- 1Department of Pediatrics, BenQ Medical Center, The Affiliated BenQ Hospital of Nanjing Medical University, Nanjing, Jiangsu, China
- 2Department of Epidemiology, Harvard T.H. Chan School of Public Health, Boston, MA, United States
- 3Center for Global Health, Perelman School of Medicine, University of Pennsylvania, Philadelphia, PA, United States
- 4Department of Orthodontics and Dentofacial Orthopedics, Henry M. Goldman School of Dental Medicine, Boston University, Boston, MA, United States
- 5Department of Obstetrics & Gynecology, National Taiwan University Hospital & College of Medicine, Taipei, Taiwan
- 6Clinical Trial Research Center, China Medical University Hospital, Taichung, Taiwan
- 7Department of Environmental Health, University of Cincinnati, Cincinnati, OH, United States
- 8Department of Biostatistics and Epidemiology, Perelman School of Medicine, University of Pennsylvania, Philadelphia, PA, United States
- 9Division of Allergy, Immunology and Rheumatology, Chung Shan Medical University Hospital, Taichung, Taiwan
- 10Institute of Medicine, Chung Shan Medical University, Taichung, Taiwan
- 11Graduate Institute of Integrated Medicine, China Medical University, Taichung, Taiwan
Background: Environmental etiology of primary Sjögren’s syndrome (pSS), an autoimmune disease, has been proposed. This study determined whether the exposure to air pollutants was an independent risk factor for pSS.
Methods: Participants were enrolled from a population-based cohort registry. Daily average concentrations of air pollutants from 2000 to 2011 were divided into 4 quartiles. Adjusted hazard ratios (aHRs) of pSS for exposure to air pollutants were estimated in a Cox proportional regression model adjusting for age, sex, socioeconomic status, and residential areas. A subgroup analysis stratified by sex was conducted to validate the findings. Windows of susceptibility indicated years of exposure which contributed the most to the observed association. Ingenuity Pathway Analysis was used to identify underlying pathways of air pollutant-associated pSS pathogenesis, using Z-score visualization.
Results: Two hundred patients among 177,307 participants developed pSS, with a mean age of 53.1 years at acumulative incidence of 0.11% from 2000 to 2011. Exposure to carbon monoxide (CO), nitric oxide (NO), and methane (CH4) was associated with a higher risk of pSS. Compared to those exposed to the lowest concentration level, the aHRs for pSS were 2.04 (95%CI=1.29-3.25), 1.86 (95%CI=1.22-2.85), and 2.21 (95%CI=1.47-3.31) for those exposed to high levels of CO, NO, and CH4, respectively. The findings persisted in the subgroup analysis, in which females exposed to high levels of CO, NO, and CH4 and males exposed to high levels of CO were associated with significantly great risk of pSS. The cumulative effect of air pollution on pSS was time-dependent. The underlying cellular mechanisms involved chronic inflammatory pathways including the interleukin-6 signaling pathway.
Conclusion: Exposure to CO, NO, and CH4 was associated with a high risk of pSS, which was biologically plausible.
1 Introduction
Sjögren’s syndrome (SS) is an autoimmune disease in which the destruction of the epithelium of the exocrine glands, including the salivary glands and lacrimal glands, leads to the hallmark symptoms of sicca syndrome (1). Patients with SS may also present articular, cutaneous, pulmonary, and muscular complications, with symptoms that may plateau, worsen or go into remission (1). SS affects about 35 million people worldwide, with manifestations of significant fatigue, joint and muscle pain, impairing their quality of life, and the development of extra glandular manifestations, including non-Hodgkin’s lymphoma in about 30% to 50% of patients (2). The incidence of primary SS (pSS) is estimated to be 7 cases per 100,000 people per year in the general population with a female-to-male predominance of 9:1 (1). It is significantly higher in middle-aged women than in men as the prevalence rate is 60.82 cases per 100,000 residents with a female-male ratio of 10.72, and a mean onset age of 60.8 ± 15.2 years (3, 4). As with most autoimmune diseases, genetic, environmental, and hormonal factors are incorporated in SS pathogenesis (1). Although the pathogenic pathways underlying SS have yet been completely elucidated (5), studies have described that the etiology and pathogenesis of SS are related to genetic susceptibility to environmental factors, viral infection, vitamin D deficiency, smoking, endocrine alternations and the role of angiogenesis (6).
On the other hand, air pollution has been considered as one consequence of globalization involving industrial development, fossil fuel burning, and climate change. With the rising awareness of air pollution and environmentalism, how urban air pollution could negatively affect human health (7), as a constituent of the ecosystems in the ecotoxicological context, has been brought to the attention of healthcare professionals. Studies have provided evidence that air pollution is associated with enhanced incidence of cardiovascular and respiratory diseases (8). In terms of rheumatic inflammatory diseases, inhalation of air pollutants has been proposed to be an independent risk factor for systemic inflammatory responses, which is associated with the development and progression of autoimmune diseases, such as systemic autoimmune rheumatic diseases (SARD), juvenile idiopathic arthritis (JIA), and rheumatoid arthritis (RA) (9). However, it remains unclear whether the exposure to air pollutants is also a vital factor for the pathogenesis of SS. Therefore, the aim of the present population-based cohort study was to determine whether the exposure to air pollutants could be an environmental risk factor for pSS.
2 Materials and methods
2.1 Data source
With the National Health Insurance Research Database (NHIRD), covering 99% of the population of Taiwan, 25 million people, this population-based cohort study was conducted. The NHIRD consisted of data on reimbursement claims sourced from the National Health Insurance (NHI) program (10–12), a single-payer National Health Insurance (NHI) program which was instituted in 1995 and covered nearly 99% of Taiwan’s population by the end of 2008 (13). Outpatient visits, hospital admissions, prescriptions, procedures, and disease diagnostic records were retrieved from NHIRD (14–26).
Air pollution data including concentrations of carbon monoxide (CO), nitrogen monoxide (NO), and methane (CH4) were obtained from the Taiwan Air Quality-Monitoring Database (TAQMD). This database was released by a governmental agency, Taiwan Air Quality Monitoring Network, which collected daily ambient air pollution data from 78 community-based monitoring stations, available on a real-time basis since 1993. Pollutant data on particulate matter (PM)10 (μg/m3), PM2.5 (μg/m3), ozone (O3) (parts per million, ppm), CO (ppm), CH4 (ppm), sulfur dioxide (SO2, parts per billion, ppb), NO2 (ppb), and NO (ppb), were retrieved for their daily mean concentrations from January 1st, 2000 to December 31th, 2011. The endpoint of the follow-up period of this study was the diagnosis of pSS, or December 2011. The daily average exposed air pollutant concentrations for each participant were estimated using the Inverse Distance Weighting Method (IDW method). As one means of spatial interpolation, the IDW method was used to give the predicted values of unknown points by weighting the average of known points. In the present study, data on air pollution within two years prior to the diagnosis of pSS were accessed, with the IDW method used to estimate the concentrations of air pollutants based on values of the concentrations measured by monitoring stations surrounding the registered address of each participant, considering the distance between the monitoring station and the registered address of the participant.
2.2 Study population
Populations residing in areas with ambient air quality-monitoring stations were set as the study population. Among them, those with a history of SS before 2000 were excluded. All participants were traced from January 1st, 2000 until the endpoint of the study period. Confounding factors including age, gender, annual individual income, and urbanization levels where the participants lived, were adjusted. The urbanization level was defined following the National Health Research Institutes (NHRI) classification system, in which all residential areas were stratified into 7 urbanization levels considering their population density, resident education level, agricultural activity, and healthcare accessibility (physician number per 100,000 residents) (27). Due to the lack of participants from rural areas of levels 4-7, this study included areas of levels 1-4, with level 1 as the most urbanized and level 4 as the least urbanized. Annual incomes were classified into 4 groups: less than 5,760 USD, 5,760–7,320 USD, 7,320–8,400 USD, and over 8,400 USD.
