- 1Department of Parasitology, College of Veterinary Medicine, Sichuan Agricultural University, Chengdu, China
- 2Department of Chemistry, College of Life and Basic Science, Sichuan Agricultural University, Chengdu, China
One of the most common rabbits coccidia species, Eimeria magna is mainly parasitic in the ileal and jejunal epithelial cells. E. magna infection can affect the growth performance of rabbits or cause other secondary diseases. Traditional methods of anticoccidial treatment typically result in drug resistance and drug residue. Therefore, vaccination is a promising alternative. Gametocyte antigen 56 (GAM56) and rhoptry kinase family proteins (ROPs) are involved in oocyst wall formation and parasite invasion, respectively. A virulence factor, ROP17 contains a serine/threonine kinase catalytic domain. In this study, recombinant E. magna GAM56 (rEmGAM56) and ROP17 (rEmROP17) proteins were obtained from a prokaryotic expression system and their reactogenicity was investigated with immunoblotting. To assess the potential of rEmGAM56 and rEmROP17 as coccidiosis vaccines, New Zealand White rabbits were subcutaneously immunized with 100 μg rEmGAM56 (rGC group) or rEmROP17 (rRC group) twice at 2-week intervals followed by homologous oocyst challenge. The rabbit serum was collected weekly to detect the specific antibody levels. The cytokine levels of pre-challenge serum were measured by enzyme-linked immunosorbent assay and the rabbits were observed and recorded post-challenge for the onset of clinical symptoms. The weight gain, oocyst output, and feed conversion ratio were calculated at the end of the experiment. The results showed that both rEmGAM56 and rEmROP17 had good reactogenicity. The rEmGAM56- or rEmROP17-immunized rabbits had milder clinical symptoms and feed conversion ratios of 3.27:1 and 3.37:1, respectively. The rEmGAM56-immunized rabbits had 81.35% body weight gain and 63.85% oocyst output reduction; the rEmROP17-immunized rabbits had 79.03% body weight gain and 80.10% oocyst output reduction. The ACI of rGC and rRC groups were 162.35 and 171.03, respectively. The specific antibody levels increased rapidly after immunization. Significantly increased interleukin (IL)-2, interferon (IFN)-γ, and IL-17 levels were evident in the rGC and rRC groups (p < 0.05). The rEmGAM56 and rEmROP17 elicited humoral and cellular responses, which protected against E. magna infection in rabbits. Thus, rEmGAM56 and rEmROP17 are potential vaccine candidates against E. magna, and rEmROP17 performed better than rEmGAM56.
1. Introduction
Rabbit coccidiosis is a highly contagious protozoan disease, and the prevalence in rabbitries is more than 90% even with the use of anticoccidials (1, 2). To date, 11 Eimeria spp. have been identified as the valid species of rabbit coccidiosis, among which, although Eimeria magna is a mildly pathogenic species but it is widely distributed in rabbitries (3, 4). E. magna can infect rabbits of all ages, especially juvenile rabbits (5, 6). E. magna parasitizes the ileum and jejunum of rabbits, causing depression, watery stool, diarrhea, reduced growth performance, reduced feed conversion efficiency, and even death of rabbits (7–9). Epidemiological investigations have suggested the high infection prevalence (17-42%) and oocyst output intensity of E. magna (5, 10, 11). Meanwhile, due to the development of the resistance to robenidine, some E. magna strains are predominant in rabbit breeding (12). Moreover, coccidiosis infection may cause other secondary diseases in subclinical conditions. Therefore, rabbit coccidiosis is responsible for large economic losses in the rabbit industry (5, 11).
Traditional coccidiosis control is heavily reliant on anticoccidials, but concerns over drug resistance and drug residue encouraged the search for new control strategies such as vaccines (13). Current research on rabbit E. magna vaccines has mainly focused on live attenuated vaccines (14–17). However, live anticoccidial vaccines are expensive to produce and carry the risk of virulence reversal (18). Compared with live attenuated vaccines, recombinant antigen-based subunit vaccines are high-stability and easy to mass produce, which significantly reduces the antigen production cost (19).
Secreted by rhoptry, rhoptry kinase family proteins (or rhoptry bulb proteins, ROPs) are critical in host cell invasion (20). Recently, it was reported that ROP16 (21), ROP17 (22, 23), and ROP18 (24) confer protection against apicomplexan infections. Gametocyte antigen 56 (GAM56) of chicken E. maxima demonstrated good antigenicity and immunogenicity (25) where the homologous gene of GAM56 was identified in E. tenella and E. acervulina (26). Chickens immunized with anti- E. tenella (Et)GAM56 antibody were protected against coccidiosis by passive immunity (27).
In the present study, the E. magna (Em)GAM56 and EmROP17 genes were selected and cloned based on our E. magna transcriptome data. Recombinant GAM56 (rEmGAM56) and ROP17 (rEmROP17) proteins were obtained from a prokaryotic expression system. Then, we investigated the immune responses and protective effects of rEmGAM56 and rEmROP17 against homologous challenge with E. magna in rabbits. To our knowledge, this is the first report of the two recombinant subunit vaccines against E. magna.
2. Materials and methods
2.1. Parasites and animals
The E. magna Chinese isolate was kindly provided by Xianyong Liu of China Agricultural University (Beijing, China) (9) and was preserved and passaged at our laboratory. Sixty coccidia-free New Zealand White rabbits (35 days old, 0.84 ± 0.108 kg, 30 females and 30 males, n = 5 females and 5 males per group) were randomly grouped then raised according to Wei et al. (28). The rabbits were housed in pairs in flame-sterilized steel cages, and a plastic partition was placed at the bottom of each cage to prevent the experimental rabbits from contacting feces. Anticoccidial drugs were discontinued 1 week before the challenge infection and pathogenic examination was performed every other day to ensure that no coccidia oocysts were detected. The rabbits were vaccinated with a bivalent vaccine against rabbit hemorrhagic disease virus and Pasteurella multocida when they were 30 days old.
The experimental groups were the rEmGAM56 (rGC) and rEmROP17 (rRC) groups (rEmGAM56- or rEmROP17-immunized and E. magna-challenged); the positive control groups were the unimmunized-challenged (UC, sterile phosphate-buffered saline [PBS] mock-immunized and E. magna-challenged), Quil-A-challenged (QC, saponin derivative Quil-A mock-immunized and E. magna-challenged), and rTrx-His-S-challenged (rTC) groups (recombinant pET-32a tag protein mock-immunized and E. magna-challenged); and the negative control unimmunized-unchallenged (UU) group (sterile PBS mock-immunized without E. magna challenge). The rabbits were immunized at 35 days of age, and a booster immunization was conducted 2 weeks later (49 days old); then the rabbits were challenged 2 weeks after the booster immunization (63 days old) (Table 1).
2.2. EmGAM56 and EmROP17 sequence analysis
The EmGAM56 and EmROP17 open reading frames (ORF) and amino acid sequences were obtained using ORF Finder (https://www.ncbi.nlm.nih.gov/orffinder/). The molecular weight (MW) of the proteins was predicted with the ExPASy proteomics server (http://web.Expasy.org/protparam/). The transmembrane regions and signal peptides of the proteins were analyzed with TMHMM Server v.2.0 (http://www.cbs.dtu.dk/services/TMHMM/#opennewwindow) and the SignalP 4.1 server (http://www.cbs.dtu.dk/services/SignalP/), respectively. B cell epitopes were predicted using the Immune Epitope Database Analysis Resource (http://tools.immuneepitope.org/bcell/). Multiple sequence alignment was performed using Jalview 2.11.2.0 (29).
2.3. Cloning, expression, and purification
Total RNAs of E. magna (unsporulated oocysts, sporulated oocysts, merozoites, and gametocytes) were extracted using a commercial kit (Tiangen, Beijing, China) and the complementary DNAs (cDNAs) were synthesized (Thermo Fisher Scientific, Waltham, MA, USA). Then the resulting cDNAs were mixed and used as a template for PCR amplification.
