- 1Department of Anesthesiology, Tianjin Medical University General Hospital, Tianjin, China
- 2Tianjin Research Institute of Anesthesiology, Tianjin, China
- 3Department of Critical Care Medicine, Tianjin Medical University General Hospital, Tianjin, China
Objectives: Sepsis-associated encephalopathy (SAE) patients in the intensive care unit (ICU) and perioperative period are administrated supplemental oxygen. However, the correlation between oxygenation status with SAE and the target for oxygen therapy remains unclear. This study aimed to examine the relationship between oxygen therapy and SAE patients.
Methods: Patients diagnosed with sepsis 3.0 in the intensive care unit (ICU) were enrolled. The data were collected from the Medical Information Mart for Intensive Care IV (MIMIC IV) database and the eICU Collaborative Research Database (eICU-CRD) database. The generalized additive models were adopted to estimate the oxygen therapy targets in SAE patients. The results were confirmed by multivariate Logistic, propensity score analysis, inversion probability-weighting, doubly robust model, and multivariate COX analyses. Survival was analyzed by the Kaplan-Meier method.
Results: A total of 10055 patients from eICU-CRD and 1685 from MIMIC IV were included. The incidence of SAE patients was 58.43%. The range of PaO2 (97-339) mmHg, PaO2/FiO2 (189-619), and SPO2≥93% may reduce the incidence of SAE, which were verified by multivariable Logistic regression, propensity score analysis, inversion probability-weighting, and doubly robust model estimation in MIMIC IV database and eICU database. The range of PaO2/FiO2 (189-619) and SPO2≥93% may reduce the hospital mortality of SAE were verified by multivariable COX regression.
Conclusions: SAE patients in ICU, including perioperative period, require conservative oxygen therapy. We should maintain SPO2≥93%, PaO2 (97-339) mmHg and PaO2/FiO2 (189-619) in SAE patients.
Introduction
Sepsis-associated encephalopathy (SAE) refers to cognitive dysfunction that can be attributed to systemic inflammatory responses in the absence of direct infection of the central nervous system (1). The incidence of SAE is over 70% of patients admitted to the intensive care unit (ICU) (2). SAE correlates with higher mortality (50.3%), longer hospital stays, and poorer long-term outcomes (3).
Patients admitted to the ICU and perioperative period are administered supplemental oxygen as low partial pressure of arterial oxygen (PaO2) is detrimental. However, a high PaO2 correlates with increased mortality, as confirmed in previous studies (4, 5). In a medical-surgical population of adult critically ill patients, arterial oxygen saturation (SPO2) supplementation titrated to 94%-98% correlates with favorable outcomes (6). The frequent oxygen exposure above the protocol goal (PaO2 >80 mmHg and FiO2 >0.5) correlates with worse clinical outcomes in patients who develop acute respiratory distress syndrome (7). PaO2 between (77-220) mmHg and the PaO2/FiO2 ratio in the range of 314-788 also correlates with favorable neurologic outcomes (8). Lower or higher oxygenation targets correlate with worse patient outcomes in ICU and perioperative period.
Potentially adjusted factors contributing to SAE include acute renal failure, hyperglycemia >10 mmol/l, hypercapnia >45 mmHg, and hypernatremia >145 mmol/l (3). The relationships between lower or higher oxygen therapy targets and the incidence and survival in SAE patients remain unclear. This study aimed to assess the correlation of SpO2, PaO2, and PaO2/FiO2 with SAE in ICU and perioperative period, and elucidate the optimal oxygen therapy targets in SAE patients.
Materials and methods
Study settings
We collected information on patients admitted to the ICU between 2008-2019 from the MIMIC-IV 0.4 and between 2014-2015 from the eICU-CRD v2.0 (NO. 33690380) database. MIMIC IV comprises 69619 and eICU-CRD of 200859 ICU admissions. The eICU database is a multi-center dataset. These were approved by the institutional review boards of the Massachusetts Institute of Technology and Beth Israel Deaconess Medical Center (9). The requirement for individual patient consent was waived because the project does not impact clinical care and all patient confidential information was anonymized. MIMIC-IV and eICU included the demographics, laboratory measurements, microbiology cultures diagnoses, and other patient data. The MIMIC IV database (version 1.0) is publicly available on https://physionet.org/content/mimiciv/1.0/ and the eICU is publicly available on https://eICU-crd.mit.edu/about/eICU/. The raw data were extracted by employing structure query language (SQL) with Navicat and further processed using the R software.
Patients
Sepsis was diagnosed with an acute change in the total sequential organ failure assessment (SOFA) score ≥2 and documented or suspected infection complied with the sepsis-3.0 criteria (10). The patients with infection sites or prescriptions of antibiotics and samples of bodily fluids for microbiological culture had suspected infection. In line with the existing literature, the microbiological sample must have been collected within 24 h when the antibiotic was first administered, and at the first occurrence of microbiological sampling, the antibiotic administration would be within 72 h (11). The SOFA score was defined in the first 24 h of the admission of the patient to the ICU.
SAE in this study was defined as the Glasgow Coma Scale (GCS) <15 or diagnosed delirium (3, 12, 13). Consciousness disorder with clear causes was excluded. GCS has been established as a clinically effective tool to characterize SAE and distinguish it from sepsis (3, 14). Specifically for the sedated or postoperative patients, GCS that was evaluated before sedation or surgery was extracted.
Inclusion criteria were as follows: 1) patients aged over 18 years; 2) ICU stays with more than 24 h of oxygen therapy, and 3) patients complying with the diagnostic criteria as in sepsis 3.0.
Following were the exclusion criteria (3, 12): 1) patients with brain injury (e.g., traumatic brain injury, meningitis, encephalitis intracerebral hemorrhage, cerebral embolism, ischemic stroke, epilepsy, brain tumor or intracranial infection, and any other cerebrovascular disease); 2) mental disorders and neurological disease; 3) chronic alcohol or drug abuse; 4) metabolic encephalopathy, hepatic encephalopathy, hypertensive encephalopathy, hypoglycemic coma, and other liver disease or kidney disease that affected consciousness; 5) severe electrolyte imbalances or glycemic disturbances, including hyponatremia (<120 mmol/l), hyperglycemia (>180 mg/dl), or hypoglycemia (<54 mg/dl); 6) those without GCS assessment; 7) missing values of SpO2, FiO2, PaO2 or no signs of administration of supplemental oxygen. Hyponatremia, hyperglycemia, and hypoglycemia can cause metabolic encephalopathy. Patients with any of the above-mentioned conditions were excluded.
