- 1Beijing Ditan Hospital, Capital Medical University, Beijing, China
- 2Clinical Center for HIV/AIDS, Capital Medical University, Beijing, China
- 3Infectious Disease Department, Sichuan Provincial People’s Hospital, University of Electronic Science and Technology of China, Chengdu, China
After long-term anti-retroviral therapy (ART) treatment, most human immunodeficiency virus (HIV)/Acquired Immure Deficiency Syndrome (AIDS) patients can achieve virological suppression and gradual recovery of CD4+ T-lymphocyte (CD4+ T cell) counts. However, some patients still fail to attain normal CD4+ T cell counts; this group of patients are called immune non-responders (INRs), and these patients show severe immune dysfunction. The potential mechanism of poor immune reconstitution (PIR) remains unclear and the identification of uniform biomarkers to predict the occurrence of PIR is particularly vital. But limited information is available on the relationship between circulating markers of INRs and immune recovery. Hence, this review summarises alterations in the intestine microbiota and associated markers in the setting of PIR to better understand host-microbiota-metabolite interactions in HIV immune reconstitution and to identify biomarkers that can predict recovery of CD4+ T cell counts in INRs.
1 Introduction
With the widespread use of anti-retroviral therapy (ART), the viral load (VL) of most human immunodeficiency virus (HIV)/Acquired Immure Deficiency Syndrome (AIDS) patients has been controlled, and peripheral blood CD4+ T-lymphocyte (CD4+ T cell) counts have returned to relatively normal levels, but approximately 15-30% of ART-treated people living with HIV (PLWH) still have low CD4+ T cell counts despite adequate control of viral replication (1, 2). People who suffer from this poor immune reconstitution (PIR) are called immune non-responders (INRs) (3). Persistently low CD4+ T cell counts can not only accelerate the progression of Acquired Immune Deficiency Syndrome (AIDS), but also accompany a high mortality rate from AIDS and non-AIDS-related illnesses, causing substantial difficulties in the management of infected individuals (4) (Figure 1). There are no universal criteria for PIR. The Department of Health and Human Services (DHHS) defined that HIV/AIDS patients with CD4+ T cell count still below 350 or 500 cells/µl after 4-7 years of ART are considered to be INRs (5).
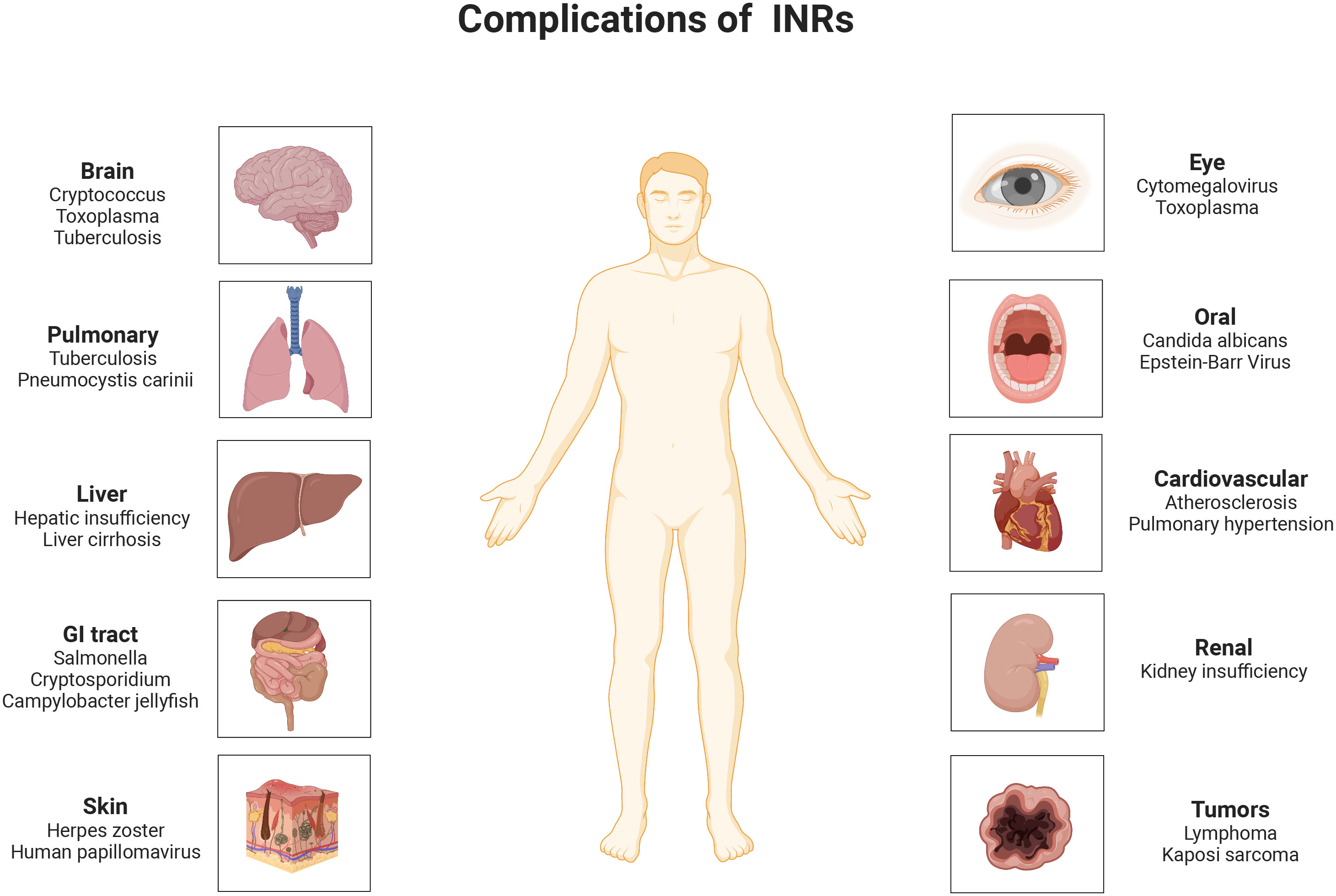
Figure 1 Opportunistic infections and diseases that are likely to complicate in INRs. INRs, immune non-responders.
Previous studies examining respiratory fungal communities in HIV/AIDS patients with lung disease have found a strong relationship between lung microbiome and immune status. As CD4 counts decreased, the species diversity of lung bacteria increased but the number declined. At the same time, the quantity and variety of fungus grew as one’s immunological condition deteriorated (6). The primary hallmark of HIV infection is the loss of CD4+ T cells. This is most evident in the gut-associated lymphoid tissue (GALT), which houses the majority of lymphocytes in the body (7). Even in the presence of ART, this failed recovery of immune ecological function is associated with an increased risk of microbial translocation, triggers of immune activation, and low-grade chronic inflammation (8, 9). In addition to the intestinal flora, its metabolites and other relevant markers regulate important host activities, such as energy metabolism, intercellular communication, and host immunity (10). Although the pathogenesis of PIR is unclear, it may involve peripheral inflammation, intestinal epithelial impairment, immune damage, and intestinal homing of aberrant immune cells (11). Host metabolic factors are associated with poor CD4+ T cell recovery in HIV. It has been found that adipose tissue may affect peripheral CD4+ T cell recovery and that excessive activation of CD4+ T cell glycolysis may lead to CD4+ T cell depletion in HIV infection, and these changes may be reflected in circulating metabolite profiles (12, 13).
Intestinal “translocation” of bacteria or other microorganisms refers to the non-physiological passage of gastrointestinal flora through the intestinal epithelial barrier and lamina propria, eventually reaching local mesenteric lymph nodes, and from there to the body circulation (14). Under normal conditions, translocated microorganisms and microbial products are phagocytosed in the lamina propria and mesenteric lymph nodes (15). However, if the host immune system is compromised, these defense mechanisms may fail, allowing bacteria to escape and survive in distant extra-intestinal sites (16). The intestinal flora and the immune system interact and influence each other. The intestinal flora is closely related to the development of T helper 17 (Th17) cells and plays a crucial part in maintaining the integrity of the intestinal mucosa and the function of the intestinal mucosal barrier (17). The intestinal flora promotes the development of intestinal B cells and protects the intestinal mucosa from exogenous pathogens (18). At the same time, intestinal flora can induce the differentiation of immunosuppressive Treg cells and down-regulate the immune response of the body (19), and can also regulate the immune function of NK cells. Most importantly, intestinal flora can promote tolerogenic differentiation of T cells and enhance the immune tolerance of the intestinal mucosa.
Due to the harmful impact of PIR and the complexity of the underlying mechanisms, it has become essential and urgent to shed light on some biomarkers that reflect immune activation and persistent low-grade inflammation in PLWH on ART (20). However, limited information is available on the relationship between circulating markers and immune recovery in ART-treated PLWH. Meanwhile, with the progression of science and technology, the utilization of body fluids as a sample source to obtain relevant disease information is a breakthrough after high-throughput sequencing technology was applied to molecular diagnosis, which has the advantages of low cost, low adverse effects, and repeatable sampling (21). Therefore, we conducted this review to outline the variations in circulating biomarkers and the link between these markers and immune recovery in INRs, which may help to understand and monitor immune recovery after ART initiation in PLWH.
2 Intestinal flora disorders in INRs
HIV infection alters the composition of the intestinal flora non-specifically and diminishes its abundance, characterized by a rise in pathogenic bacteria and a fall in beneficial bacteria, dominated by the absence of bifidobacteria, anaerobic vibrios, clostridia, and Akkermansia (7). One study found that fecal flora alpha diversity declined in PLWH and was independently associated with the patients’ immune status, and that patients with lower CD4+ T cell counts had decreased alpha diversity, suggesting that remodeling the intestinal flora may restore immune function in PLWH (22). Microbial diversity plays a crucial role in host immune homeostasis, and the introduction of ART could not fully restore the diversity of the gut microbiota (23). The metagenomic sequencing results of Yirui Xie’s team showed that the INRs and IRs could not be fully restored from the dysregulated gut microbiota in HIV infection. This was demonstrated by the relative greater abundance of Fusarium, Ruminococcus, and Aeromonas megaterium; while Escherichia coli, Algae, Bifidobacterium, Rectobacterium, and Rhodobacter were less abundant in IRs and INRs than in uninfected controls (UCs) (24). Esther Merlini et al. observed that INRs were deficient in probiotic-like lactic acid bacteria both before and after treatment, which was associated with the failure of ART to control microbial translocation of polymicrobial flora in the peripheral blood circulation, and the polymicrobial flora did not change substantially in any way as a result of treatment (25). Edda Russo et al. also detected a rise in algae and a reduction in bacteria in the feces of INRs (26). Danfeng Lu et al. reported lower levels of luminococci in INRs than in IRs and observed differences in alpha diversity between the two groups (27). Judit Villar-García et al. revealed significantly higher inflammatory markers in INRs and more Trichodermaceae and Bacillariidae families in the intestinal flora than in IRs (28). And Gabriella d’Ettorre et al. conducted 48 weeks of probiotic supplementation in INRs and noticed a decrease in both inflammatory markers and T cell activation levels. Even if viral replication is effectively suppressed, further research is needed to restore the diversity of the intestinal flora in PLWH (29). Wei Lu et al. found that Faecalibacterium prausnitzii, unclassified Subdoligranulum sp. and Coprococcus were enriched in INRs. These species were butyric acid-producing and strongly correlated with CD4+ T cell counts and T cell immune activation (30). Soo Ching Lee et al. found a significant enrichment of Clostridium perfringens in INRs and a negative correlation between Clostridium perfringens abundance and CD4+ T cell count but a positive correlation with CD4+ T cell activation and CD4 Treg cell count (31). Krystelle Nganou-Makamdop et al. found that the ratio of Serratia to other bacterial genera changed rapidly following ART. The high ratio in the first year led to inflammation and the first wave of immune reconstitution. In the second year, systemic T cell homeostasis was restored as a result of innate cytokine downregulation and a decrease in Serratia abundance (32). In summary, gut flora may be a breakthrough for HIV immune reconstitution, and detecting circulating flora metabolites and other related markers is probably the most direct way to observe it (Figure 2).