2.3 Definition of exposure and outcome
The exposure to air pollution was defined using daily average concentrations of each air pollutant from 2000 to the endpoint. The daily average concentrations were classified into four groups using interquartile range (IQR), with three cut-off points (25th, 50th and 75th percentiles) ranging from the lowest concentration level, Quartile (Q) 1, to the highest concentration level, Q4. The American College of Rheumatology (ACR) and the European League Against Rheumatism (EULAR) Consensus Group criteria was followed to diagnose pSS as the outcome of this cohort study (28), for which the diagnosis of pSS was made based on anti-SSA (Ro) antibody positivity, focal lymphocytic sialadenitis, abnormal ocular staining, Schirmer’s tests, and unstimulated salivary flow rates (28). The diagnosis of pSS had to be confirmed in at least two outpatient visits or at least one hospitalization record within two years, all of which were peer-reviewed by a rheumatologist.
2.4 Statistical analyses
The Chi-squared test was used to determine the differences in age, sex, urbanization level, annual income, season, and the incidence of pSS. A multivariable Cox proportional hazard regression model adjusted for confounding factors was used to derive the association between the exposure to air pollutants and the risk of new-onset pSS. Incidence rates (IRs) and adjusted hazard ratios (aHRs) for new-onset pSS in participants exposed to each concentration level of air pollutants were derived. HRs for quartile levels Q2, Q3, and Q4, were compared to the reference level, Q1. All analyses were conducted with SAS version 9.4 (SAS Institute, Cary, NC, USA). A two-sided P-value less than 0.05 was considered statistically significant.
2.5 Bioinformatic analyses
Canonical Pathway Analysis (29–32) was conducted on Ingenuity Pathway Analysis (IPA) software (QIAGEN, Hilden, Germany) using differentially expressed (DE) RNA-seq data of airway epithelial cells exposed to coarse (n=3), fine (n=3), and ultrafine particulate matter (PM) (n=3) versus control group (n=3) obtained from the National Center for Biotechnology Information-Gene Expression Omnibus database (GEO; GSE7010), as well as parotid gland tissue from patients with pSS (n=3) versus healthy adults (n=3) obtained from GEO (GSE40611). Positive and negative z-scores indicated up-regulation and down-regulation of targeted pathways, respectively. Molecular Activation Prediction (MAP) was performed to model the mechanisms by which air pollutants may trigger or regulate the pathogenesis of pSS.
3 Results
177,307 residents with available data for both their exposure to air pollutants and electronic medical records were included in this study. The mean age of patients who developed pSS (N=200) was 53.1 years, with 60.5% over 50 years of age (N= 121) and 85% of patients were females (N=170). Among all included air pollutants, exposure to CO, NO, and CH4, was significantly associated with higher risks of pSS.
3.1 Baseline characteristics and the incidence of pSS in individuals exposed to CO, NO, and CH4
Participants exposed to CO in Q1 level were the oldest, with a median age of 41.1 years, and exhibited the least incidence of pSS (0.07%). Participants exposed to Q3 level of CO had a younger median age of 38.7 years and exhibited the highest incidence of pSS (0.18%) (Table 1).
Participants exposed to NO in the Q1 level were the oldest, with the median age of 41.0 years; whereas those exposed to the Q3 level were the youngest, with a median age of 37.7 years. Compared with participants exposed to Q1, Q2, and Q3 levels of NO concentration, those exposed to Q4 level exhibited the highest incidence of pSS (0.14%), with the median age of 39.5 years (Table 1). Overall, the exposure to NO was significantly associated with the incidence of pSS (Table 1).
Participants exposed to CH4 in the Q4 level were the oldest, with a median age of 41.6 years. Participants exposed to Q4 level had the highest incidence of pSS (0.24%), compared with those exposed to lower concentration levels. More participants exposed to Q4 level of CH4 lived in level 1 urbanization areas (35.0%) (Table 1).
3.2 Incidence rates and risks of pSS in individuals exposed to CO, NO, and CH4
The association between exposed levels of CO, NO, and CH4 and the incidence of pSS was dose-dependent. Subjects exposed to high concentrations (Q3 and Q4 levels) of CO, NO, and CH4, presented with significantly higher IRs of pSS, compared to those exposed to Q1 level of these air pollutants (Table 2). Likewise, after adjusting for demographics including age, sex, socioeconomic status, and urbanization levels of residing areas, the risk of pSS in those exposed to Q3 or Q4 levels were significantly higher than that in those exposed to to the Q1 level of each air pollutant (Table 2 and Figure 1). In particular, the aHR was 3.05 (95% CI = 1.96–4.72) for individuals exposed to Q3 level of CO, 1.88 (95% CI = 1.21-2.92) for those exposed to Q3 level of NO, and 2.21 (95% CI = 1.47-3.31) for participants exposed to Q4 level of CH4 (Table 2 and Figure 1).
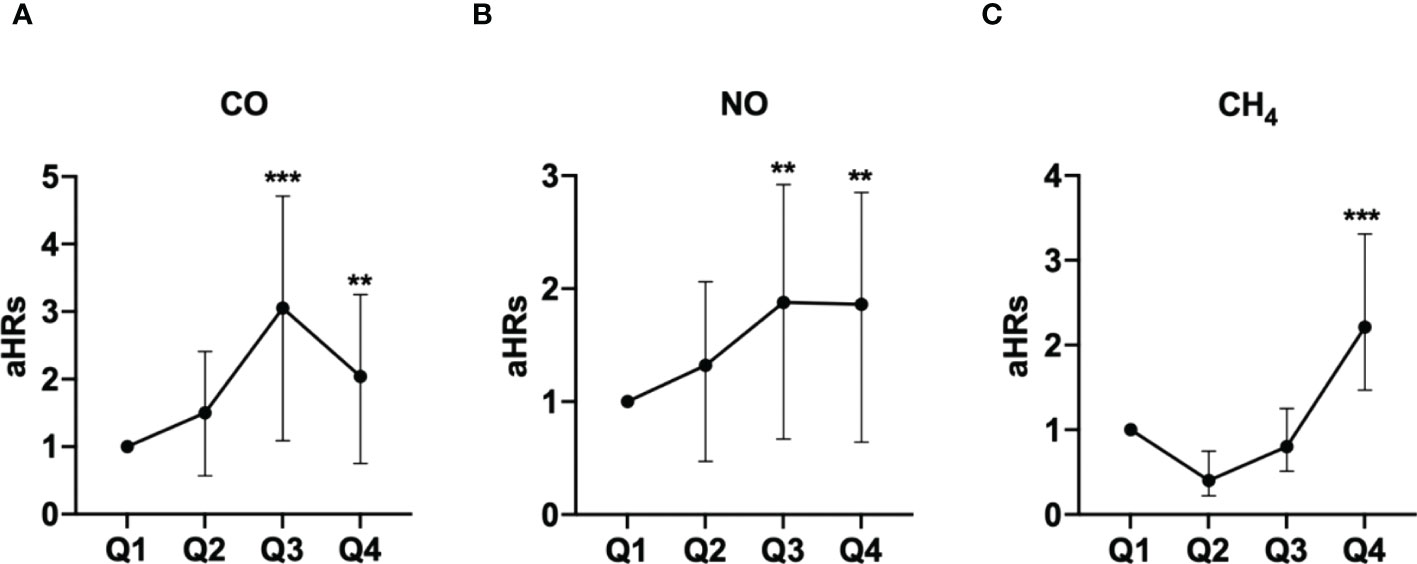
Figure 1 Illustrated hazard ratios (HRs) of pSS in individuals exposed to carbon monoxide (CO) (A), nitric oxide (NO) (B), and methane (CH4) (C). **p<0.01; ***p<0.001.