Data collection
Patient information (e.g., age, gender, length of hospital stay, and hospital mortality), laboratory indicators, co-existing illnesses, sites of infection, microbiology types, and advanced cardiac life support (e.g., mechanical ventilation and vasopressors) were extracted as the demographic data using SQL. The laboratory indicators of the patients were collected within the first 24 h of their ICU stay, including pulse oxygen saturation (SPO2), partial pressure of carbon dioxide (PaCO2), partial pressure of oxygen (PaO2), and a fraction of inspired oxygen (FiO2). The coexisting illnesses were determined following the recorded International Classification of Diseases (ICD)-9 and ICD-10 codes (e.g., hypertension, diabetes, pulmonary disease, and kidney disease). Disease severity scores included the SOFA score and GCS. Only the data of the first ICU admission of the corresponding patients have been included.
Statistical analysis
The data were analyzed using the R software. Data distributions were analyzed by the Shapiro-Wilk test. Herein, all the data exhibited skewed distributions. Continuous data (age, PaCO2, FiO2, PaO2, SPO2, length of hospital stay, SOFA, and GCS) were expressed as median and interquartile ranges (IQR). Other categorical data were expressed in counts and proportions. Continuous variables were examined using the non-parametric Mann-Whitney U-test. Furthermore, categorical variables were compared using the Fisher exact test.
PaO2 and PaO2/FiO2 show a nonlinear correlation with the incidence in SAE patients. The generalized additive models (15) were adopted to estimate the association between median PaO2, PaO2/FiO2, and SAE, and the rage of PaO2, PaO2/FiO2 target was determined for reducing the incidence and hospital mortality among SAE patients.
The range of PaO2, SPO2, PaO2/FiO2 targets for incidence and hospital mortality of SAE patients as determined using multivariate Logistic, COX regression models, propensity score analysis, inversion probability-weighting, and doubly robust model for further validation of the oxygen values. An independent association between blood oxygen index levels and patients’ SAE was inferred through the doubly robust estimation method. Multivariate Logistic regression and Extreme Gradient Boosting (XGBoost) were used to create propensity score models for the 7 covariables (Figure 3) in sepsis patients with SAE. A cohort of inverse probability of treatment weighting (IPTW) was generated from the estimated propensity scores. Afterward, we performed a Logistic Regression on the weighted cohort to adjust for remaining unbalanced variables in the propensity score model between SAE groups and non-SAE groups, resulting in a double robust analysis. To determine whether IPTW reduced the imbalance of covariate distribution, the standardized mean difference (SMD) of the original cohort was compared with the SMD of the IPTW cohort. The Kaplan-Meier curves were analyzed using Log-rank tests.
Results
Baseline characteristics
A total of 51395 patients met the sepsis 3.0 criteria in the MIMIC IV database and the eICU database; among them, 39655 patients were excluded from the analysis due to intracerebral hemorrhage, encephalitis, brain tumor, brain injury, mental disorders, drug abuse, alcoholism, Alzheimer’s disease, metabolic encephalopathy, hepatic encephalopathy, the absence of FiO2 over 21%, missing records of the oxygen index, or no records of GCS scores; three patients were younger than 18 years and other conditions. A total of 11740 patients were included in the final analysis (Figure 1).
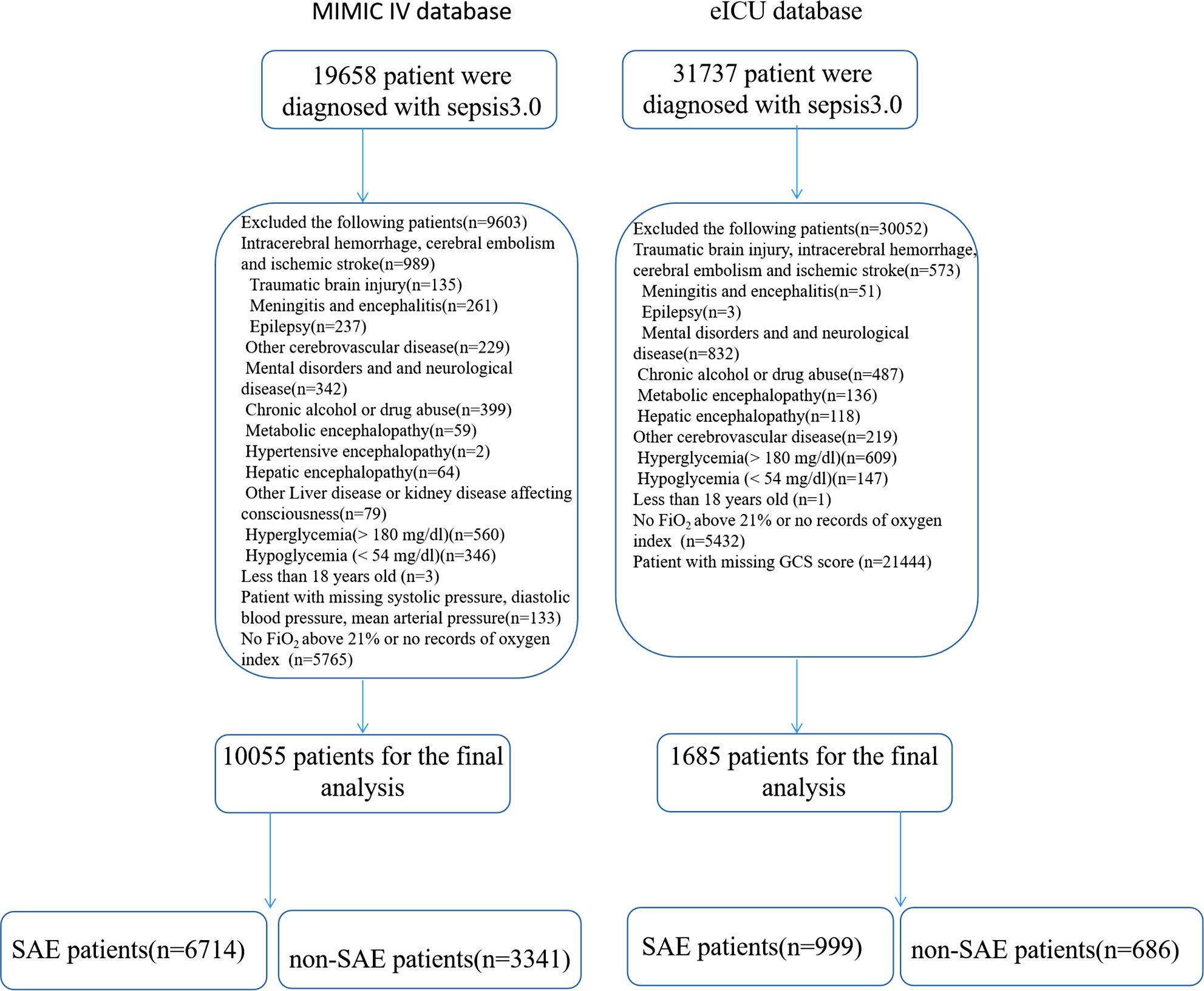
Figure 1 Flow chart for patient selection. ICU, intensive care unit; PaCO2, partial pressure of carbon dioxide; FiO2, the fraction of inspired oxygen.
