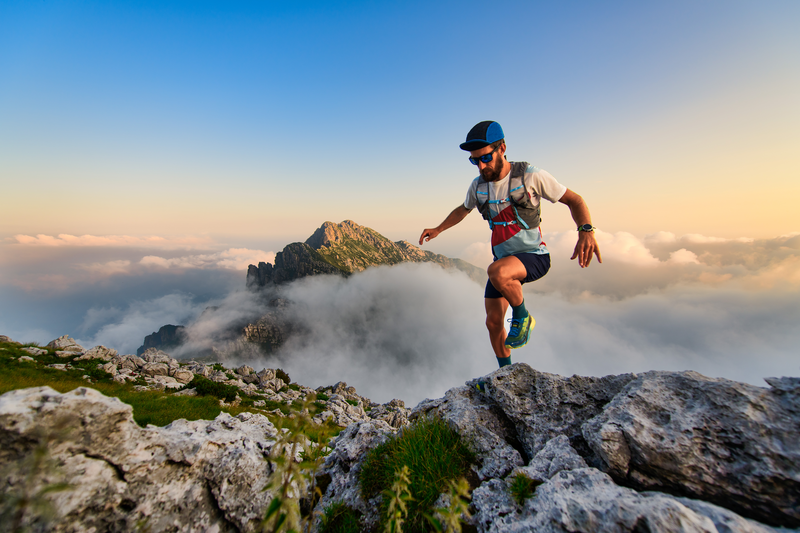
94% of researchers rate our articles as excellent or good
Learn more about the work of our research integrity team to safeguard the quality of each article we publish.
Find out more
ORIGINAL RESEARCH article
Front. Immunol. , 21 November 2022
Sec. Primary Immunodeficiencies
Volume 13 - 2022 | https://doi.org/10.3389/fimmu.2022.1035571
This article is part of the Research Topic SARS-COV-2 infections within Inborn Errors of Immunity Populations. View all 7 articles
Severe acute respiratory syndrome coronavirus 2 (SARS-CoV-2) is a single-stranded RNA virus that causes coronavirus disease 2019 (COVID-19). One of the main topics of conversation in these past months in the world of immunology has been the issue of how patients with immune defects will fare if they contract this infection. To date there has been limited data on larger cohorts of patients with Inborn Errors of Immunity (IEI) diagnosed with COVID-19. Here, we review the data of COVID-19 infections in a single center cohort of 113 patients from the Mount Sinai Immunodeficiency program, who had 132 infections between January 2020 and June 2022. This included 56 males and 57 females, age range 2 - 84 (median 42). The mortality rate was 3%. Comparison between admitted patients revealed a significantly increased risk of hospitalization amongst the unvaccinated patients, 4% vaccinated vs 40% unvaccinated; odds ratio 15.0 (95% CI 4.2 – 53.4; p <0.00001). Additionally, COVID anti-spike antibody levels, determined in 36 of these patients post vaccination and before infection, were highly variable.