These findings persisted in a subgroup analysis stratified by sex, in which the risks of pSS among females exposed to Q3 (aHR =2.83, 95% CI = 1.77-4.52) and Q4 (aHR =1.84, 95% CI = 1.12-3.02) levels of CO, Q3 (aHR =1.64, 95% CI = 1.02-2.65) and Q4 (aHR =1.79, 95% CI = 1.13-2.81) levels of NO, and Q4 level of CH4 (aHR =2.34, 95% CI = 1.52-3.62), were significantly greater than females exposed to Q1 levels of respective air pollutants; parallelly, males exposed to Q3 (aHR =4.97, 95% CI = 1.37-18.1) and Q4 (aHR =3.99, 95% CI = 1.05-15.1) levels of CO also had significantly higher risks of pSS, compared to males exposed to Q1 level of CO (Table 3 and Figure 2).
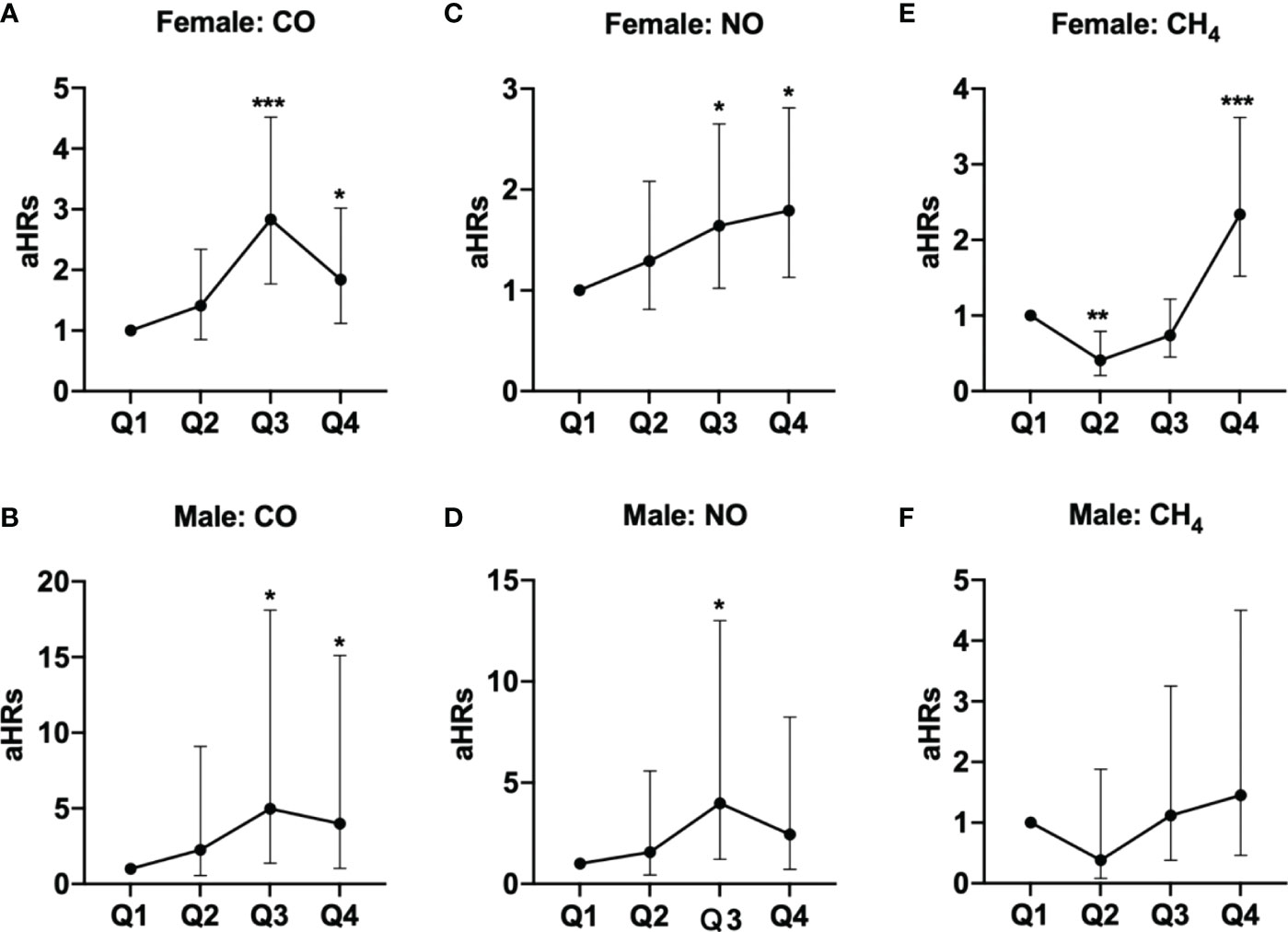
Figure 2 Illustrated hazard ratios (HRs) of pSS in females and males exposed to (A, female; B, male) CO, (C, female; D, male) NO, and (E, female; F, male) CH4. *p<0.05; ***p<0.001.
Overall, the dose-dependent effect of CO, NO, and CH4 on the risk of new-onset pSS was observed; on the other hand, the risks of pSS following the exposure to PM10, PM2.5, and NOx, were not consistently increased in individuals exposed to all high concentrations (Supplementary Figure 1 and Supplementary Table 1). Collectively, these findings, suggested an elevated risk of pSS subsequent to the exposure to air pollutants that where small molecules, but not air pollutants of large sizes. Moreover, windows of susceptibility indicated years of exposure that contributed the most to the observed association, in which years between 2007 and 2011 were of high incidence of pSS (9.50%, 16.00%, and 13.00% of all incident cases of pSS happened in 2007, 2009, and 2011, respectively) (Figure 3). This suggested the time-dependent cumulative effect of the exposure to air pollutants on pSS.
3.3 Modeled mechanisms of air pollutant-triggered pSS
Canonical pathway analyses indicated multiple air pollutant-associated inflammatory pathways that may trigger pSS, for which their degrees of upregulation were associated with particle sizes, with smaller sizes of air pollutants exacerbating larger extents of inflammation (Figure 4). Among pathways expressed in airway epithelial cells exposed to coarse, fine and ultrafine PM, and in parotid glands of patients with pSS, the highly expressed inflammatory pathways included (1): interleukin (IL)-6 signaling, with Z-scores being 3.272 for pSS, 2.714 for exposure to ultrafine PM, 2.121 for exposure to fine PM, and 1.663 for exposure to coarse PM, (2) Toll-like receptor signaling, with Z-scores being 1.633 for pSS, 2.449 for exposure to ultrafine PM, 2 for exposure to fine PM, and 1.342 for exposure to coarse PM, (3) acute phase response signaling, with Z-scores being 2.921 for pSS, 2.111 for exposure to ultrafine PM, 1.414 for exposure to fine PM, and 0.816 for exposure to coarse PM, (4) adrenomedullin signaling, with Z-scores being 2.401 for pSS, 1.89 for exposure to ultrafine PM, 1.633 for exposure to fine PM, and 1.342 for exposure to coarse PM, (5) NF-κB signaling, with Z-scores being 2.502 for pSS, 1.633 for exposure to ultrafine PM, 0.816 for exposure to fine PM, and 0.707 for exposure to coarse PM, (6) neuroinflammation signaling, with Z-scores being 4.899 for pSS, 1.508 for exposure to ultrafine PM, 0.707 for exposure to fine PM, and -0.378 for exposure to coarse PM, (7) B cell signaling pathway with Z-scores being 4.737 for pSS, 1.508 for exposure to ultrafine PM, 0.707 for exposure to fine PM, and 1.633 for exposure to coarse PM, and (8) osteoarthritis pathway with Z-scores being 3.157 for pSS, 1.387 for exposure to ultrafine PM, 1.387 for exposure to fine PM, and 0.333 for exposure to coarse PM.
