, which would provide more evidence for further syntheses. In the later stages, we will continue to pay attention to new research in this field and strive to collect more adequate and specific data, aiming to demonstrate a better cancer risk landscape for RA patients.
Comprehensive analysis indicated that RA patients using DMARDs (only csDMARDs or bDMARDs, not suitable for csDMARDs combined with bDMARDs) had elevated risks of lung and renal cancer, but reduced risks of breast and endometrial cancer.
The higher risks were also shown in cancers of the hematologic and cutaneous system (different malignancies at different risks). In addition, TMBs were not associated with elevated cancer risks in RA patients following immunosuppressive therapy. These relationships provided clinicians guidance on the individualized treatment of RA patients and the prevention of site-specific malignancies.
The original contributions presented in the study are included in the article/Supplementary Material. Further inquiries can be directed to the corresponding authors.
This study has been approved by Institutional Review Board (IRB) of Guangdong Provincial People’s Hospital. Because the study is a retrospective analysis, the ethics committee waived informed consent for all patients.
This study was proposed by YZ. YZ, JRL and PH extracted data. YZ, RGR and ZX analyzed data. YZ wrote the initial draft manuscript. JPL and PH revised the original text and carried out English editing. ZH and GQ revised the final manuscript. All authors have contributed to the revision of the manuscript. YZ is the guarantor. All authors contributed to the article and approved the submitted version.
This work was supported by a grant from the 2020–2021 Popularization of Science and Technology Innovation Special Project of Guangdong Province of China (2020A1414070007); the Science and Technology Program of Guangzhou, China (202206010103); and the Natural Science Foundation of Guangdong Province (2022A1515012469).
The authors appreciate Qinyi Xu (MA, College of Comparative Law, China University of Political Science and Law) for editing the manuscript.
The authors declare that the research was conducted in the absence of any commercial or financial relationships that could be construed as a potential conflict of interest.
All claims expressed in this article are solely those of the authors and do not necessarily represent those of their affiliated organizations, or those of the publisher, the editors and the reviewers. Any product that may be evaluated in this article, or claim that may be made by its manufacturer, is not guaranteed or endorsed by the publisher.
The Supplementary Material for this article can be found online at: https://www.frontiersin.org/articles/10.3389/fimmu.2022.1050876/full#supplementary-material
CI, confidence interval; RA, rheumatoid arthritis; TMB, tumor mutational burden; SIR, standardized incidence ratio; LR, linear regression; MARD, disease-modifying anti-rheumatic drug; csDMARD, conventional synthetic DMARD; bDMARD, biologic DMARD; NHL, non-Hodgkin lymphoma; HL, Hodgkin lymphoma; NMSC, non-melanoma skin cancer; SCC, squamous cell carcinoma; BCC, basal cell carcinoma; RR, relative risk; ORR, objective response rate; Treg, regulatory T cells; Teff, T effector cells; CGP, comprehensive genomic profiling; ICI, immune checkpoint inhibitor; PRISMA, Preferred Reporting Items for Systematic Reviews and Meta‐Analysis; BMI, body mass index.
