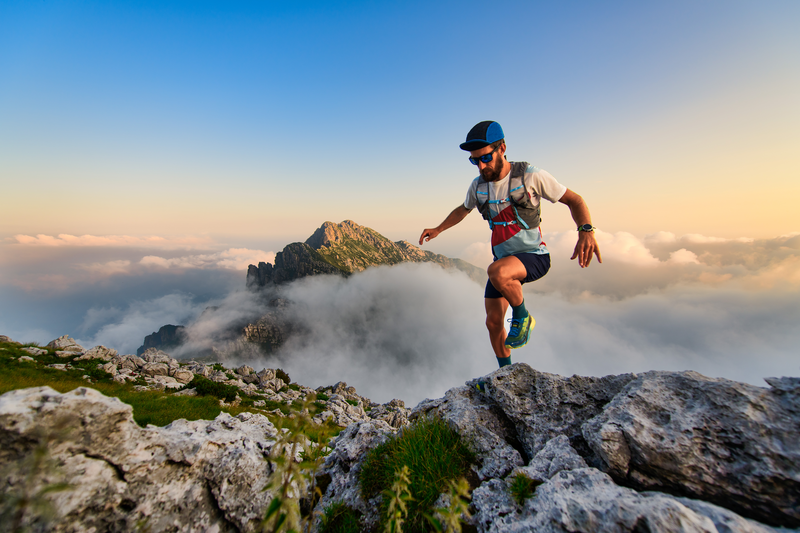
95% of researchers rate our articles as excellent or good
Learn more about the work of our research integrity team to safeguard the quality of each article we publish.
Find out more
REVIEW article
Front. Immunol. , 28 October 2022
Sec. Immunological Tolerance and Regulation
Volume 13 - 2022 | https://doi.org/10.3389/fimmu.2022.1032575
This article is part of the Research Topic Expert Opinions and Perspectives in Immunological Tolerance and Regulation: 2023 View all 7 articles
Parts of this article's content have been modified or rectified in:
Erratum: Type 1 regulatory T cell-mediated tolerance in health and disease
Type 1 regulatory T (Tr1) cells, in addition to other regulatory cells, contribute to immunological tolerance to prevent autoimmunity and excessive inflammation. Tr1 cells arise in the periphery upon antigen stimulation in the presence of tolerogenic antigen presenting cells and secrete large amounts of the immunosuppressive cytokine IL-10. The protective role of Tr1 cells in autoimmune diseases and inflammatory bowel disease has been well established, and this led to the exploration of this population as a potential cell therapy. On the other hand, the role of Tr1 cells in infectious disease is not well characterized, thus raising concern that these tolerogenic cells may cause general immune suppression which would prevent pathogen clearance. In this review, we summarize current literature surrounding Tr1-mediated tolerance and its role in health and disease settings including autoimmunity, inflammatory bowel disease, and infectious diseases.
Immunological tolerance is vital in a healthy immune system to prevent excessive inflammation and autoimmunity, and many cell types contribute to tolerance [reviewed in (1)]. Self-tolerance is largely mediated by FOXP3+ regulatory T cells (Treg) while type 1 regulatory T cells (Tr1) contribute greatly to peripheral tolerance and to some degree, self-tolerance. Tr1 cells were first discovered when a severe combined immunodeficiency (SCID) patient received a mismatched allogeneic fetal liver and thymus transplant and developed stable mixed chimerism (2, 3). This patient had high levels of serum IL-10, and further analysis revealed that T cell clones from this patient produced substantial amounts of IL-10 rapidly after T cell receptor (TCR) stimulation but produced low amounts of IL-2 (3). Interestingly, IL-2 production was rescued by stimulating the cells in a TCR-independent manner. Follow-up studies established that Tr1 cells were a subset of regulatory T cells that produced large amounts of IL-10 and TGF-β, variable amounts of IFN-γ comparable to undifferentiated CD4+ T cells (Th0), and little to no IL-2 and IL-4, therefore making the cytokine profile distinct from Th1 and Th2 cells (4). Tr1 cells proliferated poorly in response to cognate antigen, and this proliferative defect could be rescued by IL-2; it was later demonstrated that Tr1 cells proliferated extensively in response to IL-15 as well (5). Tr1 cells were highly suppressive through secretion of IL-10 and TGF-β, and they suppressed colitis in a mouse T-cell transfer model (4). Secretion of IL-10 can directly limit effector T cell responses, and it can also indirectly suppress T cell responses by limiting the antigen presenting capacity of antigen presenting cells (6–9). We and others showed that Tr1 cells also have cytotoxic potential which can contribute to tolerance via elimination of myeloid antigen presenting cells (APC) (10–13). Tr1 cells also suppress through PD-1/PD-L1- and CTLA-4-mediated mechanisms (14). In addition to modulating T cell responses directly and through APCs, Tr1 cells can also regulate antibody production by B cells (15, 16). Due to their potent, antigen-specific suppression, Tr1 cells have garnered considerable attention for clinical applications [reviewed in (17, 18)]. In contrast to FOXP3+ Tregs, antigen-specific Tr1 cells can easily be generated in vitro and in vivo, and polyclonal Tr1 cells can also mediate tolerance via modulation of APC function and subsequent induction of antigen-specific Tr1 cells – a process known as infectious tolerance (19). Tr1 cells have clinical applications in inflammatory diseases such as inflammatory bowel diseases (IBD), autoimmune disease (AID) ranging from type 1 diabetes (T1D) to multiple sclerosis (MS), and even in infectious diseases. In this review, we will provide a summary of the role of Tr1-mediated tolerance in health and various disease settings.
Tr1 cells are a subset of peripherally induced FOXP3- regulatory CD4+ T cells which can limit immune responses to foreign and autoantigens. Prior to the discovery of surface markers to identify Tr1 cells, they were often described based on their hallmark cytokine profile. FOXP3-IL-10+ CD4+ T cells were often referred to as Tr1 cells, though this profile alone is insufficient to identify Tr1 cells. For instance, suppressive IL-10+CTLA-4+ Th2 cells can arise in the mouse, but these are distinct from Tr1 cells as they secrete high amounts of IL-4, IL-5, and IL-13 (20). In many instances, co-production of IL-10 and IFN-γ has been used to identify Tr1 cells, Tr1-like cells, or Th1 cells expressing IL-10, thus making it difficult to determine the actual cell type being studied. Using co-production of IL-10 and IFN-γ as a metric is not an ideal method to identify Tr1 cells, as human Th1 cells may co-produce these cytokines yet are distinct from Tr1 cells, despite their important role in limiting inflammatory Th1 responses [reviewed in (21)]. It is important to note these differences, as the pathways driving IL-10 production by Th1 cells are impaired in several diseases (22–24). Moreover, if the regulatory capacity of these cells is transient, as has been demonstrated (23), then these cells could potentially reacquire an effector profile and therefore not contribute to resolution of inflammation and could even exacerbate immunopathology. One notable difference between these cell types can be seen by the transcription factors they express: IL-10-producing Th1 cells express high levels of Tbx21 and Egr2 but low Gata3 (25), whereas Tr1 cells isolated from the peripheral blood of healthy donors had elevated GATA3 expression compared to memory CD4+ T cells with no changes in TBX21 or EGR2 (26). While high GATA3 expression was not reported in in vitro-differentiated Tr1 cells (27), TBX21 and EGR2 expression was not elevated, thus suggesting these may not be features of human Tr1 cells. Additionally, IL-10-producing Th1 cells are often not anergic and proliferate extensively in response to antigen [reviewed in (21)]. Currently, it is unclear how else these two populations may vary. This is an area of research which is being actively pursued utilizing advances in molecular technology including ATAC sequencing to build a better understanding of these cell types.
To better characterize Tr1 cells, extensive efforts aimed to identify cell surface markers unique to Tr1 cells. The first step toward this demonstrated that human CD45RA-CD25-CD127- CD4+ T cells produced IL-10 and IFN-γ upon in vitro stimulation, and that these cells were suppressive (28). Interestingly, these cells appeared to have recently undergone activation in vivo, as they displayed high expression of Ki67, ICOS, HLA-DR, and CTLA-4. Despite their Ki67 expression, these cells were not proliferative; as with classically described Tr1 cells, the proliferative defect was rescued with IL-2 treatment. Near the same time, another group demonstrated that LAG-3 expression identified a population of murine CD25- CD4+ T cells which produced IL-10 and low amounts of IL-2, IL-4, IL-5, IFN-γ, and TGF-β (29). Like Tr1 cells, the CD25-LAG-3+ CD4+ T cells were anergic and capable of suppressing colitis. This phenotype was further refined once transcriptomic analyses were performed on Tr1 clones, revealing that CD49b and LAG-3 were uniquely co-expressed by Tr1 cells (27). Sorting of memory CD49b+LAG-3+ CD4+ T cells from both mice and humans revealed that these were the major IL-10-producing subset of CD4+ T cells, and they were highly suppressive in vitro compared to other subsets, including CD49b-LAG-3+ cells, suggesting that these two markers accurately identify Tr1 cells when used together. CD49b+LAG-3+ CD4+ T cells were also enriched in the blood of thalassemic patients who developed persistent mixed chimerism after allogeneic stem cell transplantation (27). Intestinal Tr1 cells from mice and humans also co-express CCR5 and PD-1 (30), and murine CCR5+PD-1+ cells are nearly all CD49b+LAG-3+, suggesting these markers work equally well and can likely be used together to more precisely identify endogenous Tr1 cells. Other markers can also aid in the identification of Tr1 cells. For instance, CD226 is highly expressed on Tr1 cells and is important for Tr1-mediated suppression (12). Staining for other co-inhibitory receptors such as Tim-3, TIGIT, and CTLA-4 can also aid in identifying Tr1 cells (31). Beyond being markers for Tr1 cells, LAG-3 and TIM-3 both have minor roles in Tr1-mediated suppression (31). This is consistent with FOXP3+ Tregs which have increased suppressive capacity when expressing LAG-3 or TIM-3 (32, 33). Discovery of these markers has permitted rapid progress in the field, but challenges remain. Indeed, many of the markers used to identify Tr1 cells can be induced transiently in cells which adopt a suppressive state (23). Despite this progress, a uniform definition of Tr1 cells still eludes the field, in large part due to the lack of a described lineage-defining transcription factor. Several transcription factors such as cMaf and Blimp-1 were shown to be essential for murine Tr1 differentiation and function (34, 35), but only a couple of these have findings (such as AHR/cMaf regulation of IL-10 production (36)) have been recapitulated in human Tr1 cells. AHR and IRF4 contribute to the suppressive capacity of Tr1 cells (37), but the involvement of these transcription factors in human Tr1 differentiation remains unknown. Eomes is the only transcription factor that has been demonstrated to play a role in the differentiation of a subset of human Tr1 cells (11, 38, 39). However, bulk RNA sequencing revealed that Eomes was not differentially expressed in circulating Tr1 cells compared to non-Tr1 memory CD4+ T cells (26), so it is unlikely to be a lineage-defining transcription factor for all Tr1 cells, and instead may only identify a subset of Tr1 cells. The discovery of a universal lineage-defining transcription factor will be a huge leap forward for the field. However, even lineage-defining transcription factors can be insufficient to identify a population. For instance, using FOXP3 to identify Tregs is unreliable, as human effector CD4+ T cells upregulate both CD25 and FOXP3 following stimulation; discovery of an epigenetic marker for FOXP3+ Tregs overcame this problem (40). Recent studies of Tr1 cells in a mouse model have begun exploring the epigenetic profile of murine Tr1 cells (41), but a unique epigenetic marker has not yet been identified.
Since Tr1 cells are induced in the periphery (29) and are therefore antigen-experienced, they are thought to be memory CD4+ T cells and behave as such. Evidence to the contrary was introduced in an airway allergy model in which Tr1-like cells substantially reduced airway inflammation in an acute inflammatory setting but showed no role during re-challenge despite being present as resident memory cells (42). The conclusion that Tr1-like cells did not contribute to tolerogenic memory was primarily based on the finding that inflammation was not enhanced in the recall response upon the in vivo depletion of IL-10-producing T cells. However, there were several caveats for this finding: first, the recall response was assessed only one day after challenge and a protective role of Tr1-like cells may not be visible or necessary that early in a recall response; second, the difference in frequency of Tr1-like cells between control or depleted animals was substantially less in the recall challenge, whereas there was a great difference in the frequency of IL-10-producing cells in control animals during acute infection – again, this could be reflective of the kinetics of Tr1-like cell activation and expansion; third, the activation of Tr1-like cells may be context-specific in vivo and there could be a threshold of inflammation to trigger Tr1 cell activation, such as when excessive tissue damage is present; and fourth, the Tr1 response during primary infection could contribute to tolerogenic memory via limiting the formation of pathogenic Th2 resident memory cells, and depletion of Tr1-like cells immediately prior to re-challenge would not affect this phenomenon. Further efforts are required to determine whether Tr1 cells contribute to tolerogenic memory in allergy models. In the context of influenza vaccination, a population of lung-resident memory Tr1 cells are induced and further expand upon re-challenge, though a protective role for Tr1 memory cells was not assessed as their presence was an unexpected finding (43). In other instances, a role for memory Tr1 cells in promoting tolerance has been demonstrated. For instance, gliadin-specific Tr1 cells exist in the intestinal mucosa of celiac disease patients during disease as well as in the absence of cognate antigen (44),. These Tr1 cells responded to cognate antigen and robustly secreted IL-10. The longevity of Tr1 cells has also clearly been demonstrated in humans, both in the SCID patient where Tr1 cells were first discovered to be contributing to long-term tolerance (3) but also in patients treated with a Tr1-enriched cell therapy product for the prevention of graft-versus-host disease (GvHD) in conjunction with allogeneic stem cell transplantation to treat high-risk myeloid malignancies (14). In fact, TCR sequencing of a Tr1-enriched cell therapy product before infusion and of peripheral CD4+ T cells at various timepoints post-infusion revealed that Tr1 clones which were present at infusion could still be detected in circulation one year later (14). Current data suggest that Tr1 cells indeed contribute to tolerogenic memory, though more efforts should focus on mechanisms and kinetics of tolerogenic memory responses elicited by Tr1 cells.
