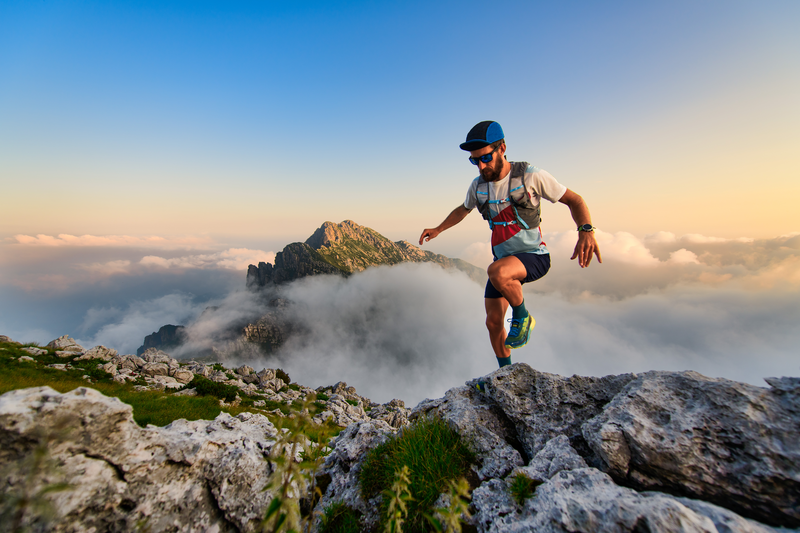
94% of researchers rate our articles as excellent or good
Learn more about the work of our research integrity team to safeguard the quality of each article we publish.
Find out more
ORIGINAL RESEARCH article
Front. Immunol. , 17 November 2022
Sec. Comparative Immunology
Volume 13 - 2022 | https://doi.org/10.3389/fimmu.2022.1037517
This article is part of the Research Topic Novel Strategies and Insights in Aquatic Vaccines View all 6 articles
Immunoglobulin (Ig) M is an important immune effector that protects organisms from a wide variety of pathogens. However, little is known about the immune response of gut mucosal IgM during bacterial invasion. Here, we generated polyclonal antibodies against common carp IgM and developed a model of carp infection with Aeromonas hydrophila via intraperitoneal injection. Our findings indicated that both innate and adaptive immune responses were effectively elicited after A. hydrophila infection. Upon bacterial infection, IgM+ B cells were strongly induced in the gut and head kidney, and bacteria-specific IgM responses were detected in high levels both in the gut mucus and serum. Moreover, our results suggested that IgM responses may vary in different infection strategies. Overall, our findings revealed that the infected common carp exhibited high resistance to this representative enteropathogenic bacterium upon reinfection, suggesting that IgM plays a key role in the defense mechanisms of the gut against bacterial invasion. Significantly, the second injection of A. hydrophila induces strong local mucosal immunity in the gut, which is essential for protection against intestinal pathogens, providing reasonable insights for vaccine preparation.
Teleost fish are an important link in vertebrate evolution and an indispensable component of comparative immunology, which has both innate and adaptive immune systems (1). The adaptive immune system responds to a multitude of antigens and pathogenic microorganisms by producing specific immunoglobulins (Igs), which are more commonly known as antibodies. Igs secreted by B lymphocytes, an important immune effector, play a vital role in protecting fish against various pathogens (2). Igs come in two physical forms: the membrane-bound form located on the surface of B cells (i.e., B cell receptors [BCRs]) and the soluble form secreted by plasma cells (i.e., antibodies) (3). The main functions of BCRs, as antigen-bound receptors, are to bind, internalize, and target antigens and to present the antigenic peptide to helper T cells (4). The soluble form of Igs plays an important role in recognizing and binding to antigens to conduct various immune effector defensive processes, including antigen neutralization, complement activation, monocyte opsonization, and antibody-dependent cell-mediated cytotoxicity (ADCC) (5, 6).
Igs occur in vertebrates from cartilaginous fish to mammals (7). Five classes of Igs (i.e., IgM, IgD, IgG, IgA, and IgE) have been recognized in mammals. In contrast, only three Ig isotypes (i.e., IgM, IgD, and IgT) have been reported in teleost fish (8). IgM is the earliest identified Ig isotype, which exists predominantly as tetramers in serum and mucus (9, 10). Previous studies have shown that IgM concentrations are far higher compared to IgD or IgT in both systemic tissues and mucosal tissues of teleost fish (11, 12). Importantly, IgM has also known to involved in both systemic and mucosal immunity (13, 14). Furthermore, the membrane-bound IgM+ B lymphocytes of teleost fish are important subsets of B cells involved in innate and adaptive immune responses (15). Although IgD is the second Ig isotype discovered in teleost fish, its exact function is yet to be elucidated (16). A previous study demonstrated that IgD may enhance mucosal homeostasis (17). IgT, a recently identified member of the Ig family, is functionally equivalent to the IgA of mammals and is mainly involved in mucosal immunity (18). In addition, many antibodies against IgM have been developed in several teleost fish in recent years, which has greatly contributed to the development of fish Ig research (19–25). Although the IgM heavy chain gene of common carp (Cyprinus carpio) has been cloned (26), studies on IgM function are still lacking, especially in terms of the mucosal response to bacterial infection.
Common carp is among the major freshwater economic species worldwide, especially in China, with a total production of 2.8 million tons in 2021, exceeding one-tenth of the nation’s freshwater fish breeding production (27). Bacterial diseases such as Aeromonas hydrophila, Aeromonas veronii, and Flavobacterium columnare are important factors affecting the health and development of the current carp cultivation industry (28–30). A. hydrophila is a very common bacteria in aquatic environments worldwide (31). This opportunistic zoonotic bacterium can cause hemorrhagic septicemia, abdominal dropsy, and skin ulceration, and is considered the most devastating pathogen of Cyprinoid fish (28, 32). Previous studies have demonstrated that A. hydrophila induces intestinal inflammation in zebrafish and grass carp (33, 34). Moreover, the IgM expression in the intestine of rainbow trout was significantly increased after infection with Streptococcus iniae (35). However, the immune response and function of IgM against A. hydrophila infection in the common carp intestine remains largely unexplored.
To fill the aforementioned knowledge gaps, here we prepared polyclonal antibodies against the common carp IgM. Afterward, we developed a model of common carp infection with A. hydrophila via intraperitoneal injection. In this study, we found that A. hydrophila infection elicited a strong immune response and histopathologic changes in the gut. Notably, bacterial infection induced increases in IgM+ B cells and A. hydrophila-specific IgM titers in mucosal and systemic immunity. More importantly, our results demonstrated that common carp that were reinfected with A. hydrophila recovered faster from the pathological changes in their gut. Furthermore, A. hydrophila-specific IgM titers and survival rates were also higher in the reinfected carp. Therefore, the results in this paper provide crucial insights into the role of IgM in bacterial enteritis of teleost fish.
Completed IgM heavy chains of common carp cDNA sequences were obtained from NCBI (GenBank accession no. MH352354.1) and were synthesized artificially. We predicted the functional domains through the IMGT tool (http://www.imgt.org/3Dstructure-DB/cgi/DomainGapAlign.cgi). The heavy chain constant domains (CH2-CH4, 993bp) were amplified with primers F/R (F:5’-CGCGGATCCGATGTTCGCGCAACCGTT-3’ R:5’-CCGCTGGAGTTACGGTTTACAAAACGCCGG-3’) and 2×Taq Master Mix (Vazyme, China). The PCR products were purified using the FastPure® Gel DNA Extraction Mini Kit (Vazyme, China), digested with restriction enzymes BamH I and Xho I (Takara, Japan), and ligated to the pET-32a vector. Then the constructed plasmid was transformed into Escherichia coli BL21 (DE3) (Vazyme, China), inoculated on Luria-Bertani (LB) solid medium, and cultured at 37°C overnight. The expression and purification of recombinant IgM protein (rIgM) expression were performed by referring to the previous method of Wang et al. with minor modifications (36). Positive clones on the plates were then selected and inoculated in a 500 ml LB liquid medium (with 100 μg/ml ampicillin), then cultured at 37°C with shaking at 220 rpm. When the medium reached approximately 0.6 at OD600nm, isopropyl-β-d-thiogalactopyranoside (IPTG; Roche, Switzerland) was added at a final concentration of 1 mM and incubated at 28°C for 3.5 h. The rIgM was purified according to the manufacturer’s instructions by a nickel-nitrilotriacetic acid column (Ni-NTA; Qiagen, Germany). 400 μg purified rIgM and Freund’s Complete Adjuvant (FCA; Sigma, USA) were fully mixed in equal proportions and then used for injection immunization of Japanese white rabbits (2-3 months old). The rabbits were booster-immunized with 150 μg of purified rIgM mixed with Freund’s Incomplete Adjuvant (FIA; Sigma, USA) four times. After immunization, blood was collected from rabbits, and IgG was purified from rabbit serum with protein A agarose (Thermo Fisher Scientific, USA). Then we purified the obtained rabbit IgG by affinity chromatography to obtain the anti-carp IgM antibody. Briefly, the affinity column was prepared by coupling rIgM to CNBr-activated Sepharose 4B according to the manufacturer’s instructions (GE Healthcare, USA). For isolation anti-carp IgM pAb, the purified rabbit IgG sample was applied to the column equilibrated in 1× phosphate buffered saline (PBS, pH 7.2). Then incubate in the shaker for 2 h. After several washes of affinity column with PBS, bound IgM were eluted with 0.1 M glycine (pH 2.5), and immediately neutralized with 1 M Tris (pH 9.0). The neutralized antibody was displaced into PBS using a PD-10 Desalting Columns (GE Healthcare, USA) according to the manufacturer’s instructions and stored at -80°C. The specificity of the polyclonal antibody against common carp IgM was detected by western blot and immunofluorescence.
