- 1Department of Veterinary Microbiology and Pathology, College of Veterinary Medicine, Washington State University, Pullman, WA, United States
- 2Department of Parasitology, Faculty of Veterinary Medicine, University of Firat, Elazig, Turkey
- 3Parasitology and Animal Diseases Department, Veterinary Research Institute, National Research Center, Dokki, Giza, Egypt
- 4Animal Disease Research Unit, United States Department of Agricultural - Agricultural Research, Pullman, WA, United States
The apicomplexan tickborne parasites Babesia bovis and B. bigemina are the major causative agents of bovine babesiosis, a disease that negatively affects the cattle industry and food safety around the world. The absence of correlates of protection represents one major impediment for the development of effective and sustainable vaccines against bovine babesiosis. Herein we superinfected cattle with attenuated and virulent strains of B. bovis to investigate immune correlates of protection against acute bovine babesiosis. Three 6-month-old Holstein calves were infected intravenously (IV) with the in vitro culture attenuated Att-S74-T3Bo B. bovis strain (106 infected bovine red blood cells (iRBC)/calf) while three age-matched Holstein calves were inoculated IV with normal RBC as controls (106 RBC/calf). All Att-S74-T3Bo-infected calves showed a significant increase in temperature early after inoculation but recovered without treatment. Att-S74-T3Bo-infected calves also developed: (a) monocytosis, neutropenia, and CD4+ lymphopenia in peripheral blood on days 3 to 7 post-inoculation; (b) significant levels of TNFα, CXCL10, IFNγ, IL-4, and IL-10 in sera at day 6 after infection; and (c) IgM and IgG against B. bovis antigens, starting at days 10 and 30 post-inoculation, respectively. At 46 days post-Att-S74-T3Bo inoculation, all experimental calves were infected IV with the homologous virulent B. bovis strain Vir-S74-T3Bo (107 iRBC/calf). All Att-S74-T3Bo-infected calves survived superinfection with Vir-S74-T3Bo without displaying signs of acute babesiosis. In contrast, control animals showed signs of acute disease, starting at day 10 post-Vir-S74-T3Bo infection, and two of them were humanely euthanized at days 13 and 14 after inoculation due to the severity of their symptoms. Also, control calves showed higher (P<0.05) parasite load in peripheral blood compared to animals previously exposed to Att-S74-T3Bo. No significant alterations in the profile of leukocytes and cytokines were observed in Att-S74-T3Bo-inoculated after Vir-S74-T3Bo infection. In conclusion, data demonstrate novel changes in the profile of blood immune cells and cytokine expression in peripheral blood that are associated with protection against acute bovine babesiosis. These identified immune correlates of protection may be useful for designing effective and sustainable vaccines against babesiosis in cattle.
Introduction
Bovine babesiosis is an economically important tickborne disease of cattle caused by apicomplexan hemoparasites of the genus Babesia (1). Babesia bovis and B. bigemina are the primary causative agents of the disease, and it is estimated that more than 500 million cattle are at risk of contracting the disease in tropical and temperate endemic regions of the world (2–6). Pathogenesis of acute bovine babesiosis is characterized by severe anemia, fever, anorexia, and prostration, which may lead to death or establishment of persistent infection in animals that survive the acute phase of the disease. Acute B. bovis infection can also be presented by adhesion of infected red blood cells (iRBC) in the capillaries of the brain, liver, and lungs, among other vital organs, a condition that resembles severe malaria, resulting in high mortality (7, 8). Acaricides to decrease tick infestation, anti-babesicidal drugs, and live attenuated B. bovis and B. bigemina vaccines are the currently available control strategies against bovine babesiosis. The use of acaricides can select for resistant tick populations and cause toxicity for animals and environment (9). Therapeutic drugs are expensive, unpractical for large herds of cattle, and can also potentially lead to drug resistant parasites (10–12). Live attenuated vaccine strains are relatively efficient but are only used in some endemic areas due to several constraints, including: (a) variable parasite attenuation for naïve adult animals and, consequently, the utilization of these vaccines is usually restricted to young calves (<1-year old); (b) risk to transmit contaminating blood-borne pathogens; (c) the need of Babesia-naïve calves to produce the attenuated vaccine strains; (d) potential risk for reversion of virulence; (e) vaccinated animals become carriers/reservoirs of Babesia and a potential source for parasite tick acquisition/transmission; (f) vaccine batch variation with implications on quality control; and (g) the need for a cold chain for vaccine distribution and administration (1, 13–15). Combined, these factors clearly illustrate that more efficient and sustainable strategies to control bovine babesiosis are urgently needed.
It is well established that young cattle (<6 months of age) are more resistant to acute bovine babesiosis than adults (>1-year old animals) (16–18). The increased resistance of young animals to Babesia infection is spleen-dependent and associated with early activation of innate immune responses (19–22). Specifically, protection against B. bovis is correlated with the development of a pro-inflammatory phenotype that is initiated by splenic myeloid cells, such as monocytes and dendritic cells, which induces lymphocytes to produce IFNγ (19, 21, 23). Considering the role of splenic immune cells during B. bovis infection, delay in producing IFNγ with concomitant expression of IL-10 is associated with progression of acute disease (19, 20). Interestingly, cattle that survive the acute phase of babesiosis caused by B. bovis become chronically infected and reservoirs for tick acquisition but are protected against clinical disease upon reinfection with related parasite strains (1, 14, 24, 25). Collectively, these previous observations demonstrate that protective immunity against Babesia parasites is achievable, and a better understanding of this mechanism could help towards developing effective vaccines against bovine babesiosis.
Following the genome sequencing of the B. bovis T2Bo strain (26), a marked increase in understanding the parasite’s biology has emerged from functional studies using genetically modified parasites and high-throughput approaches (27–32). Therefore, several novel parasite blood and tick stage antigens have been discovered and evaluated in vaccine trials (31, 33–35). Availability of genome sequences and development of genetic tools for B. bovis also allowed the use of transfected parasites as a delivery system for tick vaccine antigens (36, 37). Despite the progress, an efficient subunit vaccine against B. bovis, or any other Babesia spp., has not yet been achieved. This failure is associated in part with the absence of well-defined immune correlates of protection, which represents an important knowledge gap that has hampered the development of effective and sustainable vaccines against babesiosis.
To address the knowledge gap on correlates of immune protection against B. bovis, in this study, we investigated the immune responses of cattle infected with the in vitro culture attenuated non-tick transmissible B. bovis S74-T3Bo strain (Att-S74-T3Bo) (37, 38). Development of protective immunity was assessed by superinfecting Att-S74-T3Bo-inoculated animals with the homologous virulent B. bovis S74-T3Bo strain (Vir-S74-T3Bo). Att-S74-T3Bo-infected calves developed an early significant monocytosis, neutropenia and CD4+ lymphopenia in peripheral blood. In addition, these animals showed a balanced profile of pro- and anti-inflammatory cytokines in peripheral blood and were fully protected against infection with Vir-S74-T3Bo. By focusing on peripheral blood, herein we identified novel immune correlates of protection that may have implications for the development of anti-B. bovis vaccines.
Material and methods
B. bovis strains
The B. bovis strain S74-T3Bo, which originated from the parental T2Bo strain (26), was used in this study. A batch of the S74-T3Bo strain has been maintained in culture, under standard conditions, as previously described (39), for more than 10 years. This strain has been shown to be attenuated for cattle and non-tick transmissible (37, 38), and consequently, termed Att-S74-T3Bo in this present study. Stabilates with the homologous virulent S74-T3Bo strain (Vir-S74-T3Bo), derived from experimentally infected cattle, were used to infect calves, as described in previous studies (19, 38, 40).
Att-S74-T3Bo and Vir-S74-T3Bo infections
A group of three male Holstein calves, 6 months of age, were inoculated intravenously (IV) with Att-S74-T3Bo iRBC (106 iRBC/calf). Inoculum was prepared from a fresh parasite culture. Another group of three male Holstein calves, 6 months of age, were inoculated IV with 106 normal RBC/calf and served as controls. After inoculation, all animals were monitored daily for signs of acute bovine babesiosis, including temperature, packed cell volume (PCV), B. bovis load in peripheral blood, and behavioral alterations. At day 46 after Att-S74-T3Bo inoculation, infected and control calves were infected IV with 107 Vir-74-T3Bo iRBC. This parasite dose has been shown to induce severe acute disease in cattle in previous studies (40–43). Following Vir-74-T3Bo infection, all calves were monitored daily for clinical signs of acute bovine babesiosis, as described above. All animal experiments were approved by the University of Idaho Institutional Animal Care and Use Committee (IACUC protocol number 2020-51).
B. bovis real-time quantitative PCR
Parasite load in peripheral blood of animals after infection was measured by real-time quantitative PCR (qPCR), as previously described (42). Briefly, peripheral blood from experimental animals was collected via jugular venipuncture into Vacutainer® tubes containing ethylenediamine tetra acetic acid (EDTA) (BD Company, Franklin Lakes, NJ) at several time-points after infection. Total genomic DNA from whole blood was extracted using the QIAamp® DNA Blood Mini Kit (QIAGen, Valencia, CA) following the manufacture’s protocol. Genomic DNA was used for qPCR to amplify the single copy B. bovis msa-1 gene. For the msa-1 qPCR, specific primers (5’ gatgcgtttgcacatgctaag 3’ and 5’ cgggtacttcggtgctctca 3’) and probe (FAM 5’-cacgctcaagtaggaaattttgttaaacctgga-3’ TAMRA) were used following the assay conditions described elsewhere (42).
Indirect ELISA to B. bovis rhoptry associated protein 1 C-terminal segment
The presence of antibodies in serum of infected animals against the C-terminal segment of the immunodominant antigen rhoptry associated protein 1 (RAP-1 CT) was evaluated by indirect ELISA (iELISA), as previously described (44). Briefly, peripheral blood was collected in Vacutainer® tubes with no anticoagulant, and serum samples were obtained by standard procedures. For iELISA, 96-well Immulon™ 2HB microtiter plates (Thermo Fisher Scientific, Waltham, MA) were coated overnight at 4 °C with 50 µl of recombinant B. bovis RAP-1 CT (2 µg/ml). Plates were then washed three times using 200 µl blocking buffer (0.2% I-Block™ in 1xPBS with 0.1% Tween 20) and blocked with 300 µl of the same buffer for one hour at 30 °CC. After blocking, bovine serum samples were diluted 1/10 in blocking buffer, and 50 µl was added to triplicate individual wells. Plates were incubated for one hour at 30 °CC and then washed five times in 200 µl blocking buffer. After that, 50 µl of a 1/1000 dilution of anti-bovine total IgG, IgG1, IgG2, or IgM peroxidase labeled secondary antibodies (SeraCare, Milford, MA) were added to each well, and plates were incubated for 45 minutes at 30 °CC. After incubation, plates were washed four times using 200 µl blocking buffer and two times with 200 µl 1xPBS with 0.1% Tween 20. Fifty-five µl of SureBlue™ TMB (SeraCare, Milford, MA) was then added to each well and plates were incubated for 10 minutes. Reaction was stopped by adding 55 µl TMB stop solution (SeraCare, Milford, MA), and absorbance was measured at 450 nm using the SpectraMax® 190 plate reader (Molecular Devices, San Jose, CA). Average values with standard deviations were then calculated and plotted to a chart.
