- 1Department of Immunology, Graduate School of Medicine and Faculty of Medicine, The University of Tokyo, Tokyo, Japan
- 2Department of Pharmacotherapeutics, Research Institute of Pharmaceutical Sciences and Faculty of Pharmacy, Musashino University, Tokyo, Japan
The γδT cells that produce IL-17 (γδT17 cells) play a key role in various pathophysiologic processes in host defense and homeostasis. The development of γδT cells in the thymus requires γδT cell receptor (γδTCR) signaling mediated by the spleen tyrosine kinase (Syk) family proteins, Syk and Zap70. Here, we show a critical role of Syk in the early phase of γδT cell development using mice deficient for Syk specifically in lymphoid lineage cells (Syk-conditional knockout (cKO) mice). The development of γδT cells in the Syk-cKO mice was arrested at the precursor stage where the expression of Rag genes and αβT-lineage-associated genes were retained, indicating that Syk is required for γδT-cell lineage commitment. Loss of Syk in γδT cells weakened TCR signal-induced phosphorylation of Erk and Akt, which is mandatory for the thymic development of γδT17 cells. Syk-cKO mice exhibited a loss of γδT17 cells in the thymus as well as throughout the body, and thereby are protected from γδT17-dependent psoriasis-like skin inflammation. Collectively, our results indicate that Syk is a key player in the lineage commitment of γδT cells and the priming of γδT17 cell differentiation.
Introduction
γδT cells exert multiple functions while acting as innate effector cells that produce cytokines and/or kill infected or malignant cells. Recently, accumulating evidence has suggested the versatile roles played by a subset of IL-17-producing γδT cells (γδT17 cells) in inflammatory disorders (1), tumor progression (2), tissue regeneration (3–5), and fibrosis (6) as well as affording protection from bacterial and fungal infection (7).
The development of both αβT and γδT cells occurs in the thymus. αβT lineage cells undergo a differentiation process through CD4− CD8− (double negative, DN), CD4+ CD8+ (double positive, DP), and CD4+ CD8- (CD4 single positive, CD4SP) or CD4- CD8+ (CD8SP) thymocytes, during which the T cell receptor (TCR) β and α chains are sequentially rearranged and immunocompetent yet self-tolerant αβTCRs are selected upon the interaction with self-peptide/major histocompatibility complex (8). In contrast, γδT lineage cells originate from DN thymocytes, in which the TCRγ and δ chains are simultaneously rearranged (9). Unlike the well-studied mechanisms of αβT cell development, the claim that the γδTCR-ligand interaction is required for γδT cell differentiation is still controversial. The effector function of γδT cells is reportedly determined in the thymus, where ligand-dependent strong or ligand-independent weak γδTCR signals lead to IFNγ-producing (γδT1) or IL-17-producing γδT (γδT17) cells, respectively (10–12). The effector function of γδT cells has a profound connection with TCR-Vγ repertoire. The γδT cells expressing Vγ4 or Vγ6 cells preferentially produce IL-17, while Vγ1, Vγ5, or Vγ7 γδT cells mainly produce IFNγ. It is also known that these distinct γδT cell subsets home to lymphoid and mucosal tissues depending on the TCR-Vγ chains they expressed (e.g., Vγ6 cells in the mucosal epithelia such as dermis and lung, Vγ7 cells in the intestine, Vγ5 cells in the epidermis, and Vγ1/Vγ4 cells in the lymphoid tissues).
Immune receptors including the TCR require the Spleen tyrosine kinase (Syk) family protein tyrosine kinases, Syk or Zap70, to activate intracellular signaling events (13). Zap70 is uniquely required for αβTCR signaling and αβT cell development (14). In contrast, Syk is involved in signal transduction in other receptor types such as the B cell receptor, Fc receptors, C-type lectin receptors, and erythropoietin receptors, and play critical roles in various physiological and pathological conditions (13, 15). In the early stage of αβT cell development, Zap70 and Syk play a redundant role in pre-TCR signaling (16, 17).
We previously have shown that Syk as well as Zap70 are activated upon γδTCR signaling and that mice lacking Syk but not Zap70 exhibit reduced γδTCR signaling in γδT cells (18). These results with genetically or pharmacologically manipulated T-progenitor cells demonstrated that Syk-mediated γδTCR signal activates both the Lat/MAPK and PI3K/Akt pathways so as to induce γδT17 cell differentiation. However, these findings were obtained using γδT cells from fetal or neonatal mouse thymus, since systemic Syk-deficient mice are perinatally lethal due to an abnormality in lymphatic vascular development (19, 20). Thus, the contribution of Syk to γδT cell development in the adult stage is not yet fully understood.
In this study, we generated lymphoid lineage-specific Syk-deficient mice (hereafter called Syk conditional knockout mice: Syk-cKO mice). The results clearly demonstrate that, in adult mice, Syk is required for the thymic development of γδT cells, but not αβT cells. A substantial fraction of thymic γδT cells in Syk-cKO mice are in an immature state since they express Rag recombinases as well as the pTα chain, indicating that Syk drives γδT-cell lineage commitment. Furthermore, Syk-cKO mice completely lacked the development of γδT17 cells in the thymus and were largely protected from imiquimod-induced psoriasis-like dermatitis. These results offer conclusive evidence that Syk-mediated TCR signaling is critical for both γδT-cell lineage commitment and γδT17 cell priming.
Materials and methods
Animals
C57BL/6N mice were purchased from SLC Japan (Shizuoka, Japan). Sykflox mice (21) were purchased from the Jackson laboratory (No. 017309). Cd127-Cre mice were described previously (22). The mice were maintained under specific pathogen-free conditions in our animal facility and euthanized by overdose of inhalational anesthetics. All animal experiments were performed with the approval of the Institutional Animal Care and Use Committee of the University of Tokyo, and conducted in accordance with institutional procedures.
Cell preparation
Thymocytes, splenocytes, and lymph node cells were prepared by homogenizing the organs. Lung and skin cells were prepared by digesting minced tissues with 0.2% collagenase D (Roche) and 0.01% DNase I (Roche) at 37°C for 30 minutes. The digested tissues were disrupted by using a syringe and 18-gauge needle. The enzymatic reaction was stopped by adding PBS containing 2 mM EDTA and 2% FCS. The homogenized or digested cells were passed through 100-μm nylon mesh to obtain single cell suspensions. To prepare IEL from the small intestine, gut fragments were cut open and incubated with PBS containing 30 mM EDTA at 4°C for 30 minutes. Then the incubated fragments were washed and shaken in cold PBS to collect the small intestine epithelial fraction. The lymphocyte fractions were isolated from the small intestine using a 40-80% Percoll gradient (GE Healthcare).
Flow cytometry analysis
FACSCantoII (BD Bioscience) was used for the flow cytometry analysis. Cells were treated with an Fc blocker (anti-CD16/32, clone 2.4G2, TONBO Bioscience) prior to cell surface staining. The cells were stained with the indicated antibodies at the final concentration of 1 to 2 μg/ml. To exclude dead cells, 7-aminoactionomycin D (7AAD) was added to the cell suspension at the final concentration of 0.3 μg/ml. For the intracellular staining, a Foxp3 Staining Buffer Set (eBioscience) was used according to their manufacturer’s protocol. The antibodies used for flow cytometry were as follows: CD4 (GK1.5), CD5 (53-7.3), CD8α (53-6.7), TCRβ (H57-597), CD3 (17A2), B220 (RA3-6B2), Ly6C (HK1.4), NK1.1(PK136), CD45RB (C363-16A), CD44 (IM7), CD25 (PC61), CD24 (30-F1), CD27 (LG.3A10), Gr-1 (RB6-8C5), TCRδ (GL3), TCR-Vγ1 (2.11), TCR-Vγ4 (UC3-10A6), TCR-Vγ5 (536), TCR-Vγ7 (GL1), IL-17A (TC11-18H10.1), IFN-γ (XMG1.2), phospho-ERK (p-ERK, 197G2) and phospho-AKT (p-Akt; D9E). The monoclonal antibody 17D1, specific for TCR-Vγ6/Vδ1 and TCR-Vγ5/Vδ1, was provided by Robert E. Tigelaar (Yale University, New Haven, Connecticut, USA) and used as described previously (23). Anti-Vγ7 monoclonal antibody (GL1) was provided by the late professor Leo Lefrançois (University of Connecticut Health Center, Farmington, Connecticut, USA) (24).