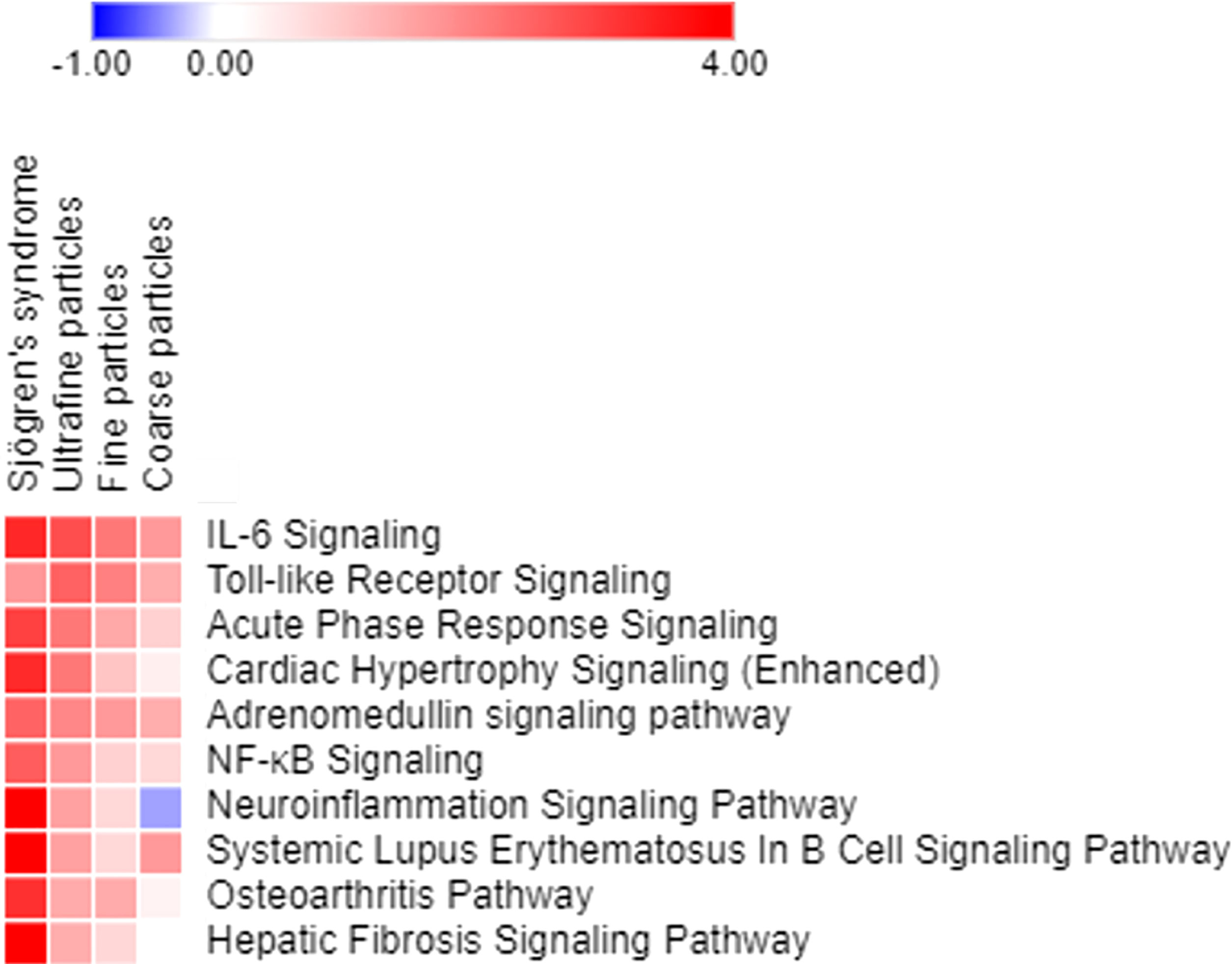
Figure 4 Pathways expressed in parotid glands of patients with pSS and primary airway epithelial cells exposed to ultrafine, fine, and coarse PM, ordered based on the sum of z scores.
To further decipher the network that addressed new-onset pSS following the exposure to air pollutants, findings of the MAP analysis (33, 34) supported that IL-6 signaling was activated in both the pathogenesis of pSS (Figure 5A) and the exposure to ultrafine (Figure 5B) or fine PM (Supplementary Figure 1A), in which transcription factor NF-IL6 was activated and subsequently induced the production of multiple inflammatory cytokines, including IL-6 and IL-8, while STAT3 was phosphorylated to transport downstream signaling and the production of acute-phase proteins, such as C-reactive protein (CRP). To the contrary, exposure to coarse PM only activated NF-IL6- but not STAT3-mediated signaling (Supplementary Figure 1B).
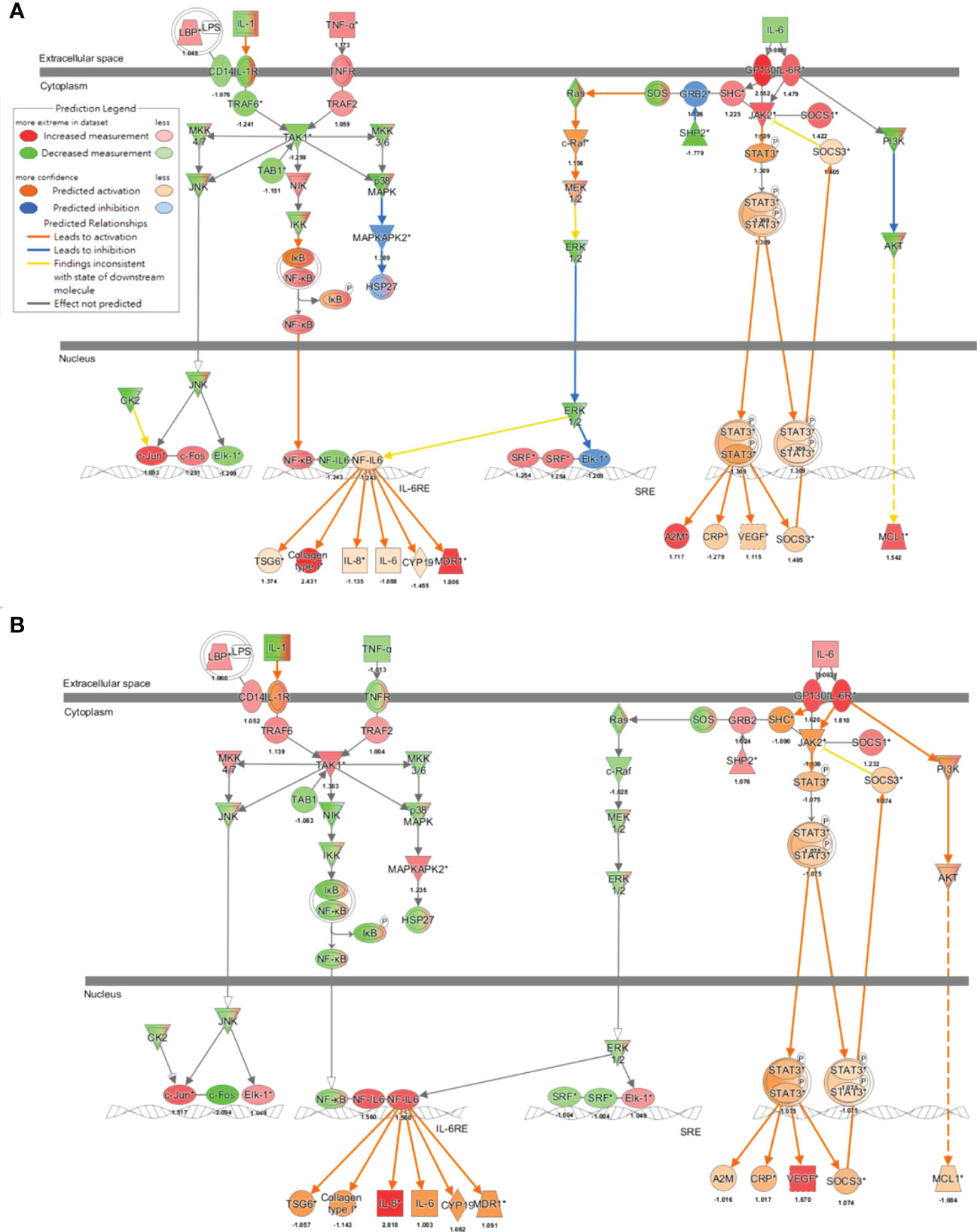
Figure 5 Air pollutant-associated IL-6 signaling pathway underlying the pathogenesis of pSS. Molecular Activation Prediction through transcriptomic data from parotid glands of patients with pSS (A) and airway epithelial cells exposed to ultrafine PM (B), indicated mechanisms involved in IL-6 signaling.