The generation of Tr1 cells in vivo likely occurs through multiple mechanisms involving presentation of both autoantigens and persistent foreign antigens accompanied by signaling from APCs (10, 28, 29, 45–47). There is substantial evidence demonstrating that IL-10-producing APCs promote Tr1 development in vivo. The first indirect evidence came from the SCID patient in which Tr1 cells were discovered; monocytes from this patient highly expressed IL-10 mRNA (3). Indeed, IL-10+ monocytes have been demonstrated to induce a protective Tr1-like population in vivo during viral infection (48). Other IL-10-producing APCs such as IL-10-producing tolerogenic dendritic cells (DC-10), tolerogenic dendritic cells expressing the scavenger asialoglycoprotein receptor (ASGPR), and macrophages play important roles in tolerance in vivo by promoting Tr1 differentiation (46, 49, 50). Despite the prominent role of IL-10 which was clearly demonstrated in Tr1 differentiation, there were cases in which Tr1 cells were differentiated in the absence of exogenous IL-10 due to non-classical co-stimulation such as through CD2, CD55, ICOS, or B7H1 (51–54). However, the extent to which these pathways contribute to Tr1 development in vivo remains uncertain. Finally, co-stimulation through CD46 has also been described to induce Tr1-like cells (22, 55–57), but these are likely IL-10-producing Th1 cells instead of bona fide Tr1 cells [reviewed in (21)]. While distinct, it is important to mention that IL-10-producing Th1 cells can also contribute to peripheral tolerance. Irrespective of its role in inducing Tr1 differentiation, murine and human Tr1 cells require IL-10 signaling to function (54, 58). Beyond IL-10, other cytokines have been implicated in Tr1 differentiation as well. IFN-α complements IL-10 to promote Tr1 cells in vitro (59). Similarly, induction of functional Tr1 cells through co-stimulation with B7H1 required IFN-γ (54). This is in line with a recent report which suggested IFN-γ signaling was vital for Tr1 cell formation in vivo (60), though this remains to be seen in human. Extensive studies have been carried out on the role of IL-27 in regulating IL-10 and promoting Tr1 cell differentiation, but this has almost exclusively been performed in mouse models (61, 62). It was recently reported that IL-27 had only modest effects on IL-10 production in human CD4+ T cells (63). However, IL-27 promoted IL-10 production by Tr1-like cells overexpressing the transcription factor, EOMES (11). Accordingly, the effect of IL-27 on human Tr1 cell induction remains poorly characterized. Similar to IL-27, TGF-β contributes to murine Tr1 cell differentiation (61) but data from experiments with human cells do not support a role for TGF-β signaling in Tr1 differentiation (59, 64). Beyond these extensively studied cytokines, other soluble mediators such as melatonin can induce Tr1-like cells (37, 65). Taken together, the existing data strongly suggest that Tr1 cells differentiate following stimulation by tolerogenic APCs in the presence of IL-10 with possible roles for non-conventional co-stimulation and other soluble mediators. With recent advances in epigenetic tracking and gene editing of human CD4+ T cells (66–68), it is likely that the biology governing Tr1 cell differentiation will become more transparent.
Ultimately, the field suffers from the lack of a universal definition of Tr1 cells. Based on the wealth of data available, we consider Tr1 cells to be memory CD4+ T cells that suppress pathogenic immune responses in a TCR-dependent manner. They are FOXP3- and have high expression of co-inhibitory receptors in addition to CD49b, CCR5, CD2, CD18, and CD226. They produce high amounts of IL-10 and TGF- β, intermediate amounts of IFN- γ and IL-5, and little to no IL-2, IL-4, and IL-17. Tr1 cells also secrete IL-22 which could be important in tolerance by mediating tissue repair (69, 70), and this could be another useful cytokine to identify Tr1 cells. Activated Tr1 cells are also cytotoxic and can eliminate myeloid cells through the secretion of perforins and granzymes. These criteria are summarized in Figure 1.
Figure 1 Hallmark Features and Functions of Tr1 Cells. Tr1 cells display high surface expression of CD49b, CCR5, CD2, CD18, CD226, and many co-inhibitory receptors such as LAG-3, PD-1, TIM-3, CTLA-4, and PD-L1. Tr1 cells characteristically secrete large amounts of IL-10 and TGF-β, intermediate amounts of IFN-γ and IL-5, and little to no IL-2 and IL-4. Tr1 cells have also been recently described to produce IL-22. Tr1 cells can suppress pathogenic immune responses through various mechanisms such as impairing antibody production by B cells, inducing PD-L1-mediated apoptosis in B cells, direct cytolysis of myeloid antigen presenting cells, reducing antigen presenting capacities by antigen presenting cells, induction of infectious tolerance, and direct inhibition of effector T cell responses through cytokine- and contact-dependent mechanisms. Created with BioRender.com.
Tr1 cells are present in the circulation of healthy individuals (27) and also reside in tissues such as healthy colon (30, 31) and tonsils (15). Additionally, DC-10 cells were found in human decidual tissue where they are poised to give rise to Tr1 cells during pregnancy (71), and a population of Tr1-like cells was recently found in human decidual tissue, thereby suggesting Tr1 cells may contribute to fetal-maternal tolerance in an IL-10-dependent manner (72). Tr1 cells isolated from peripheral blood were activated in response to persistent antigens of self and foreign origin but were not activated in response to vaccine-associated antigens (28). In one such instance, islet-specific Tr1 cells were identified in non-diabetic individuals and were able to suppress antigen-specific effector T cell responses by eliminating APCs (10), thus representing a way Tr1 cells in health may prevent the onset of diabetes. Tr1 cells can be specific for many foreign antigens, but a substantial population is induced in the periphery following exposures to antigens associated with commensal microbes (29). Findings from IL-10-deficient mice revealed the importance of tolerance to commensal bacteria, as they only developed colitis when colonized with intestinal microbes (73). Much of what we know about Tr1 cells in health comes from studies correlating the frequency of Tr1 cells in healthy donors to those with inflammatory and autoimmune diseases. Intriguingly, the frequency of Tr1 cells amongst IL-10-producing cells is substantially reduced in ulcerative colitis (UC) and Crohn’s disease (CD) patients (30, 31). Desmoglein-3-specific Tr1 cells were found more frequently in healthy donors than in patients with pemphigus vulgaris, suggesting a protective role for Tr1 cells in prevention of the disease (45). Likewise, Tr1 cells were found more frequently in peripheral blood and synovial fluid of healthy donors and osteoarthritis patients than in rheumatoid arthritis (RA) patients, suggesting a protective role in the development of RA (74). Indeed, the frequency of Tr1 cells and Th1 cells at the site of inflammation was inversely correlated in RA patients. Taken together, these data indicate that Tr1 cells in healthy individuals protect against the development of autoimmune and inflammatory disease.
AIDs are a group of more than 80 disorders in which autoreactive immune cells are unable to distinguish self from non-self, resulting in highly inflammatory immune responses directed toward tissues containing the antigen. Globally, it is estimated that 3-5% of people have an AID and the incidence is increasing (75). In patients suffering from AIDs, regulatory T cells, including Tr1 cells, are unable to prevent this break of tolerance in affected tissues. For many of the AIDs, the antigens that are targeted by the host immune system are known and therefore antigen-specific Tr1 cell therapies can be designed. Such therapies could be a promising alternative to the current standard of care since general immunosuppressive drugs and biologicals may possibly result in increased susceptibility to infectious diseases. More recently, autoantigen-containing nanoparticles (NPs) have shown promising results to induce tolerance in vivo and the use of these biomaterials avoid high standards of manufacturing for cell therapies. In this section, we will focus on the role of Tr1 cells in T1D, MS, and other AIDs and how they can be applied to cure patients. The roles of Tr1 cells in various autoimmune and inflammatory diseases are summarized in Table 1.
T1D is characterized by a mainly Th1-mediated inflammatory reaction targeted towards insulin-producing β-cell islets in the pancreas, resulting in hyperglycemia and many co-morbidities. Interestingly, the HLA-DRB1*0401 haplotype is associated with development of multiple AIDs, including T1D and MS. T1D patients display a shift towards the presence of proinflammatory IFN-γ-producing Th1 cells while in healthy donors a strong bias towards protective IL-10 production can be observed upon islet peptide stimulation (81). Moreover, first degree relatives of T1D patients show a similar frequency of IFN-γ- and IL-10-producing T cells using ELISPOT assays although their T cells produce more IL-10 spot-forming units (SFUs). This indicates that, regardless of IFN-γ secretion, the ability of T cells to produce sufficient levels of IL-10 is an important factor for tolerance to pancreatic peptides (82). The idea that Tr1 cells, as most potent producers of IL-10, are important for T1D protection is further illustrated by the correlation of IL-10-producing CD4+ T cells at disease onset and future blood glucose levels (83). Interestingly, patients suffering from juvenile T1D have more protective antigen-specific Th2/Tr1 clones compared to inflammatory Th1 clones, which is in stark contrast to adult T1D patients (84). Early in vivo studies showed that anti-CD3 treatment induces tolerance in non-obese diabetic (NOD) mice, but this approach was largely ineffective in humans and was often accompanied by cytokine release syndrome caused by the crosslinking of the anti-CD3 FcR with autoreactive T cells (101). Therefore, Herold et al. designed an anti-CD3 antibody having an FcR with reduced binding capacity which resulted in an IL-10-mediated induction of tolerance in T1D patients (85). Interestingly, intestinal Tr1 cells may be an important source of these in vivo induced protective IL-10-producing CD4+ T cells as Tr1 cells that arise in the small intestine protected NOD/SCID mice from T1D development upon co-transfer with diabetogenic BDC2.5 T cells (76). To further support this finding, rectally injected Tr1 cells were able to migrate to the pancreas to prevent T1D onset. Tr1 cells isolated from the gut mucosa, the main site of Tr1 induction, are thus able to confer protection to autoreactive T cells in a T1D context and possibly other AIDs (76). Since the use of anti-CD3 antibodies resulted in undesired side effects, other approaches for inducing tolerance in T1D were investigated. Rapamycin, known to induce and maintain FOXP3+ Tregs, was used in combination with IL-10 to induce tolerance to insulin-producing pancreatic islets in the NOD mouse model (77). In these mice, splenic CD4+ T cells produced larger amounts of IL-10 which thus could be the cause of a significant delay in diabetes onset. Furthermore, Tr1 cells isolated from the spleens of these mice suppressed the proliferation of diabetic splenocytes and transfer of pancreatic islets from treated mice to NOD/SCID mice showed that these T cells were indeed protective. Injection of IL-10 alone did not protect NOD mice from diabetes which might indicate that rapamycin first needs to reduce effector T cell proliferation before IL-10 is able to maintain a stable Tr1 cell population, as illustrated by a synergistic effect of rapamycin and IL-10 treatment. These studies show that both anti-CD3 treatments and the use of rapamycin and IL-10 might induce tolerance mediated by polyclonal Tr1 cells. Importantly, however, these in vivo induced polyclonal Tr1 cells might result in infectious tolerance and therefore more recent in vivo studies investigated the potential of autoantigens to generate antigen-specific Tr1 cells for peptide immunotherapy. Chen et al. utilized the 65 kDa glutamic acid decarboxylase (GAD65) to immunize NOD mice to establish antigen-specific Tr1-mediated tolerance (78). Co-transfer of diabetogenic T cells and Tr1 clones into lymphopenic NOD/SCID hosts resulted in protection from T1D in an IL-10-dependent manner. A study in T1D patients illustrated that immunotherapy using the immunodominant proinsulin peptide resulted in a decrease in insulin dependency which thus could be attributed to tolerance induction although the precise mechanism was not elucidated (86). Rather than injecting soluble peptides, NPs capable of encapsulating disease-causing peptides are a valuable alternative for treating AID patients. Using NPs harboring multiple cognate diabetogenic peptides, Prasad et al. demonstrated that this approach induced tolerance upon adoptive transfer of T1D-specific proinflammatory T cells (79). Although Foxp3+ Tregs were increased in both the spleen and the pancreas of host mice, splenocytes produced increased levels of IL-10 which might implicate a possible role for CD49b+LAG-3+ Tr1 cells. Another study exploiting the same approach found similar results using NPs containing insulin peptides, which is the cognate antigen of the BDC2.5 TCR-transgenic mouse model. Adoptive transfer experiments again resulted in the protection from T1D in an antigen-specific manner as illustrated by tetramer staining on antigen-specific regulatory T cells (102). The formal proof that tolerogenic Tr1 cells are induced in vivo upon administration of antigen-containing NPs came from a study in which multiple diabetogenic peptides were loaded onto recombinant MHC-II (pMHC-II) (80). G6pc2-/- NOD mice treated with these pMHC-II NPs showed an expansion of pre-existing Tr1 cells since only BDC2.5 memory T cells were protective upon adoptive transfer of NOD/SCID mice whereas their naïve counterparts were not. Interestingly, from a translational approach, viral challenge of pMHC-II NP-treated mice resulted in protection from both T1D and viral infections. Adoptive transfer experiments using DR4-specific peripheral blood mononuclear cells (PBMCs) isolated from T1D patients also resulted in an increase in diabetes-free survival upon co-injection of pMHC-II NPs. These results highlight that antigen-specific Tr1 cells induced by pMHC-II NPs can mediate tolerance while still allowing a potent immune response towards pathogens.