Cell stimulation
For the induction of the TCR signal, 1.0 ×107 thymocytes were resuspended in RPMI 1640 complete medium (25) that had been prewarmed at 37°C for 5 minutes, then an equal volume of prewarmed complete medium containing biotinylated anti-CD3ε antibody (60 μg/ml; 145-2C11; Biolegend) and streptavidin (21 μg/ml; SouthernBiotech) was added. At the indicated timepoint, 4% paraformaldehyde was added to stop the stimulation. For cytokine production, cells were incubated in RPMI 1640 complete medium in the presence of phorbol myristate acetate (PMA; 2.5 ng/ml), ionomycin (1 μg/ml) and brefeldin A (1 μg/ml) at 37°C for 4 hours.
Quantitative mRNA analysis
Total RNA was extracted from FACS-sorted cells using the RNeasy Micro Kit (QIAGEN) and reverse transcribed with Superscript III (Invitrogen, Thermo Fisher Scientific). Quantitative PCR was performed with SYBR Premix ExTaq (TaKaRa) and the StepOne Real-Time PCR System (Life Technologies, Thermo Fisher Scientific). The results were normalized to the Gapdh expression levels. The primer sequence used in this study is described in Supplementary Material.
Imiquimod-induced dermatitis
Daily application of 5 mg Beselna cream (5% IMQ; Mochida Pharmaceutical) or an equal amount of control vaseline cream (Wako) on mouse ears was performed to induce psoriasis-like dermatitis. Ear thickness was measured with a micrometer. On day 5, cervical lymph nodes were isolated and analyzed by flow cytometry.
Statistical analysis
All data are represented as the mean ± SEM. Differences with a P-value of <0.05 were considered significant. For statistical analysis, Prism 7 software (GraphPad Software Inc.) was used. Two-tailed unpaired t-tests were used for comparing two groups, and one-way ANOVA tests were used when comparing three groups or more.
Results
Impaired thymic γδT cell development in Syk-cKO mice
We crossed Sykflox mice with Cd127-Cre mice that express Cre recombinase under the control of the endogenous CD127 (IL-7R) promoter to specifically delete the Syk gene in lymphoid cells (21, 22). The generated Cd127-Cre Sykflox/flox (Syk-cKO) mice are assumed to lack the expression of Syk in both αβT cells and γδT cells as well as other lymphoid lineage cells, including B cells, natural killer (NK) cells, and innate lymphoid cells.
At the age of 4 to 5 weeks, Syk-cKO mice had a normal number of thymocytes without affecting the frequency of DN, DP, CD4SP or CD8SP cells (Figures 1A–C). In the spleen and lung, the number of B cells was markedly reduced in Syk-KO mice, while the number the NK cells was not significantly altered (Figure S1A in Supplementary Material). Consistent with previous findings that Syk controls pre-TCR signaling (17), Syk-cKO mice exhibited increases in the frequency of DN3 cells and decreases in the frequency of DN4 cells, resulting in a partial reduction of the DN4/DN3 ratio (Figure 1D and Figure S2). Both the frequency and number of DN1 (CD25– CD44+) cells were significantly reduced in Syk-cKO mice. This can be explained by the impaired development of B cells in Syk-cKO mice, as the CD25– CD44+ cell population contains many thymic B cells (26, 27). The number of DP, CD4SP, and CD8SP cells was comparable between the Syk-cKO mice and control mice, indicating that Syk-deficiency has no apparent effect on αβT cell development.
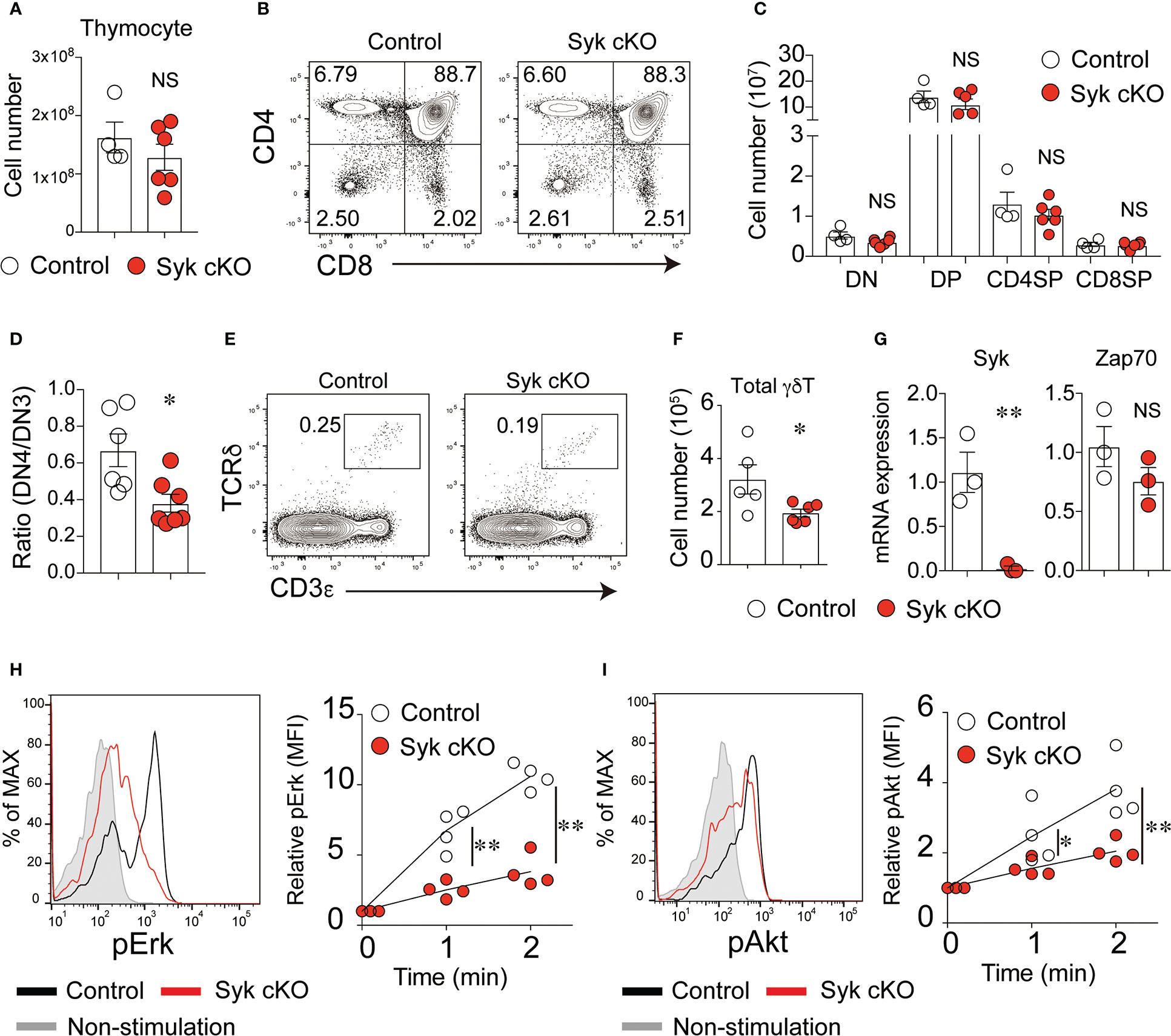
Figure 1 Syk is required for the development of γδT cells but not of αβT cells. (A) The number of thymocytes from 5-week-old control mice (Cd127-cre Syk flox/wt) or Syk-cKO mice (Cd127-cre Syk flox/flox). Each circle indicates an individual mouse. (B) Flow cytometric profiles for CD4 and CD8α expression in the total thymocytes from the indicated mice. (C) The graph indicates the number of DN, DP, CD4SP and CD8SP cells. (D) The ratio of CD25lo CD44lo DN (DN4) to CD25hi CD44lo DN (DN3) cells is shown. (E) Flow cytometric profiles for CD3ε and TCRδ expression in the total thymocytes from the indicated mice. (F) The number of thymic γδT cells is shown. (G) mRNA expression of Syk and Zap70 in sorted thymic γδT cells from control and Syk-cKO mice. Gene expression was normalized to Gapdh mRNA. (H, I) The phosphorylation of Erk (H) and Akt (I) induced by anti-CD3ε stimulation in thymic γδT cell. Representative flow cytometric profiles of the phospho-Erk (pErk) and phospho-Akt (pAkt) levels after 2-minutes of stimulation are shown. Graphs indicate the changes in the mean fluorescence intensity (MFI) of pErk and pAkt in the stimulated cells relative to non-stimulated cells. All data represent the mean ± SEM of at least two independent experiments. *P < 0.05 and **P < 0.01, by unpaired t-test. NS, not significant.