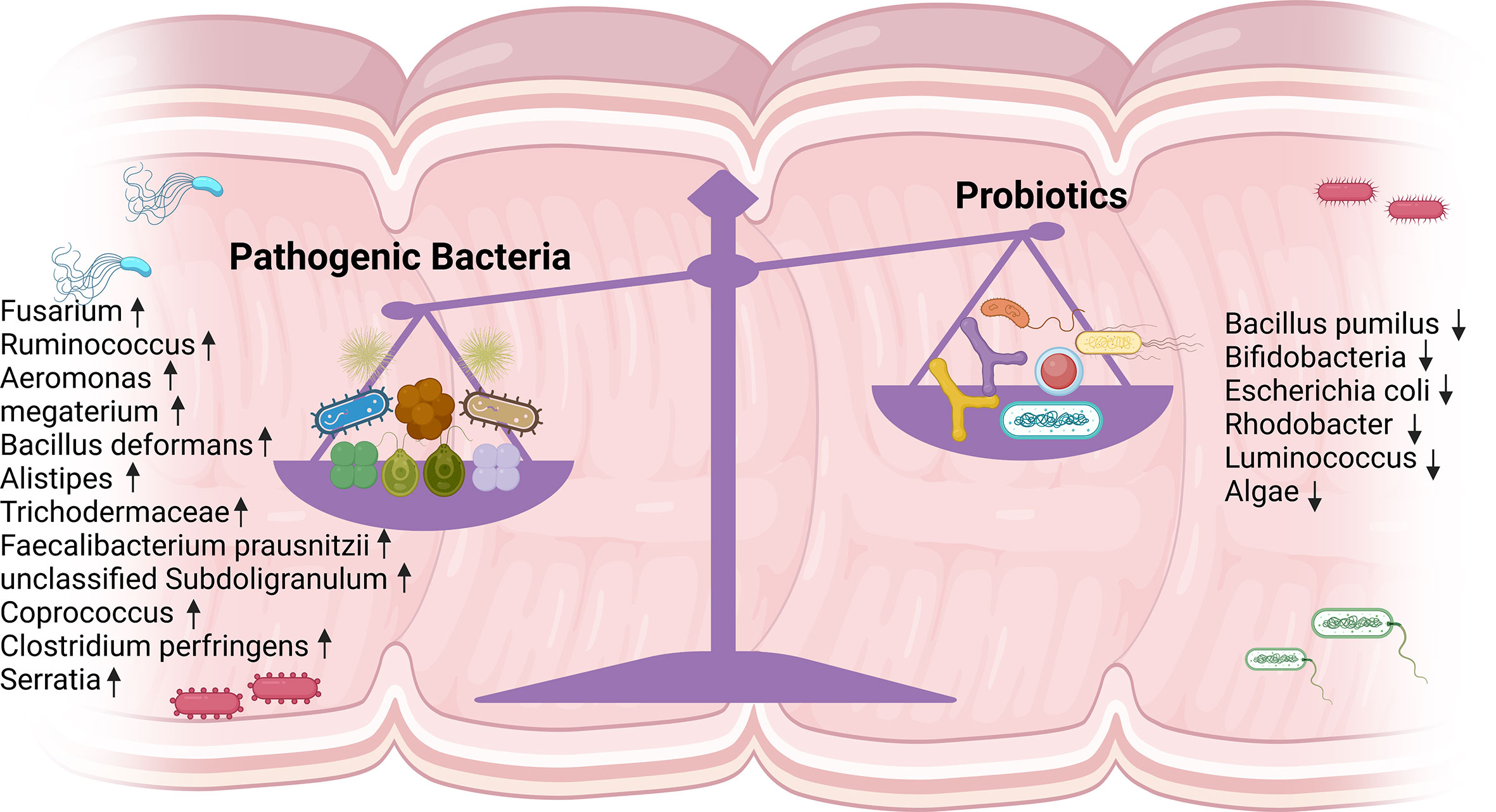
Figure 2 Altered intestinal flora in INRs. A noticeable increase in pathogenic bacteria and a significant decrease in probiotics were detected. INRs, immune non-responders.
3 Fecal markers
The intestinal microecology is a critical part of the body’s immune system, with intestinal epithelial cells, intestinal flora, and immune cells interacting with each other to maintain the balance of the intestinal microecology (33). HIV infection causes CD4+ T cell depletion, which primarily affects the intestine, severely damages the intestinal epithelium, and enables microbial translocation. Additionally, the modification can activate innate and adaptive immune responses in the blood and gut. Thus, long-term immune activation induces low-grade inflammation in the gut and systemically, resulting in enteropathy (34, 35). Immune activation, pro-inflammatory cytokines, biomarkers of gut damage, microbial translocation, and systemic inflammation are reduced after ART initiation, but remain higher than in uninfected controls (36, 37).
3.1 Short-chain fatty acids
Short-chain fatty acids (SCFA) are vital bacterial metabolites that regulate the production of immune mediators for the repair and maintenance of epithelial integrity (38). In addition, SCFA regulates T cell activity and reduces the overexpression of histone deacetylases, such as butyric and valeric acid (39). SCFA is an important connection between the immune system and the microbiome, essential for maintaining homeostasis in the gut, and ultimately plays a role in HIV infection (40). Edda Russo et al. observed variations in the composition of fecal microbiota between IRs and INRs. Fecal isobutyric acid, isovaleric acid, and 2-methylbutyric acid were more significantly increased in IRs, compared with INRs; and they were highly expressed in both groups than in uninfected controls (26). However, they did not change dramatically in the blood. Changes in the gut flora system of PLWH receiving ART may be a consequence and potential cause of systemic immune recovery.
3.2 Cytokines
HIV infection is accompanied by an increase in circulating interleukin-18 (IL-18) and a concomitant decrease of its antagonist interleukin-18 binding protein (IL-18BP). HIV infection is also coupled with intestinal inflammation and a loss of intestinal integrity, contributing to increased intestinal permeability and microbial transport. Ossama Allam et al. discovered increased IL-18, IL-1β, and IL-8 in intestinal biopsies of PLWH, which were not related to the ability to reconstitute immunity (41). Mariana Del Rocio Ruiz-Briseño et al. also observed significantly higher levels of IL-1β, IL-8, and IL-18 in the feces of all PLWH treated with ART, but no significant difference in the levels of pro-inflammatory factors in the feces of INRs and IRs (42).
3.3 Intestinal inflammation-associated protein
HIV infection is characterized by the depletion of T helper cell 17 cells (Th17 cells), influencing the development of antimicrobial peptides, mucosal regeneration, and neutrophil recruitment. Two key enzymes secreted by neutrophils are fecal calprotectin and lactoferrin, both of which are non-invasive indicators of intestinal inflammation and associated with a variety of gastrointestinal inflammatory diseases (43–45). Mariana Del Rocio Ruiz-Briseño et al. showed that calprotectin and lactoferrin levels were both increased in PLWH and that 17% of the INRs and 5.6% of the IRs exceeded the threshold for lactoferrin associated with intestinal inflammation (5.6 µ g/g in feces). Moreover, 50% of INRs showed elevated lactoferrin and calcineurin concentrations, and low CD4+ T cell counts were associated with fecal calcineurin levels (42). When fecal calprotectin levels in HIV-positive children receiving ART were evaluated in another study from Uganda, these kids had excessive quantities of the protein, which was correlated with the development of the illness (46). Changes in gut homeostasis may manifest as activation of the innate immune system and systemic T cells, both of which may contribute to chronic inflammation. Lack of immune rebuilding may be associated with persistent immune activation and inflammation, particularly in the intestinal environment.
4 Plasma markers
The destruction of the intestinal mucosa in PLWH causes translocation of the flora and the continuous entry of inflammatory mediators into circulation, creating a chronic immune activation (47). The persistence of low virus levels in their viral reservoirs, such as plasma and monocytes, despite virological suppression with ART, may have contributed to the ongoing immune activation. Such low levels of viremia are more prevalent in patients with PIR (48). Sustained immune activation may impair the initial T cell pool and lead to CD4+ T cell depletion (49). Immune activation is higher in INRs and increased T cell activation is predictive of disease progression in HIV infection (50–53). Biomarkers, such as immune activation, inflammation, and flora displacement in serum, are among the main features of HIV infection and disease progression. Serum samples are easily accessible, easy to store and monitor, and more acceptable to patients (54, 55).
4.1 Soluble immune mediators
4.1.1 Soluble CD14
Soluble CD14 (sCD14), a ligand for bacterial Lipopolysaccharide (LPS) (56), is detached with the activation of monocytes and macrophages. sCD14 is considered a soluble marker of microbial translocation, inflammation, and innate immune activation, and is associated with all-cause mortality in HIV infection (57, 58). PLWH have been found to have higher levels of sCD14 than UCs (59, 60). A multinational prospective study detected systemic inflammation in African HIV patients with controlled viral loads, and found that plasma sCD14 and C-reactive protein (CRP) concentrations were negatively correlated with subsequent recovery of CD4+ T cell counts during ART (61). Gema Méndez-Lagares et al. also revealed that ART did not normalize sCD14 levels in PLWH. Instead, compared to UCs, PLWH had significantly higher plasma sCD14 levels before ART initiation, one year after ART initiation, and five years after ART initiation. In addition, baseline CD4+ T cell counts were negatively correlated with baseline sCD14 levels (62). Also, Richard M Dunham et al. discovered that plasma sCD14 levels were significantly higher in INRs than in UCs, but the difference between INRs and IRs was insignificant. And sCD14 levels were negatively correlated with peripheral CD4+ T cell levels, a relationship that appeared to be more robust when considering INRs alone (63). Because monocytes/macrophages of neonatal or cord blood origin are highly replicative and more infectious for HIV, the degree of monocyte/macrophage activation and resulting inflammation in perinatally infected children may differ from that in infected adults (64, 65). In HIV-infected children, LPS and sCD14 levels were also increased and persisted even after viral control, and lymphocyte activation was improved by ART (66).