The specific forward (F) and reverse (R) primers for EmGAM56 and EmROP17 were designed based on E. magna transcriptome data: EmGAM56-F 5′-CGGGATCCATGGAACCCTCTACCATTGAG-3′ and EmGAM56-R 5′-GCGTCGACTTAGAAAGGCATGCCTGC-3′; EmROP17-F 5′-CGGGATCCATGTACAGCCTCTTACAAGGTCAC-3′ and EmROP17-R 5′-GCGTCGACCTACTCTGAGCTTTTTCCTTCACT-3′, and contained BamHI and SalI restriction enzyme sites (underlined) (Takara, Dalian, China). The purified PCR amplification products were cloned into pET-32a(+) plasmids, then the recombinant plasmids pET-32a(+)-EmGAM56 and pET-32a(+)-EmROP17 were sequenced (Sangon, Shanghai, China) and transformed into Escherichia coli BL21 for protein expression (1 mM isopropyl-β-d-thiogalactoside [IPTG]). The rEmGAM56 and rEmROP17 proteins were purified (HisTrap HP, Cytiva, Marlborough, MA, USA) then separated using 12% sodium dodecyl sulfate–polyacrylamide gel electrophoresis (SDS-PAGE). The purified fusion Trx-His-S tag protein (with no insert fragment) was cryopreserved in our laboratory.
2.4. Western blotting
The anti-E. magna positive serum and negative serum were provided by the Sichuan Agricultural University Department of Parasitology.
After 12% SDS-PAGE separation, the rEmGAM56 and rEmROP17 were transferred onto nitrocellulose membranes (Boster, Wuhan, China). The membranes were blocked for 2 h using 5% (w/v) skimmed milk solution in Tris-buffered saline (TBS) at room temperature, then incubated overnight at 4°C with anti-E. magna positive serum (1:200 v/v dilution in TBS) and negative serum (1:200 v/v dilution in TBS). After four washes with TBST (TBS+0.05% Tween-20), the membranes were incubated with horseradish peroxidase-conjugated goat anti-rabbit IgG (1:2000 v/v dilution, EarthOx Life Sciences, Millbrae, CA, USA) for 2 h at room temperature. After four washes, the immunoreactive protein bands were detected using a Metal Enhanced DAB Substrate Kit (20×) (Solarbio, Beijing, China).
2.5. Immunization and challenge
The trial design and immune procedures are detailed in Table 1 and Figure 1. The rabbits were sacrificed 2 weeks after the challenge.
2.6. Evaluation of protective efficacy
To evaluate the safety of the rEmGAM56 and rEmROP17 proteins, we observed the health status and body weight gain after immunization of all experimental rabbits. The body weight of each rabbit was recorded before the first immunization, booster immunization, and challenge. The weight gain after immunization was calculated as the weight before challenge minus the weight before the first immunization to verify whether the immunization affected the weight gain of the experimental rabbits.
For protective efficacy, there is currently no specific standard for evaluating recombinant subunit vaccines against rabbit coccidiosis. In this study, after rabbits were challenged with E. magna, we observed whether the rabbits developed diarrhea and decreased appetite, and the protective effects were evaluated according to the following parameters among groups (n = 10 per group) (1): the survival rate (%) = the number of surviving rabbits/the initial number of rabbits × 100 (2); the body weight gain after challenge (g) = weight before sacrifice (g) − weight before challenge (g); (3) after the rabbits had been sacrificed, 2 g feces was collected from the rectum, and the amount of oocysts excreted per g feces (OPG) was calculated using the McMaster method (30), the oocyst decrease ratio (%) = (the OPG of UC group − the OPG of experimental group)/the OPG of UC group × 100 (4); feed conversion ratio = feed consumption (g)/rabbit’s mass after challenge (g), to be specific, after the challenge, the initial weight of feed for each group was recorded, and rabbits were given the same amount of feed every day; at the end of the experiment, the remaining feed of each group was weighed again (final weight), and the feed consumption of each group was obtained by subtracting the final weight from the initial weight; the feed conversion ratio was obtained by dividing the feed consumption by the total weight gain of all rabbits in each group after challenge (31); (5) ACI = (relative rate of weight gain + survival rate) − (lesion value + oocyst value), and the ACI ≥ 180 is considered good or effective, 160 ≤ ACI < 179 is considered moderately effective, ACI < 160 is considered poor effective (32–34).
2.7. Serum IgG level detection
The sera of all rabbits were collected pre-immunization, and then collected weekly after immunization. All serum samples were stored at −20°C.
The specific antibody levels of the immunized rabbits were evaluated using indirect enzyme-linked immunosorbent assay (ELISA) based on rEmGAM56 and rEmROP17 (35). The optimal concentration of rEmGAM56 and rEmROP17 was 0.94 μg/well and 1.13 μg/well, respectively. The optimal serum dilution ratio was 1:160.
2.8. Serum cytokine level detection
The rabbit serum interleukin (IL)-2, IL-4, IL-10, IL-17, interferon gamma (IFN-γ), and transforming growth factor beta 1 (TGF-β1) levels were detected using commercial ELISA kits (Cusabio, Wuhan, China). For each group, serum of six rabbits were randomly selected.
2.9. Statistical analysis
Differences among the groups were assessed using one-way analysis of variance (ANOVA) with IBM SPSS Statistics 22.0 (IBM, Armonk, NY, USA). GraphPad Prism 8.0.2 (GraphPad Software Inc., La Jolla, CA, USA) was used to produce all the graphs. P < 0.05 and < 0.01 were considered significant and extremely significant, respectively.
3. Results
3.1. EmGAM56 and EmROP17 sequence features
The EmGAM56 gene (GenBank accession number: OM451230) ORF was 1371 bp (encoding a protein with a predicted MW of 51 kDa) while that of the EmROP17 gene (GenBank accession number: OM451229) was 1725 bp (encoding a protein with a predicted MW of 63 kDa). Neither EmGAM56 nor EmROP17 contain a transmembrane region, but the signal peptides were predicted at 1–20 and 1–22 amino acids, respectively. The EmGAM56 and EmROP17 target fragment sizes without signal peptides were 1314 bp and 1662 bp, respectively.
Multiple sequence alignment revealed that the EmGAM56 amino acid sequences had high variability but shared high homology with rabbit E. stiedae (89.44%). The EmROP17 amino acid sequences shared 66.96% identity with E. stiedae ROP17 proteins and 28.01~37.95% identity with that of other apicomplexans (Figure 2).
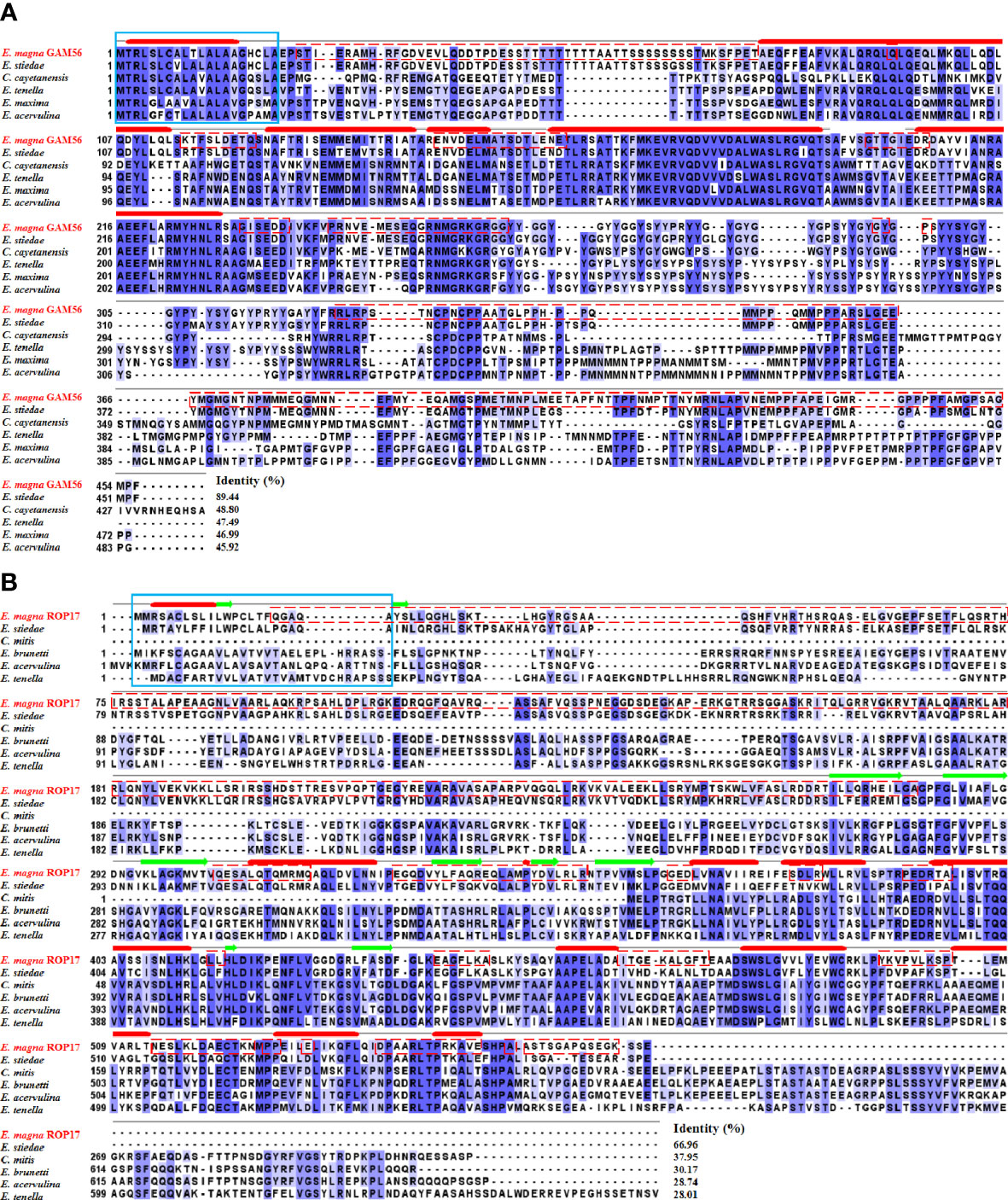
Figure 2 Multiple sequence alignment of GAM56 and ROP17 from different species. (A) Multiple sequence alignment of EmGAM56 with GAM56 proteins from E stiedae (GenBank accession number: OL622034), Cyclospora cayetanensis (UniProt: A0A1D3D9G4), E tenella (UniProt: U6KUA4), E maxima (UniProt: U6M5G7), and E acervulina (UniProt: U6GGM3). (B) Multiple sequence alignment of EmROP17 with ROP17 proteins from E stiedae (GenBank accession number: OM451231), E mitis (UniProt: U6KAV7), E brunetti (UniProt: U6LD89), E acervulina (UniProt: U6GVC3), and E tenella (UniProt: U6KG78). Blue shading indicates conserved residues. Dashed red outlines represent B cell epitopes. The signal peptides are marked with a solid blue outline. The thick solid red lines indicate predicted alpha helix, and the green arrows indicate predicted beta sheet.