In contrast, the number of CD3+ TCRδ+ γδT cells was significantly reduced in the thymus of Syk-cKO mice (Figures 1E, F). The cell surface expression of TCRδ and CD3ε in Syk-deficient γδT cells was lower than that in control cells (Figure S3). In the thymic γδT cells isolated from Syk-cKO mice, the expression of functional Syk mRNA was totally absent, whereas the expression of Zap70 was comparable to that in control mice (Figure 1G). We measured the phosphorylation of Erk and Akt proteins in γδT cells upon anti-CD3ε stimulation to examine the effect of Syk deficiency on γδTCR signaling. Despite a normal expression of Zap70, TCR signal-induced phosphorylation of Erk and Akt was significantly diminished in Syk-cKO γδT cells (Figures 1H, I). These results indicate that Syk is required for optimum γδT cell development and γδTCR signaling.
Failure of γδ-selection in Syk-cKO mice
We hypothesized that the dampened γδTCR signaling in Syk-cKO mice influences the differentiation processes of γδT cells. To evaluate the effect of Syk deficiency on the thymic γδT cell differentiation, we examined cell surface expression of CD5 and CD24; the former is a widely used indicator of TCR signaling (28), whereas the latter is expressed by immature T cells (29). CD5lo CD24hi γδT cells in control mice were only 2.9 ± 0.74% of total γδT cells, whereas this population was drastically increased to 38.5 ± 0.9% in Syk-cKO mice (Figures 2A, B). The frequency of CD5hi CD24hi γδT cells was 76.7 ± 3.8% and 42.0 ± 1.0% of the total γδT cells in the control and Syk-cKO mice, respectively. The frequency of CD5hi CD24lo γδT cells was not significantly different between the control and Syk-cKO mice.
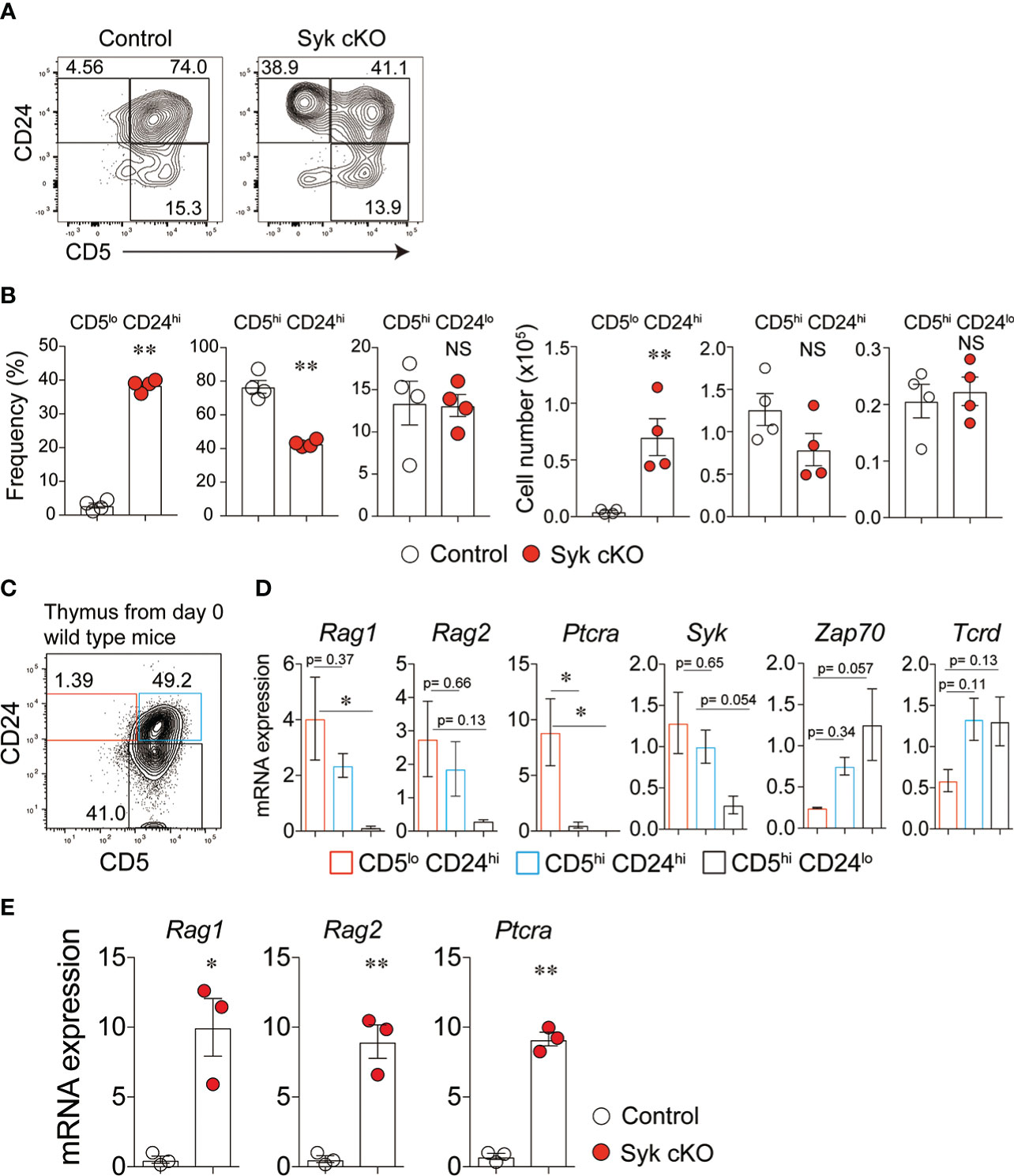
Figure 2 Impaired γδ-selection in Syk-cKO mice. (A, B) Flow cytometric profiles of CD5 and CD24 expression in total γδT cells from thymuses of control or Syk-cKO mice (A) and the number and frequency of the indicated γδT cell subset are shown (B). (C) Flow cytometric profiles of CD5 and CD24 expression in thymic γδT cells from 0-day-old wild-type mice. (D) mRNA expression of the Rag1, Rag2, Ptcra (pTα), Syk, Zap70 and Tcrd in γδT cell subsets shown in (C). Gene expression was normalized to Gapdh, and those in total thymic γδT cells were arbitrarily set to 1. (E) mRNA expression of Rag1, Rag2 and Ptcra in thymic γδT cells from the indicated mice (n = 3). Gene expression was normalized to Gapdh. All data represent the mean ± SEM. In (A) and (B), data are obtained from two independent experiments. *P < 0.05 and **P < 0.01, by 1-way ANOVA (D) or unpaired t-test (B, E). NS, not significant
To characterize the γδT cell subpopulations defined by CD24 and CD5 expression, we examined the gene expression profiles in γδT cell populations isolated from neonatal wild-type mouse thymus (Figure 2C). CD5lo CD24hi γδT cells exhibited high-level expression of recombination activating gene 1 (Rag1), Rag2 and Ptcra (pTα) (Figure 2D), indicating that CD5lo CD24hi γδT cells retain the potential to rearrange TCR genes and to differentiate into the αβT-cell lineage. The expression of these genes was down-regulated in CD5hi CD24hi and CD5hi CD24lo γδT cells. Thus, the CD5lo CD24hi cells represent γδT precursors that complete γδTCR rearrangement yet are uncommitted to the γδT cell lineage. Indeed, γδT cells isolated from Syk-cKO mice displayed a markedly higher expression of Rag1, Rag2 and Ptcra compared with those from control mice (Figure 2E). In general, the expression of Syk and Zap70 is inversely regulated during the development of thymic T cells: Syk is highly expressed until DN3 thymocyte stages and downregulated after β-selection, while Zap70 expression is induced after β-selection and maintained thereafter (9). Our results showed that the expression of Syk is highest in CD5lo CD24hi γδT cells but gradually decreased in CD5hi CD24hi and CD5hi CD24lo γδT cells (Figure 2D). On the other hand, the expressional pattern of Zap70 was opposite to that of Syk. These results indicate that Syk is required for the earliest step in γδT cell differentiation (previously referred to as “γδ-selection”) the step at which γδT precursors are committed towards the γδT cell lineage at the expense of a more limited αβT cell lineage potential (9).