In summary, systemic inflammation and monocyte/macrophage activation were associated with limited CD4+ T cell recovery during ART. Long-term innate immune activation may contribute to an HIV-associated inflammatory state, and reduced inflammation may improve CD4+ T cell recovery during ART (Table 1).
4.1.2 Soluble CD163
Soluble CD163 (sCD163), a soluble molecule linked to inflammation and monocyte/macrophage activation, is another hallmark of innate immunity. Following inflammatory stimulation, sCD163 can be detached from macrophages by proteolytic cleavage of sheddase ADAM-17 and is therefore considered a marker of inflammation (70). A multicenter cohort study reported that levels of inflammatory and immune activation biomarkers, including sCD163, declined following ART initiation (71). Some studies have also reported no elevated sCD163 levels in PLWH after ART (72, 73). Stefanie Kroeze et al. have identified in a multinational African cohort of late HIV that monocyte/macrophage activation (sCD163, sCD14), inflammation (C-X-C motif chemokine 10 (CXCL10) and CRP), and microbial translocation (lipopolysaccharide-binding protein (LBP)) were persistently elevated (74), which raised the possibility of inadequate CD4+ T cell recovery and virus relapse (61). Rebeccah A McKibben et al. found elevated levels of sCD163, sCD14, and C-C Motif Chemokine Ligand 2 (CCL2) in PLWH receiving treatment and that these metrics were associated with atherosclerosis. This led to the conclusion that monocyte/macrophage activation may increase the risk of cardiovascular disease in PLWH (71).
The studies above have focused on comparing HIV-suppressed patients after ART with UCs, and few studies have focused on plasma sCD163 in INRs. Xiaopeng Dai et al. found that plasma sCD163 levels were shown to be higher in INRs compared with UCs, and that ART boosted sCD163 levels in IRs (68). Additionally, the percentage of platelet-CD4+ T cell aggregates was positively linked with the expression of sCD14 and sCD163, which were connected to an enhanced risk of cardiovascular disease (CVD) and can function as indicators of the evolution of HIV illness (75–77).
4.2 Fatty acid metabolites
Shi Qian et al. reported significant differences in levels of more than 30 metabolites among INRs, IRs, and UCs using untargeted metabolomics, with lipids accounting for the majority. Compared with UCs, PLWH had reduced levels of certain sterols, while INRs had higher levels of fatty acids and acyl carotenoids than UCs and IRs. Of these, acylcarnitine was the primary differential metabolite. Myristyl carnitine (MC), palmitoylcarnitine (PC), stearoylcarnitine (SC), and oleoylcarnitine (OC) were significantly elevated in the plasma of INRs, and their expressions were inversely connected to CD4+ T cell counts (78).
4.3 Amino acid metabolites
4.3.1 Tryptophan
Tryptophan is an essential amino acid metabolized predominantly via the kynurenine pathway and indoleamine 2,3-dioxygenase 1 (IDO1) is the rate-limiting enzyme for its catabolism (79). The ratio of plasma concentrations of kynurenine to tryptophan (KTR) was used as an indirect measure of IDO activity (80). Julie C. Gaardbo et al. found that PLWHs retaining high KTR exhibited a lower percentage of naïve T cells and preserved an unfavorable distribution of CD4 and CD8 cells, which was consistent with impaired immune reconstitution. In addition, KTR was positively correlated with immune activation, senescence, and apoptosis (81). An in vitro study by Peter Terness et al. also showed that downstream products of tryptophan catabolism have toxic effects on T cell responses, inhibiting T cell proliferation and inducing death (82).
Indole-3-propionic acid (IPA), a tryptophan deamidation product produced by the intestinal microbiota, has anti-inflammatory effects and is down-regulated during HIV infection. IPA is vital for intestinal barrier integrity and immune cell functionality, and reflects impaired homeostasis within the gastrointestinal epithelium (83). Furthermore, reduced plasma IPA levels were associated with reduced gut microbial diversity (84). Therefore, the decrease in circulating IPA in PLWH after ART may be a result of changes in gut microbial activity, dysbiosis, and ongoing low inflammation (85–87). Sofia Nyström et al. found IPA to be one of the most significant metabolites that distinguished PLWH from UCs. Plasma expression of IPA and tryptophan was lower in PLWH than in UCs (88).
4.3.2 Cysteine
Cysteine supplies the redox-active sulfhydryl radicals of glutathione, one of the most significant natural antioxidants (89), and oxidative stress has previously been seen in PLWH (90, 91). Cysteine is necessary for T cell growth, which produces reactive oxygen species (ROS) (92). Thomas R. Ziegler et al. found no differences in amino acid concentrations in plasma of INRs and IRs, but PLWH had significantly lower concentrations of total, essential, branched and sulphur amino acids, and 12 individual amino acids than UCs (93). In contrast, Sofia Nyström et al. found that plasma cysteine levels were higher in HIV patients with rapid immune recovery than those with slow immune recovery. Cysteine levels were also elevated in the HIV rapid immune recovery group, compared to UCs. The rapid immune recovery group also had higher concentrations of several metabolic pathways, including homocysteine degradation, cysteine metabolism, and taurine and hypotaurine etabolism, and there was a significant connection between cysteine levels and CD4+ T cell count (88). In short, elevated plasma cysteine levels may contribute to the maintenance of redox homeostasis and rapid recovery of CD4+ T cell function, and facilitate effective immune reconstitution after ART.
4.3.3 Glycine and serine
ROS levels impact cellular status, with low levels contributing to cell proliferation and high levels leading to cellular senescence or death (94, 95). When cellular metabolism increases and ROS elevates, serine metabolism can maintain cell survival by synthesizing reductive substances to resist excess ROS (96–98). Glycine and serine metabolism is one of the most prominent changes correlated to HIV, where serine and polysaccharides can affect the proliferation and immune function of CD4+ T cells in UCs (99). Serine hydroxymethyltransferase catalyzes the conversion of serine to glycine, which in turn directly regulates methionine and serine to replenish single-carbon metabolism (100). The intricate mechanism of single-carbon metabolism is what indirectly regulates cysteine levels and is crucial for preserving cellular redox equilibrium (101). In PLWH with slow immune recovery, levels of these amino acids were reduced (88). Thus, altered serine and glycine metabolism may aid immune recovery by regulating inflammation-induced oxidative stress in PLWH.
4.4 Cytokines
4.4.1 Interleukin-6
Interleukin-6 (IL-6), a multipotent cytokine widely distributed in the human body, is involved in the growth and differentiation of a variety of cells and plays a vital role in the body’s acute phase response and immune response against infection (102). Carey L Shive et al. found that plasma IL-6 and sCD14 levels were higher in INRs than in IRs, and that IL-6 levels were positively correlated with the expressions of T cell exhaustion and senescence markers (67). Richard M Dunham et al. assessed IL-6 mRNA and protein levels in the colon. They found that although IL-6 levels were higher in IRs than in UCs and tended to be higher in INRs than in UCs, there was no statistical difference between IRs and INRs, nor was there a correlation between blood IL-6 levels and colonic IL-6 mRNA levels (63).
4.4.2 Interferon-inducible protein-10
Interferon-inducible protein-10 (IP-10), induced by type I and type II interferons, is involved in transporting immune cells to sites of inflammation and is considered a crucial pro-inflammatory factor in the course of HIV disease. In acute HIV infection, plasma IP-10 levels could predict rapid disease progression and were negatively related to CD4+ T cell counts (103). With ART intervention, IP-10 levels were reduced, but not to normal levels. And IP-10 was consistently associated with HIV disease progression (based on CD4+ T cell count) during this period, suggesting that IP-10 could be used as an indicator of HIV infection or as a target for HIV therapy (104). Carey L Shive found that IP-10 was elevated in INRs and was accompanied by a higher rate of T cell exhaustion and senescence (67).
4.4.3 Interleukin-7 and interleukin-7 receptor
Interleukin-7 (IL-7) is a cytokine that plays a crucial role in the development, maintenance, and renewal of T lymphocytes (105). Studies have reported elevated plasma circulating IL-7 levels in PLWH and its negative correlation with CD4+ T cell counts (106, 107). In addition, the IL-7 level is a reliable indicator of the virological reaction to ART in PLWH (107). Consistent with adults, IL-7 levels were significantly higher in HIV-infected children than in age-matched UCs and were negatively correlated with CD4+ T cell percentage, but not absolute CD4+ T cell count (108–110). In addition, an elevation in plasma IL-7 has also been reported in INRs, consistent with a decrease in T lymphocytes, which may be associated with temporary support of thymic output or peripheral T cell renewal (106, 111, 112). Rethi and her colleagues demonstrated that T cells isolated from PLWH and cultured in the presence of IL-7 had a survival disadvantage, compared with T cells from UCs, suggesting that reduced responsiveness to IL-7 may play a role in disease progression (113).
Marco Marziali et al. observed increased serum IL-7 and decreased naive and thymic naive CD4+ T cells in INRs, which was correlated with reduced IL-7Rα in both cell subsets. In addition, increased immune activation, reduced Treg frequency, and increased amplification of the Vβ family were also detected; suggesting that reduced IL-7Rα expression was linked to sustained immune activation and altered Treg frequency, also partially explaining the low levels of CD4+ T cell observed in INRs (114). In conclusion, alterations in the IL7/IL7Rα pathway may influence the elevation of CD4+ T cells in PLWH with sustained viral load suppression and thus function in the pathogenesis of PIR.
4.4.4 Interleukin-1α and tumor necrosis factor-α
Interleukin-1α (IL-1α), engaged in the formation of acute and chronic inflammation, can activate T, B, and natural killer (NK) cells, increase the neutrophil number and promote the expression of inflammatory cell adhesion molecules (115). TNF-α binds to two receptors, the type I TNF receptor (TNFRI) and the type II TNF receptor (TNFRII), which initiate apoptosis or necroptosis (116). Danfeng Lu et al. demonstrated that INRs had a poorer ratio of CD4/CD8, a worse nutritional condition, and greater amounts of serum cytokines, including tumor necrosis factor-α (TNF-α), interferon-gamma-inducible protein-10 (IP-10) and interleukin-1α (IL-1α), indicating increased chronic inflammation in INRs. Further, lower CD4+ T cell counts in INRs were linked to decreased intestinal flora abundance and higher serum pro-inflammatory cytokines. TNF-α and IL-1α levels were also adversely correlated with CD4+ T cell counts (27).