Immunoblot
The presence of anti-B. bovis antibodies after infection was also examined by immunoblot. Briefly, total antigens (2 µg/lane) from uninfected bovine RBC, Att-S74-T3Bo iRBC (2% percentage of parasitized erythrocytes, PPE), or Vir-S74-T3Bo iRBC (2% PPE) were ran through 4-20% TGX™ gels (Bio-Rad Laboratories, Hercules, CA). Antigens were then transferred to nitrocellulose membranes using iBlot 2™ (Invitrogen, Waltham, MA), and the membranes were blocked in 5% milk overnight. Membranes were then washed one time in 1xPBS with 0.1% Tween 20 (PBS-T) and incubated with a 1/50 dilution of bovine serum in 5% milk for one hour rocking at 30 °CC. After that, membranes were washed three times using PBS-T and incubated with a 1/1000 dilution of anti-bovine IgG peroxidase labeled secondary antibodies (SeraCare, Milford, MA) in 5% milk for 30 minutes rocking at 30 °CC. Membranes were then washed again three times in PBS-T and sprayed with WesternBright® ECL-spray (Advansta, San Jose, CA). Visualization of immune complexes was performed using the Azure™ Imaging System (Azure Biosystems, Dublin, CA).
Blood cell count
Cell blood count was evaluated in all experimental animals after B. bovis infection using the ProCyte One™ Hematology Analyzer (IDEXX Laboratories, Westbrook, ME). Peripheral blood from the experimental animals was collected at several time-points after infection, as described above into Vacutainer® tubes containing EDTA (BD Company, Franklin Lakes, NJ). After collection, whole blood samples were homogenized for 5 minutes and the numbers of total leukocytes, lymphocytes, monocytes, neutrophils, RBC, and reticulocytes were measured. Results of each leukocyte population are presented as 1,000 cells/μl of blood, and RBC and reticulocyte are shown as 1,000,000 cells/μl of blood.
Phenotype of peripheral blood mononuclear cells by flow cytometric analysis
Peripheral blood from all experimental animals was collected at several time-points after infection in Vacutainer® tubes containing EDTA. Peripheral blood mononuclear cells (PBMC) were isolated using Histopaque®-1077 (Sigma, St. Louis, MO), as per a standard protocol. Cells were then labeled with monoclonal antibodies to surface markers (Table S1), followed by secondary antibodies (Table S2) using standard flow cytometry protocols, as previously described (45). Flow cytometric analysis was performed using the Guava® easyCyte™ flow cytometer (Luminex, Austin, TX), and data were acquired using the InCyte™ guavaSoft™ software version 3.1.1 (Luminex, Austin, TX). A single-color panel was used for phenotyping CD14+ monocytes, CD335+ NK cells, CD4+ T cells, CD8+ T cells, γδ T cells, and B cells in PBMC. A minimum of 20,000 events were collected for each cell population. After acquisition, data were analyzed using FCS Express™, version 6 (DeNovo™ Software, Pasadena, CA). Results are presented as percentage of target cell populations in PBMC.
Cytokine measurements
Concentration of cytokines in serum from infected animals was measured by ELISA. Bovine IFNγ was assessed by a single-metabolite assay (Bio-Rad Laboratories, Hercules, CA) following the manufacture’s protocol, using the SpectraMax® 190 plate reader (Molecular Devices, San Jose, CA). Results for IFNγ are presented as pg/ml and minimal detectable level was 25 pg/ml. Bovine IL-1β, IL-4, IL-6, IL-8, IL-10, CXCL10, and TNFα were evaluated by MILLIPLEX® Bovine Cytokine/Chemokine Magnetic Bead (Millipore-Sigma, Burlington, MA) following the manufacture’s protocol. Minimal detectable levels were as follows: 0.1 pg/ml IL-1β, 12.8 pg/ml IL-4, 2.6 pg/ml IL-6, 2.2 pg/ml IL-8, 0.96 pg/ml IL-10, 0.6 pg/ml CXCL10, and 12.8 pg/ml TNFα. Standard curves and quality controls for each cytokine in the multiplex assay were prepared following the manufacture’s protocol. The LX-200™ instrument (Luminex, Austin, TX) was used for the multiplex analysis. Data acquisition was done by xPONENT® software (Luminex, Austin, TX), and results were analyzed by Belysa® software (Millipore-Sigma, Burlington, MA).
Data analysis
Graphpad Prism software version 9 (GraphPad Software, San Diego, CA) was used for data analysis and to generate graphs. Results of temperature, PCV, parasite load, percentage of cells in blood, percentage of PBMC, and cytokines in serum are shown as mean and standard deviation of experimental and control samples. Means were compared with a two-tailed t test, and a P value of <0.05 was considered significant.
Results
Att-S74-T3Bo B. bovis infection of cattle
We have previously shown that the Att-S74-T3Bo B. bovis strain has an attenuated phenotype in cattle, and it is not tick transmissible (37, 38). However, no previous experiments have been performed to investigate the ability of this strain to induce a protective immune response in cattle against experimental infection with a virulent B. bovis strain. Therefore, as a starting point of our study, we systematically defined the characteristics of the Att-S74-T3Bo acute infection in cattle. A group of three calves was inoculated IV with 106 iRBC and monitored daily for signs of acute bovine babesiosis. Att-S74-T3Bo-inoculated animals showed mild but significant (P<0.05) elevation of temperature on days 6 (39.2 °CC [±0.41]) and 7 (39.1 °CC [±0.34]) post-infection compared to uninfected control calves (Figure 1A). Concomitantly, infected animals had decreased PCV on days 6 to 10 after infection; however, no statistical significance was observed compared to control animals (Figure 1B). Peak of parasite load (approx. 5x103 parasites/100 µl of blood) occurred at day 6 after Att-S74-T3Bo inoculation. Parasite DNA was then intermittently detected from days 12 to 30 post-inoculation (Figure 1C). Despite the increase in temperature, drop in PCV, and parasitemia, the Att-S74-T3Bo-infected calves did not develop additional signs of acute babesiosis, such as anorexia and neurological symptoms, and were able to control acute infection, transitioning to subclinical infection without the administration of anti-pyretic or babesicidal therapeutics. Collectively, results confirmed that the Att-S74-T3Bo B. bovis strain establishes infection in calves, which is characterized by moderate parasite replication in peripheral blood, significant increase in temperature, and marginal drop in PCV.
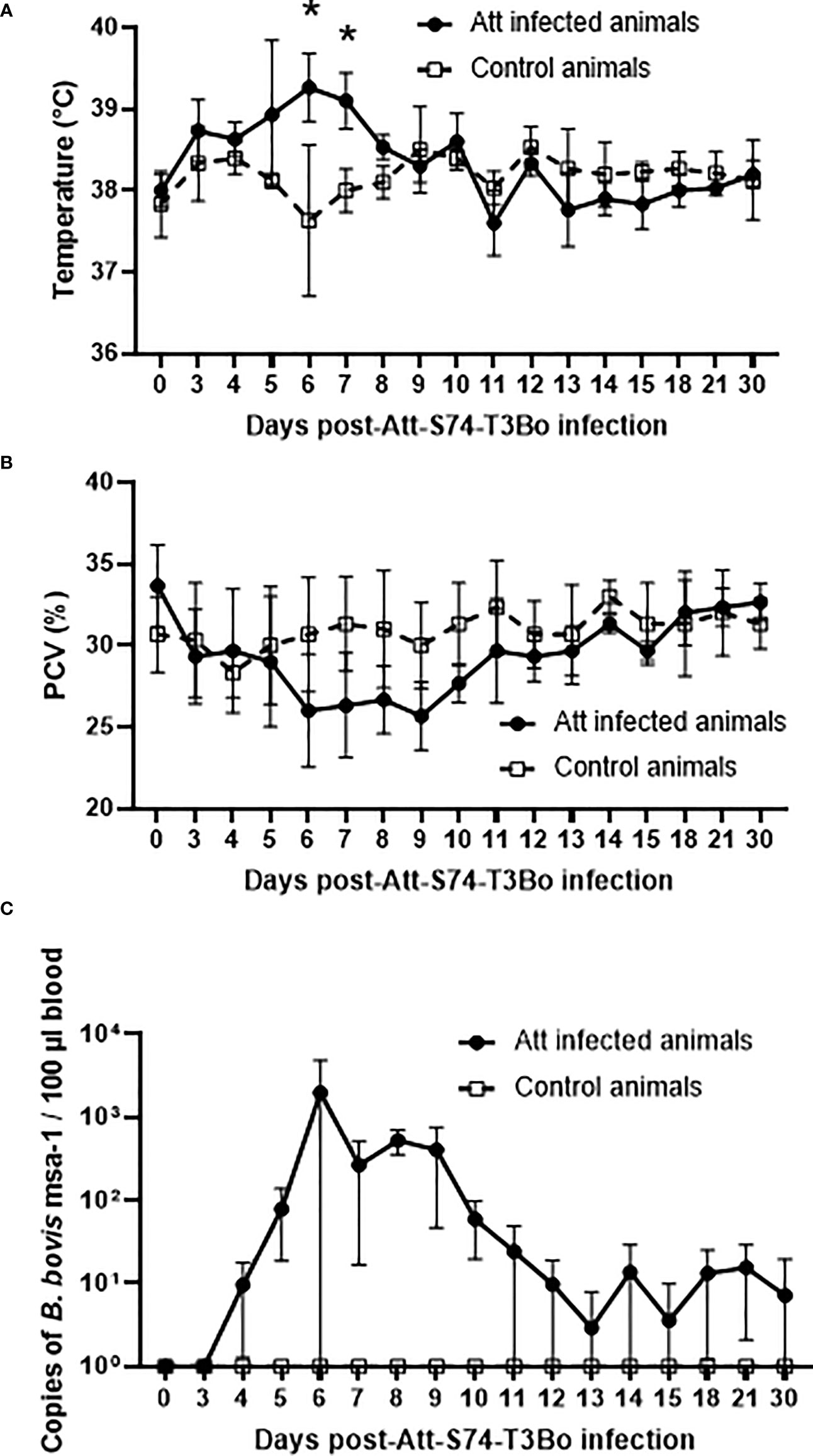
Figure 1 Temperature (A), packed cell volume (PCV) (B), and B. bovis load in peripheral blood accessed by real-time quantitative PCR (C) after infection with the in vitro culture attenuated B bovis strain Att-S74-T3Bo. Att infected animals (n=3). Control animals (n=3). * P<0.05.
Cattle infected with Att-S74-T3Bo B. bovis are protected against Vir-S74-T3Bo
After demonstrating that Att-S74-T3Bo infected calves without causing severe acute disease, we further investigated whether these animals were protected against the homologous virulent strain Vir-S74-T3Bo. To test this proposition, Att-S74-T3Bo-inoculated and control calves were infected IV with 107 Vir-S74-T3Bo iRBC at day 46 after the Att-S74-T3Bo infection. All animals were monitored daily for clinal signs of acute bovine babesiosis and parasite load in peripheral blood. A significant increase (P<0.05) in temperature, combined with a marked drop in PCV, was observed in all three control animals starting at day 11 after Vir-S74-T3Bo infection compared to the calves previously inoculated with Att-S74-T3Bo (Figures 2A, B). Following the regulations of the approved IACUC protocol, two calves in the control group were humanely euthanized at days 13 and 14 after Vir-S74-T3Bo infection due to the severity of the acute disease. Temperature of the control calf that did not succumb to acute disease returned to physiological levels around day 16 post-infection; however, the animal’s PCV values remained low until day 21 after infection (Figure 2B). No significant alterations in temperature and PCV were observed in the calves previously infected with Att-S74-T3Bo (Figures 2A, B). Parasite load in Att-S74-T3Bo-infected calves reached a peak of 5x103 copies of B. bovis msa-1/100 µl of blood at day 9 after Vir-S74-T3Bo superinfection, and then it was intermittently detected until 45 days post-superinfection when the experiment was terminated (Figure 2C). In contrast, control animals showed a significant increase (P<0.05) in parasite load starting at day 10 after Vir-S74-T3Bo infection, which peaked at day 13 post-infection (approximately 106 copies of B. bovis msa-1/100 µl of blood). The control calf that survived acute disease exhibited a decrease in parasite load to approximately 104-105 copies of B. bovis msa-1/100 µl of blood, starting at day 15 post-Vir-S74-T3Bo infection (Figure 2C). Altogether, results demonstrate that calves previously infected with Att-S74-T3Bo were fully protected against the virulent homologous strain Vir-S74-T3Bo.