The 10-15 g common carp used for this experiment were obtained from a fish farm in Chongqing, China, and placed in an aquarium containing a recirculating aquaculture system. Fish were fed with 153 commercial fish floating feed pellets (Tongwei Group, China) twice per day and acclimated at 28°C for at least 2 weeks. The feeding was terminated 48 h before injection and sampling. Animal procedures were approved by the Animal Experiment Committee of Institute of Hydrobiology, Chinese Academy of Sciences and carried out according to the relative guidelines.
A. hydrophila strain AH1 was obtained from the Laboratory of Aquatic Animal Medicine of the College of Fisheries at Huazhong Agricultural University (Hubei, China). The AH1 strain was streaked consecutively on LB solid medium and cultured at 28°C for 24 hours. Then, single colonies were picked and inoculated into LB liquid medium and cultured at 28°C for 12 h with 200rpm shaking. A. hydrophila suspension was centrifuged at 5000 g for 10 min, bacteria were resuspended with PBS and the concentration was adjusted to 1.6 × 107 CFU/ml for challenge use. We designed two types of challenge experiments. The first was an infection of common carp by intraperitoneal injection with 100 μl of A. hydrophila (1.6 × 107 CFU/ml), tissues (foregut, midgut, hindgut, spleen, and head kidney) from at least six fish were collected at 0.5, 1, 2, 4, 7, 14, 28 days post-infection (DPI), respectively, and fluids (serum and gut mucus) were taken on 28 DPI (Figure 2A). For the second type of challenge, a second intraperitoneal injection was performed with the same dose of A. hydrophila on day 28 after the first infection. Tissues, serum, and gut mucus were collected at 28 (28 DPI surviving group, [28DPI-S], before the second challenge) and 35 (35 DPI surviving group, [35DPI-S]) days post-primary infection. As the control group, the fish were injected with 100 μl of PBS. Tissue samples were used to evaluate morphological change and measure the expression of the immune-related genes, and serum and gut mucus were used to detect the changes in the IgM by western blot.
For common carp sampling, fish were anesthetized with MS-222. The blood was taken from the tail vein, centrifuged at 5000 g for 10 min to remove the plasma, collected the serum, and immediately stored at -80°C before use. To collect skin mucus, the mucus was gently scraped from the surface of common carp skin and then placed in a sterile Petri dish as previously described (37). To collect gut mucus, the gut of common carp was opened longitudinally, and the incubation buffer (1 ×PBS, containing 1 × protease inhibitor cocktail [Roche, Switzerland], 1mM phenylmethylsulfonyl fluoride [Sigma, Germany]) was added at a ratio of 4000 μl/g to the surface of the gut and then the surface mucus was gently scraped with a cell scraper to a sterile Petri dish. The sampling locations of foregut, midgut, and hindgut were shown in Supplementary Figure 2B. To obtain gill, buccal, nasal, and pharyngeal mucus, perfusion was performed using PBS to remove blood present in the tissue, and after dissection and removal of the tissue, it was rinsed three times with PBS to remove the remaining blood, added to the protease inhibition buffer described above (gill, added at a ratio of 2000 μl/g; buccal, added at a ratio of 10,000 μl/g; nasal, added at a ratio of 10,000 μl/g; pharyngeal, added at a ratio of 4000 μl/g), placed in sterile Petri dishes, and incubated overnight with gentle shaking at 4°C as previously described (38–41). After the tissue was gently blown, the culture supernatant was collected. All mucus suspensions were then collected into Eppendorf tubes and centrifuged at 400 g for 10 min at 4°C to remove existing the carp cells and debris. To remove bacteria from the mucus, the cell-free mucus was further centrifuged at 10,000 g for 10 min at 4°C and the mucus suspension was then stored at -80°C before use.
Mucus and serum samples were resolved on 4-15% SDS-PAGE Ready Gel (Bio-Rad, USA). PVDF membranes (Bio-Rad, USA) were first activated with methanol and the gels were transferred onto PVDF membranes for western blot analysis. PVDF membranes were blocked with 8% skim milk after the transfer and then incubated with anti-carp IgM (0.2 μg/ml) antibody. As isotype, the rabbit IgG (purified from rabbit blood before immunization) was used at the same concentrations. After washing four times, added HRP Goat Anti-Rabbit IgG (H+L) (ABclonal, China) and incubated for 45 min at RT. After washing four times, the ECL Substrate (Epizyme, China) was incubated with PVDF membranes. For quantitative analysis of IgM in serum and gut mucus, the PVDF membranes were scanned under the Amersham Imager 800 Imaging System (GE Healthcare, USA), and the signal intensity of each band was analyzed with ImageQuant TL software (GE Healthcare, USA). Thereafter, the concentration of IgM was determined by plotting the obtained signal strength values on a standard curve generated for each blot using known amounts of purified carp IgM. For purification of native IgM in common carp serum, the same procedure was used as for the purification of pAb as described above. Briefly, the affinity column was prepared by coupling anti-carp IgM pAb to CNBr-activated Sepharose 4B (GE Healthcare, USA). Then a serum sample pooled from several individual carp was diluted 1:2 in PBS (pH 7.2) was applied to the column equilibrated in PBS. The bound IgM was eluted in the same manner, and the concentration was determined using the Micro BCA™ Protein Assay Kit according to the manufacturer’s instructions (Thermo Fisher Scientific, USA).
As for histological studies, hematoxylin-eosin (H&E) staining was performed according to the previous method of Kong et al. (41). Images were captured for at least 6 individuals using a microscope, and then the length and width of at least 3 gut villi per image were measured using the measurement tool of cellSens Standard software (Olympus, Japan). IgM+ B cells were detected according to the method previously described by Yu et al. with minor modifications (40). Briefly, sections were subjected to antigen retrieval using EDTA-2Na, followed by incubation with rabbit anti-carp IgM pAb (1.5 μg/ml) overnight at 4°C. As isotype, the rabbit IgG (purified from rabbit blood before immunization) was used at the same concentrations. After washing four times with PBS, sections were stained with Goat anti-Rabbit IgG (H+L) Highly Cross-Adsorbed Secondary Antibody, Alexa Fluor™ Plus 488 (Invitrogen, USA) at 3.3 μg/ml each for 40 min at RT. After washing four times with PBS, all sections were stained with 1 μg/ml DAPI (4’, 6-diamidino-2-phenylindole; Invitrogen, USA) for 8 min at RT before mounting. All images were then captured using an Olympus BX53 fluorescence microscope (Olympus, Japan) and analyzed by cellSens Standard imaging processing software (Olympus, Japan).
DNA was extracted by using the DNeasy® Blood & Tissue Kit (QIAGEN, German) according to the manufacturer’s protocol. Briefly, the tissues were cut up and mixed with 180 μl ATL and 20 μl proteinase K. The tissues were incubated at 56°C until they were completely lysed. Add 200 μl AL and incubate at 56°C for 10 min and then add 200 μl anhydrous ethanol. Transfer supernatant to DNeasy Mini spin column and centrifuge at 7000 g for 1 min. After washing the hybrids with AW1 and AW2, elution was performed with Buffer AE, collected the eluted DNA. The concentration of DNA was determined using a Nanodrop 2000 spectrophotometer (Thermo Fisher Scientific, USA). To test bacterial loads, we selected Act virulence genes from A. hydrophila for qPCR. Total RNA was extracted by homogenization in 1 ml TRIzol (Invitrogen, USA) using steel beads and shaking (60 HZ for 1 min; WONBIO, China) following the manufacturer’s instructions. Extracted RNA was measured by spectrophotometer for concentration and checked for integrity with agarose gel (Monad, China). To eliminate differences in gene expression levels for each sample, RNA was reverse transcribed to cDNA and then analyzed by qPCR according to the method of Yu et al. (40). The relative mRNA levels of IgM in different tissues were calculated by taking the reciprocal of the value of IgM/40S. The relative fold changes were calculated using the 2-ΔΔCt method, and 40S was used as a control gene for normalization of expression. The specific primers used in this experiment are listed in Supplementary Table 1.
