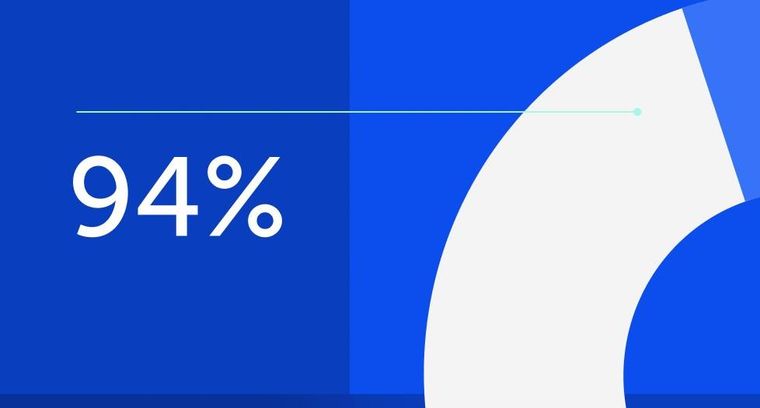
94% of researchers rate our articles as excellent or good
Learn more about the work of our research integrity team to safeguard the quality of each article we publish.
Find out more
REVIEW article
Front. Immunol., 16 September 2022
Sec. Cancer Immunity and Immunotherapy
Volume 13 - 2022 | https://doi.org/10.3389/fimmu.2022.1027838
This article is part of the Research TopicAdenosine Pathways in Cancer Immunity and ImmunotherapyView all 14 articles
Gastric cancer (GC) is one of the most common malignancy and leading cause of cancer-related deaths worldwide. Due to asymptomatic or only nonspecific early symptoms, GC patients are usually in the advanced stage at first diagnosis and miss the best opportunity of treatment. Immunotherapies, especially immune checkpoint inhibitors (ICIs), have dramatically changed the landscape of available treatment options for advanced-stage cancer patients. However, with regards to existing ICIs, the clinical benefit of monotherapy for advanced gastric cancer (AGC) is quite limited. Therefore, it is urgent to explore an optimal target for the treatment of GC. In this review, we summarize the expression profiles and prognostic value of 20 common immune checkpoint-related genes in GC from Gene Expression Profiling Interactive Analysis (GEPIA) database, and then find that the adenosinergic pathway plays an indispensable role in the occurrence and development of GC. Moreover, we discuss the pathophysiological function of adenosinergic pathway in cancers. The accumulation of extracellular adenosine inhibits the normal function of immune effector cells and facilitate the effect of immunosuppressive cells to foster GC cells proliferation and migration. Finally, we provide insights into potential clinical application of adenosinergic-targeting therapies for GC patients.
Gastric cancer (GC) is a major source of global cancer mortality with limited treatment options and poor patient survival. It is the fourth most commonly occurring cancer in men and the seventh in women (1). For patients with early gastric cancer (EGC) and low risk of lymph node metastasis, endoscopic submucosal dissection (ESD) or radical surgical resection alone is potentially curative (2, 3). Unfortunately, due to no apparent symptom or only indigestion-like clinical manifestations, such as inappetence, gastroesophageal reflux, and belching, patients with EGC often miss the best treatment opportunity because of negligence (2). Although endoscopic screening significantly increases the detection of EGC and improves prognosis (4). Skill among endoscopists varies greatly, and numerous patients are still missed for various reasons (5). As the disease progresses, hemorrhage, perforation, obstruction, cachexia, and other symptoms of advanced cancer gradually appear. GC is already in the advanced stage once detected in patients, which has a poor ending due to ineffective therapies and multiple resistance (6). Therefore, accurately diagnosing EGC and effectively treating advanced gastric cancer (AGC) patients who have lost the chance of radical surgical resection are two serious health problems all over the world.
For the patients who are suffering from GC, the treatments are mainly surgical excision, chemotherapy, targeted therapy, immunotherapy, and other comprehensive strategies (7). Among them, radical gastrectomy with D2 lymphadenectomy, with or without neoadjuvant therapy, is the only potentially curative treatment option (8). However, increasing numbers of studies have shown that surgery cannot benefit patients with unresectable AGC and post-operative complication is a negative predictor of long-term survival outcomes for them (9). Systemic chemotherapy with multiple drug regimens is the main therapy choice to further prolong the survival of post- or non-operative AGC patients (10). Despite relevant progress, the impact of chemotherapy on AGC patients’ survival is still unsatisfactory, especially patients with multiple distant metastases (1). Additionally, as an emerging, attractive, and effective treatment, targeted therapy has shown promising effects in a part of GC patients, even if the beneficiary degree not definite (11). As the most common target in GC, the frequency of human epidermal growth factor receptor 2 (HER2) overexpression ranges from 4.4% to 53.4%, with a mean of 17.9% (12). Coupled with drug resistance developed during treatment, management of AGC patients by targeted therapy remains a challenge. Despite new therapeutic options, AGC remains associated with a poor prognosis compared with other cancers, on account of inactive immunogenicity and vast heterogeneity represent a barrier to disease management (13, 14).
Immunotherapies, especially immune checkpoint inhibitors (ICIs) and chimeric antigen receptor-modified T (CAR T) cell therapies, have been used continuously for decades, as lifesaving procedures for millions of patients with hematological malignancy (15). As the most extensively used ICIs at present, checkpoint inhibitor-based immunotherapies that target the cytotoxic T lymphocyte-associated antigen 4 (CTLA-4) and the programmed cell death receptor 1/programmed cell death ligand 1 (PD-1/PD-L1) pathway have achieved impressive success in the treatment of different cancer types (16). Nevertheless, there still exists various challenges that have severely limited the clinical application of immunotherapies in AGC, for instance, the ineffectiveness and serious side effects (6). For AGC patients, anti-CTLA-4 and anti-PD-1/PD-L1 monoclonal antibodies cannot acquire satisfactory curative effect without the assistance of other cancer treatments (17–20). Some clinical trials have shown positive effects on overall response and disease control in combination with ICIs and other therapies, yet responses are slight and heterogeneous (17). Therefore, it is urgent to explore a more effective immunotherapy method to prolong the survival of AGC patients.
In this review, we find that CD73 is the most important immune checkpoint affecting the prognosis of GC patients by analyzing the Gene Expression Profiling Interactive Analysis (GEPIA) database. In addition, we also describe the mechanism of CD39-CD73-adenosine signaling pathway in immune regulation of cancers and discuss its role in the occurrence and development of GC. At the end of the article, we also put forward some prospects about treating GC with the help of targeting CD39-CD73-adenosine axis.
ICI, especially inhibition of PD-1/PD-L1 axis, is a new standard of immunotherapy in the treatment of advanced or metastatic GC and is represented in various combinations with and without other treatments within clinical trials (21). However, its curative effect is related to individual differences to a certain degree. For example, in a randomized, open-label, phase 3 trial (NCT02370498), the PD-1/PD-L1 blockade cannot significantly improve overall survival (OS) and progression-free survival (PFS) versus paclitaxel for PD-L1-positive GC (all P > 0.6) (22). In another phase 3 randomized clinical trial (NCT02494583), the PD-1/PD-L1 blockade plus chemotherapy was not superior to chemotherapy for OS (12.3 vs. 10.8 months; HR, 0.85; 95% CI, 0.62-1.17; P = 0.16) (19). Collectively, the immunotherapy of GC needs a more appropriate immune checkpoint to obtain superior efficacy.
To further confirm which target plays the most indispensable role in GC, we input 20 common immune checkpoint-related genes into the GEPIA server for in-depth analysis (Table 1). Among them, we found that 9 genes were confirmed to have significant differential expression in GC (Figure 1). Moreover, the expression levels of PDCD1 (encode PD-1), CD274 (encode PD-L1), and CTLA-4 genes in GC not change compared with adjacent tissues, which was consistent with the above-mentioned treatment results.
Figure 1 The analysis of immune checkpoint-related genes expression in GC by GEPIA database. The results revealed that 9 genes were confirmed to have significant differential expression in GC compared to the normal tissues. Among them, higher expression was observed in HHLA2, ENTPD1, PVR, CD24, NT5E, TIGIT, CD276, and CD47 and lower expression was observed in LGALS9C. Red color represents tumor tissue (n=408), and gray color represents normal tissue (n=211). STAD, stomach adenocarcinoma. * P < 0.05.
Furthermore, we investigated whether the expression of various immune checkpoint-related genes was correlated with prognosis in GC patients (Figure 2). The results of GEPIA analysis showed that only the high expression of NT5E (encode CD73) is more likely to encounter GC patients death earlier and shorten survival time (p<0.05). Additionally, with the help of immunohistochemistry, single-sample gene set enrichment analysis and flow cytometry, extensive related studies have reported that CD73 expression is upregulated in GC which is proved to be an independent adverse prognosticator for the patients (61–63).
Figure 2 Kaplan-Meier survival curves comparing the high and low expression of immune checkpoint-related genes in GC by GEPIA database. The results showed that only the high expression of NT5E (encode CD73) was correlated with poor prognosis of GC patients (p<0.05). The red line indicates the high expression group of genes (n=192) and the blue line represents the low expression group of genes (n=191).