3.2. Expression, purification, and western blotting
The rEmGAM56 (~48 kDa) and rEmROP17 (~54 kDa) were expressed in the supernatant of E. coli BL21 cells after IPTG induction (Figure 3, lane 1). The MW of the recombinant proteins included the ~20 kDa fusion tag protein encoded by pET-32a(+) plasmid. After HisTrap HP affinity column purification, the recombinant proteins were separated using 12% SDS-PAGE (Figure 3, lane 2).
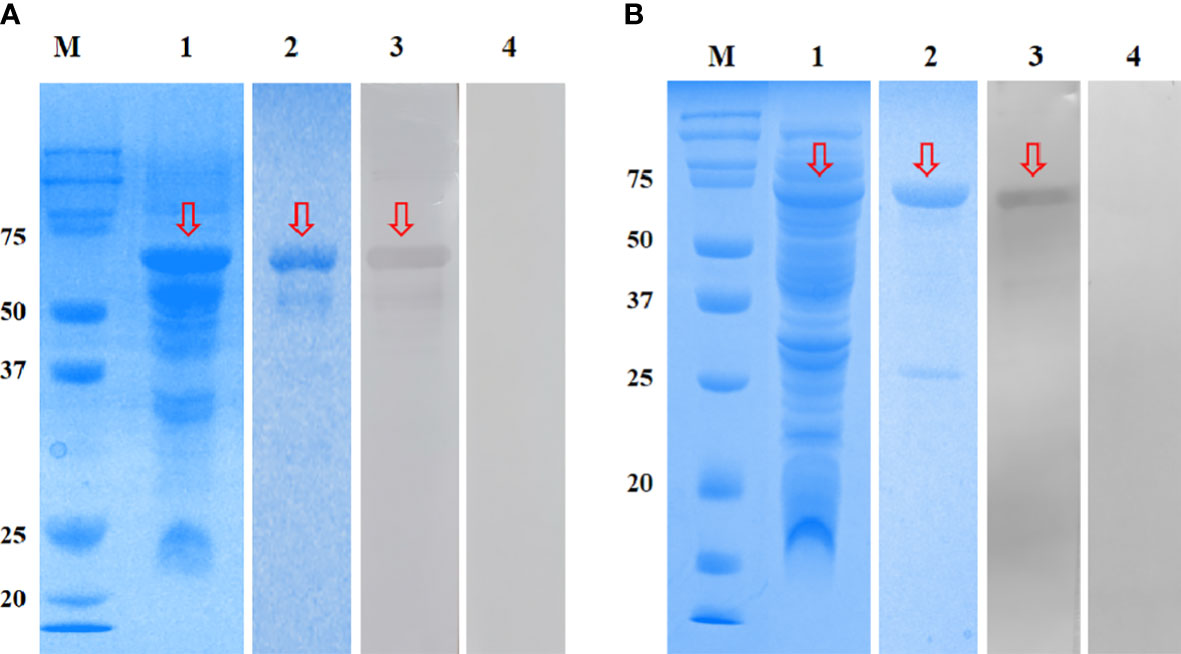
Figure 3 SDS-PAGE and Western blotting analysis of rEmGAM56 (A) and rEmROP17 (B). Lane M: Protein molecular weight markers; lane 1: crude extracts expressed by BL21 (DE3); lane 2: purified recombinant proteins; lane 3: purified recombinant proteins incubated with anti-E. magna positive serum; lane 4: purified recombinant proteins incubated with negative serum from coccidia-free rabbits. Arrows indicate the bands of interest.
The rEmGAM56 and rEmROP17 were reacted with anti-E. magna positive serum and specific bands were observed on the NC membranes (Figure 3, lane 3), while the coccidia-free rabbit serum yielded no specific bands (Figure 3, lane 4). These results indicated that both rEmGAM56 and rEmROP17 had strong reactogenicity.
3.3. Protective efficacy of rEmGAM56 and rEmROP17
No statistically significant differences were observed for weight gain after immunization among the six groups (p > 0.05) (Table 2), nor were obvious adverse reactions observed in the immunized rabbits. This result suggested that rEmGAM56 and rEmROP17 had good safety at the experimental doses.
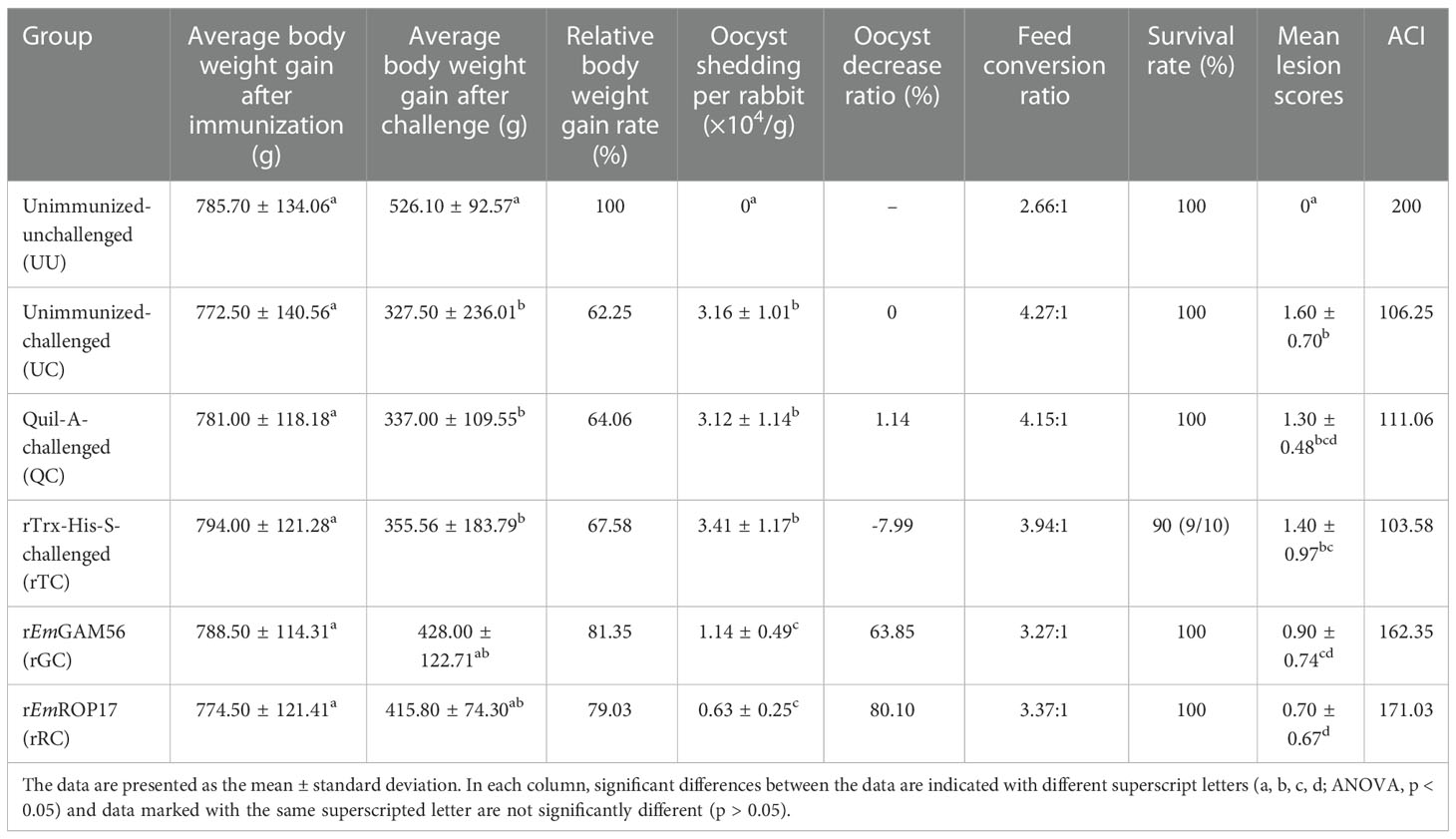
Table 2 Protective effects of rEmGAM56 and rEmROP17 against E. magna infection under different evaluation indicators.