Syk is essential for the development of γδT17 cells
We next examined the effector function of γδT cells in Syk-cKO mice. The mRNA expression of Rorc and Sox13, essential transcriptional factors for the γδT17 cell differentiation program, was significantly reduced in Syk-deficient γδT cells, whereas Egr3 and Tbx21, both of which promote thymic γδT1 cell differentiation, were normally expressed (Figure 3A) (30). To investigate the functional difference of Syk-deficient thymic γδT cells, we analyzed cell surface expression of CD44 and CD45RB that segregates mature γδT cells into γδT17 cells and γδT1 cells (10). The frequency of CD45RBlo CD44hi CD24lo γδT cells that correspond to γδT17 cells was 34.3 ± 3.6% and 5.2 ± 0.6% in control and Syk cKO mice, respectively (Figure 3B). In contrast, the frequency of CD45RBlo CD44hi CD24lo cells (mainly γδT1 cells) was statistically unchanged (Figure 3B). Consistent with this, production of IL-17 upon PMA/ionomycin stimulation was barely detected in thymic γδT cells from Syk-cKO mice (Figure 3C). These mice also exhibited a complete loss of γδT17 cells in the spleen and lungs, indicating a pivotal role for Syk in the development of γδT 17 cells. In contrast, the number of γδT1 cells was normal in the thymus and lungs of Syk-cKO mice, although it was partially (not significantly) reduced in the spleen (Figure 3C). Given that the effector function of γδT cells is correlated with TCR-Vγ chains (31), we examined the TCR-Vγ repertoire in various tissues. The number of Vγ4+ and Vγ6+ γδT cells (mostly γδT17 cells) was substantially reduced in the thymus, spleen, lung, small intestine and skin of Syk-cKO mice, while the number of Vγ1+, Vγ5+ and Vγ7+ γδT cells (mostly γδT1 cells) was undiminished with the exception of splenic Vγ1+ γδT cells (Figure 3D and Figure S4). Therefore, these results indicate that Syk is specifically required for the development of γδT17 cells but is largely dispensable for other subsets of γδT cells, including γδT1.
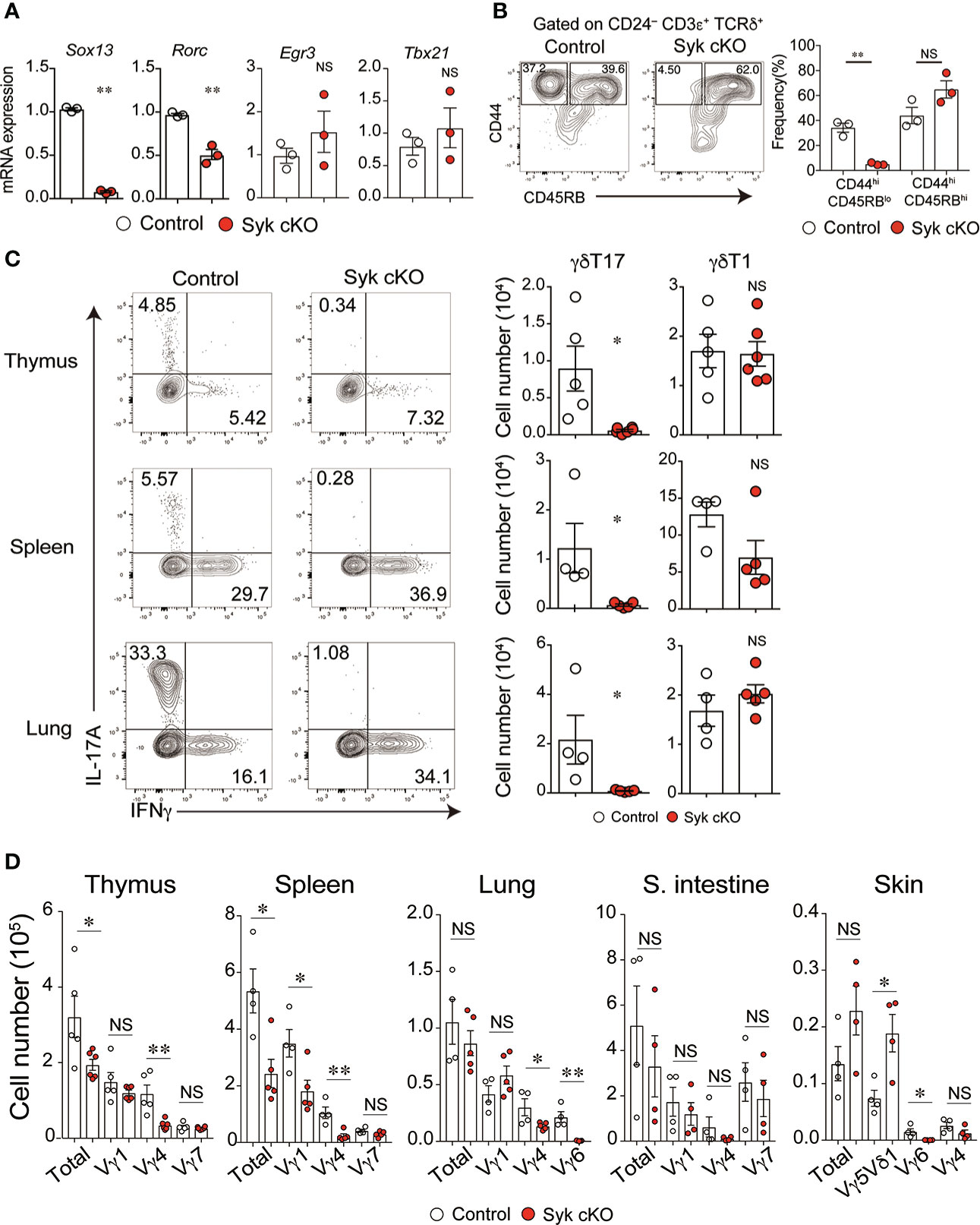
Figure 3 The impaired development of γδT17 cells but not γδT1 cells in Syk-cKO mice. (A) mRNA expression of Sox13, Rorc, Egr3 and Tbx21 in thymic γδT cells from control (Cd127-cre Syk flox/wt, n = 3) or Syk-cKO mice (Cd127-cre Syk flox/flox, n = 3). Gene expression was normalized to Gapdh. (B) Flow cytometric profiles of CD44 and CD45RB expression in CD24lo γδT cells from thymuses of control or Syk-cKO mice. The graph indicates the frequency of the indicated γδT cell subset. (C) Intracellular staining for IL-17A and IFNγ production in thymic, splenic and lung γδT cells from the indicated mice (n = 5 to 6). Cell staining was performed after a stimulation with PMA and ionomycin. The number of γδT17 and γδT1 cells is shown. (D) Graphs show the number of Vγ1+, Vγ4+, Vγ5Vδ1+, Vγ6+ and Vγ7+ γδT cells as well as total γδT cells in the indicated tissues (n = 4 to 6). All data represent the mean ± SEM. In (B–D), three independent experiments were performed. *P < 0.05 and **P < 0.01, by unpaired t-test. NS, not significant
Amelioration of skin inflammation in Syk-cKO mice
The accumulated evidence demonstrates that γδT17 cells play a central role in the pathogenesis of psoriatic dermatitis (1). We examined the significance of the role of Syk in the inflammatory condition by use of an imiquimod (IMQ)-induced psoriasis model. IMQ-treated control mice exhibited an increase in ear skin thickness, along with a robust expansion of cervical lymph node (LN) cells, including γδT cells (Figures 4A–C). However, Syk-cKO mice treated with IMQ exhibited a significant reduction in skin inflammation and an unaltered number of cervical LN γδT cells (Figures 4A–C). Furthermore, the IMQ-induced expansion of γδT17 cells in the cervical LN was not observed in Syk-cKO mice, while the γδT1 cell as well as αβT cell number was unchanged between the control and Syk-cKO mice (Figures 4D, E and Figure S5). The IL-17 produced in the skin promotes the recruitment of neutrophils to draining LN and dermis (32, 33). At the steady state, there are no obvious changes in the number of Gr-1hi Ly6Cint cells that contain neutrophils (34) in the spleen and lung from Syk-cKO mice (Figure S1B). However, the number of Gr-1+ Ly6Cint cells in cervical LN was significantly elevated in the IMQ-treated control mice, but not in Syk-cKO mice (Figure 4E). Consistent with this, the increase of Vγ4+ and Vγ6+ γδT cells upon IMQ treatment was also abrogated in Syk-cKO mice (Figure 4F). In addition to this, the proportion of CD27lo γδT cells characteristics of γδT17 cells (35) was significantly reduced in Syk-cKO mice (Figure 4G). These results indicate the requirement of Syk for the γδT17-mediated inflammatory responses.