Overall, these inflammatory pathways, especially the IL-6 signaling, were upregulated more in response to the exposure to ultrafine particles than that to the exposure to coarse particles (Figure 4), which was in line with significant findings in the cohort study on small gaseous molecules.
4 Discussion
In this population-based cohort study, an independent correlation between exposure to small molecule air pollutants and new-onset pSS was observed. Individuals exposed to great concentrations of CH4, NO, CO, presented with significantly great risks of SS. After adjusting for age, sex, annual income, and urbanization levels of the areas where the participants lived, the association was still notable, for which our findings were not dominated by population density or socioeconomic status. The upregulated pathways in response to the exposure to air pollutants were proinflammatory, and their degrees of upregulation were related to PM sizes. Moreover, in accordance with the above-mentioned findings that small-molecule air pollutants, instead of PM10 or PM2.5, were associated with increased risks of pSS, the inflammatory pathways were most highly expressed in samples exposed to ultrafine particles, followed by those exposed to fine particles, then those exposed to coarse particles; these scale-dependent findings support the clinical relevance of findings in the present cohort study on significantly great risk of pSS following exposure to small molecule air pollutants (35).
Although previous studies suggested that socioeconomic status may affect the occurrence and development of autoimmune diseases (36), in the present study, the effect of air pollution on pSS was independent of socioeconomic status and where the participants lived. Moreover, results of canonical pathway analyses in the present study illustrated that such an association can be based on significantly triggered inflammatory pathways including the IL-6 signaling pathway that were highly expressed in airway epithelial cells and salivary glands. These findings were similar to previous studies suggesting that inhalation of air pollutants may directly affect the lungs, causing acute and chronic inflammation in the respiratory system (37), and may be associated with non-pulmonary diseases, such as type 2 diabetes, cancer, neurodegenerative diseases, and autoimmune rheumatoid diseases (38, 39). As such, together with previous studies (38, 39), findings of the present study allowed for the conjecture that air pollutants may not only directly induce local pulmonary inflammation but also trigger systemic chronic inflammatory responses, which was to the mechanisms by which air pollutants trigger cardiovascular diseases (8).
As to whether the exposure to air particulates could exacerbate or trigger SS, few studies have addressed the impact of airborne pollutants on SS. Most of these studies were either cross-sectional or animal studies. For instance, one animal study demonstrated that inhaled residual oil fly ash (ROFA) exacerbated lung response in SS mice models, mimicking the harmful effects of airborne pollution on the airway of patients with SS (40). Another cross-sectional study indicated that ocular abnormalities and eye irritation in patients with SS exposed to nitrogen dioxide were significantly more severe than control patients (41). These studies suggested a possible effect of air pollution on SS patients, yet did not provide evidence for whether the exposure to air pollutants was an independent risk factors for pSS, due to the lack of longitudinal and temporal data.
The respiratory immune system as the first-line defense against inhaled agents, when dysregulated by microorganisms, allergens or pollutants, has been known to trigger host immune responses (42) and autoantibody induction (43) that may initiate pSS (44). When these pollutants are transferred from lungs to blood, the onset of systemic inflammatory responses usually involves B cell stimulation, in which autoantibodies could be generated and lead to autoimmunity (38). In the present study, ambient exposure to severe air pollution involving CO, NO, and CH4 was associated with the risk of pSS, with previous studies suggesting that these three pollutants can trigger the production of excessive inflammatory mediators that may perturb immunity and cause pulmonary toxicity (45, 46). Juxtaposing findings in previous studies and the modeled air pollution-dependent pathogenesis of pSS as demonstrated in the present study, it is recognized that IL-6 plays a role in initiating Th17/Treg imbalance that may contribute to autoimmune responses and chronic inflammation, through driving Th17 immunity and inhibiting the peripheral generation of Foxp3+ regulatory T cells (47); furthermore, these IL-6-dependent autoimmune responses may be triggered by the exposure to air pollutants (34) and have been reported to be associated with the severity of pSS (48), providing the biological plausibility of findings in the present study. Specifically, epidemiological findings in the present study on significantly high risk of pSS following the exposure to air pollutants of smaller molecular sizes, such as CO, NO, and CH4, instead of PM10 and PM2.5, were in line with bioinformatic trends that as well indicated the biological effect of these pollutants on the triggered inflammatory pathways in airway epithelium, monocytes (49), and endothelium (50), which demonstrated a link between exposure to air pollutants and subsequent autoimmune responses.
Among all pSS-associated air pollutants that were identified in the present study, the findings on the correlation between pSS and the exposure to NO, echoed with studies suggesting that an increment of NO levels in the human body may disturb immune response, impair organelle function of alveolar macrophages, and elevate cellular levels of IFN-γ and MIP-1α, which can result in disrupted pulmonary immune homeostasis in the alveoli (51). As such, it is also worthwhile to uncover the mechanisms through which NO-mediated damage of alveolar macrophages may contribute to autoimmune pathogenesis underlying pSS, for which studies have suggested that epigenetic events in the immune cells can trigger pSS (6, 45), and that DNA methylation can be induced by exposure to particulate matter (52).
Furthermore, findings in the present study on the great risk of pSS in individuals exposed to high concentrations of air pollutants, were in line with studies implying the impact of environmental pollutants on dry eye disease in patients with SS and in SS models (53), for which increased levels of TNF-α and NF-κB in the cornea were observed (53, 54). Such an ocular involvement was followed by induced apoptosis in corneal superficial/basal epithelium that led to abnormal differentiation and proliferation of the ocular surface plus a reduced number of goblet cells in the conjunctival fornix (53), which was similar to our findings on air pollution-associated NF-κB upregulation. Additionally, since lung involvement as one of the major systemic complications of pSS (55) has been suggested to manifest as low transfer factors for carbon monoxide (55), our findings indicated that apart from dry eye (56–58) and dry mouth symptoms, the consecutive exposure to air pollutants may exacerbate pSS-related lung pathologies including chronic obstructive pulmonary disease and interstitial lung disease (55).