Table 1 summarized the characteristics and outcomes of sepsis in the MIMIC IV database. Relative to the non-SAE group in the original cohort, patients in the SAE group were more likely to suffer from Klebsiella, Escherichia coli, or fungus infection. The SAE group showed higher respiratory rates, FiO2, and lower PaO2, SPO2, and PaO2/FiO2, relative to the non-SAE group. Patients in the SAE group exhibited higher SOFA and hospital mortality with a longer length of hospital stay.
Generalized additive models to estimate the optimal oxygen therapy targets for incidence of SAE
Figure 2 illustrated the correlation between the incidence of SAE and the median SPO2, PaO2, and PaO2/FiO2 determined using the generalized additive models in MIMIC IV database. The generalized additive models demonstrated a nonlinear correlation between PaO2 and PaO2/FiO2 with SAE incidence. SPO2<93%, PaO2<97 mmHg and >339 mmHg, and PaO2/FiO2<189 and >619 were associated with increased incidence of SAE, as shown in Table 2.
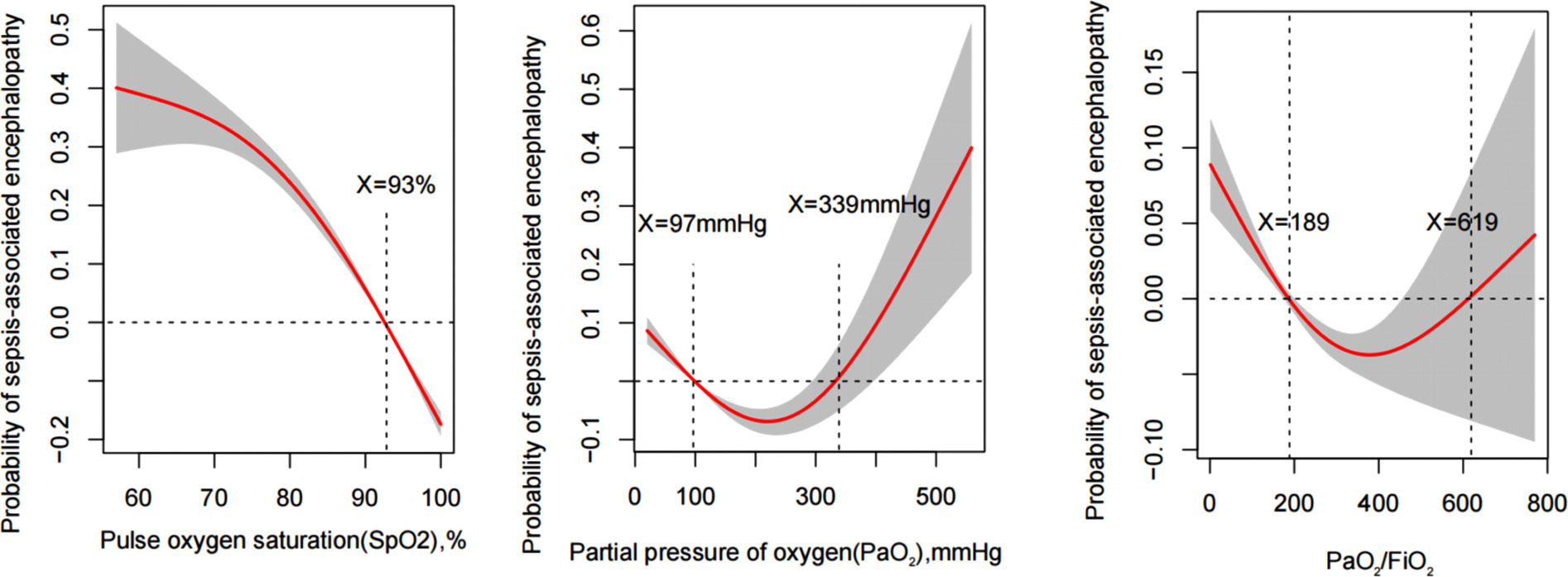
Figure 2 Generalized additive model plots for the median of SPO2, PaO2, and PaO2/FiO2 indicating the probability of sepsis-associated encephalopathy incidence.
Multivariate logistic analysis for risk factors of SAE incidence
According to the results of the generalized additive model, as shown in Figure 2, PaO2 (97-339) mmHg, PaO2/FiO2 (189-619), and SPO2≥93% may reduce the incidence of SAE. After adjusting for confounders, PaO2 (97-339) mmHg [odds ratio (OR): 0.566, 95% confidence interval (CI): 0.471-0.681, p<0.001], PaO2/FiO2 (189-619) [OR:0.513, 95% CI: 0.452-0.582, p<0.001], and SPO2≥93% [OR:0.324, 95% CI: 0.272-0.387, p<0.001] were identified as protective factors against SAE conduct internal assessment in the MIMIC IV database (Supplementary Material 1). Besides, using the eICU database for external evaluation (Supplementary Material 2), after adjusting for confounders, PaO2 (97-339) mmHg [OR: 0.703, 95% CI: 0.547-0.903, p=0.006], PaO2/FiO2 (189-619) [OR:0.750, 95% CI: 0.555-0.982, p=0.048], and SPO2≥93% [OR:0.779, 95% CI: 0.631-0.961, p=0.020] were identified as protective factors (Supplementary Materials 1, 2 ).