Using the pull-down assay as described previously (42, 43), we measured the titers of A. hydrophila-specific IgM in foregut mucus, midgut mucus, hindgut mucus, and serum. Briefly, the suspension of A. hydrophila cultured to OD600nm = 0.7 was washed three times with PBS. Then 40 μl of bacterial suspension were added to the incubation system, and the mucus was diluted at 1:2, 1:10, 1:40, 1:100, and the serum was diluted at 1:10, 1:100, 1:1000, 1:4000, respectively, and the system was supplemented to 300 μl with 1% BSA-PBS. After incubation at RT for 4 h or at 4°C for 12h, the supernatant was discarded by centrifugation at 10,000 g for 5 min and the sediment was washed three times with PBS. Add 2 × Laemmli Sample Buffer (Bio-Rad, USA) to elute bound proteins and mix well, boil at 95°C for 8 min. The prepared samples were separated by SDS-PAGE and then detected for the presence of IgM using western blot and the polyclonal antibody against common carp IgM as described above.
The data were shown as the mean ± SEM and normality and homogeneity of variance were checked before the statistical analysis. The statistical differences between groups were analyzed using an unpaired Student’s t-test, one-way analysis of variance (ANOVA) with Bonferroni correction, and log-rank (Mantel-Cox) test (Prism version 6.01; GraphPad). A value of p < 0.05 or less was considered statistically significant.
To prepare polyclonal antibodies against common carp IgM, we first obtained the complete cDNA sequence of IgM heavy chain from NCBI (GenBank accession: no. MH352354.1) and cloned the CH2-CH4 domains into the prokaryotic expression plasmid (Figure 1A). Then we purified and identified the soluble expression products. As we expected, the purified recombinant proteins exhibited the main band with molecular weights of ~56 kilodaltons (kDa) (Figure 1B). The rIgM was then used as an immunogen to develop against common carp IgM antibody. The rabbit polyclonal antibody (pAb) against common carp IgM was analyzed by western blot using common carp serum dilution. The results showed significant bands at ~800 kDa and ~75 kDa under non-reducing and reducing conditions, respectively, and no bands for the antibody (rabbit IgG) of the isotype control (Figure 1C). By immunofluorescence assay, we found that green fluorescent signals were observed on histological sections from the skin, gill, foregut, midgut, hindgut, buccal cavity, pharynx, nose, spleen, and head kidney of the healthy common carp, and isotype control was negative, which suggested that the pAb could specifically react with membrane-bound IgM-positive B lymphocytes (Figure 1D; Supplementary Figure 1A).
Figure 1 The common carp IgM polyclonal antibody (pAb) production and characterization. (A) Structural schematic of pET-32a-IgM recombinant plasmids. (B) Coomassie blue staining of recombinant protein of IgM resolved by SDS-PAGE. Red arrowheads indicate the recombinant IgM. (C) Western blot analysis with pAb to common carp serum. Left margin, molecular size in kilodaltons (kDa). MK, marker; NR, nonreducing conditions; RE, reducing conditions. (D) Immunofluorescence staining of IgM+ B cells in different tissues of common carp. Tissue paraffinic sections were stained with rabbit anti-carp IgM (green) and nuclei were stained with DAPI (blue) (isotype-matched control antibody staining, Figure S1A). White arrowheads point to cells stained for IgM. White dotted lines outline the border. Scale bar, 20 μm. EP, epidermis; DE, dermis; PL, primary lamella; SL, secondary lamella; LU, lumen; LP, lamina propria; BC, buccal cavity; BE, buccal epithelium; PC, pharyngeal cavity; PE, pharyngeal epithelium; NC, nasal cavity; OE, olfactory epithelium.
We also investigated the expression pattern of igm in healthy common carp by qPCR. As shown that igm expression was found in all examined tissues and measured at higher levels in the systemic tissues (i.e., head kidney and spleen) than in the mucosal tissues (i.e., skin, gill, foregut, midgut, hindgut, buccal cavity, pharynx, and nose) (Supplementary Figure 1B). In addition, the concentrations of IgM in serum and mucus were examined at the protein level in this study, and we found that the concentration of IgM in mucus was much lower than in serum (Supplementary Figure 1C). Together, these data indicated that the carp IgM pAb herein was workable and could be used for western blot and immunofluorescence analysis.
To evaluate the common carp immune responses to bacterial pathogenic challenges, we developed a common carp bacterial infection model via intraperitoneal injection of A. hydrophila (Figure 2A). In the infected group, typical symptoms of A. hydrophila appeared, such as ascites, anal redness and swelling, body congesting, and enteritis (Figure 2B). In addition, we found that the walls of the foregut, midgut, and hindgut were congested (Supplementary Figure 2A). Using H&E staining, we observed severe pathological changes in the gut of common carp after A. hydrophila infection, including gut villus damage as well as a decrease in the gut villus aspect ratio (Figures 2C–E). The aspect ratio of gut villi reduced significantly at 0.5 DPI and gradually recovered to the normal level until 28 d (Figure 2F, G). Notably, the hindgut recovery was faster than foregut and midgut, with no significant difference in the infected group compared with control fish at 7 DPI (Figure 2H). Together, our results confirmed that the A. hydrophila infection model was successful in the common carp.
Figure 2 Scheme of the infection strategy with A. hydrophila via intraperitoneal injection. (A) Fish were injected with A. hydrophila and sacrificed at 0.5, 1, 2, 4, 7, 14, and 28 DPI for sample collection, while fish were injected with PBS as control. (B) The clinical observation following challenge with A. hydrophila. (C–E) Histological examination by H&E staining of foregut (C), midgut (D), and hindgut (E) from control and infected fish (n = 6). Scale bars, 50 μm. (F–H) The length-width ratio of foregut (F), midgut (G), and hindgut (H) intestinal folds in these groups of fish from (C–E) (n = 6). *P < 0.05, **P < 0.01, and ***P < 0.001 (one-way ANOVA with Bonferroni correction). Data are representative of at least three independent experiments (mean ± SEM).
To evaluate the kinetics of immune responses in common carp post A. hydrophila infection, we measured the expression levels of 13 immune-related genes in the foregut, midgut, hindgut, spleen, and head kidney tissues of common carp at 0.5, 1, 2, 4, 7, 14, and 28 DPI by qPCR, which including antimicrobial peptides (AMPs) genes (hepcidin; NK-lysin 2, nkl2; Apolipoproteins A-1, apoa-1; and Apolipoproteins A-14, apoa-14), inflammatory genes (inflammatory 1-β, il1-β; inflammatory 6, il6; inflammatory 8, il8; and inflammatory 2, il2), matrix metalloproteinase 9 (mmp-9), and immunoglobulin (Ig) heavy chain genes (igm, igd, igt1, and igt2). In this study, we found that most of the genes increased significantly in foregut, midgut, hindgut, spleen, and head kidney tissues after A. hydrophila infection (Figures 3A–E). As AMPs, the expression of hepcidin and nkl2 were upregulated significantly at 0.5 DPI. Interestingly, the apoa-1 and apoa-14 genes decreased significantly at first (0.5 and 1 DPI) and then increased significantly. The higher expression levels of proinflammatory cytokines, such as il1-β, il6, and il8, were also expressed at 0.5 and 1 DPI. However, the inflammatory cytokine il2 was significantly upregulated at the initial phase of inflammation in common carp gut, as well as at the later phase of tissue remodeling (Figures 3A–C). It is worth noting that the genes of igm and igt1 were expressed earlier than igd and igt2 in the spleen and head kidney (Figures 3D, E), and the up-regulation of igm expression can persist up to 14 days after A. hydrophila infection. In addition, the expression of igm and igt2 were upregulated in the foregut, midgut, and hindgut post the second infection (Supplementary Figure 3). Taken together, these data supported a strong innate and adaptive immune response were generated in systemic and mucosal tissues following intraperitoneal infection with A. hydrophila in common carp.
Figure 3 Immune response in gut, spleen, and head kidney tissues of common carp following A. hydrophila infection. (A–E) Heat map illustrates results from qPCR of mRNAs for selected immune-related genes in bacteria-challenged fish vs. control group measured at 0.5, 1, 2, 4, 7, 14, and 28 DPI following with A. hydrophila in the foregut (A), midgut (B), hindgut (C), spleen (D), and head kidney (E) organs of common carp (n = 6). Color value: log2 (fold change). Data are representative of at least three different independent experiments.