In December 2019, a novel single-stranded RNA Coronavirus, SARS-CoV-2, emerged in Wuhan, China (1). SARS-CoV-2 enters human cells via the angiotensin-converting enzyme-2 receptor expressed predominantly in the lung and intestinal epithelial cells, alveolar cells, and vascular endothelial cells and is mainly transmitted by exposure to infectious respiratory fluids (2, 3). SARS-CoV-2 causes an infectious disease with variable presentation ranging from a mild common cold to severe respiratory failure (3, 4). In Spring of 2020, New York City became the epicenter of the severe acute respiratory syndrome coronavirus 2 (SARS-CoV-2) pandemic (5). As of July 2022, a total of 564.1 million confirmed cases globally and 6,371,354 cumulative deaths had been reported by the World Health Organization (WHO) (6). Since emerging, COVID-19 has remained an ongoing global pandemic (5). An increased risk for severe disease and death has been noted among elderly patients and persons with preexisting medical conditions (7). Human inborn errors of immunity (IEI) otherwise referred to as primary immunodeficiency (PID) result from monogenic germline mutations, resulting in loss or gain of function of the encoded protein (8). In patients with IEI, the course of COVID-19 may vary from asymptomatic, to death; however, overall, there has been demonstration of high morbidity and mortality relative to the general population (9–11). In one study, 63% of 94 patients with primary immune deficiency who developed COVID-19, required hospitalization, with case-fatality rate of approximately 10% (12). In addition to limited studies on COVID-19 in IEI population, vaccine efficacy remains unclear. Data regarding the effectiveness of anti–SARS-CoV-2 vaccine in 26 patients with IEI, showed that the majority of patients were able to respond to vaccines, with levels of anti-SARS-CoV-2 IgG antibodies, ie. Anti-spike (anti-S) IgG antibodies, evaluated using commercial automated assay, as well as neutralizing antibodies, examined by angiotensin-converting enzyme 2 (ACE2)-RBD inhibition (ELISA), and cellular responses (13). However, 62 patients with predominantly antibody deficiency, characterized by low helper T cells, low B cells, and/or low class-switched memory B cells, were at risk for low antibody response to SARS-CoV-2 immunization, which however, improved following additional doses of vaccination in the majority of patients (10). The Mount Sinai Immune Deficiency program follows approximately 900 - 1,000 patients with primary immune deficiency, including infants referred for newborn screening for severe T cell defects, patients with agammaglobulinemia, a wide range of antibody defects, complement, T cell or neutrophil defects. In this study, we report the clinical course, follow-up, and outcome of COVID-19 in patients with IEI and correlate outcomes of disease with vaccination status.
We analyzed a large cohort of IEI patients followed in the Mount Sinai Immune Deficiency program, who had a confirmed diagnosis of COVID-19, whether by PCR or rapid antigen testing, between March 2020 and July 2022. Spike-Antibody levels of a subset of these patients with IEI (n = 36) who had been vaccinated against SARS-CoV-2 before infection, were measured. Data including the patient demographics, clinical complications related to their IEI disease, treatments, antibody levels, vaccine status and outcomes were collected by either phone, chart review or at the time of in person follow up (Table 1). Data were collected and managed using Research Electronic Data Capture (REDCap) electronic data capture tools (14, 15). Statistical programing was done in MATLAB 2020a The MathWorks, Inc., Natick, Massachusetts, United States. This study was approved by the Institutional review board at Mount Sinai Medical Center.
Antibody testing (IgG anti SARS CoV-2 spike antibody), Elecsys® Anti−SARS−CoV−2 for patients was done using the same commercial SARS-CoV-2 Semi-Quant Total Ab Chemiluminescent Immunoassay (CLIA). Results reported until May 2022 were between 0.8-2500 U/mL.
One hundred and thirteen of our patients had a total of 132 confirmed Sars-COV-2 infections. The first recorded infection was in March 2020. The peak number of cases was observed in December 2021 when 27 new infections occurred (Figure 1); this coincided with the Omicron variant wave (BA.1.1529) in New York City (16). (Figure 2). The infected patients included 56 males and 57 females, of age range 2 to 84, (median age 42). The clinical parameters and course of COVID-19 in this cohort are shown (Tables 1, 2). Fifteen patients had agammaglobulinemia (13 had X-Linked agammaglobulinemia, XLA), 63 patients had common variable immunodeficiency (CVID), 15 had hypogammaglobulinemia, 5 had IgA deficiency (with or without IgG2 deficiency), 2 had specific antibody deficiency, and 3 had hyper IgM syndrome. The other patients had Kabuki syndrome, Di-George Syndrome, autoimmune polyendocrinopathy-candidiasis-ectodermal dystrophy (APCED), complement C5 deficiency, hyper IgE syndrome, Wiskott-Aldrich, Good syndrome, activated PI3K Delta Syndrome and a homozygous IFN gamma R1 mutation (Table 1, Figure 3). Comorbidities summarized in (Table 1) were as follows; autoimmune hemolytic anemia (AIHA) (n = 5), asthma (n = 12), bronchiectasis (n = 9), chronic obstructive pulmonary disease (COPD) (n = 1), immune thrombocytopenic purpura (ITP) (n = 16), hemolytic malignancy (n = 8), solid organ malignancy (n = 6), and nodular regenerative hyperplasia (n = 2). Nine of these patients had other comorbidities, including diabetes mellitus, thyroid disease, coronary artery disease, obesity and organ transplant.