Propensity match analysis
To further confirm the reliability of the results, the data in the MIMIC IV database is used for internal verification and the data in the eICU database is used for external verification by propensity score analysis, inversion probability-weighting, and doubly robust model. A propensity matching scoring model was constructed using 7 covariates with statistically significant differences in Table 1 of the original cohort in the MIMIC IV database (Figure 3). For standardizing the differences between the SAE group and the non-SAE group, the estimated propensity scores were used. Covariates were well balanced between classes after IPTW (<0.1) (Figure 3). After matching the difference covariates, PaO2, SPO2, and PaO2/FiO2 were significantly different between the SAE group and the non-SAE group (Table 1). To evaluate the relationship between the PaO2, SPO2, and PaO2/FiO2 levels (estimated as per generalized additive model) and SAE incidence, we used propensity-matching score, proportion score IPTW and a doubly robust model with two databases to statistical analysis. The estimation models led to the same conclusion in the MIMIC IV and eICU database that SPO2≥93%, PaO2/FiO2 (189-619), and PaO2(97-339) mmHg were protective factors for patients with SAE (Table 2).
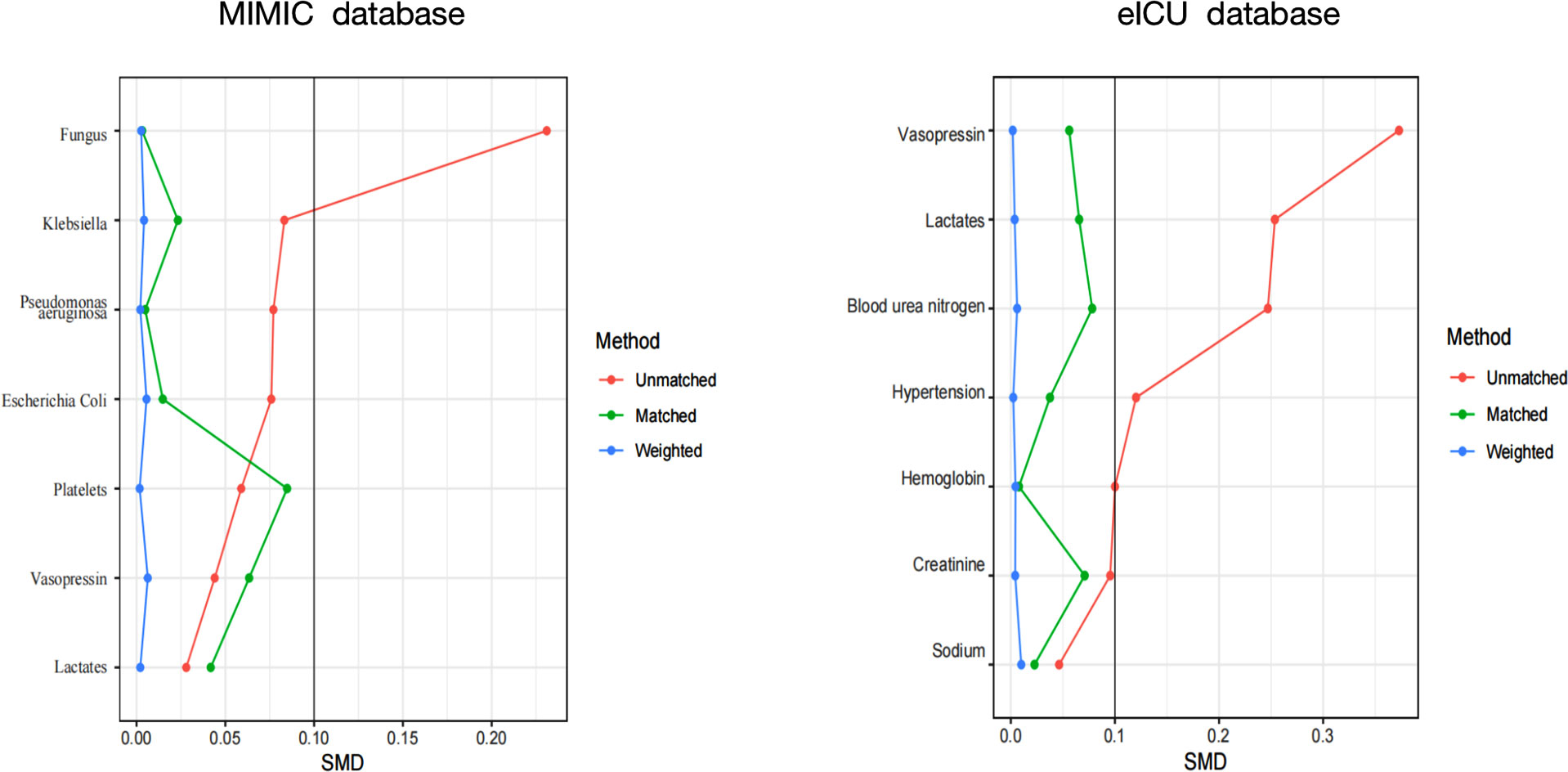
Figure 3 A SMD of the original cohort was compared with the SMD of the IPTW cohorts. SMD, standardized mean difference.
Prognostic analyses of patients with SAE
To further examine the effect of SAE on the prognoses of patients with sepsis, their survival was analyzed by the Kaplan-Meier method. Patients in the non-SAE group showed better survival rates than the SAE group (p<0.001) (Figure 4).
