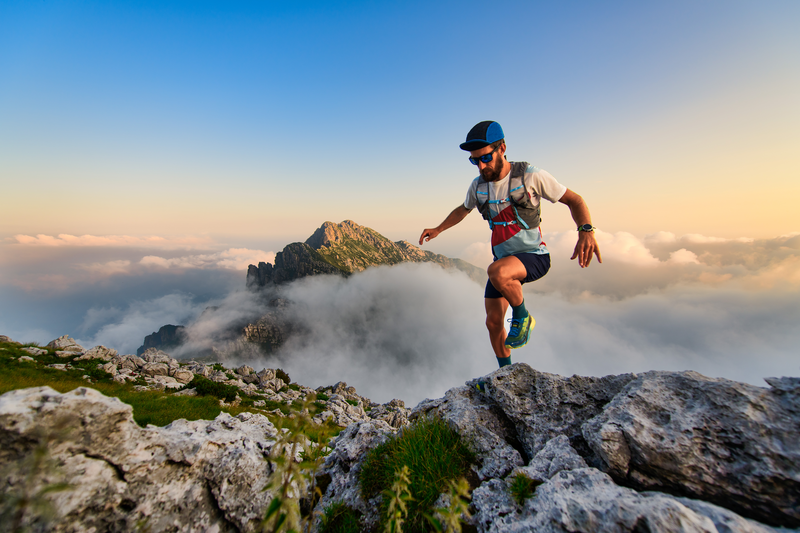
94% of researchers rate our articles as excellent or good
Learn more about the work of our research integrity team to safeguard the quality of each article we publish.
Find out more
REVIEW article
Front. Immunol. , 28 October 2022
Sec. Cancer Immunity and Immunotherapy
Volume 13 - 2022 | https://doi.org/10.3389/fimmu.2022.1049812
This article is part of the Research Topic Biomarkers and Immunotherapy of Hepatic-Biliary-Pancreatic Cancers View all 53 articles
Biliary tract cancers (BTCs), including cholangiocarcinoma and gallbladder carcinoma, originate from the biliary epithelium and have a poor prognosis. Surgery is the only choice for cure in the early stage of disease. However, most patients are diagnosed in the advanced stage and lose the chance for surgery. Early diagnosis could significantly improve the prognosis of patients. Bile has complex components and is in direct contact with biliary tract tumors. Bile components are closely related to the occurrence and development of biliary tract tumors and may be applied as biomarkers for BTCs. Meanwhile, arising evidence has confirmed the immunoregulatory role of bile components. In this review, we aim to summarize and discuss the relationship between bile components and biliary tract cancers and their ability as biomarkers for BTCs, highlighting the role of bile components in regulating immune response, and their promising application prospects.
Biliary tract cancers (BTCs) constitute a diverse group of malignancies emerging from the biliary epithelium, including cholangiocarcinoma and gallbladder carcinoma (1). According to its anatomical site, cholangiocarcinoma can be divided into intrahepatic cholangiocarcinoma(iCCA), perihilar cholangiocarcinoma(pCCA) and distal cholangiocarcinoma(dCCA) (2–4). iCCA, pCCA, and dCCA have distinct clinicopathologic characteristics in terms of epidemiology, molecular characteristics, clinical features, management, and outcomes (5). BTCs account for about 3% of all gastrointestinal tumors, and their incidence has increased in recent years (6). BTCs have an abysmal prognosis, with a five-year survival rate of about 10% (4). Radical resection is the only cure chance for BTCs. However, since patients are usually asymptomatic or only exhibit non-specific symptoms in the early stage, most patients are diagnosed at the advanced stage, missing the opportunity for radical surgery (7). Therefore, early diagnosis of BTCs is vital to improve patient prognosis.
The diagnosis of BTCs is based on a combination of imaging techniques, including ultrasound, CT, MRI, and tumor biopsy (5, 8).CT scanning is convenient and economical and is usually the first choice for diagnosing BTCs. MRI can provide high-resolution anatomical information about the biliary system, while MRCP is audio-visual (1). ERCP can directly display the biliary system and improve the accuracy of diagnosis. ERCP can also be used to confirm the diagnosis of cholangiocarcinoma by brushing cytology or biopsy (9). However, widely accepted biomarkers for diagnosing and dynamically monitoring the disease are still lacking. Currently, widely applied tumor markers, such as serum CA199 and CEA, have limited diagnostic value for BTCs (10). Tumor markers can be used for tumor screening, early detection, differential diagnosis and staging, prognosis judgment, efficacy monitoring, recurrence and metastasis monitoring, and guiding individualized treatment. The ideal tumor markers should have high sensitivity and specificity and reflect the tumor’s dynamic changes. The detection of tumor markers should be simple, fast, and accurate. Tumor markers have great application prospects in cancer. Thus, many researchers are working on searching for biomarkers used in the diagnosis, treatment response, and prognosis of BTCs (11–13). Tumor immunotherapy is a new and hot field. In addition, understanding the interaction between molecules and immune system is the premise of implementing immunotherapy.
Bile is produced by hepatocytes and secreted into the intestine through the biliary system. The compositions of bile are complex. Bile is composed mainly of bile acids, phospholipids, cholesterol, bilirubin, proteins, inorganic salts, etc. Proteins account for about 7% of the total bile compositions (14). Under the condition of disease, the ingredients of bile can be changed, especially in terms of BTCs, which is directly in touch with bile. Therefore, compared with blood or other body fluids, bile is an essential source for searching for tumor markers in the biliary system. Current advances in omics techniques have enabled researchers to discover new and valuable biomarkers in biological fluids (5). This review focuses on changes in bile composition and their interaction with the immune system. Changes in small molecules, proteins, ctDNA, miRNA, and extracellular vesicles have also been discussed.
When tumors of the biliary system occur, small molecules in the bile are altered. Existing studies have focused on differences in bile acid molecules and lipids in bile. The production of bile acids in the liver is the body’s primary way of cholesterol metabolism. The primary bile acids, such as cholic and chenodeoxycholic acid, are synthesized from cholesterol in the liver, then secreted into the intestine to produce secondary bile acids through the action of intestinal bacteria. Primary and secondary bile acids are unconjugated and can be combined with glycine or taurine to form conjugated bile acids (15). The intestine can reabsorb more than 95% of all bile acids; the rest is excreted with the stool (16).
Bile acids can be divided into hydrophobic and hydrophilic bile acids according to their affinity for water. Hydrophobic and hydrophilic bile acids play opposite roles in tumor carcinogenesis. Hydrophobic bile acids, such as deoxycholic acids (DCA) and lithocholic acid (LCA), can promote tumorigenesis, whereas hydrophilic bile acids, such as ursodeoxycholic acid (UDCA), can prevent tumorigenesis (17–19). However, the relationship between the composition of bile acid in bile and the malignant transformation of biliary tract tumors has not been fully elucidated. Compared with patients with biliary stones or controls (liver transplant donors without biliary disease), patients with biliary system tumors had lower concentrations of total bile acids and lower ratios of DCA and LCA. For patients with bilirubin ≤ 2.0mg/dL, biliary tract tumor patients also showed lower total bile acid concentration and a lower percentage of DCA and LCA compared with the control group, suggesting its value in the early diagnosis of biliary tract tumors (20). A later study further confirmed the reduction in total bile acid concentrations. They also found an elevated ratio of primary bile acids and conjugated bile acids in CCA patients compared with BBD or PC, which is consistent with previous results (21, 22), indicating that conjugated bile acids can promote the growth of bile tract cancers. They further identified GCA as a positive biomarker and TUCDA as a negative biomarker for cholangiocarcinoma (23). Researchers have also found that compared with benign stenosis, the conjugated bile acids(C)/unconjugated bile acids(U) ratio of malignant stenosis patients is relatively low. As the C/U ratio decreases, the diagnosis rate of inflammation and tumors increases (24). The synthesis of bile acids is dynamically regulated and bile acid concentration can be a real-time and reliable indicator of liver function (25).However, the regulatory mechanisms of bile acid synthesis is complex and have not yet been fully elucidated. The results observed in those studies may reflect a partial imbalance in the process of bile acid during tumorigenesis.
In addition to digestive function, bile acids also participate in the regulation of immune function. Primary and secondary bile acids can inhibit the expression of pro-inflammatory cytokines from monocytes, macrophages, dendritic cells, and Kupffer cells (26). Bile acid metabolites affect the host immune response by directly regulating the balance of TH17 and Tregs (27). One LCA derivative, 3-oxolCa, can inhibit TH17 cell differentiation. Another LCA derivative, IsoalloLCA, can enhance Treg differentiation. Biliary primary and secondary bile acids can regulate the expression of RORγ+ regulatory T cells via bile acid nuclear receptor (28). Primary bile acids increase the expression of CXCL6, thereby causing aggregation of hepatic CXCR6+ NKT cells and producing anti-tumor effects through IFN-γ, while secondary bile acids have opposite effects (29). SIRT5 is a metabolic regulator involved in the occurrence and development of a variety of tumors and plays context-specific roles. A recent study found that the decreased expression of SITR5 in hepatocellular carcinoma patients leads to increased bile acid synthesis. Bile acids act as signaling molecules to stimulate their nuclear receptors and promote the polarization of M2-type macrophages, thereby creating an immunosuppressive tumor microenvironment and promoting tumor growth (30). It is reasonable to infer that bile acids play a role in the occurrence and development of biliary tract tumors by interacting with the immune system. In conclusion, bile acids play a complex role in immune regulation, and understanding the role of bile acids in immunity can promote the implementation of immunotherapy.