Figure 1 Timeline of confirmed COVID-19 infections in our patients with IEI. Month-by-month data of confirmed COVID-19 infections presented for patients who were infected blue line, and infected and hospitalized red line.
Figure 2 Prevalance of COVID-19 variants in circulation in New York City from January 2021 to July 2022. Data generated from Coronavirus Disease 2019 (COVID-19) in New York City (NYC), from the NYC Department of Health and Mental Hygiene https://github.com/nychealth/coronavirus-data.
Of the 132 confirmed infections, 17 patients had two separate infections and one patient (with XLA) was infected on 3 separate occasions. The most commonly recorded symptoms with this illness were fever (84 of 132), dry cough (76 of 132), fatigue/weakness (38 of 132), dyspnea (35 of 132), congestion (31 of 132), diarrhea (7 of 16), rhinorrhea (20 of 132), headache (20 of 132), chills (18 of 132), diarrhea (18 of 132) and loss of smell/taste (12 of 132).
Vaccinations for COVID-19 became available, starting with BNT162b2 (Pfizer) (17) which was granted emergency use authorization (EUA) on December 11, 2021. Shortly after this, the mRNA-1273 (Moderna) (18), and Ad26.COV2.S (Johnson and Johnson) (19) vaccines were granted EUA. In our cohort, 88 patients were vaccinated, while 25 patients declined. Of the 88 vaccinated subjects, 68 patients were infected at least once after vaccination leading to a total of 73 infections. Examining the timing of infections and vaccinations for these subjects, 18 of the vaccinated subjects had one infection prior to being vaccinated (Figure 4A), while 54 other patients had only one episode of COVID-19 post vaccination, with a mean time to infection post vaccination of 185 days (range 22 – 470) (Figure 4B). In contrast, 16 other vaccinated patients had infections both before and after vaccination. For this group of patients, the mean time for reinfection from most recent vaccination was 178 (26 – 294) days, and the mean time from infection to reinfection was 227 (range 46 – 440) days Figure 4C. For the 25 subjects who had declined vaccination, there were 27 infections, (two with re-infections) with a mean time between infections of 294 days (range 149 – 439) (Figure 4D).
Figure 4 Time between vaccination and infection. Timeline demarcated by: (A) Single infection diagnosed prior to vaccination (B) Single infection diagnosed post vaccination (C) Multiple infections in vaccinated patients. (D) Infection(s) in unvaccinated population. Note, (C) includes patients with multiple infections both pre and post infection. The blue diamond and red triangle represents a vaccination and infection time point respectively.
Of the 132 infections 104 cases were managed on an outpatient basis, while 28 patients were admitted to the hospital ward; 16 of these required supplemental oxygen therapy, five patients required the highest level of care in the intensive care unit and were intubated (Table 2). Infected patients received azithromycin, and/or hydroxychloroquine (n = 14), early in the pandemic. Other treatments included convalescent plasma (n = 19), remdesivir (n = 11) dexamethasone (n = 11). When this became available, 28 people received monoclonal antibody therapy for COVID-19 (infusions included Bamlanivimab/Etesevimab, Casirivimab/Imdevimab and Sotrovimab). Of the patients who received monoclonal therapy, 3 patients were hospitalized and 25 continued management as outpatients post infusion. After January 14, 2021, 62 additional infections were confirmed, however, did not receive monoclonal therapy upon diagnosis. Four out of 62 infections were hospitalized (Table 2). There was no statistical difference regarding outcomes for hospitalization with monoclonal therapy; odds ratio 0.6 (95% CI 0.1 – 2.8). When approved, Paxlovid was administered to 14 patients.