Ecto-5’-nucleotidase (NT5E), also known as CD73, is a cytomembrane protein linked to the cell membrane via a glycosylphosphatidylinositol (GPI) anchor that regulates the conversion of extracellular adenosine monophosphate (eAMP) to adenosine contributing to immunosuppression (64). CD39, also termed ectonucleoside triphosphate diphosphohydrolase-1 (ENTPD1), catalyzes the hydrolysis of extracellular adenosine triphosphate (eATP) and adenosine diphosphate (eADP) into eAMP to provide raw materials for CD73 (65). As the end product of CD39-CD73 axis, adenosine mediates immunosuppression within the tumor microenvironment (TME) through triggering adenosine receptors on the membrane surface, including A1R (encoded by ADORA1), A2AR (encoded by ADORA2A), A2BR (encoded by ADORA2B), and A3R (encoded by ADORA3) (66).
Based on these, we analyzed the associations between 20 common immune checkpoint-related genes and survival contribution in GC by GEPIA database. In general, compared with other immune checkpoints, CD73 showed the most obvious detrimental role in GC patients (Figure 3A). In addition, according to the analysis of corresponding genes expression and the TNM stage, we also found that the expression of CD39 and CD73 was higher in GC patients with clinic stage II, stage III, or stage IV than that in stage I, which revealed that these upregulated genes might be associated with tumor progression positively (Figure 3B). However, the role of adenosine receptors in GC patients still needs to be further evaluated (Figure 3C).
Figure 3 The analysis of adenosinergic pathway-related genes expression in GC by GEPIA database. (A) The risk assessment of 20 common immune checkpoint-related genes affecting the prognosis of GC patients. By comparing the survival contribution of multiple genes via Mantel-Cox test, we found that NT5E (encode CD73) showed the most obvious detrimental role in GC patients (n=383). (B) The expression levels of ENTPD1 and NT5E in different tumor stages of GC. With the progression of GC, the expression of ENTPD1 and NT5E also increased. (C) The expression levels of adenosine receptors in GC patients. The analysis showed that only ADORA2B expression (encode A2BR) increased in GC compared to the normal tissues and only ADORA2A (encode A2AR) was positively correlated with the progression of GC. Red color represents tumor tissue (n=408), and gray color represents normal tissue (n=211). STAD, stomach adenocarcinoma; HR, hazard ratio. * P < 0.05.
Taken together, the CD39-CD73-adenosine signaling pathway, as the most important immune checkpoint in GC, mediates the immunosuppressive mechanism by which tumors escape immunosurveillance and impede anti-tumor immunity within the TME. Thereinto, CD73 is an optimal target for the immunotherapy of GC.
Under normal circumstances, ATP is almost exclusively present inside cells as the main energy currency, participating in virtually all biological processes (67). eATP, as an extracellular messenger, is set by both passive and active release mechanisms and degradation processes (68, 69). Measurement of eATP levels in different biological context reveals that healthy tissues present very low levels (10–100 nanomoles per liter) of this nucleotide in the pericellular space, while in sites of tissue damage, inflammation, hypoxia, ischemia or TME it can reach high levels (100–500 micromoles per liter) to promote inflammatory responses (Figure 4) (70, 71).
Figure 4 Immune regulation of adenosine signaling in the TME. Cell stress promotes eATP production and contributes to chronic inflammation via P2Rs. Within the TME, accumulated eATP can be degraded to ADO by the sequential action of the ectonucleotidases CD39 and CD73 or other alternative pathways such as ALP or PAP-mediated process. In addition, the sequential catabolism of NAD+ by CD38, CD203a and CD73 also can generate ADO and the high concentration of intracellular ADO can be transported outside the cell via ENTs or CNTs to maintain balance. The bioavailability of extracellular ADO is regulated by adenosine-converting enzymes such as ADK and ADA, which converts ADO into AMP and inosine respectively. High concentrations of ADO binding to adenosine receptors to inhibit the activation of immune cells and stimulate immunosuppressive cells to promote the immune escape of cancers. eATP, extracellular adenosine triphosphate; eAMP, extracellular adenosine monophosphate; NK cell, natural killer cell; DC, dendritic cell; Treg, regulatory T cell; TAM, tumor-associated macrophage; CAF, cancer associated fibroblast; MDSC, myeloid-derived suppressor cell; MSC, mesenchymal stromal cell; ADO, adenosine; NAD+, nicotinamide adenine dinucleotide; ADPR, adenosine diphosphate ribose; ADA, adenosine deaminase; ADK, adenosine kinase; ENT, equilibrative nucleoside transporter; CNT, concentrative nucleoside transporter; P2Rs, P2 purinergic receptors; PAP, prostatic acid phosphatase; ALP, alkaline phosphatase; cAMP, cyclic adenosine monophosphate.
There are two families of P2 purinergic receptors (P2Rs) for eATP: ATP-gated ion channels known as P2X receptors (P2X1-7) and G protein–coupled P2Y receptors (P2Y1, P2Y2, P2Y4, P2Y6, P2Y11b, P2Y12, P2Y13, P2Y14c) (69). Among them, the P2X7 receptor (P2X7R), as the most structurally and functionally distinct P2R subtype, appears to be a main player in host-tumor cell interactions because of involvement in apoptotic, inflammatory, and tumor progression pathways (72, 73). During innate immune responses, the key role of P2X7R is to activate the assembly of nucleotide-binding domain (NOD) like receptor protein 3 (NLRP3) inflammasome rapidly, which could consecutively facilitate caspase-1 meditated maturation and release of the pro-inflammatory cytokines interleukin-1β and interleukin-18 to participate in both defense and inflammatory responses (74, 75). For adaptive immune responses, eATP signals via P2X7R to boost the activation, proliferation, and chemotaxis of immune cells with consequent stimulation of CD8+ and CD4+ T cell mediated anti-tumor responses (74, 76, 77). The production of pro-inflammatory cytokines, such as interleukin-1β and interleukin-18, are involved in the activation of B and NK cells (78). Additionally, the stimulation of P2X7R inhibits the tissue-specific immunosuppressive potential of regulatory T cells (Tregs) and facilitated their conversion to T helper 17 (Th17) cells during chronic inflammation (79). On the contrary, P2X7R antagonism increases Tregs and reduces clinical and histological graft-versus-host disease in a humanized mouse model (80). Overall, eATP can provide a variety of strategies to enhance the ability to eliminate malignant cells.
The human body always keeps a delicate balance between injury and repair to avoid overcorrection. Over time eATP becomes less inflammatory or even anti-inflammatory due to the recruitment of Tregs and induction of ectoenzymes such as CD39 and CD73 (Figure 4) (69). As the critical components of the extracellular adenosinergic pathway, CD39 converts eATP and eADP to eAMP, and then CD73 converts eAMP to immunosuppressive adenosine (81). Moreover, another pathway generating adenosine involves participation of extracellular nicotinamide adenine dinucleotide (NAD+), CD38, CD203a, and CD73 (82). Like CD39 and CD73, alkaline phosphatase (ALP) and prostatic acid phosphatase (PAP) also can catalyze the conversion of eATP to adenosine (83, 84). Furthermore, the high concentration of intracellular adenosine can be transported outside the cell via equilibrative nucleoside transporters (ENTs) and concentrative nucleoside transporters (CNTs) to maintain balance (85).
The levels of extracellular adenosine are regulated by adenosine-converting enzymes such as adenosine kinase (ADK) and adenosine deaminase (ADA). Among them, ADK adds the residue of phosphoric acid to adenosine and converts it into AMP and ADA separates an amino group from adenosine with the formation of inosine (86). However, in the TME, high concentrations of adenosine binding to the corresponding receptors to inhibit the activation and expansion of various immune cells and promote the immune escape of cancers (86). The four known subtypes of adenosine receptors (A1R, A2AR, A2BR, and A3R), all of which are G-protein coupled receptors (GPCRs), have distinct expression patterns and mediate diverse signaling pathways (87). Regarding the respective role of adenosine receptors, it has been demonstrated that among the four subtypes, adenosine binding to A2AR and A2BR causes an increase in intracellular cyclic adenosine monophosphate (cAMP) and consequently the functional inhibition of immune cells, while A1R and A3R activation leads to tumor growth, cell proliferation and survival in some cases (88–90).
Adenosine accumulated in the TME is a major cause of immunosuppression (Figure 4). As the main force to eliminate malignant cells, the impairment of CD8+ T cells function and metabolic fitness are mediated by the A2AR/PKA/mTORC1 pathway as the main axis, due to the persistent high concentration of adenosine (91). Blocking the interaction of receptor with adenosine by a small-molecule A2AR antagonist can increase the recruitment of CD8+ T cells into the tumor and broaden the circulating T cell repertoire (92). Similarly, existing studies also indicate that immunosuppressive adenosine can impair the parenchymal CD4+ T cell and B cell response and infiltration (93, 94). Although NK cells rarely infiltrate cancers, their presence in tumor biopsies has been shown to positively associate with increased survival (95). As an intrinsic negative regulator of NK-cell maturation and anti-tumor immune responses, A2AR-mediated adenosine signaling can obviously limit tumor-infiltrating NK cells proliferation and activation (96). At the interface between the innate and adaptive immune system, dendritic cells (DCs) play key roles in inflammation and tumor immunity (97). However, adenosine and cAMP signaling can not only prevent DC maturation and development of effector functions but also skew DC differentiation towards a tolerogenic phenotype with defective CD8+ T cell priming capacity (98).