Lipids include phospholipids, glycolipids, and cholesterol and their esters. Oxidized phospholipids(oxPLs) are essential in tumor cell apoptosis (31). Ten oxPLs were detected in bile from patients with diseases including CCA, PSC, PC, and BBD. Among them, ON-PC(1-palmitoyl-2(9-oxononanoyl)-sn-glycero-3-phosphatidylcholine) and S-PC(1-palmitoyl-2-succinoyl-sn-glycero-3-phosphatidylcholine) were significantly increased in CCA compared with other biliary stenosis patients. The sensitivity and specificity of ON-PC for distinguishing CCA from other biliary strictures were 85.7% and 80.3%, respectively. The sensitivity and specificity were increased to 100% and 83.3% by combining ON-PC and S-PC (32). The lipid peroxidation product 4- hydroxynonenal (4-HNE) was raised in gallbladder cancer patients compared with stones or controls. These findings indicate that lipids and their metabolites are also important bile markers for BTCs (33).
Metabolomics was applied to analyze low molecular metabolites in the body (10). The techniques used in metabolomics studies typically include Fourier transform infrared spectroscopy, gas chromatography/mass spectrometry(GC/MS), or lipid chromatography/mass spectrometry(LC/MS) (34). A new nuclear magnetic resonance spectra (NMR)-based metabolomics approach was also established to compare differences in the bile of patients with biliary tract cancer and benign biliary tract disease. A specific NMR spectroscopy can distinguish the two groups with 86% and 81% sensitivity and specificity, respectively (35). Lysophosphatidylcholine, phenylalanine, 2-octenoylcarnitine, and tryptophan levels in biliary tract cancer were decreased in patients of BTCs compared with benign diseases (36, 37).1H-MRS method was also applied to analyze the composition of bile. The levels of phosphatidylcholine and Taurine in bile were decreased in CCA patients compared to benign groups. The four regions in the final classifier (which represents phosphatidylcholine, bile acids, lipid, and cholesterol) can distinguish the two groups with a sensitivity and specificity of 88.9% and 87.1%, respectively (38). Another study examined volatile organic compounds in bile headspaces (gas above the sample) and found that several compounds (ethanol, acrylonitrile, acetonitrile, acetaldehyde, benzene, carbon disulfide, dimethyl sulfide, 2-propanolol) were decreased in patients with CCA compared with PSCs. A model was established based on the bile concentration of acrylonitrile, 3-methyl hexane, and benzene to distinguish patients with or without CCA. The sensitivity and specificity of the model are 90.5% and 72.7%, respectively (39).
Studies have focused on changes in other small molecules in the bile of patients with biliary tract cancer. The levels of glutathione (GSH), peroxide, ferrous iron (Fe2+), glutathione peroxidase (GPX), and farnesyl transferase/geranylgeranyltransferase type-1 subunit alpha (FNTA) in bile were investigated to illustrate the ferroptosis level of eCCA. The ability of these substances to distinguish between eCCA and common bile duct stones was further investigated. Compared with patients with common bile duct stones, the levels of these substances in bile are significantly reduced. Bile can be used as a biomarker source for diagnosing eCCA, especially for differentiating eCCA from benign bile duct stenosis (40). The lactate level in bile differs between the malignant disease group and non-malignant diseases or healthy people and may serve as a biomarker to distinguish benign people and malignancies (41). The content of ions and heavy metals in bile also changes in the diseased state. Compared with gallstones, patients with gallbladder cancer have decreased zinc levels and elevated copper and copper/zinc ratio (42). They tend to have higher concentrations of heavy metals such as chromium and cadmium (43).
Bile is believed to be the most relevant body fluid, which can closely indicate the pathological conditions of the biliary tract. Proteins are involved in tumor development (44–47). More and more data on protein biomarkers in bile is emerging. Serum CA199 and CEA are widely used markers for diagnosing biliary duct cancer, although the sensitivity and specificity are relatively low (48). Consequently, researchers have also studied the possibility of CA199 and CEA as bile biomarkers of CCA. The results indicate that bile CA199 and CEA are not valuable in distinguishing benign and malignant obstructive biliary tract disease (49). Thus, more potential bile markers with high sensitivity and specificity are urgently needed. Proteins in bile that may serve as tumor markers are summarized in Table 1.
The mucin family is a group of glycosylated macromolecules. Some mucins are abnormally expressed in cancer cells and are involved in tumorigenesis and progression (70). Several mucin family members also exist in the bile and can serve as bile markers. MUC2 and MUC16 were the first identified mucin family members which were differentially expressed in bile between CCA and controls using proteomics methods (71), although the sensitivity and specificity of bile MUC2 and MUC16 in diagnosing CCA still need further validation. Previous studies have shown that intratumoral MUC4 and MUC5AC are associated with the diagnosis and prognosis of biliary tract tumors (72, 73). Therefore, whether bile MUC4 and MUC5AC are indicators of biliary tract tumors has attracted attention. Bile MUC4 was identified as a particular marker of BTCs (74). However, whether bile MUC5AC expression could distinguish benign and malignant biliary tract diseases is still controversial. A study reported that bile MUC5AC couldn’t distinguish benign from malignant biliary tract diseases (74). However, another study showed that bile MUC5AC could distinguish benign and malignant patients with higher diagnostic accuracy than serum MUC5AC (AUC=0.85 in bile vs. AUC=0.82 in serum). Evaluating MUC5AC in bile and serum simultaneously and based on the serum-to-bile ratio can achieve better diagnostic performance (AUC=0.97) (50).
As glycosylated macromolecules, studies have also focused on the performance of glyco-alteration of glycoproteins as a diagnostic marker of CCA. In a study, researchers have established a new sandwich ELISA system to detect the glyco-alteration of MUC1. The results revealed that bile Wisteria floribunda Agglutinin (WFA)-Positive Mucin 1 is a promising CCA biomarker, which can effectively distinguish CCA from benign biliary tract diseases with sensitivity and specificity of 90.0% and 76.3%, respectively and the AUC was 0.86 (51).
Neutrophil gelatinase-associated lipocalin (NGAL), also called lipocalin-2 (LCN2), is a 25-kDa multifunctional protein released by activated neutrophils. NGAL plays a role in the onset and development of tumors (75). Zabron et al. compared the bile protein profiles of benign and malignant diseases. Biliary NGAL was the most valuable biomarker in distinguishing between benign and malignant groups. The area under the receiver-operating characteristic curve was 0.76 in differentiating malignant from benign pancreaticobiliary disease with sensitivity and specificity of 94% and 55%, respectively (52). Its diagnostic effect was validated in an independent cohort, and this capability is independent of CA199. The combination of bile NGAL and serum CA199 can improve the diagnostic accuracy for malignancy. Another study showed that bile NGAL levels could distinguish pancreaticobiliary cancer and benign biliary diseases with sensitivity and specificity of 73.3% and 72.2%, respectively. The area under the curve was 0.74 (53). In addition, Chiang et al. reported that biliary NGAL could differentiate CCA patients from gallstone patients with sensitivity and specificity of 87% and 75%, respectively. The AUC was 0.81. CCA patients with higher NGAL expression were also found to have poor overall survival (OS) of patients with CCA (54).
Bile samples from patients having biliary stenosis caused by CCA or other malignant tumors were analyzed by the proteomics method (76). A total of 127 differentially expressed proteins were identified. Several of them have been reported to be associated with pancreatic cancer. Immunoblotting assays were performed to validate these markers’ expression, and significant CEACAM6 elevation in CCA has been identified. A subsequent study explored the role of bile CEACAM6 in diagnosing CCA. Bile CEACAM6 could discriminate cholangiocarcinoma from benign disease (AUC=0.738) (55). CEACAM6 was further screened and validated as a biomarker to distinguish malignant and benign bile duct stenosis with sensitivity and specificity of 93% and 83%, respectively. The AUC was 0.92. The combination of bile CEACAM6 and serum CA199 can improve the diagnostic accuracy for malignancy (AUC 0.96) (56).
CCA cells can secrete several cytokines and promote tumor progression by paracrine. Some of the cytokines secreted by CCA can also enter bile and may serve as biomarkers. Among them, IGF-1 and VEGF were the most studied. IGF-1 is a bioactive protein polypeptide, and it has been previously reported that serum IGF-1 levels are associated with prostate, breast, pancreatic, lung, and colorectal cancer (77, 78). VEGF promotes tumor angiogenesis, and the relationship between serum VEGF levels and various tumors has also been reported (79, 80). Expression levels of IGF-1 and VEGF in the bile and serum of extrahepatic cholangiocarcinoma, pancreatic cancer, and benign biliary abnormalities were measured to evaluate their role as a tumor marker (57). Biliary IGF-1 perfectly differentiated extrahepatic cholangiocarcinoma from benign biliary abnormalities or pancreatic cancer. The AUC was 1. Bile VEGF levels have no differentiating effect on biliary obstruction. Meanwhile, another study shows the level of bile VEGF could distinguish pancreatic patients from other etiologies of biliary stricture (58). Biliary VEGF-1 may help rule out distal common bile duct cancer (81).
Some cytokines related to chronic inflammation, such as IL-6 and TNF-a, play a role in the development of tumors by interacting with the immune system. Chronic inflammation-driven cytokines released from cholangiocytes, fibroblasts, or immune cells can promote the development of CCA (82).
BTCs are always complicated with an anomalous arrangement of pancreaticobiliary ducts and affect bile digestive enzymes, especially for pCCA and dCCA. Abnormal digestive enzymes and their relative product expression in bile may serve as biomarkers. One study used the quantitative proteomics method to compare bile proteins in six CCA patients with three different gross-appearance tumor types to identify possible biomarkers. They selected α-1- antitrypsin (AAT) for further validation. Fecal AAT, which can indirectly reflect bile AAT, can differentiate CCA from controls with a sensitivity and specificity of 80% and 75%, respectively. The AUC was 0.83 (83). Elevated amylase activity in bile is also associated with pancreaticobiliary reflux, a significant risk factor for biliary system tumors (84, 85). Therefore, high biliary amylase activity is associated with BTCs tumorigenesis (86). However, pancreaticobiliary reflux is affected by the location and extent of biliary tract obstruction. The amylase level in bile is not specific for diagnosing biliary tract cancers (87, 88). Compared with patients with gallbladder stones, the pancreatic elastase (PE) level in CCA patients is elevated. Biliary PE can distinguish patients with CCA from that with gallstones. The combined measurement of PE and amylase and the PE to amylase ratio can improve the accuracy of CCA diagnosis (AUC=0.0877) (59).