To evaluate the adaptive IgM+ B cells responses, we performed a second intraperitoneal challenge of common carp using the same dose of A. hydrophila as the first at 28 DPI (Figure 4A). Using immunofluorescence microscopy, the results showed a significant increase in the number of IgM+ B cells in the foregut, midgut, and hindgut of 28DPI-S fish, which were ∼2.1-, ∼2.1-, and ∼2.0-fold higher compared to the control fish (Figures 4B–D, G–I). And we detected a moderate increase of IgM+ B cells in the head kidney of the 28DPI-S fish, which was ∼1.5-fold higher when compared to control group (Figure 4F, K). Notably, 35DPI-S fish showed significantly increased numbers of IgM+ B cells in the foregut, midgut, hindgut, and head kidney, which were ∼2.5-, ∼2.7-, ∼2.5-, and ∼1.8-fold, respectively, when compared to the control fish (Figures 4G–I, K). However, the trend of IgM+ B cells in the spleen was not significant compared to the control fish (Figure 4E, J).
Figure 4 Scheme of the reinfection strategy and accumulation of IgM+ B cells of common carp infected with A. hydrophila. (A) Scheme of the rechallenge strategy with A. hydrophila via intraperitoneal injection. Fish were injected with A. hydrophila at 0 days and reinfected at 28 DPI with the same bacterial dose, and the resulting surviving fish were sacrificed at 28 and 35 days after the primary infection (28 DPI surviving group, [28DPI-S]) and 35 (35 DPI surviving group, [35DPI-S]). The three groups of fish include Control (PBS + PBS), Con+Cha (PBS + A. hydrophila), and Infe+Cha (A. hydrophila + A. hydrophila). (B–F) Representative differential interference contrast (DIC) images of immunofluorescence staining on common carp foregut (B), midgut (C), hindgut (D), spleen (E), and head kidney (F) paraffin-sections from uninfected (Control) fish, 28DPI-S, and 35DPI-S fish, stained for IgM (green) (Isotype-matched control antibody staining is shown in Figure S1A); nuclei (blue) were stained with DAPI (blue). Scale bars, 20 μm. (G–K) The number of IgM+ B cells of Control, 28DPI-S, and 35DPI-S fish counted from (B–F) (n = 6). ns, not significant, *P < 0.05, **P < 0.01, and ***P < 0.001 (unpaired Student’s t-test). Data are representative of at least three independent experiments (mean ± SEM).
To further study the gut bacteria-specific IgM responses in common carp after A. hydrophila infection, we performed a western blot analysis. Here, the results showed that the concentration of IgM in the foregut and midgut mucus of the 28DPI-S fish increased moderately and significantly (Figures 5A, B). The protein level of IgM in the foregut mucus, midgut mucus, and hindgut mucus of 35DPI-S fish was increased significantly, which was ∼3.2-, ∼1.9-, and ∼2.8- fold higher than that of the control fish, respectively (Figures 5A–C). In serum, the concentrations of IgM increased ∼3.0-fold and ∼2.4-fold in 28DPI-S and 35DPI-S fish, respectively (Figure 5D). The increase in the level of IgM protein led us to hypothesize that IgM plays an important role in the defense against bacterial invasion of the organism. Next, we verified the above hypothesis using a pull-down assay to detect the ability of IgM to bind to A. hydrophilic in serum and gut mucus. Immunoblot analysis showed that bacteria-specific IgM binding in a 1:2 midgut mucus (∼2.1-fold) and hindgut mucus (∼3.5-fold) dilution from 28DPI-S fish and up to 1:100 in dilutions of midgut mucus and hindgut mucus in 35DPI-S fish, an increase of ∼2.8-fold and ∼2.1-fold, respectively, compared to control fish (Figures 5F, G, J, K). We found that bacteria-specific IgM binding was detected at 1:10 (∼2.2-fold) foregut mucus dilution in the 28DPI-S fish, in contrast to midgut and hindgut results where the binding was detected only at 1:40 (∼3.1-fold) foregut mucus dilution in the 35DPI-S fish (Figures 5E, I). In addition, there was a significant increase in binding of bacteria-specific IgM in the diluted serum of the 28DPI-S fish and 35DPI-S fish compared with control fish, reaching 1:1000 (∼1.8-fold) and 1:4000 (∼2.8-fold), respectively (Figures 5H, L). In a word, these results indicated that IgM plays an important role in the gut tissue against bacterial invasion.
Figure 5 IgM responses in the gut mucus and serum from 28DPI-S and 35DPI-S fish. (A–D) The concentration of IgM protein in foregut mucus (A), midgut mucus (B), hindgut mucus (C), and serum (D) of Control, 28DPI-S, and 35DPI-S fish (n = 6). (E–H) Western blot analysis of IgM-specific binding to A. hydrophila in foregut mucus (E), midgut mucus (F), hindgut mucus (G), and serum (H) (mucus dilution 1:2; serum dilution 1:10) from 28DPI-S and 35DPI-S fish. (I–L) IgM-specific binding to A. hydrophila in dilutions of foregut mucus (I), midgut mucus (J), hindgut mucus (K), and serum (L) from 28DPI-S and 35DPI-S fish, evaluated by densitometric analysis of immunoblots and presented as relative values to those of control fish (n = 6). ns, not significant, *P < 0.05, **P < 0.01, and ***P < 0.001 (unpaired Student’s t-test). Data are representative of at least three independent experiments (mean ± SEM).
In reinfection model, common carp were euthanized at 35 DPI to evaluate pathological changes in the gut tissues and bacterial loads in the foregut, midgut, hindgut, spleen, and head kidney tissues from the three groups (Control, Con+Cha, Infe+Cha) (Figures 6A–E). Using H&E staining, we found that the aspect ratio of gut villi was not significantly different between control fish and reinfected-7d fish (Figure 6D). By qPCR, we detected that upon reinfection, the reinfected fish had markedly lower cytotoxic enterotoxin (Act) expression of A. hydrophila compared with those of the first infected fish (Figure 6E, Supplementary Figure 4). Importantly, we found a higher survival rate in the Infe+Cha group compared with the Con+Cha group (Figure 6F). These data strongly suggested that pre-infection with A. hydrophila protects common carp from the bacterial reinfection, and mucosal adaptive immunity had been induced in common carp against bacteria invasion.
Figure 6 Histological examination, bacterial loads, and survival rates of common carp after rechallenging with A. hydrophila. (A–C) Histological examination by H&E staining of foregut (A), midgut (B), and hindgut (C) from Control (Con-7d), Con+Cha (Infe-7d), and Infe+Cha (Reinfe-7d) groups of fish. (D) The length-width ratio of foregut, midgut, and hindgut intestinal folds from (A–C). (E) The expression levels of A. hydrophila in control, infected-7d, and reinfected-7d fish were measured in the gut of common carp (n = 6). (F) Cumulative survival of control, infected, and reinfected fish. Statistical differences were evaluated by log-rank (Mantel-Cox) test. ns, not significant, *P < 0.05, **P < 0.01, and ***P < 0.001 (one-way ANOVA with Bonferroni correction). Data are representative of at least three independent experiments (mean ± SEM).
Fish live in complex aqueous environments and are therefore uniquely susceptible to diseases caused by a variety of pathogens (44). In response to these threats, teleost fish have evolved robust innate and adaptive immune systems, thus ensuring an appropriate immune response upon antigen challenge while maintaining overall tissue and microbiota homeostasis (45–47). Igs are important effector molecules of the adaptive immune system in vertebrates, which play critical roles in defending the host against various pathogens (48). In teleost fish, IgM is the most abundant Ig isotype and plays a crucial role in both systemic and mucosal tissues (13, 14). The gut of teleost fish represents the prevalent mucosa-associated lymphoid tissue (MALT), directly interacts with foreign pathogens from aquatic environment, and is often considered the major site of invasion (49). A. hydrophila is a highly infectious enteropathogenic bacterium commonly found in freshwater, which causes intestinal inflammation and hemorrhagic septicemia, resulting in considerable economic losses (50). However, the IgM function in the development of bacterial enteritis in teleost fish is relatively little known. Here, we generated polyclonal antibodies against common carp IgM to investigate the function of gut IgM in protecting against enteropathogenic bacterial invasion.