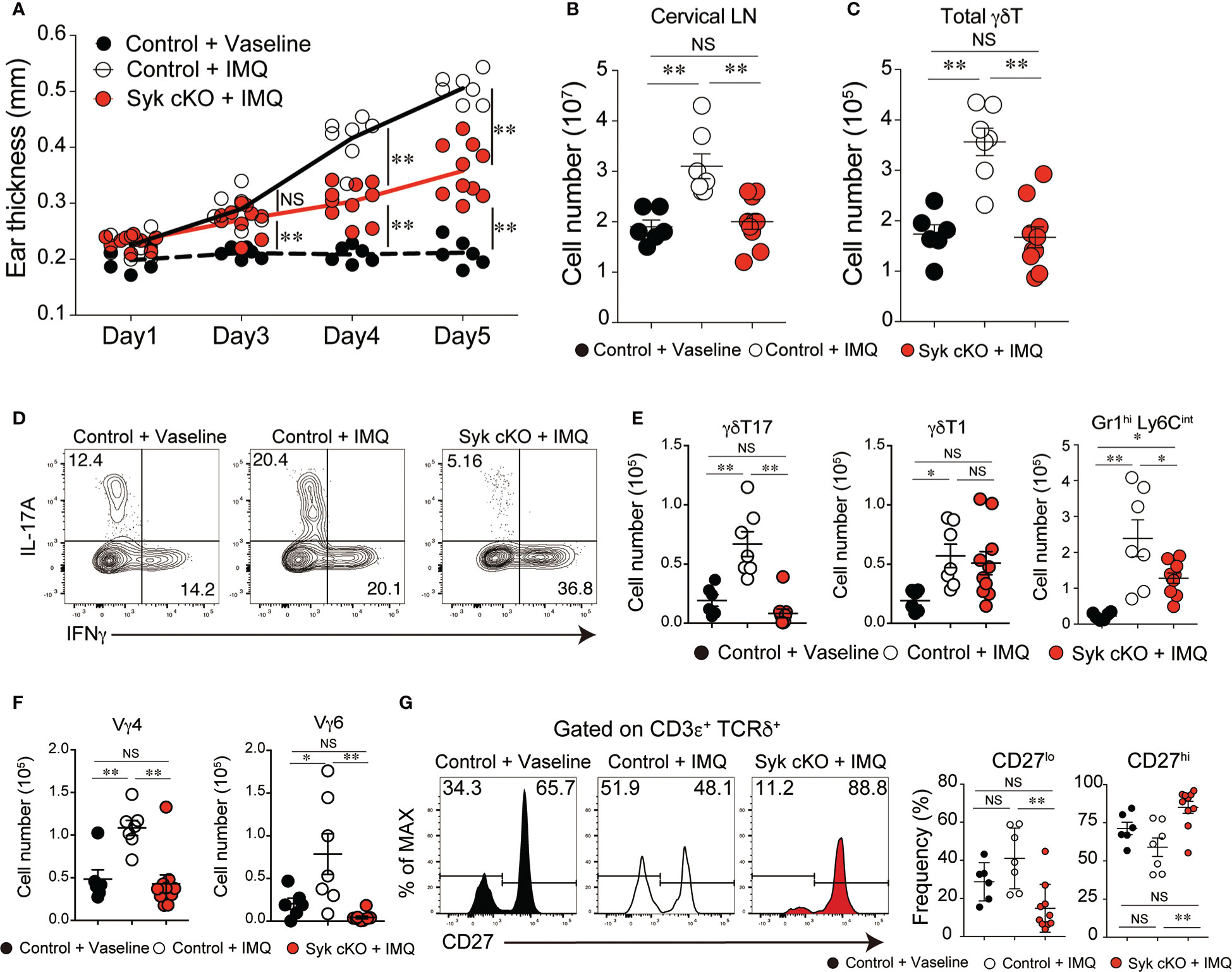
Figure 4 Amelioration of imiquimod (IMQ)-inducible dermatitis in Syk-cKO mice. Control (Cd127-cre Syk flox/wt) and Syk-cKO (Cd127-cre Syk flox/flox) mice were treated daily for 5 days with IMQ or Vaseline applied onto the ear. On day 5, the cervical lymph node (cLN) was isolated and analyzed. (A–C) The kinetics of ear swelling (A), the number of total cLN cells (B) and the number of γδT cells in the cLN (C) are shown. (D) Intracellular staining of IL-17A and IFNγ for γδ T cells in the cLN from mice shown in (A–C). (E) The number of γδT17, γδ T1 and Gr1+ CD11b+ cells in the cLN. (F) The number of Vγ4+ and Vγ6+ γδ T cells in the cLN. (G) Flow cytometric profiles of CD27 expression in total γδT cells from indicated mice. Graphs show the frequency of CD27lo or CD27hi γδT cells. Data were obtained from 6 to 10 mice in each group from two independent experiments. All data represent the mean ± SEM. *P < 0.05 and **P < 0.01, by 1-way ANOVA. NS, not significant
Discussion
In this study, we generated lymphoid lineage-specific Syk-deficient mice to study the role of Syk in the thymic development of γδT cells and γδTCR signaling. A large proportion of the γδT cells in the Syk-cKO mice was found to be in the early differentiation stage prior to γδT-lineage commitment, as indicated by the substantial expression of Rag genes and pTα. Syk-cKO mice also displayed a complete loss of γδT17 cell subsets and an altered γδTCR repertoire. These alternations in Syk-cKO mice resulted in a significant amelioration of skin inflammation. These results indicate that the Syk-mediated γδTCR signaling drives both γδT cell commitment and the γδT17 differentiation program.