Extensive literature shows that eATP-mediated activation of purinergic receptor is necessary for the maturation and release of interleukin-1β by activated macrophages (99). Nevertheless, adenosine generated by eATP likely contributes to the differentiation and recruitment of tumor-associated macrophages (TAMs) which further amplify adenosine-dependent immunosuppression via additional ectonucleotidase activity of cancer cells (100). Myeloid-derived suppressor cells (MDSCs) are considered to be an important contributor to the immunosuppressive TME and thus an obstacle for many cancer immunotherapies. The metabolite adenosine plays a vital role in MDSCs mobilization through several mechanisms to inhibit T cell functions and promote cancer progression (101). In addition, elevated adenosine upregulates CD73 on cancer associated fibroblasts (CAFs) via A2BR-mediated pathway, thereby inciting the adenosine-A2BR-CD73 feedforward circuitry, which further augments immunosuppression by activating the non-redundant adenosine-A2AR pathway in immune cells to inhibit immune activation (102). For mesenchymal stromal cells (MSCs), the modulation of the adenosine overall promotes a more aggressive phenotype of cancers and more serious immunosuppressive function (103). Recently, Abhishek Tripathi et al. found a strong correlation between CD73, CD39 and A2AR expression, and Treg gene expression signature. Adenosine activates the high-affinity A2AR receptor, which in turn inhibits infiltrating NK cells and cytotoxic T lymphocytes (CTLs) activity and increases Tregs proliferation to further promote immunosuppression (104). Beyond the task of providing an immune-tolerant TME by helping to determine the activity of immune and inflammatory cells, the adenosine system directly regulates cancer growth and metastatic dissemination through specific receptors that are expressed on cancer cells (105).
Overall, in the context of cancer, the accumulation of extracellular adenosine inhibits the normal function of immune effector cells and facilitate the effect of immunosuppressive cells to foster malignant cells proliferation and migration.
Extracellular release of the central cellular energy metabolite ATP has although evolved as a natural signal for cellular distress, immunogenic cell death (ICD) and the recruitment and activation of immune cells (106). Ectonucleotidases which up-regulated in many types of cancer, such as CD39 and CD73, rapidly metabolize eATP to immunosuppressive adenosine, thereafter exacerbating immunosuppression in the TME (107).
Similar to other malignancies, the expression of CD39 and CD73 is synergistically increased in GC, causing a poor outcome for patients (61, 108). Under the dysfunction of mitochondria, GC cells preferentially utilize both glycolytic and pentose phosphate pathways rather than electron transport chains to desperately generate ATP, classically recognized as the Warburg effect, to provide substrates for adenosine production (109). Importantly, CD73 is also a hypoxia-responsive gene and promotes the Warburg effect of GC dependent on its enzyme activity to further amplifying adenosine signal transduction (110). Immunosuppressive adenosine can enhance the stemness of GC to resist treatment and promote the expression of epithelial-mesenchymal transition-associated genes to stimulate GC cell invasion and metastasis via interaction with A2AR and subsequent activation of the PI3K/AKT/mTOR pathway (111, 112). Furthermore, pathway and gene set enrichment analysis of transcriptome data revealed the modulation role of adenosine in RICS/RhoA signaling, which subsequently inhibited phosphorylation of LIMK/cofilin and promoted β-catenin activation to induce metastasis of GC (63).
Long-term accumulation of adenosine in GC helps to establish the immunosuppressive TME and promote tumor development through its interaction with tumor parenchyma and stromal cells (113). For immune cells, tumor-associated Tregs express more CD39 and CD73 in GC tissue. They also can decompose eATP to adenosine and in turn not only induce apoptosis and inhibit the proliferation of CD8+ T cells through the A2AR pathway but also prevent the infiltration of effector T cells into the TME (114, 115). Moreover, Hanyuan Liu et al. found that CD73 high expression GC showed a specific microenvironment with more CD8+ T cell infiltration via recruiting 902 GC patients to examine CD73 expression and immune contexture, but these CD8+ T cells displayed a dysfunctional phenotype for anti-tumor immunity (62). As a bypass pathway for adenosine production, restraining the conversion of NAD+ to adenosine can improve the function of effector CD8+ T cells and induce the apoptosis of GC cells simultaneously (116).
Though lots of systemic and in-depth researches on the role of the CD39-CD73-adenosine axis in diseases have been implemented, such as cardiovascular diseases, autoimmune disease, gut inflammation, and other cancers, immune checkpoint therapy targeting adenosine pathway in GC is still in the early phase (113, 117–119). With the use of small molecule inhibitors and monoclonal antibodies targeting adenosine pathway, an increasing number of clinical trials designed for GC treatment are ongoing, yet few successful experiences have been identified thus far (Table 2). Therefore, further exploration is still needed to complement the deficiencies of this immunotherapy method for GC patients.
The considerable heterogeneity and immunosuppressive TME represent major obstacles to accurate diagnosis and effective treatment in GC patients, leading to ineffective immunotherapy (120). For tumor heterogeneity, the molecular classification of GC extends the potential for personalized treatments to benefit each patient and fulfill the concept of precision medicine (121). The development of GC is a complex process displaying polytropic cell and molecular landscape within the TME, which supports tumor growth, metastasis, and recurrence, and function as the soil for gastric tumorigenesis (122). There is increasing evidence that reprogrammed energy metabolism contributes to the development of tumor suppressive immune microenvironment and influences the course of GC (123).
As a common metabolite, immunosuppressive adenosine has been intensively studied in many benign and malignant diseases, nevertheless, few researchers are currently exploring this avenue in GC. Although the efficacy of multiple small-molecule antagonists and antibodies of CD39-CD73-adenosine signaling pathway are being verified in a variety of diseases, deficiencies such as inefficacy and excessive inflammation cannot be ignored. Based on both, further research should mainly focus on the following aspects to obtain better curative effect:
Adenosine signaling, as one of the key components in regulating normal immune responses, induces immune tolerance to prevent an overreaction with self and the development of autoimmune disease (124). Due to the clinical experience with adenosine pathway inhibitors in oncology is limited, long-term exposure to these drugs and their association with other anti-tumor treatments could potentially lead to the emergence of systemic multiorgan toxicity (125). Therefore, the development of new drugs should also pay attention to its safety.
Multiple pathways can contribute to the production of adenosine, some of them by traditional CD39/CD73-dependent mechanisms, others by alternative pathways. In order to disrupt the adenosine production, Nathalie Bonnefoy et al. generated two antibodies, IPH5201 and IPH5301, targeting human membrane-associated and soluble forms of CD39 and CD73, respectively, and efficiently blocking the hydrolysis of immunogenic ATP into immunosuppressive adenosine. Their results suggested that the concomitant blockade of both CD39 and CD73 immunosuppressive enzymes can limit adenosine-mediated T cell inhibition, thereby enhancing anti-tumor immunity (126). Similarly, the simultaneous inhibition CD39 and CD73 cell surface ectonucleotidases by small molecular inhibitors can enhance the mobilization of bone marrow residing stem cells by decreasing the extracellular level of adenosine (127). In addition, co-targeting CD73 and A2AR strategy is also a promising novel therapeutic strategy for future hepatocellular carcinoma management (128). More interestingly, the alternative pathways can compensate the lack of adenosine production when the CD39/CD73/adenosine axis is blocked (129). Hence, a strong rationale exists for combining several inhibitions with the aim of more completely blunting adenosine production and signaling, but no similar research has been conducted on GC. It is worth noting that the combination therapy may improves the treatment outcome but it also carries more side-effect burden.