Antibodies against IgM have been reported in much teleost fish (19–25). In this study, the rIgM was employed as an immunogen to generate polyclonal antibodies. Under reducing conditions, the results showed that the molecular weight of rIgM was approximately 56 kDa, indicating that we successfully expressed the rIgM protein in E. coli. Generally, IgM occurs as tetramers with a molecular weight of approximately 800 kDa in the teleost fish, and its monomer consists of two heavy chains (~75 kDa) and two light chains (~25 kDa) (19, 24, 51–53). In our study, western blot analysis revealed that the polyclonal antibody (pAb) against carp IgM specifically reacted with the band corresponding to the heavy chain (~75 kDa) as expected, and IgM predominantly occurred as tetramers (~800 kDa) in common carp serum, which is consistent with the previous study (54). Moreover, the immunofluorescence results indicated that the IgM pAb could specifically recognize the IgM+ B cells of common carp. Notably, the qPCR and immunoblotting results showed that the expression and concentration of IgM in mucosal tissues were lower than those in systemic tissues, which was consistent with previous results (25, 37, 38, 40, 55). Overall, these findings indicated that the IgM pAb prepared herein could be used as a monitoring tool to assess the immune status of common carp.
To evaluate the immune responses of IgM in the gut against bacterial invasion, we established an A. hydrophila infection model in common carp via intraperitoneal injection. As expected, the fish exhibited the typical symptoms of A. hydrophila infection, including body surface hemorrhage, abdominal dropsy, and enteritis (28). Moreover, severe histological changes occurred in the gut of common carp upon infection, including gut villi shedding and lower aspect ratio of the villus structure, which was consistent with the known symptoms of A. hydrophila-infected grass carp (32). Notably, we found that the most serious symptoms occurred at 0.5-1 DPI, after which they gradually improved, indicating that A. hydrophila is an acute infectious gastroenteritis type bacterium. Overall, our results demonstrated that A. hydrophila successfully induces intestinal inflammation via intraperitoneal injection.
Innate immunity serves as the first line of defense against pathogen invasion. Upon A. hydrophila infection, consistent with the gut significant histopathological lesions, the results showed that six immune-related genes were significantly up-regulated in the foregut, midgut, hindgut, spleen, and head kidney, as early as 0.5 DPI. Hepcidin, an important antimicrobial peptide (AMP), plays a crucial role in host defense against bacterial invasion (56). Here, we found that the mRNA expression levels of hepcidin were quickly up-regulated in the foregut, midgut, hindgut, spleen, and head kidney post A. hydrophila infection. This demonstrated that hepcidin may be an important component of the innate immunity of carp and participates in mucosal and systemic immune responses against bacterial infection. NK-lysin is a potent antimicrobial peptide that is widely distributed in vertebrates (57). Upon A. hydrophila infection, the nkl2 gene was significantly activated, suggesting that Nkl2 plays an important role in the immune response against microbial pathogens of common carp. Matrix metalloproteinase 9 (Mmp-9), a member of the zinc-dependent endopeptidase family, is associated with vital inflammatory processes in mammals (58). At the beginning of infection, significant up-regulation of mmp-9 expression was detected in the foregut, midgut, hindgut, spleen, and head kidney, suggesting the important role of Mmp-9 in the innate immune response. Moreover, the mRNA expression of proinflammatory cytokines such as il1-β, il6, and il8 was also significantly increased in the foregut, midgut, and hindgut of common carp immediately after A. hydrophila infection. The results were similarly reported in zebrafish infected with Staphylococcus aureus and in common carp with SMB-induced enteritis (59, 60). Apolipoprotein A (Apo A) is a multifunctional protein that participates in lipid metabolism and transport. A previous study demonstrated that Apo A-1 has bacteriostatic activity against A. hydrophila and bactericidal activity against E. coli (61). Interestingly, apoa-1 and apoa-14 were first downregulated and then upregulated during the infection process, which was consistent with the findings of a previous study in carp infected with SVCV (62). The expression of apoa-1 and apoa-14 were upregulated in the foregut, midgut, and hindgut of common carp post the second infection. Overall, our results suggested that Apo A plays an important role in the resistance to invasion by A. hydrophila.
Similar to mammals, adaptive immunity in teleost fish plays a vital role in preventing bacterial infection (42, 63). Previous studies have shown that IL2 has regulatory effects on immune gene expression and may be involved in the differentiation of Treg and Th1/2 cells, in addition to enhancing NK cell activity (64, 65). Interestingly, il2 upregulation occurred both at the early and late stages in the gut, indicating that IL2 plays an indispensable role in both innate and adaptive immunity in fish mucosal tissues. Igs are crucial components of adaptive immunity, and the expression of igm, igd, and igt was up-regulated in the foregut, midgut, hindgut, spleen, and head kidney tissues in this study. Interestingly, although the igm responses in the spleen and head kidney were activated earlier than in gut tissues, up-regulation of igm expression was also observed in the gut during the later phase of tissue remodeling. Meanwhile, upregulation of igm expression occurred in the foregut, midgut, hindgut, spleen, and head kidney post the second infection, which suggested that the IgM plays a vital role in adaptive immunity. Taken together, our results showed that both innate and adaptive immune responses could be effectively elicited after A. hydrophila infection.
A previous study showed that the expression of igm increased dramatically in gut of rainbow trout to which Flavobacterium psychrophilum was administered via intraperitoneal injection, and IgM was a main participant in the adaptive immune response to this pathogen challenge (66). Similar to mammals, the adaptive immune system of teleost fish also possesses immune memory, which produces a rapid and strong immune response upon pathogen reinfection (67). Thus, we developed a reinfection model, which demonstrated the induction of adaptive immune responses in the gut. In brief, IgM+ B cells were significantly increased in the foregut, midgut, hindgut, and head kidney of the 28DPI-S fish and 35DPI-S fish compared to the control fish. Notably, the accumulation of IgM+ B cells was also increased in the spleen, albeit not significantly, which may be due to the migration of cells into the gut from the spleen (68, 69). A large number of IgM+ B cells were detected in the gut of 35DPI-S fish, which coincided with increases in IgM concentration in the gut mucus of the same individuals. Higher bacteria-specific IgM titers were found in the gut mucus and serum in the 35DPI-S fish when compared to the 28DPI-S fish, indicating that IgM plays an important role in both systemic and mucosal immunity. Similarly, a study performed by Castro et al. showed that IgM+ B cells increased significantly in the peritoneal cavity of rainbow trout after injection with E. coli and viral hemorrhagic septicemia virus (VHSV), and these cells increased their IgM secretion (70). In contrast, bacteria-specific IgM titers were not found in the mucosal tissues (i.e., skin, gill, and buccal cavity) of rainbow trout after F. columnare infection by immersion in our previous studies (42, 43, 71). In another study that examined the GALTs of rainbow trout that had survived Ceratomyxa shasta infection via immersion, parasite-specific IgM responses were only detected in serum samples (18). Based on these observations, we hypothesized that IgM responses vary in an infection strategy-specific manner. Furthermore, we found that the expression of igt was stronger in the gut than in the spleen and head kidney after bacterial infection, especially after the second infection, implying that IgT plays an important role in the mucosa to defend against A. hydrophila infection. This finding is in agreement with the results of Zhang et al. and also applies to the skin, gill, and oral mucosal tissues, and in later work, we will develop antibodies against common carp IgT for further validation (18, 42, 43, 71).
More importantly, our previous studies demonstrated that the survivor rainbow trout re-exposed to this bacterium or virus exhibited high resistance (42, 72). In this study, we found that common carp reinfected with A. hydrophila exhibited higher survival rates and the pathological changes in the gut recovered faster compared to those in individuals infected for the first time. These findings suggested that local humoral immunity might be elicited in carp GALT thus contributing to protection upon reinfection. After rechallenging with A. hydrophila, based on the significant decrease in bacterial load, we hypothesized that IgM+ B cells secrete A. hydrophila-specific IgM to neutralize the bacteria, thereby preventing the pathogens from replicating and multiplying in the target cells. However, further studies are required to explain the mechanisms through which IgM neutralizes pathogens. Moreover, previous studies have demonstrated that A. hydrophila has developed resistance to commercial antibiotics, thus highlighting the importance of vaccines as an effective prevention measure (50). Injection is the most common route of vaccine administration, and therefore it is important to determine whether local mucosal immunity in the gut can be induced by injection. Previous studies hypothesized that the immune responses induced by mucosal (gut) and parenteral immunization are asymmetrical (73). In a previous study, when antigens were delivered via the gut, mucosal and systemic immune responses were elicited, as demonstrated by an increase in the amounts of circulating IgM (74). In contrast, delivering the dinitrophenol (a model antigen) by intraperitoneal injection resulted in strong systemic responses, whereas mucosal responses were virtually absent (75). However, our results demonstrated that intraperitoneal injection with A. hydrophila elicited a strong mucosal and systemic immune response in common carp. In other words, extraintestinal exposure to enteropathogenic bacteria triggered both immune responses equally in our infection model. Therefore, the above-described results suggested that IgM+ B cell and IgM responses may vary in a pathogen-specific manner. From a practical perspective, further research on the pathogen-specific responses of IgM in fish mucosa would greatly contribute to improving delivery strategies and adjuvant formulations. In turn, this would enable the creation of more effective vaccines to induce the generation of specific antibodies in the plasma cells of fish.