Previous studies on the function of Syk in T cell development encountered difficulties because systemic Syk-deficient mice are perinatally lethal (19, 20) and T cell development requires in vivo thymus activity (8). We have previously investigated γδT cell development in fetal and neonatal Syk-deficient mice, but the requirement of Syk for γδT cell development in the adult stage was inadequately addressed (18, 36). Some pioneering studies using embryonic chimera mice or hematopoietic stem cell transplantation into immunodeficient mice demonstrated the role of Syk in epidermal and intestinal γδT cells (37, 38). However, these chimeric mouse models might not fully recapitulate the physiological conditions required for proper γδT cell development, as later studies suggested that certain γδT cell subsets require certain developmental stage-specific interplay between the progenitors and the thymic microenvironment for their full functional maturation (31, 39). The present results using Syk-cKO mice clearly demonstrate that Syk plays a pivotal role in γδT-cell lineage commitment as well as γδT17 cell priming. In the Syk-cKO mice, Vγ5Vδ1+ and Vγ7+ γδT cells normally populate in the skin and small intestine, respectively, indicating that Syk is dispensable for the development of Vγ5Vδ1+ and Vγ7+ γδT cells, most likely due to the compensatory function of Zap70 (16, 17, 40). This is consistent with previous results using fetal thymocytes from systemic Syk-deficient mice (18). Thus, the results presented here offer a conclusive answer to the long-standing question on the physiological role of Syk in γδT cell development.
It has been previously reported that γδTCR as well as preTCR induces downstream signaling in a ligand-independent manner (9, 41, 42). In Syk-cKO mice, the development of a large proportion of γδT cells were arrested at the stage of CD5lo CD24hi γδ precursors that still retain αβT-lineage potential. Our present findings, along with previous results, account for the molecular events in the initial phase of γδT cell development as follows: when both TCRγ and TCRδ chains are successfully rearranged and expressed in DN cells (these cells are defined as γδ-precursors), the fledgling γδTCR-CD3 complex induces Syk-mediated intracellular signaling in a ligand-independent manner. This initial TCR signaling upregulates γδT cell signature genes and downregulates those genes that are associated with TCR rearrangement and αβT cell differentiation. Thus, this sequential but rapidly unfolding process, referred to as “γδ-selection”, represents a checkpoint to ensure the generation of productive γδTCR distinct from the αβT cell lineage.
The Syk-mediated initial γδTCR signaling also involves the priming of γδT17 cell differentiation. This process is dependent on the unique ability of Syk to activate the PI3K-Akt pathway (18). It has been shown that γδT17 cells originate from distinct progenitors identified as cKit-CD24hiCD25-CD44+Sox13+ and this progenitor population is generated independently of γδTCR signaling (43). Thus, Syk-mediated γδTCR signaling may play a role in driving the progenitor cells to complete the differentiation program towards γδT17 cells. A recent study demonstrated that mice bearing mutations in CD3ε with attenuated γδTCR signaling exhibited decreased generation of γδT17 cells in the thymus (44). The weakened γδTCR signaling in this mouse strain is due to the lack of recruitment of adaptor protein Nck to the γδTCR-CD3 complex, indicating the requirement of Nck-mediated γδTCR signaling for γδT17 cell differentiation. Future studies are needed to determine how Nck and Syk cooperate for the activation of the PI3K-Akt pathway in order to drive γδT17 cell differentiation program.
A previous study has demonstrated that not only γδT cells but also RORγt+ ILCs contribute to the initiation of IMQ-induced psoriasis-like dermatitis (45). ILCs express NKp44 and NKp46 that is capable of coupling with immunoreceptor tyrosine-based activation motifs (ITAM)-containing molecules such as CD3ζ, DAP12, FcRγ. Either Syk or Zap70 is recruited to these ITAM-containing molecules (46), and may mediate the activation of intracellular signals in ILCs. In Syk-cKO mice, ILCs are expected to lack Syk alleles since ILCs are cells downstream of common lymphoid progenitors (CLP). Although quantitative and/or qualitative changes in ILCs from Syk-deficient mice have not been explored, it is possible that Syk is required for the exertion of ILC function. Because of this, we could not completely exclude the possibility that the amelioration of IMQ-induced dermatitis observed in Syk-cKO mice is caused by not only defects of γδT17 cells but also impairments of ILCs. In addition, our Syk-cKO mice have impairment of B cell development, which may also influence the induction of inflammatory responses. The use of Tcrd-driven Cre-expressing mice (47) would overcome this experimental limitation and allow a more rigorous elucidation of the role of Syk in early γδT cell development.
We detected a modest amount of γδT17 cells from the IMQ-treated Syk-cKO mice despite an almost complete lack of γδT17 cells in Syk-cKO mice at the steady state. Papotto et al., have previously shown that γδT17 cells can be induced from naïve γδT cells and/or expanded in LNs under the experimental autoimmune encephalomyelitis (EAE)-condition (48). Thus, it is likely that the γδT17 cells that emerged under the IMQ-condition are peripherally induced and/or expanded cells. Considering the fact that Zap70 is expressed on mature γδT cells and TCR signal enhances γδT17 differentiation in vitro (48), it is strongly suggested that Zap70, but not Syk, plays a central role in the regulation of γδTCR signal transduction for the peripheral induction and/or expansion of γδT17 cells.
In conclusion, it is shown that Syk plays a central role in both γδT cell commitment and γδT17 cell differentiation. These findings provide better insight into the molecular mechanisms underlying the effector determination of γδT cells and suggest that the Syk-mediated signaling pathways might be a therapeutic target in γδT17-dependent inflammatory diseases.
Data availability statement
The original contributions presented in the study are included in the article/Supplementary Material. Further inquiries can be directed to the corresponding author.
Ethics statement
The animal study was reviewed and approved by Institutional Animal Care and Use Committee of the University of Tokyo.
Author contributions
RM and TNi designed and performed most of the experiments. TNa performed the experiments. RM, TNi and HT interpreted the results and wrote the manuscript. HT supervised the project. All authors contributed to the article and approved the submitted version.
Funding
This work was supported by Grants-in-Aid for Research from the Japan Society for the Promotion of Science (JSPS) (KAKENHI 19K16602, 19J00248, 19H04802, 21H05046, 21K18254, 22H02886, 21K19371 and 22K07114), the Japan Agency for Medical Research and Development (AMED) (22gm1210008, JP223fa6270001 to HT), and the Japan Foundation for Applied Enzymology (TNi), a research grant from the Astellas Foundation for Research on Metabolic Disorders (no. 1102 to RM), Takeda Science Foundation (to RM).
Acknowledgments
We thank Dr. Hans-Reimer Rodewald (German Cancer Research Center) for providing the Cd127-Cre mouse strain, Dr. Robert E. Tigelaar (Yale University) for providing the 17D1 antibody, and the late professor Leo Lefrançois (University of Connecticut Health Center) for providing the GL1 antibody. We also would like to thank Sachiko Nitta and Koichi Kubo for technical assistance and all our laboratory members for helpful discussions.
Conflict of interest
The authors declare that the research was conducted in the absence of any commercial or financial relationships that could be construed as a potential conflict of interest.
Publisher’s note
All claims expressed in this article are solely those of the authors and do not necessarily represent those of their affiliated organizations, or those of the publisher, the editors and the reviewers. Any product that may be evaluated in this article, or claim that may be made by its manufacturer, is not guaranteed or endorsed by the publisher.
Supplementary material
The Supplementary Material for this article can be found online at: https://www.frontiersin.org/articles/10.3389/fimmu.2022.1045881/full#supplementary-material
Supplementary Figure 1 | Peripheral B cells, NK cells and neutrophiles in Syk-cKO mice. (A) Representative flow cytometric profiles of B220 and NK1.1 expression in spleen and lung cells. The graphs depict the number of B cells (B220+) and NK cells (NK1.1+). (B) Representative flow cytometric profiles of Gr-1 and Ly6C expression in spleen and lung cells. The neutrophils were distinguished based on the cell surface expression of Gr-1 and Ly6C according to a previous report by Cunin et al. (34). The graphs depict the number of neutrophils (Gr-1+ Ly6Cint). In (A, B), 5 to 8-week-old control mice (Cd127-cre Syk flox/wt, n = 4) or Syk-cKO mice (Cd127-cre Syk flox/flox, n = 6) were used. All data represent the mean ± SEM of four independent experiments. *P < 0.05 and **P < 0.01, by unpaired t-test.