Systemic immunosuppression greatly affects the chemotherapeutic anti-tumor effect. CD39 cell-surface expression and activity is increased in patients with acute myeloid leukemia (AML) upon chemotherapy compared with diagnosis, and enrichment in CD39-expressing blasts is a marker of adverse prognosis in the clinic (130). Furthermore, extracellular vesicles from B cells through CD39 and CD73 vesicle-incorporated proteins hydrolyze eATP from chemotherapy-treated tumor cells into adenosine, thus impairing CD8+ T cell responses (131). As receptor for adenosine signaling, elevated A2AR expression was also detected in recurrent tumor tissues with induction chemotherapy (132). These phenomena offer a preclinical proof for the administration of adenosine signaling inhibitors in combination with chemotherapy in cancers, possibly including GC. Notably, the addition of HER2-targeted therapies to first-line chemotherapy has improved the OS of patients with HER2-positive GC, and has become the standard-of-care treatment for this group of patients (133). In breast cancer, high levels of CD73 gene expression are associated significantly with poor clinical outcome and promote resistance to HER2 antibody therapy (134). However, whether inhibitors of adenosinergic signaling pathway can be used to increase the efficacy of HER2-targeted therapy in GC needs to be further demonstrated. Various forms of immunotherapy are proving to be effective at restoring T cell-mediated immune responses that can lead to marked and sustained clinical responses, especially ICIs and CAR T-cell therapy. However, the efficacy of various immunotherapies for solid tumor is still mediocre because of immunosuppression in the TME. Hypoxia and cell damage, as common phenomena in solid tumors, are strongly linked to hallmarks of cancers and facilitate the production of immunosuppressive adenosine. The studies revealed that targeted blockade of CD73 can enhance the therapeutic activity of anti-PD-1 and anti-CTLA-4 monoclonal antibodies and may thus potentiate therapeutic strategies targeting ICIs for colorectal cancer, breast cancer, and prostate cancer (126, 135). Previous studies have shown that adenosine generated by tumor cells potently inhibits CAR T-cell responses through activation of A2AR. Therefore, using either A2AR antagonists or genetic targeting of A2AR using short hairpin RNA can profoundly increase CAR T-cell efficacy, particularly when combined with PD-1 blockade (136). In addition, disrupting A2AR gene in human CAR T-cell with CRISPR-Cas9 increased the anti-tumor function and prevented the exhaustion of CAR T-cells (137). Mechanistically, human A2AR-edited CAR T-cells are significantly resistant to adenosine-mediated transcriptional changes, resulting in enhanced production of cytokines including interferon-γ and tumor necrosis factor-α, and increased expression of JAK-STAT signaling pathway associated genes (138). The purpose of combination therapy is to combine separate mechanisms of action that will make malignant cells more sensitive to therapeutic agent and acquire better curative effect, but no similar research has been conducted on GC.
In addition to the above methods, accelerating the metabolism of adenosine within TME also can restore an anti-tumor immune competence. Emanuele Sasso et al. encoded adenosine deaminase (ADA)into an oncolytic targeted herpes virus to improve enzyme secretion for the metabolism of adenosine, and the clearance of adenosine within the TME reversed HER2-positive breast cancer resistance to trastuzumab (139).
The growth and progression of solid tumors are strongly affected by adenosine metabolic changes and interplay with the TME that sustain tumor development and immune escape. We explored the expression pattern and prognostic value of common immune checkpoints in GC patients via GEPIA database. Compared with other targets, adenosinergic pathway plays an indispensable role in the occurrence and development of GC, especially CD73. The components of adenosinergic pathway on both GC cells and immune cells sustains immunosuppressive TME by affecting multiple aspects of the immune response. Furthermore, some emerging antagonists of adenosinergic pathway show therapeutic potential in the preliminary studies of other malignancies. Therefore, these findings uncovered a mechanism by which immunosuppressive adenosine participates in the immune tolerance of GC, implying the potential of adenosinergic pathway as a therapeutic target or predictive marker for GC patients. However, On the basis of the limited evidence available as of now, elaborate clinical evaluation is further warranted to confirm whether the adenosinergic-targeting therapies are suitable for GC patients.
J-QW: writing of original manuscript. L-YD: revision of the manuscript. X-JC: language modification of the manuscript. All authors contributed to the article and approved the submitted version.
This work was supported by Wenzhou Science & Technology Bureau Foundation (Grant No. Y2020144 to X-JC) and National Natural Science Foundation of China under (Grant No. 81902151 to L-YD).
All authors contributed to the conception of the study and the preparation and approval of the paper.
The authors declare that the research was conducted in the absence of any commercial or financial relationships that could be construed as a potential conflict of interest.
All claims expressed in this article are solely those of the authors and do not necessarily represent those of their affiliated organizations, or those of the publisher, the editors and the reviewers. Any product that may be evaluated in this article, or claim that may be made by its manufacturer, is not guaranteed or endorsed by the publisher.
GC, gastric cancer; ICI, immune checkpoint inhibitor; AGC, advanced gastric cancer; GEPIA, Gene Expression Profiling Interactive Analysis; EGC, early gastric cancer; HER2, human epidermal growth factor receptor 2; CAR, T-cell chimeric antigen receptor-modified T cell; CTLA-4, cytotoxic T lymphocyte-associated antigen 4; PD-1/PD-L1, programmed cell death receptor 1/programmed cell death ligand 1; OS, overall survival; PFS, progression-free survival; eAMP, extracellular adenosine monophosphate; eADP, extracellular adenosine diphosphate; eATP, extracellular adenosine triphosphate; TME, tumor microenvironment; NK, natural killer cell; Tregs, regulatory T cells; DC, dendritic cell.
1. Sexton RE, Al Hallak MN, Diab M, Azmi AS. Gastric cancer: A comprehensive review of current and future treatment strategies. Cancer Metastasis Rev (2020) 39(4):1179–203. doi: 10.1007/s10555-020-09925-3
2. Li GZ, Doherty GM, Wang J. Surgical management of gastric cancer: A review. JAMA Surg (2022) 157(5):446–54. doi: 10.1001/jamasurg.2022.0182
3. Ngamruengphong S, Ferri L, Aihara H, Draganov PV, Yang DJ, Perbtani YB, et al. Efficacy of endoscopic submucosal dissection for superficial gastric neoplasia in a Large cohort in north America. Clin Gastroenterol Hepatol (2021) 19(8):1611–9.e1. doi: 10.1016/j.cgh.2020.06.023
4. Li WQ, Qin XX, Li ZX, Wang LH, Liu ZC, Fan XH, et al. Beneficial effects of endoscopic screening on gastric cancer and optimal screening interval: A population-based study. Endoscopy (2021) 54(9):848–58. doi: 10.1055/a-1728-5673
5. He X, Wu L, Dong Z, Gong D, Jiang X, Zhang H, et al. Real-time use of artificial intelligence for diagnosing early gastric cancer by magnifying image-enhanced endoscopy: A multicenter diagnostic study (with videos). Gastrointest Endosc (2022) 95(4):671–8.e4. doi: 10.1016/j.gie.2021.11.040
6. Jin X, Liu Z, Yang D, Yin K, Chang X. Recent progress and future perspectives of immunotherapy in advanced gastric cancer. Front Immunol (2022) 13:948647. doi: 10.3389/fimmu.2022.948647