Rabbits in the positive control groups demonstrated a slight loss of appetite and weight loss 2 weeks after the challenge; only a few rabbits had diarrhea, most of which manifested as soft unformed feces. No obvious clinical symptoms developed in the rGC and rRC groups, and a minority of rabbits had soft unformed feces. Gross postmortem examination of the positive control groups revealed obvious hemorrhagic spots in the ileum and lower jejunum while the immunized groups had few or no hemorrhagic spots (Figure 4).
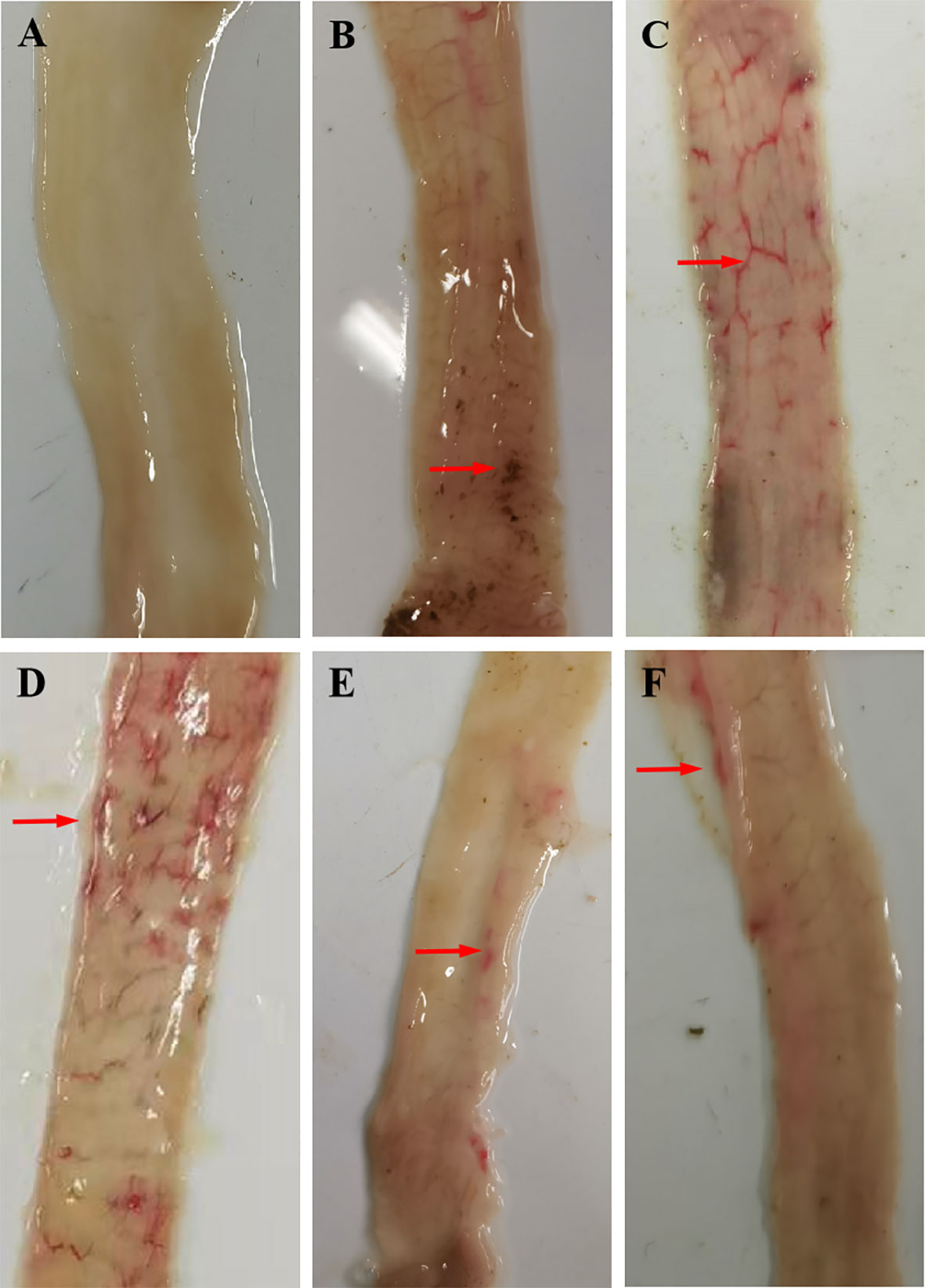
Figure 4 Gross postmortem examination. (A) UU, (B) UC, (C) QC, (D) rTC, (E) rGC, and (F) rRC groups.
Compared with the UC group, the relative body weight gain rate of the rGC and rRC groups was 81.35% and 79.03%, respectively (p > 0.05). In addition, the rabbits immunized with rEmGAM56 (63.85% oocyst reduction ratio) and rEmROP17 (80.10% oocyst reduction ratio) had significantly lower oocyst output (p < 0.05).
The rGC (3.27:1) and rRC (3.37:1) groups had better feed conversion ratios in comparison with the UC (4.27:1), QC (4.15:1), and rTC (3.94:1) positive control groups. In addition, the results of ACI indicated that rEmGAM56 and rEmROP17 could provide moderately effective protection.
3.4. IgG responses against rEmGAM56 and rEmROP17
The serum specific IgG levels of the rGC and rRC groups increased significantly after immunization (Figure 5). The specific IgG levels of the rGC group peaked at week 3 but decreased at week 5 while that of the rRC group continued to increase after the challenge. The rTC group also exhibited increased antibody levels, indicating that the inclusion of the Trx-His-S tag in the rEmGAM56 and rEmROP17 proteins increased the antibody levels. Nevertheless, the rTC group had lower antibody levels than the rGC and rRC groups.