4.4.5 Lipopolysaccharide
Lipopolysaccharide (LPS) can trigger inflammation and immune activation by stimulating toll-like receptor 4 (TLR4) on monocytes and other naturally occurring immune cells and is used as a biomarker for microbial translocation. Soluble CD14 (sCD14) plays an essential role in signaling by transferring LPS to the TLR4 complex. Levels of LPS and sCD14 are commonly correlated with intestinal injury, microbial translocation, and inflammation (34). Mariana Del Rocio Ruiz-Briseño et al. found that LPS levels of UCs, IRs, and INRs all showed high variability, and no statistical significance was observed among the three groups (42). However, Esther Merlini et al. found similarly elevated plasma levels of LPS and its ligand sCD14 in both partial immune responders (PRs) and INRs, and this elevation was not reduced by ART (25). These results suggested that microbial translocation could not be fully controlled by ART and was associated with insufficient CD4+ T cell reconstitution.
4.4.6 Hypersensitive C-reactive protein
Hypersensitive C-reactive protein (hsCRP) is an inflammatory biomarker that is widely used in a variety of diseases and this immediate reaction raises the risk of disease progression and cardiovascular disease (95). One study found that INRs displayed significantly higher levels of hsCRP, compared with UCs. Although hsCRP is non-specific, its measurement, together with other immune activation and inflammation-related biomarkers, provides information on the status of systemic inflammation (42). In INRs, increases in hsCRP and sCD14 suggest that low-grade systemic inflammation continues despite virological control, which may affect the body’s ability to restore CD4+ T cells. Another study found that 17% of IRs and 33% INRs had hsCRP levels above 3 mg/dL; greater risk of death, cardiovascular disease, and the emergence of opportunistic infections were connected to this threshold (117).
4.4.7 Transforming growth factor-β
Transforming growth factor-β (TGF-β) is an essential anti-inflammatory cytokine that promotes the development of regulatory T cells (118). Carey L. Shive et al. found that plasma TGF-β levels in INRs were low and the proportion of circulating CD4+ regulatory T cells was reduced, which may make it challenging to control inflammation. In addition, T cell exhaustion and senescence phenotypes were negatively correlated with TGF-β expression and positively correlated with plasma IP-10 and IL-6 levels (67). Thus, there may be a mechanistic link between low TGF-β levels, reduced CD4+ T cell recovery, impaired Treg function, and dysregulated T cell phenotype.
4.4.8 Vascular-cell adhesion molecule
Vascular-Cell Adhesion Molecule (VCAM) is not expressed in the resting state, but mainly on the surface of cytokine-activated vascular endothelial cells, mediating the adhesion of lymphocytes and leukocytes to vascular endothelial cells. Under pathological conditions like inflammation, the number and function of VCAM can be significantly upregulated and involved in tissue damage (119). Rodney K Rousseau et al. discovered that soluble VCAM was the only plasma marker elevated in INRs, in comparison with complete responders (CRs). All other plasma biomarkers, including TNF, sCD14, CRP, intercellular cell adhesion molecule (ICAM), monocyte chemotactic protein-1 (MCP-1), gamma-interferon (IFNγ), I-FABP, D-dimer, IL-8, IL-6, and Kyn/Trp, were not significantly different between INRs and CRs (120).
4.4.9 Others
Regarding intestinal permeability, Mariana Del Rocio Ruiz-Briseño et al. measured serum intestinal fatty acid binding protein (I-FABP) and soluble growth stimulated expression gene 2 protein (sST2) levels. Although no significant differences were detected, both I-FABP and sST2 were elevated in IRs and INRs, which may reflect intestinal injury that exacerbated the inflammatory phenotype in PLWH (42). Soluble TNF receptors (sTNF-RI and sTNF-RII, detached upon TNF binding) were measured in plasma as biomarkers of TNF activity. sTNF-RI and sTNF-RII levels did not differ between IRs and INRs. Levels of sTNF-RII were higher in INRs than in UCs, and were more comparable to those observed in viremic subjects (121).
4.5 Fibrosis markers
In HIV early infection, lymphoid tissue fibrosis can cause CD4+ T cell depletion and immune system dysfunction, and this process may not fully reverse even if ART is started (122, 123). Hyaluronic acid (HA), a component of the extracellular matrix generated during wound healing, is present in elevated concentrations in fibrotic diseases. C-X-C Motif Chemokine Ligand 4 (CXCL4) concentrations rise in response to pro-fibrotic stimuli, and decreased CXCL4 levels in PLWH may imply that HIV effectively evades immunity (124–127). Pre-ART HA and CXCL4 concentrations were found not to vary according to final immune reconstitution status. In INRs, HA concentrations were higher and CXCL4 concentrations were lower after treatment. In paired pre/post-treatment samples, there was a tremendous increase in HA and a more significant decrease in CXCL4 in INRs, compared with UCs. It is hypothesized that increased circulating HA and lower circulating CXCL4 concentrations due to lymphoid tissue fibrosis were associated with PIR (128).
4.6 MicroRNAs
Small endogenous RNAs called microRNAs (miRNAs) control post-transcriptional gene expression. Studies have revealed that due to their chemical stability and anti-RNase action, endogenous circulating miRNAs are reliable blood biomarkers (129–131). Circulating miRNAs in serum may be used as indicators of early HIV infection (132), HIV disease progression (133–135), HIV-associated neurological disorders (136–138), and HIV-related liver injury (139, 140). Yuping Fu et al. recognized that numerous miRNAs were found to be downregulated in INRs, compared with IRs; and the miRNA let-7d-5p was identified as a possible biomarker for INRs (141). In contrast, Junnan Lv et al. found that the expressions of five miRNAs (miR-580, miR-627, miR-138-5p, miR-16-5p, and miR-323-3p) were upregulated in the plasma of INRs, compared with IRs. One year after ART, the expressions of these miRNAs were negatively correlated with the increase in CD4+ T cell count and percentage. These five miRNAs were utilized to create a predictive algorithm that could successfully and precisely distinguish INRs from IRs to predict PIR following ART. Furthermore, miR-16-5p might prevent the growth of CD4+ T cells by regulating calcium flow (142). These prognostic miRNA biomarkers may enhance the early detection of PIR.
4.7 Others
Gut bacterial-derived solutes (GBDSs), such as p-cresol sulfate (PCS) and indoxyl sulfate (IS), could impair mitochondrial fitness and thus result in an INR-specific reduction in CD4+ T cells; and fecal samples of INRs were enriched with bacterial genera capable of producing PCS (23). Ghneim K et al. found that senescent INRs were associated with higher HIV persistence and were driven by the plasma metabolome and microbes with a rich butyrate/bile acid (BA) (143). BA tolerance is an essential property of intestinal colonizing bacteria (144), and the interactions between BA metabolism and the intestinal microbiota are complex. Accumulating toxic bile acids may lead to inflammation (145), and targeting the BA-microbiota axis may be a potential way to improve HIV immune function (146).
5 Limitations of this review
This review also has some limitations. First, there are few studies related to circulating markers in INRs, especially in feces. In addition, the accuracy and potential value of these biomarkers in evaluating CD4+ T cell recovery in INRs need to be validated in larger sample cohorts. Finally, due to the difference in sample size, the disparity of diagnostic criteria, and the methodological heterogeneity of the included studies, we did not assess the quality and risk of bias of the included studies as a whole, nor did we perform a meta-analysis of individual biomarkers in this review.
6 Conclusions and perspectives
ART increases CD4+ T cell counts and partially rebuilds the immune system in PLWH, but still fails to resolve microbial translocation or monocyte/macrophage activation, thereby increasing long-term morbidity and mortality in non-AIDS-associated inflammatory conditions (Figure 3). Furthermore, we revealed that the systematic study of diverse circulating markers might deepen the understanding of the immunopathogenesis of HIV and provide new tactics for clinical treatment (Table 2). Considering the vital role of circulating markers in immune programming and regulation, future studies on changes of gut flora and circulating biomarkers in INRs should be conducted in more detail to elucidate the regulatory mechanisms of the circulating microenvironment on CD4+ T cell reconstitution. Overall, this review offers a new perspective on the impact of HIV infection, ART, and the microbiota immune axis on immune recovery at the metabolic level.
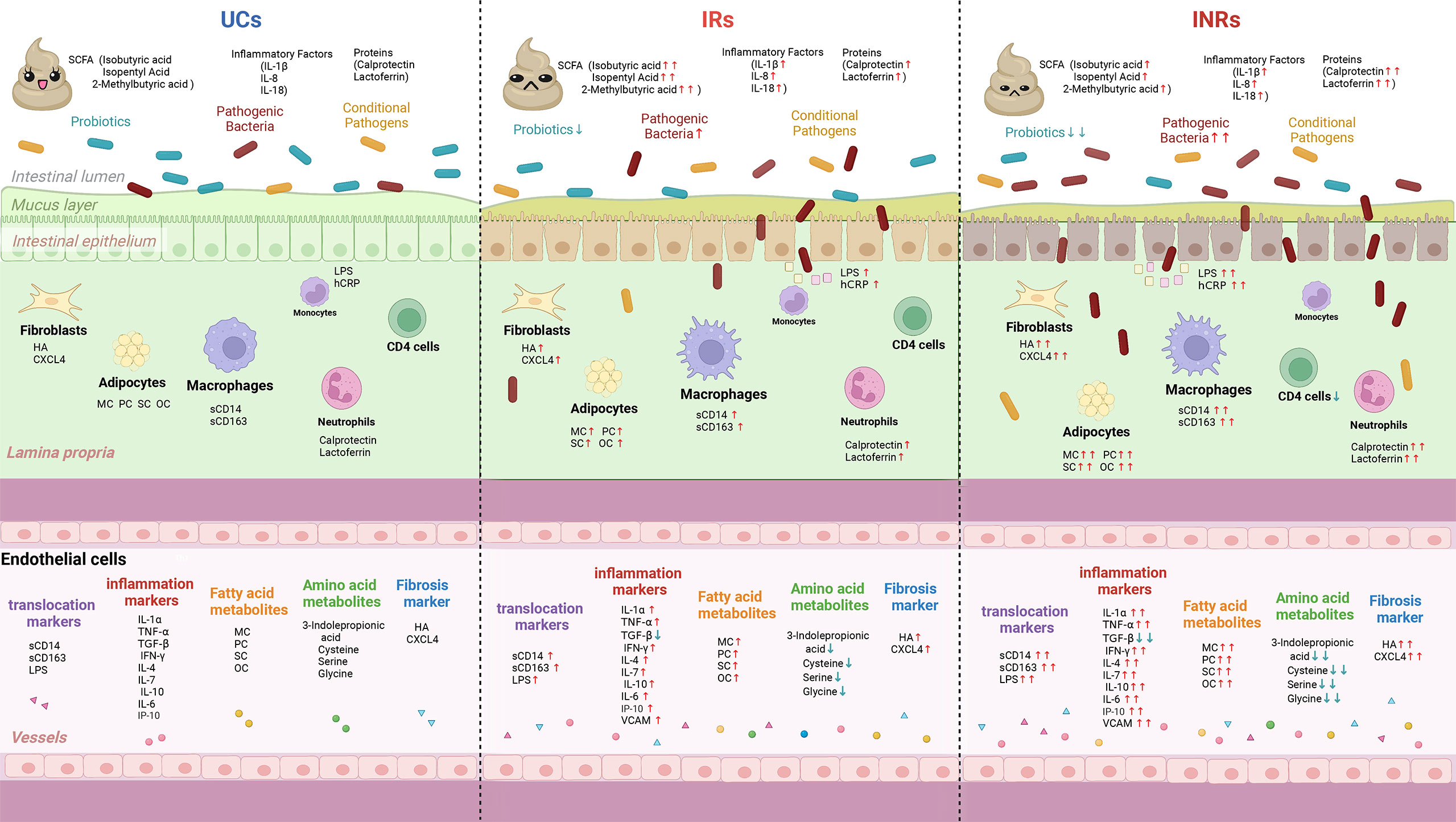
Figure 3 Schematic summary of microbial translocation, immune cells involved, and altered circulating markers in UCs, IRs, and INRs. UCs, uninfected controls; IRs, immune responders; INRs, immune non-responders; SCFA, short chain fatty acids; sCD14, soluble CD14; sCD163, soluble CD163; IL-6, interleukin-6; IL-7, interleukin-7; IL-7R, interleukin-7 receptor; IL-1α, interleukin-1α; IL-1β, interleukin-1β; IL-8, interleukin-8; IL-18, interleukin-18; IP-10, interferon-inducible protein 10; LPS, lipopolysaccharide; hsCRP, hypersensitive C-reactive protein; TGF-β, transforming growth factor-β; VCAM, vascular adhesion molecules; HA, hyaluronic acid; CXCL4, C-X-C motif chemokine ligand 4; MC, myristyl carnitine; PC, palmitoylcarnitine; SC, stearoylcarnitine; OC, oleoylcarnitine; ↑, increase in comparison with uninfected controls; ↓, decrease in comparison with uninfected controls.