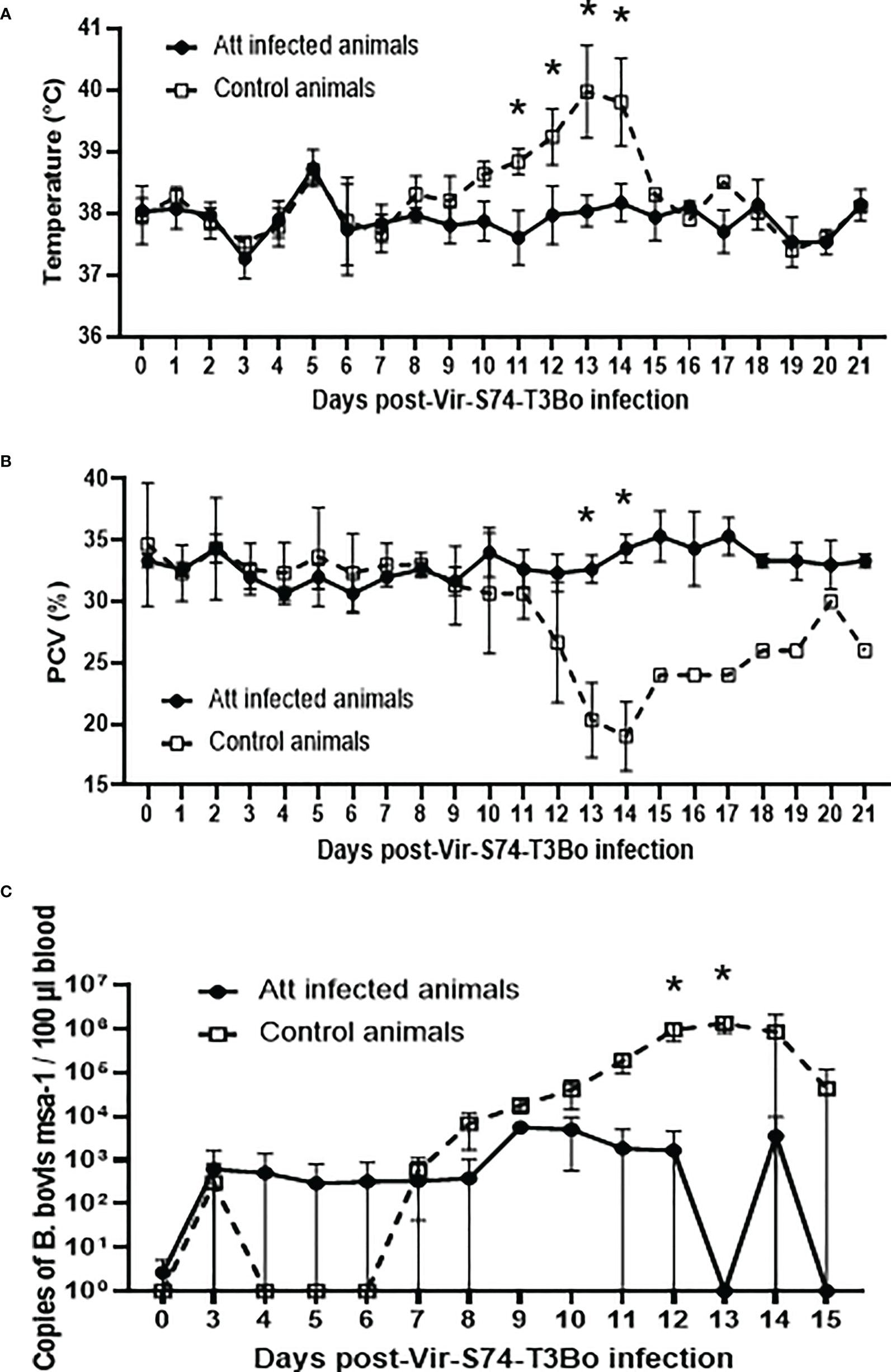
Figure 2 Temperature (A), packed cell volume (PCV) (B), and B. bovis load in peripheral blood accessed by real-time quantitative PCR (C) after infection with the virulent B bovis strain Vir-S74-T3Bo. Att infected animals (n=3). Control animals (n=3; two animals of the control group were humanely euthanized at days 13 and 14 after Vir-S74-T3Bo inoculation due to the severity of the disease). * P<0.05.
Alterations in peripheral blood cells of B. bovis-protected cattle
The observation that Att-S74-T3Bo infection protects cattle against a homologous virulent strain prompted us to investigate alterations in blood cells in the protected animals (Figure 3). Despite a marginal decreased in total leukocytes and lymphocytes at day 3 after Att-S74-T3Bo infection, and subsequently through days 10 and 14 after Vir-S74-T3Bo infection in control animals, no significant alterations were observed in these cell populations among experimental animals (Figures 3A–D). In contrast, data demonstrated a significant (P<0.05) monocytosis on days 7 and 10 post-Att-S74-T3Bo infection (Figure 3E). No alterations in monocytes were detected in Att-S74-T3Bo-inoculated calves after superinfection; however, the one control animal that survived Vir-S74-T3Bo infection showed an increase of monocytes starting at day 16 after infection (Figure 3F). A significant decrease in neutrophils in peripheral blood was observed starting at day 3 post-Att-S74-T3Bo inoculation, which returned to physiological levels at day 10 post-infection (Figure 3G). Neutrophils also decreased significantly in the control animals starting at 12 days after Vir-S74-T3Bo infection, but no alterations were detected in Att-S74-T3Bo-inoculated animals post-Vir-S74-T3Bo superinfection (Figure 3H). Att-S74-T3Bo infection did not alter the total number of RBC (Figure 3I), confirming our observation of the absence of significant changes in PCV after infection. On the other hand, control animals developed a dramatic and significant decrease in the number of RBC starting at day 12 after Vir-S74-T3Bo infection (Figure 3J). No significant changes in the absolute numbers of reticulocytes after infections with Att-S74-T3Bo and Vir-S74-T3Bo were observed, except for the one control calf that survived Vir-S74-T3Bo infection that showed a peak of reticulocytes at day 18 post-infection (Figures 3K, L). Combined, these results demonstrate significant alterations of monocytes and neutrophils in peripheral blood starting at day 3 after Att-S74-T3Bo infection. No significant changes were observed in blood cells of the protected Att-S74-T3Bo-inoculated animals after superinfection with Vir-S74-T3Bo. Interestingly, the control calf that did not succumb to Vir-S74-T3Bo infection developed a similar but delayed pattern of alterations for neutrophils and monocytes compared to protected Att-S74-T3Bo-infected animals.
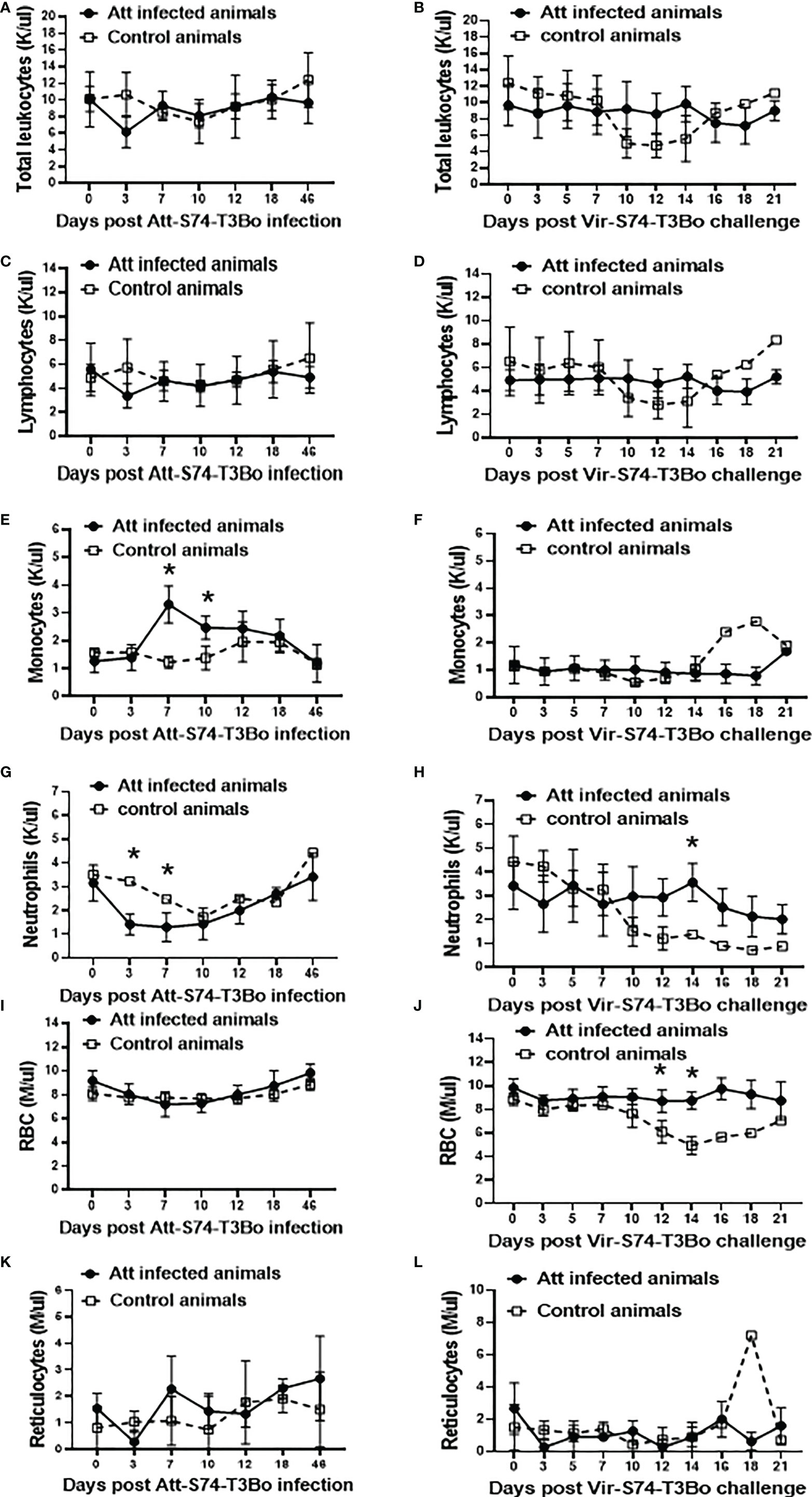
Figure 3 Absolute numbers of total leukocytes (A, B), lymphocytes (C, D), monocytes (E, F), neutrophils (G, H), red blood cells (RBC) (I, J), and reticulocytes (K, L) after infections with the in vitro culture attenuated B. bovis strain Att-S74-T3Bo and the virulent B. bovis strain Vir-S74-T3Bo. Att infected animals (n=3). Control animals (n=3; two animals of the control group were humanely euthanized at days 13 and 14 after Vir-S74-T3Bo inoculation due to the severity of the disease). * P<0.05.