Supplementary Figure 2 | Frequency and number of DN cell subpopulation in Syk-cKO mice. (A, B) The number (A) and frequency (B) of CD44+ CD25– (DN1), CD44+ CD25+ (DN2), CD44– CD25+ (DN3) and CD44– CD25– (DN4) cells in CD4– CD8– thymocytes from 5 to 8-week-old control mice (Cd127-cre Syk flox/wt, n = 6) or Syk-cKO mice (Cd127-cre Syk flox/flox, n = 7) are shown. All data represent the mean ± SEM of four independent experiments. All data represent the mean ± SEM of four independent experiments. *P < 0.05 and **P < 0.01, by 1-way ANOVA.
Supplementary Figure 3 | Cell surface expression of CD3ε and TCRδ in Syk-deficient thymic γδT cells. The mean fluorescence intensity (MFI) of CD3ε and TCRδ as gated in Figure 1E is shown. 4 to 6-week-old control mice (Cd127-cre Syk flox/wt, n = 6) or Syk-cKO mice (Cd127-cre Syk flox/flox, n = 7) were used. All data represent the mean ± SEM of three independent experiments. *P < 0.05 and **P < 0.01, by unpaired t-test.
Supplementary Figure 4 | The frequency of γδT cell subsets in the thymus and peripheral tissues. Graphs show the frequency of Vγ1+, Vγ4+, Vγ5Vδ1+, Vγ6+ and Vγ7+ γδT cells in the indicated tissues (n = 4 to 6). All data represent the mean ± SEM of three independent experiments. *P < 0.05 and **P < 0.01, by unpaired t-test.
Supplementary Figure 5 | αβT cells and B cells in IMQ-treated Syk-cKO mice. Representative flow cytometric profiles of B220 and TCRβ expression in cervical lymph node (cLN) from IMQ- or Vaseline-treated mice as shown in . The graphs depict the number of CD4+ T cells (CD4+ TCRβ+), CD8+ T cells (CD4+ TCRβ+) and B cells (B220+). Vaseline-treated control mice (Cd127-cre Syk flox/wt, n= 6), IMQ-treated control (n = 7) and IMQ-treated Syk-cKO (Cd127-cre Syk flox/flox n = 10) was used. All data represent the mean ± SEM of two independent experiments. *P < 0.05 and **P < 0.01, by 1-way ANOVA.
Supplementary Figure 6 | List of primers for quantitative PCR used in this study.
References
1. Mills KHG. IL-17 and IL-17-producing cells in protection versus pathology. Nat Rev Immunol (2022) 5:1–17. doi: 10.1038/s41577-022-00746-9
2. Silva-Santos B, Mensurado S, Coffelt SB. γδ T cells: pleiotropic immune effectors with therapeutic potential in cancer. Nat Rev Cancer (2019) 19(7):392–404. doi: 10.1038/s41568-019-0153-5
3. Ribot JC, Lopes N, Silva-Santos B. γδ T cells in tissue physiology and surveillance. Nat Rev Immunol (2021) 21(4):221–32. doi: 10.1038/s41577-020-00452-4
4. Konieczny P, Xing Y, Sidhu I, Subudhi I, Mansfield KP, Hsieh B, et al. Interleukin-17 governs hypoxic adaptation of injured epithelium. Science (2022) 377(6602):eabg9302. doi: 10.1126/science.abg9302
5. Mann AO, Hanna BS, Muñoz-Rojas AR, Sandrock I, Prinz I, Benoist C, et al. IL-17A-producing γδT cells promote muscle regeneration in a microbiota-dependent manner. J Exp Med (2022) 219(5):e20211504. doi: 10.1084/jem.20211504
6. Chung L, Maestas DR Jr., Lebid A, Mageau A, Rosson GD, Wu X, et al. Interleukin 17 and senescent cells regulate the foreign body response to synthetic material implants in mice and humans. Sci Transl Med (2020) 12(539):eaax3799. doi: 10.1126/scitranslmed.aax3799
7. Shibata K. Close link between development and function of gamma-delta T cells. Microbiol Immunol (2012) 56(4):217–27. doi: 10.1111/j.1348-0421.2012.00435.x
8. Irla M. Instructive cues of thymic T cell selection. Annu Rev Immunol (2022) 40:95–119. doi: 10.1146/annurev-immunol-101320-022432
9. Muro R, Takayanagi H, Nitta T. T Cell receptor signaling for γδT cell development. Inflamm Regener (2019) 39:6. doi: 10.1186/s41232-019-0095-z
10. Sumaria N, Grandjean CL, Silva-Santos B, Pennington DJ. Strong TCRγδ signaling prohibits thymic development of IL-17A-Secreting γδ T cells. Cell Rep (2017) 19(12):2469–76. doi: 10.1016/j.celrep.2017.05.071
11. Turchinovich G, Hayday AC. Skint-1 identifies a common molecular mechanism for the development of interferon-gamma-Secreting versus interleukin-17-Secreting gamma delta T cells. Immunity (2011) 35(1):59–68. doi: 10.1016/j.immuni.2011.04.018
12. Munoz-Ruiz M, Ribot JC, Grosso AR, Goncalves-Sousa N, Pamplona A, Pennington DJ, et al. TCR signal strength controls thymic differentiation of discrete proinflammatory gammadelta T cell subsets. Nat Immunol (2016) 17(6):721–7. doi: 10.1038/ni.3424
13. Mócsai A, Ruland J, Tybulewicz VL. The SYK tyrosine kinase: A crucial player in diverse biological functions. Nat Rev Immunol (2010) 10(6):387–402. doi: 10.1038/nri2765
14. Negishi I, Motoyama N, Nakayama K, Senju S, Hatakeyama S, Zhang Q, et al. Essential role for ZAP-70 in both positive and negative selection of thymocytes. Nature (1995) 376(6539):435–8. doi: 10.1038/376435a0
15. Duprez V, Blank U, Chrétien S, Gisselbrecht S, Mayeux P. Physical and functional interaction between p72(syk) and erythropoietin receptor. J Biol Chem (1998) 273(51):33985–90. doi: 10.1074/jbc.273.51.33985
16. Cheng AM, Negishi I, Anderson SJ, Chan AC, Bolen J, Loh DY, et al. The syk and ZAP-70 SH2-containing tyrosine kinases are implicated in pre-T cell receptor signaling. Proc Natl Acad Sci USA (1997) 94(18):9797–801. doi: 10.1073/pnas.94.18.9797
17. Palacios EH, Weiss A. Distinct roles for syk and ZAP-70 during early thymocyte development. J Exp Med (2007) 204(7):1703–15. doi: 10.1084/jem.20070405
18. Muro R, Nitta T, Nakano K, Okamura T, Takayanagi H, Suzuki H. γδTCR recruits the Syk/PI3K axis to drive proinflammatory differentiation program. J Clin Invest (2018) 128(1):415–26. doi: 10.1172/jci95837
19. Turner M, Mee PJ, Costello PS, Williams O, Price AA, Duddy LP, et al. Perinatal lethality and blocked b-cell development in mice lacking the tyrosine kinase syk. Nature (1995) 378(6554):298–302. doi: 10.1038/378298a0
20. Cheng AM, Rowley B, Pao W, Hayday A, Bolen JB, Pawson T. Syk tyrosine kinase required for mouse viability and b-cell development. Nature (1995) 378(6554):303–6. doi: 10.1038/378303a0
21. Saijo K, Schmedt C, Su IH, Karasuyama H, Lowell CA, Reth M, et al. Essential role of src-family protein tyrosine kinases in NF-kappaB activation during b cell development. Nat Immunol (2003) 4(3):274–9. doi: 10.1038/ni893
22. Schlenner SM, Madan V, Busch K, Tietz A, Läufle C, Costa C, et al. Fate mapping reveals separate origins of T cells and myeloid lineages in the thymus. Immunity (2010) 32(3):426–36. doi: 10.1016/j.immuni.2010.03.005
23. Roark CL, Aydintug MK, Lewis J, Yin X, Lahn M, Hahn YS, et al. Subset-specific, uniform activation among V gamma 6/V delta 1+ gamma delta T cells elicited by inflammation. J Leukoc Biol (2004) 75(1):68–75. doi: 10.1189/jlb.0703326