Tr1 cells also play a key role in MS. MS is an AID characterized by an excessive inflammatory immune response in which autoreactive T cells target the central nervous system (CNS) white matter. Early reports investigating the role of Tr1 cells in MS pathogenesis showed that an alteration in the expression of the isoforms of the co-stimulatory molecule CD46, important for a shift from a proinflammatory to an anti-inflammatory immune response, resulted in a reduced ability to induce IL10-producing CD4+ T cells in MS patients (91). Moreover, a follow-up study showed that impaired glycosylation of CD46 upon TCR stimulation in MS patients prevented the shift from IFN-γ-producing T cells toward IL-10-secreting T cells (57). Barrat et al. were among the first to show that IL-10-producing CD4+ T cells were able to protect from experimental autoimmune encephalitis (EAE), a mouse model of MS (87). Here, the combination of vitamin D3 (VitD3) and dexamethasone (Dex), both known to be immunosuppressive, enriched for IL-10-producing CD4+ T cells in vitro. Of note, these cells were presumably not a homogenous population of Tr1 cells as they did not produce IFN-γ which is a hallmark cytokine of bona fide Tr1 cells. To demonstrate the antigen-specificity of these IL-10-producing CD4+ T cells, EAE mice were first intracranially injected using OVA and subsequently injected with OVA-specific VitD3/Dex-induced Tr1-like cells. These cells were able to significantly reduce both the EAE clinical score and T cell infiltration in the CNS in an antigen-specific manner. Another study investigated the potential of IL27-pulsed dendritic cells (DCs) to induce tolerance in an EAE mouse model (88). These IL27-primed tolerogenic DCs were able to upregulate IL-10 secretion in Tr1-polarizing cultures using naïve 2D2 CD4+ T cells, a population isolated from TCR-transgenic mice specific for myelin oligodendrocyte glycoprotein (MOG). Vaccination of EAE mice with IL27-conditioned DCs showed to improve EAE although the specific role of Tr1 cells was not addressed in these experiments (88). Interestingly, however, Il27ra knockout mice showed an increase in EAE clinical score and a decreased production of IL10-producing splenic CD4+ T cells which might indicate a loss of Tr1 cells due to the absence of tolerogenic DCs. Of note, although the impact of IL-27 signaling on the induction of IL10 expression through Blimp-1 and c-Maf during Tr1 differentiation has been demonstrated in mice, similar results have not been observed in human (35). Evidence that Tr1 cells are instrumental for tolerance in EAE came from a study that immunized mice using the autoantigen MOG in the presence of retinoic acid and IL-2 as adjuvants (89). While these mice had an increase in IL-10-producing CD49b+LAG-3+ Tr1 cells that protected from EAE progression due to a decrease in Th17 and Th1 cells, FOXP3+ Treg abundance was not altered. Of note, the same experiments were also performed in Il10-/- mice which showed that although Tr1 cells mainly inhibit immune responses via IL-10 secretion, these mice were still protected from EAE. This finding might indicate that, in MS, Tr1 cells may contribute to immunomodulation via alternative mechanisms or they might instruct other tolerogenic cells to mediate tolerance but studies in human should be performed. Another interesting study showed that melatonin has the potential to reduce disease severity in MS patients as melatonin is an important mediator of disease activity resulting from seasonal changes. Indeed, administration of melatonin in multiple EAE models increased IL-10 production by Tr1 cells while Th17 differentiation was inhibited and thus the use of melatonin could be an interesting avenue for tolerance induction (65). From a translational perspective, the use of NPs in EAE also proved to protect mice from disease in an antigen-specific manner. In an early report, myelin epitope-containing microparticles prevented EAE development via uptake through the macrophage receptor with collagenous structure (MARCO) which eventually resulted in a reduction of pathogenic IL-17+ and IFN-γ+ CD4+ T cells. However, the possible impact of Tr1 cells to restore balance in these mice was not investigated (103). Next to the use of pMHC-II NPs to treat T1D in mice, Clemente-Casares et al. also investigated the use of pMHC-II NPs containing MOG to prevent EAE progression (80). Here, the authors found that antigen-specific CD49b+LAG-3+ Tr1 cells were increased in treated mice with concomitant absence of demyelination of white matter in the cerebellum compared to untreated controls. Finally, Kenison et al. used nanoliposomes (NLPs) that contain both the MOG peptide and an aryl hydrocarbon receptor (AhR) agonist to establish tolerance in EAE mice via induction of tolerogenic DCs (90). Indeed, these NLPs were able to generate antigen-specific IL-10-producing CD4+ T cells which mediated the reduction of IL-17+ and IFN-γ+ pathogenic T cells. However, the presence of Tr1 cells by validation of CD49b and LAG-3 expression was not investigated.
In addition to T1D and MS, Tr1 cells also promote tolerance in other AIDs such as systemic lupus erythematosus (SLE), RA, psoriasis, and celiac disease. Similar to what has been observed in MS patients, patients with lupus nephritis, which is a major cause of SLE, the CD46 co-stimulatory pathway was affected and prevented the conversion of Th1 to IL-10-producing Th1 cells (24). Interestingly, it has also been shown that although Tr1 cells are more abundant in the peripheral blood compared to healthy donors, Tr1 cells isolated from SLE patients are unable to restrict IgG production by B cells which indicates that Tr1 cells play a crucial role in limiting autoantibody production by autoreactive B cells (16). This finding was further supported by experiments in which co-transfer of B cells and Tr1 cells in OVA-immunized Rag1-/- mice resulted in a decrease of OVA-specific IgG production of the transferred B cells. However, their protective role in MS might not be strictly attributable to IL-10-mediated tolerance induction since a recent publication demonstrated that IL-10 produced by CXCR5-CXCR3+PD-1high CD4+ T cells stimulates antibody production by autoreactive B cells (92). Also, in a mouse model of RA, there was evidence that Tr1 cells are key to instruct tolerance towards RA autoantigens (93). This work demonstrated that Col-Tregs (collagen type II-specific Tr1 cells), induced ex vivo by the incubation of collagen type II TCR-specific splenocytes with IL-10 and collagen type II antigen, resulted in the generation of RA-specific Tr1 cells capable of reducing erosion, cell infiltration, B cell response and hyperplasia in the paws from arthritic mice. These Col-Tregs also produced IFN-γ which might indeed indicate that these cells are enriched for bona fide Tr1 cells. In psoriasis, Tr1 cells might also play an important role in preserving tolerance. Kim et al. found that psoriasis patients, in contrast to SLE patients, had less CD49b+LAG-3+ Tr1 cells in the peripheral blood and this observation correlated to disease severity (94). Furthermore, the authors illustrated that Tr1 cells were present in non-lesional skin while in psoriatic lesions there was an increase in infiltrating T cells and here Tr1 cells could not be detected, leading to the idea that the absence of Tr1 cells allows Th1-mediated inflammation of the skin. Finally, Tr1 cells are also implicated in celiac disease, a condition characterized by the intolerance to gluten in the gut of affected individuals. An important study by Gianfrani et al. generated gliadin-specific IL-10-producing cells by culture of cells from patient-derived mucosal explants on a PBMC feeder in the presence of the gliadin antigen and IL-10 (44). These clones produced large amounts of IL-10 and IFN-γ and, importantly, were anergic to gliadin-specific stimulation which is an important feature of Tr1 cells. Moreover, these gliadin-specific IL-10-producing clones inhibited the proliferation of responder T cells in an antigen-specific fashion. Recently, a double-blinded placebo-controlled clinical trial using TAK101, a NP containing a multi-epitope gliadin extract, showed to induce tolerance with a reduction in pathogenic effector T cells in patients which paves the way for novel and less toxic therapies for celiac disease (101).
In summary, it has been shown using in vivo models that Tr1 cells are critical to protect from T1D and MS development while their role in other AIDs has not been fully investigated. IL-10 plays a prominent role in establishing tolerance in SLE, RA, psoriasis, and celiac disease which might indicate that bona fide CD49b+LAG-3+ Tr1 cells are involved but this requires more investigation. Induction of antigen-specific tolerance in AIDs, either via cell therapy or other modalities, would transform the therapeutic management of these diseases.
IBD comprises a group of severe diseases of the gastrointestinal (GI) tract in which an autoinflammatory immune response is mounted against enteric antigens in genetically susceptible hosts. Over the past few decades, the prevalence of IBD, including very early inflammatory bowel disease (VEO-IBD), CD, and UC, has substantially increased mainly in western and industrialized countries (104). IBD is a complex and multifactorial disease mainly characterized by severe colitis and weight loss with important co-morbidities. In the intestinal microenvironment of IBD patients, many key players of the immune system target otherwise harmless enteric and environmental antigens in which both FOXP3+ Tregs and Tr1 cells have lost their ability to suppress these undesired immune responses. Here, we focus on the role of Tr1 cells in IBD and their immunosuppressive abilities via the production of IL-10.
IL-10-producing cells are mainly found in the in the lamina propria of the GI tract (105–107). Although Tr1 cells produce high amounts of IL-10 and have broad immunosuppressive properties, it recently has been demonstrated using adoptive transfer of IL-10+FOXP3- Tr1 cells in lymphopenic hosts that there is substantial spatial heterogeneity among Tr1 cells. For instance, IL-10+FOXP3- splenic Tr1 cells are less protective against colitis development in contrast to their intestinal counterparts (31). The heterogeneity in IL-10-producing cells is further reflected in single cell studies using gut biopsies from IBD patients in which only a small cluster of IL-10-producing lymphocytes represents bona fide Tr1 cells, characterized by high expression of multiple co-inhibitory receptors (31). In general, Tr1 cells isolated from IBD patients produce less IL-10 than their FOXP3+ Treg counterparts upon ex vivo stimulation, which highlights that loss of Tr1-specific IL-10 is an important mediator of IBD (23, 30). Importantly, it has also been shown that proinflammatory cytokines implicated in IBD, such as IL-23 and IL-1β, cause this loss of IL-10 secretion by Tr1 cells which illustrates that the proinflammatory microenvironment present in IBD patients prevents Tr1-mediated tolerance (30).
The first formal evidence of the protective role of IL-10-producing Tr1 cells was provided by Groux et al. Here, it was demonstrated that clonally expanded OVA-specific Tr1 cells were able to prevent colitis in the CD4+CD45RBhigh adoptive transfer mouse model in an antigen-specific manner (4). Of note, these findings might also indicate that Tr1 cells could potentially provide bystander suppression to enteric antigens (108). The crucial role of IL-10 in gut tolerance was further shown by the inability of Il10-deficient CD4+CD45RBlow T lymphocytes, containing both Tr1 and FOXP3+ Treg cells, to restore homeostasis in the bowels of mice that were injected using inflammatory CD4+CD45RBhigh T cells (96). Importantly, in the same study, injections of IL-10 did not ameliorate IBD symptoms which highlights the need for T cell-derived IL-10 to induce long-term tolerance in IBD patients (96). Studies using this CD4+CD45RBhigh adoptive transfer model also demonstrated the role of gut microbiota in shaping intestinal tolerance since CD4+CD45RBhigh T cells isolated from germ-free mice were unable to induce colitis in hosts (109). Interestingly, these adoptive transfer studies in mice are performed using polyclonal T cells which indicates that these cells might induce de novo antigen-specific Tr1 and Treg cells for long-term tolerance in IBD. Since the CD4+CD45RBlow subset contains both Tr1 cells and FOXP3+ Treg cells, FOXP3+ Treg cells may also mediate tolerance via IL-10 and this has been elegantly shown by a Foxp3CreIl10LoxP mouse model that indeed showed intestinal inflammation (110). Interestingly, the intestinal inflammation observed in these Foxp3CreIl10LoxP mice is very similar to the gut pathology of Il10-/- mice while inflammation of the skin is only seen in the latter which might indicate that homeostasis in the skin is mainly mediated by Tr1 cells and myeloid cells, such as macrophages and DCs (111). Combined, these studies thus show that although Tr1 cells produce large amounts of IL-10, the total level of IL-10 produced by intestinal T cells needs to reach a certain threshold to maintain tolerance. This has been further demonstrated by a study in which both FOXP3+ Tregs and Tr1 cells control Th17-mediated colitis in an IL-10-dependent manner as pathogenic Th17 cells expressing a dominant negative form of the IL-10 receptor were unresponsive to IL-10-mediated suppression (97). In addition, IL-10 also plays a critical role in amplifying the immunosuppressive role of tolerogenic T cells as loss of the IL-10 receptor on both FOXP3+ Tregs and Tr1 cells results in the loss of suppression of pathogenic Th17 responses in the gut (58, 112). Here, the loss of IL-10 signaling in Tr1 cells only resulted in a decrease of IL-10 secretion while other immunosuppressive mechanisms such as CTLA-4 and Granzyme B function were unaffected. This finding thus indicates that IL-10 is crucial for both dampening the production of inflammatory cytokines by pathogenic T cells and for amplifying the immunosuppressive ability of Tr1 cells and FOXP3+ Tregs (58). However, one study showed that only injection of OVA-specific Tr1 cells, and not FOXP3+ Tregs, multiple weeks after colitis onset resolved intestinal inflammation which indicates that mainly Tr1 cells are able to expand in vivo to control inflammation and ongoing disease and ongoing disease (90).