1. Scott DL, Wolfe F, Huizinga TWJ. Rheumatoid arthritis. Lancet (2010) 376:1094–108. doi: 10.1016/S0140-6736(10)60826-4
2. Smolen JS. Insights into the treatment of rheumatoid arthritis: A paradigm in medicine. J Autoimmun (2020) 110:102425. doi: 10.1016/j.jaut.2020.102425
3. James SLG, Abate D, Abate KH, Abay SM, Abbafati C, Abbasi N, et al. Global, regional, and national incidence, prevalence, and years lived with disability for 354 diseases and injuries for 195 countries and territories, 1990-2017: A systematic analysis for the global burden of disease study 2017. Lancet (2018) 392:1789–858. doi: 10.1016/S0140-6736(18)32279-7
4. Smolen JS, Aletaha D, McInnes IB. Rheumatoid arthritis. Lancet (2016) 388:2023–38. doi: 10.1016/S0140-6736(16)30173-8
5. Avina-Zubieta JA, Thomas J, Sadatsafavi M, Lehman AJ, Lacaille D. Risk of incident cardiovascular events in patients with rheumatoid arthritis: A meta-analysis of observational studies. Ann Rheum Dis (2012) 71:1524–9. doi: 10.1136/annrheumdis-2011-200726
6. England BR, Thiele GM, Anderson DR, Mikuls TR. Increased cardiovascular risk in rheumatoid arthritis: mechanisms and implications. BMJ (2018) 361:k1036. doi: 10.1136/bmj.k1036
7. Lortholary O, Fernandez-Ruiz M, Baddley JW, Manuel O, Mariette X, Winthrop KL, et al. Infectious complications of rheumatoid arthritis and psoriatic arthritis during targeted and biological therapies: a viewpoint in 2020. Ann Rheum Dis (2020) 79:1532–43. doi: 10.1136/annrheumdis-2020-217092
8. Galloway JB, Hyrich KL, Mercer LK, Dixon WG, Fu B, Ustianowski AP, et al. Anti-TNF therapy is associated with an increased risk of serious infections in patients with rheumatoid arthritis especially in the first 6 months of treatment: Updated results from the British society for rheumatology biologics register with special emphasis on risks in the elderly. Rheumatol (Oxford) (2011) 50:124–31. doi: 10.1093/rheumatology/keq242
9. Cho S-K, Lee J, Han M, Bae S-C, Sung Y-K. The risk of malignancy and its incidence in early rheumatoid arthritis patients treated with biologic DMARDs. Arthritis Res Ther (2017) 19:277. doi: 10.1186/s13075-017-1482-y
10. Lin Y-J, Anzaghe M, Schülke S. Update on the pathomechanism, diagnosis, and treatment options for rheumatoid arthritis. Cells (2020) 9. doi: 10.3390/cells9040880
11. Aletaha D, Smolen JS. Diagnosis and management of rheumatoid arthritis: A review. JAMA (2018) 320:1360–72. doi: 10.1001/jama.2018.13103
12. Yun H, Xie F, Delzell E, Chen L, Levitan EB, Lewis JD, et al. Risk of hospitalised infection in rheumatoid arthritis patients receiving biologics following a previous infection while on treatment with anti-TNF therapy. Ann Rheum Dis (2015) 74:1065–71. doi: 10.1136/annrheumdis-2013-204011
13. Park JK, Lee YL, Shin K, Kang EH, Ha Y-J, Park JW, et al. The impact of temporary methotrexate discontinuation for 1 week versus 2 weeks on seasonal influenza vaccination in patients with rheumatoid arthritis. Arthritis Rheumatol (2022). doi: 10.1002/art.42318
14. Simon TA, Thompson A, Gandhi KK, Hochberg MC, Suissa S. Incidence of malignancy in adult patients with rheumatoid arthritis: a meta-analysis. Arthritis Res Ther (2015) 17:212. doi: 10.1186/s13075-015-0728-9
15. Dreyer L, Mellemkjær L, Andersen AR, Bennett P, Poulsen UE, Ellingsen TJ, et al. Incidences of overall and site specific cancers in TNFα inhibitor treated patients with rheumatoid arthritis and other arthritides - a follow-up study from the DANBIO registry. Ann Rheum Dis (2013) 72:79–82. doi: 10.1136/annrheumdis-2012-201969
16. Wadström H, Pettersson A, Smedby KE, Askling J. Risk of breast cancer before and after rheumatoid arthritis, and the impact of hormonal factors. Ann Rheum Dis (2020) 79:581–6. doi: 10.1136/annrheumdis-2019-216756
17. Mercer LK, Lunt M, Low ALS, Dixon WG, Watson KD, Symmons DPM, et al. Risk of cancer in patients receiving non-biologic disease-modifying therapy for rheumatoid arthritis compared with the UK general population. Rheumatol (Oxford) (2013) 52:91–8. doi: 10.1093/rheumatology/kes350
18. Chalmers ZR, Connelly CF, Fabrizio D, Gay L, Ali SM, Ennis R, et al. Analysis of 100,000 human cancer genomes reveals the landscape of tumor mutational burden. Genome Med (2017) 9:34. doi: 10.1186/s13073-017-0424-2
19. Moher D, Liberati A, Tetzlaff J, Altman DG. Preferred reporting items for systematic reviews and meta-analyses: the PRISMA statement. PLoS Med (2009) 6:e1000097. doi: 10.1371/journal.pmed.1000097
20. Wells G, Shea B, O’Conell D, Peterson J, Welch V, Losos M, et al. The Newcastle-Ottawa scale (NOS) for assessing the quality of non randomised studies in meta-analyses. (2001).