24. Goodman T, Lefrancois L. Intraepithelial lymphocytes. anatomical site, not T cell receptor form, dictates phenotype and function. J Exp Med (1989) 170(5):1569–81. doi: 10.1084/jem.170.5.1569
25. Muro R, Takayanagi H, Nitta T. Retroviral gene transduction into T cell progenitors for analysis of T cell development in the thymus. Methods Mol Biol (2020) 2111:193–203. doi: 10.1007/978-1-0716-0266-9_16
26. Ceredig R. The ontogeny of b cells in the thymus of normal, CD3 epsilon knockout (KO), RAG-2 KO and IL-7 transgenic mice. Int Immunol (2002) 14(1):87–99. doi: 10.1093/intimm/14.1.87
27. Xiao S, Zhang W, Manley NR. Thymic b cell development is controlled by the b potential of progenitors via both hematopoietic-intrinsic and thymic microenvironment-intrinsic regulatory mechanisms. PloS One (2018) 13(2):e0193189. doi: 10.1371/journal.pone.0193189
28. Hayes SM, Li L, Love PE. TCR signal strength influences alphabeta/gammadelta lineage fate. Immunity (2005) 22(5):583–93. doi: 10.1016/j.immuni.2005.03.014
29. Fang X, Zheng P, Tang J, Liu Y. CD24: from a to z. Cell Mol Immunol (2010) 7(2):100–3. doi: 10.1038/cmi.2009.119
30. Muñoz-Ruiz M, Sumaria N, Pennington DJ, Silva-Santos B. Thymic determinants of γδ T cell differentiation. Trends Immunol (2017) 38(5):336–44. doi: 10.1016/j.it.2017.01.007
31. Haas JD, Ravens S, Duber S, Sandrock I, Oberdorfer L, Kashani E, et al. Development of interleukin-17-producing gammadelta T cells is restricted to a functional embryonic wave. Immunity (2012) 37(1):48–59. doi: 10.1016/j.immuni.2012.06.003
32. van der Fits L, Mourits S, Voerman JS, Kant M, Boon L, Laman JD, et al. Imiquimod-induced psoriasis-like skin inflammation in mice is mediated via the IL-23/IL-17 axis. J Immunol (2009) 182(9):5836–45. doi: 10.4049/jimmunol.0802999
33. Cai Y, Shen X, Ding C, Qi C, Li K, Li X, et al. Pivotal role of dermal IL-17-producing γδ T cells in skin inflammation. Immunity (2011) 35(4):596–610. doi: 10.1016/j.immuni.2011.08.001
34. Cunin P, Lee PY, Kim E, Schmider AB, Cloutier N, Pare A, et al. Differential attenuation of β2 integrin-dependent and -independent neutrophil migration by Ly6G ligation. Blood Adv (2019) 3(3):256–67. doi: 10.1182/bloodadvances.2018026732
35. Ribot JC, deBarros A, Pang DJ, Neves JF, Peperzak V, Roberts SJ, et al. CD27 is a thymic determinant of the balance between interferon-gamma- and interleukin 17-producing gammadelta T cell subsets. Nat Immunol (2009) 10(4):427–36. doi: 10.1038/ni.1717
36. Sumaria N, Martin S, Pennington DJ. Constrained TCRγδ-associated syk activity engages PI3K to facilitate thymic development of IL-17A-secreting γδ T cells. Sci Signal (2021) 14(692):eabc5884. doi: 10.1126/scisignal.abc5884
37. Mallick-Wood CA, Pao W, Cheng AM, Lewis JM, Kulkarni S, Bolen JB, et al. Disruption of epithelial gamma delta T cell repertoires by mutation of the syk tyrosine kinase. Proc Natl Acad Sci USA (1996) 93(18):9704–9. doi: 10.1073/pnas.93.18.9704
38. Colucci F, Guy-Grand D, Wilson A, Turner M, Schweighoffer E, Tybulewicz VL, et al. A new look at syk in alpha beta and gamma delta T cell development using chimeric mice with a low competitive hematopoietic environment. J Immunol (2000) 164(10):5140–5. doi: 10.4049/jimmunol.164.10.5140
39. Cai Y, Xue F, Fleming C, Yang J, Ding C, Ma Y, et al. Differential developmental requirement and peripheral regulation for dermal Vγ4 and Vγ6T17 cells in health and inflammation. Nat Commun (2014) 5:3986. doi: 10.1038/ncomms4986
40. Kong GH, Bu JY, Kurosaki T, Shaw AS, Chan AC. Reconstitution of syk function by the ZAP-70 protein tyrosine kinase. Immunity (1995) 2(5):485–92. doi: 10.1016/1074-7613(95)90029-2
41. Jensen KD, Su X, Shin S, Li L, Youssef S, Yamasaki S, et al. Thymic selection determines gammadelta T cell effector fate: antigen-naive cells make interleukin-17 and antigen-experienced cells make interferon gamma. Immunity (2008) 29(1):90–100. doi: 10.1016/j.immuni.2008.04.022
42. Irving BA, Alt FW, Killeen N. Thymocyte development in the absence of pre-T cell receptor extracellular immunoglobulin domains. Science (1998) 280(5365):905–8. doi: 10.1126/science.280.5365.905
43. Spidale NA, Sylvia K, Narayan K, Miu B, Frascoli M, Melichar HJ, et al. Interleukin-17-Producing γδ T cells originate from SOX13(+) progenitors that are independent of γδTCR signaling. Immunity (2018) 49(5):857–872.e855. doi: 10.1016/j.immuni.2018.09.010
44. Borroto A, Alarcón B, Navarro MN. Mutation of the polyproline sequence in CD3ϵ evidences TCR signaling requirements for differentiation and function of pro-inflammatory Tγδ17 cells. Front Immunol (2022) 13 799919:799919. doi: 10.3389/fimmu.2022.799919
45. Pantelyushin S, Haak S, Ingold B, Kulig P, Heppner FL, Navarini AA, et al. Rorγt+ innate lymphocytes and γδ T cells initiate psoriasiform plaque formation in mice. J Clin Invest (2012) 122(6):2252–6. doi: 10.1172/jci61862
46. Colonna M. Innate lymphoid cells: Diversity, plasticity, and unique functions in immunity. Immunity (2018) 48(6):1104–17. doi: 10.1016/j.immuni.2018.05.013
47. Zhang B, Wu J, Jiao Y, Bock C, Dai M, Chen B, et al. Differential requirements of TCR signaling in homeostatic maintenance and function of dendritic epidermal T cells. J Immunol (2015) 195(9):4282–91. doi: 10.4049/jimmunol.1501220
Keywords: γδT cell, TCR signal, SYK (spleen tyrosine kinase), IL-17, thymus
Citation: Muro R, Narita T, Nitta T and Takayanagi H (2023) Spleen tyrosine kinase mediates the γδTCR signaling required for γδT cell commitment and γδT17 differentiation. Front. Immunol. 13:1045881. doi: 10.3389/fimmu.2022.1045881
Received: 16 September 2022; Accepted: 23 December 2022;
Published: 12 January 2023.
Edited by:
Alexandre M. Carmo, Universidade do Porto, PortugalReviewed by:
Noe Rodriguez-Rodriguez, MRC Laboratory of Molecular Biology (LMB), University of Cambridge, United KingdomAndreas Krueger, University of Giessen, Germany
Yoontae Lee, Pohang University of Science and Technology, Republic of Korea
Copyright © 2023 Muro, Narita, Nitta and Takayanagi. This is an open-access article distributed under the terms of the Creative Commons Attribution License (CC BY). The use, distribution or reproduction in other forums is permitted, provided the original author(s) and the copyright owner(s) are credited and that the original publication in this journal is cited, in accordance with accepted academic practice. No use, distribution or reproduction is permitted which does not comply with these terms.
*Correspondence: Takeshi Nitta, bml0LWltQG0udS10b2t5by5hYy5qcA==