Phenotypic changes in PBMC following Att-S74-T3Bo and Vir-S74-T3Bo infections
After showing significant alterations in monocytes and neutrophils in whole blood of protected calves, we investigated in more details the dynamics of PBMC populations in Att-S74-T3Bo-infected animals following Vir-S74-T3Bo superinfection. To that end, we used flow cytometric analysis to examine the percentage of CD4+ T cells, CD8+ T cells, γδ T cells, B cells, NK cells, and monocytes in PBMC during acute infection with Att-S74-T3Bo following superinfection with Vir-S74-T3Bo (Figure 4). Flow cytometry results of PBMC stained for CD4+ T cells, CD8+ T cells, γδ T cells, B cells, NK cells, and monocytes from a representative uninfected calf are presented in Figure 4A. Calves infected with Att-S74-T3Bo developed a moderate but significant lymphocytopenia of CD4+ T cells at day 7 post-infection (Figure 4B). No alterations in CD8+ T cells, γδ T cells, B cells, and NK cells were observed after Att-S74-T3Bo inoculation (Figure 4B). Percentage of monocytes in PBMC increased significantly in Att-S74-T3Bo-infected calves at day 7 post-infection compared to controls (Figure 4B), confirming our previous results in whole blood. No significant alterations were detected in CD4+ T cells, CD8+ T cells, γ™; T cells, B cells, and monocytes after Vir-S74-T3Bo infection (Figure 4C). A significant decrease in NK cells at day 9 after Vir-S74-T3Bo infection was observed in control calves in comparison to Att-S74-T3Bo-inoculated animals (Figure 4C). As demonstrated in whole blood, the calf that survived Vir-S74-T3Bo infection presented a marked increase of monocytes in PBMC; however, this alteration was delayed compared to the Att-S74-T3Bo-inoculated animals and occurred at day 15 post-Vir-S74-T3Bo infection (Figure 4C). In summary, data demonstrated early monocytosis combined with decrease of neutrophils and CD4+ T cells in peripheral blood of Att-S74-T3Bo-inoculated calves that were protected against Vir-S74-T3Bo. In addition, the control calf that survived infection did present monocytosis and neutropenia late after Vir-S74-T3Bo inoculation compared to Att-S74-T3Bo-inoculated protected animals.
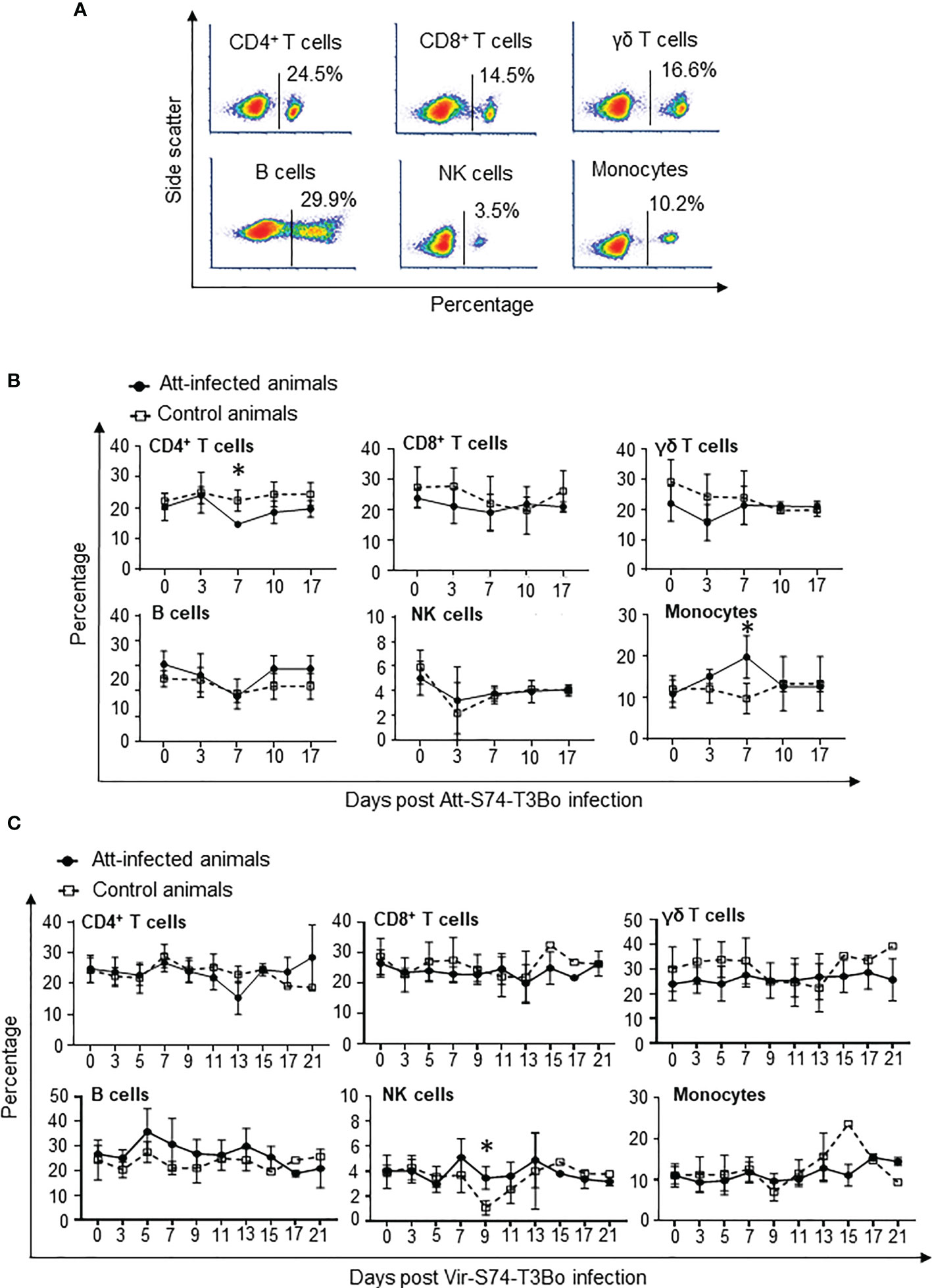
Figure 4 Kinetics of peripheral blood mononuclear cells (PBMC) after infections with the in vitro culture attenuated B. bovis strain Att-S74-T3Bo and the virulent B. bovis strain Vir-S74-T3Bo. Flow cytometric analysis showing PBMC from a normal, uninfected representative calf stained for CD4+ T cells, CD8+ T cells, γδ T cells, CD14+ monocytes, and CD335+ natural killer cells (NK) (A). Kinetics of CD4+ T cells, CD8+ T cells, γδ T cells, B lymphocytes, CD14+ monocytes, and CD335+ NK cells after Att-S74-T3Bo infection (B). Kinetics of CD4+ T cells, CD8+ T cells, γδ T cells, B lymphocytes, CD14+ monocytes, and CD335+ NK cells following Vir-S74-T3Bo infection (C). Att infected animals (n=3). Control animals (n=3; two animals of the control group were humanely euthanized at days 13 and 14 after Vir-S74-T3Bo inoculation due to the severity of the disease). * P<0.05.
Protective immune responses following Att-S74-T3Bo infection
After demonstrating alterations in the profile of blood cells following infections with Att-S74-T3Bo and Vir-S74-T3Bo, we investigated the immune responses induced in Att-S74-T3Bo-inoculated protected animals. Immunoblot results indicated that all three Att-S74-T3Bo-infected animals developed IgG antibodies to parasite antigens (Figure S1). Immunoblot data also showed that no antibodies to B. bovis antigens were detected in serum of the control calves 14 days after Vir-S74-T3Bo infection, when all three animals were exhibiting marked signs of acute babesiosis and two of them were euthanized due to the severity of the disease (Figure S1). In fact, antibodies to parasite antigens were detected in serum of the control calf that survived Vir-S74-T3Bo infection only at day 21 post-inoculation, by the time that acute disease had been resolved. iELISA was performed using the immune dominant B. bovis RAP-1 CT as antigen to investigate the level of IgM, total IgG, IgG1, and IgG2 after Att-S74-T3Bo and Vir-S74-T3Bo infections. Data demonstrated detectable levels of anti-RAP-1 CT IgM in all three Att-S74-T3Bo-infected animals starting at 10 days post-infection (Figure 5A). After that, the levels of IgM remained marginal and peaked dramatically again at day 21 after Vir-S74-T3Bo superinfection (67 days after Att-S74-T3Bo infection). Levels of total IgG and IgG1 against RAP-1 CT showed a sharp increase starting at day 30 after Att-S74-T3Bo infection, and it remained at those levels until the end of the experiment at 21 days post-Vir-S74-T3Bo superinfection (Figures 5B, C). Comparatively, anti-RAP-1 CT IgG2 levels were lower than IgG1, and one Att-S74-T3Bo-infected animal developed only marginal levels of this immunoglobulin subclass (Figure 5D). Noteworthy, no boost effect was observed in the levels of anti-RAP-1 CT IgG after Vir-S74-T3Bo superinfection. No presence of IgM, total IgG, IgG1, and IgG2 to RAP-1 CT were detected in control calves in the first 14 days after Vir-S74-T3Bo infection (Figure 5), confirming our immunoblot results described above. In fact, the control calf that survived Vir-S74-T3Bo infection developed only minimal levels of IgM and IgG starting at day 21 after inoculation when the symptoms of acute disease had been resolved (Figures 2, 3, 5). Next, we evaluated the presence of soluble polypeptides for IFNγ, IL-1β, IL-4, IL-6, IL-8, IL-10, CXCL10, and TNFα in serum of animals following infections with Att-S74-T3Bo and Vir-S74-T3Bo (Figure 6). Quantitative analysis revealed significant levels of the pro-inflammatory cytokines TNFα, IFNγ, and CXCL10 at day 6 after Att-S74-T3Bo infection compared to uninfected control animals (Figure 6A). Also, the Att-S74-T3Bo-infected calves developed significant levels of IL-10 at days 3 and 6 post-infection, and IL-4 at day 12 post-infection (Figure 6A). IL-6 and IL-8 were not detected in sera from Att-S74-T3Bo-infected animals (data not shown). No significant differences were found in the levels of TNFα, CXCL10, and IFNγ after infection with Vir-S74-T3Bo, despite the slightly higher concentration of these cytokines in serum from calves previously inoculated with Att-S74-T3Bo compared to controls (Figure 6B). No significant levels of IL-10 were detected in Att-S74-T3Bo-infected and control animals after Vir-S74-T3Bo infection, except for an increase of this cytokine in control calves at 13 days post-infection, at the time two control animals were euthanized due to the severity of acute disease (Figure 6B). Interestingly, Att-S74-T3Bo-inoculated animals showed significant levels of IL-4 at days 3 and 6 post-Vir-S74-T3Bo infection compared to controls (Figure 6B). No detectable levels of IL-6 and IL-8 were found in sera after Vir-S74-T3Bo infection (data not shown). Altogether, these data indicate that Att-S74-T3Bo infection results in the development of protective immune responses in calves characterized by early expression of a balance of inflammatory and regulatory cytokines in peripheral blood, and significant levels of antibodies to B. bovis antigen late during infection. In addition, no significant alteration in the levels of IFNγ, IL-1β, IL-4, IL-6, IL-8, IL-10, CXCL10, and TNFα was observed in peripheral blood after Vir-S74-T3Bo infection by comparing Att-S74-T3Bo-inoculated and naïve control animals.