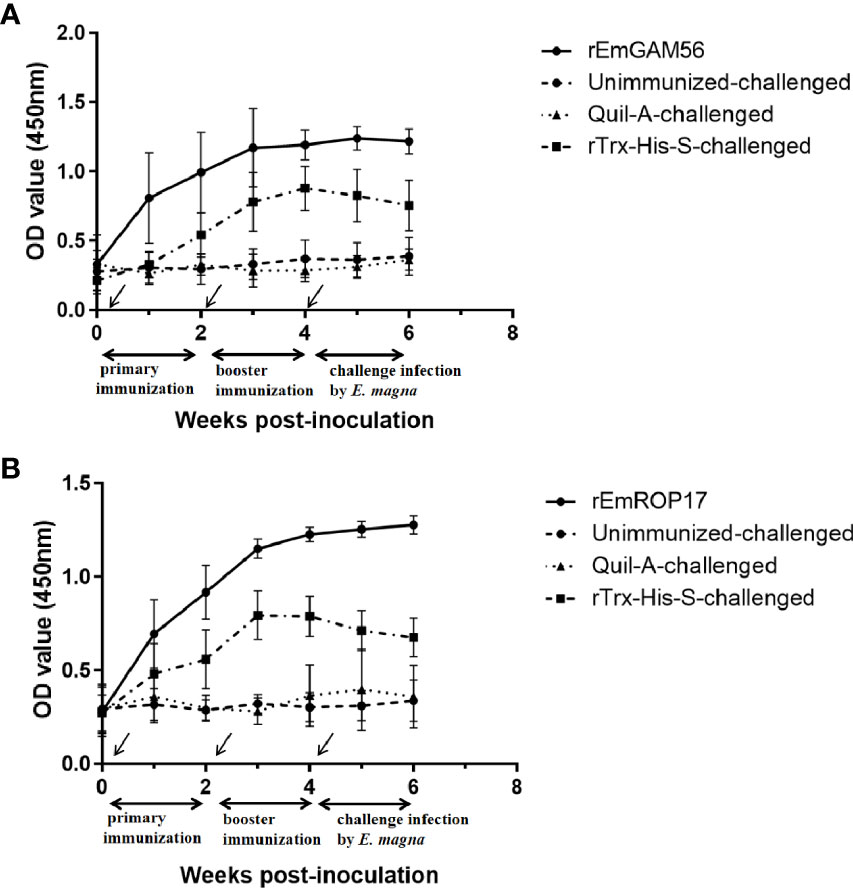
Figure 5 The changes in serum anti-rEmGAM56 (A) and rEmROP17 (B) IgG levels after the first immunization (week 0), booster immunization (week 2), and challenge with E magna (week 4).
3.5. rEmGAM56- and rEmROP17-induced serum cytokine levels
The serum cytokine levels were estimated 2 weeks after the booster vaccination. In the rGC group, serum IL-17 and IFN-γ levels were significantly increased (p < 0.05) and serum IL-2 levels were significantly higher (p < 0.05) than that of the UC and rTC groups, but there was no significant difference with the QC group (p > 0.05). The rRC group had significantly increased serum IL-2, IL-17, and IFN-γ levels (p < 0.05). There was no significant difference in the serum TGF-β1 levels among the groups (Figure 6).
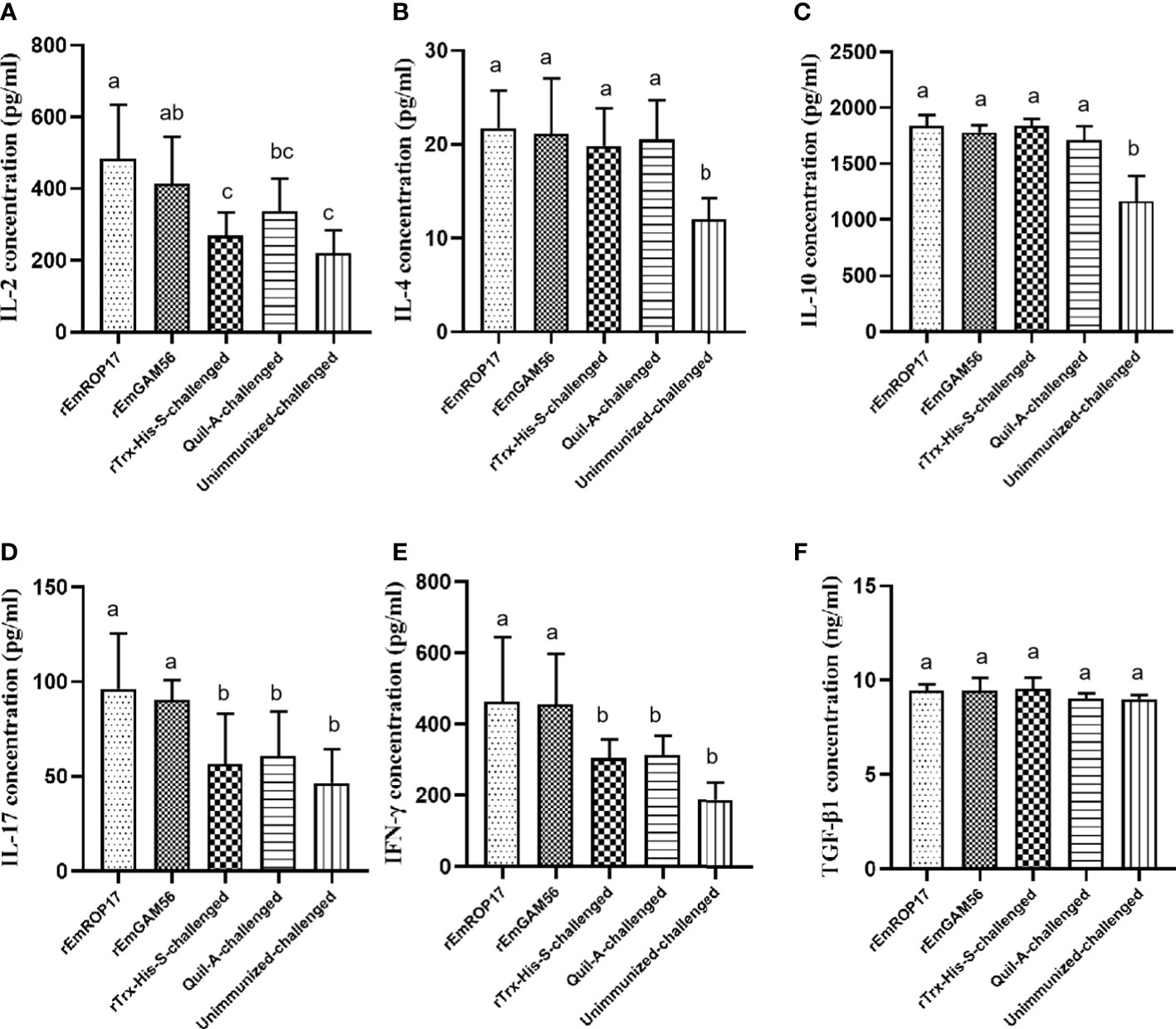
Figure 6 The serum IL-2 (A), IL-4 (B), IL-10 (C), IL-17 (D), IFN-γ (E), and TGF-β1 (F) levels 2 weeks after booster vaccination. Different superscript letters (a, b) indicate a significant difference (p < 0.05). The same superscript letters indicate no significant difference (p > 0.05).
4. Discussion
Rabbit coccidiosis is a common and highly contagious parasitic protozoan disease (3, 11). Anticoccidials are the main control method of rabbit coccidiosis but can be accompanied by drug resistance and drug residue. Previous work on anticoccidial vaccines predominantly focused on live vaccines, specifically the precocious lines. Several precocious lines of rabbit Eimeria spp. have been successfully selected and characterized so far, such as that for E. magna, and immunogenicity studies have been conducted (8). Mohamed et al. (17) reported 97% oocyst output reduction in rabbits following vaccination with 3500 oocysts from an E. magna precocious line. However, live anticoccidial vaccines are expensive to produce and carry the risk of virulence reversal. Therefore, recombinant subunit vaccines are a promising vaccination strategy as they are easier to mass-produce than live vaccines and have a longer shelf life (19). Some studies that explored the protective effects of recombinant subunit vaccines against chicken coccidiosis yielded good results (36, 37). For rabbit coccidiosis, early studies have reported that the soluble antigens in the bile and coproantigen from E. stiedae-infected rabbits could induce protection against E. stiedae infection (38–40). Meanwhile, our previous studies found that rabbits immunized with the recombinant protein-based subunit vaccines also displayed good protective effects against E. stiedae or E. magna infection (41, 42). These studies showed the feasibility of developing vaccines using the immunodominant antigens of rabbit coccidia.
Produced by Eimeria spp. gametocytes, GAM56 is involved in oocyst wall formation (43, 44). The E. maxima GAM56 antigen is one of the main components of CoxAbic®, where chickens immunized with its antibody had 78% oocyst output reduction (27, 45). Moreover, chickens immunized with a DNA vaccine carrying EmaxiGAM56 had 89.7% relative body weight gain and 53.7% oocyst output reduction (46). Here, the rabbits immunized with rEmGAM56 had significantly reduced oocyst output (63.85% reduction rate, p < 0.05) and up to 81.35% relative body weight gain.