In conclusion, our study successfully developed a pAb against common carp IgM, which can be used as an effective tool for vaccine evaluation. Moreover, we constructed a model of carp infected with A. hydrophila via intraperitoneal injection, which can cause strong systemic and mucosal immunity. Importantly, our results demonstrated that reinfected common carp had a lower bacterial load, higher survival rates and higher bacteria-specific IgM titers compared to the common carp that had only been infected once. Therefore, IgM memory B cells may resist the invasion of A. hydrophila by being rapidly and massively activated and exerting various functions. Furthermore, our results suggest that IgM immune responses could vary in different infection strategies. Nevertheless, additional studies are required to elucidate the mechanisms through which IgM participates in the response against bacterial invasion in common carp.
The original contributions presented in the study are included in the article/Supplementary Material. Further inquiries can be directed to the corresponding author.
The animal study was reviewed and approved by the Animal Experiment Committee of Institute of Hydrobiology, Chinese Academy of Sciences.
QM and ZD performed most of the experiments and wrote the manuscript. QM analyzed the data. WK designed infection model and revised manuscript. XW and JY helped with most of the experiments. WJ, JS, and ZX designed the experiments and revised the manuscript. All authors contributed to the article and approved the submitted version.
This work was supported by grants from the National Natural Science Foundation of China (32225050, 32073001, and 31873045) and a grant from the State Key Laboratory of Freshwater Ecology and Biotechnology (2022FB13).
The authors declare that the research was conducted in the absence of any commercial or financial relationships that could be construed as a potential conflict of interest.
All claims expressed in this article are solely those of the authors and do not necessarily represent those of their affiliated organizations, or those of the publisher, the editors and the reviewers. Any product that may be evaluated in this article, or claim that may be made by its manufacturer, is not guaranteed or endorsed by the publisher.
The Supplementary Material for this article can be found online at: https://www.frontiersin.org/articles/10.3389/fimmu.2022.1037517/full#supplementary-material
1. Zhu LY, Nie L, Zhu G, Xiang LX, Shao JZ. Advances in research of fish immune-relevant genes: a comparative overview of innate and adaptive immunity in teleosts. Dev Comp Immunol (2013) 39(1-2):39–62. doi: 10.1016/j.dci.2012.04.001
2. Fillatreau S, Six A, Magadan S, Castro R, Sunyer JO, Boudinot P. The astonishing diversity of ig classes and b cell repertoires in teleost fish. Front Immunol (2013) 4:28. doi: 10.3389/fimmu.2013.00028
3. Ross DA, Wilson MR, Miller NW, Clem LW, Warr GW. Evolutionary variation of immunoglobulin mu heavy chain RNA processing pathways: origins, effects, and implications. Immunol Rev (1998) 166:143–51. doi: 10.1111/j.1600-065x.1998.tb01259.x
4. Brooks K, Knight AM. Lowering the affinity between antigen and the b cell receptor can enhance antigen presentation. Eur J Immunol (2004) 34(3):837–43. doi: 10.1002/eji.200324357
5. Heyman B. Regulation of antibody responses via antibodies, complement, and fc receptors. Annu Rev Immunol (2000) 18:709–37. doi: 10.1146/annurev.immunol.18.1.709
6. Uribe C, Folch H, Enriquez R, Moran G. Innate and adaptive immunity in teleost fish: a review. Vet Med (2011) 56:486–503. doi: 10.17221/3294-VETMED
7. Bilal S, Etayo A, Hordvik I. Immunoglobulins in teleosts. Immunogenetics (2021) 73(1):65–77. doi: 10.1007/s00251-020-01195-1
8. Parra D, Takizawa F, Sunyer JO. Evolution of b cell immunity. Annu Rev Anim Biosci (2013) 1:65–97. doi: 10.1146/annurev-animal-031412-103651
9. Koumans-van Diepen JC, Egberts E, Peixoto BR, Taverne N, Rombout JH. B cell and immunoglobulin heterogeneity in carp (Cyprinus carpio l.); an immuno(cyto)chemical study. Dev Comp Immunol (1995) 19(1):97–108. doi: 10.1016/0145-305x(94)00061-j
10. Coscia MR, Simoniello P, Giacomelli S, Oreste U, Motta CM. Investigation of immunoglobulins in skin of the Antarctic teleost Trematomus bernacchii. Fish Shellfish Immunol (2014) 39(2):206–14. doi: 10.1016/j.fsi.2014.04.019
11. Bengtén E, Clem LW, Miller NW, Warr GW, Wilson M. Channel catfish immunoglobulins: repertoire and expression. Dev Comp Immunol (2006) 30(1-2):77–92. doi: 10.1016/j.dci.2005.06.016
12. Solem ST, Stenvik J. Antibody repertoire development in teleosts-a review with emphasis on salmonids and gadus morhua l. Dev Comp Immunol (2006) 30(1-2):57–76. doi: 10.1016/j.dci.2005.06.007
13. Sheng XZ, Xu GJ, Tang XQ, Zhan WB. Monoclonal antibodies recognizing mucus immunoglobulin and surface immunoglobulin-positive cells of flounder (Paralichthys olivaceus). Vet Immunol Immunopathol (2012) 145(1-2):143–50. doi: 10.1016/j.vetimm.2011.10.022
14. Sheng XZ, Qian XY, Tang XQ, Xing J, Zhan WB. Polymeric immunoglobulin receptor mediates immune excretion of mucosal IgM-antigen complexes across intestinal epithelium in flounder (Paralichthys olivaceus). Front Immunol (2018) 9:1562. doi: 10.3389/fimmu.2018.01562
15. Li J, Barred DR, Zhang YA, Boshra H, Gelman AE, Lapatra S, et al. B lymphocytes from early vertebrates have potent phagocytic and microbicidal abilities. Nat Immunol (2006) 7(10):1116–24. doi: 10.1038/ni1389
16. Edholm ES, Bengtén E, Stafford JL, Sahoo M, Taylor EB, Miller NW, et al. Identification of two IgD+ b cell populations in channel catfish. Ictalurus punctatus. J Immunol (2010) 185(7):4082–94. doi: 10.4049/jimmunol.1000631
17. Perdiguero P, Martín-Martín A, Benedicenti O, Díaz-Rosales P, Morel E, Muñoz-Atienza E, et al. Teleost IgD+IgM- b cells mount clonally expanded and mildly mutated intestinal IgD responses in the absence of lymphoid follicles. Cell Rep (2019) 29(13):4223–4235.e5. doi: 10.1016/j.celrep.2019.11.101
18. Zhang YA, Salinas I, Li J, Parra D, Bjork S, Xu Z, et al. IgT, a primitive immunoglobulin class specialized in mucosal immunity. Nat Immunol (2010) 11(9):827–35. doi: 10.1038/ni.1913
19. Cui ZW, Zhang XY, Wu CS, Zhang YA, Zhou Y, Zhang XJ. Membrane IgM+ plasma cells in grass carp (Ctenopharyngodon idella): Insights into the conserved evolution of IgM+ plasma cells in vertebrates. Dev Comp Immunol (2020) 106:103613. doi: 10.1016/j.dci.2020.103613
20. Velázquez J, Rodríguez A, Aragón H, Haidar A, González M, Valdés R, et al. Monoclonal antibody against Nile tilapia (Oreochromis niloticus) IgM heavy chain: A valuable tool for detection and quantification of IgM and IgM+ cells. Fish Shellfish Immunol (2021) 110:44–54. doi: 10.1016/j.fsi.2020.12.007
21. Huang YP, Yuan XY, Mu PF, Li QH, Ao JQ, Chen XH. Development of monoclonal antibody against IgM of large yellow croaker (Larimichthys crocea) and characterization of IgM+ b cells. Fish Shellfish Immunol (2019) 91:216–22. doi: 10.1016/j.fsi.2019.05.035
22. Santos MD, Saito-Taki T, Takano T, Kondo H, Hirono I, Aoki T. Characterization of polyclonal antibodies against Japanese flounder IgM derived from recombinant IgM constant region proteins. Fish Shellfish Immunol (2009) 27(2):374–8. doi: 10.1016/j.fsi.2009.03.008
23. Bunnoy A, Na-Nakorn U, Srisapoome P. Development of a monoclonal antibody specific to the IgM heavy chain of bighead catfish (Clarias macrocephalus): A biomolecular tool for the detection and quantification of IgM molecules and IgM+ cells in clarias catfish. Biomolecules (2020) 10(4):567. doi: 10.3390/biom10040567
24. Yang S, Tang XQ, Sheng XZ, Xing J, Zhan WB. Development of monoclonal antibodies against IgM of sea bass (Lateolabrax japonicus) and analysis of phagocytosis by mIgM+ lymphocytes. Fish Shellfish Immunol (2018) 78:372–82. doi: 10.1016/j.fsi.2018.04.042
25. Yang S, Chen WQ, He FF, Fu SM, Jin ZH, Zheng CC, et al. Comparison of the roles of IgM in systemic and mucosal immunity via tissue distribution analysis in largemouth bass (Micropterus salmoides). Aquaculture (2020) 527:735488. doi: 10.1016/j.aquaculture.2020.735488
26. Nakao M, Moritomo T, Tomana M, Fujiki K, Yano T. Isolation of cDNA encoding the constant region of the immuoglobulin heavy-chain from common carp (Cyprinus carpio l.). Fish Shellfish Immunol (1998) 8(6):425–34. doi: 10.1006/fsim.1998.0149
27. Fishery Bureau of Department of Agriculture of China. China Fishery statistical yearbook. Beijing: China Agriculture Press (2022).