Author contributions
All authors made intellectual contributions to this work. QX and FZ had the idea for this review, QX wrote the article, and FY, LY, HZ, and FZ supervised the manuscript. All authors contributed to the article and approved the submitted version.
Funding
This work was supported by the 13th Five-year Plan, Ministry of Science and Technology of China (2018ZX10302-102), Beijing Municipal Administration of Hospitals’ Ascent Plan (DFL20191802), and Beijing Municipal Administration of Hospitals Clinical Medicine Development of Special Funding Support (ZYLX202126). The funders had no role in study design, data collection, analysis, publication decisions, or manuscript preparation.
Acknowledgments
Thanks to all authors for their contribution to this manuscript.
Conflict of interest
The authors declare that the research was conducted in the absence of any commercial or financial relationships that could be construed as a potential conflict of interest.
Publisher’s note
All claims expressed in this article are solely those of the authors and do not necessarily represent those of their affiliated organizations, or those of the publisher, the editors and the reviewers. Any product that may be evaluated in this article, or claim that may be made by its manufacturer, is not guaranteed or endorsed by the publisher.
References
1. Ghaffari G, Passalacqua DJ, Caicedo JL, Goodenow MM, Sleasman JW. Two-year clinical and immune outcomes in human immunodeficiency virus-infected children who reconstitute CD4 T cells without control of viral replication after combination antiretroviral therapy. Pediatrics (2004) 114:e604-611. doi: 10.1542/peds.2004-0274
2. Deeks SG. Immune dysfunction, inflammation, and accelerated aging in patients on antiretroviral therapy. Top HIV Med (2009) 17:118–23.
3. Yang X, Su B, Zhang X, Liu Y, Wu H, Zhang T. Incomplete immune reconstitution in HIV/AIDS patients on antiretroviral therapy: Challenges of immunological non-responders. J Leukoc Biol (2020) 107:597–612. doi: 10.1002/jlb.4mr1019-189r
4. Gaardbo JC, Hartling HJ, Gerstoft J, Nielsen SD. Incomplete immune recovery in HIV infection: mechanisms, relevance for clinical care, and possible solutions. Clin Dev Immunol (2012) 2012:670957. doi: 10.1155/2012/670957
5. Siberry GK, Abzug MJ, Nachman S. Executive summary: 2013 update of the guidelines for the prevention and treatment of opportunistic infections in HIV-exposed and HIV-infected children. Pediatr Infect Dis J (2013) 32:1303–7. doi: 10.1097/inf.0000000000000080
6. Chen Z, Tian Y, Wang Y, Zhao H, Chen C, Zhang F. Profile of the lower respiratory tract microbiome in human immunodeficiency Virus/Acquired immunodeficiency syndrome and lung disease. Front Microbiol (2022) 13:888996. doi: 10.3389/fmicb.2022.888996
7. Rocafort M, Noguera-Julian M, Rivera J, Pastor L, Guillén Y, Langhorst J, et al. Evolution of the gut microbiome following acute HIV-1 infection. Microbiome (2019) 7:73. doi: 10.1186/s40168-019-0687-5
8. Hsue PY, Waters DD. HIV Infection and coronary heart disease: mechanisms and management. Nat Rev Cardiol (2019) 16:745–59. doi: 10.1038/s41569-019-0219-9
9. Feinstein MJ, Hsue PY, Benjamin LA, Bloomfield GS, Currier JS, Freiberg MS, et al. Characteristics, prevention, and management of cardiovascular disease in people living with HIV: A scientific statement from the American heart association. Circulation (2019) 140:e98–e124. doi: 10.1161/cir.0000000000000695
10. He B, Liu Y, Hoang TK, Tian X, Taylor CM, Luo M, et al. Antibiotic-modulated microbiome suppresses lethal inflammation and prolongs lifespan in treg-deficient mice. Microbiome (2019) 7:145. doi: 10.1186/s40168-019-0751-1
11. Doitsh G, Greene WC. Dissecting how CD4 T cells are lost during HIV infection. Cell Host Microbe (2016) 19:280–91. doi: 10.1016/j.chom.2016.02.012
12. Palmer CS, Ostrowski M, Gouillou M, Tsai L, Yu D, Zhou J, et al. Increased glucose metabolic activity is associated with CD4+ T-cell activation and depletion during chronic HIV infection. Aids (2014) 28:297–309. doi: 10.1097/qad.0000000000000128
13. Cenderello G, De Maria A. Discordant responses to cART in HIV-1 patients in the era of high potency antiretroviral drugs: clinical evaluation, classification, management prospects. Expert Rev Anti Infect Ther (2016) 14:29–40. doi: 10.1586/14787210.2016.1106937
14. Braun V, Helbig S, Patzer SI, Pramanik A, Römer C. Import and export of bacterial protein toxins. Int J Med Microbiol (2015) 305:238–42. doi: 10.1016/j.ijmm.2014.12.006
15. Berg RD. Bacterial translocation from the gastrointestinal tract. Trends Microbiol (1995) 3:149–54. doi: 10.1016/s0966-842x(00)88906-4
16. Alexander JW, Gianotti L, Pyles T, Carey MA, Babcock GF. Distribution and survival of escherichia coli translocating from the intestine after thermal injury. Ann Surg (1991) 213:558–66. doi: 10.1097/00000658-199106000-00005
17. Planas D, Routy JP, Ancuta P. New Th17-specific therapeutic strategies for HIV remission. Curr Opin HIV AIDS (2019) 14:85–92. doi: 10.1097/coh.0000000000000522
18. Strugnell RA, Wijburg OL. The role of secretory antibodies in infection immunity. Nat Rev Microbiol (2010) 8:656–67. doi: 10.1038/nrmicro2384
19. Russler-Germain EV, Rengarajan S, Hsieh CS. Antigen-specific regulatory T-cell responses to intestinal microbiota. Mucosal Immunol (2017) 10:1375–86. doi: 10.1038/mi.2017.65
20. Abdel-Mohsen M, Richman D, Siliciano RF, Nussenzweig MC, Howell BJ, Martinez-Picado J, et al. Recommendations for measuring HIV reservoir size in cure-directed clinical trials. Nat Med (2020) 26:1339–50. doi: 10.1038/s41591-020-1022-1
22. Nowak P, Troseid M, Avershina E, Barqasho B, Neogi U, Holm K, et al. Gut microbiota diversity predicts immune status in HIV-1 infection. Aids (2015) 29:2409–18. doi: 10.1097/qad.0000000000000869
23. Ferrari B, Da Silva AC, Liu KH, Saidakova EV, Korolevskaya LB, Shmagel KV, et al. Gut-derived bacterial toxins impair memory CD4+ T cell mitochondrial function in HIV-1 infection. J Clin Invest (2022) 132(9):e149571. doi: 10.1172/jci149571
24. Xie Y, Sun J, Wei L, Jiang H, Hu C, Yang J, et al. Altered gut microbiota correlate with different immune responses to HAART in HIV-infected individuals. BMC Microbiol (2021) 21:11. doi: 10.1186/s12866-020-02074-1
25. Merlini E, Bai F, Bellistrì GM, Tincati C, d'Arminio Monforte A, Marchetti G. Evidence for polymicrobic flora translocating in peripheral blood of HIV-infected patients with poor immune response to antiretroviral therapy. PloS One (2011) 6:e18580. doi: 10.1371/journal.pone.0018580
26. Russo E, Nannini G, Sterrantino G, Kiros ST, Di Pilato V, Coppi M, et al. Effects of viremia and CD4 recovery on gut "microbiome-immunity" axis in treatment-naïve HIV-1-infected patients undergoing antiretroviral therapy. World J Gastroenterol (2022) 28:635–52. doi: 10.3748/wjg.v28.i6.635
27. Lu D, Zhang JB, Wang YX, Geng ST, Zhang Z, Xu Y, et al. Association between CD4(+) T cell counts and gut microbiota and serum cytokines levels in HIV-infected immunological non-responders. BMC Infect Dis (2021) 21:742. doi: 10.1186/s12879-021-06491-z
28. Villar-García J, Güerri-Fernández R, Moya A, González A, Hernández JJ, Lerma E, et al. Impact of probiotic saccharomyces boulardii on the gut microbiome composition in HIV-treated patients: A double-blind, randomised, placebo-controlled trial. PloS One (2017) 12:e0173802. doi: 10.1371/journal.pone.0173802
29. d'Ettorre G, Rossi G, Scagnolari C, Andreotti M, Giustini N, Serafino S, et al. Probiotic supplementation promotes a reduction in T-cell activation, an increase in Th17 frequencies, and a recovery of intestinal epithelium integrity and mitochondrial morphology in ART-treated HIV-1-positive patients. Immun Inflamm Dis (2017) 5:244–60. doi: 10.1002/iid3.160
30. Lu W, Feng Y, Jing F, Han Y, Lyu N, Liu F, et al. Association between gut microbiota and CD4 recovery in HIV-1 infected patients. Front Microbiol (2018) 9:1451. doi: 10.3389/fmicb.2018.01451
31. Lee SC, Chua LL, Yap SH, Khang TF, Leng CY, Raja Azwa RI, et al. Enrichment of gut-derived fusobacterium is associated with suboptimal immune recovery in HIV-infected individuals. Sci Rep (2018) 8:14277. doi: 10.1038/s41598-018-32585-x
32. Nganou-Makamdop K, Talla A, Sharma AA, Darko S, Ransier A, Laboune F, et al. Translocated microbiome composition determines immunological outcome in treated HIV infection. Cell (2021) 184:3899–3914.e3816. doi: 10.1016/j.cell.2021.05.023
33. Scott NA, Andrusaite A, Andersen P, Lawson M, Alcon-Giner C, Leclaire C, et al. Antibiotics induce sustained dysregulation of intestinal T cell immunity by perturbing macrophage homeostasis. Sci Transl Med (2018) 10(464):eaao4755. doi: 10.1126/scitranslmed.aao4755