Sixty-eight patients had been vaccinated for COVID-19 prior to infection with SARS-CoV-2. Sixty-three of these received 2 doses as a part of the initial series for Moderna and Pfizer mRNA vaccines, while 5 patients received one dose of the Johnson and Johnson vaccine). Within the cohort of 68 patients who were infected post vaccination, there were 73 infections; of these, 3 patients required hospital admission, and all required supplemental oxygen therapy. A comparison between admitted patients revealed a significantly increased risk of hospitalization in the unvaccinated patients [4% vaccinated vs 40% unvaccinated; odds ratio (OR) 15.0 (95% CI 4.2 – 53.4; p <0.00001)] (Figure 5). There were no ICU admissions in the vaccinated cohort. Also, during the time period after vaccinations became available (January 2021), there were also 20 patients who recorded an infection and remained unvaccinated, 6 of these patients were admitted to the hospital (3 of whom were age <15).
Figure 5 Comparison of disease severity between vaccinated and unvaccinated patients. Location of management (hospitalization vs outpatient) used as a proxy of disease severity. Comparison revealed a significantly increased risk of hospitalization amongst the unvaccinated patients (4% vaccinated vs 40% unvaccinated; odds ratio 15.0 (95% CI 4.2 – 53.4; p <0.00001)).
We analyzed the need for hospitalization by underlying diagnosis of IEI, separating patients with predominant antibody deficiencies [including agammaglobulinemia, CVID, hypogammaglobulinemia, IgA deficiency (with or without IgG2 deficiency), specific antibody deficiency, hyper IgM syndrome or Good syndrome] from those the other diagnosis encountered [Kabuki syndrome, Di-George syndrome, APCED, C5 deficiency, hyper IgE syndrome, Wiskott-Aldridge syndrome, activated PI3K delta syndrome or interferon gamma receptor deficiency] (Table 3). We noted that patients with these primarily antibody deficiencies were 14 times more likely to be hospitalized if infected while unvaccinated as compared to after vaccination. (OR 14.7 (95% CI 4.1 – 52.8); p <0.000001). Those with the other diagnoses were 4.5 more likely to be hospitalized if infected while unvaccinated as compared to those who had been vaccinated OR 4.5 (95% CI 0.2 – 80.6; p < 0.5, however this was not statistically significant).
The majority of infected patients survived, and fully recovered from acute disease. Four patients, early in the pandemic died from COVID-19 (CVID, n = 2; hypogammaglobulinemia, n = 1; IgA-IgG2 deficiency, n = 1) (5). Three of the patients who died had preexisting immune deficiency-associated autoimmune/inflammatory complications; 2 of these also had preexisting IEI-associated chronic lung disease (bronchiectasis, n = 1; interstitial lung disease, n = 1). In addition, one patient was a kidney transplant recipient who had previously had lymphoma. The age of the patients who died ranged from 39 to 76 years (5) (20). None had been vaccinated, as this was before the FDA authorized vaccines became available in January 2021. The five patients who were intubated and required intensive care unit level care were also unvaccinated.
Thirty-six of the 58 patients (Figures 4B, C) who were not infected prior to vaccination, had serum COVID anti-spike antibody levels checked prior to their confirmed infection (Figure 6). The amounts varied widely from 0 to 2500 U/mL. The antibody present in serum of these patients represents vaccination responses and/or contributions from immunoglobulin treatments, as this antibody began to appear in Ig products this interval (21, 22). There was no correlation between antibody level and need for hospitalization, based on the available data. However, it was observed that 2 of the hospitalized patients had an antibody level of < 10 U/ml. Both patients (ages 46 and 69) had a diagnosis of agammaglobulinemia (one with confirmed XLA), and one had known lung disease (bronchiectasis).
Figure 6 Correlation between disease severity and spike antibody level taken prior to infection. Antibodies were derived from multiple source including vaccination, immunoglobulin product, monoclonal infusions and convalescent plasma. Note, spike antibody test limit was 2500 U/ml.