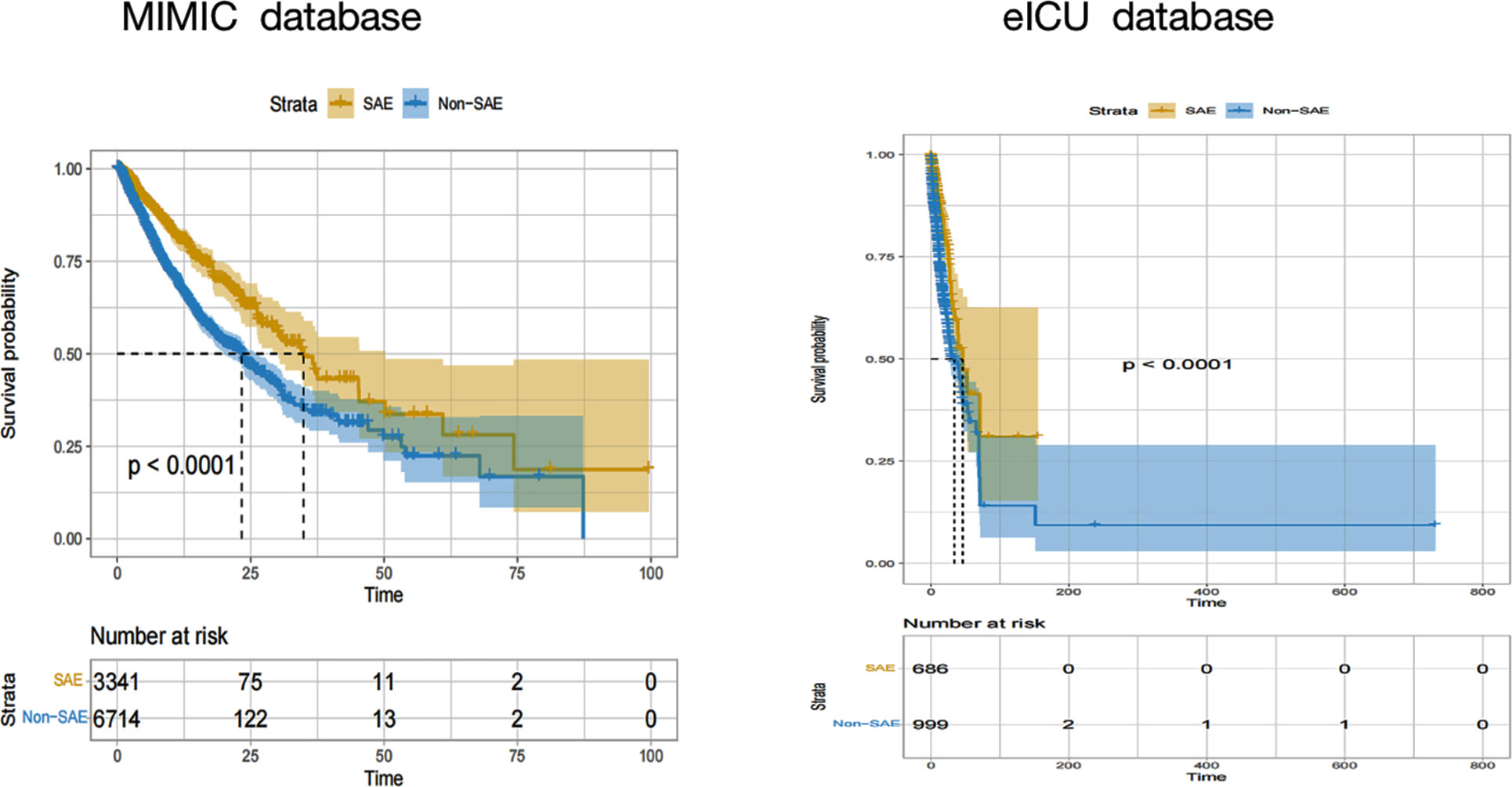
Figure 4 Kaplan-Meier hospital survival curves for SAE patients. SAE, sepsis-associated encephalopathy.
Demographic and clinical characteristics of SAE
Table 1 and Figure 4 illustrated that SAE patients had poor prognoses. To further examine the effect of oxygen therapy on the prognoses of patients with SAE, we divided the SAE patients into the survival and non-survival groups in the MIMIC IV and eICU database. The results as listed in Supplementary Material 3 showed that non-surviving patients had higher PaO2 and PaO2/FiO2.
Multivariate COX analysis for risk factors of hospital mortality in SAE
According to the results of the generalized additive model, as shown in Figure 2, after adjusting for confounders, SPO2≥93% and PaO2/FiO2(189-619) were independent protective factors for SAE prognoses in the MIMIC IV and eICU database (Table 3; Supplementary Materials 4, 5).
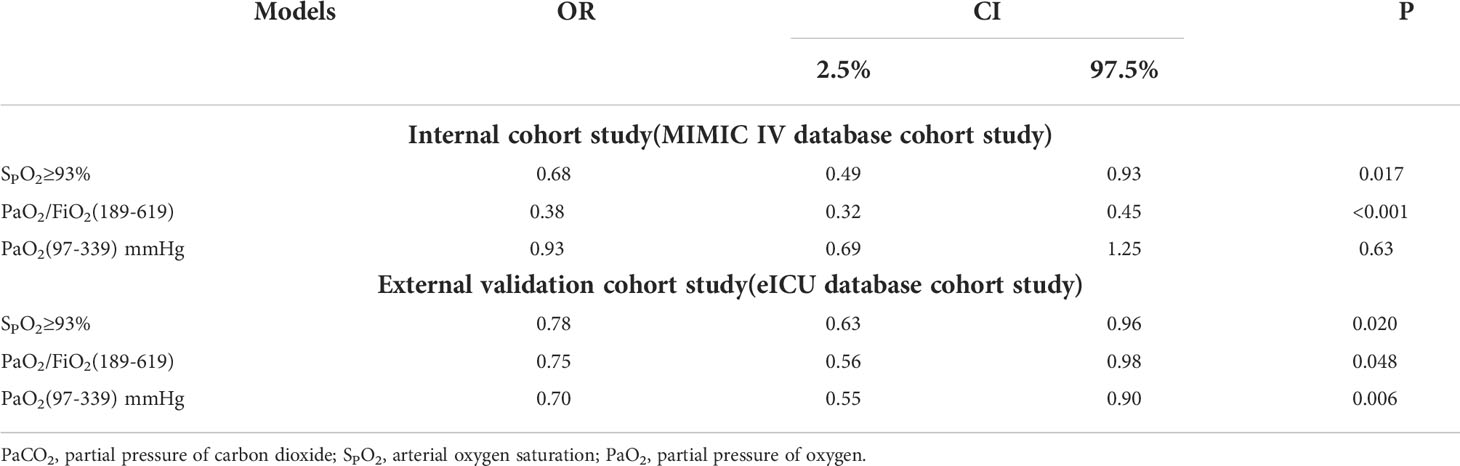
Table 3 Multiple COX regression model analysis of blood oxygen index to hospital mortality in sepsis with encephalopathy.