ROPs are important in Apicomplexa host invasion (20). Some ROPs (such as ROP16, ROP17, ROP18) are serine/threonine kinases that act as virulence factors (47, 48). Several ROPs have been tested for immunogenicity, including ROP17 (49). BALB/c mice immunized with recombinant Toxoplasma gondii ROP17 protein received apparent protection against chronic infection, and the liver and brain parasite burdens were reduced by 59.17% and 49.08%, respectively; in immunized mice, survival increased by 50% against lethal infection (22). Liu et al. (23) identified the ROP17 of chicken E. tenella, where challenge experiments revealed that immunization with rEtROP17 protein significantly reduced oocyst output, and the body weight gain was up to 84.14%. In the present study, rEmROP17-immunized rabbits had 79.03% body weight gain and 80.10% oocyst output reduction. The results demonstrated that both the rEmGAM56 and rEmROP17 proteins conferred protection against E. magna infection and that rEmROP17 performed better for reducing oocyst output.
Cytokines play a role in fighting coccidiosis. A Th1 immune response marker, IFN-γ is considered a key factor for preventing coccidiosis (50). Chicken IFN-γ inhibited Eimeria sporozoite development in vitro and its recombinant protein also exerted an anticoccidial effect (51, 52). IL-2 exerted an anticoccidial effect by inducing T cell proliferation and increasing CD8+ and CD4+ T cell ratios (50). IFN-γ and IL-2 also acted as anticoccidial vaccine adjuvants to enhance the immune response to vaccine antigens (53). Combining vaccine antigens with IFN-γ or IL-2 further improved the anticoccidial index of chickens (54–56). In the present study, the IL-2 and IFN-γ levels of the immunized rabbits were significantly increased post-vaccination (p < 0.05), indicating that rEmGAM56 and rEmROP17 stimulated Th1-type immune responses. We also observed increased IL-17 levels in the rGC and rRC groups (p < 0.05). IL-17 is important in responses against parasite infection (57). Wild-type mice treated with IL-17A neutralizing antibody had prolonged survival after being challenged with T. gondii (58). Ding et al. (55) reported that simultaneous immunization with recombinant 3-1E protein and IL-17 gene in ovo enhanced the immune protection against E. maxima infection in chickens. Geriletu et al. (59) also reported that the IL-17 gene enhanced the anticoccidial effect of antigens.
In the present study, both the rEmGAM56 and rEmROP17 proteins exerted moderately protective effects against E. magna infection, and rEmROP17 performed better for reducing oocyst output. Immunized with rEmGAM56 and rEmROP17 could induce humoral immunity in the rabbits, and the specific IgG was significantly increased. Recent research has proven that antibodies are involved in the occurrence of Eimeria infection (60, 61), where antigen-specific antibodies inhibited adhesion to host cells (62). Moreover, it was shown that there was an excellent correlation between antibody titer and protection (63). As an obligate intracellular parasite, Eimeria spp. has a complex life cycle including the asexual and sexual replicative stages, and the asexual replicative stages (sporozoites and merozoites) lead to the most damage to the intestinal tissues (64, 65). ROPs play an important role during the early stages of host invasion, and ROP17 is expressed in the sporozoites and merozoites (23, 66, 67). Gametocyte antigens are involved in oocyst wall formation in the later sexual replicative stage (43, 44). Therefore, we speculated that the anti-rEmROP17 antibody might interact with sporozoites or merozoites, and inhibit the invasion of intestinal epithelial cells by them to alleviate intestinal damage; while the anti-rEmGAM56 antibody mainly plays the role of inhibiting the oocyst wall formation, but at this time, the parasites have completed asexual replicative stages, causing irreversible damage to the intestinal tissues, this may lead to a better protective effect of rEmROP17. Meanwhile, the high IFN-γ, IL-2, and IL-17 levels in the immunized rabbits further inhibited the intracellular infection of E. magna. Together, these effects might eventually lead to significant differences in oocyst output and body weight gain.
5. Conclusions
The rEmGAM56 and rEmROP17 proteins conferred protective immunity against E. magna infection in rabbits. The relative body weight gain of the rEmGAM56- and rEmROP17-immunized rabbits was 81.35% and 79.03%, respectively, and the oocyst output reduction rate was 63.85% and 80.10%, respectively. The rEmROP17 performed better in reducing oocyst output. The rEmGAM56 and rEmROP17 proteins elicited cellular and humoral immune responses and are potential vaccine candidates against E. magna.
Data availability statement
The datasets presented in this study can be found in online repositories. The names of the repository/repositories and accession number(s) can be found below: https://www.ncbi.nlm.nih.gov/genbank/, OM451230 https://www.ncbi.nlm.nih.gov/genbank/, OM451229.
Ethics statement
The animal study was reviewed and approved by The Sichuan Agricultural University Animal Care and Use Committee of reviewed and approved the animal study protocol (SYXK 2019–189). All animal procedures used in this study were performed in accordance with the Guide for the Care and Use of Laboratory Animals (National Research Council, Bethesda, MD, USA) and the recommendations of the Animal Research: Reporting of In Vivo Experiments (ARRIVE) guidelines (http://www.nc3rs.org.uk/arrive-guidelines). All applicable institutional and national guidelines for the care and use of animals were followed.
Author contributions
JXi participated in the design of the study, fed the experimental animals, and performed the experiments, statistical analysis, and manuscript writing. HC fed the experimental animals and performed the experiments. RZ, JP, and BJ contributed to the sample collection. GY participated in the design of the study. XG, YX, RH, JXu, XP, and GY assisted in the study design. All authors read and approved the final manuscript.
Funding
This work was supported by the National Key Research and Development Program of China (2017YFD0501200). The funder had no role in the design of the study, data collection, analysis, and interpretation, and writing the manuscript.
Acknowledgments
The authors thank Xin Bai, Wei He, Ge Hao, and Changming Xiong for their contributions to the sample collection. We also thank the native English-speaking scientists of Elixigen (Huntington Beach, CA, USA) for editing our manuscript..
Conflict of interest
The authors declare that the research was conducted in the absence of any commercial or financial relationships that could be construed as a potential conflict of interest.
Publisher’s note
All claims expressed in this article are solely those of the authors and do not necessarily represent those of their affiliated organizations, or those of the publisher, the editors and the reviewers. Any product that may be evaluated in this article, or claim that may be made by its manufacturer, is not guaranteed or endorsed by the publisher.