34. Pastor L, Langhorst J, Schröder D, Casellas A, Ruffer A, Carrillo J, et al. Different pattern of stool and plasma gastrointestinal damage biomarkers during primary and chronic HIV infection. PloS One (2019) 14:e0218000. doi: 10.1371/journal.pone.0218000
35. Yaseen MM, Abuharfeil NM, Yaseen MM, Shabsoug BM. The role of polymorphonuclear neutrophils during HIV-1 infection. Arch Virol (2018) 163:1–21. doi: 10.1007/s00705-017-3569-9
36. Wilson EM, Sereti I. Immune restoration after antiretroviral therapy: the pitfalls of hasty or incomplete repairs. Immunol Rev (2013) 254:343–54. doi: 10.1111/imr.12064
37. Mait-Kaufman J, Fakioglu E, Mesquita PM, Elliott J, Lo Y, Madan RP. Chronic HIV infection is associated with upregulation of proinflammatory cytokine and chemokine and alpha defensin gene expression in colorectal mucosa. AIDS Res Hum Retroviruses (2015) 31:615–22. doi: 10.1089/aid.2014.0085
38. Kelly CJ, Zheng L, Campbell EL, Saeedi B, Scholz CC, Bayless AJ, et al. Crosstalk between microbiota-derived short-chain fatty acids and intestinal epithelial HIF augments tissue barrier function. Cell Host Microbe (2015) 17:662–71. doi: 10.1016/j.chom.2015.03.005
39. Lee J, d'Aigle J, Atadja L, Quaicoe V, Honarpisheh P, Ganesh BP, et al. Gut microbiota-derived short-chain fatty acids promote poststroke recovery in aged mice. Circ Res (2020) 127:453–65. doi: 10.1161/circresaha.119.316448
40. Parada Venegas D, de la Fuente MK, Landskron G, González MJ, Quera R, Dijkstra G, et al. Short chain fatty acids (SCFAs)-mediated gut epithelial and immune regulation and its relevance for inflammatory bowel diseases. Front Immunol (2019) 10:277. doi: 10.3389/fimmu.2019.00277
41. Allam O, Samarani S, Mehraj V, Jenabian MA, Tremblay C, Routy JP, et al. HIV Induces production of IL-18 from intestinal epithelial cells that increases intestinal permeability and microbial translocation. PloS One (2018) 13:e0194185. doi: 10.1371/journal.pone.0194185
42. Ruiz-Briseño MDR, De Arcos-Jiménez JC, Ratkovich-González S, Sánchez-Reyes K, González-Hernández LA, Andrade-Villanueva JF, et al. Association of intestinal and systemic inflammatory biomarkers with immune reconstitution in HIV+ patients on ART. J Inflamm (Lond) (2020) 17:32. doi: 10.1186/s12950-020-00262-4
43. Däbritz J, Musci J, Foell D. Diagnostic utility of faecal biomarkers in patients with irritable bowel syndrome. World J Gastroenterol (2014) 20:363–75. doi: 10.3748/wjg.v20.i2.363
44. Lopes S, Andrade P, Rodrigues-Pinto E, Afonso J, Macedo G, Magro F. Fecal marker levels as predictors of need for endoscopic balloon dilation in crohn's disease patients with anastomotic strictures. World J Gastroenterol (2017) 23:6482–90. doi: 10.3748/wjg.v23.i35.6482
45. Mumolo MG, Bertani L, Ceccarelli L, Laino G, Di Fluri G, Albano E, et al. From bench to bedside: Fecal calprotectin in inflammatory bowel diseases clinical setting. World J Gastroenterol (2018) 24:3681–94. doi: 10.3748/wjg.v24.i33.3681
46. Hestvik E, Olafsdottir E, Tylleskar T, Aksnes L, Kaddu-Mulindwa D, Ndeezi G, et al. Faecal calprotectin in HIV-infected, HAART-naïve Ugandan children. J Pediatr Gastroenterol Nutr (2012) 54:785–90. doi: 10.1097/MPG.0b013e318241a683
47. Cassol E, Malfeld S, Mahasha P, van der Merwe S, Cassol S, Seebregts C, et al. Persistent microbial translocation and immune activation in HIV-1-infected south africans receiving combination antiretroviral therapy. J Infect Dis (2010) 202:723–33. doi: 10.1086/655229
48. Mavigner M, Delobel P, Cazabat M, Dubois M, L'Faqihi-Olive FE, Raymond S, et al. HIV-1 residual viremia correlates with persistent T-cell activation in poor immunological responders to combination antiretroviral therapy. PloS One (2009) 4:e7658. doi: 10.1371/journal.pone.0007658
49. Hazenberg MD, Otto SA, van Benthem BH, Roos MT, Coutinho RA, Lange JM, et al. Persistent immune activation in HIV-1 infection is associated with progression to AIDS. Aids (2003) 17:1881–8. doi: 10.1097/00002030-200309050-00006
50. Nakanjako D, Ssewanyana I, Mayanja-Kizza H, Kiragga A, Colebunders R, Manabe YC, et al. High T-cell immune activation and immune exhaustion among individuals with suboptimal CD4 recovery after 4 years of antiretroviral therapy in an African cohort. BMC Infect Dis (2011) 11:43. doi: 10.1186/1471-2334-11-43
51. Massanella M, Negredo E, Pérez-Alvarez N, Puig J, Ruiz-Hernández R, Bofill M, et al. CD4 T-cell hyperactivation and susceptibility to cell death determine poor CD4 T-cell recovery during suppressive HAART. Aids (2010) 24:959–68. doi: 10.1097/QAD.0b013e328337b957
52. Hunt PW, Brenchley J, Sinclair E, McCune JM, Roland M, Page-Shafer K, et al. Relationship between T cell activation and CD4+ T cell count in HIV-seropositive individuals with undetectable plasma HIV RNA levels in the absence of therapy. J Infect Dis (2008) 197:126–33. doi: 10.1086/524143
53. Hazenberg MD, Hamann D, Schuitemaker H, Miedema F. T Cell depletion in HIV-1 infection: how CD4+ T cells go out of stock. Nat Immunol (2000) 1:285–9. doi: 10.1038/79724
54. Jasinska AJ, Dong TS, Lagishetty V, Katzka W, Jacobs JP, Schmitt CA, et al. Shifts in microbial diversity, composition, and functionality in the gut and genital microbiome during a natural SIV infection in vervet monkeys. Microbiome (2020) 8:154. doi: 10.1186/s40168-020-00928-4
55. Hampel H, O'Bryant SE, Molinuevo JL, Zetterberg H, Masters CL, Lista S, et al. Blood-based biomarkers for Alzheimer disease: mapping the road to the clinic. Nat Rev Neurol (2018) 14:639–52. doi: 10.1038/s41582-018-0079-7
56. Gioannini TL, Weiss JP. Regulation of interactions of gram-negative bacterial endotoxins with mammalian cells. Immunol Res (2007) 39:249–60. doi: 10.1007/s12026-007-0069-0
57. Sandler NG, Wand H, Roque A, Law M, Nason MC, Nixon DE, et al. Plasma levels of soluble CD14 independently predict mortality in HIV infection. J Infect Dis (2011) 203:780–90. doi: 10.1093/infdis/jiq118
58. Romero-Sánchez M, González-Serna A, Pacheco YM, Ferrando-Martínez S, Machmach K, García-García M, et al. Different biological significance of sCD14 and LPS in HIV-infection: importance of the immunovirology stage and association with HIV-disease progression markers. J Infect (2012) 65:431–8. doi: 10.1016/j.jinf.2012.06.008
59. Eller MA, Blom KG, Gonzalez VD, Eller LA, Naluyima P, Laeyendecker O, et al. Innate and adaptive immune responses both contribute to pathological CD4 T cell activation in HIV-1 infected ugandans. PloS One (2011) 6:e18779. doi: 10.1371/journal.pone.0018779
60. Marchetti G, Cozzi-Lepri A, Merlini E, Bellistrì GM, Castagna A, Galli M, et al. Microbial translocation predicts disease progression of HIV-infected antiretroviral-naive patients with high CD4+ cell count. Aids (2011) 25:1385–94. doi: 10.1097/QAD.0b013e3283471d10
61. Kroeze S, Rossouw TM, Steel HC, Wit FW, Kityo CM, Siwale M, et al. Plasma inflammatory biomarkers predict CD4+ T-cell recovery and viral rebound in HIV-1 infected africans on suppressive antiretroviral therapy. J Infect Dis (2021) 224:673–8. doi: 10.1093/infdis/jiaa787
62. Méndez-Lagares G, Romero-Sánchez MC, Ruiz-Mateos E, Genebat M, Ferrando-Martínez S, Muñoz-Fernández M, et al. Long-term suppressive combined antiretroviral treatment does not normalize the serum level of soluble CD14. J Infect Dis (2013) 207:1221–5. doi: 10.1093/infdis/jit025
63. Dunham RM, Vujkovic-Cvijin I, Yukl SA, Broadhurst MJ, Loke P, Albright RG, et al. Discordance between peripheral and colonic markers of inflammation during suppressive ART. J Acquir Immune Defic Syndr (2014) 65:133–41. doi: 10.1097/01.qai.0000437172.08127.0b
64. Sundaravaradan V, Saxena SK, Ramakrishnan R, Yedavalli VR, Harris DT, Ahmad N. Differential HIV-1 replication in neonatal and adult blood mononuclear cells is influenced at the level of HIV-1 gene expression. Proc Natl Acad Sci U.S.A. (2006) 103:11701–6. doi: 10.1073/pnas.0602185103
65. Sperduto AR, Bryson YJ, Chen IS. Increased susceptibility of neonatal monocyte/macrophages to HIV-1 infection. AIDS Res Hum Retroviruses (1993) 9:1277–85. doi: 10.1089/aid.1993.9.1277
66. Goulder PJ, Lewin SR, Leitman EM. Paediatric HIV infection: the potential for cure. Nat Rev Immunol (2016) 16:259–71. doi: 10.1038/nri.2016.19
67. Shive CL, Freeman ML, Younes SA, Kowal CM, Canaday DH, Rodriguez B, et al. Markers of T cell exhaustion and senescence and their relationship to plasma TGF-β levels in treated HIV+ immune non-responders. Front Immunol (2021) 12:638010. doi: 10.3389/fimmu.2021.638010
68. Dai XP, Wu FY, Cui C, Liao XJ, Jiao YM, Zhang C, et al. Increased platelet-CD4(+) T cell aggregates are correlated with HIV-1 permissiveness and CD4(+) T cell loss. Front Immunol (2021) 12:799124. doi: 10.3389/fimmu.2021.799124