21. DerSimonian R, Laird N. Meta-analysis in clinical trials. (1986) 7:177–88. doi: 10.1016/0197-2456(86)90046-2
22. Ades A, Lu G, Higgins J. The interpretation of random-effects meta-analysis in decision models. (2005) 25:646–54. doi: 10.1177/0272989X05282643
23. Higgins JPT, Thompson SG, Deeks JJ, Altman DG. Measuring inconsistency in meta-analyses. BMJ (2003) 327:557–60. doi: 10.1136/bmj.327.7414.557
24. Egger M, Smith GD, Schneider M, Minder C. Bias in meta-analysis detected by a simple, graphical test. (1997) 315:629–34. doi: 10.1136/bmj.315.7109.629
25. Geborek P, Bladström A, Turesson C, Gulfe A, Petersson IF, Saxne T, et al. Tumour necrosis factor blockers do not increase overall tumour risk in patients with rheumatoid arthritis, but may be associated with an increased risk of lymphomas. Ann Rheum Dis (2005) 64:699–703. doi: 10.1136/ard.2004.030528
26. Dreyer L, Cordtz RL, Hansen IMJ, Kristensen LE, Hetland ML, Mellemkjaer L, et al. Risk of second malignant neoplasm and mortality in patients with rheumatoid arthritis treated with biological DMARDs: A Danish population-based cohort study. Ann Rheum Dis (2018) 77:510–4. doi: 10.1136/annrheumdis-2017-212086
27. Mercer LK, Green AC, Galloway JB, Davies R, Lunt M, Dixon WG, et al. The influence of anti-TNF therapy upon incidence of keratinocyte skin cancer in patients with rheumatoid arthritis: Longitudinal results from the British society for rheumatology biologics register. Ann Rheum Dis (2012) 71:869–74. doi: 10.1136/annrheumdis-2011-200622
28. Ekström K, Hjalgrim H, Brandt L, Baecklund E, Klareskog L, Ekbom A, et al. Risk of malignant lymphomas in patients with rheumatoid arthritis and in their first-degree relatives. Arthritis Rheum (2003) 48:963–70. doi: 10.1002/art.10939
29. Wolfe F, Michaud K. Biologic treatment of rheumatoid arthritis and the risk of malignancy: analyses from a large US observational study. Arthritis Rheum (2007) 56:2886–95. doi: 10.1002/art.22864
30. Raaschou P, Simard JF, Asker Hagelberg C, Askling J. Rheumatoid arthritis, anti-tumour necrosis factor treatment, and risk of squamous cell and basal cell skin cancer: cohort study based on nationwide prospectively recorded data from Sweden. BMJ (2016) 352:i262. doi: 10.1136/bmj.i262
31. Askling J, Fored CM, Brandt L, Baecklund E, Feltelus LBN, et al. Risks of solid cancers in patients with rheumatoid arthritis and after treatment with tumour necrosis factor antagonists. Ann Rheum Dis (2005) 64:1421–6. doi: 10.1136/ard.2004.033993
32. Pallavicini FB, Caporali R, Sarzi-Puttini P, Atzeni F, Bazzani C, Gorla R, et al. Tumour necrosis factor antagonist therapy and cancer development: Analysis of the LORHEN registry. Autoimmun Rev (2010) 9:175–80. doi: 10.1016/j.autrev.2009.07.006