Dhar et al. (89) discussed the role of pyruvate kinase M2(PKM2) in the development of cholangiocarcinoma. They proposed that PKM2 can be used as a diagnostic marker of BTCs and is associated with prognosis. A subsequent study (60) showed that biliary PKM2 had low sensitivity (52.9%), but the specificity was high (94.1%) for the diagnosis of malignant and benign bile duct stenosis. The AUC was 0.77.
In the first published bile proteomics, Mac-2-binding protein (Mac2BP) was identified as the potential biomarker for CCA. The value of Mac2BP as a diagnostic marker for cholangiocarcinoma was further validated in the following study (61). Bile Mac2BP can distinguish between malignant and benign biliary diseases with sensitivity and specificity of 69% and 67%, and the AUC was 0.70. The diagnostic effect is similar to biliary CA199 (AUC 0.69), and the combination of bile Mac2BP and CA19-9 can improve the accuracy of diagnosis (AUC 0.75).
Our group (62) applied ELISA to detect survivin and CA199 in bile. Biliary survivin could differentiate CCA and benign biliary obstruction with sensitivity and specificity of 67.27% and 80.85%, and the AUC was 0.78. Combining biliary survivin and CA199 could improve diagnostic accuracy (AUC=0.838).
Wang et al. (90) analyzed the proteins of gallbladder bile samples from gallbladder cancer, gallbladder adenoma, and chronic calculous cholecystitis patients using 2D LC-MS/MS. They proposed S100A8 as a potential biomarker for GBC and used immunohistochemical analysis to confirm it. Another study showed that S100P levels in bile were significantly elevated in patients with CCA, and bile S100P could distinguish between patients with CCA and choledocholithiasis with sensitivity and specificity of 92.9% and 70.0%, respectively (63).
Some other bile proteins including total fibronectin(tFN) and cellular fibronectin(cFN) (64), sB7-HB3 (65), sCD97 (66), LR11 (67), minichromosome maintenance protein 5(Mcm5) (68), HSP27, and HSP70 (69) was also reported to be bile biomarkers to distinguish benign and malignant biliary tract diseases with different sensitivity and specificity. However, these biomarkers still need further validation.
The term liquid biopsy was first used to describe how the same diagnostic information can be obtained from blood samples as tissue samples. In oncology, the term broadly refers to the sampling and analysis of various biological fluids (91). Analytes include cell-free DNA(cfDNA), cell-free RNA(cfRNA), circulating tumor cells (CTCs), and extracellular vesicles (EVs) (91). The interaction between liquid biopsy components and anti-cancer immunity is a new area of research (92). CfDNA refers to fragmented DNA found in non-cellular components of blood, which are usually double-stranded fragments of about 150-200 base pairs in length (93). In cancer patients, the cfDNA released from tumor cells is called circulating tumor DNA (ctDNA) and constitutes part of the total cfDNA (94, 95).
Assessing the ctDNA in bile is a promising liquid biopsy method, especially for patients with insufficient tumor tissue to biopsy. However, whether the mutation profiles detected by ctDNA reliably reproduce the mutation profiles obtained by tumor biopsy remains a question (96). Bile contact with tumor cells directly, Therefore, detecting genetic alterations in bile may be more valuable than in plasma for biliary tract cancers. Several studies (97, 98) have demonstrated the feasibility of detecting genetic mutations of ctDNA in tumors of the biliary system, with a high compatibility rate with tissue biopsies. A recent study shows genetic mutations of ctDNA in bile are similar to those in tissues and are more effective than in plasma (97). Similar conclusions were reached in another study (98). cfDNA in bile samples is mainly long DNA fragments, significantly different from plasma samples. Targeted deep sequencing can reliably detect mutant variants in bile cfDNA. Tumor stage and location affect the sensitivity of mutation detection in bile cfDNA samples. In patients with GBC, the positive rate of mutation detected in bile ctDNA was 58.3%, which is higher than cytology. The mutation compliance rate between tissue DNA and bile ctDNA in GBC patients was 85.7%. KRAS and TP53 are frequent mutation genes in tumors of the biliary system. Several previous studies have evaluated the diagnostic effects of KRAS and P53 mutations in bile on tumors of the biliary system (99–102). Overall, the frequency of KRAS and P53 mutations in bile is too low to rely on these molecular markers to establish a reliable diagnosis.
It was initially thought that higher cfDNA might indicate tumor growth. However, many other diseases contribute to a similar increase. Thus, recent research has focused on epigenetic features of cfDNA, such as methylation (103). One study showed that hypermethylation of UCHL1 and RUNX3 in pancreaticobiliary fluid might be useful for diagnosing biliary duct cancers (104). INK4a/ARF’s promoter methylation status in bile might be useful for diagnosing benign and malignant biliary tract diseases (105). Shen et al. (106) established and validated a methylation panel in the bile, which can improve the sensitivity of detecting BTCs.
ctDNA can be used to diagnose biliary duct cancers at an early stage. Recently, a prospective study using ddPCR to detect the methylation status of four genes (CDO1, CNRIP1, SEPT9, VIM) in bile showed that detecting DNA methylation markers in bile allowed for more accurate and early detection of CCA patients in PSCs (107). Another prospective study suggests that next-generation sequencing (NGS) mutational analysis of bile cell-free DNA (cfDNA) in the initial stages of biliary stenosis can significantly improve the detection of malignant tumors. In addition, it can also test for the presence of mutations suitable for targeted therapy (108).
miRNAs are small non-coding RNA molecules of 18-25 nucleotides in length, which can regulate gene expression by binding to corresponding mRNA sites (109). Extracellular miRNAs are stable and detectable in other body fluids (110). There are significant differences in miRNA concentration and composition in different diseases. Therefore, miRNA can be used as a biomarker to evaluate the body’s condition (111). Extracellular miRNAs are not homogenous groups and exist in various body fluids in different forms. Its origin and function are not fully understood. It is currently believed that some extracellular miRNAs are packaged in apoptotic bodies, shedding microvesicles, exosomes, or high-density lipoprotein (HDL) particles. Most extracellular miRNAs (90%-95%) bind to AGO proteins (110).Therefore, attention should be paid to the origin of the miRNAs when evaluating their roles as biomarkers.
Extracellular vesicles are all kinds of vesicles with membrane structures released by cells, and their contents include proteins, lipids, nucleic acids, and so on. According to the diameter and occurrence mode, it can be divided into exosomes, microvesicles, and apoptotic bodies (112, 113). Tumor-derived exosomes are likely to serve as potential cancer markers.
Shigehara et al. (114) compared the difference of miRNA in the whole bile between patients with malignant and benign biliary tract diseases for the first time and pointed out that miR-145 and miR-9 could be used as diagnostic markers of BTCs (Table 2), especially miR-9, which has higher diagnostic accuracy. A subsequent study showed that RNA from free-floating cells degrades rapidly. The bile processing process can produce unpredictable deviations. Therefore, they focused on extracellular vesicles in bile instead of the whole bile and established a miRNA panel based on extracellular vesicles in bile to diagnose CCA with a sensitivity and specificity of 67% and 96%, respectively (115). However, miRNA in extracellular vesicles only accounts for a small part of extracellular miRNA. Thus, one study suggested that extracellular vesicles were not a reliable miRNA source for CCA diagnosis. They recommend cell-free bile rather than whole bile or extracellular vesicles in bile to find potential miRNA markers (118). miRNA profiles in the bile of PSC, PSC with CCA, and CCA patients were different, among which miR-142, miR-640, miR-1537, and miR-3187 were significantly different in PSC and CCA patients (116) (Table 2). In another study, extracellular miR-30d-5p and miR-92a-3p were increased dramatically in the bile of CCA patients compared with benign biliary disease (BBD) patients (117) (Table 2). miR-30d-5p showed the best performance in distinguishing CCA and BBD patients. In addition, it has been demonstrated that high methylation of miR-1247, -200a, and -200b in bile may help determine benign and malignant biliary tract diseases (119).
The extracellular vesicles can also be used to distinguish between patients with benign and malignant diseases. As aforementioned, an established miRNA panel (Table 3) in the extracellular vesicles can be used to diagnose CCA (115). Ge X et al. (120)compared the expression of lncRNAs in bile exosomes from CCA patients and biliary obstruction patients. They identified that two lncRNAs were significantly elevated in CCA, and the combined use of two lncRNAs showed better diagnostic value (Table 3). Besides, both of them were associated with CCA prognosis. Bile exosome circCCAC1 can distinguish CCA from benign hepatobiliary diseases (AUC=0.857) while tissue circCCAC1 is associated with the prognosis of patients (122). In addition to non-coding RNA, proteins in extracellular vesicles can also be used as markers for patients with CCA. Ikeda, C et al. (121)used EDEG to separate human bile EVs and analyzed proteins in extracellular vesicles for the first time. They found that Claudin-3 in bile EVs can distinguish between CCA and patients with bile duct stones with a sensitivity and specificity of 87.5% and 87.5%, respectively (Table 3). The concentration of EVs in bile may be relevant to diagnosing malignant biliary tract disease. One study showed that the concentration of EVs in bile can distinguish malignant from non-malignant CBD stenosis with 100% accuracy (123).