69. Merlini E, Tincati C, Biasin M, Saulle I, Cazzaniga FA, d'Arminio Monforte A, et al. Stimulation of PBMC and monocyte-derived macrophages via toll-like receptor activates innate immune pathways in HIV-infected patients on virally suppressive combination antiretroviral therapy. Front Immunol (2016) 7:614. doi: 10.3389/fimmu.2016.00614
70. Hearps AC, Martin GE, Rajasuriar R, Crowe SM. Inflammatory co-morbidities in HIV+ individuals: learning lessons from healthy ageing. Curr HIV/AIDS Rep (2014) 11:20–34. doi: 10.1007/s11904-013-0190-8
71. McKibben RA, Margolick JB, Grinspoon S, Li X, Palella FJ Jr., Kingsley LA, et al. Elevated levels of monocyte activation markers are associated with subclinical atherosclerosis in men with and those without HIV infection. J Infect Dis (2015) 211:1219–28. doi: 10.1093/infdis/jiu594
72. Reid MJA, Ma Y, Golovaty I, Okello S, Sentongo R, Feng M, et al. Association of gut intestinal integrity and inflammation with insulin resistance in adults living with HIV in Uganda. AIDS Patient Care STDS (2019) 33:299–307. doi: 10.1089/apc.2019.0032
73. Siedner MJ, Zanni M, Tracy RP, Kwon DS, Tsai AC, Kakuhire B, et al. Increased systemic inflammation and gut permeability among women with treated HIV infection in rural Uganda. J Infect Dis (2018) 218:922–6. doi: 10.1093/infdis/jiy244
74. Kroeze S, Wit FW, Rossouw TM, Steel HC, Kityo CM, Siwale M, et al. Plasma biomarkers of human immunodeficiency virus-related systemic inflammation and immune activation in Sub-Saharan Africa before and during suppressive antiretroviral therapy. J Infect Dis (2019) 220:1029–33. doi: 10.1093/infdis/jiz252
75. Burdo TH, Lentz MR, Autissier P, Krishnan A, Halpern E, Letendre S, et al. Soluble CD163 made by monocyte/macrophages is a novel marker of HIV activity in early and chronic infection prior to and after anti-retroviral therapy. J Infect Dis (2011) 204:154–63. doi: 10.1093/infdis/jir214
76. Chevalier MF, Petitjean G, Dunyach-Rémy C, Didier C, Girard PM, Manea ME, et al. The Th17/Treg ratio, IL-1RA and sCD14 levels in primary HIV infection predict the T-cell activation set point in the absence of systemic microbial translocation. PloS Pathog (2013) 9:e1003453. doi: 10.1371/journal.ppat.1003453
77. Leeansyah E, Malone DF, Anthony DD, Sandberg JK. Soluble biomarkers of HIV transmission, disease progression and comorbidities. Curr Opin HIV AIDS (2013) 8:117–24. doi: 10.1097/COH.0b013e32835c7134
78. Qian S, Chen X, Wu T, Sun Y, Li X, Fu Y, et al. The accumulation of plasma acylcarnitines are associated with poor immune recovery in HIV-infected individuals. BMC Infect Dis (2021) 21:808. doi: 10.1186/s12879-021-06525-6
79. Mellor AL, Munn DH. IDO expression by dendritic cells: tolerance and tryptophan catabolism. Nat Rev Immunol (2004) 4:762–74. doi: 10.1038/nri1457
80. Favre D, Mold J, Hunt PW, Kanwar B, Loke P, Seu L, et al. Tryptophan catabolism by indoleamine 2,3-dioxygenase 1 alters the balance of TH17 to regulatory T cells in HIV disease. Sci Transl Med (2010) 2:32ra36. doi: 10.1126/scitranslmed.3000632
81. Gaardbo JC, Trøsied M, Stiksrud B, Midttun Ø., Ueland PM, Ullum H, et al. Increased tryptophan catabolism is associated with increased frequency of CD161+Tc17/MAIT cells and lower CD4+ T-cell count in HIV-1 infected patients on cART after 2 years of follow-up. J Acquir Immune Defic Syndr (2015) 70:228–35. doi: 10.1097/qai.0000000000000758
82. Terness P, Bauer TM, Röse L, Dufter C, Watzlik A, Simon H, et al. Inhibition of allogeneic T cell proliferation by indoleamine 2,3-dioxygenase-expressing dendritic cells: mediation of suppression by tryptophan metabolites. J Exp Med (2002) 196:447–57. doi: 10.1084/jem.20020052
83. Wlodarska M, Luo C, Kolde R, d'Hennezel E, Annand JW, Heim CE, et al. Indoleacrylic acid produced by commensal peptostreptococcus species suppresses inflammation. Cell Host Microbe (2017) 22:25–37.e26. doi: 10.1016/j.chom.2017.06.007
84. Wilmanski T, Rappaport N, Earls JC, Magis AT, Manor O, Lovejoy J, et al. Blood metabolome predicts gut microbiome α-diversity in humans. Nat Biotechnol (2019) 37:1217–28. doi: 10.1038/s41587-019-0233-9
85. Rajasuriar R, Booth D, Solomon A, Chua K, Spelman T, Gouillou M, et al. Biological determinants of immune reconstitution in HIV-infected patients receiving antiretroviral therapy: the role of interleukin 7 and interleukin 7 receptor α and microbial translocation. J Infect Dis (2010) 202:1254–64. doi: 10.1086/656369
86. Rajasuriar R, Wright E, Lewin SR. Impact of antiretroviral therapy (ART) timing on chronic immune activation/inflammation and end-organ damage. Curr Opin HIV AIDS (2015) 10:35–42. doi: 10.1097/coh.0000000000000118
87. Yap SH, Abdullah NK, McStea M, Takayama K, Chong ML, Crisci E, et al. HIV/Human herpesvirus co-infections: Impact on tryptophan-kynurenine pathway and immune reconstitution. PloS One (2017) 12:e0186000. doi: 10.1371/journal.pone.0186000
88. Nyström S, Govender M, Yap SH, Kamarulzaman A, Rajasuriar R, Larsson M. HIV-Infected individuals on ART with impaired immune recovery have altered plasma metabolite profiles. Open Forum Infect Dis (2021) 8:ofab288. doi: 10.1093/ofid/ofab288
89. Bansal A, Simon MC. Glutathione metabolism in cancer progression and treatment resistance. J Cell Biol (2018) 217:2291–8. doi: 10.1083/jcb.201804161
90. Buhl R, Jaffe HA, Holroyd KJ, Wells FB, Mastrangeli A, Saltini C, et al. Systemic glutathione deficiency in symptom-free HIV-seropositive individuals. Lancet (1989) 2:1294–8. doi: 10.1016/s0140-6736(89)91909-0
91. Watson WH, Ritzenthaler JD, Peyrani P, Wiemken TL, Furmanek S, Reyes Vega AM, et al. Plasma cysteine/cystine and glutathione/glutathione disulfide redox potentials in HIV and COPD patients. Free Radic Biol Med (2019) 143:55–61. doi: 10.1016/j.freeradbiomed.2019.07.031
92. Levring TB, Hansen AK, Nielsen BL, Kongsbak M, von Essen MR, Woetmann A, et al. Activated human CD4+ T cells express transporters for both cysteine and cystine. Sci Rep (2012) 2:266. doi: 10.1038/srep00266
93. Ziegler TR, Judd SE, Ruff JH, McComsey GA, Eckard AR. Amino acid concentrations in HIV-infected youth compared to healthy controls and associations with CD4 counts and inflammation. AIDS Res Hum Retroviruses (2017) 33:681–9. doi: 10.1089/aid.2015.0369
94. Schumacker PT. Reactive oxygen species in cancer: a dance with the devil. Cancer Cell (2015) 27:156–7. doi: 10.1016/j.ccell.2015.01.007
95. Schumacker PT. Reactive oxygen species in cancer cells: live by the sword, die by the sword. Cancer Cell (2006) 10:175–6. doi: 10.1016/j.ccr.2006.08.015
96. Antonov A, Agostini M, Morello M, Minieri M, Melino G, Amelio I. Bioinformatics analysis of the serine and glycine pathway in cancer cells. Oncotarget (2014) 5:11004–13. doi: 10.18632/oncotarget.2668
97. Possemato R, Marks KM, Shaul YD, Pacold ME, Kim D, Birsoy K, et al. Functional genomics reveal that the serine synthesis pathway is essential in breast cancer. Nature (2011) 476:346–50. doi: 10.1038/nature10350
98. Liou GY, Storz P. Reactive oxygen species in cancer. Free Radic Res (2010) 44:479–96. doi: 10.3109/10715761003667554
99. Ma EH, Bantug G, Griss T, Condotta S, Johnson RM, Samborska B, et al. Serine is an essential metabolite for effector T cell expansion. Cell Metab (2017) 25:345–57. doi: 10.1016/j.cmet.2016.12.011
100. Ducker GS, Rabinowitz JD. One-carbon metabolism in health and disease. Cell Metab (2017) 25:27–42. doi: 10.1016/j.cmet.2016.08.009
101. Amelio I, Cutruzzolá F, Antonov A, Agostini M, Melino G. Serine and glycine metabolism in cancer. Trends Biochem Sci (2014) 39:191–8. doi: 10.1016/j.tibs.2014.02.004
102. Head BM, Mao R, Keynan Y, Rueda ZV. Inflammatory mediators and lung abnormalities in HIV: A systematic review. PloS One (2019) 14:e0226347. doi: 10.1371/journal.pone.0226347
103. Liovat AS, Rey-Cuillé MA, Lécuroux C, Jacquelin B, Girault I, Petitjean G, et al. Acute plasma biomarkers of T cell activation set-point levels and of disease progression in HIV-1 infection. PloS One (2012) 7:e46143. doi: 10.1371/journal.pone.0046143
104. Thorman J, Björkman P, Marrone G, Tolera Balcha T, Tesfaye F, Abdissa T, et al. Interferon-γ-Inducible protein 10 (IP-10) kinetics after antiretroviral treatment initiation in Ethiopian adults with HIV. Microbiol Spectr (2021) 9:e0181021. doi: 10.1128/Spectrum.01810-21
105. Ma A, Koka R, Burkett P. Diverse functions of IL-2, IL-15, and IL-7 in lymphoid homeostasis. Annu Rev Immunol (2006) 24:657–79. doi: 10.1146/annurev.immunol.24.021605.090727
106. Napolitano LA, Grant RM, Deeks SG, Schmidt D, De Rosa SC, Herzenberg LA, et al. Increased production of IL-7 accompanies HIV-1-mediated T-cell depletion: implications for T-cell homeostasis. Nat Med (2001) 7:73–9. doi: 10.1038/83381