33. Abásolo L, Júdez E, Descalzo MA, González-Alvaro I, Jover JA, Carmona L, et al. Cancer in rheumatoid arthritis: Occurrence, mortality, and associated factors in a south European population. Semin Arthritis Rheum (2008) 37:388–97. doi: 10.1016/j.semarthrit.2007.08.006
34. Solomon DH, Kremer JM, Fisher M, Curtis JR, Furer V, Harrold LR, et al. Comparative cancer risk associated with methotrexate, other non-biologic and biologic disease-modifying anti-rheumatic drugs. Semin Arthritis Rheum (2014) 43:489–97. doi: 10.1016/j.semarthrit.2013.08.003
35. Calip GS, Patel PR, Adimadhyam S, Xing S, Wu Z, Sweiss K, et al. Tumor necrosis factor-alpha inhibitors and risk of non-Hodgkin lymphoma in a cohort of adults with rheumatologic conditions. Int J Cancer (2018) 143:1062–71. doi: 10.1002/ijc.31407
36. Amari W, Zeringue AL, McDonald JR, Caplan L, Eisen SA, Ranganathan P, et al. Risk of non-melanoma skin cancer in a national cohort of veterans with rheumatoid arthritis. Rheumatol (Oxford) (2011) 50:1431–9. doi: 10.1093/rheumatology/ker113
37. Waljee AK, Higgins PDR, Jensen CB, Villumsen M, Cohen-Mekelburg SA, Wallace BI, et al. Anti-tumour necrosis factor-α therapy and recurrent or new primary cancers in patients with inflammatory bowel disease, rheumatoid arthritis, or psoriasis and previous cancer in Denmark: A nationwide, population-based cohort study. Lancet Gastroenterol Hepatol (2020) 5:276–84. doi: 10.1016/S2468-1253(19)30362-0
38. Lim XR, Xiang W, Tan JWL, Koh LW, Lian TY, Leong KP, et al. Incidence and patterns of malignancies in a multi-ethnic cohort of rheumatoid arthritis patients. Int J Rheum Dis (2019) 22:1679–85. doi: 10.1111/1756-185X.13655
39. Wu C-Y, Chen D-Y, Shen J-L, Ho HJ, Chen C-C, Kuo KN, et al. The risk of cancer in patients with rheumatoid arthritis taking tumor necrosis factor antagonists: a nationwide cohort study. Arthritis Res Ther (2014) 16:449. doi: 10.1186/s13075-014-0449-5
40. Harigai M, Nanki T, Koike R, Tanaka M, Watanabe-Imai K. Risk for malignancy in rheumatoid arthritis patients treated with biological disease-modifying antirheumatic drugs compared to the general population: A nationwide cohort study in Japan. Mod Rheumatol (2016) 26:642–50. doi: 10.3109/14397595.2016.1141740
41. Setoguchi S, Solomon DH, Weinblatt ME, Katz JN, Avorn J, Glynn RJ, et al. Tumor necrosis factor alpha antagonist use and cancer in patients with rheumatoid arthritis. Arthritis Rheum (2006) 54:2757–64. doi: 10.1002/art.22056
42. Buchbinder R, Barber M, Heuzenroeder L, Wluka AE, Giles G, Hall S, et al. Incidence of melanoma and other malignancies among rheumatoid arthritis patients treated with methotrexate. Arthritis Rheum (2008) 59:794–9. doi: 10.1002/art.23716