Supplementary Material
The Supplementary Material for this article can be found online at: https://www.frontiersin.org/articles/10.3389/fimmu.2022.1037949/full#supplementary-material
References
1. Duszynski DW, Couch L. The biology and identification of the coccidia (Apicomplexa) of rabbits of the world. Rev Colomb Entomol (2013) 37:340. doi: 10.1584/jpestics.W11-09
2. Pilarczyk B, Tomza-Marciniak A, Pilarczyk R, Januś E, Stanek P, Seremak B, et al. The effect of the sex, age, and breed of farmed rabbits and the choice of management system on the extensity and intensity of Eimeria infection. Vet World (2020) 13:1654–60. doi: 10.14202/vetworld.2020.1654-1660
3. Bhat T, Jithendran K, Kurade N. Rabbit coccidiosis and its control: A review. World Rabbit Sci (1996) 4:37–41. doi: 10.4995/wrs.1996.269
4. Kvicerová J, Pakandl M, Hypsa V. Phylogenetic relationships among Eimeria spp. (Apicomplexa, eimeriidae) infecting rabbits: Evolutionary significance of biological and morphological features. Parasitology (2008) 135:443–52. doi: 10.1017/S0031182007004106
5. Kornaś S, Kowal J, Wierzbowska I, Basiaga M, Nosal P, Niedbała P. The Alice - "Follow the white rabbit" - parasites of farm rabbits based on coproscopy. Ann Parasitol (2015) 61:257–61. doi: 10.17420/ap6104.16
6. Pakandl M, Hlásková L, Poplstein M, Chromá V, Vodicka T, Salát J, et al. Dependence of the immune response to coccidiosis on the age of rabbit suckling. Parasitol Res (2008) 103:1265. doi: 10.1007/s00436-008-1123-0
7. Li TS, Zou Y, Ma YT, Ma YY, Chen H, Liang XX, et al. Molecular characterization of Eimeria spp. and Blastocystis in rabbits in Shandong province, China. Parasitol Res (2020) 119:1547–51. doi: 10.1007/s00436-020-06652-0
8. Pakandl M. Coccidia of rabbit: A review. Folia Parasitol (Praha) (2009) 56:153–66. doi: 10.14411/fp.2009.019
9. Tao G, Wang Y, Li C, Gu X, Cui P, Fang S, et al. High pathogenicity and strong immunogenicity of a Chinese isolate of Eimeria magna pérard, 1925. Parasitol Int (2017) 66:207. doi: 10.1016/j.parint.2017.01.014
10. Jing F, Yin G, Liu X, Suo X, Qin Y. Large-Scale survey of the prevalence of Eimeria infections in domestic rabbits in China. Parasitol Res (2011) 110:1495–500. doi: 10.1007/s00436-011-2653-4
11. Yin G, Goraya MU, Huang J, Suo X, Huang Z, Liu X. Survey of coccidial infection of rabbits in sichuan province, southwest China. Springerplus (2016) 5:1–4. doi: 10.1186/s40064-016-2586-6
12. Peeters JE, Geeroms R, Norton CC. Eimeria magna: Resistance against robenidine in the rabbit. Vet Rec (1987) 121:545–6. doi: 10.1136/vr.121.23.545
13. Licois D, Coudert P, Drouet-Viard F, Boivin M. Eimeria magna: pathogenicity, immunogenicity and selection of a precocious line. Vet Parasitol (1995) 60:27–35. doi: 10.1016/0304-4017(94)00768-8
14. Drouet-Viard F, Coudert P, Licois D, Boivin M. Vaccination against Eimeria magna coccidiosis using spray dispersion of precocious line oocysts in the nest box. Vet Parasitol (1997) 70:61. doi: 10.1016/S0304-4017(96)01134-X
15. Fang S, Gu X, El-Ashram S, Li X, Yu X, Guo B, et al. Immune protection provided by a precocious line trivalent vaccine against rabbit eimeria. Vet Parasitol (2019) 275:108927. doi: 10.1016/j.vetpar.2019.108927
16. Akpo Y, Kpodékon MT, Djago Y, Licois D, Youssao IAK. Vaccination of rabbits against coccidiosis using precocious lines of Eimeria magna and Eimeria media in Benin. Vet Parasitol (2012) 184:73–6. doi: 10.1016/j.vetpar.2011.08.012
17. Bachene MS, Temim S, Ainbaziz H, Bachene A, Suo X. A vaccination trial with a precocious line of eimeria magna in Algerian local rabbits Oryctolagus cuniculus. Vet Parasitol (2018) 261:73–6. doi: 10.1016/j.vetpar.2018.08.013
18. Peek HW, Landman WJM. Coccidiosis in poultry: anticoccidial products, vaccines and other prevention strategies. Vet Q (2011) 31:143–61. doi: 10.1080/01652176.2011.605247
19. Jenkins MC. Advances and prospects for subunit vaccines against protozoa of veterinary importance. Vet Parasitol (2001) 101:291–310. doi: 10.1016/S0304-4017(01)00557-X
20. Sparvoli D, Lebrun M. Unraveling the elusive rhoptry exocytic mechanism of apicomplexa. Trends Parasitol (2021) 37:622–37. doi: 10.1016/j.pt.2021.04.011
21. Cao A, Liu Y, Wang J, Li X, Wang S, Zhao Q, et al. Toxoplasma gondii: Vaccination with a DNA vaccine encoding T- and b-cell epitopes of SAG1, GRA2, GRA7 and ROP16 elicits protection against acute toxoplasmosis in mice. Vaccine (2015) 33:6757–62. doi: 10.1016/j.vaccine.2015.10.077
22. Wang HL, Zhang TE, Yin LT, Pang M, Guan L, Liu HL, et al. Partial protective effect of intranasal immunization with recombinant Toxoplasma gondii rhoptry protein 17 against toxoplasmosis in mice. PloS One (2014) 9:e108377. doi: 10.1371/journal.pone.0108377
23. Liu X, Mu B, Zheng W, Meng Y, Yu L, Gao W, et al. Identification and protective efficacy of Eimeria tenella rhoptry kinase family protein 17. Anim (Basel) (2022) 12:556. doi: 10.3390/ani12050556
24. Zhu YC, He Y, Liu JF, Chen J. Adjuvantic cytokine IL-33 improves the protective immunity of cocktailed DNA vaccine of ROP5 and ROP18 against Toxoplasma gondii infection in mice. Parasite (2020) 27:26. doi: 10.1051/parasite/2020021
25. Belli SI, Mai K, Skene CD, Gleeson MT, Witcombe DM, Katrib M, et al. Characterisation of the antigenic and immunogenic properties of bacterially expressed, sexual stage antigens of the coccidian parasite, eimeria maxima. Vaccine (2004) 22:4316–25. doi: 10.1016/j.vaccine.2004.04.019
26. Belli SI, Ferguson D, Katrib M, Slapetova I, Mai K, Slapeta J, et al. Conservation of proteins involved in oocyst wall formation in Eimeria maxima, Eimeria tenella and eimeria acervulina. Int J Parasitol (2009) 39:1063–70. doi: 10.1016/j.ijpara.2009.05.004
27. Wiedmer S, Alnassan AA, Volke B, Thabet A, Daugschies A, Lendner M, et al. Passive immunization with eimeria tenella gametocyte antigen 56 (EtGAM56) specific antibodies and active immunization trial with the epitope containing peptide. Vet Parasitol (2017) 247:100–7. doi: 10.1016/j.vetpar.2017.09.019
28. Wei W, Shen N, Xiao J, Tao Y, Luo Y, Angel C, et al. Expression analysis and serodiagnostic potential of microneme proteins 1 and 3 in eimeria stiedai. Genes (2020) 11:725. doi: 10.3390/genes11070725
29. Waterhouse AM, Procter JB, Martin D, Clamp M, Barton GJ. Jalview version 2–a multiple sequence alignment editor and analysis workbench. Bioinformatics (2009) 25:1189–91. doi: 10.1093/bioinformatics/btp033
30. Bortoluzzi C, Paras KL, Applegate TJ, Verocai GG. Comparison between McMaster and mini-FLOTAC techniques for the enumeration of Eimeria maxima oocysts in poultry excreta. Vet Parasitol (2018) 254:21–5. doi: 10.1016/j.vetpar.2018.02.039
31. Drouilhet L, Gilbert H, Balmisse E, Ruesche J, Tircazes A, Larzul C, et al. Genetic parameters for two selection criteria for feed efficiency in rabbits. J Anim Sci (2013) 91:3121–8. doi: 10.2527/jas.2012-6176
32. Johnson J, Reid WM. Anticoccidial drugs: lesion scoring techniques in battery and floor-pen experiments with chickens. Exp Parasitol (1970) 28:30–6. doi: 10.1016/0014-4894(70)90063-9
33. Zhao AY, Duan JS. Pathogenicity and immunogenicity a new isolated strain of e. Mitis J Beijing Agric Coll (2006) 21:50–2. doi: 10.3969/j.issn.1002-3186.2006.01.013
34. McManus EC, Campbell WC, Cuckler AC. Development of resistance to quinoline coccidiostats under field and laboratory conditions. J Parasitol (1968) 54:1190–3. doi: 10.2307/3276989
35. Crowther JR. The ELISA guidebook. Methods Mol Biol (2000) 149:III–IV, 1-413. doi: 10.1385/1592590497
36. Venkatas J, Adeleke MA. A review of Eimeria antigen identification for the development of novel anticoccidial vaccines. Parasitol Res (2019) 118:1701–10. doi: 10.1007/s00436-019-06338-2
37. Blake DP, Pastor-Fernández I, Nolan MJ, Tomley FM. Recombinant anticoccidial vaccines - a cup half full? Infect Genet Evol (2017) 55:358–65. doi: 10.1016/j.meegid.2017.10.009
38. Omata Y, Sueda M, Koyama T, Tanabe S, Uzuka Y, Sarashina T, et al. Identification and the role of soluble antigens detected in bile from Eimeria stiedai-infected rabbits. J Parasitol (2001) 87:287–91. doi: 10.1645/0022-3395(2001)087[0287:IATROS]2.0.CO;2
39. Hanada S, Umemoto Y, Omata Y, Koyama T, Nishiyama K, Kobayashi Y, et al. Eimeria stiedai merozoite 49-kDa soluble antigen induces protection against infection. J Parasitol (2003) 89:613–17. doi: 10.1645/0022-3395(2003)089[0613:ESMKSA]2.0.CO;2
40. Abdel Megeed KN, Abuel Ezz NM, Abdel-Rahman EH. Protective effect of Eimeria stiedae coproantigen against hepatic coccidiosis in rabbits. J Egypt Soc Parasitol (2005) 35:581–95.