7. Zhu X, Su T, Wang S, Zhou H, Shi W. New advances in nano-drug delivery systems: Helicobacter pylori and gastric cancer. Front Oncol (2022) 12:834934. doi: 10.3389/fonc.2022.834934
8. Bausys A, Mazeikaite M, Bickaite K, Bausys B, Bausys R, Strupas K. The role of prehabilitation in modern esophagogastric cancer surgery: A comprehensive review. Cancers (Basel) (2022) 14(9):2096. doi: 10.3390/cancers14092096
9. Li Z, Bai B, Zhao Y, Yu D, Lian B, Liu Y, et al. Severity of complications and long-term survival after laparoscopic total gastrectomy with D2 lymph node dissection for advanced gastric cancer: A propensity score-matched, case-control study. Int J Surg (2018) 54(Pt A):62–9. doi: 10.1016/j.ijsu.2018.04.034
10. Jiang H, Cui J, Chu H, Xu T, Xie M, Jing X, et al. Targeting Il8 as a sequential therapy strategy to overcome chemotherapy resistance in advanced gastric cancer. Cell Death Discovery (2022) 8(1):235. doi: 10.1038/s41420-022-01033-1
11. Li H, Huang H, Zhang T, Feng H, Wang S, Zhang Y, et al. Apatinib: A novel antiangiogenic drug in monotherapy or combination immunotherapy for digestive system malignancies. Front Immunol (2022) 13:937307. doi: 10.3389/fimmu.2022.937307
12. Zhu Y, Zhu X, Wei X, Tang C, Zhang W. Her2-targeted therapies in gastric cancer. Biochim Biophys Acta Rev Cancer (2021) 1876(1):188549. doi: 10.1016/j.bbcan.2021.188549
13. Myer NM, Shitara K, Chung HC, Lordick F, Kelly RJ, Szabo Z, et al. Evolution of predictive and prognostic biomarkers in the treatment of advanced gastric cancer. J Cancer Res Clin Oncol (2022) 148(8):2023–43. doi: 10.1007/s00432-021-03902-1
14. Kumar V, Ramnarayanan K, Sundar R, Padmanabhan N, Srivastava S, Koiwa M, et al. Single-cell atlas of lineage states, tumor microenvironment, and subtype-specific expression programs in gastric cancer. Cancer Discovery (2022) 12(3):670–91. doi: 10.1158/2159-8290.Cd-21-0683
15. Finck AV, Blanchard T, Roselle CP, Golinelli G, June CH. Engineered cellular immunotherapies in cancer and beyond. Nat Med (2022) 28(4):678–89. doi: 10.1038/s41591-022-01765-8
16. Havel JJ, Chowell D, Chan TA. The evolving landscape of biomarkers for checkpoint inhibitor immunotherapy. Nat Rev Cancer (2019) 19(3):133–50. doi: 10.1038/s41568-019-0116-x
17. Högner A, Moehler M. Immunotherapy in gastric cancer. Curr Oncol (2022) 29(3):1559–74. doi: 10.3390/curroncol29030131
18. Myint ZW, Goel G. Role of modern immunotherapy in gastrointestinal malignancies: A review of current clinical progress. J Hematol Oncol (2017) 10(1):86. doi: 10.1186/s13045-017-0454-7
19. Shitara K, Van Cutsem E, Bang YJ, Fuchs C, Wyrwicz L, Lee KW, et al. Efficacy and safety of pembrolizumab or pembrolizumab plus chemotherapy vs chemotherapy alone for patients with first-line, advanced gastric cancer: The keynote-062 phase 3 randomized clinical trial. JAMA Oncol (2020) 6(10):1571–80. doi: 10.1001/jamaoncol.2020.3370
20. Li S, Gao J, Xu Q, Zhang X, Huang M, Dai X, et al. A signature-based classification of gastric cancer that stratifies tumor immunity and predicts responses to pd-1 inhibitors. Front Immunol (2021) 12:693314. doi: 10.3389/fimmu.2021.693314
21. Takei S, Kawazoe A, Shitara K. The new era of immunotherapy in gastric cancer. Cancers (Basel) (2022) 14(4):1054. doi: 10.3390/cancers14041054
22. Shitara K, Özgüroğlu M, Bang YJ, Di Bartolomeo M, Mandalà M, Ryu MH, et al. Molecular determinants of clinical outcomes with pembrolizumab versus paclitaxel in a randomized, open-label, phase iii trial in patients with gastroesophageal adenocarcinoma. Ann Oncol (2021) 32(9):1127–36. doi: 10.1016/j.annonc.2021.05.803
23. Wang J, Sun J, Liu LN, Flies DB, Nie X, Toki M, et al. Siglec-15 as an immune suppressor and potential target for normalization cancer immunotherapy. Nat Med (2019) 25(4):656–66. doi: 10.1038/s41591-019-0374-x
24. Sun J, Lu Q, Sanmamed MF, Wang J. Siglec-15 as an emerging target for next-generation cancer immunotherapy. Clin Cancer Res (2021) 27(3):680–8. doi: 10.1158/1078-0432.Ccr-19-2925
25. Podojil JR, Miller SD. Potential targeting of B7-H4 for the treatment of cancer. Immunol Rev (2017) 276(1):40–51. doi: 10.1111/imr.12530
26. John P, Wei Y, Liu W, Du M, Guan F, Zang X. The B7x immune checkpoint pathway: From discovery to clinical trial. Trends Pharmacol Sci (2019) 40(11):883–96. doi: 10.1016/j.tips.2019.09.008
27. Rieder SA, Wang J, White N, Qadri A, Menard C, Stephens G, et al. B7-H7 (Hhla2) inhibits T-cell activation and proliferation in the presence of tcr and Cd28 signaling. Cell Mol Immunol (2021) 18(6):1503–11. doi: 10.1038/s41423-020-0361-7
28. Wei Y, Ren X, Galbo PM Jr., Moerdler S, Wang H, Sica RA, et al. Kir3dl3-Hhla2 is a human immunosuppressive pathway and a therapeutic target. Sci Immunol (2021) 6(61):eabf9792. doi: 10.1126/sciimmunol.abf9792
29. Nimmerjahn F. Keeping T-he killers at bay Via fcγriib. Immunity (2020) 52(1):9–11. doi: 10.1016/j.immuni.2019.12.012
30. Morris AB, Farley CR, Pinelli DF, Adams LE, Cragg MS, Boss JM, et al. Signaling through the inhibitory fc receptor fcγriib induces Cd8(+) t cell apoptosis to limit T cell immunity. Immunity (2020) 52(1):136–50.e6. doi: 10.1016/j.immuni.2019.12.006
31. Moesta AK, Li XY, Smyth MJ. Targeting Cd39 in cancer. Nat Rev Immunol (2020) 20(12):739–55. doi: 10.1038/s41577-020-0376-4
32. Li XY, Moesta AK, Xiao C, Nakamura K, Casey M, Zhang H, et al. Targeting Cd39 in cancer reveals an extracellular atp- and inflammasome-driven tumor immunity. Cancer Discovery (2019) 9(12):1754–73. doi: 10.1158/2159-8290.Cd-19-0541
33. Freed-Pastor WA, Lambert LJ, Ely ZA, Pattada NB, Bhutkar A, Eng G, et al. The Cd155/Tigit axis promotes and maintains immune evasion in neoantigen-expressing pancreatic cancer. Cancer Cell (2021) 39(10):1342–60.e14. doi: 10.1016/j.ccell.2021.07.007
34. Kučan Brlić P, Lenac Roviš T, Cinamon G, Tsukerman P, Mandelboim O, Jonjić S. Targeting pvr (Cd155) and its receptors in anti-tumor therapy. Cell Mol Immunol (2019) 16(1):40–52. doi: 10.1038/s41423-018-0168-y
35. Wang X, Liu M, Zhang J, Brown NK, Zhang P, Zhang Y, et al. Cd24-siglec axis is an innate immune checkpoint against metaflammation and metabolic disorder. Cell Metab (2022) 34(8):1088–103.e6. doi: 10.1016/j.cmet.2022.07.005
36. Barkal AA, Brewer RE, Markovic M, Kowarsky M, Barkal SA, Zaro BW, et al. Cd24 signalling through macrophage siglec-10 is a target for cancer immunotherapy. Nature (2019) 572(7769):392–6. doi: 10.1038/s41586-019-1456-0
37. Xiong Z, Ampudia Mesias E, Pluhar GE, Rathe SK, Largaespada DA, Sham YY, et al. Cd200 checkpoint reversal: A novel approach to immunotherapy. Clin Cancer Res (2020) 26(1):232–41. doi: 10.1158/1078-0432.Ccr-19-2234
38. Choueiry F, Torok M, Shakya R, Agrawal K, Deems A, Benner B, et al. Cd200 promotes immunosuppression in the pancreatic tumor microenvironment. J Immunother Cancer (2020) 8(1):e000189. doi: 10.1136/jitc-2019-000189
39. Malissen N, Macagno N, Granjeaud S, Granier C, Moutardier V, Gaudy-Marqueste C, et al. Hvem has a broader expression than pd-L1 and constitutes a negative prognostic marker and potential treatment target for melanoma. Oncoimmunology (2019) 8(12):e1665976. doi: 10.1080/2162402x.2019.1665976
40. Mintz MA, Felce JH, Chou MY, Mayya V, Xu Y, Shui JW, et al. The hvem-btla axis restrains T cell help to germinal center b cells and functions as a cell-extrinsic suppressor in lymphomagenesis. Immunity (2019) 51(2):310–23.e7. doi: 10.1016/j.immuni.2019.05.022
41. Yang R, Sun L, Li CF, Wang YH, Yao J, Li H, et al. Galectin-9 interacts with pd-1 and Tim-3 to regulate T cell death and is a target for cancer immunotherapy. Nat Commun (2021) 12(1):832. doi: 10.1038/s41467-021-21099-2