28. Harikrishnan R, Balasundaram C. Modern trends in Aeromonas hydrophila disease management with fish. Rev Fisheries Sci (2005) 13(4):281–320. doi: 10.1080/10641260500320845
29. Sheng TG, Song GG, Yue TT, Zhang JH, Wang WD, Yang ZG, et al. Whole-genome sequencing and antimicrobial resistance analysis of multidrug-resistant Aeromonas veronii strain JC529 from a common carp. J Glob Antimicrob Resist (2021) 27:118–22. doi: 10.1016/j.jgar.2021.08.007
30. Jia YF, Li ZS, Du QY, Chang ZJ. Transcriptome analysis of immune-related gene expression in yellow river carp (Cyprinus carpio var.) after challenge with Flavobacterium columnare. Microb Pathog (2021) 160:105148. doi: 10.1016/j.micpath.2021.105148
31. Janda JM, Abbott SL. The genus Aeromonas: taxonomy, pathogenicity, and infection. Clin Microbiol Rev (2010) 23(1):35–73. doi: 10.1128/CMR.00039-09
32. Podok P, Xu LJ, Xu D, Lu LQ. Different expression profiles of interleukin 11 (IL-11), intelectin (ITLN) and purine nucleoside phosphorylase 5a (PNP 5a) in crucian carp (Carassius auratus gibelio) in response to cyprinid herpesvirus 2 and Aeromonas hydrophila. Fish Shellfish Immunol (2014) 38(1):65–73. doi: 10.1016/j.fsi.2014.03.001
33. Wang Y, Re Z, Fu L, Su X. Two highly adhesive lactic acid bacteria strains are protective in zebrafish infected with Aeromonas hydrophila by evocation of gut mucosal immunity. J Appl Microbiol (2016) 120(2):441–51. doi: 10.1111/jam.13002
34. Song XH, Zhao J, Bo YX, Liu ZJ, Wu K, Gong CL. Aeromonas hydrophila induces intestinal inflammation in grass carp (Ctenopharyngodon idella): An experimental model. Aquaculture (2014) 434:171–8. doi: 10.1016/j.aquaculture.2014.08.015
35. Shiry N, Khoshnoodifar K, Alavinia SJ. Cutaneous mucosal immune-parameters and intestinal immune-relevant genes expression in streptococcal-infected rainbow trout (Oncorhynchus mykiss): A comparative study with the administration of florfenicol and olive leaf extract. Fish Shellfish Immunol (2020) 107:403–10. doi: 10.1016/j.fsi.2020.10.023
36. Wang HQ, Zhou L, Yang M, Luo XC, Li YW, Dan XM. Identification and characterization of myeloperoxidase in orange-spotted grouper (Epinephelus coioides). Fish Shellfish Immunol (2018) 72:230–6. doi: 10.1016/j.fsi.2017.10.063
37. Xu Z, Parra D, Gómez D, Salinas I, Zhang YA, von Gersdorff Jørgensen L, et al. Teleost skin, an ancient mucosal surface that elicits gut-like immune responses. Proc Natl Acad Sci U.S.A. (2013) 110(32):13097–102. doi: 10.1073/pnas.1304319110
38. Xu Z, Takizawa F, Parra D, Gómez D, von Gersdorff Jørgensen L, LaPatra SE, et al. Mucosal immunoglobulins at respiratory surfaces mark an ancient association that predates the emergence of tetrapods. Nat Commun (2016) 7:10728. doi: 10.1038/ncomms10728
39. Yu YY, Kong WG, Xu HY, Huang ZY, Zhang XT, Ding LG, et al. Convergent evolution of mucosal immune responses at the buccal cavity of teleost fish. iScience (2019) 19:821–35. doi: 10.1016/j.isci.2019.08.034
40. Yu YY, Kong WG, Yin YX, Dong F, Huang ZY, Yin GM, et al. Mucosal immunoglobulins protect the olfactory organ of teleost fish against parasitic infection. PloS Pathog (2018) 14(11):e1007251. doi: 10.1371/journal.ppat.1007251
41. Kong WG, Yu YY, Dong S, Huang ZY, Ding LG, Cao JF, et al. Pharyngeal immunity in early vertebrates provides functional and evolutionary insight into mucosal homeostasis. J Immunol (2019) 203(11):3054–67. doi: 10.4049/jimmunol.1900863
42. Zhang XT, Yu YY, Xu HY, Huang ZY, Liu X, Cao JF, et al. Prevailing role of mucosal igs and b cells in teleost skin immune responses to bacterial infection. J Immunol (2021) 206(5):1088–101. doi: 10.4049/jimmunol.2001097
43. Xu HY, Dong F, Zhai X, Meng KF, Han GK, Cheng GF, et al. Mediation of mucosal immunoglobulins in buccal cavity of teleost in antibacterial immunity. Front Immunol (2020) 11:562795. doi: 10.3389/fimmu.2020.562795
44. Parra D, Korytář T, Takizawa F, Sunyer JO. B cells and their role in the teleost gut. Dev Comp Immunol (2016) 64:150–66. doi: 10.1016/j.dci.2016.03.013
45. Baldissera MD, Souza CF, Dias JB, Da Silva TO, Tavares GC, Valladão G, et al. Branchial bioenergetics dysfunction as a relevant pathophysiological mechanism in freshwater silver catfish (Rhamdia quelen) experimentally infected with Flavobacterium columnare. Microb Pathog (2020) 138:103817. doi: 10.1016/j.micpath.2019.103817
46. Gomez D, Sunyer JO, Salinas I. The mucosal immune system of fish: the evolution of tolerating commensals while fighting pathogens. Fish Shellfish Immunol (2013) 35(6):1729–39. doi: 10.1016/j.fsi.2013.09.032
47. Gómez GD, Balcázar JL. A review on the interactions between gut microbiota and innate immunity of fish. FEMS Immunol Med Microbiol (2008) 52(2):145–54. doi: 10.1111/j.1574-695X.2007.00343.x
48. Netea MG, Schlitzer A, Placek K, Joosten L, Schultze JL. Innate and adaptive immune memory: an evolutionary continuum in the host’s response to pathogens. Cell Host Microbe (2019) 25(1):13–26. doi: 10.1016/j.chom.2018.12.006
49. Zhang FM, Li MJ, Lv C, Wei GC, Wang C, Wang YM, et al. Molecular characterization of a new IgZ3 subclass in common carp (Cyprinus carpio) and comparative expression analysis of IgH transcripts during larvae development. BMC Vet Res (2021) 17(1):159. doi: 10.1186/s12917-021-02844-7
50. Wang QC, Ji W, Xu Z. Current use and development of fish vaccines in China. Fish Shellfish Immunol (2020) 96:223–34. doi: 10.1016/j.fsi.2019.12.010
51. Miyadai T, Ootani M, Tahara D, Aoki M, Saitoh K. Monoclonal antibodies recognising serum immunoglobulins and surface immunoglobulin-positive cells of puffer fish, torafugu (Takifugu rubripes). Fish Shellfish Immunol (2004) 17(3):211–22. doi: 10.1016/j.fsi.2004.03.005
52. Wu R, Shen J, Lai X, He T, Li Y. Development of monoclonal antibodies against serum immunoglobulins from gibel carp (Carassius auratus gibelio) and their applications in serodiagnosis of inapparent infection and evaluation of vaccination strategies. Fish Shellfish Immunol (2020) 96:69–77. doi: 10.1016/j.fsi.2019.11.059
53. Yang S, Tang XQ, Sheng XZ, Xing J, Zhan WB. Development of monoclonal antibodies against IgM of half-smooth tongue sole (Cynoglossus semilaevis) and analysis of phagocytosis of fluorescence microspheres by mIgM+ lymphocytes. Fish Shellfish Immunol (2017) 66:280–8. doi: 10.1016/j.fsi.2017.05.019