43. Cordtz R, Mellemkjær L, Glintborg B, Hetland ML, Madsen OR, Hansen IMJ, et al. Risk of virus-associated cancer in female arthritis patients treated with biological DMARDs-a cohort study. Rheumatol (Oxford) (2016) 55:1017–22. doi: 10.1093/rheumatology/kew012
44. Askling J, Fored CM, Baecklund E, Brandt L, Backlin C, Ekbom A, et al. Haematopoietic malignancies in rheumatoid arthritis: lymphoma risk and characteristics after exposure to tumour necrosis factor antagonists. Ann Rheum Dis (2005) 64:1414–20. doi: 10.1136/ard.2004.033241
45. Warren GW, Cummings KM. Tobacco and lung cancer: risks, trends, and outcomes in patients with cancer. Am Soc Clin Oncol Educ Book (2013), 359–64. doi: 10.1200/EdBook_AM.2013.33.359
46. Sugiyama D, Nishimura K, Tamaki K, Tsuji G, Nakazawa T, Morinobu A, et al. Impact of smoking as a risk factor for developing rheumatoid arthritis: a meta-analysis of observational studies. Ann Rheum Dis (2010) 69:70–81. doi: 10.1136/ard.2008.096487
47. Peacock A, Leung J, Larney S, Colledge S, Hickman M, Rehm J, et al. Global statistics on alcohol, tobacco and illicit drug use: 2017 status report. Addiction (2018) 113:1905–26. doi: 10.1111/add.14234
48. Smitten AL, Simon TA, Hochberg MC. & suissa, s. a meta-analysis of the incidence of malignancy in adult patients with rheumatoid arthritis. Arthritis Res Ther (2008) 10:R45. doi: 10.1186/ar2404
49. Wu X, Peng H, Wen Y, Cai X, Li C, Zhong R, et al. Rheumatoid arthritis and risk of lung cancer: Meta-analysis and mendelian randomization study. Semin Arthritis Rheum (2021) 51:565–75. doi: 10.1016/j.semarthrit.2021.03.015
50. Hunter TM, Boytsov NN, Zhang X, Schroeder K, Michaud K, Araujo AB, et al. Prevalence of rheumatoid arthritis in the united states adult population in healthcare claims databases, 2004-2014. Rheumatol Int (2017) 37:1551–7. doi: 10.1007/s00296-017-3726-1
51. Myasoedova E, Crowson CS, Kremers HM, Therneau TM, Gabriel SE. Is the incidence of rheumatoid arthritis rising?: results from Olmsted county, Minnesota, 1955-2007. Arthritis Rheum (2010) 62:1576–82. doi: 10.1002/art.27425
52. Wilsher M, Voight L, Milne D, Teh M, Good N, Kolbe J, et al. Prevalence of airway and parenchymal abnormalities in newly diagnosed rheumatoid arthritis. Respir Med (2012) 106:1441–6. doi: 10.1016/j.rmed.2012.06.020
53. Norton S, Koduri G, Nikiphorou E, Dixey J, Williams P, Young A, et al. A study of baseline prevalence and cumulative incidence of comorbidity and extra-articular manifestations in RA and their impact on outcome. Rheumatol (Oxford) (2013) 52. doi: 10.1093/rheumatology/kes262