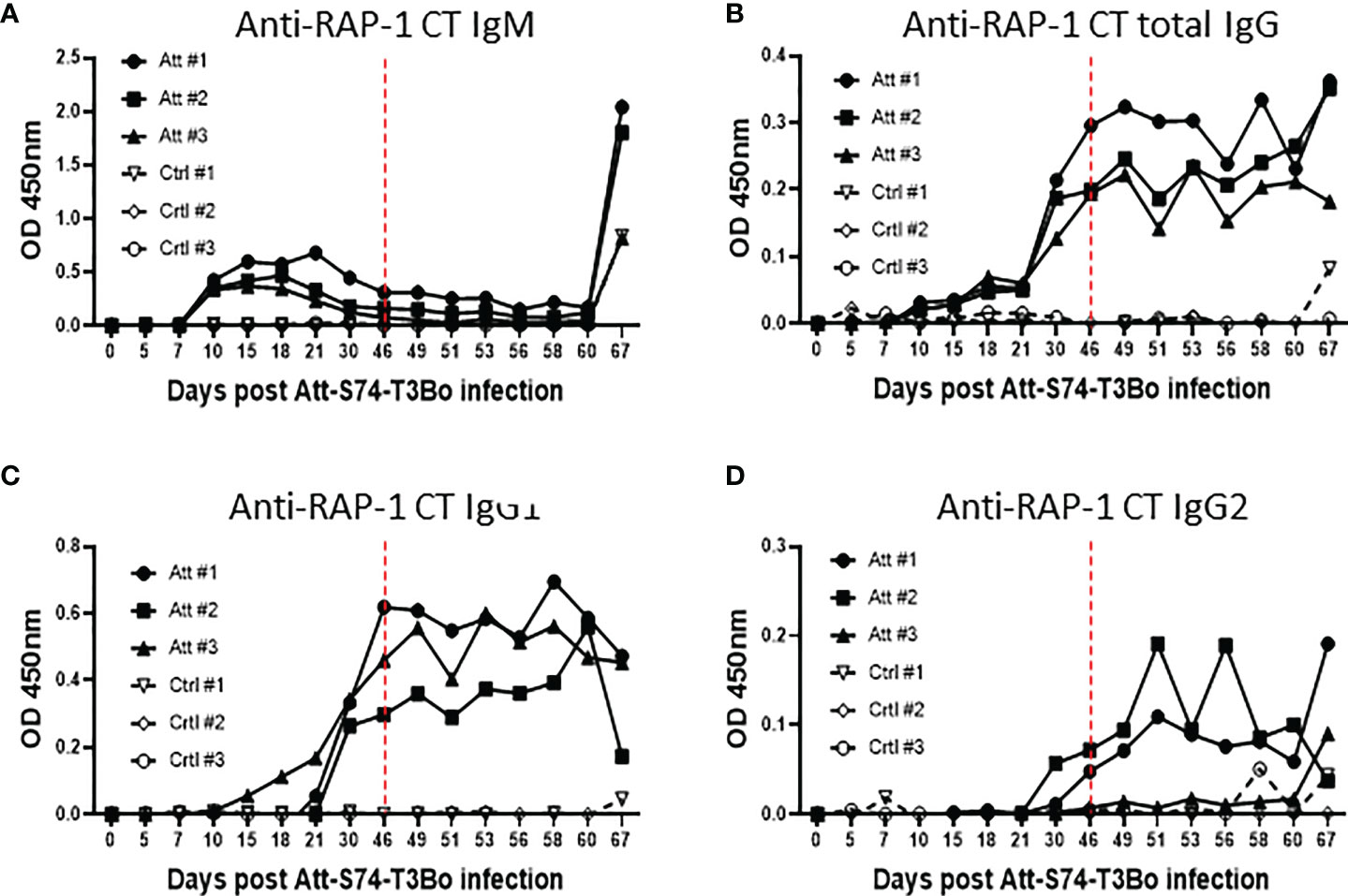
Figure 5 Humoral immune response accessed by indirect ELISA using the immunodominant antigen rhoptry associated protein 1 C-terminal (RAP-1 CT) after infections with the in vitro culture attenuated B. bovis strain Att-S74-T3Bo and the virulent B. bovis strain Vir-S74-T3Bo. Anti-RAP-1 CT IgM (A). Total anti-RAP-1 CT IgG (B). Anti-RAP-1 CT IgG1 (C). Anti-RAP-1 CT IgG2 (D). Results are presented as optical density (OD) of individual experimental animals. Att, animals infected with Att-S74-T3Bo and superinfected with Vir-S74-T3Bo. Ctrl, control animals infected with Vir-S74-T3Bo. Dashed red lines indicate day zero of Vir-S74-T3Bo infection.
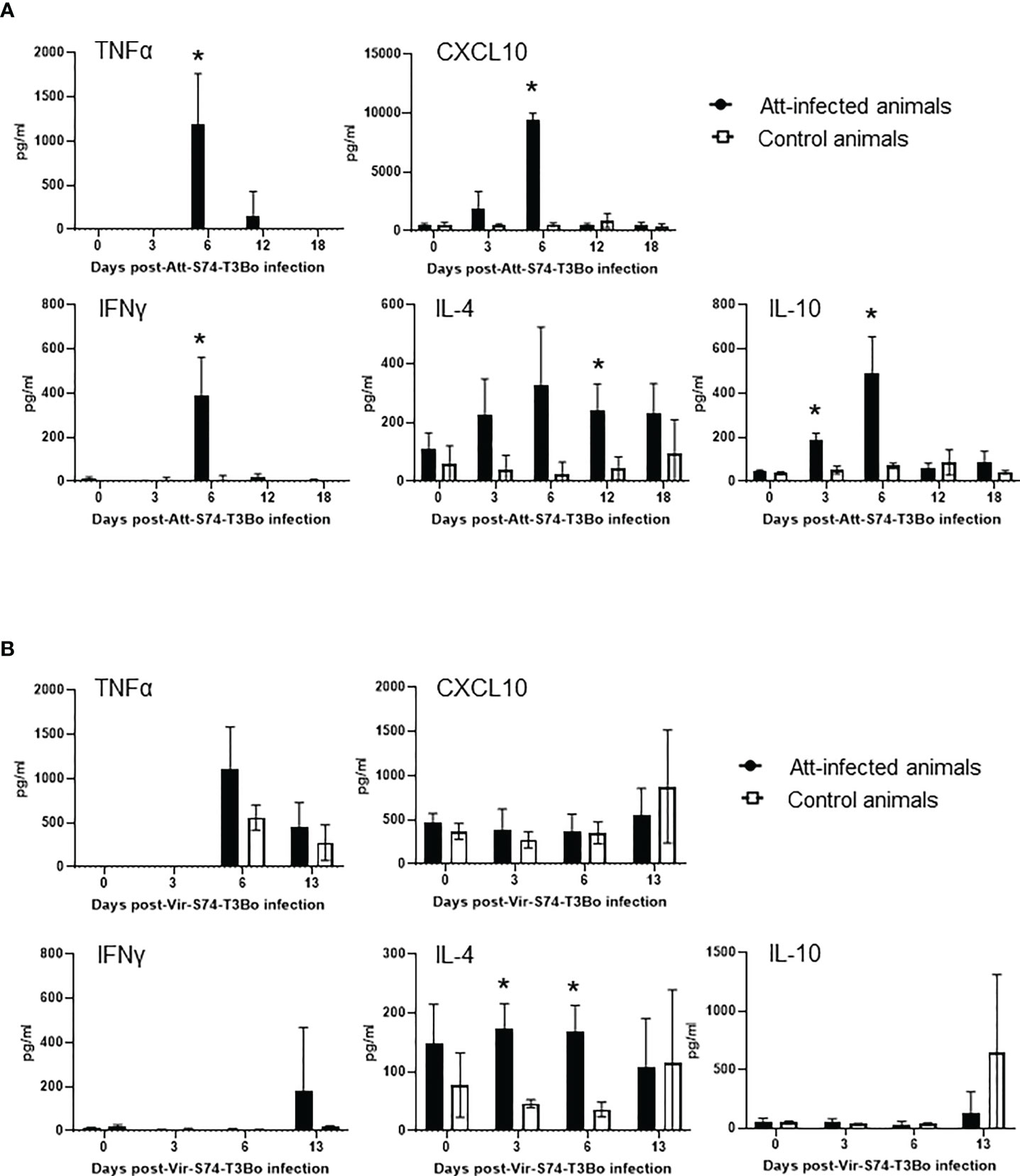
Figure 6 Presence of TNFα, CXCL10, IFNγ, IL-4, and IL-10 in peripheral blood of cattle infected with the in vitro culture attenuated B. bovis strain Att-S74-T3Bo (A) following superinfection with the virulent B. bovis strain Att-S74-T3Bo (B). Att infected animals (n=3). Control animals (n=3; two animals of the control group were humanely euthanized at days 13 and 14 after Vir-S74-T3Bo inoculation due to the severity of the disease). * P<0.05.
Discussion
The absence of well-defined immune correlates of protection and proper understanding of babesiosis pathogenesis are considered important aspects that hinder the development of effective vaccines against Babesia parasites. In this study we addressed these knowledge gaps by using cattle, the natural host of B. bovis. By infecting calves with the in vitro culture attenuated B. bovis strain Att-S74-T3Bo, followed by superinfection with the homologous virulent strain Vir-S74-T3Bo, we identified novel correlates of protection against acute babesiosis and revealed critical aspects of the infection caused by attenuated and virulent parasites. After Att-S74-T3Bo inoculation, we observed signs of acute infection in all calves characterized by marginal clinical signs and a marked increase in monocytes with concomitant decrease in neutrophils and CD4+ T cells in peripheral blood. These alterations in the kinetics of monocytes, neutrophils, and CD4+ T cells coincided with a significant increase in serum concentration of TNFα, IFNγ, CXCL10, and IL-10 early after infection. Data indicate that infection with this attenuated B. bovis strain elicited both innate and acquired immune responses, which protected the animals against superinfection with a homologous virulent strain. In Figure 7, we provide a synopsis of the present data and propose a model for the immunological and clinical events following Att-S74-T3Bo infection in naïve cattle (Figure 7A), Vir-S74-T3Bo superinfection in Att-S74-T3Bo-protected cattle (Figure 7B), and Vir-S74-T3Bo infection in naïve cattle (Figure 7C). Collectively, the results demonstrate that Att-S74-T3Bo infection induces early activation of immune responses, corroborating and expanding results from previously published studies in B. bovis (19–22).