In addition to being a marker of disease, the potential therapeutic role of EVs in disease is gradually being discovered (124). EVs can target receptor cells, increase drug concentration in target tissues, and reduce drug toxicity and side effects. The outer membrane of the vesicle increases drug stability and prevents drug degradation. The study of vesicle-related functions has become a research hotspot and is expected to play a role in the early diagnosis and treatment of many diseases.
BTCs are a group of highly heterogeneous diseases with abysmal prognosis. Finding a method for early diagnosis of BTCs in a non-invasive or minimally invasive way and evaluating the treatment effect to improve the prognosis of patients with BTCs is promising. The search for tumor markers is a feasible approach. Many previous studies have focused on finding a substance in the blood, evaluating its relationship with BTCs, and determining whether it can be used as a tumor marker. Blood samples are readily available, but blood often reflects the body’s overall conditions and cannot be accurately localized to an organ or tissue. The components in the blood may interfere with each other and mask the characteristic information of the disease, especially in the early stages (125). Therefore, many studies have focused on finding tumor markers in other body fluids. Bile is directly exposed to BTCs, and tumors will release certain substances into bile. Thus, bile may contain tumor-specific information, an essential source for searching for tumor markers. The acquisition of bile is invasive, and it is difficult to obtain information about a healthy person’s bile (14). While in the disease state, some treatments such as ERCP, PTCD, and surgery become essential sources of bile without adding additional trauma to patients. Therefore, bile biomarkers research is more valuable. This study focused on bile and summarized the research progress of the relationship between bile components and BTCs.
Metabolomics is used to analyze low molecular metabolites in bile to determine the pathophysiology of the human. Bile acids and lipids are two kinds of small molecules that are most concerned at present. However, the results of different studies are inconsistent or even opposite. Many factors are involved, including pretreatment before bile testing, tumor anatomical site and methods of bile collection, individual differences in disease severity, and various other factors affecting the results. For the early diagnosis of BTCs, more attention was paid to whether bile composition changes in the absence of biliary obstruction (20). Bile acids and lipids are involved in the occurrence and development of BTCs, and the mechanism needs to be further studied.
Proteomics studies the composition and variation of proteins in cells, tissues, or living organisms. Clinical detection of disease is mainly based on protein analysis. Bile protein analysis is challenging to carry out because of the interference of many other substances (14). Thus, pretreatment is required before proteomic analysis. The proteome is a fluctuating description of the host’s response to disease. Many minor but significant changes may occur in the early stage of the disease (126).Therefore, combining multiple markers is more effective than single markers (127). Protein changes arise during the malignant transformation of healthy cells (128–130). Researchers currently focus on finding overexpressed proteins that flow into body fluids due to disease as disease biomarkers (131–133). Since bile is directly in contact with BTCs, tumor markers of BTC are more likely to be identified in bile. The use of mass spectrometry to analyze proteomic patterns in tissues and body fluids, combined with bioinformatics tools to distinguish patterns in normal, benign, or malignant disease states, namely, proteomic pattern diagnosis (134). Thus, artificial intelligence (AI) is required for processing high-throughput omics data.
Liquid biopsy is a hot field now, which can be used to detect cfDNA, cfRNA, CTCs, EVs, etc. (91). Compared with the traditional methods, it can comprehensively reflect the overall state of the tumor in a minimally invasive and fast way, and it applies to a wide range of people. Liquid biopsies are good supplements for patients who have difficulty obtaining biopsy tissues or have insufficient tissues for genetic testing. Liquid biopsies can be used to diagnose and guide the treatment of patients (135). The low ctDNA content of the samples used for liquid biopsies, especially in the early stages, poses a great challenge to the detection of ctDNA (135). ctDNA is currently the most widely used in clinical practice. ctDNA can be used as a tumor marker of BTCs by detecting the related mutation status of ctDNA (125). ctDNA in bile provides a comprehensive view of the tumor genome, reflecting DNA released from multiple tumor regions. It is dynamic. Thus, ctDNA in bile can monitor treatment response, drug-resistant, tumor heterogeneity, and detect residual disease after surgery. In conclusion, detecting ctDNA in bile is an auspicious method.
Multiple miRNAs have been found in the blood as markers of BTCs. However, miRNAs are rarely reported in bile. Extracellular miRNAs are heterogeneous and may play an essential role in the occurrence and development of BTCs (110). Extracellular miRNAs may act as tumor markers for BTCs and are associated with prognosis. EVs are a recently emerging class of molecules that play an essential role in cell-to-cell communication, with exosomes being the most mature (113). There are also growing studies of EVs in bile. EVs contain various substances such as proteins, nucleic acids, etc. These substances within EVs or the concentration of EVs in bile may play an essential role in the occurrence and development of BTCs and may act as biomarkers for BTCs. EVs can also be used in the treatment of diseases. Bile-specific exosomes may lead to better therapeutic outcomes. Bile enters the intestine through the biliary tract and may be helpful in the treatment of intestinal diseases (124).
In addition to traditional chemotherapy and targeted therapy, tumor immunotherapy is a revolutionary breakthrough. Instead of acting on tumor cells, it activates the body’s immune system to produce an anti-tumor effect. Understanding the mechanism of interaction between molecules and the immune system is immunotherapy’s premise. Anti-tumor immunotherapy is promising theoretically and ideologically. However, it still seems a long way to go.
Bile biomarkers are promising and meaningful in diagnosis and prognosis judgement of CCA. However, bile collection is invasive, and it is difficult to obtain normal bile from a healthy donor (14). Setting the control group to patients with benign diseases may lead to unpredictable biases. Instead, finding a non-invasive way, such as blood, feces, etc., to get an accurate diagnosis is preferred. However, this requires building a relationship between bile and the clinically readily available samples such as blood, urine, stool, etc. Besides, single bile biomarker is hard to reach a high specificity and a high sensitivity simultaneously. An accurate BTCs diagnosis involves combining data from multiple omics, including genomics, epigenomics, transcriptomics, proteomics, and metabolomics. The high-throughput multi-dimensional information, combined with AI technology, makes it possible to diagnose BTCs more accurately and improve the judgment of patient prognosis.
Y-CL, K-SL, Z-LL, Y-CT, and X-QH collected the references. Y-CL and K-SL analyzed the references and wrote the paper. X-YL, A-DS, L-MZ, L-ZS, SL, Z-DY, S-HH, G-LS, YS, Y-JL, FH, and M-HZ read the paper and provided revising advice. Z-LZ contributed to study supervision and revised the manuscript. All authors contributed to the article and approved the submitted version.
This work was supported by the National Natural Science Foundation of China (Grant No. 81900728, 82072676,82172791,82203766), Shandong Province Natural Science Foundation (Grant No. ZR2019MH008, ZR2020MH238, ZR2021QH079), Shandong Province Key R&D Program(Major Scientific Innovation Projects,2021CXGC011105), Shandong Medical and Health Technology Development Project (Grant No. 2018WSB20002), Clinical Research Foundation of Shandong University (Grant No. 2020SDUCRCA018), Key Research and Development Program of Shandong Province (Grant No. 2019GSF108254). The funders had no role in study design, data collection, analysis, interpretation, and manuscript writing.
The authors declare that the research was conducted in the absence of any commercial or financial relationships that could be construed as a potential conflict of interest.
All claims expressed in this article are solely those of the authors and do not necessarily represent those of their affiliated organizations, or those of the publisher, the editors and the reviewers. Any product that may be evaluated in this article, or claim that may be made by its manufacturer, is not guaranteed or endorsed by the publisher.