42. Giovannone N, Liang J, Antonopoulos A, Geddes Sweeney J, King SL, Pochebit SM, et al. Galectin-9 suppresses b cell receptor signaling and is regulated by I-branching of n-glycans. Nat Commun (2018) 9(1):3287. doi: 10.1038/s41467-018-05770-9
43. Targeting Cd73 may improve immunotherapy efficacy in glioblastoma. Cancer Discovery (2020) 10(2):175. doi: 10.1158/2159-8290.Cd-rw2020-005
44. Young A, Ngiow SF, Barkauskas DS, Sult E, Hay C, Blake SJ, et al. Co-Inhibition of Cd73 and A2ar adenosine signaling improves anti-tumor immune responses. Cancer Cell (2016) 30(3):391–403. doi: 10.1016/j.ccell.2016.06.025
45. Crunkhorn S. Understanding Lag3 effects on T cells. Nat Rev Drug Discovery (2022) 21(6):416. doi: 10.1038/d41573-022-00083-5
46. Mullard A. Lag3 pushes immuno-oncology’s leading edge. Nat Rev Drug Discovery (2022) 21(3):167–9. doi: 10.1038/d41573-022-00036-y
47. Mullard A. Immuno-oncology target tigit attracts a new contender. Nat Rev Drug Discovery (2021) 20(8):576. doi: 10.1038/d41573-021-00123-6
48. Banta KL, Xu X, Chitre AS, Au-Yeung A, Takahashi C, O’Gorman WE, et al. Mechanistic convergence of the tigit and pd-1 inhibitory pathways necessitates Co-blockade to optimize anti-tumor Cd8(+) T cell responses. Immunity (2022) 55(3):512–26.e9. doi: 10.1016/j.immuni.2022.02.005
49. Huang X, Zhang X, Li E, Zhang G, Wang X, Tang T, et al. Vista: An immune regulatory protein checking tumor and immune cells in cancer immunotherapy. J Hematol Oncol (2020) 13(1):83. doi: 10.1186/s13045-020-00917-y
50. ElTanbouly MA, Zhao Y, Nowak E, Li J, Schaafsma E, Le Mercier I, et al. Vista is a checkpoint regulator for naïve T cell quiescence and peripheral tolerance. Science (2020) 367(6475):eaay0524. doi: 10.1126/science.aay0524
51. Kennedy R, Klein U. A T cell-b cell tumor-suppressive axis in the germinal center. Immunity (2019) 51(2):204–6. doi: 10.1016/j.immuni.2019.07.006
52. Jones A, Bourque J, Kuehm L, Opejin A, Teague RM, Gross C, et al. Immunomodulatory functions of btla and hvem govern induction of extrathymic regulatory T cells and tolerance by dendritic cells. Immunity (2016) 45(5):1066–77. doi: 10.1016/j.immuni.2016.10.008
53. Goodman A, Patel SP, Kurzrock R. Pd-1-Pd-L1 immune-checkpoint blockade in b-cell lymphomas. Nat Rev Clin Oncol (2017) 14(4):203–20. doi: 10.1038/nrclinonc.2016.168
54. Jiang X, Wang J, Deng X, Xiong F, Ge J, Xiang B, et al. Role of the tumor microenvironment in pd-L1/Pd-1-Mediated tumor immune escape. Mol Cancer (2019) 18(1):10. doi: 10.1186/s12943-018-0928-4
55. Kanchan RK, Doss D, Khan P, Nasser MW, Mahapatra S. To kill a cancer: Targeting the immune inhibitory checkpoint molecule, B7-H3. Biochim Biophys Acta Rev Cancer (2022) 1877(5):188783. doi: 10.1016/j.bbcan.2022.188783
56. Yang S, Wei W, Zhao Q. B7-H3, a checkpoint molecule, as a target for cancer immunotherapy. Int J Biol Sci (2020) 16(11):1767–73. doi: 10.7150/ijbs.41105
57. Du X, Tang F, Liu M, Su J, Zhang Y, Wu W, et al. A reappraisal of ctla-4 checkpoint blockade in cancer immunotherapy. Cell Res (2018) 28(4):416–32. doi: 10.1038/s41422-018-0011-0
58. Rowshanravan B, Halliday N, Sansom DM. Ctla-4: A moving target in immunotherapy. Blood (2018) 131(1):58–67. doi: 10.1182/blood-2017-06-741033
59. Feng M, Jiang W, Kim BYS, Zhang CC, Fu YX, Weissman IL. Phagocytosis checkpoints as new targets for cancer immunotherapy. Nat Rev Cancer (2019) 19(10):568–86. doi: 10.1038/s41568-019-0183-z
60. Logtenberg MEW, Scheeren FA, Schumacher TN. The Cd47-sirpα immune checkpoint. Immunity (2020) 52(5):742–52. doi: 10.1016/j.immuni.2020.04.011
61. Jiang T, Xu X, Qiao M, Li X, Zhao C, Zhou F, et al. Comprehensive evaluation of Nt5e/Cd73 expression and its prognostic significance in distinct types of cancers. BMC Cancer (2018) 18(1):267. doi: 10.1186/s12885-018-4073-7
62. He X, Gu Y, Cao Y, Hu B, Fang H, Fei Y, et al. Impact of intratumoural Cd73 expression on prognosis and therapeutic response in patients with gastric cancer. Eur J Cancer (2021) 157:114–23. doi: 10.1016/j.ejca.2021.08.006
63. Xu Z, Gu C, Yao X, Guo W, Wang H, Lin T, et al. Cd73 promotes tumor metastasis by modulating Rics/Rhoa signaling and emt in gastric cancer. Cell Death Dis (2020) 11(3):202. doi: 10.1038/s41419-020-2403-6
64. Liu Z, Wu X, Wang Q, Li Z, Liu X, Sheng X, et al. Cd73-adenosine a(1)R axis regulates the activation and apoptosis of hepatic stellate cells through the plc-Ip(3)-Ca(2+)/Dag-Pkc signaling pathway. Front Pharmacol (2022) 13:922885. doi: 10.3389/fphar.2022.922885
65. Nagate Y, Ezoe S, Fujita J, Okuzaki D, Motooka D, Ishibashi T, et al. Ectonucleotidase Cd39 is highly expressed on atll cells and is responsible for their immunosuppressive function. Leukemia (2021) 35(1):107–18. doi: 10.1038/s41375-020-0788-y
66. Franco R, Rivas-Santisteban R, Navarro G, Reyes-Resina I. Adenosine receptor antagonists to combat cancer and to boost anti-cancer chemotherapy and immunotherapy. Cells (2021) 10(11):2831. doi: 10.3390/cells10112831
67. Takenaka MC, Robson S, Quintana FJ. Regulation of the T cell response by Cd39. Trends Immunol (2016) 37(7):427–39. doi: 10.1016/j.it.2016.04.009
68. Kepp O, Bezu L, Yamazaki T, Di Virgilio F, Smyth MJ, Kroemer G, et al. Atp and cancer immunosurveillance. EMBO J (2021) 40(13):e108130. doi: 10.15252/embj.2021108130
69. Linden J, Koch-Nolte F, Dahl G. Purine release, metabolism, and signaling in the inflammatory response. Annu Rev Immunol (2019) 37:325–47. doi: 10.1146/annurev-immunol-051116-052406
70. de Andrade Mello P, Coutinho-Silva R, Savio LEB. Multifaceted effects of extracellular adenosine triphosphate and adenosine in the tumor-host interaction and therapeutic perspectives. Front Immunol (2017) 8:1526. doi: 10.3389/fimmu.2017.01526
71. Garcia-Jacobo RE, Bergamin LS, Vultaggio-Poma V, Thorstenberg ML, Tarantini M, García-Hernández MH, et al. The purinergic landscape of type 2 diabetes mellitus. Molecules (2022) 27(6):1838. doi: 10.3390/molecules27061838
72. Di Virgilio F, Sarti AC, Falzoni S, De Marchi E, Adinolfi E. Extracellular atp and P2 purinergic signalling in the tumour microenvironment. Nat Rev Cancer (2018) 18(10):601–18. doi: 10.1038/s41568-018-0037-0
73. McCarthy AE, Yoshioka C, Mansoor SE. Full-length P2x(7) structures reveal how palmitoylation prevents channel desensitization. Cell (2019) 179(3):659–70.e13. doi: 10.1016/j.cell.2019.09.017
74. Cao F, Hu LQ, Yao SR, Hu Y, Wang DG, Fan YG, et al. P2x7 receptor: A potential therapeutic target for autoimmune diseases. Autoimmun Rev (2019) 18(8):767–77. doi: 10.1016/j.autrev.2019.06.009
75. Zhang T, Tsutsuki H, Islam W, Ono K, Takeda K, Akaike T, et al. Atp exposure stimulates glutathione efflux as a necessary switch for Nlrp3 inflammasome activation. Redox Biol (2021) 41:101930. doi: 10.1016/j.redox.2021.101930
76. Ahn Y, Seo J, Lee EJ, Kim JY, Park MY, Hwang S, et al. Atp-P2x7-Induced inflammasome activation contributes to melanocyte death and Cd8(+) T-cell trafficking to the skin in vitiligo. J Invest Dermatol (2020) 140(9):1794–804.e4. doi: 10.1016/j.jid.2019.12.035
77. Scott BM, Gutiérrez-Vázquez C, Sanmarco LM, da Silva Pereira JA, Li Z, Plasencia A, et al. Self-tunable engineered yeast probiotics for the treatment of inflammatory bowel disease. Nat Med (2021) 27(7):1212–22. doi: 10.1038/s41591-021-01390-x
78. Kaplanski G. Interleukin-18: Biological properties and role in disease pathogenesis. Immunol Rev (2018) 281(1):138–53. doi: 10.1111/imr.12616
79. Schenk U, Frascoli M, Proietti M, Geffers R, Traggiai E, Buer J, et al. Atp inhibits the generation and function of regulatory T cells through the activation of purinergic P2x receptors. Sci Signaling (2011) 4(162):ra12–ra. doi: 10.1126/scisignal.2001270