The Mount Sinai Immunodeficiency Program previously reported the first group of 16 patients who developed COVID-19 early in the pandemic. The proportion of deaths in the original series (25%) was greater than that in the general population with COVID-19 reported at New York City hospitals (10.2%) at that time (5). From July 2020 to November 2021, the incidence of additional cases in our cohort remained relatively low, with 21% of cases occurring in that time range (Figure 1). This was perhaps due to behavioral practices such as masking, social distancing, and strict self-isolation. However, as variants such as the Omicron variant (BA.1) began to circulate (16), the infection rate began to rise in late 2021. Although the number of reported infections increased, we did not observe a large increase in rates of hospitalization with subsequent variants. In fact, there were no additional mortalities after the initial 4 patients reported (5). This was likely due to differences in viral strains, large variation in age range within our cohort, improved treatment measures, availability of convalescent plasma, monoclonal antibody infusions, and prophylactic therapies such as monoclonal infusions and vaccinations. Although analyses of monoclonal therapy in these patients revealed no statistical differences regarding outcomes for hospitalizations (odds ratio 0.6 (95% CI 0.1 – 2.8)) this could be due to a smaller size cohort of patients receiving this therapy, and/or patients having additional protection from vaccination. Additionally, the monoclonal antibodies available earlier in the pandemic no longer demonstrated the same efficacy with regard to later circulating variants (23).
For this cohort, there was an overall mortality rate of 3%; However, all deaths were early in the pandemic (5). Even though 3% is lower compared to other cohorts reported, with inpatient mortality rate at 40% (24), the 3% mortality rate seen in our cohort would still represent a high morbidity and mortality relative to the general population (1.1% in the US) (9–12, 25). Other studies had similar findings seen in our cohort, where patients through different phases of the pandemic experienced a mild clinical course with limited symptoms (26). Higher mortality in earlier studies could be explained by differences in viral strains, and additional modalities of treatment including use of steroids, plasma, antivirals, and monoclonal infusions for confirmed infections as well as prophylaxis with vaccines and monoclonal infusions.
Thirty-six patients had anti spike antibody levels evaluated prior to infection; these levels were highly variable and revealed no correlation with prevention of hospitalization, demonstrating that the level, and/or value of the antibodies tested is far from clear. Neutralizing antibodies were not examined. In one study, immune deficient patients showed a wide range of anti-spike antibody titers, ranging from undetectable levels to normal-to-high titers. In fact, when patients with XLA were excluded, 18 of 22 of the examined patients (81.8%) tested positive for anti-S antibodies (13). However, in another study, subjects with antibody deficiency were found to have lower mean anti-spike antibody levels, compared to matched healthy controls, leading to the assumption that patients were less protected (10). In addition to antibody, the role of T cell responses may also be critical components of the immune protection against SARS-CoV-2 (27–29).
Comparisons between admitted patients revealed a significantly increased risk of hospitalization amongst the unvaccinated patients [4% vaccinated vs 40% unvaccinated; odds ratio 15.0 (95% CI 4.2 – 53.4; p <0.00001)] (Figure 5) which suggest that vaccination may provide some level of protection against severe disease (10). This may be due to the generation of neutralizing antibody as well as augmented T cell responses. In one study, vaccines induced a strong cellular response with a trend toward statistical significance when convalescent individuals with IEI and no prior history of infection were compared with healthy vaccinated donors (13).
In summary, the clinical impact of COVID-19 in patients with primary immune deficiency varies from mild symptoms to death. The proportion of deaths in this series (3%) was greater than that in the general population with COVID-19 reported in New York (0.28% crude mortality rate) and in the US (1.1% mortality rate) (25, 30). However, over time, the patients who have become infected, have been less ill and have required few hospitalizations, similar to that of the general population, potentially due to less virulent variants of SARS-CoV-2 (31). However, the role of additional treatments such as our early use of plasma (32), and more recently, monoclonal antibodies and Paxlovid have been essential therapies.