1. Valle JW, Kelley RK, Nervi B, Oh DY, Zhu AX. Biliary tract cancer. Lancet. (2021) 397(10272):428–44. doi: 10.1016/S0140-6736(21)00153-7
2. Liu J, Ren G, Li K, Liu Z, Wang Y, Chen T, et al. The Smad4-MYO18A-PP1A complex regulates β-catenin phosphorylation and pemigatinib resistance by inhibiting PAK1 in cholangiocarcinoma. Cell Death Differ (2022) 29(4):818–31. doi: 10.1038/s41418-021-00897-7
3. Wang Y, Chen T, Li K, Mu W, Liu Z, Shi A, et al. Recent advances in the mechanism research and clinical treatment of anti-angiogenesis in biliary tract cancer. Front Oncol (2021) 11:777617. doi: 10.3389/fonc.2021.777617
4. Chen T, Li K, Liu Z, Liu J, Wang Y, Sun R, et al. WDR5 facilitates EMT and metastasis of CCA by increasing HIF-1α accumulation in myc-dependent and independent pathways. Mol Ther (2021) 29(6):2134–50. doi: 10.1016/j.ymthe.2021.02.017
5. Banales J, Marin J, Lamarca A, Rodrigues P, Khan S, Roberts L, et al. Cholangiocarcinoma 2020: The next horizon in mechanisms and management. Nat Rev Gastroenterol hepatol (2020) 17(9):557–88. doi: 10.1038/s41575-020-0310-z
6. Banales JM, Cardinale V, Carpino G, Marzioni M, Andersen JB, Invernizzi P, et al. Expert consensus document: Cholangiocarcinoma: Current knowledge and future perspectives consensus statement from the European network for the study of cholangiocarcinoma (ENS-CCA). Nat Rev Gastroenterol Hepatol (2016) 13(5):261–80. doi: 10.1038/nrgastro.2016.51
7. Cillo U, Fondevila C, Donadon M, Gringeri E, Mocchegiani F, Schlitt HJ, et al. Surgery for cholangiocarcinoma. Liver Int (2019) 39 Suppl 1(Suppl Suppl 1):143–55. doi: 10.1111/liv.14089
8. Liu Y, Sun J, Guo S, Liu Z, Zhu M, Zhang ZL. The distal classification and management of choledochal cyst in adults: Based on the relation between cyst and pancreatic duct. Med (Baltimore). (2017) 96(12):e6350. doi: 10.1097/MD.0000000000006350
9. Rösch T, Meining A, Frühmorgen S, Zillinger C, Schusdziarra V, Hellerhoff K, et al. A prospective comparison of the diagnostic accuracy of ERCP, MRCP, CT, and EUS in biliary strictures. Gastrointest Endosc. (2002) 55(7):870–6. doi: 10.1067/mge.2002.124206
10. Macias R, Banales J, Sangro B, Muntané J, Avila M, Lozano E, et al. The search for novel diagnostic and prognostic biomarkers in cholangiocarcinoma. Biochim Biophys Acta Mol basis disease. (2018) 1864:1468–77. doi: 10.1016/j.bbadis.2017.08.002
11. Nault JC, Villanueva A. Biomarkers for hepatobiliary cancers. Hepatology. (2021) 73 Suppl 1:115–27. doi: 10.1002/hep.31175
12. Sun R, Liu Z, Qiu B, Chen T, Li Z, Zhang X, et al. Annexin10 promotes extrahepatic cholangiocarcinoma metastasis by facilitating EMT via PLA2G4A/PGE2/STAT3 pathway. EBioMedicine. (2019) 47:142–55. doi: 10.1016/j.ebiom.2019.08.062
13. Liu Z, Sun R, Zhang X, Qiu B, Chen T, Li Z, et al. Transcription factor 7 promotes the progression of perihilar cholangiocarcinoma by inducing the transcription of c-myc and FOS-like antigen 1. EBioMedicine. (2019) 45:181–91. doi: 10.1016/j.ebiom.2019.06.023
14. Farina A, Dumonceau J, Lescuyer P. Proteomic analysis of human bile and potential applications for cancer diagnosis. Expert Rev proteomics. (2009) 6(3):285–301. doi: 10.1586/epr.09.12
15. Marion S, Desharnais L, Studer N, Dong Y, Notter MD, Poudel S, et al. Biogeography of microbial bile acid transformations along the murine gut. J Lipid Res (2020) 61(11):1450–63. doi: 10.1194/jlr.RA120001021
16. Chiang JY. Bile acid metabolism and signaling. Compr Physiol (2013) 3(3):1191–212. doi: 10.1002/cphy.c120023
17. Oyama K, Shiota G, Ito H, Murawaki Y, Kawasaki H. Reduction of hepatocarcinogenesis by ursodeoxycholic acid in rats. Carcinogenesis. (2002) 23(5):885–92. doi: 10.1093/carcin/23.5.885
18. Thompson M. Bile acids in the assessment of hepatocellular function. Toxicol pathol (1996) 24(1):62–71. doi: 10.1177/019262339602400109
19. Einarsson K. Effect of ursodeoxycholic acid on hepatic cholesterol metabolism. Scandinavian J Gastroenterol Supplement. (1994) 204:19–23. doi: 10.3109/00365529409103620
20. Park JY, Park BK, Ko JS, Bang S, Song SY, Chung JB. Bile acid analysis in biliary tract cancer. Yonsei Med J (2006) 47(6):817–25. doi: 10.3349/ymj.2006.47.6.817
21. Hashim Abdalla MS, Taylor-Robinson SD, Sharif AW, Williams HR, Crossey MM, Badra GA, et al. Differences in phosphatidylcholine and bile acids in bile from Egyptian and UK patients with and without cholangiocarcinoma. HPB (Oxford). (2011) 13(6):385–90. doi: 10.1111/j.1477-2574.2011.00296.x
22. Sharif AW, Williams HR, Lampejo T, Khan SA, Bansi DS, Westaby D, et al. Metabolic profiling of bile in cholangiocarcinoma using in vitro magnetic resonance spectroscopy. HPB (Oxford). (2010) 12(6):396–402. doi: 10.1111/j.1477-2574.2010.00185.x
23. Song WS, Park HM, Ha JM, Shin SG, Park HG, Kim J, et al. Discovery of glycocholic acid and taurochenodeoxycholic acid as phenotypic biomarkers in cholangiocarcinoma. Sci Rep (2018) 8(1):11088. doi: 10.1038/s41598-018-29445-z
24. Krupa Ł, Staroń R, Dulko D, Łozińska N, Mackie A, Rigby N, et al. Importance of bile composition for diagnosis of biliary obstructions. Molecules (Basel Switzerland) (2021) 26(23):7329. doi: 10.3390/molecules26237279
25. Kurumiya Y, Nagino M, Nozawa K, Kamiya J, Uesaka K, Sano T, et al. Biliary bile acid concentration is a simple and reliable indicator for liver function after hepatobiliary resection for biliary cancer. Surgery. (2003) 133(5):512–20. doi: 10.1067/msy.2003.142
26. Postler T, Ghosh S. Understanding the holobiont: How microbial metabolites affect human health and shape the immune system. Cell Metab (2017) 26(1):110–30. doi: 10.1016/j.cmet.2017.05.008
27. Hang S, Paik D, Yao L, Kim E, Trinath J, Lu J, et al. Bile acid metabolites control T(H)17 and t(reg) cell differentiation. Nature. (2019) 576(7785):143–8. doi: 10.1038/s41586-019-1785-z
28. Song X, Sun X, Oh SF, Wu M, Zhang Y, Zheng W, et al. Microbial bile acid metabolites modulate gut RORγ(+) regulatory T cell homeostasis. Nature. (2020) 577(7790):410–5. doi: 10.1038/s41586-019-1865-0
29. Ma C, Han M, Heinrich B, Fu Q, Zhang Q, Sandhu M, et al. Gut microbiome-mediated bile acid metabolism regulates liver cancer via NKT cells. Science (2018) 360(6391):eaan5931. doi: 10.1126/science.aan5931
30. Sun R, Zhang Z, Bao R, Guo X, Gu Y, Yang W, et al. Loss of SIRT5 promotes bile acid-induced immunosuppressive microenvironment and hepatocarcinogenesis. J hepatol (2022) 77(2):453–66. doi: 10.1016/j.jhep.2022.02.030
31. Volinsky R, Kinnunen P. Oxidized phosphatidylcholines in membrane-level cellular signaling: from biophysics to physiology and molecular pathology. FEBS J (2013) 280(12):2806–16. doi: 10.1002/hep.31175
32. Navaneethan U, Gutierrez N, Venkatesh P, Jegadeesan R, Zhang R, Jang S, et al. Lipidomic profiling of bile in distinguishing benign from malignant biliary strictures: a single-blinded pilot study. Am J gastroenterol (2014) 109(6):895–902. doi: 10.1038/ajg.2014.60
33. Pandey M, Shukla VK, Singh S, Roy SK, Rao BR. Biliary lipid peroxidation products in gallbladder cancer: increased peroxidation or biliary stasis? Eur J Cancer Prev (2000) 9(6):417–22. doi: 10.1097/00008469-200012000-00007
34. Jusakul A, Khuntikeo N, Haigh W, Kuver R, Ioannou G, Loilome W, et al. Identification of biliary bile acids in patients with benign biliary diseases, hepatocellular carcinoma and cholangiocarcinoma. Asian Pacific J Cancer prevention: APJCP (2012) 13 Suppl:77–82. doi: 10.7314/APJCP.2012.13.KKSuppl.77
35. Wen H, Yoo SS, Kang J, Kim HG, Park JS, Jeong S, et al. A new NMR-based metabolomics approach for the diagnosis of biliary tract cancer. J Hepatol (2010) 52(2):228–33. doi: 10.1016/j.jhep.2009.11.002
36. Xu X, Cheng S, Ding C, Lv Z, Chen D, Wu J, et al. Identification of bile biomarkers of biliary tract cancer through a liquid chromatography/mass spectrometry-based metabolomic method. Mol Med Rep (2015) 11(3):2191–8. doi: 10.3892/mmr.2014.2973
37. Khan SA, Cox IJ, Thillainayagam AV, Bansi DS, Thomas HC, Taylor-Robinson SD. Proton and phosphorus-31 nuclear magnetic resonance spectroscopy of human bile in hepatopancreaticobiliary cancer. Eur J Gastroenterol Hepatol (2005) 17(7):733–8. doi: 10.1097/00042737-200507000-00007
38. Albiin N, Smith IC, Arnelo U, Lindberg B, Bergquist A, Dolenko B, et al. Detection of cholangiocarcinoma with magnetic resonance spectroscopy of bile in patients with and without primary sclerosing cholangitis. Acta Radiol (2008) 49(8):855–62. doi: 10.1080/02841850802220092
39. Navaneethan U, Parsi MA, Lourdusamy V, Bhatt A, Gutierrez NG, Grove D, et al. Volatile organic compounds in bile for early diagnosis of cholangiocarcinoma in patients with primary sclerosing cholangitis: a pilot study. Gastrointest Endosc. (2015) 81(4):943–9.e1. doi: 10.1016/j.gie.2014.09.041
40. Han JY, Ahn KS, Baek WK, Suh SI, Kim YH, Kim TS, et al. Usefulness of bile as a biomarker via ferroptosis and cysteine prenylation in cholangiocarcinoma; role of diagnosis and differentiation from benign biliary disease. Surg Oncol (2020) 34:174–81. doi: 10.1016/j.suronc.2020.04.019
41. Nishijima T, Nishina M, Fujiwara K. Measurement of lactate levels in serum and bile using proton nuclear magnetic resonance in patients with hepatobiliary diseases: its utility in detection of malignancies. Japanese J Clin Oncol (1997) 27(1):13–7. doi: 10.1093/jjco/27.1.13
42. Gupta SK, Singh SP, Shukla VK. Copper, zinc, and Cu/Zn ratio in carcinoma of the gallbladder. J Surg Oncol (2005) 91(3):204–8. doi: 10.1002/jso.20306
43. Shukla V, Prakash A, Tripathi B, Reddy D, Singh S. Biliary heavy metal concentrations in carcinoma of the gall bladder: case-control study. BMJ (Clinical Res ed). (1998) 317(7168):1288–9. doi: 10.1136/bmj.317.7168.1288
44. Liu Z, Liu J, Chen T, Wang Y, Shi A, Li K, et al. Wnt-TCF7-SOX9 axis promotes cholangiocarcinoma proliferation and pemigatinib resistance in a FGF7-FGFR2 autocrine pathway. Oncogene. (2022) 41(20):2885–96. doi: 10.1038/s41388-022-02313-x
45. Wang Y, Li K, Zhao W, Liu Z, Liu J, Shi A, et al. Aldehyde dehydrogenase 3B2 promotes the proliferation and invasion of cholangiocarcinoma by increasing integrin beta 1 expression. Cell Death Dis (2021) 12(12):1158. doi: 10.1038/s41419-021-04451-8
46. Li Z, Liu J, Chen T, Sun R, Liu Z, Qiu B, et al. HMGA1-TRIP13 axis promotes stemness and epithelial mesenchymal transition of perihilar cholangiocarcinoma in a positive feedback loop dependent on c-myc. J Exp Clin Cancer Res (2021) 40(1):86. doi: 10.1186/s13046-021-01890-1
47. Chen T, Liu H, Liu Z, Li K, Qin R, Wang Y, et al. FGF19 and FGFR4 promotes the progression of gallbladder carcinoma in an autocrine pathway dependent on GPBAR1-cAMP-EGR1 axis. Oncogene. (2021) 40(30):4941–53. doi: 10.1038/s41388-021-01850-1
48. Björnsson E, Kilander A, Olsson R. CA 19-9 and CEA are unreliable markers for cholangiocarcinoma in patients with primary sclerosing cholangitis. Liver. (1999) 19(6):501–8. doi: 10.1111/j.1478-3231.1999.tb00083.x
49. Chen C, Shiesh S, Tsao H, Lin X. The assessment of biliary CA 125, CA 19-9 and CEA in diagnosing cholangiocarcinoma–the influence of sampling time and hepatolithiasis. Hepato-gastroenterology. (2002) 49(45):616–20.