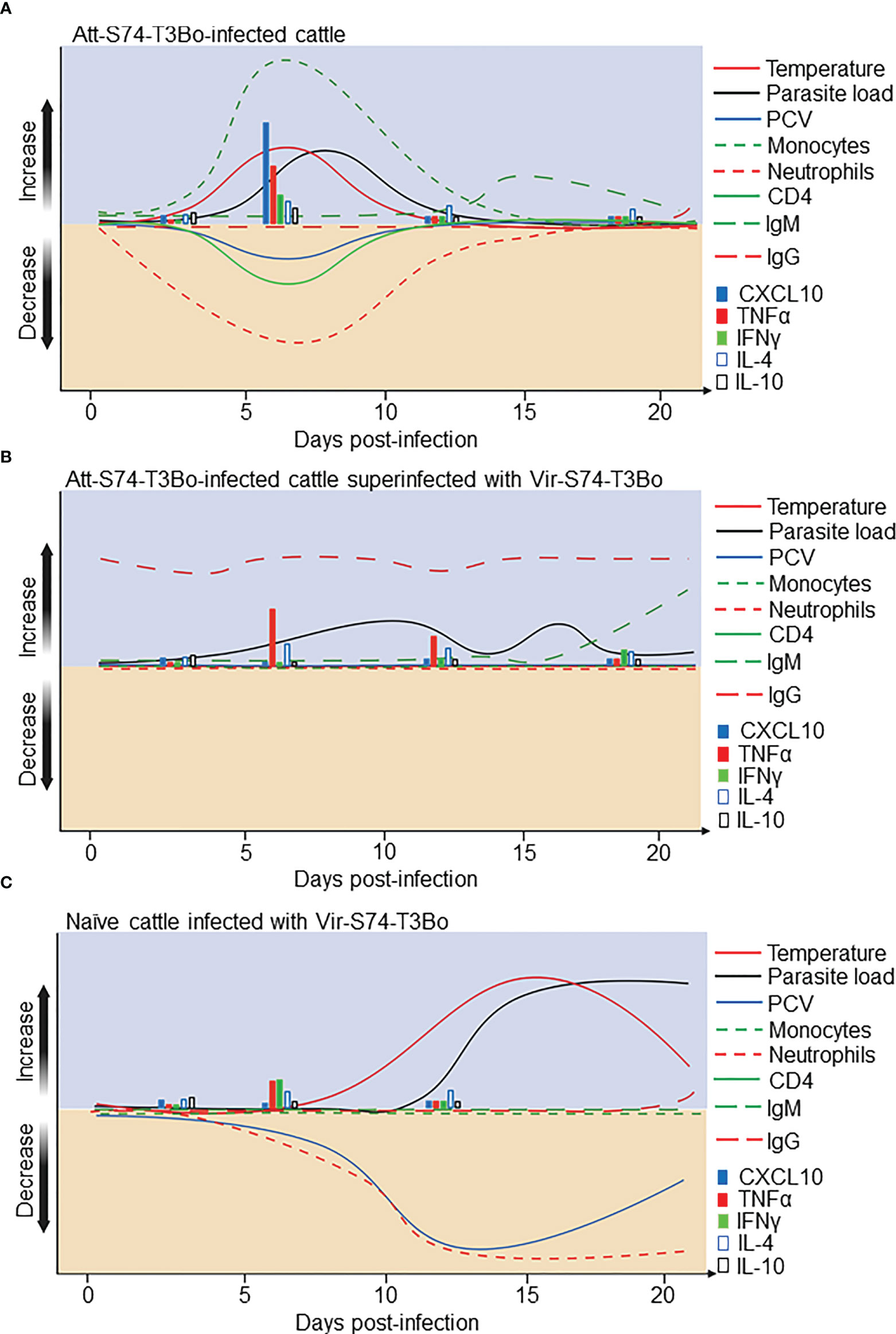
Figure 7 Proposed model for the immunological events after infection with the in vitro culture attenuated B. bovis strain Att-S74-T3Bo and superinfection with the virulent B. bovis strain Vir-S74-T3Bo. (A) Att-S74-T3Bo infection in naïve animals. (B) Vir-S74-T3Bo superinfection in Att-S74-T3Bo-protected. (C) Vir-S74-T3Bo infection in naïve animals.
Cells of the monocyte/macrophage linage have been shown to play an important role in protection against apicomplexan parasites, such as Babesia spp. and Plasmodium spp., among other parasite species (46–49). The present data reinforce this concept, considering the pronounced monocytosis in Att-S74-T3Bo-infected calves that were protected against superinfection with a homologous virulent parasite strain. Even though the early monocytosis observed in peripheral blood of protected animals is novel, it is important to highlight that this is in agreement with previous results in the bovine spleen and underscores the importance of early activation of myeloid cells in the development of protective immune responses against B. bovis (19, 23). The functional relevance of the observed monocytosis in peripheral blood is currently unknown; however, we speculate that a host and/or parasite factor(s) may induce monocytopoiesis in the bone marrow early during infection. Subsequently, these monocytes may migrate from the bone marrow to the peripheral blood and then to the spleen, playing a role as phagocytic cells and a source of cytokines and oxidation reagents that control parasite growth. Noteworthy, the one control calf that did not succumb to Vir-S74-T3Bo infection also developed neutropenia and monocytosis starting at days 10 and 16 post-infection, respectively, indicating a delay compared to protected Att-S74-T3Bo-inoculated animals. Also, an interesting result of our study was the CD4+ T cell lymphopenia observed at day 7 after Att-S74-T3Bo infection. It is possible that after infection, CD4+ T cells migrate from peripheral blood to the spleen, a major organ during infection with blood pathogens, such as Babesia (50–52). This recruitment of CD4+ T cells to the spleen early after infection may be important, considering that this T cell population is associated with protection against Babesia infection (22, 53). We observed no alterations in CD8+, γδ, and B cells in peripheral blood after Att-S74-T3Bo and Vir-S74-T3Bo infections. Our results on the dynamics of CD4+ and CD8+ T cells after B. bovis infection differ from previous observations following infection with an in vitro culture attenuated B. bigemina strain (54). This discrepancy may be due to differences in the pathogenesis of babesiosis caused by B. bovis and B. bigemina, and/or distinct biological characteristics of the attenuated parasite strains used in the two studies. In addition, we found that increase in B. bovis parasite load did not affect the number of reticulocytes in peripheral blood, despite the reduction of RBC in infected animals. This contrasts with data showing that high parasitemia of B. microti in vivo in peripheral blood is prevented by an increase in the number of reticulocytes, underlining a significant biological difference between B. bovis and B. microti (53, 55).
Initial innate immune events elicited by encountering Babesia parasites may define the magnitude, quality, and outcome of the infection. It has been previously described that early activation of innate immune responses in the spleen is associated with protection against acute bovine babesiosis (19–22, 56, 57). Here, we demonstrated that Att-S74-T3Bo infection induces alterations in innate immune myeloid and lymphoid cells in peripheral blood, eliciting significant levels of the pro-inflammatory cytokines TNFα, CXCL10, and IFNγ in sera as early as 3 days post-infection. Interestingly, the regulatory cytokines IL-10 and IL-4 were also detected during acute infection with Att-S74-T3Bo, which implies that this attenuated strain induced protective responses characterized by a balanced profile of pro- and anti-inflammatory cytokines in peripheral blood. Considering the absence of significant expression of pro-inflammatory cytokines in sera of naïve animals infected with Vir-S74-T3Bo compared to calves inoculated with Att-S74-T3Bo, it is possible to speculate that virulent B. bovis parasites may sabotage the bovine immune system, as previously demonstrated in the mouse model during B. microti infection (58). This premise is also supported by the lack of alterations in the kinetics of immune cells and the absence of antibodies to B. bovis antigens after infection of naïve animals that either succumbed to acute infection or survived the acute phase of the disease. In that context, it is also possible to infer that there is currently no evidence that the cattle immune system is involved in the pathogenesis of acute bovine babesiosis caused by B. bovis. This potential escape from and/or downregulation of the immune system provoked by virulent B. bovis contrasts with findings in other apicomplexan parasites that induce overwhelming responses that are associated with immunopathogenesis (45, 59–61). Further studies are needed to understand the molecular components of virulent B. bovis, in comparison to attenuated strains, that may be responsible for subverting the immune system of cattle, leading to delayed immune responses and pathogenesis.
Attenuation of Babesia parasites by in vitro cultivation has been demonstrated before (54, 62–64), and we have previously reported that the in vitro culture attenuated B. bovis strain Att-S74-T3Bo is a non-clonal parasite line with lower genetic diversity compared to its homologous virulent strain (38). This genetic alteration in Att-S74-T3Bo has likely occurred during the long-term in vitro cultivation period of this strain and may be responsible for its attenuated phenotype; however, the actual mechanism of attenuation remains unknown. The present study demonstrates that animals inoculated with Att-S74-T3Bo developed mild signs of acute disease and a significantly lower parasitemia compared to naïve animals infected with Vir-S74-T3Bo. Yet, this observation cannot solely explain the attenuated phenotype of the strain, considering the alterations in immune blood cells and elicitation of immune responses following Att-S74-T3Bo infection. In previous studies, we showed that cattle infected with either 2x108 or 107 Att-S74-T3Bo parasites presented mild signs of acute disease and recovered without treatment (37, 38). Here in the present work, we demonstrated that calves infected with a lower parasite dose (106 iRBC/calf) were not only infected but also developed protective immune responses against a homologous virulent B. bovis strain. Even though the attenuated phenotype of Att-S74-T3Bo has been confirmed by our work, additional studies are needed to investigate the molecular genetic mechanisms involved in attenuation of this strain compared to homologous and heterologous virulent parasites. Such mechanisms may involve the ability of virulent parasites to adhere to capillaries of vertebrates and/or express immune modulators. Also, it remains to be determined why naïve animals do not respond or present a delayed response to virulent B. bovis parasites. The relevance of this question is highlighted in our study where two naïve control animals that succumbed to acute disease on days 13 and 14 post-Vir-S74-T3Bo infection showed no alterations in the level of immune cells and cytokine profile compared to animals previously infected with attenuated parasites.
It has been demonstrated that antibodies against B. bovis antigens can neutralize the parasite growth in vitro and that passive transfer of sera from immune animals can protect naïve cattle from acute babesiosis (65–68). However, it is still unclear if the humoral immune response plays a role in protecting naïve animals from clinical signs of acute disease during primary infection. Here we show that all three naïve control calves developed severe acute disease, while two of them succumbed to acute infection, the third survived Vir-S74-T3Bo infection. Considering that the control calf that was able to control acute disease after Vir-S74-T3Bo inoculation without developing detectable antibodies, it is reasonable to speculate that humoral immune response is not essential for protection against a primary infection with a virulent parasite strain. Yet, it is possible that antibodies may play a critical role in protection against development of acute disease upon reinfection, as demonstrated in our study where Att-S74-T3Bo-infected calves, which developed significant levels of antibodies to parasite antigens, where fully protected against Vir-S74-T3Bo. The absence of significant alterations in the kinetics of immune cells and in the profile of cytokine expression in Att-S74-T3Bo-infected animals after Vir-S74-T3Bo superinfection also suggests that antibodies may play a role in protection upon reinfection. Additional studies are needed to evaluate the importance of the humoral immune response during primary and subsequent infections with B. bovis, and to identify novel antigens and/or epitopes that may be relevant for the elicitation of protective antibodies.
Understanding the immunological mechanisms induced by infection with attenuated B. bovis strains that protect cattle against acute disease upon primary infection and subsequent exposure to the parasite is critical to reveal immune correlates of protection and mechanisms of pathogenesis. This knowledge may lead to the rational design of vaccines to control bovine babesiosis. In that respect, based on the present data, we conclude that calves infected with Att-S74-T3Bo develop early activation of immune responses characterized by monocytosis, neutropenia, lymphopenia of CD4+ T cells, and a balanced expression profile of pro- and anti-inflammatory cytokines in peripheral blood (Figure 7). Strikingly, Att-S74-T3Bo-infected animals were protected against Vir-S74-T3Bo. Therefore, the immunological alterations in peripheral blood presented in this study can be considered correlates of protection against bovine babesiosis caused by B. bovis. The identification of novel immune markers of protection against B. bovis acute infection represents a step forward in our understanding of the protective mechanisms involved in bovine babesiosis. Based on the results, we propose that prospective vaccine trials should focus on live attenuated parasites, genetically modified live parasites, Babesia antigens, and novel adjuvants to induce early activation of myeloid cells, such as monocytes and neutrophils, and CD4+ T cells. In addition, future anti-Babesia vaccine evaluations should consider the development of a balanced profile of pro- and regulatory cytokines, especially TNFα, CXCL10, IFNγ, IL-10, and IL-4 in peripheral blood as a correlate of protection against acute disease. Considering the development of protective immunity in calves after infection with Att-S74-T3Bo, further investigations are needed to address the potential efficacy of this attenuated strain in preventing acute babesiosis in highly susceptible adult cattle and in eliciting protection against heterologous strains of B. bovis. Collectively, the findings of this study are relevant and represent a significant step toward the development effective vaccines against bovine babesiosis.