The raw data supporting the conclusions of this article will be made available by the authors, without undue reservation.
The studies involving human participants were reviewed and approved by Institutional review board at Mount Sinai Medical Center. Written informed consent to participate in this study was provided by the participants’ legal guardian/next of kin.
All authors listed in this research contributed to the final publication. KC main author and primary investigator designed and executed the project, NF designed algorithms in MATLAB to process data and participated in compiling the paper, SJ participated in data collection, JF participated in data collection, AL participated in data collection, KR participated in data collection, DS participated in data collection, FJ participated in data collection, SA contributed to writing and review of the paper, H-eH contributed to writing and review of the paper, CC-R is the Senior investigator of the project.
The authors declare that the research was conducted in the absence of any commercial or financial relationships that could be construed as a potential conflict of interest.
All claims expressed in this article are solely those of the authors and do not necessarily represent those of their affiliated organizations, or those of the publisher, the editors and the reviewers. Any product that may be evaluated in this article, or claim that may be made by its manufacturer, is not guaranteed or endorsed by the publisher.
1. Huang C, Wang Y, Li X, Ren L, Zhao J, Hu Y, et al. Clinical features of patients infected with 2019 novel coronavirus in wuhan, China. Lancet (2020) 395(10223):497–506. doi: 10.1016/S0140-6736(20)30183-5
2. Stadnytskyi V, Bax CE, Bax A, Anfinrud P. The airborne lifetime of small speech droplets and their potential importance in SARS-CoV-2 transmission. Proc Natl Acad Sci U.S.A. (2020) 117(22):11875–7. doi: 10.1073/pnas.2006874117
3. Yao Y, Wang H, Liu Z. Expression of ACE2 in airways: Implication for COVID-19 risk and disease management in patients with chronic inflammatory respiratory diseases. Clin Exp Allergy (2020) 50(12):1313–24. doi: 10.1111/cea.13746
4. Esenboga S, Ocak M, Akarsu A, Bildik HN, Cagdas D, Iskit AT, et al. COVID-19 in patients with primary immunodeficiency. J Clin Immunol (2021) 41(7):1515–22. doi: 10.1007/s10875-021-01065-9
5. Ho HE, Mathew S, Peluso MJ, Cunningham-Rundles C. Clinical outcomes and features of COVID-19 in patients with primary immunodeficiencies in new York city. J Allergy Clin Immunol Pract (2021) 9(1):490–3.e2. doi: 10.1016/j.jaip.2020.09.052
6. World Health Organization. WHO coronavirus (COVID-19) dashboard. Geneva: WHO (2021). Available at: https://covid19.who.int/table.
7. Sanyaolu A, Okorie C, Marinkovic A, Patidar R, Younis K, Desai P, et al. Comorbidity and its impact on patients with COVID-19. SN Compr Clin Med (2020) 2(8):1069–76. doi: 10.1007/s42399-020-00363-4
8. Bousfiha A, Jeddane L, Picard C, Al-Herz W, Ailal F, Chatila T, et al. Human inborn errors of immunity: 2019 update of the IUIS phenotypical classification. J Clin Immunol (2020) 40(1):66–81. doi: 10.1007/s10875-020-00758-x
9. Jalil M, Pietras J, Ahmed SN, Daniels P, Hostoffer R. COVID-19 infection in patients with humoral immunodeficiency: A case series and literature review. Allergy Rhinol (Providence) (2022) 13:21526575221096044. doi: 10.1177/21526575221096044