50. Danese E, Ruzzenente O, Ruzzenente A, Iacono C, Bertuzzo F, Gelati M, et al. Assessment of bile and serum mucin5AC in cholangiocarcinoma: diagnostic performance and biologic significance. Surgery. (2014) 156(5):1218–24. doi: 10.1016/j.surg.2014.05.006
51. Matsuda A, Kuno A, Kawamoto T, Matsuzaki H, Irimura T, Ikehara Y, et al. Wisteria floribunda agglutinin-positive mucin 1 is a sensitive biliary marker for human cholangiocarcinoma. Hepatology. (2010) 52(1):174–82. doi: 10.1002/hep.23654
52. Zabron AA, Horneffer-van der Sluis VM, Wadsworth CA, Laird F, Gierula M, Thillainayagam AV, et al. Elevated levels of neutrophil gelatinase-associated lipocalin in bile from patients with malignant pancreatobiliary disease. Am J Gastroenterol (2011) 106(9):1711–7. doi: 10.1038/ajg.2011.187
53. Budzynska A, Nowakowska-Dulawa E, Marek T, Boldys H, Nowak A, Hartleb M. Differentiation of pancreatobiliary cancer from benign biliary strictures using neutrophil gelatinase-associated lipocalin. J Physiol Pharmacol (2013) 64(1):109–14.
54. Chiang KC, Yeh TS, Wu RC, Pang JS, Cheng CT, Wang SY, et al. Lipocalin 2 (LCN2) is a promising target for cholangiocarcinoma treatment and bile LCN2 level is a potential cholangiocarcinoma diagnostic marker. Sci Rep (2016) 6:36138. doi: 10.1038/srep36138
55. Rose JB, Correa-Gallego C, Li Y, Nelson J, Alseidi A, Helton WS, et al. The role of biliary carcinoembryonic antigen-related cellular adhesion molecule 6 (CEACAM6) as a biomarker in cholangiocarcinoma. PloS One (2016) 11(3):e0150195. doi: 10.1371/journal.pone.0150195
56. Farina A, Dumonceau J, Antinori P, Annessi-Ramseyer I, Frossard J, Hochstrasser D, et al. Bile carcinoembryonic cell adhesion molecule 6 (CEAM6) as a biomarker of malignant biliary stenoses. Biochim Biophys Acta (2014) 1844(5):1018–25. doi: 10.1016/j.bbapap.2013.06.010
57. Alvaro D, Macarri G, Mancino MG, Marzioni M, Bragazzi M, Onori P, et al. Serum and biliary insulin-like growth factor I and vascular endothelial growth factor in determining the cause of obstructive cholestasis. Ann Intern Med (2007) 147(7):451–9. doi: 10.7326/0003-4819-147-7-200710020-00003
58. Navaneethan U, Gutierrez NG, Jegadeesan R, Venkatesh PG, Poptic E, Liu X, et al. Vascular endothelial growth factor levels in bile distinguishes pancreatic cancer from other etiologies of biliary stricture: a pilot study. Dig Dis Sci (2013) 58(10):2986–92. doi: 10.1007/s10620-013-2764-0
59. Chen C, Tsai W, Wu H, Syu M, Wu C, Shiesh S. Diagnostic role of biliary pancreatic elastase for cholangiocarcinoma in patients with cholestasis. Clinica chimica acta; Int J Clin Chem (2008) 390:82–9. doi: 10.1016/j.cca.2008.01.011
60. Navaneethan U, Lourdusamy V, Poptic E, Hammel J, Sanaka M, Parsi M. Comparative effectiveness of pyruvate kinase M2 in bile, serum carbohydrate antigen 19-9, and biliary brushings in diagnosing malignant biliary strictures. Digestive Dis Sci (2015) 60(4):903–9. doi: 10.1007/s10620-014-3397-7
61. Koopmann J, Thuluvath PJ, Zahurak ML, Kristiansen TZ, Pandey A, Schulick R, et al. Mac-2-binding protein is a diagnostic marker for biliary tract carcinoma. Cancer. (2004) 101(7):1609–15. doi: 10.1002/cncr.20469
62. Liu Y, Sun J, Zhang Q, Jin B, Zhu M, Zhang Z. Identification of bile survivin and carbohydrate antigen 199 in distinguishing cholangiocarcinoma from benign obstructive jaundice. biomark Med (2017) 11(1):11–8. doi: 10.2217/bmm-2016-0178
63. Sato Y, Harada K, Sasaki M, Nakanuma Y. Clinicopathological significance of S100 protein expression in cholangiocarcinoma. J Gastroenterol Hepatol (2013) 28(8):1422–9. doi: 10.1111/jgh.12247
64. Körner T, Kropf J, Hackler R, Brenzel A, Gressner AM. Fibronectin in human bile fluid for diagnosis of malignant biliary diseases. Hepatology. (1996) 23(3):423–8. doi: 10.1002/hep.510230305
65. Liu Y, Cheng C, Bai L, Yao F, Shi S, Zhang Y. Value of bile soluble B7H3 for the diagnosis of malignant biliary strictures: Results of a retrospective study. Surg Oncol (2019) 28:195–200. doi: 10.1016/j.suronc.2019.01.010
66. Meng ZW, Liu MC, Hong HJ, Du Q, Chen YL. Expression and prognostic value of soluble CD97 and its ligand CD55 in intrahepatic cholangiocarcinoma. Tumour Biol (2017) 39(3):1010428317694319. doi: 10.1177/1010428317694319
67. Terai K, Jiang M, Tokuyama W, Murano T, Takada N, Fujimura K, et al. Levels of soluble LR11/SorLA are highly increased in the bile of patients with biliary tract and pancreatic cancers. Clin Chim Acta (2016) 457:130–6. doi: 10.1016/j.cca.2016.04.010
68. Ayaru L, Stoeber K, Webster GJ, Hatfield AR, Wollenschlaeger A, Okoturo O, et al. Diagnosis of pancreaticobiliary malignancy by detection of minichromosome maintenance protein 5 in bile aspirates. Br J Cancer. (2008) 98(9):1548–54. doi: 10.1038/sj.bjc.6604342
69. Sato Y, Harada K, Sasaki M, Yasaka T, Nakanuma Y. Heat shock proteins 27 and 70 are potential biliary markers for the detection of cholangiocarcinoma. Am J Pathol (2012) 180(1):123–30. doi: 10.1016/j.ajpath.2011.09.010
70. Hollingsworth MA, Swanson BJ. Mucins in cancer: protection and control of the cell surface. Nat Rev Cancer. (2004) 4(1):45–60. doi: 10.1038/nrc1251
71. Kristiansen T, Bunkenborg J, Gronborg M, Molina H, Thuluvath P, Argani P, et al. A proteomic analysis of human bile. Mol Cell proteomics: MCP. (2004) 3(7):715–28. doi: 10.1074/mcp.M400015-MCP200
72. Ruzzenente A, Iacono C, Conci S, Bertuzzo F, Salvagno G, Ruzzenente O, et al. A novel serum marker for biliary tract cancer: diagnostic and prognostic values of quantitative evaluation of serum mucin 5AC (MUC5AC). Surgery. (2014) 155(4):633–9. doi: 10.1016/j.surg.2013.12.003
73. Shibahara H, Tamada S, Higashi M, Goto M, Batra S, Hollingsworth M, et al. MUC4 is a novel prognostic factor of intrahepatic cholangiocarcinoma-mass forming type. Hepatol (Baltimore Md). (2004) 39(1):220–9. doi: 10.1002/hep.20031
74. Matull WR, Andreola F, Loh A, Adiguzel Z, Deheragoda M, Qureshi U, et al. MUC4 and MUC5AC are highly specific tumour-associated mucins in biliary tract cancer. Br J Cancer. (2008) 98(10):1675–81. doi: 10.1038/sj.bjc.6604364
75. Bolignano D, Donato V, Lacquaniti A, Fazio MR, Bono C, Coppolino G, et al. Neutrophil gelatinase-associated lipocalin (NGAL) in human neoplasias: a new protein enters the scene. Cancer Lett (2010) 288(1):10–6. doi: 10.1016/j.canlet.2009.05.027
76. Farina A, Dumonceau J, Frossard J, Hadengue A, Hochstrasser D, Lescuyer P. Proteomic analysis of human bile from malignant biliary stenosis induced by pancreatic cancer. J Proteome Res (2009) 8(1):159–69. doi: 10.1021/pr8004925