Discussion
As demonstrated from the primary outcome of this cohort study, oxygen therapy may be correlated with SAE incidence. The PaO2 range of 97 to 339 mmHg, the PaO2/FiO2 ratio between 189 and 619, and SPO2≥93% may be correlated with reducing SAE incidence. The PaO2 range of 97 to 339 mmHg and the PaO2/FiO2 ratio between 189 and 619 may be correlated with reduce SAE hospital mortality. Conservative oxygen therapy should be performed for SAE patients.
As reported in existing studies, the SAE incidence can reach up to 70% (2), in line with the results of this study (58.43%), which suggested a high SAE incidence. The results of the cohort study showed that hospital mortality was 13.6% in SAE patients, the hospital mortality of SAE group was significantly higher than that of non-SAE group, and that SAE patient with a higher SOFA score and longer hospital stay. These results with consistent with the findings of a previous study (3). It has been reported that 45% of SAE patients show long-term cognitive dysfunction after hospital discharge (16). Taken together, SAE shows a high incidence and poor prognosis. However, specific treatment for SAE is rare, and early identification of potentially modifiable factors with the optimal chance of avoiding incidence, long-term cognitive dysfunction, and reducing mortality are needed. In this large cohort study, the PaO2 range of 97 to 339 mmHg and the PaO2/FiO2 ratio between 189 and 619 were associated with reduced incidence and hospitalization mortality of SAE. Thus, lower or higher oxygenation could induce SAE.
Hyperoxemia is correlated with neurological injury in patients with traumatic brain injury and aneurysmal subarachnoid hemorrhage (17, 18). Hyperoxemia leads to the production of reactive oxygen species, thereby destroying cells and further promoting inflammatory responses (18). Moreover, active oxygen can cause an increase in the production of free radicals of oxygen, whereby excess free radicals can stimulate the hypersensitive arterial system, resulting in vasospasm (19). Inflammatory responses, oxygen-free radicals, and vasospasm are vital mechanisms underlying SAE (20, 21). Nguyen Mai et al. propose a conceptual model of lung-brain coupling, the use of supplemental oxygen can induce cardiac arrest, neuronal injury, neuroinflammation, and memory deficits (22). The results of this study demonstrated that PaO2 >339 mmHg and PaO2/FiO2>619 may be increase the incidence of encephalopathy in patients with sepsis. Hyperoxemia has been proven to be correlated with SAE. The neurological injury in sepsis patients attributed to hyperoxia may be due to the above-mentioned reasons. A subsequent study is thus required to evaluate the underlying pathophysiological mechanism in the future (23).
Neurological injury attributed to hypoxemia has been extensively confirmed (24, 25). Kim I Chisholm et al. report that sepsis leads to increased sensitivity of cortical mitochondria to hypoxemia, and such increased sensitivity is mirrored by a decrease in cortical tissue oxygen tension in mice (26). Transcription-dependent mechanisms triggered by hypoxia and reticulum stress could activate AMP-activated protein kinase, thereby stimulating the inflammatory activities. AMP protein is vital and is stimulated by immune factors in the brain, and consequently, the MAP signaling pathway is inhibited, decreased meta apoptosis and autophagy (22, 27). Hypoxia increases the levels of lactate/pyruvate, decreases the glutathione/oxidized glutathione ratio, upregulates inflammatory cytokine cascades, activates the apoptosis pathway, all leading to the damage of the cerebral cortex and neurons (23, 28). The results of this study further demonstrated that PaO2 <97mmHg, PaO2/FiO2<189, and SPO2<93% may cause changes in consciousness among sepsis patients. To mitigate the neurological injury by hypoxia or hyperoxia and incidence of SAE, PaO2 with 97-339 mmHg, PaO2/FiO2 in the range of 189-619, and SPO2≥93% should be taken in SAE patients.
Low oxygen saturations are considered detrimental. A liberal oxygen strategy is correlated with mortality, especially in ICU patients, as oxygen is extensively used in the ICU, and patients are commonly exposed to high oxygenation (29). The correlation between exposure to hyperoxia and mortality has been reported in ICU in previous studies (30, 31). Specifically for critically ill patients or those on ventilator-assisted breathing, to reduce mortality, the assessment of optimal oxygen saturation is particularly important. Willem van den Boom et al. report that the optimal range of SPO2 is 94%–98% which is correlated with decreased hospital mortality among critically ill patients (32). The proportion of time of oxygen saturation of 95%–99% correlates with reduced mortality in critically ill patients on mechanical ventilation, as reported by Dawei Zhou et al. (33). According to Derek K Chu et al., in acutely ill adults, liberal oxygen therapy increases mortality, and oxygen saturation of 94%-96% adversely affects the patients (34). In this study, the SPO2<93% was significantly associated with hospital mortality among SAE patients. The range of PaO2 (97-339) mmHg and PaO2/FiO2 (189-619) are associated with lower hospital mortality in SAE patients. The findings support that SAE patients should be administrated with conservative oxygen therapy for reducing the incidence and hospital mortality.
Limitation
First, the definition of SAE is compliance with GCS<15 score, and patients diagnosed with delirium according to ICD9 and ICD10. Though brain hemorrhage, brain trauma, and other diseases were excluded, the absence of brain computed tomography scans, magnetic resonance imaging, electroencephalogram, and other examinations to assess the nervous system, the result information bias maybe in the SAE cohort. Second, this was an observational study, and the causal correlation between oxygen therapy and the incidence and mortality of SAE could not be proved. However, the correlation between oxygen therapy and SAE was demonstrated in this large cohort study by multiple databases and Multiple statistical methods. The findings provide certain clinical reference values. Finally, due to the interrelationship between diseases, some confounding factors remained, thereby covering up or exaggerating the relationship between study factors and SAE.
Conclusions
In conclusion, high or low PaO2, PaO2/FiO2 and SPO2 were correlated with the incidence of SAE. The range of PaO2 (97-339) mmHg, PaO2/FiO2 (189-619) and SPO2 ≥93% were identified in SAE patients in ICU and perioperative period. A reference target was provided which is expected to aid clinicians in preventing the occurrence and reducing the incidence and hospital mortality of SAE. PaO2 (97-339) mmHg, PaO2/FiO2 (189-619) and SPO2 ≥93% as reference targets for subsequent experiments.