10. Barmettler S, Yang NJ, Lam T, Naranbhai V, Dighe AS, Burke KE, et al. Response to SARS-CoV-2 initial series and additional dose vaccine in patients with predominant antibody deficiency. J Allergy Clin Immunol: In Pract (2022) 10(6):P1622–1634.E4 doi: 10.1016/j.jaip.2022.03.017
11. Delavari S, Abolhassani H, Abolnezhadian F, Babaha F, Iranparast S, Ahanchian H, et al. Impact of SARS-CoV-2 pandemic on patients with primary immunodeficiency. J Clin Immunol (2021) 41(2):345–55. doi: 10.1007/s10875-020-00928-x
12. Meyts I, Bucciol G, Quinti I, Neven B, Fischer A, Seoane E, et al. Coronavirus disease 2019 in patients with inborn errors of immunity: An international study. J Allergy Clin Immunol (2021) 147(2):520–31. doi: 10.1016/j.jaci.2020.09.010
13. Hagin D, Freund T, Navon M, Halperin T, Adir D, Marom R, et al. Immunogenicity of pfizer-BioNTech COVID-19 vaccine in patients with inborn errors of immunity. J Allergy Clin Immunol (2021) 148(3):739–49. doi: 10.1016/j.jaci.2021.05.029
14. Harris PA, Taylor R, Thielke R, Payne J, Gonzalez N, Conde JG. Research electronic data capture (REDCap)--a metadata-driven methodology and workflow process for providing translational research informatics support. J BioMed Inform (2009) 42(2):377–81. doi: 10.1016/j.jbi.2008.08.010
15. Harris PA, Taylor R, Minor BL, Elliott V, Fernandez M, O'Neal L, et al. The REDCap consortium: Building an international community of software platform partners. J BioMed Inform (2019) 95:103208. doi: 10.1016/j.jbi.2019.103208
16. Hygiene NDoHaM. Coronavirus disease 2019 (COVID-19) in New York City. (NYC: Gov Resource) (2022). Available at: https://www.nyc.gov/site/doh/covid/covid-19-main.page
17. Thomas SJ, Moreira ED, Kitchin N, Absalon J, Gurtman A, Lockhart S, et al. Safety and efficacy of the BNT162b2 mRNA covid-19 vaccine through 6 months. N Engl J Med (2021) 385(19):1761–73. doi: 10.1056/NEJMoa2110345
18. Baden LR, El Sahly HM, Essink B, Kotloff K, Frey S, Novak R, et al. Efficacy and safety of the mRNA-1273 SARS-CoV-2 vaccine. N Engl J Med (2021) 384(5):403–16. doi: 10.1056/NEJMoa2035389
19. Sadoff J, Gray G, Vandebosch A, Cardenas V, Shukarev G, Grinsztejn B, et al. Safety and efficacy of single-dose Ad26.COV2.S vaccine against covid-19. N Engl J Med (2021) 384(23):2187–201. doi: 10.1056/NEJMc2107809
20. Harada K, Ho HE, Cunningham-Rundles C. COVID-19 prevalence and outcomes in patients receiving biologic therapies at an infusion center in New York City. Clin Immunol (2021) 230:108803. doi: 10.1016/j.clim.2021.108803
21. Cousins K, Singh G, Aboelregal N, Ho H, Krammer F, Cummnigham-Rundles C. Detection of SARS-COV-2 antibodies in immunglobulin products. Ann Allergy Asthma Immunol 127(5):S6. doi: 10.1016/j.anai.2021.08.029
22. Hirsiger JR, Weigang S, Walz AC, Fuchs J, Daly ML, Eggimann S, et al. Passive immunization against COVID-19 by anti-SARS-CoV-2 spike IgG in commercially available immunoglobulin preparations in severe antibody deficiency. J Allergy Clin Immunol Pract (2022) 10(9):2452–5.e3. doi: 10.1016/j.jaip.2022.06.020
23. VanBlargan LA, Errico JM, Halfmann PJ, Zost SJ, Crowe JE Jr., Purcell LA, et al. An infectious SARS-CoV-2 B.1.1.529 omicron virus escapes neutralization by therapeutic monoclonal antibodies. Nat Med (2022) 28(3):490–5. doi: 10.1038/s41591-021-01678-y
24. Castano-Jaramillo LM, Yamazaki-Nakashimada MA, O'Farrill-Romanillos PM, Muzquiz Zermeno D, Scheffler Mendoza SC, Venegas Montoya E, et al. COVID-19 in the context of inborn errors of immunity: a case series of 31 patients from Mexico. J Clin Immunol (2021) 41(7):1463–78. doi: 10.1007/s10875-021-01077-5