54. Wang D, Zhang J, Lau J, Wang S, Taneja V, Matteson EL, et al. Mechanisms of lung disease development in rheumatoid arthritis. Nat Rev Rheumatol (2019) 15:581–96. doi: 10.1038/s41584-019-0275-x
55. Khan KA, Kennedy MP, Lee Crush ME, Prendeville S, Maher MM, Declan Burke L, et al. Radiological characteristics, histological features and clinical outcomes of lung cancer patients with coexistent idiopathic pulmonary fibrosis. Lung (2015) 193:71–7. doi: 10.1007/s00408-014-9664-8
56. Huang Q-C, Huang R-Y. The cyclooxygenase-2/thromboxane A2 pathway: a bridge from rheumatoid arthritis to lung cancer? Cancer Lett (2014) 354:28–32. doi: 10.1016/j.canlet.2014.08.024
57. Chuang H-C, Wang X, Tan T-H. MAP4K family kinases in immunity and inflammation. Adv Immunol (2016) 129:277–314. doi: 10.1016/bs.ai.2015.09.006
58. Chen Y-M, Chuang H-C, Lin W-C, Tsai C-Y, Wu C-W, Gong N-R, et al. Germinal center kinase-like kinase overexpression in T cells as a novel biomarker in rheumatoid arthritis. Arthritis Rheum (2013) 65:2573–82. doi: 10.1002/art.38067
59. Chuang H-C, Chang C-C, Teng C-F, Hsueh C-H, Chiu L-L, Hsu P-M, et al. MAP4K3/GLK promotes lung cancer metastasis by phosphorylating and activating IQGAP1. Cancer Res (2019) 79:4978–93. doi: 10.1158/0008-5472.CAN-19-1402
60. Hakulinen T, Isomaki H, Knekt P. Rheumatoid arthritis and cancer studies based on linking nationwide registries in Finland. Am J Med (1985) 78:29–32. doi: 10.1016/0002-9343(85)90242-6
61. Doody MM, Linet MS, Glass AG, Friedman GD, Pottern LM, Boice JD, et al. Leukemia, lymphoma, and multiple myeloma following selected medical conditions. Cancer Causes Control (1992) 3:449–56. doi: 10.1007/BF00051358
62. Symmons DP. Neoplasms of the immune system in rheumatoid arthritis. Am J Med (1985) 78:22–8. doi: 10.1016/0002-9343(85)90241-4
63. Zintzaras E, Voulgarelis M, Moutsopoulos HM. The risk of lymphoma development in autoimmune diseases: a meta-analysis. Arch Intern Med (2005) 165:2337–44. doi: 10.1001/archinte.165.20.2337
64. Starkebaum G. Rheumatoid arthritis and lymphoma: risky business for b cells. J Rheumatol (2007) 34:243–6.
65. Küppers R. B cells under influence: transformation of b cells by Epstein-Barr virus. Nat Rev Immunol (2003) 3:801–12. doi: 10.1038/nri1201
66. Gridley G, McLaughlin JK, Ekbom A, Klareskog L, Adami H-O, Hacker DG, et al. Incidence of cancer among patients with rheumatoid arthritis. J Natl Cancer Inst (1993) 85:307–11. doi: 10.1093/jnci/85.4.307
67. Mellemkjaer L, Linet MS, Gridley G, Frisch M, Moller H, Olsen JH, et al. Rheumatoid arthritis and cancer risk. Eur J Cancer (1996) 32A:1753–7. doi: 10.1016/0959-8049(96)00210-9
68. Chakravarty EF, Michaud K, Wolfe F. Skin cancer, rheumatoid arthritis, and tumor necrosis factor inhibitors. J Rheumatol (2005) 32:2130–5.