80. Cuthbertson P, Geraghty NJ, Adhikary SR, Casolin S, Watson D, Sluyter R. P2x7 receptor antagonism increases regulatory T cells and reduces clinical and histological graft-Versus-Host disease in a humanised mouse model. Clin Sci (Lond) (2021) 135(3):495–513. doi: 10.1042/cs20201352
81. Yang R, Elsaadi S, Misund K, Abdollahi P, Vandsemb EN, Moen SH, et al. Conversion of atp to adenosine by Cd39 and Cd73 in multiple myeloma can be successfully targeted together with adenosine receptor A2a blockade. J Immunother Cancer (2020) 8(1):e000610. doi: 10.1136/jitc-2020-000610
82. Horenstein AL, Chillemi A, Zini R, Quarona V, Bianchi N, Manfredini R, et al. Cytokine-induced killer cells express Cd39, Cd38, Cd203a, Cd73 ectoenzymes and P1 adenosinergic receptors. Front Pharmacol (2018) 9:196. doi: 10.3389/fphar.2018.00196
83. Jackson EK, Cheng D, Verrier JD, Janesko-Feldman K, Kochanek PM. Interactive roles of Cd73 and tissue nonspecific alkaline phosphatase in the renal vascular metabolism of 5’-amp. Am J Physiol Renal Physiol (2014) 307(6):F680–5. doi: 10.1152/ajprenal.00312.2014
84. Alarcón S, Toro M, Villarreal C, Melo R, Fernández R, Ayuso Sacido A, et al. Decreased equilibrative nucleoside transporter 1 (Ent1) activity contributes to the high extracellular adenosine levels in mesenchymal glioblastoma stem-like cells. Cells (2020) 9(8):1914. doi: 10.3390/cells9081914
85. Aherne CM, Collins CB, Rapp CR, Olli KE, Perrenoud L, Jedlicka P, et al. Coordination of Ent2-dependent adenosine transport and signaling dampens mucosal inflammation. JCI Insight (2018) 3(20):e121521. doi: 10.1172/jci.insight.121521
86. Zhulai G, Oleinik E, Shibaev M, Ignatev K. Adenosine-metabolizing enzymes, adenosine kinase and adenosine deaminase, in cancer. Biomolecules (2022) 12(3):418. doi: 10.3390/biom12030418
87. Sek K, Mølck C, Stewart GD, Kats L, Darcy PK, Beavis PA. Targeting adenosine receptor signaling in cancer immunotherapy. Int J Mol Sci (2018) 19(12):3837. doi: 10.3390/ijms19123837
88. Muller-Haegele S, Muller L, Whiteside TL. Immunoregulatory activity of adenosine and its role in human cancer progression. Expert Rev Clin Immunol (2014) 10(7):897–914. doi: 10.1586/1744666x.2014.915739
89. Mazziotta C, Rotondo JC, Lanzillotti C, Campione G, Martini F, Tognon M. Cancer biology and molecular genetics of a(3) adenosine receptor. Oncogene (2022) 41(3):301–8. doi: 10.1038/s41388-021-02090-z
90. Gao ZG, Jacobson KA. A(2b) adenosine receptor and cancer. Int J Mol Sci (2019) 20(20):5139. doi: 10.3390/ijms20205139
91. Mastelic-Gavillet B, Navarro Rodrigo B, Décombaz L, Wang H, Ercolano G, Ahmed R, et al. Adenosine mediates functional and metabolic suppression of peripheral and tumor-infiltrating Cd8(+) T cells. J Immunother Cancer (2019) 7(1):257. doi: 10.1186/s40425-019-0719-5
92. Fong L, Hotson A, Powderly JD, Sznol M, Heist RS, Choueiri TK, et al. Adenosine 2a receptor blockade as an immunotherapy for treatment-refractory renal cell cancer. Cancer Discovery (2020) 10(1):40–53. doi: 10.1158/2159-8290.Cd-19-0980
93. Amaral EP, Machado de Salles É, Barbosa Bomfim CC, Salgado RM, Almeida FM, de Souza PC, et al. Inhibiting adenosine receptor signaling promotes accumulation of effector Cd4+ T cells in the lung parenchyma during severe tuberculosis. J Infect Dis (2019) 219(6):964–74. doi: 10.1093/infdis/jiy586
94. Zhou SN, Zhang N, Liu HH, Xia P, Zhang C, Song JW, et al. Skewed Cd39/Cd73/Adenosine pathway contributes to b-cell hyperactivation and disease progression in patients with chronic hepatitis b. Gastroenterol Rep (Oxf) (2021) 9(1):49–58. doi: 10.1093/gastro/goaa048
95. Neo SY, Yang Y, Record J, Ma R, Chen X, Chen Z, et al. Cd73 immune checkpoint defines regulatory nk cells within the tumor microenvironment. J Clin Invest (2020) 130(3):1185–98. doi: 10.1172/jci128895
96. Young A, Ngiow SF, Gao Y, Patch AM, Barkauskas DS, Messaoudene M, et al. A2ar adenosine signaling suppresses natural killer cell maturation in the tumor microenvironment. Cancer Res (2018) 78(4):1003–16. doi: 10.1158/0008-5472.Can-17-2826
97. Perez CR, De Palma M. Engineering dendritic cell vaccines to improve cancer immunotherapy. Nat Commun (2019) 10(1):5408. doi: 10.1038/s41467-019-13368-y
98. Challier J, Bruniquel D, Sewell AK, Laugel B. Adenosine and camp signalling skew human dendritic cell differentiation towards a tolerogenic phenotype with defective Cd8(+) T-cell priming capacity. Immunology (2013) 138(4):402–10. doi: 10.1111/imm.12053
99. Zumerle S, Calì B, Munari F, Angioni R, Di Virgilio F, Molon B, et al. Intercellular calcium signaling induced by atp potentiates macrophage phagocytosis. Cell Rep (2019) 27(1):1–10.e4. doi: 10.1016/j.celrep.2019.03.011
100. Montalbán Del Barrio I, Penski C, Schlahsa L, Stein RG, Diessner J, Wöckel A, et al. Adenosine-generating ovarian cancer cells attract myeloid cells which differentiate into adenosine-generating tumor associated macrophages - a self-amplifying, Cd39- and Cd73-dependent mechanism for tumor immune escape. J Immunother Cancer (2016) 4:49. doi: 10.1186/s40425-016-0154-9
101. Groth C, Hu X, Weber R, Fleming V, Altevogt P, Utikal J, et al. Immunosuppression mediated by myeloid-derived suppressor cells (Mdscs) during tumour progression. Br J Cancer (2019) 120(1):16–25. doi: 10.1038/s41416-018-0333-1
102. Yu M, Guo G, Huang L, Deng L, Chang CS, Achyut BR, et al. Cd73 on cancer-associated fibroblasts enhanced by the a(2b)-mediated feedforward circuit enforces an immune checkpoint. Nat Commun (2020) 11(1):515. doi: 10.1038/s41467-019-14060-x
103. Pietrobono D, Giacomelli C, Marchetti L, Martini C, Trincavelli ML. High adenosine extracellular levels induce glioblastoma aggressive traits modulating the mesenchymal stromal cell secretome. Int J Mol Sci (2020) 21(20):7706. doi: 10.3390/ijms21207706
104. Tripathi A, Lin E, Xie W, Flaifel A, Steinharter JA, Stern Gatof EN, et al. Prognostic significance and immune correlates of Cd73 expression in renal cell carcinoma. J Immunother Cancer (2020) 8(2):e001467. doi: 10.1136/jitc-2020-001467
105. Antonioli L, Blandizzi C, Pacher P, Haskó G. Immunity, inflammation and cancer: A leading role for adenosine. Nat Rev Cancer (2013) 13(12):842–57. doi: 10.1038/nrc3613
106. Draganov D, Lee PP. Purinergic signaling within the tumor microenvironment. Adv Exp Med Biol (2021) 1270:73–87. doi: 10.1007/978-3-030-47189-7_5
107. Mao C, Yeh S, Fu J, Porosnicu M, Thomas A, Kucera GL, et al. Delivery of an ectonucleotidase inhibitor with ros-responsive nanoparticles overcomes adenosine-mediated cancer immunosuppression. Sci Transl Med (2022) 14(648):eabh1261. doi: 10.1126/scitranslmed.abh1261
108. Cai XY, Wang XF, Li J, Dong JN, Liu JQ, Li NP, et al. High expression of Cd39 in gastric cancer reduces patient outcome following radical resection. Oncol Lett (2016) 12(5):4080–6. doi: 10.3892/ol.2016.5189
109. Nakachi S, Okamoto S, Tamaki K, Nomura I, Tomihama M, Nishi Y, et al. Impact of anti-diabetic sodium-glucose cotransporter 2 inhibitors on tumor growth of intractable hematological malignancy in humans. BioMed Pharmacother (2022) 149:112864. doi: 10.1016/j.biopha.2022.112864
110. Cao X, Zhu Z, Cao Y, Hu J, Min M. Cd73 is a hypoxia-responsive gene and promotes the warburg effect of human gastric cancer cells dependent on its enzyme activity. J Cancer (2021) 12(21):6372–82. doi: 10.7150/jca.62387
111. Shi L, Wu Z, Miao J, Du S, Ai S, Xu E, et al. Adenosine interaction with adenosine receptor A2a promotes gastric cancer metastasis by enhancing Pi3k-Akt-Mtor signaling. Mol Biol Cell (2019) 30(19):2527–34. doi: 10.1091/mbc.E19-03-0136
112. Liu G, Yang S, Liu Y, Xu Y, Qiu H, Sun J, et al. The adenosine-A2a receptor regulates the radioresistance of gastric cancer Via Pi3k-Akt-Mtor pathway. Int J Clin Oncol (2022) 27(5):911–20. doi: 10.1007/s10147-022-02123-x