Studies in human and mice have clearly shown that Tr1 cell therapy holds great promise as an alternative treatment capable of inducing long-term tolerance in the bowels of IBD patients. For instance, the use of autologous OVA-Tr1 cells has been tested in a phase I/IIa clinical trial with a maximum effect 5 weeks after administration but follow up studies were never performed (NCT02327221) (100). A more recent study by Cook et al. showed that polyclonal activation of CD4+ T cells isolated from the peripheral blood of IBD patients enabled the ex vivo expansion of IL-10-producing CD49b+LAG-3+ Tr1 cells (69). These clonally expanded Tr1 cells were able to reduce the secretion of the proinflammatory cytokines TNF-α and IL-1β by myeloid cells. In addition, these Tr1 cells not only secreted IL-22 which promoted barrier integrity of epithelial cell lines, but they were also able to induce mucin production by goblet cells in patient-derived gut organoids, both features that were not seen with expanded FOXP3+ Tregs (69). These preliminary results demonstrate that Tr1 cells, rather than FOXP3+ Tregs, might be promising as a novel cell therapy for IBD patients. Next to these ex vivo expanded Tr1 cells, tolerogenic DCs might also represent an interesting approach for resolving IBD. Administration of GSK-J4, a histone demethylase inhibitor, resulted in the retinoic acid-mediated induction of tolerogenic DCs in the dextran sulfate sodium (DSS) colitis mouse model with concomitant production of IL-10 by CD4+ T cells (98). However, the induction of CD49b+LAG-3+ Tr1 cells was not shown in this model and since GSK-J4 might affect other important pathways as a broad epigenetic modifier, the use of this compound might result in multiple undesired off-target effects (98). Finally, many clinical trials are now investigating the potential use of mesenchymal stem cells (MSCs) in reducing IBD severity. Using the 2,4,6-trinitrobenzenesulfonic acid (TNBS) colitis mouse model, it has been shown that MSCs are capable of expanding the existing Tr1 pool. However, co-expression of CD44 and IL-10 was used to define these putative Tr1 cells while the presence of both CD49b and LAG-3 was not shown (99).
As the most important source of IL-10 in the gut, Tr1 cells play a non-redundant role in the protection from IBD in human. Together with other genetic risk, the composition of the gut microbiome is an important factor in the onset and progression of IBD. IBD patients that have mutations in the IL10 or IL10R genes suffer from very severe intestinal disease which indeed highlights that Tr1-mediated IL-10 signaling maintains tolerance to commensal microbiota in the gut. The enteric antigens that induce IBD have been extensively studied but the bacteria that are implicated might differ substantially between individuals. Nevertheless, future clinical trials investigating the use of polyclonal Tr1 alone or together with anti-inflammatory drugs might prove promising to induce long-term tolerance in the bowels of affected patients.
While it is very well established that Tr1-mediated tolerance confers protection in autoimmune contexts, the role for Tr1 cells in infectious disease is ill-defined. Shortly after their discovery, research began to address whether Tr1 cells are friend or foe in infectious disease, a context where the balance between inflammatory and anti-inflammatory responses is critical to permit pathogen clearance without excessive pathology. In a murine model of Bordetella pertussis infection, antigen-specific Tr1 cell clones were produced from lung-infiltrating T cells of infected mice (113). The resulting clones were suppressive in vitro and caused a small delay in viral clearance upon adoptive transfer in combination with Th1 cells. However, it was not demonstrated whether immunopathology was affected in this model. In contrast, a more recent paper demonstrated that Tr1 cells did not impair the immune response to acute viral infection (114). In this case, mice which were treated with Tr1 cells (LAG-3+ CD4+ T cells) to prevent allograft rejection were infected with lymphocytic choriomeningitis virus (LCMV), but viral clearance and generation of antigen-specific effector T cells was comparable to what was observed in non-transplanted/non-treated C57BL/6 mice. Consistent with this finding, human T cell populations containing allo-antigen-specific Tr1 cells proliferated effectively when challenged with foreign antigens and 3rd party APCs (14, 64). We previously demonstrated that CD49b+LAG-3+ Tr1 cells were induced in vivo during Nippostrongylus brasiliensis infection, though their emergence in the lungs and mediastinal lymph nodes corresponded with the resolution of inflammation after pathogen clearance (27). Similarly, Tr1 cells were recently shown to be present within the lung during influenza infection in mice (43). Remarkably, Omokanye et al. demonstrated that Tr1 cells exist within the influenza-specific lung-resident memory T cell population 6 months post-vaccination, and the Tr1 cells clonally expanded following re-challenge. Despite the induction of influenza-specific Tr1 cells in this model, mice were able to survive lethal infection with low morbidity (115). While no functional experiments were performed to establish a role for the Tr1 cells in this model, the authors speculated that the Tr1 cells likely serve as a mechanism to limit pathology within the lung during acute infection, similar to what has been observed for FOXP3+ Tregs during influenza infection (116–120). In support of this hypothesis, recent evidence suggests a protective role of Tr1-like cells in murine gamma herpesvirus infection in which infection induced IL-10-producing monocytes within the lung that led to generation of Tr1-like cells (48). Though the cells were not referred to as Tr1 cells, their single-cell RNA sequencing revealed these cells had a Tr1-like transcriptional profile exemplified by high expression of Gzmb, Gzma, Il10, Lag3, Maf, Ctla4, Id2, Nkg7, and Ccr5. Upon monocyte depletion, these cells were absent and instead a population of cytotoxic CD4+ T cells persisted and elicited severe tissue damage within the lungs, suggesting a protective role of Tr1-like cells generated by IL-10-producing monocytes during acute infection. Overall, Tr1 cells do not seem to hinder effective immune responses needed for pathogen clearance during acute infections but on the other hand exert a protective role against excessive inflammation and immune activation induced by the pathogen.
While the study of Tr1 cells in acute infection is rather limited, significant efforts have aimed at determining whether Tr1-mediated tolerance plays a part in recurrent or chronic infections. One of the first reports on the topic examined PBMCs from patients infected with Onchocerca volvulus who developed patent infection instead of sterilizing immunity (121). Hyporesponsiveness to antigen was driven by IL-10 and TGF-β, and T cell clones from one patient revealed a Tr1-like cytokine profile. Surprisingly, antigen-specific Tr1-like cell clones could not be generated from previously infected patients who developed immunity. In another setting, the frequency of Tr1 cells correlated with disease progression in HIV-infected individuals, but a causative link was not established, and more data are needed to determine whether Tr1 cells contribute to HIV pathogenesis (122). The frequency of Tr1 cells was also associated with a severe form of paracoccidioidomycosis, but the frequency of Tr1 cells in these patients was only slightly elevated compared to healthy controls and patients with a mild form of chronic infection (123). Conversely, patients with an acute form of paracoccidiomycosis had a substantial increase in circulating Tr1 cells. In the context of chronic viral infection, patients with chronic hepatitis C virus (HCV) infection presented with elevated levels of serum IL-10 (124). Using T cells from these patients, HCV core-specific cell lines and clones were generated which adopted either Th1 or Tr1 cytokine profiles. While no functional studies were performed to demonstrate the ability of the Tr1 cell lines and clones to suppress HCV-specific immune responses, similar work provided more insight. PBMCs from HCV-infected patients produced substantial amounts of IL-10 upon stimulation with another HCV protein, nonstructural protein 4, and IFN-γ production was minimal, but IFN-γ production was rescued in the presence of an IL-10-neutralizing antibody (125). However, it was then demonstrated that IL-10 production largely came from monocytes in this model and the monocyte-derived IL-10 was sufficient to suppress DC maturation and T cell responses to polyclonal and antigen-specific stimulations. Nevertheless, these data suggest that HCV-derived proteins could elicit antigen-specific Tr1 differentiation in vivo which could potentially impair effector responses against HCV, though this remains to be demonstrated. Several reports have explored the hypothesis that Tr1 cells contribute to HCV recurrence following liver transplantation but a direct causative role for Tr1 cells has not been demonstrated (126–129).
The most cohesive body of literature surrounding the role of Tr1-mediated tolerance during infectious disease arose in the context of malaria infection. Protective immunity to malaria develops slowly and follows repeated infection [reviewed in (130)]. This form of immunity is often referred to as “clinical immunity” and it does not constitute a sterilizing immune response (131). Children living in malaria-endemic settings have a much higher probability of asymptomatic infection following repeated exposure. Thus, clinical immunity is defined by health despite parasitemia. Children repeatedly exposed to malaria in endemic settings develop a substantial proportion of antigen-specific IL-10+IFN-γ+ CD4+ T cells (132), prompting the question whether Tr1-mediated tolerance may contribute to the development of clinical immunity to Plasmodium infections. Multiple studies utilizing mouse models of malaria infection demonstrated a protective role of Tr1-like cells. One study utilizing Plasmodium chabaudi chabaudi demonstrated that engraftment of wildtype but not IL-10-deficient CD4+ T cells protected Rag-/- mice from serious weight loss, hypothermia, and mortality following infection (133). A separate study using Plasmodium yoelii demonstrated that although Rag-/- mice engrafted with WT as opposed to IL-10-deficient CD4+ T cells had increased parasitemia following infection, these mice experienced less severe weight loss and a modest improvement in survival duration (134). These two studies demonstrate, using separate mouse models, that CD4+ T cell-derived IL-10 promotes health while potentially inhibiting parasite clearance. However, Tr1-like cells may not influence parasitemia to the same degree as FOXP3+ Tregs, which significantly increased parasite levels in the blood of Plasmodium yoelii-infected mice (134, 135). Additionally, FOXP3+ Tregs provided insufficient protection against pathology during malaria infection, and IL-10 from effector CD4+ T cells sufficiently limited pathology even in the absence of FOXP3+ Tregs (133). Thus, compared to Tregs, Tr1-like cells provide superior protection against immune-mediated pathologies while seemingly inhibiting anti-parasite immune responses to a lesser degree. As the latter report (133) claimed that the major source of protective IL-10 came from effector CD4+ T cells, it should be carefully considered whether these studies were looking at true Tr1 cells or IL-10-producing Th1 cells, which are distinct [reviewed in (21)]. While one group suggested the cells in their model were Tr1 cells and not IL-10-producing effectors based on the absence of IFN-γ, IL-4, IL-13, and IL-17a mRNA expression (136), recent single-cell RNA sequencing experiments in a mouse model of malaria revealed an emergence of Tr1-like cells from the Th1 lineage, but the transcriptomic profile between Th1 cells and Tr1-like cells were nearly identical, with only two genes besides Il10 being differentially expressed between the two cell subsets (137, 138). Indeed, it is well documented that effector T cells may upregulate IL-10 in inflammatory settings to self-limit inflammation [reviewed in (139)].