Data availability statement
Publicly available datasets were analyzed in this study. This data can be found here: The MIMIC IV database (version 1.0) is publicly available at https://mimic-iv.mit.edu/and the eICU database is publicly available at https://eicu-crd.mit.edu/about/eicu/. Any researcher who adheres to the data use requirements is permitted access to these databases. The codes are available at https://github.com/MIT-LCP/mimic-iv.
Author contributions
YL, LZ, KX, and YHY conceived the central ideas of the study. YY, KZ, YJ, and ZW collected the data. YL and LZ wrote the first draft of the manuscript. KX and YHY revised the paper, worked on the English, and drafted the final version of the manuscript. All authors contributed to the article and approved the submitted version.
Funding
This work was supported by the grant from the Medical and Health Science and Technology Plan Project of Inner Mongolia Autonomous Region Health Commission (202202326); Natural Science Foundation of Inner Mongolia Autonomous Region (2022MS08002); Science and Technology Support Key Program Affiliated to the Key Research and Development Plan of Tianjin Science and Technology Project (18YFZCSY00560); Natural Science Foundation of China (81772043, 81971879, 82072150); Tianjin Key Medical Discipline (Specialty) Construction Project (TJYXZDXK-036A).
Conflict of interest
The authors declare that the research was conducted in the absence of any commercial or financial relationships that could be construed as a potential conflict of interest.
Publisher’s note
All claims expressed in this article are solely those of the authors and do not necessarily represent those of their affiliated organizations, or those of the publisher, the editors and the reviewers. Any product that may be evaluated in this article, or claim that may be made by its manufacturer, is not guaranteed or endorsed by the publisher.
Supplementary material
The Supplementary Material for this article can be found online at: https://www.frontiersin.org/articles/10.3389/fimmu.2022.1035298/full#supplementary-material
Abbreviations
SAE, sepsis-associated encephalopathy; PaCO2, partial pressure of carbon dioxide; FiO2, fraction of inspired oxygen; PaO2, partial pressure of oxygen; SpO2 pulse oxygen saturation
arterial oxygen saturation; SOFA, sequential organ failure assessment; GCS, Glasgow coma scale; ICU, intensive care unit; MIMIC-IV, Medical Information Mart for Intensive Care IV.
References
1. Eidelman LA, Putterman D, Putterman C, Sprung CL. The spectrum of septic encephalopathy. definitions, etiologies, and mortalities. JAMA (1996) 275(6):470–3. doi: 10.1001/jama.1996.03530300054040
2. Ely WE. Delirium as a predictor of mortality in mechanically ventilated patients in the intensive care unit. JAMA (2004) 291(14):1753. doi: 10.1001/jama.291.14.1753
3. Sonneville R, de Montmollin E, Poujade J, Garrouste-Orgeas M, Souweine B, Darmon M, et al. Potentially modifiable factors contributing to sepsis-associated encephalopathy. Intensive Care Med (2017) 43(8):1075–84. doi: 10.1007/s00134-017-4807-z
4. Bellani G, Laffey JG, Pham T, Fan E, Brochard L, Esteban A, et al. Epidemiology, patterns of care, and mortality for patients with acute respiratory distress syndrome in intensive care units in 50 countries. JAMA (2016) 315(8):788–800. doi: 10.1001/jama.2016.0291
5. Schjrring OL, Jensen A, Nielsen CG, Ciubotariu A, Rasmussen BS. Arterial oxygen tensions in mechanically ventilated ICU patients and mortality: A retrospective, multicentre, observational cohort study. Br J Anaesth (2020) 124(4):420–9. doi: 10.1016/j.bja.2019.12.039
6. Rinaldi L MA, Morelli A, Antonelli M, Singer M. Effect of conservative vs conventional oxygen therapy on mortality among patients in an intensive care unit: The oxygen-ICU randomized clinical trial. JAMA (2016) 316(15):1583–9. doi: 10.1001/jama.2016.11993
7. Aggarwal NR, Brower RG, Hager DN, Thompson BT, Netzer G, Shanholtz C, et al. Oxygen exposure resulting in arterial oxygen tensions above the protocol goal was associated with worse clinical outcomes in acute respiratory distress syndrome. Crit Care Med (2017) 46(4):517–24. doi: 10.1097/CCM.0000000000002886
8. Chang WT, Wang CH, Lai CH, Yu HY, Chou NK, Wang CH, et al. Optimal arterial blood oxygen tension in the early postresuscitation phase of extracorporeal cardiopulmonary resuscitation: A 15-year retrospective observational study. Crit Care Med (2019) 47(11):1549–56. doi: 10.1097/CCM.0000000000003938
9. Johnson A, Bulgarelli L, Pollard T, Horng S, Celi LA, Mark R. MIMIC-IV (version 0.4). PhysioNet (2020). doi: 10.13026/a3wn-hq05
10. Singer M, Deutschman CS, Seymour CW, Shankar-Hari M, Annane D, Bauer M, et al. The third international consensus definitions for sepsis and septic shock (Sepsis-3). JAMA (2016) 315(8):801–10. doi: 10.1001/jama.2016.0287
11. Seymour CW, Liu VX, Iwashyna TJ, Brunkhorst FM, Rea TD, Scherag A, et al. Assessment of clinical criteria for sepsis: For the third international consensus definitions for sepsis and septic shock (Sepsis-3). JAMA (2016) 315(8):762–74. doi: 10.1001/jama.2016.0288
12. Yang Y, Liang S, Geng J, Wang Q, Wang P, Cao Y, et al. Development of a nomogram to predict 30-day mortality of patients with sepsis-associated encephalopathy: A retrospective cohort study. J Intensive Care (2020) 8(1):45. doi: 10.1186/s40560-020-00459-y