25. Center JsHUoMCR. Mortality analyses. (Johns Hopkins School of Medicine: Coronavirus Resource Center) (2022). Available at: https://coronavirus.jhu.edu/data/mortality
26. Giardino G, Milito C, Lougaris V, Punziano A, Carrabba M, Cinetto F, et al. The impact of SARS-CoV-2 infection in patients with inborn errors of immunity: the experience of the Italian primary immunodeficiencies network (IPINet). J Clin Immunol (2022) 42(5):935–46. doi: 10.1007/s10875-022-01264-y
27. Oberhardt V, Luxenburger H, Kemming J, Schulien I, Ciminski K, Giese S, et al. Rapid and stable mobilization of CD8(+) T cells by SARS-CoV-2 mRNA vaccine. Nature (2021) 597(7875):268–73. doi: 10.1038/s41586-021-03841-4
28. Moss P. The T cell immune response against SARS-CoV-2. Nat Immunol (2022) 23(2):186–93. doi: 10.1038/s41590-021-01122-w
29. Zhao J, Zhao J, Perlman S. T Cell responses are required for protection from clinical disease and for virus clearance in severe acute respiratory syndrome coronavirus-infected mice. J Virol (2010) 84(18):9318–25. doi: 10.1128/JVI.01049-10
30. Worldometer. Coronavirus (COVID-19) mortality rate 2022 . Available at: http://www.worldemeters.info/coronavirus/coronavirus-death-rate/.
31. Wen W, Chen C, Tang J, Wang C, Zhou M, Cheng Y, et al. Efficacy and safety of three new oral antiviral treatment (molnupiravir, fluvoxamine and paxlovid) for COVID-19a meta-analysis. Ann Med (2022) 54(1):516–23. doi: 10.1080/07853890.2022.2034936
Keywords: coronavirus disease 2019 (COVID-19), severe acute respiratory syndrome coronavirus 2 (SARS-CoV-2), inborn errors of immunity (IEI), primary immunodeficiency (PID), common variable immunodeficiency (CVID), X-linked agammaglobulinemia (XLA), covid vaccination, monoclonal therapy
Citation: Cousins K, DeFelice N, Jeong S, Feng J, Lee ASE, Rotella K, Sanchez D, Jaber F, Agarwal S, Ho H-e and Cunningham-Rundles C (2022) SARS-COV-2 infections in inborn errors of immunity: A single center study. Front. Immunol. 13:1035571. doi: 10.3389/fimmu.2022.1035571
Received: 02 September 2022; Accepted: 28 October 2022;
Published: 21 November 2022.
Edited by:
Leif Gunnar Hanitsch, Charité Universitätsmedizin, GermanyReviewed by:
Mónica Martínez-Gallo, Vall d’Hebron University Hospital, SpainCopyright © 2022 Cousins, DeFelice, Jeong, Feng, Lee, Rotella, Sanchez, Jaber, Agarwal, Ho and Cunningham-Rundles. This is an open-access article distributed under the terms of the Creative Commons Attribution License (CC BY). The use, distribution or reproduction in other forums is permitted, provided the original author(s) and the copyright owner(s) are credited and that the original publication in this journal is cited, in accordance with accepted academic practice. No use, distribution or reproduction is permitted which does not comply with these terms.
*Correspondence: Kimberley Cousins, S2ltYmVybGV5LmNvdXNpbnNAbXNzbS5lZHU=
Disclaimer: All claims expressed in this article are solely those of the authors and do not necessarily represent those of their affiliated organizations, or those of the publisher, the editors and the reviewers. Any product that may be evaluated in this article or claim that may be made by its manufacturer is not guaranteed or endorsed by the publisher.
Research integrity at Frontiers
Learn more about the work of our research integrity team to safeguard the quality of each article we publish.