Beyond findings in the mouse, recent findings have shed light on the role of Tr1-like cells in human malaria. In endemic settings, the frequency of IL-10-producing CD4+ T cells correlated with the number of malaria exposures (132). In line with this finding, T cells were less proliferative when isolated from children with a higher incidence of infection compared to T cells isolated from children with less frequent infections. This proliferative defect was at least partially IL-10-dependent (132). Surprisingly, the frequency of Tr1-like cells in malaria-exposed children did not increase with age, while the frequency of malaria-specific Th1 cells did (140). Additionally, the frequency of IL-10+ CD4+ T cells correlated with protection against symptomatic infection and induction of asymptomatic infection (140), consistent with the hypothesis that Tr1 cells can protect against excessive pathology during infection and may contribute to clinical immunity. It was previously reported that the most suppressive population of IL-10-producing CD4+ T cells in mice are CD49b+, LAG-3+, and highly express co-inhibitory receptors such as TIM-3, TIGIT, CTLA-4, and PD-1 (31). A CTLA-4+PD-1+ population of CD4+ T cells was identified in the blood of patients who had recently travelled to malaria-endemic regions and became infected (141). These cells co-produced IFN-γ and IL-10 in an antigen-specific manner, but IL-10 production was not detected upon polyclonal stimulation. Conversely, the CTLA-4+PD-1+ cells suppressed effector T cell responses in vitro when stimulated with infected red blood cells or with anti-CD3/CD28, suggesting suppression elicited by these cells was not dependent on IL-10. Indeed, cell-cell contact was necessary for suppression. Notably, these cells proliferated in response to antigen, and they displayed rapid contraction when patients were treated with antimalarials, which is consistent with what was observed for IL-10-producing Th1 cells in a mouse model of Plasmodium infection (138). While the frequency of CTLA-4+ CD4+ T cells was elevated in patients with severe cerebral malaria, there was no correlation between the frequency of CTLA-4 or PD-1 expression and parasitemia (141). Whether these were Tr1 cells or a subset of effectors induced specifically in the context of malaria should be explored. Follow-up studies from this group demonstrated that both CD4+ T cells and CD8+ T cells from malaria-infected individuals adopted a Tr1-like phenotype and cytokine profile, illustrated by high expression of LAG-3 and co-inhibitory receptors accompanied by production of IL-10, IFN-γ, and granzyme B (142). While functional studies were not performed with the human cells, analogous cells were induced in a murine model of malaria infection (142). The murine LAG-3+ cells were suppressive irrespective of CD49b expression, and the frequency of LAG-3+ cells in various tissues dwindled to levels seen in naïve mice within days of anti-malarial administration, suggesting these were not long-lived memory cells. Unexpectedly, the co-inhibitory receptor-rich CD8+ T cells had enhanced antigen-specific cytotoxic function (142, 143). Based on the presently available evidence, it can be concluded that Tr1-like cells prevent symptomatic malaria infection by protecting against immune-mediated pathology, as demonstrated in mice and humans (133–135, 140). Whether the Tr1-like cells are bona fide Tr1 cells instead of Th1 cells expressing IL-10 remains to be seen, but regardless, the IL-10-producing CD4+ T cells in malaria limit disease-associated pathology and may contribute to clinical immunity.
Collectively, these data suggest that across different infection models and in both acute and chronic settings, Tr1 cells and Tr1-like cells limit immune-mediated pathology during infection. In some contexts, it is possible that Tr1-mediated tolerance could contribute to delayed pathogen clearance or favor recurrent infection, though more data are warranted to draw strong conclusions. Whether Tr1 cells inhibit pathogen clearance could also depend on the microenvironment governing their function during infection. Indeed, FoxP3+ Tregs exert different immunoregulatory functions at various stages of infection (119). Further studies should rigorously explore the role of Tr1 cells across the full duration of infection and in re-infection models to distinguish context-dependent roles of Tr1 cells during infection. This information will be vital as Tr1 cells receive more attention in clinical settings.
Tr1 cells have a vital role in promoting peripheral tolerance. However, they are not the only regulatory cell involved in tolerance, as natural and inducible FOXP3+ Tregs and other emerging regulatory cell types undoubtedly contribute [reviewed in (144)]. This begs the question: why do we have Tr1 cells? They could exist as a fail-safe for when other regulatory cells are insufficient to limit an immune response. For instance, in immunodysregulation polyendocrinopathy enteropathy X-linked syndrome (IPEX) patients, deleterious mutations in FOXP3 result in a failure of Tregs to suppress autoimmunity. Intriguingly, T cells from IPEX patients can differentiate into Tr1 cells (145), and increased frequencies of Tr1 cells correlate with diminished disease severity (146). Another reason Tr1 cells may exist is the broad antigen specificity Tr1 cells can acquire. FOXP3+ Tregs are generated in the thymus and recognize self-antigens, though the antigenic repertoire does not cover the entirety of self-antigens [reviewed in (147)]. Accordingly, Tr1 cells may fill the void by inducing tolerance to self-antigens which are not represented in the mosaic thymic antigen library. Tr1 cells can also mediate tolerance to persistent foreign antigens. Indeed, Tr1 cells have been identified that are specific for allergens such as Derp2 from house dust mite, Ara h1 and Ara h2 from peanuts, gliadin from gluten, bee venom, and commensal microbes (13, 29, 44, 47, 148–150). While Tr1 cells may potentially respond to a wider array of persistent antigens than FOXP3+ Tregs, there is no doubt that both cell types are critical in inducing and maintaining tolerance and they can cooperate to control disease through disparate mechanisms, thereby establishing tolerance when one cell type alone may be insufficient (44, 69, 72, 77, 151–153).
As our collective understanding of Tr1 biology continues to grow, we can appreciate their role in tolerance in various disease settings as well as possible limitations such as in infectious diseases. The suppressive function of Tr1 cells has been mostly investigated in T1D, cell and organ transplantation, and IBD models while their role in other autoimmune diseases has not been extensively investigated. By continuing to push forward, we can strive to uniformly characterize human Tr1 cells to ultimately use them as novel therapies for inducing tolerance in autoimmune and autoinflammatory diseases. Further knowledge will support the development of novel Tr1-based tolerogenic therapies and allow us to apply them in a safe and efficacious manner.
All authors contributed to the article and approved the submitted version.
The authors would like to thank Jason Nideffer for critical reading and thoughtful scientific discussions during the preparation of this review.
The authors declare that the research was conducted in the absence of any commercial or financial relationships that could be construed as a potential conflict of interest.
All claims expressed in this article are solely those of the authors and do not necessarily represent those of their affiliated organizations, or those of the publisher, the editors and the reviewers. Any product that may be evaluated in this article, or claim that may be made by its manufacturer, is not guaranteed or endorsed by the publisher.
1. Bluestone JA, Anderson M. Tolerance in the age of immunotherapy. New Engl J Med (2020) 383:1156–66. doi: 10.1056/nejmra1911109
2. Roncarolo MG, Yssel H, Touraine J-L, Bacchetta R, Gebuhrer L, de Vries JE, et al. Antigen recognition by MHC-incompatible cells of a human mismatched chimera. J Exp Med (1988) 168:2139–51. doi: 10.1084/jem.168.6.2139
3. Bacchetta R, Bigler M, Touraine J-L, Parkman R, Tovo P-A, Abrams J, et al. High levels of interleukin 10 production In vivo are associated with tolerance in SCID patients transplanted with HLA mismatched hematopoietic stem cells. J Exp Med (1994) 179:493–502. doi: 10.1084/jem.179.2.493
4. Groux H, O’Garra A, Bigler M, Rouleau M, Antonenko S, de Vries JE, et al. A CD4+ t-cell subset inhibits antigen-specific t-cell responses and prevents colitis. Nature (1997) 389:737–42. doi: 10.1038/39614
5. Bacchetta R, Sartirana C, Levings MK, Bordignon C, Narula S, Roncarolo MG. Growth and expansion of human t regulatory type 1 cells are independent from TCR activation but require exogenous cytokines. Eur J Immunol (2002) 32:2237–45. doi: 10.1002/1521-4141(200208)32:8<2237::AID-IMMU2237>3.0.CO;2-2
6. de Waal-Malefyt R, Haanen J, Spits H, Roncarolo M-G, te Velde A, Figdor C, et al. Interleukin 10 (IL-10) and viral IL-10 strongly reduce antigen-specific human t cell proliferation by diminishing the antigen-presenting capacity of monocytes via downregulation of class II major histocompatibility complex expression. J Exp Med (1991) 174:915–24. doi: 10.1084/jem.174.4.915
7. Ding L, Linsley PS, Huang LY, Germain RN, Shevach EM. IL-10 inhibits macrophage costimulatory activity by selectively inhibiting the up-regulation of B7 expression. J Immunol (1993) 151:1224–34.
8. Willems F, Marchant A, Delville J-P, Gerard C, Delvaux A, Velu T, et al. Interleukin-10 inhibits B7 and intercellular adhesion molecule-1 expression on human monocyt es. Eur J Immunol (1994) 24:1007–9. doi: 10.1002/eji.1830240435
9. Chang C-H, Furue M, Tamaki K. B7-1 expression of langerhans cells is upregulated by proinflammatory cytokines, and is down-regulated by interferon-y or by int erleukin-10. Eur J Immunol (1995) 25:394–8. doi: 10.1002/eji.1830250213
10. Tree TIM, Lawson J, Edwards H, Skowera A, Arif S, Roep BO, et al. Naturally arising human CD4 t-cells that recognize islet autoantigens and secrete interleukin-10 regulate proinflammatory t-cell responses via linked suppression. Diabetes (2010) 59:1451–60. doi: 10.2337/db09-0503
11. Gruarin P, Maglie S, de Simone M, Häringer B, Vasco C, Ranzani V, et al. Eomesodermin controls a unique differentiation program in human IL-10 and IFN-g coproducing regulatory t cells. Eur J Immunol (2019) 49:96–111. doi: 10.1002/eji.201847722
12. Magnani CF, Alberigo G, Bacchetta R, Serafini G, Andreani M, Roncarolo MG, et al. CD2 and CD226 defines a novel mechanism of suppression by human Tr1 cells. Eur J Immunol (2011) 41:1652–62. doi: 10.1002/eji.201041120
13. Meiler F, Zumkehr J, Klunker S, Rückert B, Akdis CA, Akdis M. In vivo switch to IL-10-secreting t regulatory cells in high dose allergen exposure. J Exp Med (2008) 205:2887–98. doi: 10.1084/jem.20080193
14. Chen PP, Cepika A, Agarwal-hashmi R, Saini G, Uyeda MJ, Louis DM, et al. Alloantigen-specific type 1 regulatory t cells suppress through CTLA-4 and PD-1 pathways and persist long-term in patients. Sci Transl Med (2021) 13:eabf5264. doi: 10.1126/scitranslmed.abf5264
15. Sumitomo S, Nakachi S, Okamura T, Tsuchida Y, Kato R, Shoda H, et al. Identification of tonsillar CD4+CD25–LAG3+ t cells as naturally occurring IL-10-producing regulatory t cells in human lymphoid tissue. J Autoimmun (2017) 76:75–84. doi: 10.1016/j.jaut.2016.09.005
16. Facciotti F, Gagliani N, Häringer B, Alfen JS, Penatti A, Maglie S, et al. IL-10–producing forkhead box protein 3–negative regulatory t cells inhibit b-cell responses and are involved in systemic lupus erythematosus. J Allergy Clin Immunol (2016) 137:318–321.e5. doi: 10.1016/j.jaci.2015.06.044
17. Roncarolo MG, Gregori S, Bacchetta R, Battaglia M, Gagliani N. The biology of t regulatory type 1 cells and their therapeutic application in immune-mediated diseases. Immunity (2018) 49:1004–19. doi: 10.1016/j.immuni.2018.12.001
18. Sayitoglu EC, Freeborn RA, Roncarolo MG. The yin and yang of type 1 regulatory t cells : From discovery to clinical application. Front Immunol (2021) 12:693105. doi: 10.3389/fimmu.2021.693105
19. Qin S, Cobbold SP, Pope H, Elliott J, Kioussis D, Davies J, et al. “Infectious” transplantation tolerance. Science (1993) 259:974–7. doi: 10.1126/science.8094901