Data availability statement
The original contributions presented in the study are included in the article/Supplementary Material. Further inquiries can be directed to the corresponding authors.
Ethics statement
The animal study was reviewed and approved by University of Idaho Institutional Animal Care and Use Committee.
Author contributions
Conception and design: RB, CS. Collection and assembly of data: RB, JL, SO, CS. Data analysis and interpretation: RB, JL, SO, HA, NT, MU, CS. Manuscript writing: RB, JL, SO, HA, NT, MU, CS. Final approval of manuscript: All authors.
Funding
We acknowledge financial support from the USDA National Institute of Food and Agriculture (NIFA) (Award Number: 2020-67015-31809; Proposal Number: 2019-05375, Accession Number: 1022541), the International Development Research Center (IDRC) (Livestock Vaccine Innovation Fund (Grant 108525), funded by the Canadian Government and the Bill and Melinda Gates Foundation), and the United States Department of Agriculture (ARS-USDA CRIS 2090- 32000-040-00-D).
Acknowledgments
We wish to express our gratitude to Paul Lacy, Jinna Navas, and Megan Jacks for their excellent technical assistance and animal care.
Conflict of interest
The authors declare that the research was conducted in the absence of any commercial or financial relationships that could be construed as a potential conflict of interest.
Publisher’s note
All claims expressed in this article are solely those of the authors and do not necessarily represent those of their affiliated organizations, or those of the publisher, the editors and the reviewers. Any product that may be evaluated in this article, or claim that may be made by its manufacturer, is not guaranteed or endorsed by the publisher.
Supplementary material
The Supplementary Material for this article can be found online at: https://www.frontiersin.org/articles/10.3389/fimmu.2022.1045608/full#supplementary-material
Supplementary Figure 1 | Immunoblots using sera from cattle infected with the in vitro culture attenuated B. bovis strain Att-S74-T3Bo (n=3) and the virulent B. bovis strain Vir-S74-T3Bo (n=3). DPI, days post-infection. DPSI, days post-superinfection. nRBC, normal red blood cells. M, molecular weight in kilodaltons (KDa).
References
1. Bock RE, de Vos AJ, Kingston TG, Shiels IA, Dalgliesh RJ. Investigations of breakdowns in protection provided by living babesia bovis vaccine. Vet Parasitol (1992) 43(1-2):45–56. doi: 10.1016/0304-4017(92)90047-D
2. Bock R, Jackson L, de Vos A, Jorgensen W. Babesiosis of cattle. Parasitology (2004) 129 Suppl:S247–69. doi: 10.1017/S0031182004005190
3. Madison-Antenucci S, Kramer LD, Gebhardt LL, Kauffman E. Emerging tick-borne diseases. Clin Microbiol Rev (2020) 33(2):e00083–18. doi: 10.1128/cmr.00083-18
4. Schnittger L, Rodriguez AE, Florin-Christensen M, Morrison DA. Babesia: A world emerging. Infect Genet Evol (2012) 12(8):1788–809. doi: 10.1016/j.meegid.2012.07.004
5. Ozubek S, Bastos RG, Alzan HF, Inci A, Aktas M, Suarez CE. Bovine babesiosis in Turkey: Impact, current gaps, and opportunities for intervention. Pathogens (2020) 9(12):1041. doi: 10.3390/pathogens9121041
6. He L, Bastos RG, Sun Y, Hua G, Guan G, Zhao J, et al. Babesiosis as a potential threat for bovine production in China. Parasit Vectors (2021) 14(1):460. doi: 10.1186/s13071-021-04948-3
7. Sondgeroth KS, McElwain TF, Allen AJ, Chen AV, Lau AO. Loss of neurovirulence is associated with reduction of cerebral capillary sequestration during acute babesia bovis infection. Parasit Vectors (2013) 6(1):181. doi: 10.1186/1756-3305-6-181
8. Sondgeroth KS, McElwain TF, Ueti MW, Scoles GA, Reif KE, Lau AO. Tick passage results in enhanced attenuation of babesia bovis. Infect Immun (2014) 82(10):4426–34. doi: 10.1128/iai.02126-14
9. George JE, Pound JM, Davey RB. Chemical control of ticks on cattle and the resistance of these parasites to acaricides. Parasitology (2004) 129 Suppl:S353–66. doi: 10.1017/S0031182003004682
10. Kuttler KL, Johnson LW. Chemoprophylactic activity of imidocarb, diminazene and oxytetracycline against babesia bovis and b. Bigemina. Vet Parasitol (1986) 21(2):107–18. doi: 10.1016/0304-4017(86)90151-2
11. Batiha GE, Beshbishy AM, Alkazmi LM, Nadwa EH, Rashwan EK, Yokoyama N, et al. In vitro and in vivo growth inhibitory activities of cryptolepine hydrate against several babesia species and theileria equi. PloS Negl Trop Dis (2020) 14(8):e0008489. doi: 10.1371/journal.pntd.0008489
12. Silva MG, Bastos RG, Stone Doggett J, Riscoe MK, Pou S, Winter R, et al. Endochin-like quinolone-300 and elq-316 inhibit babesia bovis, b. bigemina, b. caballi and theileria equi. Parasit Vectors (2020) 13(1):606. doi: 10.1186/s13071-020-04487-3
13. de Castro JJ. Sustainable tick and tickborne disease control in livestock improvement in developing countries. Vet Parasitol (1997) 71(2-3):77–97. doi: 10.1016/S0304-4017(97)00033-2
14. Bock RE, de Vos AJ. Immunity following use of Australian tick fever vaccine: A review of the evidence. Aust Vet J (2001) 79(12):832–9. doi: 10.1111/j.1751-0813.2001.tb10931.x
15. Rathinasamy V, Poole WA, Bastos RG, Suarez CE, Cooke BM. Babesiosis vaccines: Lessons learned, challenges ahead, and future glimpses. Trends Parasitol (2019) 35(8):622–35. doi: 10.1016/j.pt.2019.06.002
16. Trueman KF, Blight GW. The effect of age on resistance of cattle to babesia bovis. Aust Vet J (1978) 54(6):301–5. doi: 10.1111/j.1751-0813.1978.tb02465.x
17. Levy MG, Clabaugh G, Ristic M. Age resistance in bovine babesiosis: Role of blood factors in resistance to babesia bovis. Infect Immun (1982) 37(3):1127–31. doi: 10.1128/iai.37.3.1127-1131.1982
18. Zintl A, Gray JS, Skerrett HE, Mulcahy G. Possible mechanisms underlying age-related resistance to bovine babesiosis. Parasite Immunol (2005) 27(4):115–20. doi: 10.1111/j.1365-3024.2005.00748.x
19. Goff WL, Johnson WC, Cluff CW. Babesia bovis immunity. In vitro and in vivo evidence for il-10 regulation of ifn-gamma and inos. Ann New York Acad Sci (1998) 849:161–80. doi: 10.1111/j.1749-6632.1998.tb11046.x
20. Goff WL, Johnson WC, Tuo W, Valdez RA, Parish SM, Barrington GM, et al. Age-related innate immune response in calves to babesia bovis involves il-12 induction and il-10 modulation. Ann New York Acad Sci (2002) 969:164–8. doi: 10.1111/j.1749-6632.2002.tb04371.x
21. Goff WL, Johnson WC, Horn RH, Barrington GM, Knowles DP. The innate immune response in calves to boophilus microplus tick transmitted babesia bovis involves type-1 cytokine induction and nk-like cells in the spleen. Parasite Immunol (2003) 25(4):185–8. doi: 10.1046/j.1365-3024.2003.00625.x
22. Brown WC, Norimine J, Knowles DP, Goff WL. Immune control of babesia bovis infection. Vet Parasitol (2006) 138(1-2):75–87. doi: 10.1016/j.vetpar.2006.01.041
23. Bastos RG, Johnson WC, Brown WC, Goff WL. Differential response of splenic monocytes and dc from cattle to microbial stimulation with mycobacterium bovis bcg and Babesia bovis merozoites. Vet Immunol Immunopathology (2007) 115(3-4):334–45. doi: 10.1016/j.vetimm.2006.11.001
24. De Vos AJ, Bock RE. Vaccination against bovine babesiosis. Ann New York Acad Sci (2000) 916:540–5. doi: 10.1111/j.1749-6632.2000.tb05333.x
25. Howell JM, Ueti MW, Palmer GH, Scoles GA, Knowles DP. Persistently infected calves as reservoirs for acquisition and transovarial transmission of Babesia bovis by Rhipicephalus (Boophilus) Microplus. J Clin Microbiol (2007) 45(10):3155–9. doi: 10.1128/jcm.00766-07
26. Brayton KA, Lau AO, Herndon DR, Hannick L, Kappmeyer LS, Berens SJ, et al. Genome sequence of babesia bovis and comparative analysis of apicomplexan hemoprotozoa. PloS Pathog (2007) 3(10):1401–13. doi: 10.1371/journal.ppat.0030148
27. Suarez CE, McElwain TF. Transient transfection of purified babesia bovis merozoites. Exp Parasitol (2008) 118(4):498–504. doi: 10.1016/j.exppara.2007.10.013
28. Suarez CE, McElwain TF. Stable expression of a gfp-bsd fusion protein in babesia bovis merozoites. Int J Parasitol (2009) 39(3):289–97. doi: 10.1016/j.ijpara.2008.08.006
29. Suarez CE, McElwain TF. Transfection systems for babesia bovis: A review of methods for the transient and stable expression of exogenous genes. Veterinary Parasitol (2010) 167(2-4):205–15. doi: 10.1016/j.vetpar.2009.09.022
30. Ueti MW, Johnson WC, Kappmeyer LS, Herndon DR, Mousel MR, Reif KE, et al. Transcriptome dataset of babesia bovis life stages within vertebrate and invertebrate hosts. Data Brief (2020) 33:106533. doi: 10.1016/j.dib.2020.106533
31. Ueti MW, Johnson WC, Kappmeyer LS, Herndon DR, Mousel MR, Reif KE, et al. Comparative analysis of gene expression between babesia bovis blood stages and kinetes allowed by improved genome annotation. Int J Parasitol (2021) 51(2-3):123–36. doi: 10.1016/j.ijpara.2020.08.006
32. Johnson WC, Hussein HE, Capelli-Peixoto J, Laughery JM, Taus NS, Suarez CE, et al. A transfected babesia bovis parasite line expressing egfp is able to complete the full life cycle of the parasite in mammalian and tick hosts. Infect Immun (2022) 11(6):1127–31. doi: 10.3390/pathogens11060623
33. Bastos RG, Suarez CE, Laughery JM, Johnson WC, Ueti MW, Knowles DP. Differential expression of three members of the multidomain adhesion ccp family in babesia bigemina, babesia bovis and theileria equi. PloS One (2013) 8(7):e67765. doi: 10.1371/journal.pone.0067765
34. Alzan HF, Lau AO, Knowles DP, Herndon DR, Ueti MW, Scoles GA, et al. Expression of 6-cys gene superfamily defines babesia bovis sexual stage development within rhipicephalus microplus. PloS One (2016) 11(9):e0163791. doi: 10.1371/journal.pone.0163791
35. Hussein HE, Bastos RG, Schneider DA, Johnson WC, Adham FK, Davis WC, et al. The babesia bovis Hap2 gene is not required for blood stage replication, but expressed upon in vitro sexual stage induction. PloS Negl Trop Dis (2017) 11(10):e0005965. doi: 10.1371/journal.pntd.0005965