The strengths of the present cohort study included a large sample size with long-term follow-up, and the reliability of pSS as an outcome, as the diagnosis of pSS in this study was validated by rheumatologists, rather than questionnaires filled in by patients. Results of this study supported the hypothesis that individuals exposed to small molecule air pollutants, as opposed to PM10 or PM2.5, presented with an elevated risks of SS. The association between exposure to air pollutants and subsequent pSS may involve several chronic inflammatory pathways (59) with existing studies suggesting their relevance to autoimmune diseases such as the B cell signaling pathway (38). The findings on small molecule air pollutant-specific risk of pSS, were in accordance with scale-dependent expression levels of the above-mentioned chronic inflammatory pathways.
Although in the present study, people with previous history of pSS prior to the enrollment were excluded, there could be potential misclassification that resulted in cases who had undiagnosed pSS at baseline that were incorrectly found to develop pSS after the exposure to air pollutants. Moreover, as the exposure to air pollutants has been suggested to be associated with the risk of heart failure, stroke and myocardial infarction (60), it warrants further studies to investigate whether air pollution-associated pSS may be an early sign or concomitant complication of air pollution-associated heart diseases that could lead to hospitalization. Other limitations of this study included the lack of detailed information from electronic medical records involving lab data that may reflect the severity of SS, such as the raw data of SS disease activity index (ESSDAI) (1, 28). In addition, as the community-based monitoring stations of TAQMD may not detect indoor air quality, studies that collect data on indoor air pollutants are required to estimate the effect of indoor air quality; for instance, since NO can result from gas burning and barbecue or cooking (61), further studies that acquire data from NO sensors may validate findings in the present study. All in all, prospective cohort studies with more detailed information are needed to ascertain the observed dose-dependent effect of air pollutants on pSS and other autoimmune diseases, and to provoke patient education on environmental risk factors for pSS (62).
5 Conclusions
In conclusion, the exposure to small molecule air pollutants, especially CO, NO, and CH4, was an independent risk factor for pSS. Clinical and policy implications of this study include insights to environmental toxicology in a global health context, with more focus on ways to promote public awareness about the importance of environmental health to rheumatic and autoimmune diseases (13).
Data availability statement
The datasets presented in this study can be found in online repositories. The names of the repository/repositories and accession number(s) can be found in the article/Supplementary Material.
Author contributions
Conceptualization, KM, L-TW & WC; Methodology, KM, L-TW & C-LL; Writing – Original Draft, KM, HL & L-TW; Writing – Review & Editing, AC & JW; Funding Acquisition, KM, L-TW & WC. All authors contributed to the article and approved the submitted version.
Funding
This work was supported in part by funding from the Taiwan Ministry of Science & Technology (MOST: 108-2813-C-040-040-B to KM & 109-2326-B-002 -016-MY3 to L-TW), and a research grant from International Team for Implantology (ITI) (fund no.1577_2021 to KM).
Conflict of interest
The authors declare that the research was conducted in the absence of any commercial or financial relationships that could be construed as a potential conflict of interest.
Publisher’s note
All claims expressed in this article are solely those of the authors and do not necessarily represent those of their affiliated organizations, or those of the publisher, the editors and the reviewers. Any product that may be evaluated in this article, or claim that may be made by its manufacturer, is not guaranteed or endorsed by the publisher.
Supplementary material
The Supplementary Material for this article can be found online at: https://www.frontiersin.org/articles/10.3389/fimmu.2022.1044462/full#supplementary-material
References
1. Brito-Zeron P, Baldini C, Bootsma H, Bowman SJ, Jonsson R, Mariette X, et al. Sjogren syndrome. Nat Rev Dis Primers (2016) 2:16047. doi: 10.1038/nrdp.2016.47
2. Odani T, Chiorini JA. Targeting primary sjogren’s syndrome. Mod Rheumatol (2019) 29(1):70–86. doi: 10.1080/14397595.2018.1546268
3. Tsuboi H, Asashima H, Takai C, Hagiwara S, Hagiya C, Yokosawa M, et al. Primary and secondary surveys on epidemiology of sjogren’s syndrome in Japan. Mod Rheumatol (2014) 24(3):464–70. doi: 10.3109/14397595.2013.843765
4. Qin B, Wang J, Yang Z, Yang M, Ma N, Huang F, et al. Epidemiology of primary sjogren’s syndrome: A systematic review and meta-analysis. Ann Rheum Dis (2015) 74(11):1983–9. doi: 10.1136/annrheumdis-2014-205375
5. Chen HH, Ma KS, Dong C, Chang WJ, Gao KR, Perng WT, et al. Risk of primary sjogren's syndrome following human papillomavirus infections: A nationwide population-based cohort study. Front Immunol (2022) 13:967040. doi: 10.3389/fimmu.2022.967040
6. Pontarini E, Lucchesi D, Bombardieri M. Current views on the pathogenesis of sjogren’s syndrome. Curr Opin Rheumatol (2018) 30(2):215–21. doi: 10.1097/BOR.0000000000000473
7. Lee YY, Wu CC, Lee YJ, Chiang CH, Ma KS, Wei JC. Lung cancer attributable to environmental risk factors. Am J Respir Crit Care Med (2022). doi: 10.1164/rccm.202208-1618LE
8. Zhang JJ, Adcock IM, Bai Z, Chung KF, Duan X, Fang Z, et al. Health effects of air pollution: What we need to know and to do in the next decade. J Thorac Dis (2019) 11(4):1727–30. doi: 10.21037/jtd.2019.03.65
9. Sun G, Hazlewood G, Bernatsky S, Kaplan GG, Eksteen B, Barnabe C. Association between air pollution and the development of rheumatic disease: A systematic review. Int J Rheumatol (2016) 2016:5356307. doi: 10.1155/2016/5356307
10. Ma KS. Integrating travel history via big data analytics under universal healthcare framework for disease control and prevention in the COVID-19 pandemic. J Clin Epidemiol. (2021) 130:147–8. doi: 10.1016/j.jclinepi.2020.08.016
11. Ma KS, Tsai SY. Big data-driven personal protective equipment stockpiling framework under universal healthcare for disease control and prevention in the COVID-19 era. Int J Surg (2020) 79:290–1. doi: 10.1016/j.ijsu.2020.05.091