20. Altin JA, Goodnow CC, Cook MC. IL-10 + CTLA-4 + Th2 inhibitory cells form in a Foxp3-independent, IL-2–dependent manner from Th2 effectors during chronic inflammation. J Immunol (2012) 188:5478–88. doi: 10.4049/jimmunol.1102994
21. Cope A, le Friec G, Cardone J, Kemper C. The Th1 life cycle: Molecular control of IFN-g to IL-10 switching. Trends Immunol (2011) 32:278–86. doi: 10.1016/j.it.2011.03.010
22. Cardone J, le Friec G, Vantourout P, Roberts A, Fuchs A, Jackson I, et al. Complement regulator CD46 temporally regulates cytokine production by conventional and unconventional t cells. Nat Immunol (2010) 11:862–71. doi: 10.1038/ni.1917
23. Ahlers J, Mantei A, Lozza L, Stäber M, Heinrich F, Bacher P, et al. A Notch/STAT3-driven blimp-1/c-Maf-dependent molecular switch induces IL-10 expression in human CD4+ t cells and is defective in crohn´s disease patients. Mucosal Immunol (2022) 15:1–11. doi: 10.1038/s41385-022-00487-x
24. Tsai Y-G, Chien J-W, Chiu Y-M, Su T-C, Chiu P-F, Hsiao K-H, et al. Lupus nephritis with corticosteroid responsiveness: molecular changes of CD46-mediated type 1 regulatory t cells. Pediatr Res (2021). doi: 10.1038/s41390-021-01882-z
25. Gabrysova L, Nicolson KS, Streeter HB, Verhagen J, Sabatos-Peyton CA, Morgan DJ, et al. Negative feedback control of the autoimmune response through antigen-induced differentiation of IL-10-secreting Th1 cells. J Exp Med (2009) 206:1755–67. doi: 10.1084/jem20082118
26. Uyeda MJ, Freeborn RA, Cieniewicz B, Romano R, Chen PP, Liu JM, et al. BHLHE40 regulates IL-10 and IFN-g production in t cells but does not interfere with human type 1 regulatory t cell differentiation. Front Immunol (2021) 12:683680. doi: 10.3389/fimmu.2021.683680
27. Gagliani N, Magnani CF, Huber S, Gianolini ME, Pala M, Licona-Limon P, et al. Coexpression of CD49b and LAG-3 identifies human and mouse t regulatory type 1 cells. Nat Med (2013) 19:739–46. doi: 10.1038/nm.3179
28. Häringer B, Lozza L, Steckel B, Geginat J. Identification and characterization of IL-10/IFN-g-producing effector-like t cells with regulatory function in human blood. J Exp Med (2009) 206:1009–17. doi: 10.1084/jem.20082238
29. Okamura T, Fujio K, Shibuya M, Sumitomo S, Shoda H, Sakaguchi S, et al. CD4+CD25-LAG3+ regulatory t cells controlled by the transcription factor egr-2. Proc Natl Acad Sci (2009) 106:13974–9. doi: 10.1073/pnas.0906872106
30. Alfen JS, Larghi P, Facciotti F, Gagliani N, Bosotti R, Paroni M, et al. Intestinal IFN-g–producing type 1 regulatory t cells coexpress CCR5 and programmed cell death protein 1 and downregulate IL-10 in the inflamed guts of patients with inflammatory bowel disease. J Allergy Clin Immunol (2018) 142:1537–1547.e8. doi: 10.1016/j.jaci.2017.12.984
31. Brockmann L, Soukou S, Steglich B, Czarnewski P, Zhao L, Wende S, et al. Molecular and functional heterogeneity of IL-10-producing CD4 + t cells. Nat Commun (2018) 9:5457. doi: 10.1038/s41467-018-07581-4
32. Bauché D, Joyce-Shaikh B, Jain R, Grein J, Ku KS, Blumenschein WM, et al. LAG3 + regulatory t cells restrain interleukin-23-Producing CX3CR1 + gutresident macrophages during group 3 innate lymphoid cell-driven colitis. Immunity (2018) 49:342–352.e5. doi: 10.1016/j.immuni.2018.07.007
33. Banerjee H, Nieves-rosado H, Kulkarni A, Delgoffe GM, Ferris RL, Kane LP, et al. Expression of tim-3 drives phenotypic and functional changes in treg cells in secondary lymphoid organs and the tumor microenvironment. CellReports (2021) 36:109699. doi: 10.1016/j.celrep.2021.109699
34. Apetoh L, Quintana FJ, Pot C, Joller N, Xiao S, Kumar D, et al. The aryl hydrocarbon receptor interacts with c-maf to promote the differentiation of type 1 regulatory t cells induced by IL-27. Nat Immunol (2010) 11:854–61. doi: 10.1038/ni.1912
35. Zhang H, Madi A, Yosef N, Chihara N, Awasthi A, Pot C, et al. An IL-27-Driven transcriptional network identifies regulators of IL-10 expression across t helper cell subsets. Cell Rep (2020) 33:108433. doi: 10.1016/j.celrep.2020.108433
36. Gandhi R, Kumar D, Burns EJ, Nadeau M, Dake B, Laroni A, et al. Activation of the aryl hydrocarbon receptor induces human type 1 regulatory t cell-like and Foxp3+ regulatory t cells. Nat Immunol (2010) 11:846–53. doi: 10.1038/ni.1915
37. Tousa S, Semitekolou M, Morianos I, Banos A, Trochoutsou AI, Brodie TM, et al. Activin-a co-opts IRF4 and AhR signaling to induce human regulatory t cells that restrain asthmatic responses. Proc Natl Acad Sci USA (2017) 114:2891–900. doi: 10.1073/pnas.1616942114
38. Roessner PM, Llaó Cid L, Lupar E, Roider T, Bordas M, Schifflers C, et al. EOMES and IL-10 regulate antitumor activity of t regulatory type 1 CD4+ t cells in chronic lymphocytic leukemia. Leukemia (2021) 35:2311–24. doi: 10.1038/s41375-021-01136-1
39. Bonnal RJP, Rossetti G, Lugli E, de Simone M, Gruarin P, Brummelman J, et al. Clonally expanded EOMES+ Tr1-like cells in primary and metastatic tumors are associated with disease progression. Nat Immunol (2021) 22:735–45. doi: 10.1038/s41590-021-00930-4
40. Baron U, Floess S, Wieczorek G, Baumann K, Grützkau A, Dong J, et al. DNA demethylation in the human FOXP3 locus discriminates regulatory t cells from activated FOXP3+ conventional t cells. Eur J Immunol (2007) 37:2378–89. doi: 10.1002/eji.200737594
41. Bevington SL, Ng STH, Britton GJ, Keane P, Wraith DC, Cockerill PN. Chromatin priming renders t cell tolerance-associated genes sensitive to activation below the signaling threshold for immune response genes. Cell Rep (2020) 31:107748. doi: 10.1016/j.celrep.2020.107748
42. Yadava K, Medina CO, Ishak H, Gurevich I, Kuipers H, Shamskhou EA, et al. Natural tr1-like cells do not confer long-term tolerogenic memory. Elife (2019) 8:e44821. doi: 10.7554/eLife.44821
43. Omokanye A, Ong LC, Lebrero-Fernandez C, Bernasconi V, Schön K, Strömberg A, et al. Clonotypic analysis of protective influenza M2e-specific lung resident Th17 memory cells reveals extensive functional diversity. Mucosal Immunol (2022) 15:717–29. doi: 10.1038/s41385-022-00497-9
44. Gianfrani C, Levings MK, Sartirana C, Mazzarella G, Barba G, Zanzi D, et al. Gliadin-specific type 1 regulatory t cells from the intestinal mucosa of treated celiac patients inhibit pathogenic t cells. J Immunol (2006) 177:4178–86. doi: 10.4049/jimmunol.177.6.4178
45. Veldman C, Höhne A, Dieckmann D, Schuler G, Hertl M. Type i regulatory t cells specific for desmoglein 3 are more frequently detected in healthy individuals than in patients with pemphigus vulgaris. J Immunol (2004) 172:6468–75. doi: 10.4049/jimmunol.172.10.6468
46. Li D, Romain G, Flamar AL, Duluc D, Dullaers M, Li XH, et al. Targeting self- and foreign antigens to dendritic cells via DC-ASGPR generates IL-10-producing suppressive CD4+ t cells. J Exp Med (2012) 209:109–21. doi: 10.1084/jem.20110399
47. Kim JE, Sharma A, Sharma G, Lee SY, Shin HS, Rudra D, et al. Lactobacillus pentosus modulates immune response by inducing IL-10 producing Tr1 cells. Immune Netw (2019) 19:e39. doi: 10.4110/in.2019.19.e39
48. Maquet C, Baiwir J, Loos P, Rodriguez-Rodriguez L, Javaux J, Sandor R, et al. Ly6Chi monocytes balance regulatory and cytotoxic CD4 t cell responses to control virus-induced immunopathology. Sci Immunol (2022) 7:eabn3240. doi: 10.1126/sciimmunol.abn3240
49. Gregori S, Tomasoni D, Pacciani V, Scirpoli M, Battaglia M, Magnani CF, et al. Differentiation of type 1 t regulatory cells (Tr1) by tolerogenic DC-10 requires the IL-10-dependent ILT4/HLA-g pathway. Blood (2010) 116:935–44. doi: 10.1182/blood-2011-08-377416
50. Mfarrej B, Jofra T, Morsiani C, Gagliani N, Fousteri G, Battaglia M. Key role of macrophages in tolerance induction via t regulatory type 1 (Tr1) cells. Clin Exp Immunol (2020) 201:222–30. doi: 10.1111/cei.13440
51. Wakkach A, Cottrez F, Groux H. Differentiation of regulatory t cells 1 is induced by CD2 costimulation. J Immunol (2001) 167:3107–13. doi: 10.4049/jimmunol.167.6.3107
52. Sutavani RV, Bradley RG, Ramage JM, Jackson AM, Durrant LG, Spendlove I. CD55 costimulation induces differentiation of a discrete t regulatory type 1 cell population with a stable phenotype. J Immunol (2013) 191:5895–903. doi: 10.4049/jimmunol.1301458
53. Ito T, Yang M, Wang YH, Lande R, Gregorio J, Perng OA, et al. Plasmacytoid dendritic cells prime IL-10-producing t regulatory cells by inducible costimulator ligand. J Exp Med (2007) 204:105–15. doi: 10.1084/jem.20061660
54. Ding Q, Lu L, Wang B, Zhou Y, Jiang Y, Zhou X, et al. B7H1-ig fusion protein activates the CD4 + IFN-g receptor + type 1 t regulatory subset through IFN-g-Secreting Th1 cells. J Immunol (2006) 177:3606–14. doi: 10.4049/jimmunol.177.6.3606
55. Kemper C, Chan AC, Green JM, Brett KA, Murphy KM, Atkinson JP. Activation of human CD4+ cells with CD3 and CD46 induces a t-regulatory cell 1 phenotype. Nature (2003) 421:388–92. doi: 10.1038/nature01315
56. le Friec G, Sheppard D, Whiteman P, Karsten CM, Shamoun SAT, Laing A, et al. The CD46-Jagged1 interaction is critical for human t h 1 immunity. Nat Immunol (2012) 13:1213–21. doi: 10.1038/ni.2454
57. Choileain SN, Hay J, Thomas J, Williams A, Vermeren MM, Benezech C, et al. TCR-stimulated changes in cell surface CD46 expression generate type 1 regulatory t cells. Sci Signal (2017) 10:1–13. doi: 10.1126/scisignal.aah6163
58. Brockmann L, Gagliani N, Steglich B, Giannou AD, Kempski J, Pelczar P, et al. IL-10 receptor signaling is essential for TR1 cell function in vivo. J Immunol (2017) 198:1130–41. doi: 10.4049/jimmunol.1601045
59. Levings MK, Sangregorio R, Galbiati F, Squadrone S, de Waal Malefyt R, Roncarolo M-G. IFN-a and IL-10 induce the differentiation of human type 1 t regulatory cells. J Immunol (2001) 166:5530–9. doi: 10.4049/jimmunol.166.9.5530
60. Zhang P, Lee JS, Gartlan KH, Schuster IS, Comerford I, Varelias A, et al. Eomesodermin promotes the development of type 1 regulatory t (TR1) cells. Sci Immunol (2017) 2:eaah7152. doi: 10.1126/sciimmunol.aah7152
61. Awasthi A, Carrier Y, Peron JPS, Bettelli E, Kamanaka M, Flavell RA, et al. A dominant function for interleukin 27 in generating interleukin 10-producing antiinflammatory t cells. Nat Immunol (2007) 8:1380–9. doi: 10.1038/ni1541
62. Pot C, Jin H, Awasthi A, Liu SM, Lai C-Y, Madan R, et al. Cutting edge: IL-27 induces the transcription factor c-maf, cytokine IL-21, and the costimulatory receptor ICOS that coordinately act together to promote differentiation of IL-10-Producing Tr1 cells. J Immunol (2009) 183:797–801. doi: 10.4049/jimmunol.0901233
63. Sumida TS, Dulberg S, Schupp JC, Lincoln MR, Stillwell HA, Axisa P, et al. Type i interferon transcriptional network regulates expression of coinhibitoryreceptors in human t cells. Nat Immunol (2022) 23:632–42. doi: 10.1038/s41590-022-01152-y
64. Bacchetta R, Gregori S, Serafini G, Sartirana C, Schulz U, Zino E, et al. Molecular and functional characterization of allogantigen specific anergic t cells suitable for cell therapy. Haematologica (2010) 95:2134–43. doi: 10.3324/haematol.2010.025825
65. Farez MF, Mascanfroni ID, Méndez-Huergo SP, Yeste A, Murugaiyan G, Garo LP, et al. Melatonin contributes to the seasonality of multiple sclerosis relapses. Cell (2015) 162:1338–52. doi: 10.1016/j.cell.2015.08.025
66. Seki A, Rutz S. Optimized RNP transfection for highly efficient CRISPR / Cas9-mediated gene knockout in primary t cells. J Exp Med (2018) 215:985–97. doi: 10.1084/jem.20171626
67. Shifrut E, Carnevale J, Tobin V, Roth TL, Woo JM, Bui CT, et al. Genome-wide CRISPR screens in primary human t cells reveal key regulators of immune function. Cell (2018) 175:1958–1971.e15. doi: 10.1016/j.cell.2018.10.024
68. Schmidt R, Steinhart Z, Layeghi M, Freimer JW, Nguyen VQ, Blaeschke F, et al. CRISPR activation and interference screens in primary human t cells decode cytokine regulation. Science (2022) 375:eabj4008. doi: 10.1126/science.abj4008
69. Cook L, Stahl M, Han X, Nazli A, MacDonald KN, Wong MQ, et al. Suppressive and gut-reparative functions of human type 1 t regulatory cells. Gastroenterology (2019) 157:1584–98. doi: 10.1053/j.gastro.2019.09.002
70. Liu JM-H, Chen P, Uyeda MJ, Cieniewicz B, Sayitoglu EC, Thomas BC, et al. Pre-clinical development and molecular characterization of an engineered type 1 regulatory t-cell product suitable for immunotherapy. Cytotherapy (2021) 23:10174–1028. doi: 10.1016/j.jcyt.2021.05.010
71. Amodio G, Mugione A, Sanchez AM, Viganò P, Candiani M, Somigliana E, et al. HLA-g expressing DC-10 and CD4+ t cells accumulate in human decidua during pregnancy. Hum Immunol (2013) 74:406–11. doi: 10.1016/j.humimm.2012.11.031