113. Shi L, Yang L, Wu Z, Xu W, Song J, Guan W. Adenosine signaling: Next checkpoint for gastric cancer immunotherapy? Int Immunopharmacol (2018) 63:58–65. doi: 10.1016/j.intimp.2018.07.023
114. Shi L, Feng M, Du S, Wei X, Song H, Yixin X, et al. Adenosine generated by regulatory T cells induces Cd8(+) T cell exhaustion in gastric cancer through A2ar pathway. BioMed Res Int (2019) 2019:4093214. doi: 10.1155/2019/4093214
115. Sundström P, Stenstad H, Langenes V, Ahlmanner F, Theander L, Ndah TG, et al. Regulatory T cells from colon cancer patients inhibit effector T-cell migration through an adenosine-dependent mechanism. Cancer Immunol Res (2016) 4(3):183–93. doi: 10.1158/2326-6066.Cir-15-0050
116. Liu HY, Wang FH, Liang JM, Xiang YY, Liu SH, Zhang SW, et al. Targeting nad metabolism regulates extracellular adenosine levels to improve the cytotoxicity of Cd8+ effector T cells in the tumor microenvironment of gastric cancer. J Cancer Res Clin Oncol (2022). doi: 10.1007/s00432-022-04124-9
117. Sutton NR, Bouïs D, Mann KM, Rashid IM, McCubbrey AL, Hyman MC, et al. Cd73 promotes age-dependent accretion of atherosclerosis. Arterioscler Thromb Vasc Biol (2020) 40(1):61–71. doi: 10.1161/atvbaha.119.313002
118. Knight JS, Mazza LF, Yalavarthi S, Sule G, Ali RA, Hodgin JB, et al. Ectonucleotidase-mediated suppression of lupus autoimmunity and vascular dysfunction. Front Immunol (2018) 9:1322. doi: 10.3389/fimmu.2018.01322
119. Kulkarni N, Meitei HT, Sonar SA, Sharma PK, Mujeeb VR, Srivastava S, et al. Ccr6 signaling inhibits suppressor function of induced-treg during gut inflammation. J Autoimmun (2018) 88:121–30. doi: 10.1016/j.jaut.2017.10.013
120. Zhang M, Hu S, Min M, Ni Y, Lu Z, Sun X, et al. Dissecting transcriptional heterogeneity in primary gastric adenocarcinoma by single cell rna sequencing. Gut (2021) 70(3):464–75. doi: 10.1136/gutjnl-2019-320368
121. Ichikawa H, Nagahashi M, Shimada Y, Hanyu T, Ishikawa T, Kameyama H, et al. Actionable gene-based classification toward precision medicine in gastric cancer. Genome Med (2017) 9(1):93. doi: 10.1186/s13073-017-0484-3
122. Zhang H, Yang M, Wu X, Li Q, Li X, Zhao Y, et al. The distinct roles of exosomes in tumor-stroma crosstalk within gastric tumor microenvironment. Pharmacol Res (2021) 171:105785. doi: 10.1016/j.phrs.2021.105785
123. Zhao L, Liu Y, Zhang S, Wei L, Cheng H, Wang J, et al. Impacts and mechanisms of metabolic reprogramming of tumor microenvironment for immunotherapy in gastric cancer. Cell Death Dis (2022) 13(4):378. doi: 10.1038/s41419-022-04821-w
124. Nakahama T, Kawahara Y. Adenosine-to-Inosine rna editing in the immune system: Friend or foe? Cell Mol Life Sci (2020) 77(15):2931–48. doi: 10.1007/s00018-020-03466-2
125. Allard B, Allard D, Buisseret L, Stagg J. The adenosine pathway in immuno-oncology. Nat Rev Clin Oncol (2020) 17(10):611–29. doi: 10.1038/s41571-020-0382-2
126. Perrot I, Michaud HA, Giraudon-Paoli M, Augier S, Docquier A, Gros L, et al. Blocking antibodies targeting the Cd39/Cd73 immunosuppressive pathway unleash immune responses in combination cancer therapies. Cell Rep (2019) 27(8):2411–25.e9. doi: 10.1016/j.celrep.2019.04.091
127. Adamiak M, Bujko K, Brzezniakiewicz-Janus K, Kucia M, Ratajczak J, Ratajczak MZ. The inhibition of Cd39 and Cd73 cell surface ectonucleotidases by small molecular inhibitors enhances the mobilization of bone marrow residing stem cells by decreasing the extracellular level of adenosine. Stem Cell Rev Rep (2019) 15(6):892–9. doi: 10.1007/s12015-019-09918-y
128. Ma XL, Shen MN, Hu B, Wang BL, Yang WJ, Lv LH, et al. Cd73 promotes hepatocellular carcinoma progression and metastasis Via activating Pi3k/Akt signaling by inducing Rap1-mediated membrane localization of P110β and predicts poor prognosis. J Hematol Oncol (2019) 12(1):37. doi: 10.1186/s13045-019-0724-7
129. Wang Y, Copeland J, Shin M, Chang Y, Venton BJ. Cd73 or Cd39 deletion reveals different mechanisms of formation for spontaneous and mechanically stimulated adenosine and sex specific compensations in atp degradation. ACS Chem Neurosci (2020) 11(6):919–28. doi: 10.1021/acschemneuro.9b00620
130. Aroua N, Boet E, Ghisi M, Nicolau-Travers ML, Saland E, Gwilliam R, et al. Extracellular atp and Cd39 activate camp-mediated mitochondrial stress response to promote cytarabine resistance in acute myeloid leukemia. Cancer Discovery (2020) 10(10):1544–65. doi: 10.1158/2159-8290.Cd-19-1008
131. Zhang F, Li R, Yang Y, Shi C, Shen Y, Lu C, et al. Specific decrease in b-Cell-Derived extracellular vesicles enhances post-chemotherapeutic Cd8(+) T cell responses. Immunity (2019) 50(3):738–50.e7. doi: 10.1016/j.immuni.2019.01.010
132. Ma SR, Deng WW, Liu JF, Mao L, Yu GT, Bu LL, et al. Blockade of adenosine A2a receptor enhances Cd8(+) T cells response and decreases regulatory T cells in head and neck squamous cell carcinoma. Mol Cancer (2017) 16(1):99. doi: 10.1186/s12943-017-0665-0
133. Oh DY, Bang YJ. Her2-targeted therapies - a role beyond breast cancer. Nat Rev Clin Oncol (2020) 17(1):33–48. doi: 10.1038/s41571-019-0268-3
134. Turcotte M, Allard D, Mittal D, Bareche Y, Buisseret L, José V, et al. Cd73 promotes resistance to Her2/Erbb2 antibody therapy. Cancer Res (2017) 77(20):5652–63. doi: 10.1158/0008-5472.Can-17-0707
135. Allard B, Pommey S, Smyth MJ, Stagg J. Targeting Cd73 enhances the antitumor activity of anti-Pd-1 and anti-Ctla-4 mabs. Clin Cancer Res (2013) 19(20):5626–35. doi: 10.1158/1078-0432.Ccr-13-0545
136. Beavis PA, Henderson MA, Giuffrida L, Mills JK, Sek K, Cross RS, et al. Targeting the adenosine 2a receptor enhances chimeric antigen receptor T cell efficacy. J Clin Invest (2017) 127(3):929–41. doi: 10.1172/jci89455
137. Li N, Tang N, Cheng C, Hu T, Wei X, Han W, et al. Improving the anti-solid tumor efficacy of car-T cells by inhibiting adenosine signaling pathway. Oncoimmunology (2020) 9(1):1824643. doi: 10.1080/2162402x.2020.1824643
138. Giuffrida L, Sek K, Henderson MA, Lai J, Chen AXY, Meyran D, et al. Crispr/Cas9 mediated deletion of the adenosine A2a receptor enhances car T cell efficacy. Nat Commun (2021) 12(1):3236. doi: 10.1038/s41467-021-23331-5
Keywords: gastric cancer, CD39, CD73, adenosine, immunotherapy
Citation: Wang J, Du L and Chen X (2022) Adenosine signaling: Optimal target for gastric cancer immunotherapy. Front. Immunol. 13:1027838. doi: 10.3389/fimmu.2022.1027838
Received: 25 August 2022; Accepted: 05 September 2022;
Published: 16 September 2022.
Edited by:
Junjiang Fu, Southwest Medical University, ChinaReviewed by:
Jiao Wang, Purdue University, United StatesCopyright © 2022 Wang, Du and Chen. This is an open-access article distributed under the terms of the Creative Commons Attribution License (CC BY). The use, distribution or reproduction in other forums is permitted, provided the original author(s) and the copyright owner(s) are credited and that the original publication in this journal is cited, in accordance with accepted academic practice. No use, distribution or reproduction is permitted which does not comply with these terms.
*Correspondence: Xiangjian Chen, d3oxMzcwQDEyNi5jb20=; Linyong Du, ZHVsaW55b25nQHFxLmNvbQ==
Disclaimer: All claims expressed in this article are solely those of the authors and do not necessarily represent those of their affiliated organizations, or those of the publisher, the editors and the reviewers. Any product that may be evaluated in this article or claim that may be made by its manufacturer is not guaranteed or endorsed by the publisher.
Research integrity at Frontiers
Learn more about the work of our research integrity team to safeguard the quality of each article we publish.