12. Ma KS. Screening programs incorporating big data analytics. In: Keikhosrokiani P, editor. Big data analytics for healthcare: Datasets, techniques, life cycles, management, and applications. Elsevier: Academic Press. p. 313–27. doi: 10.1016/B978-0-323-91907-4.00023-6
13. Huang JY, Ma KS, Wang LT, Chiang CH, Yang SF, Wang CH, et al. The risk of endometrial cancer and uterine sarcoma following endometriosis or pelvic inflammatory disease. JCancers (Basel). (2023) 15(3):833. doi: 10.3390/cancers15030833
14. Ma KS, Hasturk H, Carreras I, Dedeoglu A, Veeravalli JJ, Huang JY, et al. Dementia and the risk of periodontitis: A population-based cohort study. J Dent Res (2022) 101(3):270–7. doi: 10.1177/00220345211037220
15. Ma KS, Wu MC, Thota E, Wang YH, Alqaderi HE, Wei JC. Tonsillectomy as a risk factor of periodontitis: A population-based cohort study. J Periodontol (2022) 93(5):721–31. doi: 10.1002/JPER.21-0215
16. Wu MC, Ma KS, Chen HH, Huang JY, Wei JC. Relationship between helicobacter pylori infection and psoriasis: A nationwide population-based longitudinal cohort study. Med (Baltimore). (2020) 99(24):e20632. doi: 10.1097/MD.0000000000020632
17. Perng WT, Ma KS, Hung HY, Tsai YC, Huang JY, Liao PL, et al. Dental caries and risk of newly-onset systemic lupus erythematosus: A nationwide population-based cohort study. Curr Med Res Opin (2022) 19:1–13. doi: 10.1080/03007995.2022.2159146
18. Huang HH, Ma KS, Wu MY, Hung YM, Tsao CH, Wei JC, et al. Patients with obstructive sleep apnea are at great risk of flavor disorders: A 15-year population-based cohort study. Clin Oral Investig (2022). doi: 10.1007/s00784-022-04707-3
19. Juang SE, Ma KS, Kao PE, Wei JC, Yip HT, Chou MC, et al. Human papillomavirus infection and the risk of erectile dysfunction: A nationwide population-based matched cohort study. J Pers Med (2022) 12(5):699. doi: 10.3390/jpm12050699
20. Ma KS, Lee CM, Chen PH, Yang Y, Dong YW, Wang YH, et al. Risk of autoimmune diseases following optic neuritis: A nationwide population-based cohort study. Front Med (Lausanne). (2022) 9:903608. doi: 10.3389/fmed.2022.903608
21. Ma KS, Lai JN, Thota E, Yip HT, Chin NC, Wei JC, et al. Bidirectional relationship between osteoarthritis and periodontitis: A population-based cohort study over a 15-year follow-up. Front Immunol (2022) 13:909783. doi: 10.3389/fimmu.2022.909783
22. Wu MC, Ma KS, Wang YH, Wei JC. Impact of tonsillectomy on irritable bowel syndrome: A nationwide population-based cohort study. PloS One (2020) 15(9):e0238242. doi: 10.1371/journal.pone.0238242
23. Ma KS, Lai JN, Veeravalli JJ, Chiu LT, Van Dyke TE, Wei JC. Fibromyalgia and periodontitis: Bidirectional associations in population-based 15-year retrospective cohorts. J Periodontol (2022) 93(6):877–87. doi: 10.1002/JPER.21-0256
24. Ma KS, Thota E, Huang JY, Huang YF, Wei JC. Onset of oral lichen planus following dental treatments: A nested case-control study. Oral Dis (2021). doi: 10.1111/odi.14115
25. Ma KS, Illescas Ralda MM, Veeravalli JJ, Wang LT, Thota E, Huang JY, et al. Patients with juvenile idiopathic arthritis are at increased risk for obstructive sleep apnoea: a population-based cohort study. Eur J Orthod (2022) 44(2):226–31. doi: 10.1093/ejo/cjab050
26. Ma KS, Thota E, Huang JY, Wei JC, Resnick CM. Increased risk of temporomandibular joint disorders and craniofacial deformities in patients with juvenile idiopathic arthritis: A population-based cohort study. Int J Oral Maxillofac Surg (2022) 51(11):1482–7. doi: 10.1016/j.ijom.2022.04.007
27. Liu CY, Hung YT, Chuang YL, Chen YJ, Weng WS, Liu JS. Incorporating development stratification of Taiwan townships into sampling design of large scale health interview survey. J Health Manag (2006) 14:1–22.
28. Shiboski CH, Shiboski SC, Seror R, Criswell LA, Labetoulle M, Lietman TM, et al. 2016 American College of Rheumatology/European league against rheumatism classification criteria for primary sjogren’s syndrome: A consensus and data-driven methodology involving three international patient cohorts. Arthritis Rheumatol (2017) 69(1):35–45. doi: 10.1002/art.39859
29. Thota E, Veeravalli JJ, Manchala SK, Lakkepuram BP, Kodapaneni J, Chen YW, et al. Age-dependent oral manifestations of neurofibromatosis type 1: A case-control study. Orphanet J Rare Dis (2022) 17(1):93. doi: 10.1186/s13023-022-02223-x
30. Wang LT, Ma KS. Correspondence to 'Normal human enthesis harbours conventional CD4+ and CD8+ t cells with regulatory features and inducible IL-17A and TNF expression'. Ann Rheum Dis (2022) 81(12):e254. doi: 10.1136/annrheumdis-2020-218995
31. Ma KS, Chiang CH, Chen YW, Wang LT. Correspondence to 'Bacterial citrullinated epitopes generated by porphyromonas gingivalis infection-a missing link for ACPA production'. Ann Rheum Dis (2021) 12:annrheumdis–2020-219255. doi: 10.1136/annrheumdis-2020-219255
32. Huang JW, Kuo CL, Wang LT, Ma KS, Huang WY, Liu FC, et al. Case report: In situ vaccination by autologous CD16+ dendritic cells and anti-PD-L 1 antibody synergized with radiotherapy to boost t cells-mediated antitumor efficacy in a psoriatic patient with cutaneous squamous cell carcinoma. Front Immunol (2021) 12:752563. doi: 10.3389/fimmu.2021.752563
33. Chiarella SE, Soberanes S, Urich D, Morales-Nebreda L, Nigdelioglu R, Green D, et al. beta(2)-adrenergic agonists augment air pollution-induced IL-6 release and thrombosis. J Clin Invest (2014) 124(7):2935–46. doi: 10.1172/JCI75157
34. Zhu H, Wu Y, Kuang X, Liu H, Guo Z, Qian J, et al. Effect of PM2.5 exposure on circulating fibrinogen and IL-6 levels: A systematic review and meta-analysis. Chemosphere (2021) 271:129565. doi: 10.1016/j.chemosphere.2021.129565
35. Becker S, Mundandhara S, Devlin RB, Madden M. Regulation of cytokine production in human alveolar macrophages and airway epithelial cells in response to ambient air pollution particles: Further mechanistic studies. Toxicol Appl Pharmacol (2005) 207(2 Suppl):269–75. doi: 10.1016/j.taap.2005.01.023
36. Calixto OJ, Anaya JM. Socioeconomic status. relationship Health autoimmune diseases Autoimmun Rev (2014) 13(6):641–54. doi: 10.1016/j.autrev.2013.12.002
37. Kunzli N, Kaiser R, Medina S, Studnicka M, Chanel O, Filliger P, et al. Public-health impact of outdoor and traffic-related air pollution: A European assessment. Lancet (2000) 356(9232):795–801. doi: 10.1016/S0140-6736(00)02653-2
38. Farhat SC, Silva CA, Orione MA, Campos LM, Sallum AM, Braga AL. Air pollution in autoimmune rheumatic diseases: A review. Autoimmun Rev (2011) 11(1):14–21. doi: 10.1016/j.autrev.2011.06.008