72. Salvany-Celades M, van der Zwan A, Benner M, Setrajcic-Dragos V, Bougleux Gomes HA, Iyer V, et al. Three types of functional regulatory t cells control t cell responses at the human maternal-fetal interface. Cell Rep (2019) 27:2537–47. doi: 10.1016/j.celrep.2019.04.109
73. Kühn R, Löhler J, Rennick D, Rajewsky K, Müller W. Interleukin-10-deficient mice develop chronic enterocolitis. Cell (1993) 75:263–74. doi: 10.1016/0092-8674(93)80068-P
74. Yudoh K, Matsuno H, Nakazawa F, Yonezawa T, Kimura T. Reduced expression of the regulatory CD4+ t cell subset is related to Th1/Th2 balance and disease severity in rheumatoid arthritis. Arthritis Rheum (2000) 43:617–27. doi: 10.1002/1529-0131(200003)43:3<617::AID-ANR19>3.0.CO;2-B
75. Wang L, Wang F-S, Gershwin ME. Human autoimmune diseases: a comprehensive update. J Intern Med (2015) 278:369–95. doi: 10.1111/joim.12395
76. Yu H, Gagliani N, Ishigame H, Huber S, Zhu S, Esplugues E, et al. Intestinal type 1 regulatory t cells migrate to periphery to suppress diabetogenic t cells and prevent diabetes development. Proc Natl Acad Sci (2017) 114:10443–8. doi: 10.1073/pnas.1705599114
77. Battaglia M, Stabilini A, Draghici E, Migliavacca B, Gregori S, Bonifacio E, et al. Induction of tolerance in type 1 diabetes via both CD4+CD25+ t regulatory cells and t regulatory type 1 cells. Diabetes (2006) 55:1571–80. doi: 10.2337/db05-1576
78. Chen C, Lee W-H, Yun P, Snow P, Liu C-P. Induction of autoantigenspecific Th2 and Tr1 regulatory t cells and modulation of autoimmune diabetes. J Immunol (2003) 171:733–44. doi: 10.4049/jimmunol.171.2.733
79. Prasad S, Neef T, Xu D, Podojil JR, Getts DR, Shea LD, et al. Tolerogenic ag-PLG nanoparticles induce tregs to suppress activated diabetogenic CD4 and CD8 t cells. J Autoimmun (2018) 89:112–24. doi: 10.1016/j.jaut.2017.12.010
80. Clemente-Casares X, Blanco J, Ambalavanan P, Yamanouchi J, Singha S, Fandos C, et al. Expanding antigen-specific regulatory networks to treat autoimmunity. Nature (2016) 530:434–40. doi: 10.1038/nature16962
81. Arif S, Tree TI, Astill TP, Tremble JM, Bishop AJ, Dayan CM, et al. Autoreactive t cell responses show proinflammatory polarization in diabetes but a regulatory phenotype in health. J Clin Invest (2004) 113:451–63. doi: 10.1172/JCI19585
82. Petrich de Marquesini LG, Fu J, Connor KJ, Bishop AJ, McLintock NE, Pope C, et al. IFN-g and IL-10 islet-antigen-specific t cell responses in autoantibodynegative first-degree relatives of patients with type 1 diabetes. Diabetologia (2010) 53:1451–60. doi: 10.1007/s00125-010-1739-3
83. Sanda S, Roep BO, von Herrath M. Islet antigen specific IL-10+ immune responses but notCD4+CD25+FoxP3+ cells at diagnosis predict glycemic control in type 1 diabetes. Clin Immunol (2008) 127:138–43. doi: 10.1016/j.clim.2007.12.003
84. Chujo D, Nguyen T-S, Foucat E, Blankenship D, Banchereau J, Nepom GT, et al. Adult-onset type 1 diabetes patients display decreased IGRP-specific Tr1 cells in blood. Clin Immunol (2015) 161:270–7. doi: 10.1016/j.clim.2015.08.014
85. Herold KC, Burton JB, Francois F, Poumian-Ruiz E, Glandt M, Bluestone JA. Activation of human t cells by FcR nonbinding anti-CD3 mAb, hOKT3g1(Alaala). J Clin Invest (2003) 111:409–18. doi: 10.1172/JCI16090
86. Alhadj Ali M, Liu Y-F, Arif S, Tatovic D, Shariff H, Gibson VB, et al. Metabolic and immune effects of immunotherapy with proinsulin peptide in human new-onset type 1 diabetes. Sci Transl Med (2017) 9:eaaf7779. doi: 10.1126/scitranslmed.aaf7779
87. Barrat FJ, Cua DJ, Boonstra A, Richards DF, Crain C, Savelkoul HF, et al. In vitro generation of interleukin 10–producing regulatory CD4+ t cells is induced by immunosuppressive drugs and inhibited by t helper type 1 (Th1)– and Th2-inducing cytokines. J Exp Med (2002) 195:603–16. doi: 10.1084/jem.20011629
88. Mascanfroni ID, Yeste A, Vieira SM, Burns EJ, Patel B, Sloma I, et al. IL-27 acts on DCs to suppress the t cell response and autoimmunity by inducing expression of the immunoregulatorymoleculeCD39. Nat Immunol (2013) 14:1054–63. doi: 10.1038/ni.2695
89. Raverdeau M, Christofi M, Malara A, Wilk MM, Misiak A, Kuffova L, et al. Retinoic acid-induced autoantigen-specific type 1 regulatory t cells suppress autoimmunity. EMBO Rep (2019) 20:e47121. doi: 10.15252/embr.201847121
90. Kenison JE, Jhaveri A, Li Z, Khadse N, Tjon E, Tezza S, et al. Tolerogenic nanoparticles suppress central nervous system inflammation. Proc Natl Acad Sci (2020) 117:32017–28. doi: 10.1073/pnas.2016451117
91. Astier AL, Meiffren G, Freeman S, Hafler DA. Alterations in CD46-mediated Tr1 regulatory t cells in patients with multiple sclerosis. J Clin Invest (2006) 116:3252–7. doi: 10.1172/JCI29251
92. Caielli S, Veiga DT, Balasubramanian P, Athale S, Domic B, Murat E, et al. ACD4+ t cell population expanded in lupus blood provides b cell help through interleukin-10 and succinate. Nat Med (2019) 25:75–81. doi: 10.1038/s41591-018-0254-9
93. Asnagli H, Martire D, Belmonte N, Quentin J, Bastian H, Boucard-Jourdin M, et al. Type 1 regulatory t cells specific for collagen type II as an efficient cellbased therapy in arthritis. Arthritis Res Ther (2014) 16:R115. doi: 10.1186/ar4567
94. Kim J, Lee J, Gonzalez J, Fuentes-Duculan J, Garcet S, Krueger JG. Proportion of CD4+CD49b+LAG-3+ type 1 regulatory t cells in the blood of psoriasis patients inversely correlates with psoriasis area and severity index. J Invest Dermatol (2018) 138:2669–72. doi: 10.1016/j.jid.2018.05.021
95. Kelly CP, Murray JA, Leffler DA, Getts DR, Bledsoe AC, Smithson G, et al. TAK-101 nanoparticles induce gluten-specific tolerance in celiac disease: A randomized, double-blind, placebo-controlled study. Gastroenterology (2021) 161:66–80.e8. doi: 10.1053/j.gastro.2021.03.014
96. Asseman C, Mauze S, Leach MW, Coffman RL, Powrie F. An essential role for interleukin 10 in the function of regulatory t cells that inhibit intestinal inflammation. J Exp Med (1999) 190:995–1004. doi: 10.1084/jem.190.7.995
97. Huber S, Gagliani N, Esplugues E, O’Connor W, Huber FJ, Chaudhry A, et al. Th17 cells express interleukin-10 receptor and are controlled by Foxp3– and Foxp3+ regulatory CD4+ t cells in an interleukin-10-Dependent manner. Immunity (2011) 34:554–65. doi: 10.1016/j.immuni.2011.01.020
98. Doñas C, Neira J, Osorio-Barrios F, Carrasco M, Fernández D, Prado C, et al. The demethylase inhibitor GSK-J4 limits inflammatory colitis by promoting de novo synthesis of retinoic acid in dendritic cells. Sci Rep (2021) 11:1342. doi: 10.1038/s41598-020-79122-3
99. Qi L, Wu J, Zhu S, Wang X, Lv X, Liu C, et al. Mesenchymal stem cells alleviate inflammatory bowel disease Via Tr1 cells. Stem Cell Rev Rep (2022) 18:2444–57. doi: 10.1007/s12015-022-10353-9
100. Desreumaux P, Foussat A, Allez M, Beaugerie L, Hé buterne X, Bouhnik Y, et al. Safety and efficacy of antigen-specific regulatory t-cell therapy for patients with refractory crohn’s disease. Gastroenterology (2012) 143:1207–1217.e2. doi: 10.1053/j.gastro.2012.07.116
101. Chatenoud L, Ferran C, Bach J-F. The anti-CD3-Induced syndrome: A consequence of massive in vivo cell activation. In: Fleischer B, Sjögren HO, editors. Superantigens. current topics in microbiology and immunology, vol. 174 . Berlin, Heidelberg: Springer (1991). p. 121–34. doi: 10.1007/978-3-642-50998-8_9
102. Jamison BL, Neef T, Goodspeed A, Bradley B, Baker RL, Miller SD, et al. Nanoparticles containing an insulin–ChgA hybrid peptide protect from transfer of autoimmune diabetes by shifting the balance between effector t cells and regulatory t cells. J Immunol (2019) 203:48–57. doi: 10.4049/jimmunol.1900127
103. Getts DR, Martin AJ, McCarthy DP, Terry RL, Hunter ZN, Yap WT, et al. Microparticles bearing encephalitogenic peptides induce t-cell tolerance and ameliorate experimental autoimmune encephalomyelitis. Nat Biotechnol (2012) 30:1217–24. doi: 10.1038/nbt.2434
104. Alatab S, Sepanlou SG, Ikuta K, Vahedi H, Bisignano C, Safiri S, et al. The global, regional, and national burden of inflammatory bowel disease in 195 countries and territories 1990–2017: a systematic analysis for the global burden of disease study 2017. Lancet Gastroenterol Hepatol (2020) 5:17–30. doi: 10.1016/S2468-1253(19)30333-4
105. Uhlig HH, Coombes J, Mottet C, Izcue A, Thompson C, Fanger A, et al. Characterization of Foxp3+CD4+CD25+ and IL-10-secreting CD4+CD25+ t cells during cure of colitis. J Immunol (2006) 177:5852–60. doi: 10.4049/jimmunol.177.9.5852
106. Kamanaka M, Kim ST, Wan YY, Sutterwala FS, Lara-Tejero M, Galán JE, et al. Expression of interleukin-10 in intestinal lymphocytes detected by an interleukin-10 reporter knockin tiger mouse. Immunity (2006) 25:941–52. doi: 10.1016/j.immuni.2006.09.013
107. Maynard CL, Harrington LE, Janowski KM, Oliver JR, Zindl CL, Rudensky AY, et al. Regulatory t cells expressing interleukin 10 develop from Foxp3+ and Foxp3– precursor cells in the absence of interleukin 10. Nat Immunol (2007) 8:931–41. doi: 10.1038/ni1504
108. Himmel ME, Yao Y, Orban PC, Steiner TS, Levings MK. Regulatory t-cell therapy for inflammatory bowel disease: More questions than answers. Immunology (2012) 136:115–22. doi: 10.1111/j.1365-2567.2012.03572.x
109. Asseman C, Read S, Powrie F. Colitogenic Th1 cells are present in the antigen-experienced t cell pool in normal mice: Control by CD4 + regulatory t cells and IL-10. J Immunol (2003) 171:971–8. doi: 10.4049/jimmunol.171.2.971
110. Rubtsov YP, Rasmussen JP, Chi EY, Fontenot J, Castelli L, Ye X, et al. Regulatory t cell-derived interleukin-10 limits inflammation at environmental interfaces. Immunity (2008) 28:546–58. doi: 10.1016/j.immuni.2008.02.017
111. Roers A, Siewe L, Strittmatter E, Deckert M, Schlüter D, Stenzel W, et al. T cell–specific inactivation of the interleukin 10 gene in mice results in enhanced t cell responses but normal innate responses to lipopolysaccharide or skin irritation. J Exp Med (2004) 200:1289–97. doi: 10.1084/jem.20041789
112. Chaudhry A, Samstein RM, Treuting P, Liang Y, Pils MC, Heinrich J-M, et al. Interleukin-10 signaling in regulatory t cells is required for suppression of Th17 cell-mediated inflammation. Immunity (2011) 34:566–78. doi: 10.1016/j.immuni.2011.03.018
113. McGuirk P, McCann C, Mills KHG. Pathogen-specific t regulatory 1 cells induced in the respiratory tract by a bacterial molecule that stimulates interleukin 10 production by dendritic cells: A novel strategy for evasion of protective t helper type 1 responses by bordetella pertussis. J Exp Med (2002) 195:221–31. doi: 10.1084/jem.20011288
114. Jofra T, di Fonte R, Galvani G, Kuka M, Iannacone M, Battaglia M, et al. Tr1 cell immunotherapy promotes transplant tolerance via de novo Tr1 cell induction in mice and is safe and effective during acute viral infection. Eur J Immunol (2018) 48:1389–99. doi: 10.1002/eji.201747316
115. Eliasson DG, Omokanye A, Schön K, Wenzel UA, Bernasconi V, Bemark M, et al. M2e-tetramer-specific memory CD4 t cells are broadly protective against influenza infection. Mucosal Immunol (2018) 11:273–89. doi: 10.1038/mi.2017.14
116. Antunes I, Kassiotis G. Suppression of innate immune pathology by regulatory t cells during influenza a virus infection of immunodeficient mice. J Virol (2010) 84:12564–75. doi: 10.1128/jvi.01559-10
117. Brincks EL, Roberts AD, Cookenham T, Kohlmeier JE, Blackman MA, David L. Antigen-specific memory regulatory CD4 + Foxp3 + t cells control memory responses to influenza virus infection. J Immunol (2013) 190:3438–46. doi: 10.4049/jimmunol.1203140
118. Moser EK, Hufford MM, Braciale TJ. Late engagement ofCD86 after influenza virus clearance promotes recovery in a FoxP3 + regulatory t cell dependent manner. PloS Pathog (2014) 10:e1004315. doi: 10.1371/journal.ppat.1004315
119. Rogers MC, Lamens KD, Shafagati N, Johnson M, Oury TD, Joyce S, et al. CD4 + regulatory t cells exert differential functions during early and late stages of the immune response to respiratory viruses. J Immunol (2018) 201:1253–66. doi: 10.4049/jimmunol.1800096
120. Lu C, Zanker D, Lock P, Jiang X, Deng J, Duan M, et al. Memory regulatory t cells home to the lung and control influenza a virus infection. Immunol Cell Biol (2019) 97:774–86. doi: 10.1111/imcb.12271
121. Doetze A, Satoguina J, Burchard G, Rau T, Loliger C, Fleischer B, et al. Antigenspecific cellular hyporesponsiveness in a chronic human helminth infectionismediated by T(h)3/T(r)1-type cytokines IL-10 and transforming growth factor-beta but not by a T(h)1 to T(h)2 shift. Int Immunol (2000) 12:623–30.