69. Yarchoan M, Hopkins A, Jaffee EM. Tumor mutational burden and response rate to PD-1 inhibition. N Engl J Med (2017) 377:2500–1. doi: 10.1056/NEJMc1713444
70. Huo Z, Li C, Xu X, Ge F, Wang R, Wen Y, et al. Cancer risks in solid organ transplant recipients: Results from a comprehensive analysis of 72 cohort studies. Oncoimmunology (2020) 9:1848068. doi: 10.1080/2162402X.2020.1848068
71. Kikuchi J, Hashizume M, Kaneko Y, Yoshimoto K, Nishina N, Takeuchi T, et al. Peripheral blood CD4(+)CD25(+)CD127(low) regulatory T cells are significantly increased by tocilizumab treatment in patients with rheumatoid arthritis: increase in regulatory T cells correlates with clinical response. Arthritis Res Ther (2015) 17:10. doi: 10.1186/s13075-015-0526-4
72. Huang Z, Yang B, Shi Y, Cai B, Li Y, Feng W, et al. Anti-TNF-α therapy improves treg and suppresses teff in patients with rheumatoid arthritis. Cell Immunol (2012) 279:25–9. doi: 10.1016/j.cellimm.2012.09.001
73. Nishimoto N, Kishimoto T. Humanized antihuman IL-6 receptor antibody, tocilizumab. Handb Exp Pharmacol (2008), 151–60. doi: 10.1007/978-3-540-73259-4_7
74. Dominitzki S, Fantini MC, Neufert C, Nikolaev A, Galle PR, Scheller J, et al. Cutting edge: trans-signaling via the soluble IL-6R abrogates the induction of FoxP3 in naive CD4+CD25 T cells. J Immunol (Baltimore Md 1950) (2007) 179:2041–5. doi: 10.4049/jimmunol.179.4.2041
75. Samson M, Greigert H, Ciudad M, Gerard C, Ghesquière T, Trad M, et al. Improvement of treg immune response after treatment with tocilizumab in giant cell arteritis. Clin Trans Immunol (2021) 10:e1332. doi: 10.1002/cti2.1332
76. Feldmann M. Development of anti-TNF therapy for rheumatoid arthritis. Nat Rev Immunol (2002) 2:364–71. doi: 10.1038/nri802
77. Freisling H, Arnold M, Soerjomataram I, O'Doherty MG, Ordóñez-Mena JM, Bamia C, et al. Comparison of general obesity and measures of body fat distribution in older adults in relation to cancer risk: meta-analysis of individual participant data of seven prospective cohorts in Europe. Br J Cancer (2017) 116:1486–97. doi: 10.1038/bjc.2017.106
78. Ordóñez-Mena JM, Schöttker B, Mons U, Jenab M, Freisling H, Bueno-de-Mesquita B, et al. Quantification of the smoking-associated cancer risk with rate advancement periods: meta-analysis of individual participant data from cohorts of the CHANCES consortium. BMC Med (2016) 14:62. doi: 10.1186/s12916-016-0607-5
Keywords: rheumatoid arthritis, cancer risk, immunosuppression therapy, immunosuppressant, tumor mutational burden
Citation: Zhang Y, Lin J, You Z, Tu H, He P, Li J, Gao R, Liu Z, Xi Z, Li Z, Lu Y, Hu Q, Li C, Ge F, Huo Z and Qiao G (2022) Cancer risks in rheumatoid arthritis patients who received immunosuppressive therapies: Will immunosuppressants work? Front. Immunol. 13:1050876. doi: 10.3389/fimmu.2022.1050876
Received: 22 September 2022; Accepted: 29 November 2022;
Published: 20 December 2022.
Edited by:
Yutian Zou, Sun Yat-sen University Cancer Center (SYSUCC), ChinaReviewed by:
Sohini Chakraborty, New York University, United StatesCopyright © 2022 Zhang, Lin, You, Tu, He, Li, Gao, Liu, Xi, Li, Lu, Hu, Li, Ge, Huo and Qiao. This is an open-access article distributed under the terms of the Creative Commons Attribution License (CC BY). The use, distribution or reproduction in other forums is permitted, provided the original author(s) and the copyright owner(s) are credited and that the original publication in this journal is cited, in accordance with accepted academic practice. No use, distribution or reproduction is permitted which does not comply with these terms.
*Correspondence: Guibin Qiao, Z3VpYmlucWlhb0AxMjYuY29t; Fan Ge, MjAwMEAxNjMuY29t; Zhenyu Huo, aGpjNDQ4NUAxMjYuY29t
†These authors share first authorship
Disclaimer: All claims expressed in this article are solely those of the authors and do not necessarily represent those of their affiliated organizations, or those of the publisher, the editors and the reviewers. Any product that may be evaluated in this article or claim that may be made by its manufacturer is not guaranteed or endorsed by the publisher.
Research integrity at Frontiers
Learn more about the work of our research integrity team to safeguard the quality of each article we publish.