39. Chen CY, Hung HJ, Chang KH, Hsu CY, Muo CH, Tsai CH, et al. Long-term exposure to air pollution and the incidence of parkinson’s disease: A nested case-control study. PloS One (2017) 12(8):e0182834. doi: 10.1371/journal.pone.0182834
40. Ferraro S, Orona N, Villalon L, Saldiva PH, Tasat DR, Berra A. Air particulate matter exacerbates lung response on sjogren’s syndrome animals. Exp Toxicol Pathol (2015) 67(2):125–31. doi: 10.1016/j.etp.2014.10.007
41. Galperin G, Berra M, Marquez MI, Mandaradoni M, Tau J, Berra A. Impact of environmental pollution on the ocular surface of sjogren’s syndrome patients. Arq Bras Oftalmol (2018) 81(6):481–9. doi: 10.5935/0004-2749.20180091
42. Hussell T, Bell TJ. Alveolar macrophages: Plasticity in a tissue-specific context. Nat Rev Immunol (2014) 14(2):81–93. doi: 10.1038/nri3600
43. Feghali-Bostwick CA, Gadgil AS, Otterbein LE, Pilewski JM, Stoner MW, Csizmadia E, et al. Autoantibodies in patients with chronic obstructive pulmonary disease. Am J Respir Crit Care Med (2008) 177(2):156–63. doi: 10.1164/rccm.200701-014OC
44. Nikolov NP, Illei GG. Pathogenesis of sjogren’s syndrome. Curr Opin Rheumatol (2009) 21(5):465–70. doi: 10.1097/BOR.0b013e32832eba21
45. Choi YK, Por ED, Kwon YG, Kim YM. Regulation of ROS production and vascular function by carbon monoxide. Oxid Med Cell Longev (2012) 2012:794237. doi: 10.1155/2012/794237
46. Bai F, Ni B, Liu M, Feng Z, Xiong Q, Xiao S, et al. Mycoplasma hyopneumoniae-derived lipid-associated membrane proteins induce apoptosis in porcine alveolar macrophage via increasing nitric oxide production, oxidative stress, and caspase-3 activation. Vet Immunol Immunopathol (2013) 155(3):155–61. doi: 10.1016/j.vetimm.2013.07.004
47. Korn T, Mitsdoerffer M, Croxford AL, Awasthi A, Dardalhon VA, Galileos G, et al. IL-6 controls Th17 immunity in vivo by inhibiting the conversion of conventional T cells into Foxp3+ regulatory T cells. Proc Natl Acad Sci U.S.A. (2008) 105(47):18460–5. doi: 10.1073/pnas.0809850105
48. Chen C, Liang Y, Zhang Z, Zhang Z, Yang Z. Relationships between increased circulating YKL-40, IL-6 and TNF-alpha levels and phenotypes and disease activity of primary sjogren’s syndrome. Int Immunopharmacol (2020) 88:106878. doi: 10.1016/j.intimp.2020.106878
49. Sahu D, Kannan GM, Vijayaraghavan R. Carbon black particle exhibits size dependent toxicity in human monocytes. Int J Inflam (2014) 2014:827019. doi: 10.1155/2014/827019
50. Cheng WJ, Rong Y, Shi TM, Zhou T, Liu YW, Chen WH. Size-dependent biological effects on vascular endothelial cells induced by different particulate matters. J Huazhong Univ Sci Technol Med Sci (2014) 34(3):314–21. doi: 10.1007/s11596-014-1276-2
51. Park EJ, Lee HS, Lee SJ, Park YJ, Park SI, Chang J, et al. Cigarette smoke condensate may disturb immune function with apoptotic cell death by impairing function of organelles in alveolar macrophages. Toxicol In Vitro (2018) 52:351–64. doi: 10.1016/j.tiv.2018.07.014
52. Gondalia R, Baldassari A, Holliday KM, Justice AE, Mendez-Giraldez R, Stewart JD, et al. Methylome-wide association study provides evidence of particulate matter air pollution-associated DNA methylation. Environ Int (2019) 132:104723. doi: 10.1016/j.envint.2019.03.071
53. Tan G, Li J, Yang Q, Wu A, Qu DY, Wang Y, et al. Air pollutant particulate matter 2.5 induces dry eye syndrome in mice. Sci Rep (2018) 8(1):17828. doi: 10.1038/s41598-018-36181-x
54. Yu D, Deng Q, Wang J, Chang X, Wang S, Yang R, et al. Air pollutants are associated with dry eye disease in urban ophthalmic outpatients: A prevalence study in China. J Transl Med (2019) 17(1):46. doi: 10.1186/s12967-019-1794-6
55. Kelly C, Gardiner P, Pal B, Griffiths I. Lung function in primary sjogren’s syndrome: a cross sectional and longitudinal study. Thorax (1991) 46(3):180–3. doi: 10.1136/thx.46.3.180
56. Ma DH, Hsueh YJ, Ma KS, Tsai YJ, Huang SF, Chen HC, et al. Long-term survival of cultivated oral mucosal epithelial cells in human cornea: Generating cell sheets using an animal product-free culture protocol. Stem Cell Res Ther (2021) 12(1):524. doi: 10.1186/s13287-021-02564-7
57. Ma KS, Saeed HN, Chodosh J, Wang CW, Chung YC, Wei LC, et al. Ocular manifestations of anti-neoplastic immune checkpoint inhibitor-associated stevens-johnson syndrome/toxic epidermal necrolysis in cancer patients. Ocul Surf. (2021) 22:47–50. doi: 10.1016/j.jtos.2021.06.010
58. Ma KS, Chung WH, Hsueh YJ, Chen SY, Tokunaga K, Kinoshita S, et al. Human leucocyte antigen association of patients with stevens-johnson syndrome/toxic epidermal necrolysis with severe ocular complications in han chinese. Br J Ophthalmol (2022) 106(5):610–5. doi: 10.1136/bjophthalmol-2020-317105
59. Wu KJ, Tu CC, Hu JX, Chu PH, Ma KS, Chiu HY, et al. Severity of periodontitis and salivary interleukin-1β are associated with psoriasis involvement. J Formos Med Assoc (2022) 121(10):1908–16. doi: 10.1016/j.jfma.2022.01.017
60. Al-Kindi SG, Brook RD, Biswal S, Rajagopalan S. Environmental determinants of cardiovascular disease: Lessons learned from air pollution. Nat Rev Cardiol (2020) 17(10):656–72. doi: 10.1038/s41569-020-0371-2
61. Alberts WM. Indoor air pollution: NO, NO2, CO, and CO2. J Allergy Clin Immunol (1994) 94(2 Pt 2):289–95. doi: 10.1053/ai.1994.v94.a56007
Keywords: air pollution, carbon monoxide, nitric oxide, methane, interleukin-6, Sjögren’s syndrome
Citation: Ma KS-K, Wang L-T, Chong W, Lin C-L, Li H, Chen A and Wei JC-C (2023) Exposure to environmental air pollutants as a risk factor for primary Sjögren’s syndrome. Front. Immunol. 13:1044462. doi: 10.3389/fimmu.2022.1044462
Received: 14 September 2022; Accepted: 14 December 2022;
Published: 14 February 2023.
Edited by:
Hai-Feng Pan, Anhui Medical University, ChinaReviewed by:
Xiao-Mei Li, The First Affiliated Hospital of University of Science and Technology of China (USTC), ChinaXiaofei Shi, The First Affiliated Hospital of Henan University of Science and Technology, China
Copyright © 2023 Ma, Wang, Chong, Lin, Li, Chen and Wei. This is an open-access article distributed under the terms of the Creative Commons Attribution License (CC BY). The use, distribution or reproduction in other forums is permitted, provided the original author(s) and the copyright owner(s) are credited and that the original publication in this journal is cited, in accordance with accepted academic practice. No use, distribution or reproduction is permitted which does not comply with these terms.
*Correspondence: Aimin Chen, QWltaW4uQ2hlbkBwZW5ubWVkaWNpbmUudXBlbm4uZWR1; James Cheng-Chung Wei, amNjd2VpQGdtYWlsLmNvbQ==
†These authors have contributed equally to this work