77. Renehan AG, Zwahlen M, Minder C, O’Dwyer ST, Shalet SM, Egger M. Insulin-like growth factor (IGF)-I, IGF binding protein-3, and cancer risk: systematic review and meta-regression analysis. Lancet. (2004) 363(9418):1346–53. doi: 10.1016/S0140-6736(04)16044-3
78. Lin Y, Tamakoshi A, Kikuchi S, Yagyu K, Obata Y, Ishibashi T, et al. Serum insulin-like growth factor-I, insulin-like growth factor binding protein-3, and the risk of pancreatic cancer death. Int J Cancer. (2004) 110(4):584–8. doi: 10.1002/ijc.20147
79. Park BK, Paik YH, Park JY, Park KH, Bang S, Park SW, et al. The clinicopathologic significance of the expression of vascular endothelial growth factor-c in intrahepatic cholangiocarcinoma. Am J Clin Oncol (2006) 29(2):138–42. doi: 10.1097/01.coc.0000204402.29830.08
80. Ruohola JK, Valve EM, Karkkainen MJ, Joukov V, Alitalo K, Härkönen PL. Vascular endothelial growth factors are differentially regulated by steroid hormones and antiestrogens in breast cancer cells. Mol Cell Endocrinol (1999) 149(1-2):29–40. doi: 10.1016/S0303-7207(99)00003-9
81. Patel T. Extracellular vesicle noncoding RNA: new players in the diagnosis and pathogenesis of cholangiocarcinoma. Hepatology. (2014) 60(3):782–4. doi: 10.1002/hep.27185
82. Landskron G, de la Fuente M, Thuwajit P, Thuwajit C, Hermoso MA. Chronic inflammation and cytokines in the tumor microenvironment. J Immunol Res (2014) 2014:149185. doi: 10.1155/2014/149185
83. Laohaviroj M, Potriquet J, Jia X, Suttiprapa S, Chamgramol Y, Pairojkul C, et al. A comparative proteomic analysis of bile for biomarkers of cholangiocarcinoma. Tumour Biol (2017) 39(6):1010428317705764. doi: 10.1177/1010428317705764
84. Noda Y, Fujita N, Kobayashi G, Ito K, Horaguchi J, Takasawa O, et al. Histological study of gallbladder and bile duct epithelia in patients with anomalous arrangement of the pancreaticobiliary ductal system: comparison between those with and without a dilated common bile duct. J gastroenterol (2007) 42(3):211–8. doi: 10.1007/s00535-006-1991-y
85. Tashiro S, Imaizumi T, Ohkawa H, Okada A, Katoh T, Kawaharada Y, et al. Pancreaticobiliary maljunction: retrospective and nationwide survey in Japan. J hepato-biliary-pancreatic surgery. (2003) 10(5):345–51. doi: 10.1007/s00534-002-0741-7
86. Horaguchi J, Fujita N, Noda Y, Kobayashi G, Ito K, Takasawa O, et al. Amylase levels in bile in patients with a morphologically normal pancreaticobiliary ductal arrangement. J Gastroenterol (2008) 43(4):305–11. doi: 10.1007/s00535-008-2158-9
87. Chen CY, Lin XZ, Wu HC, Shiesh SC. The value of biliary amylase and hepatocarcinoma-Intestine-Pancreas/Pancreatitis-associated (HIP/PAP-I) in diagnosing biliary malignancies. Clin Biochem (2005) 38(6):520–5. doi: 10.1016/j.clinbiochem.2005.01.012
88. Todani T, Watanabe Y, Fujii T, Uemura S. Anomalous arrangement of the pancreatobiliary ductal system in patients with a choledochal cyst. Am J Surg (1984) 147(5):672–6. doi: 10.1016/0002-9610(84)90139-9
89. Dhar D, Olde Damink S, Brindley J, Godfrey A, Chapman M, Sandanayake N, et al. Pyruvate kinase M2 is a novel diagnostic marker and predicts tumor progression in human biliary tract cancer. Cancer. (2013) 119(3):575–85. doi: 10.1002/cncr.27611
90. Wang W, Ai KX, Yuan Z, Huang XY, Zhang HZ. Different expression of S100A8 in malignant and benign gallbladder diseases. Dig Dis Sci (2013) 58(1):150–62. doi: 10.1007/s10620-012-2307-0
91. Heitzer E, Haque I, Roberts C, Speicher M. Current and future perspectives of liquid biopsies in genomics-driven oncology. Nat Rev Genet (2019) 20(2):71–88. doi: 10.1038/s41576-018-0071-5
92. Mohme M, Riethdorf S, Pantel K. Circulating and disseminated tumour cells - mechanisms of immune surveillance and escape. Nat Rev Clin Oncol (2017) 14(3):155–67. doi: 10.1038/nrclinonc.2016.144
93. Fan HC, Blumenfeld YJ, Chitkara U, Hudgins L, Quake SR. Analysis of the size distributions of fetal and maternal cell-free DNA by paired-end sequencing. Clin Chem (2010) 56(8):1279–86. doi: 10.1373/clinchem.2010.144188
94. Bettegowda C, Sausen M, Leary RJ, Kinde I, Wang Y, Agrawal N, et al. Detection of circulating tumor DNA in early- and late-stage human malignancies. Sci Transl Med (2014) 6(224):224ra24. doi: 10.1126/scitranslmed.3007094
95. Diehl F, Schmidt K, Choti MA, Romans K, Goodman S, Li M, et al. Circulating mutant DNA to assess tumor dynamics. Nat Med (2008) 14(9):985–90. doi: 10.1038/nm.1789
96. Corcoran R, Chabner B. Application of cell-free DNA analysis to cancer treatment. New Engl J Med (2018) 379(18):1754–65. doi: 10.1056/NEJMra1706174
97. Driescher C, Fuchs K, Haeberle L, Goering W, Frohn L, Opitz F, et al. Bile-based cell-free DNA analysis is a reliable diagnostic tool in pancreatobiliary cancer. Cancers (2020) 13(1):39 doi: 10.3390/cancers13010039
98. Kinugasa H, Nouso K, Ako S, Dohi C, Matsushita H, Matsumoto K, et al. Liquid biopsy of bile for the molecular diagnosis of gallbladder cancer. Cancer Biol Ther (2018) 19(10):934–8. doi: 10.1080/15384047.2018.1456604
99. Wang Y, Yamaguchi Y, Watanabe H, Ohtsubo K, Wakabayashi T, Sawabu N. Usefulness of p53 gene mutations in the supernatant of bile for diagnosis of biliary tract carcinoma: comparison with K- ras mutation. J gastroenterol (2002) 37(10):831–9. doi: 10.1007/s005350200137
100. Müller P, Ostwald C, Püschel K, Brinkmann B, Plath F, Kröger J, et al. Low frequency of p53 and ras mutations in bile of patients with hepato-biliary disease: a prospective study in more than 100 patients. Eur J Clin Invest (2001) 31(3):240–7. doi: 10.1046/j.1365-2362.2001.00800.x
101. Kubicka S, Kühnel F, Flemming P, Hain B, Kezmic N, Rudolph K, et al. K-Ras mutations in the bile of patients with primary sclerosing cholangitis. Gut. (2001) 48(3):403–8. doi: 10.1136/gut.48.3.403
102. Saurin J, Joly-Pharaboz M, Pernas P, Henry L, Ponchon T, Madjar J. Detection of ki-ras gene point mutations in bile specimens for the differential diagnosis of malignant and benign biliary strictures. Gut. (2000) 47(3):357–61. doi: 10.1136/gut.47.3.357
103. Mann J, Reeves H, Feldstein A. Liquid biopsy for liver diseases. Gut. (2018) 67(12):2204–12. doi: 10.1136/gutjnl-2017-315846
104. Kato N, Yamamoto H, Adachi Y, Ohashi H, Taniguchi H, Suzuki H, et al. Cancer detection by ubiquitin carboxyl-terminal esterase L1 methylation in pancreatobiliary fluids. World J Gastroenterol (2013) 19(11):1718–27. doi: 10.3748/wjg.v19.i11.1718
105. Klump B, Hsieh C, Dette S, Holzmann K, Kiebetalich R, Jung M, et al. Promoter methylation of INK4a/ARF as detected in bile-significance for the differential diagnosis in biliary disease. Clin Cancer research: an Off J Am Assoc Cancer Res (2003) 9(5):1773–8.