122. Koch K, Koch N, Sandaradura De Silva U, Jung N, Schulze Zur Wiesch J, Fatkenheuer G, et al. Increased frequency of CD49b/LAG-3+ type 1 regulatory t cells in HIV-infected individuals. AIDS Res Hum Retroviruses (2015) 31:1238–46. doi: 10.1089/aid.2014.0356
123. Moreira Genaro L, de Oliveira Coser L, da Silva Justo Junior A, Furquim de Castro L, Felı́cio Barreto AK, Rizzato AE, et al. Association between IL-27 and Tr1 cells in severe form of paracoccidioidomycosis. Cytokine (2020) 127:154962. doi: 10.1016/j.cyto.2019.154962
124. MacDonald AJ, Duffy M, Brady MT, McKiernan S, Hall W, Hegarty J, et al. CD4 t helper type 1 and regulatory t cells induced against the same epitopes on the core protein in hepatitis c virus-infected persons. J Infect Dis (2002) 185:720–7. doi: 10.1086/339340
125. Brady MT, MacDonald AJ, Rowan AG, Mills KHG. Hepatitis c virus nonstructural protein 4 suppresses Th1 responses by stimulating IL-10 production from monocytes. Eur J Immunol (2003) 33:3448–57. doi: 10.1002/eji.200324251
126. Carpentier A, Conti F, Stenard F, Aoudjehane L, Miroux C, Podevin P, et al. Increased expression of regulatory Tr1 cells in recurrent hepatitis c after liver transplantation. Am J Transplant (2009) 9:2102–12. doi: 10.1111/j.1600-6143.2009.02743.x
127. Fabien S, Olivier M, Khaldoun G, Vivian V, Lynda A, Laurissa O, et al. CD49b, a major marker of regulatory t-cells type 1, predicts the response to antiviral therapy of recurrent hepatitis c after liver transplantation. BioMed Res Int (2014) 2014:290878. doi: 10.1155/2014/290878
128. Utsumi M, Takaki A, Umeda Y, Koike K, Napier SC, Watanabe N, et al. Frequency of regulatory t-cell and hepatitis c viral antigen-specific immune response in recurrent hepatitis c after liver transplantation. Transpl Immunol (2014) 31:33–41. doi: 10.1016/j.trim.2014.05.006
129. Ghazal K, Morales O, Barjon C, Dahlqvist G, Aoudjehane L, Ouaguia L, et al. Early high levels of regulatory t cells and t helper 1 may predict the progression of recurrent hepatitis c after liver transplantation. Clin Res Hepatol Gastroenterol (2019) 43:273–81. doi: 10.1016/j.clinre.2018.10.005
130. Langhorne J, Ndungu FM, Sponaas AM, Marsh K. Immunity to malaria: More questions than answers. Nat Immunol (2008) 9:725–32. doi: 10.1038/ni.f.205
131. Rodriguez-Barraquer I, Arinaitwe E, Jagannathan P, Kamya MR, Rosenthal PJ, Rek J, et al. Quantification of anti-parasite and anti-disease immunity to malaria as a function of age and exposure. Elife (2018) 7:e35832. doi: 10.7554/eLife.35832
132. Jagannathan P, Eccles-James I, Bowen K, Nankya F, Auma A, Wamala S, et al. IFNg/IL-10 co-producing cells dominate the CD4 response to malaria in highly exposed children. PloS Pathog (2014) 10:e1003864. doi: 10.1371/journal.ppat.1003864
133. Freitas do Rosário AP, Lamb T, Spence P, Stephens R, Lang A, Roers A, et al. IL-27 promotes IL-10 production by effector Th1 CD4 + t cells: A critical mechanism for protection from severe immunopathology during malaria infection. J Immunol (2012) 188:1178–90. doi: 10.4049/jimmunol.1102755
134. Loevenich K, Ueffing K, Abel S, Hose M, Matuschewski K, Westendorf AM, et al. DC-derived IL-10 modulates pro-inflammatory cytokine production and promotes induction of CD4+IL-10+ regulatory t cells during plasmodium yoelii infection. Front Immunol (2017) 8:152. doi: 10.3389/fimmu.2017.00152
135. Abel S, Lückheide N, Westendorf AM, Geffers R, Roers A, Müller W, et al. Strong impact of CD4+ Foxp3+ regulatory t cells and limited effect of t cellderived IL-10 on pathogen clearance during plasmodium yoelii infection. J Immunol (2012) 188:5467–77. doi: 10.4049/jimmunol.1102223
136. Couper KN, Blount DG, Wilson MS, Hafalla JC, Belkaid Y, Kamanaka M, et al. IL-10 from CD4+CD25-Foxp3-CD127- adaptive regulatory t cells modulates parasite clearance and pathology during malaria infection. PloS Pathog (2008) 4:e1000004. doi: 10.1371/journal.ppat.1000004
137. Lönnberg T, Svensson V, James KR, Fernandez-Ruiz D, Sebina I, Montandon R, et al. Single-cell RNA-seq and computational analysis using temporal mixture modeling resolves TH1/TFH fate bifurcation in malaria. Sci Immunol (2017) 2:eaal2192. doi: 10.1126/sciimmunol.aal2192
138. Soon MSF, Lee HJ, Engel JA, Straube J, Thomas BS, Pernold CPS, et al. Transcriptome dynamics of CD4+ t cells during malaria maps gradual transit from effector to memory. Nat Immunol (2020) 21:1597–610. doi: 10.1038/s41590-020-0800-8
139. Neumann C, Scheffold A, Rutz S. Functions and regulation of t cellderived interleukin-10. Semin Immunol (2019) 44:101344. doi: 10.1016/j.smim.2019.101344
140. Boyle MJ, Jagannathan P, Bowen K, McIntyre TI, Vance HM, Farrington LA, et al. The development of plasmodium falciparum-specific IL10 CD4 t cells and protection from malaria in children in an area of high malaria transmission. Front Immunol (2017) 8:1329. doi: 10.3389/fimmu.2017.01329
141. Mackroth MS, Abel A, Steeg C, Schulze zur Wiesch J, Jacobs T. Acute malaria induces PD1+CTLA4+ effector t cells with cell-extrinsic suppressor function. PloS Pathog (2016) 12:1–24. doi: 10.1371/journal.ppat.1005909
142. Brandi J, Lehmann C, Kaminski LC, Schulze zur Wiesch J, Addo M, Ramharter M, et al. T cells expressing multiple co-inhibitory molecules in acute malaria are not exhausted but exert a suppressive function in mice. Eur J Immunol (2022) 52:312–27. doi: 10.1002/eji.202149424
143. Brandi J, Riehn M, Hadjilaou A, Jacobs T. Increased expression of multiple co-inhibitory molecules on malaria-induced CD8+ t cells are associated with increased function instead of exhaustion. Front Immunol (2022) 13:878320. doi: 10.3389/fimmu.2022.878320
144. Christoffersson G, von Herrath M. Regulatory immune mechanisms beyond regulatory t cells. Trends Immunol (2019) 40:482–91. doi: 10.1016/j.it.2019.04.005
145. Passerini L, di Nunzio S, Gregori S, Gambineri E, Cecconi M, Seidel MG, et al. Functional type 1 regulatory t cells develop regardless of FOXP3 mutations in patients with IPEX syndrome. Eur J Immunol (2011) 41:1120–31. doi: 10.1002/eji.201040909
146. Narula M, Lakshmanan U, Borna S, Schulze JJ, Holmes TH, Harre N, et al. Epigenetic and immunological indicators of IPEX disease in subjects with FOXP3 gene mutation. J Allergy Clin Immunol (2022). doi: 10.1016/j.jaci.2022.09.013
147. Lio CWJ, Hsieh CS. Becoming self-aware: The thymic education of regulatory t cells. Curr Opin Immunol (2011) 23:213–9. doi: 10.1016/j.coi.2010.11.010
148. Akdis M, Verhagen J, Taylor A, Karamloo F, Karagiannidis C, Crameri R, et al. Immune responses in healthy and allergic individuals are characterized by a fine balance between allergen-specific t regulatory 1 and t helper 2 cells. J Exp Med (2004) 199:1567–75. doi: 10.1084/jem.20032058
149. Pacciani V, Gregori S, Chini L, Corrente S, Chianca M, Moschese V, et al. Induction of anergic allergen-specific suppressor t cells using tolerogenic dendritic cells derived from children with allergies to house dust mites. J Allergy Clin Immunol (2010) 125:727–36. doi: 10.1016/j.jaci.2009.12.004
150. Pellerin L, Jenks JA, Chinthrajah S, Dominguez T, Block W, Zhou X, et al. Peanutspecific type 1 regulatory t cells induced in vitro from allergic subjects are functionally impaired. J Allergy Clin Immunol (2018) 141:202–213.e8. doi: 10.1016/j.jaci.2017.05.045
151. Foussat A, Cottrez F, Brun V, Fournier N, Breittmayer J-P, Groux H. A comparative study between t regulatory type 1 and CD4+CD25+ t cells in the control of inflammation. J Immunol (2003) 171:5018–26. doi: 10.4049/jimmunol.171.10.5018
152. Feunou P, Poulin L, Habran C, le Moine A, Goldman M, Braun MY. CD4+ CD25+ and CD4+ CD25– t cells act respectively as inducer and effector t suppressor cells in superantigen-induced tolerance. J Immunol (2003) 171:3475–84. doi: 10.4049/jimmunol.171.7.3475
Keywords: Type 1 regulatory T (Tr1) cells, immunological tolerance, autoimmunity, inflammatory bowel disease, infectious disease
Citation: Freeborn RA, Strubbe S and Roncarolo MG (2022) Type 1 regulatory T cell-mediated tolerance in health and disease. Front. Immunol. 13:1032575. doi: 10.3389/fimmu.2022.1032575
Received: 31 August 2022; Accepted: 10 October 2022;
Published: 28 October 2022.
Edited by:
Moanaro Biswas, Indiana University, United StatesReviewed by:
Kingston H. Mills, Trinity College Dublin, IrelandCopyright © 2022 Freeborn, Strubbe and Roncarolo. This is an open-access article distributed under the terms of the Creative Commons Attribution License (CC BY). The use, distribution or reproduction in other forums is permitted, provided the original author(s) and the copyright owner(s) are credited and that the original publication in this journal is cited, in accordance with accepted academic practice. No use, distribution or reproduction is permitted which does not comply with these terms.
*Correspondence: Maria Grazia Roncarolo, bWcxQHN0YW5mb3JkLmVkdQ==
†These authors have contributed equally to this work and share first authorship
Disclaimer: All claims expressed in this article are solely those of the authors and do not necessarily represent those of their affiliated organizations, or those of the publisher, the editors and the reviewers. Any product that may be evaluated in this article or claim that may be made by its manufacturer is not guaranteed or endorsed by the publisher.
Research integrity at Frontiers
Learn more about the work of our research integrity team to safeguard the quality of each article we publish.