41. Xiao J, Zheng R, Bai X, Pu J, Chen H, Gu X, et al. Preliminary evaluation of the protective effects of recombinant AMA1 and IMP1 against Eimeria stiedae infection in rabbits. Parasit Vectors (2022) 15:400. doi: 10.1186/s13071-022-05492-4
42. Pu J, Xiao J, Bai X, Chen H, Zheng R, Gu X, et al. Prokaryotic expression of Eimeria magna SAG10 and SAG11 genes and the preliminary evaluation of the effect of the recombinant protein on immune protection in rabbits. Int J Mol Sci (2022) 23:10942. doi: 10.3390/ijms231810942
43. Belli SI, Witcombe D, Wallach MG, Smith NC. Functional genomics of gam56: characterisation of the role of a 56 kilodalton sexual stage antigen in oocyst wall formation in eimeria maxima. Int J Parasitol (2002) 32:1727–37. doi: 10.1016/S0020-7519(02)00212-6
44. Belli SI, Wallach MG, Luxford C, Davies MJ, Smith NC. Roles of tyrosine-rich precursor glycoproteins and dityrosine- and 3,4-dihydroxyphenylalanine-mediated protein cross-linking in development of the oocyst wall in the coccidian parasite eimeria maxima. Eukaryot Cell (2003) 2:456–64. doi: 10.1128/EC.2.3.456-464.2003
45. Wallach M, Smith NC, Petracca M, Miller CM, Eckert J, Braun R. Eimeria maxima gametocyte antigens: potential use in a subunit maternal vaccine against coccidiosis in chickens. Vaccine (1995) 13:347–54. doi: 10.1016/0264-410X(95)98255-9
46. Xu J, Zhang Y, Tao J. Efficacy of a DNA vaccine carrying Eimeria maxima Gam56 antigen gene against coccidiosis in chickens. Korean J Parasitol (2013) 51:147–54. doi: 10.3347/kjp.2013.51.2.147
47. Qiu W, Wernimont A, Tang K, Taylor S, Lunin V, Schapira M, et al. Novel structural and regulatory features of rhoptry secretory kinases in toxoplasma gondii. EMBO J (2009) 28:969–79. doi: 10.1038/emboj.2009.24
48. El Hajj H, Demey E, Poncet J, Lebrun M, Wu B, Galéotti N, et al. The ROP2 family of Toxoplasma gondii rhoptry proteins: proteomic and genomic characterization and molecular modeling. Proteomics (2006) 6:5773–84. doi: 10.1002/pmic.200600187
49. Wang HL, Wang YJ, Pei YJ, Bai JZ, Yin LT, Guo R, et al. DNA Vaccination with a gene encoding Toxoplasma gondii rhoptry protein 17 induces partial protective immunity against lethal challenge in mice. Parasite (2016) 23:4. doi: 10.1051/parasite/2016004
50. Kim WH, Chaudhari AA, Lillehoj HS. Involvement of T cell immunity in avian coccidiosis. Front Immunol (2019) 10:2732. doi: 10.3389/fimmu.2019.02732
51. Lowenthal JW, York JJ, O'Neil TE, Rhodes S, Prowse SJ, Strom DG, et al. In vivo effects of chicken interferon-gamma during infection with eimeria. J Interferon Cytokine Res (1997) 17:551–8. doi: 10.1089/jir.1997.17.551
52. Lillehoj HS, Choi KD. Recombinant chicken interferon-gamma-mediated inhibition of Eimeria tenella development in vitro and reduction of oocyst production and body weight loss following Eimeria acervulina challenge infection. Avian Dis (1998) 42:307–14. doi: 10.2307/1592481
53. Takehara K, Kobayashi K, Ruttanapumma R, Kamikawa M, Nagata T, Yokomizo Y, et al. Adjuvant effect of chicken interferon-gamma for inactivated Salmonella enteritidis antigen. J Vet Med Sci (2003) 65:1337–41. doi: 10.1292/jvms.65.1337
54. Lillehoj HS, Choi KD, Jenkins MC, Vakharia VN, Song KD, Han JY, et al. A recombinant Eimeria protein inducing interferon-gamma production: comparison of different gene expression systems and immunization strategies for vaccination against coccidiosis. Avian Dis (2000) 44:379–89. doi: 10.2307/1592553
55. Ding X, Lillehoj HS, Quiroz MA, Bevensee E, Lillehoj EP. Protective immunity against Eimeria acervulina following in ovo immunization with a recombinant subunit vaccine and cytokine genes. Infect Immun (2004) 72:6939–44. doi: 10.1128/IAI.72.12.6939-6944.2004
56. Song X, Huang X, Yan R, Xu L, Li X. Efficacy of chimeric DNA vaccines encoding Eimeria tenella 5401 and chicken IFN-γ or IL-2 against coccidiosis in chickens. Exp Parasitol (2015) 156:19–25. doi: 10.1016/j.exppara.2015.05.003
57. Iwakura Y, Ishigame H, Saijo S, Nakae S. Functional specialization of interleukin-17 family members. Immunity (2011) 34:149–62. doi: 10.1016/j.immuni.2011.02.012
58. Min W, Kim WH, Lillehoj EP, Lillehoj HS. Recent progress in host immunity to avian coccidiosis: IL-17 family cytokines as sentinels of the intestinal mucosa. Dev Comp Immunol (2013) 41(3):418–28. doi: 10.1016/j.dci.2013.04.003
59. Geriletu, Xu L, Xurihua, Li X. Vaccination of chickens with DNA vaccine expressing Eimeria tenella MZ5-7 against coccidiosis. Vet Parasitol (2011) 177:6–12. doi: 10.1016/j.vetpar.2010.11.041
60. Wallach M. Role of antibody in immunity and control of chicken coccidiosis. Trends Parasitol (2010) 26:382–7. doi: 10.1016/j.pt.2010.04.004
61. Constantinoiu CC, Molloy JB, Jorgensen WK, Coleman GT. Characterization of the antibody response in birds following infection with wild-type and attenuated strains of Eimeria tenella and eimeria necatrix. Vet Parasitol (2011) 175:47–51. doi: 10.1016/j.vetpar.2010.09.025
62. Wang Y, Zhou X, Wang H, Sun L, Wang B, Jiang Y, et al. The role of Eimeria tenella EtCab protein in the attachment and invasion of host cells. Vet Parasitol (2021) 292:109415. doi: 10.1016/j.vetpar.2021.109415
63. Smith NC, Wallach M, Miller CM, Morgenstern R, Braun R, Eckert J. Maternal transmission of immunity to Eimeria maxima: enzyme-linked immunosorbent assay analysis of protective antibodies induced by infection. Infect Immun (1994) 62:1348–57. doi: 10.1128/iai.62.4.1348-1357.1994
64. Ryley JF, Robinson TE. Life cycle studies with Eimeria magna pérard, 1925. Parasitol Res (1976) 50:257–75. doi: 10.1007/BF02462971
65. Tian EJ, Zhou BH, Wang XY, Zhao J, Deng W, Wang HW. Effect of diclazuril on intestinal morphology and SIgA expression in chicken infected with eimeria tenella. Parasitol Res (2014) 113:4057–64. doi: 10.1007/s00436-014-4074-7
66. Jensen JB, Edgar SA. Possible secretory function of the rhoptries of Eimeria magna during penetration of cultured cells. J Parasitol (1976) 62:988–92. doi: 10.2307/3279196
Keywords: Eimeria magna, gametocyte antigen 56, rhoptry kinase family protein 17, recombinant proteins, protective effect
Citation: Xiao J, Chen H, Zheng R, Pu J, Gu X, Xie Y, He R, Xu J, Jing B, Peng X and Yang G (2023) Recombinant GMA56 and ROP17 of Eimeria magna conferred protection against infection by homologous species. Front. Immunol. 13:1037949. doi: 10.3389/fimmu.2022.1037949
Received: 06 September 2022; Accepted: 27 December 2022;
Published: 12 January 2023.
Edited by:
Mohamed A. Dkhil, Helwan University, EgyptReviewed by:
Shawky Mohamed Aboelhadid, Beni-Suef University, EgyptDandan Liu, Yangzhou University, China
Copyright © 2023 Xiao, Chen, Zheng, Pu, Gu, Xie, He, Xu, Jing, Peng and Yang. This is an open-access article distributed under the terms of the Creative Commons Attribution License (CC BY). The use, distribution or reproduction in other forums is permitted, provided the original author(s) and the copyright owner(s) are credited and that the original publication in this journal is cited, in accordance with accepted academic practice. No use, distribution or reproduction is permitted which does not comply with these terms.
*Correspondence: Guangyou Yang, guangyou1963@126.com
†These authors have contributed equally to this work