106. Shen N, Zhang D, Yin L, Qiu Y, Liu J, Yu W, et al. Bile cell−free DNA as a novel and powerful liquid biopsy for detecting somatic variants in biliary tract cancer. Oncol Rep (2019) 42(2):549–60. doi: 10.3892/or.2019.7177
107. Vedeld HM, Grimsrud MM, Andresen K, Pharo HD, von Seth E, Karlsen TH, et al. Early and accurate detection of cholangiocarcinoma in patients with primary sclerosing cholangitis by methylation markers in bile. Hepatology. (2022) 75(1):59–73. doi: 10.1002/hep.32125
108. Arechederra M, Rullán M, Amat I, Oyon D, Zabalza L, Elizalde M, et al. Next-generation sequencing of bile cell-free DNA for the early detection of patients with malignant biliary strictures. Gut. (2022) 71(6):1141–51. doi: 10.1136/gutjnl-2021-325178
109. Bartel DP. MicroRNAs: target recognition and regulatory functions. Cell. (2009) 136(2):215–33. doi: 10.1016/j.cell.2009.01.002
110. Turchinovich A, Weiz L, Burwinkel B. Extracellular miRNAs: the mystery of their origin and function. Trends Biochem Sci (2012) 37(11):460–5. doi: 10.1016/j.tibs.2012.08.003
111. Mayr C, Beyreis M, Wagner A, Pichler M, Neureiter D, Kiesslich T. Deregulated MicroRNAs in biliary tract cancer: Functional targets and potential biomarkers. BioMed Res Int (2016) 2016:4805270. doi: 10.1155/2016/4805270
112. Colombo M, Raposo G, Théry C. Biogenesis, secretion, and intercellular interactions of exosomes and other extracellular vesicles. Annu Rev Cell Dev Biol (2014) 30:255–89. doi: 10.1146/annurev-cellbio-101512-122326
113. Raposo G, Stoorvogel W. Extracellular vesicles: exosomes, microvesicles, and friends. J Cell Biol (2013) 200(4):373–83. doi: 10.1083/jcb.201211138
114. Shigehara K, Yokomuro S, Ishibashi O, Mizuguchi Y, Arima Y, Kawahigashi Y, et al. Real-time PCR-based analysis of the human bile microRNAome identifies miR-9 as a potential diagnostic biomarker for biliary tract cancer. PloS One (2011) 6(8):e23584. doi: 10.1371/journal.pone.0023584
115. Li L, Masica D, Ishida M, Tomuleasa C, Umegaki S, Kalloo A, et al. Human bile contains microRNA-laden extracellular vesicles that can be used for cholangiocarcinoma diagnosis. Hepatol (Baltimore Md). (2014) 60(3):896–907. doi: 10.1002/hep.27050
116. Voigtländer T, Gupta SK, Thum S, Fendrich J, Manns MP, Lankisch TO, et al. MicroRNAs in serum and bile of patients with primary sclerosing cholangitis and/or cholangiocarcinoma. PloS One (2015) 10(10):e0139305. doi: 10.1371/journal.pone.0139305
117. Han H, Kim M, Han J, Yun J, Kim H, Yang Y, et al. Bile-derived circulating extracellular miR-30d-5p and miR-92a-3p as potential biomarkers for cholangiocarcinoma. Hepatobiliary pancreatic Dis international: HBPD Int (2020) 19(1):41–50. doi: 10.1016/j.hbpd.2019.10.009
118. Roest H, Verhoeven C, van der Laan L. MicroRNAs in bile vesicles: finding a trade-off for biomarker discovery. Hepatol (Baltimore Md). (2015) 61(3):1094–5. doi: 10.1002/hep.27325
119. Ohtsubo K, Miyake K, Arai S, Fukuda K, Yanagimura N, Suzuki C, et al. Aberrant methylation of tumor suppressive miRNAs in bile from patients with pancreaticobiliary diseases. Anticancer Res (2019) 39(10):5449–59. doi: 10.21873/anticanres.13738
120. Ge X, Wang Y, Nie J, Li Q, Tang L, Deng X, et al. The diagnostic/prognostic potential and molecular functions of long non-coding RNAs in the exosomes derived from the bile of human cholangiocarcinoma. Oncotarget. (2017) 8(41):69995–70005. doi: 10.18632/oncotarget.19547
121. Ikeda C, Haga H, Makino N, Inuzuka T, Kurimoto A, Ueda T, et al. Utility of claudin-3 in extracellular vesicles from human bile as biomarkers of cholangiocarcinoma. Sci Rep (2021) 11(1):1195. doi: 10.1038/s41598-021-81023-y
122. Xu Y, Leng K, Yao Y, Kang P, Liao G, Han Y, et al. Cholangiocarcinoma-associated circular RNA 1, contributes to cholangiocarcinoma progression, induces angiogenesis, and disrupts vascular endothelial barriers. Hepatol (Baltimore Md). (2021) 73(4):1419–35. doi: 10.1002/hep.31493
123. Severino V, Dumonceau JM, Delhaye M, Moll S, Annessi-Ramseyer I, Robin X, et al. Extracellular vesicles in bile as markers of malignant biliary stenoses. Gastroenterology. (2017) 153(2):495–504.e8. doi: 10.1053/j.gastro.2017.04.043
124. Yao Y, Jiao D, Li Z, Zhou X, Li J, Liu Z, et al. Roles of bile-derived exosomes in hepatobiliary disease. BioMed Res Int (2021) 2021:8743409. doi: 10.1155/2021/8743409
125. Ignatiadis M, Sledge G, Jeffrey S. Liquid biopsy enters the clinic - implementation issues and future challenges. Nat Rev Clin Oncol (2021) 18(5):297–312. doi: 10.1038/s41571-020-00457-x
126. Liotta LA, Kohn EC. The microenvironment of the tumour-host interface. Nature. (2001) 411(6835):375–9. doi: 10.1038/35077241
127. Vlahou A, Schellhammer PF, Mendrinos S, Patel K, Kondylis FI, Gong L, et al. Development of a novel proteomic approach for the detection of transitional cell carcinoma of the bladder in urine. Am J Pathol (2001) 158(4):1491–502. doi: 10.1016/S0002-9440(10)64100-4
128. Zhao C, Wang B, Liu E, Zhang Z. Loss of PTEN expression is associated with PI3K pathway-dependent metabolic reprogramming in hepatocellular carcinoma. Cell Commun Signal (2020) 18(1):131. doi: 10.1186/s12964-020-00622-w
129. Sun R, Chen T, Li M, Liu Z, Qiu B, Li Z, et al. PTPN3 suppresses the proliferation and correlates with favorable prognosis of perihilar cholangiocarcinoma by inhibiting AKT phosphorylation. BioMed Pharmacother. (2020) 121:109583. doi: 10.1016/j.biopha.2019.109583
130. Xu YF, Liu HD, Liu ZL, Pan C, Yang XQ, Ning SL, et al. Sprouty2 suppresses progression and correlates to favourable prognosis of intrahepatic cholangiocarcinoma via antagonizing FGFR2 signalling. J Cell Mol Med (2018) 22(11):5596–606. doi: 10.1111/jcmm.13833
131. Zhang XM, Liu ZL, Qiu B, Xu YF, Pan C, Zhang ZL. Downregulation of EVI1 expression inhibits cell proliferation and induces apoptosis in hilar cholangiocarcinoma via the PTEN/AKT signalling pathway. J Cancer. (2020) 11(6):1412–23. doi: 10.7150/jca.31903
132. Xu YF, Liu ZL, Pan C, Yang XQ, Ning SL, Liu HD, et al. HMGB1 correlates with angiogenesis and poor prognosis of perihilar cholangiocarcinoma via elevating VEGFR2 of vessel endothelium. Oncogene. (2019) 38(6):868–80. doi: 10.1038/s41388-018-0485-8
133. Qiu B, Chen T, Sun R, Liu Z, Zhang X, Li Z, et al. Sprouty4 correlates with favorable prognosis in perihilar cholangiocarcinoma by blocking the FGFR-ERK signaling pathway and arresting the cell cycle. EBioMedicine. (2019) 50:166–77. doi: 10.1016/j.ebiom.2019.11.021
134. Wulfkuhle J, Liotta L, Petricoin E. Proteomic applications for the early detection of cancer. Nat Rev Cancer. (2003) 3(4):267–75. doi: 10.1038/nrc1043
Keywords: biliary tract cancers, bile components, biomarkers, immune effect, liquid biopsy
Citation: Li Y-c, Li K-s, Liu Z-l, Tang Y-c, Hu X-Q, Li X-y, Shi A-d, Zhao L-m, Shu L-Z, Lian S, Yan Z-d, Huang S-h, Sheng G-l, Song Y, Liu Y-j, Huan F, Zhang M-h and Zhang Z-l (2022) Research progress of bile biomarkers and their immunoregulatory role in biliary tract cancers. Front. Immunol. 13:1049812. doi: 10.3389/fimmu.2022.1049812
Received: 21 September 2022; Accepted: 12 October 2022;
Published: 28 October 2022.
Edited by:
Xuesong Gu, Beth Israel Deaconess Medical Center, Harvard Medical School, United StatesReviewed by:
Chuanzhao Zhang, Guangdong Provincial People’s Hospital, ChinaCopyright © 2022 Li, Li, Liu, Tang, Hu, Li, Shi, Zhao, Shu, Lian, Yan, Huang, Sheng, Song, Liu, Huan, Zhang and Zhang. This is an open-access article distributed under the terms of the Creative Commons Attribution License (CC BY). The use, distribution or reproduction in other forums is permitted, provided the original author(s) and the copyright owner(s) are credited and that the original publication in this journal is cited, in accordance with accepted academic practice. No use, distribution or reproduction is permitted which does not comply with these terms.
*Correspondence: Zongli Zhang, enpsenpsMTkwMEAxNjMuY29t
†These authors have contributed equally to this work
Disclaimer: All claims expressed in this article are solely those of the authors and do not necessarily represent those of their affiliated organizations, or those of the publisher, the editors and the reviewers. Any product that may be evaluated in this article or claim that may be made by its manufacturer is not guaranteed or endorsed by the publisher.
Research integrity at Frontiers
Learn more about the work of our research integrity team to safeguard the quality of each article we publish.