36. Oldiges DP, Laughery JM, Tagliari NJ, Leite Filho RV, Davis WC, da Silva Vaz I Jr, et al. Transfected babesia bovis expressing a tick gst as a live vector vaccine. PloS Negl Trop Dis (2016) 10(12):e0005152. doi: 10.1371/journal.pntd.0005152
37. Mazuz ML, Laughery JM, Lebovitz B, Yasur-Landau D, Rot A, Bastos RG, et al. Experimental infection of calves with transfected attenuated babesia bovis expressing the rhipicephalus microplus Bm86 antigen and egfp marker: Preliminary studies towards a dual anti-Tick/Babesia vaccine. Pathogens (2021) 10(2):135. doi: 10.3390/pathogens10020135
38. Alzan HF, Bastos RG, Laughery JM, Scoles GA, Ueti MW, Johnson WC, et al. A culture-adapted strain of babesia bovis has reduced subpopulation complexity and is unable to complete its natural life cycle in ticks. Front Cell Infect Microbiol (2022) 12:827347. doi: 10.3389/fcimb.2022.827347
39. Erp EE, Gravely SM, Smith RD, Ristic M, Osorno BM, Carson CA. Growth of babesia bovis in bovine erythrocyte cultures. Am J Trop Med Hyg (1978) 27(5):1061–4. doi: 10.4269/ajtmh.1978.27.1061
40. Alzan HF, Bastos RG, Ueti MW, Laughery JM, Rathinasamy VA, Cooke BM, et al. Assessment of babesia bovis 6cys a and 6cys b as components of transmission blocking vaccines for babesiosis. Parasit Vectors (2021) 14(1):210. doi: 10.1186/s13071-021-04712-7
41. Taus NS, Cywes-Bentley C, Johnson WC, Pier GB, Fry LM, Mousel MR, et al. Immunization against a conserved surface polysaccharide stimulates bovine antibodies with opsonic killing activity but does not protect against babesia bovis challenge. Pathogens (2021) 10(12):1598. doi: 10.3390/pathogens10121598
42. Bastos RG, Ueti MW, Guerrero FD, Knowles DP, Scoles GA. Silencing of a putative immunophilin gene in the cattle tick rhipicephalus (Boophilus) microplus increases the infection rate of babesia bovis in larval progeny. Parasit Vectors (2009) 2(1):57. doi: 10.1186/1756-3305-2-57
43. Bastos RG, Ueti MW, Knowles DP, Scoles GA. The rhipicephalus (Boophilus) microplus Bm86 gene plays a critical role in the fitness of ticks fed on cattle during acute babesia bovis infection. Parasit Vectors (2010) 3:111. doi: 10.1186/1756-3305-3-111
44. Goff WL, McElwain TF, Suarez CE, Johnson WC, Brown WC, Norimine J, et al. Competitive enzyme-linked immunosorbent assay based on a rhoptry-associated protein 1 epitope specifically identifies babesia bovis-infected cattle. Clin Diagn Lab Immunol (2003) 10(1):38–43. doi: 10.1128/cdli.10.1.38-43.2003
45. Bastos RG, Sears K, Dinkel KD, Knowles DP, Fry LM. Changes in the molecular and functional phenotype of bovine monocytes during t. parva infection. Infect Immun (2019) 87(12):e00703–19. doi: 10.1128/iai.00703-19
46. Li Y, Terkawi MA, Nishikawa Y, Aboge GO, Luo Y, Ooka H, et al. Macrophages are critical for cross-protective immunity conferred by babesia microti against babesia rodhaini infection in mice. Infect Immun (2012) 80(1):311–20. doi: 10.1128/iai.05900-11
47. Chua CL, Brown G, Hamilton JA, Rogerson S, Boeuf P. Monocytes and macrophages in malaria: Protection or pathology? Trends Parasitol (2013) 29(1):26–34. doi: 10.1016/j.pt.2012.10.002
48. Hu S, Wei W, Korner H. The role of monocytes in models of infection by protozoan parasites. Mol Immunol (2017) 88:174–84. doi: 10.1016/j.molimm.2017.06.020
49. Dobbs KR, Crabtree JN, Dent AE. Innate immunity to malaria-the role of monocytes. Immunol Rev (2020) 293(1):8–24. doi: 10.1111/imr.12830
50. Goff WL, Bastos RG, Brown WC, Johnson WC, Schneider DA. The bovine spleen: Interactions among splenic cell populations in the innate immunologic control of hemoparasitic infections. Veterinary Immunol immunopathology (2010) 138(1-2):1–14. doi: 10.1016/j.vetimm.2010.07.006
51. Suarez CE, Alzan HF, Silva MG, Rathinasamy V, Poole WA, Cooke BM. Unravelling the cellular and molecular pathogenesis of bovine babesiosis: Is the sky the limit? Int J Parasitol (2019) 49:183–97. doi: 10.1016/j.ijpara.2018.11.002
52. Schneider DA, Yan H, Bastos RG, Johnson WC, Gavin PR, Allen AJ, et al. Dynamics of bovine spleen cell populations during the acute response to babesia bovis infection: An immunohistological study. Parasite Immunol (2011) 33(1):34–44. doi: 10.1111/j.1365-3024.2010.01249.x
53. Skariah S, Arnaboldi P, Dattwyler RJ, Sultan AA, Gaylets C, Walwyn O, et al. Elimination of babesia microti is dependent on intraerythrocytic killing and Cd4(+) T cells. J Immunol (Baltimore Md 1950) (2017) 199(2):633–42. doi: 10.4049/jimmunol.1601193
54. Rauf U, Suleman M, Abid A, Jamil H, Menghwar H, Durrani AZ, et al. Humoral and cell-mediated immune response validation in calves after a live attenuated vaccine of babesia bigemina. Pathogens (2020) 9(11):936. doi: 10.3390/pathogens9110936
55. Borggraefe I, Yuan J, Telford SR 3rd, Menon S, Hunter R, Shah S, et al. Babesia microti primarily invades mature erythrocytes in mice. Infect Immun (2006) 74(6):3204–12. doi: 10.1128/iai.01560-05
56. Brown WC, Palmer GH. Designing blood-stage vaccines against babesia bovis and b. bigemina. Parasitol Today (1999) 15(7):275–81. doi: 10.1016/S0169-4758(99)01471-4
57. Brown WC, Norimine J, Goff WL, Suarez CE, McElwain TF. Prospects for recombinant vaccines against babesia bovis and related parasites. Parasite Immunol (2006) 28(7):315–27. doi: 10.1111/j.1365-3024.2006.00849.x
58. Djokic V, Akoolo L, Primus S, Schlachter S, Kelly K, Bhanot P, et al. Protozoan parasite babesia microti subverts adaptive immunity and enhances Lyme disease severity. Front Microbiol (2019) 10:1596. doi: 10.3389/fmicb.2019.01596
59. Rocha BC, Marques PE, Leoratti FMS, Junqueira C, Pereira DB, Antonelli L, et al. Type I interferon transcriptional signature in neutrophils and low-density granulocytes are associated with tissue damage in malaria. Cell Rep (2015) 13(12):2829–41. doi: 10.1016/j.celrep.2015.11.055
60. Edwards CL, Best SE, Gun SY, Claser C, James KR, de Oca MM, et al. Spatiotemporal requirements for Irf7 in mediating type I ifn-dependent susceptibility to blood-stage plasmodium infection. Eur J Immunol (2015) 45(1):130–41. doi: 10.1002/eji.201444824
61. Fry LM, Schneider DA, Frevert CW, Nelson DD, Morrison WI, Knowles DP. East Coast fever caused by Theileria parva is characterized by macrophage activation associated with vasculitis and respiratory failure. PloS One (2016) 11:1–20. doi: 10.1371/journal.pone.0156004
62. Sachman-Ruiz B, Lozano L, Lira JJ, Martínez G, Rojas C, Álvarez JA, et al. A comparative genomic study of attenuated and virulent strains of babesia bigemina. Pathogens (2021) 10(3):318. doi: 10.3390/pathogens10030318
63. Alvarez JA, Rojas C, Figueroa JV. An overview of current knowledge on in vitro babesia cultivation for production of live attenuated vaccines for bovine babesiosis in Mexico. Front Vet Sci (2020) 7:364(364). doi: 10.3389/fvets.2020.00364
64. Rojas-Martinez C, Rodriguez-Vivas RI, Millan JVF, Bautista-Garfias CR, Castaneda-Arriola RO, Lira-Amaya JJ, et al. Bovine babesiosis: Cattle protected in the field with a frozen vaccine containing babesia bovis and babesia bigemina cultured in vitro with a serum-free medium. Parasitol Int (2018) 67(2):190–5. doi: 10.1016/j.parint.2017.11.004
65. Mahoney DF. Studies on the protection of cattle against babesia bovis infection. In: Morrison WI, editor. The ruminant immune system in health and disease. (New York, NY, USA: Cambridge University Press) (1986). p. 539–54.
66. Hines SA, Palmer GH, Jasmer DP, McGuire TC, McElwain TF. Neutralization-sensitive merozoite surface antigens of babesia bovis encoded by members of a polymorphic gene family. Mol Biochem Parasitol (1992) 55(1-2):85–94. doi: 10.1016/0166-6851(92)90129-8
67. Suarez CE, Laughery JM, Bastos RG, Johnson WC, Norimine J, Asenzo G, et al. A novel neutralization sensitive and subdominant rap-1-Related antigen (Rra) is expressed by babesia bovis merozoites. Parasitology (2011) 138(7):809–18. doi: 10.1017/s0031182011000321
68. Hidalgo-Ruiz M, Suarez CE, Mercado-Uriostegui MA, Hernandez-Ortiz R, Ramos JA, Galindo-Velasco E, et al. Babesia bovis Ron2 contains conserved b-cell epitopes that induce an invasion-blocking humoral immune response in immunized cattle. Parasit Vectors (2018) 11(1):575. doi: 10.1186/s13071-018-3164-2
Keywords: bovine babesiosis, Babesia bovis, vaccine, Babesia immunity, Babesia pathogenesis, Babesia immune correlates of protection
Citation: Bastos RG, Laughery JM, Ozubek S, Alzan HF, Taus NS, Ueti MW and Suarez CE (2022) Identification of novel immune correlates of protection against acute bovine babesiosis by superinfecting cattle with in vitro culture attenuated and virulent Babesia bovis strains. Front. Immunol. 13:1045608. doi: 10.3389/fimmu.2022.1045608
Received: 15 September 2022; Accepted: 02 November 2022;
Published: 18 November 2022.
Edited by:
Irene S. Soares, University of São Paulo, BrazilReviewed by:
Monica Florin-Christensen, Consejo Nacional de Investigaciones Científicas y Técnicas (CONICET), Argentinalba Marina Gimenez, Institute of Biomedical Sciences, University of São Paulo, Brazil
Copyright © 2022 Bastos, Laughery, Ozubek, Alzan, Taus, Ueti and Suarez. This is an open-access article distributed under the terms of the Creative Commons Attribution License (CC BY). The use, distribution or reproduction in other forums is permitted, provided the original author(s) and the copyright owner(s) are credited and that the original publication in this journal is cited, in accordance with accepted academic practice. No use, distribution or reproduction is permitted which does not comply with these terms.
*Correspondence: Reginaldo G. Bastos, cmVnaW5hbGRvX2Jhc3Rvc0B3c3UuZWR1; Carlos E. Suarez, Y2FybG9zLnN1YXJlekB1c2RhLmdvdg==