13. Boogaard M, Schoonhoven L, Care TAJC. Haloperidol prophylaxis in critically ill patients with a high risk for delirium. Crit Care (2013) 17(1):1–11. doi: 10.1186/cc11933
14. Iwashyna TJ, Ely EW, Smith DM, Langa KM. Long-term cognitive impairment and functional disability among ssurvivors of severe sepsis. JAMA (2010) 304(16):1787. doi: 10.1001/jama.2010.1553
16. Annane D, Sharshar T. Cognitive decline after sepsis. Lancet Respir Med (2015) 3(1):61–9. doi: 10.1016/S2213-2600(14)70246-2
17. Reynolds RA, Amin SN, Jonathan SV, Tang AR, Lan M, Wang C, et al. Hyperoxemia and cerebral vasospasm in aneurysmal subarachnoid hemorrhage. Neurocrit Care (2020) 35(1):30–38. doi: 10.1093/neuros/nyaa447_295
18. Ala‐Peijari M, Koivisto T, Djafarzadeh S, Bendel S. A pilot study of hyperoxemia on neurological injury, inflammation and oxidative stress. Acta Anaesth Scandinavica (2018) 62(6):801–10. doi: 10.1111/aas.13093
19. Damiani E, Donati A, Girardis M. Oxygen in the critically ill: Friend or foe? Curr Opin Anaesthesiol (2018) 31(2):129–35. doi: 10.1097/ACO.0000000000000559
20. Ren C, Yao RQ, Zhang H, Feng YW, Yao YM. Sepsis-associated encephalopathy: A vicious cycle of immunosuppression. J Neuroinflammation (2020) 17(1):14. doi: 10.1186/s12974-020-1701-3
21. Checinski A, Polito A, Friedman D, Siami S, Annane D, Sharshar T. Sepsis-associated encephalopathy and its differential diagnosis. Crit Care Med (2010) 5(6):901–9. doi: 10.2217/fnl.10.62
22. Mai N, Miller-Rhodes K, Knowlden S, Halterman MW. The post-cardiac arrest syndrome: A case for lung–brain coupling and opportunities for neuroprotection. J Cereb Blood Flow Metab (2019) 39(6):939–58. doi: 10.1177/0271678X19835552
23. Guo R, Hou W, Dong Y, Yu Z, Stites J, Weiner CP. Brain injury caused by chronic fetal hypoxemia is mediated by inflammatory cascade activation. Reprod Sci (2010) 17(6):540–8. doi: 10.1177/1933719110364061
24. Aminah S, Meng X, Liu J, Alexandra M, Kao J, Mcquillen PS, et al. Neonatal hypoxia-ischemia causes functional circuit changes in subplate neurons. Cereb Cortex (2018) 29(2):765–76. doi: 10.1093/cercor/bhx358
25. Arjun R, Acharya S, Shender BS, Rorres C, Hrebien L, Kam M. Correlation of cognitive scores and the onset of hypoxia. Aerosp Med Hum Perform (2019) 90(5):429–39. doi: 10.3357/AMHP.5040
26. Chisholm KI, Ida KK, Davies AL, Tachtsidis I, Papkovsky DB, Dyson A, et al. Hypothermia protects brain mitochondrial function from hypoxemia in a murine model of sepsis. J Cereb Blood Flow Metab (2016) 36(11):1955–64. doi: 10.1177/0271678X15606457
27. Zhou R, Li Y, Qu Y, Huang Q, Sun X, Mu D, et al. Regulation of hippocampal neuronal apoptosis and autophagy in mice with sepsis-associated encephalopathy by immunity-related GTPase M1. CNS Neurosci Ther (2020) 26(2):177–88. doi: 10.1111/cns.13229
28. Overgaard M, Rasmussen P, Bohm AM, Seifert T, Secher NH. Hypoxia and exercise provoke both lactate release and lactate oxidation by the human brain. FASEB J Off Publ Fed Am Societies Exp Biol (2012) 26(7):3012. doi: 10.1096/fj.11-191999
29. Helmerhorst HJ, Schultz MJ, van der Voort PH, Bosman RJ, Juffermans NP, de Jonge E, et al. Self-reported attitudes versus actual practice of oxygen therapy by ICU physicians and nurses. Ann Intensive Care (2014) 4(23):23. doi: 10.1186/s13613-014-0023-y
30. Helmerhorst H, Arts DL, Schultz MJ, Voort P, Westerloo D. Metrics of arterial hyperoxia and associated outcomes in critical care. Crit Care Med (2016) 45(2):187–95. doi: 10.1097/CCM.0000000000002084
31. Eastwood G, Bellomo R, Bailey M, Taori G, Pilcher D, Young P, et al. Arterial oxygen tension and mortality in mechanically ventilated patients. Intensive Care Med (2012) 38(1):91–8. doi: 10.1007/s00134-011-2419-6
32. van den Boom W, Hoy M, Sankaran J, Liu M, Chahed H, Feng M, et al. The search for optimal oxygen saturation targets in critically ill patients: Observational data from Large ICU databases. Chest (2020) 157(3):566–73. doi: 10.1016/j.chest.2019.09.015
33. Zhou D LZ, Shi G. Zhou time spent in oxygen saturation 95-99% is associated with reduced mortality in critically ill patients with mechanical ventilation. Crit Care Med (2005) 9(2):24(1). doi: 10.1186/s13054-020-03126-8
Keywords: sepsis, sepsis-associated encephalopathy, oxygen saturation, incidence, mortality
Citation: Li Y, Zhao L, Yu Y, Zhang K, Jiang Y, Wang Z, Xie K and Yu Y (2022) Conservative oxygen therapy in critically ill and perioperative period of patients with sepsis-associated encephalopathy. Front. Immunol. 13:1035298. doi: 10.3389/fimmu.2022.1035298
Received: 02 September 2022; Accepted: 03 October 2022;
Published: 19 October 2022.
Edited by:
Chenyang Duan, Chongqing Medical University, ChinaReviewed by:
Luis Del Carpio-Orantes, Delegación Veracruz Norte, MexicoYu Chao, Anhui Medical University, China
Copyright © 2022 Li, Zhao, Yu, Zhang, Jiang, Wang, Xie and Yu. This is an open-access article distributed under the terms of the Creative Commons Attribution License (CC BY). The use, distribution or reproduction in other forums is permitted, provided the original author(s) and the copyright owner(s) are credited and that the original publication in this journal is cited, in accordance with accepted academic practice. No use, distribution or reproduction is permitted which does not comply with these terms.
*Correspondence: Keliang Xie, eGlla2VsaWFuZzIwMDlAaG90bWFpbC5jb20=; Yonghao Yu, eXl1QHRtdS5lZHUuY24=
†These authors have contributed equally to this work