54. Magnadóttir B. Comparison of immunoglobulin (IgM) from four fish species. Icel Agric Sci (1998) 12:47–59.
55. Xu J, Zhang XT, Luo YZ, Wan XY, Yao YT, Zhang LQ, et al. IgM and IgD heavy chains of yellow catfish (Pelteobagrus fulvidraco): Molecular cloning, characterization and expression analysis in response to bacterial infection. Fish Shellfish Immunol (2019) 84:233–43. doi: 10.1016/j.fsi.2018.10.012
56. Li H, Zhang FM, Guo HY, Zhu YY, Yuan JD, Yang GW, et al. Molecular characterization of hepcidin gene in common carp (Cyprinus carpio l.) and its expression pattern responding to bacterial challenge. Fish Shellfish Immunol (2013) 35(3):1030–8. doi: 10.1016/j.fsi.2013.07.001
57. Lee MO, Kim EH, Jang HJ, Park MN, Woo HJ, Han JY, et al. Effects of a single nucleotide polymorphism in the chicken NK-lysin gene on antimicrobial activity and cytotoxicity of cancer cells. Proc Natl Acad Sci U.S.A. (2012) 109(30):12087–92. doi: 10.1073/pnas.1209161109
58. Chadzinska M, Baginski P, Kolaczkowska E, Savelkoul HF, Kemenade BM. Expression profiles of matrix metalloproteinase 9 in teleost fish provide evidence for its active role in initiation and resolution of inflammation. Immunology (2008) 125(4):601–10. doi: 10.1111/j.1365-2567.2008.02874.x
59. Lin B, Chen SW, Cao Z, Lin YQ, Mo DZ, Zhang HB, et al. Acute phase response in zebrafish upon Aeromonas salmonicida and Staphylococcus aureus infection: striking similarities and obvious differences with mammals. Mol Immunol (2007) 44:295–301. doi: 10.1016/j.molimm.2006.03.001
60. Urán PA, Gonçalves AA, Taverne-Thiele JJ, Schrama JW, Verreth JA, Rombout JH. Soybean meal induces intestinal inflammation in common carp (Cyprinus carpio l.). Fish Shellfish Immunol (2008) 25:751–60. doi: 10.1016/j.fsi.2008.02.013
61. Dietrich MA, Adamek M, Bilińska B, Hejmej A, Steinhagen D, Ciereszko A. Characterization, expression and antibacterial properties of apolipoproteins a from carp (Cyprinus carpio l.) seminal plasma. Fish Shellfish Immunol (2014) 41(2):389–401. doi: 10.1016/j.fsi.2014.09.020
62. Wu ZB, Meng KF, Ding LG, Wu S, Han GK, Zhai X, et al. Dynamic interaction between mucosal immunity and microbiota drives nose and pharynx homeostasis of common carp (Cyprinus carpio) after SVCV infection. Front Immunol (2021) 12:769775. doi: 10.3389/fimmu.2021.769775
63. Li C, Yu JK, Ai K, Li HY, Zhang Y, Zhao TY, et al. IκBα phosphorylation and associated NF-κB activation are essential events in lymphocyte activation, proliferation, and anti-bacterial adaptive immune response of Nile tilapia. Dev Comp Immunol (2020) 103:103526. doi: 10.1016/j.dci.2019.103526
64. Zhu WT, Zhang YQ, Zhang JC, Yuan GL, Liu XQ, Ai TS, et al. Astragalus polysaccharides, chitosan and poly(I:C) obviously enhance inactivated Edwardsiella ictaluri vaccine potency in yellow catfish Pelteobagrus fulvidraco. Fish Shellfish Immunol (2019) 87:379–85. doi: 10.1016/j.fsi.2019.01.033
65. Lv MY, Wang XY, Qiu XY, Zeng TT, Feng SY, Yin LC, et al. Functional characterization of grass carp (Ctenopharyngodon idella) interleukin-2 in head kidney leukocytes. Fish Shellfish Immunol (2020) 97:500–8. doi: 10.1016/j.fsi.2019.12.077
66. Evenhuis JP, Cleveland BM. Modulation of rainbow trout (Oncorhynchus mykiss) intestinal immune gene expression following bacterial challenge. Vet Immunol Immunopathol (2012) 146(1):8–17. doi: 10.1016/j.vetimm.2012.01.008
67. Stosik M, Tokarz-Deptuła B, Deptuła W. Immunological memory in teleost fish. Fish Shellfish Immunol (2021) 115:95–103. doi: 10.1016/j.fsi.2021.05.022
68. Shalapour S, Lin XJ, Bastian IN, Brain J, Burt AD, Aksenov AA, et al. Inflammation-induced IgA+ cells dismantle anti-liver cancer immunity. Nature (2017) 551(7680):340–5. doi: 10.1038/nature24302
69. Pröbstel AK, Zhou X, Baumann R, Wischnewski S, Kutza M, Rojas OL, et al. Gut microbiota-specific IgA+ b cells traffic to the CNS in active multiple sclerosis. Sci Immunol (2020) 5(53):eabc7191. doi: 10.1126/sciimmunol.abc7191
70. Castro R, Abós B, González L, Granja AG, Tafalla C. Expansion and differentiation of IgM+ b cells in the rainbow trout peritoneal cavity in response to different antigens. Dev Comp Immunol (2017) 70:119–27. doi: 10.1016/j.dci.2017.01.012
71. Tongsri P, Meng KF, Liu X, Wu ZB, Yin GM, Wang QC, et al. The predominant role of mucosal immunoglobulin IgT in the gills of rainbow trout (Oncorhynchus mykiss) after infection with Flavobacterium columnare. Fish shellfish Immunol (2020) 99:654–62. doi: 10.1016/j.fsi.2020.01.044
72. Yu Y, Huang ZY, Kong WG, Dong F, Zhang XT, Zhai X, et al. Teleost swim bladder, an ancient air-filled organ that elicits mucosal immune responses. Cell Discovery (2022) 8(1):31. doi: 10.1038/s41421-022-00393-3
73. Mutoloki S, Munang’andu HM, Evensen Ø. Oral vaccination of fish-antigen preparations, uptake, and immune induction. Front Immunol (2015) 6:519. doi: 10.3389/fimmu.2015.00519
74. Dong ZR, Mu QJ, Kong WG, Qin DC, Zhou Y, Wang XY, et al. Gut mucosal immune responses and protective efficacy of oral yeast cyprinid herpesvirus 2 (CyHV-2) vaccine in Carassius auratus gibelio. Front Immunol (2022) 13:932722. doi: 10.3389/fimmu.2022.932722
Keywords: common carp, Aeromonas hydrophila, IgM, B cells, mucosal immune response
Citation: Mu Q, Dong Z, Kong W, Wang X, Yu J, Ji W, Su J and Xu Z (2022) Response of immunoglobulin M in gut mucosal immunity of common carp (Cyprinus carpio) infected with Aeromonas hydrophila. Front. Immunol. 13:1037517. doi: 10.3389/fimmu.2022.1037517
Received: 06 September 2022; Accepted: 03 November 2022;
Published: 17 November 2022.
Edited by:
Erlong Wang, Northwest A & F University, ChinaReviewed by:
Yang He, Neijiang Normal University, ChinaCopyright © 2022 Mu, Dong, Kong, Wang, Yu, Ji, Su and Xu. This is an open-access article distributed under the terms of the Creative Commons Attribution License (CC BY). The use, distribution or reproduction in other forums is permitted, provided the original author(s) and the copyright owner(s) are credited and that the original publication in this journal is cited, in accordance with accepted academic practice. No use, distribution or reproduction is permitted which does not comply with these terms.
*Correspondence: Zhen Xu, emhlbnh1QGloYi5hYy5jbg==
†These authors have contributed equally to this work
Disclaimer: All claims expressed in this article are solely those of the authors and do not necessarily represent those of their affiliated organizations, or those of the publisher, the editors and the reviewers. Any product that may be evaluated in this article or claim that may be made by its manufacturer is not guaranteed or endorsed by the publisher.
Research integrity at Frontiers
Learn more about the work of our research integrity team to safeguard the quality of each article we publish.