107. Boulassel MR, Smith GH, Gilmore N, Klein M, Murphy T, MacLeod J, et al. Interleukin-7 levels may predict virological response in advanced HIV-1-infected patients receiving lopinavir/ritonavir-based therapy. HIV Med (2003) 4:315–20. doi: 10.1046/j.1468-1293.2003.00165.x
108. Chiappini E, Galli L, Azzari C, de Martino M. Interleukin-7 and immunologic failure despite treatment with highly active antiretroviral therapy in children perinatally infected with HIV-1. J Acquir Immune Defic Syndr (2003) 33:601–4. doi: 10.1097/00126334-200308150-00008
109. Resino S, Correa R, Bellón JM, Muñoz-Fernández MA. Preserved immune system in long-term asymptomatic vertically HIV-1 infected children. Clin Exp Immunol (2003) 132:105–12. doi: 10.1046/j.1365-2249.2003.02116.x
110. Correa R, Resino S, Muñoz-Fernández MA. Increased interleukin-7 plasma levels are associated with recovery of CD4+ T cells in HIV-infected children. J Clin Immunol (2003) 23:401–6. doi: 10.1023/a:1025325718213
111. Fry TJ, Mackall CL. Interleukin-7: master regulator of peripheral T-cell homeostasis? Trends Immunol (2001) 22:564–71. doi: 10.1016/s1471-4906(01)02028-2
112. Isgrò A, Aiuti F, Mezzaroma I, Franchi F, Mazzone AM, Lebba F, et al. Interleukin 7 production by bone marrow-derived stromal cells in HIV-1-infected patients during highly active antiretroviral therapy. Aids (2002) 16:2231–2. doi: 10.1097/00002030-200211080-00020
113. Rethi B, Fluur C, Atlas A, Krzyzowska M, Mowafi F, Grützmeier S, et al. Loss of IL-7Ralpha is associated with CD4 T-cell depletion, high interleukin-7 levels and CD28 down-regulation in HIV infected patients. Aids (2005) 19:2077–86. doi: 10.1097/01.aids.0000189848.75699.0f
114. Marziali M, De Santis W, Carello R, Leti W, Esposito A, Isgrò A, et al. T-Cell homeostasis alteration in HIV-1 infected subjects with low CD4 T-cell count despite undetectable virus load during HAART. Aids (2006) 20:2033–41. doi: 10.1097/01.aids.0000247588.69438.fd
115. Afonina IS, Müller C, Martin SJ, Beyaert R. Proteolytic processing of interleukin-1 family cytokines: Variations on a common theme. Immunity (2015) 42:991–1004. doi: 10.1016/j.immuni.2015.06.003
116. Zia K, Ashraf S, Jabeen A, Saeed M, Nur EAM, Ahmed S, et al. Identification of potential TNF-α inhibitors: from in silico to in vitro studies. Sci Rep (2020) 10:20974. doi: 10.1038/s41598-020-77750-3
117. Moran CA, Sheth AN, Mehta CC, Hanna DB, Gustafson DR, Plankey MW, et al. The association of c-reactive protein with subclinical cardiovascular disease in HIV-infected and HIV-uninfected women. Aids (2018) 32:999–1006. doi: 10.1097/qad.0000000000001785
118. Batlle E, Massagué J. Transforming growth factor-β signaling in immunity and cancer. Immunity (2019) 50:924–40. doi: 10.1016/j.immuni.2019.03.024
119. Kong DH, Kim YK, Kim MR, Jang JH, Lee S. Emerging roles of vascular cell adhesion molecule-1 (VCAM-1) in immunological disorders and cancer. Int J Mol Sci (2018) 19(4):1057. doi: 10.3390/ijms19041057
120. Rousseau RK, Szadkowski L, Kovacs CM, Saikali MF, Nadeem R, Malazogu F, et al. Activation and gut-homing of peripheral T cells in HIV immunologic non-responders despite long term viral suppression. PloS One (2021) 16:e0254149. doi: 10.1371/journal.pone.0254149
121. Ajello F, La Licata R, Lodato M, Vitale F, Bonura F, Valenti R, et al. Soluble tumor necrosis factor alpha receptors (sTNF-rs) in HIV-1-infected intravenous drug users: change in circulating sTNF-r type II level and survival for AIDS patients. Eur J Epidemiol (2000) 16:209–16. doi: 10.1023/a:1007632617516
122. Sanchez JL, Hunt PW, Reilly CS, Hatano H, Beilman GJ, Khoruts A, et al. Lymphoid fibrosis occurs in long-term nonprogressors and persists with antiretroviral therapy but may be reversible with curative interventions. J Infect Dis (2015) 211:1068–75. doi: 10.1093/infdis/jiu586
123. Asmuth DM, Pinchuk IV, Wu J, Vargas G, Chen X, Mann S, et al. Role of intestinal myofibroblasts in HIV-associated intestinal collagen deposition and immune reconstitution following combination antiretroviral therapy. Aids (2015) 29:877–88. doi: 10.1097/qad.0000000000000636
124. Li F, Zhu CL, Zhang H, Huang H, Wei Q, Zhu X, et al. Role of hyaluronic acid and laminin as serum markers for predicting significant fibrosis in patients with chronic hepatitis b. Braz J Infect Dis (2012) 16:9–14. doi: 10.1016/s1413-8670(12)70267-2
125. Seven G, Karatayli SC, Köse SK, Yakut M, Kabaçam G, Törüner M, et al. Serum connective tissue markers as predictors of advanced fibrosis in patients with chronic hepatitis b and d. Turk J Gastroenterol (2011) 22:305–14. doi: 10.4318/tjg.2011.0217
126. Park SH, Kim CH, Kim DJ, Suk KT, Park JH, Cheong JY, et al. Diagnostic value of multiple biomarker panel for prediction of significant fibrosis in chronic hepatitis c. Clin Biochem (2011) 44:1396–9. doi: 10.1016/j.clinbiochem.2011.08.1151
127. Papanastasopoulou C, Papastamataki M, Karampatsis P, Anagnostopoulou E, Papassotiriou I, Sitaras N. Cardiovascular risk and serum hyaluronic acid: A preliminary study in a healthy population of Low/Intermediate risk. J Clin Lab Anal (2017) 31(1):e22010. doi: 10.1002/jcla.22010
128. Tobolowsky FA, Wada N, Martinez-Maza O, Magpantay L, Koletar SL, Palella FJ Jr., et al. Brief report: Circulating markers of fibrosis are associated with immune reconstitution status in HIV-infected men. PloS One (2018) 13:e0191606. doi: 10.1371/journal.pone.0191606
129. Huntzinger E, Izaurralde E. Gene silencing by microRNAs: contributions of translational repression and mRNA decay. Nat Rev Genet (2011) 12:99–110. doi: 10.1038/nrg2936
130. Mitchell PS, Parkin RK, Kroh EM, Fritz BR, Wyman SK, Pogosova-Agadjanyan EL, et al. Circulating microRNAs as stable blood-based markers for cancer detection. Proc Natl Acad Sci U.S.A. (2008) 105:10513–8. doi: 10.1073/pnas.0804549105
131. Grasedieck S, Sorrentino A, Langer C, Buske C, Döhner H, Mertens D, et al. Circulating microRNAs in hematological diseases: principles, challenges, and perspectives. Blood (2013) 121:4977–84. doi: 10.1182/blood-2013-01-480079
132. Biswas S, Haleyurgirisetty M, Lee S, Hewlett I, Devadas K. Development and validation of plasma miRNA biomarker signature panel for the detection of early HIV-1 infection. EBioMedicine (2019) 43:307–16. doi: 10.1016/j.ebiom.2019.04.023
133. Munshi SU, Panda H, Holla P, Rewari BB, Jameel S. MicroRNA-150 is a potential biomarker of HIV/AIDS disease progression and therapy. PloS One (2014) 9:e95920. doi: 10.1371/journal.pone.0095920
134. Narla V, Bhakta N, Freedman JE, Tanriverdi K, Maka K, Deeks SG, et al. Unique circulating MicroRNA profiles in HIV infection. J Acquir Immune Defic Syndr (2018) 79:644–50. doi: 10.1097/qai.0000000000001851
135. Reynoso R, Laufer N, Hackl M, Skalicky S, Monteforte R, Turk G, et al. MicroRNAs differentially present in the plasma of HIV elite controllers reduce HIV infection in vitro. Sci Rep (2014) 4:5915. doi: 10.1038/srep05915
136. Asahchop EL, Akinwumi SM, Branton WG, Fujiwara E, Gill MJ, Power C. Plasma microRNA profiling predicts HIV-associated neurocognitive disorder. Aids (2016) 30:2021–31. doi: 10.1097/qad.0000000000001160
137. Asahchop EL, Branton WG, Krishnan A, Chen PA, Yang D, Kong L, et al. HIV-Associated sensory polyneuropathy and neuronal injury are associated with miRNA-455-3p induction. JCI Insight (2018) 3(23):e122450. doi: 10.1172/jci.insight.122450
138. O'Meara T, Kong Y, Chiarella J, Price RW, Chaudhury R, Liu X, et al. Exosomal MicroRNAs associate with neuropsychological performance in individuals with HIV infection on antiretroviral therapy. J Acquir Immune Defic Syndr (2019) 82:514–22. doi: 10.1097/qai.0000000000002187
139. Anadol E, Schierwagen R, Elfimova N, Tack K, Schwarze-Zander C, Eischeid H, et al. Circulating microRNAs as a marker for liver injury in human immunodeficiency virus patients. Hepatology (2015) 61:46–55. doi: 10.1002/hep.27369
140. Franco S, Buccione D, Tural C, Martinez MA. Circulating microRNA signatures that predict liver fibrosis progression in patients with HIV-1/hepatitis c virus coinfections. Aids (2021) 35:1355–63. doi: 10.1097/qad.0000000000002895
141. Fu Y, Liu Y, Liu Z, Liu L, Yuan L, An X, et al. The impact of microRNA regulation on immune recovery in HIV-1-Infected patients treated during acute infection: A pilot study. BioMed Res Int (2020) 2020:5782927. doi: 10.1155/2020/5782927
142. Lv JN, Li JQ, Cui YB, Ren YY, Fu YJ, Jiang YJ, et al. Plasma MicroRNA signature panel predicts the immune response after antiretroviral therapy in HIV-infected patients. Front Immunol (2021) 12:753044. doi: 10.3389/fimmu.2021.753044
143. Shukla S, Kumari S, Bal SK, Monaco DC, Ribeiro SP, Sekaly RP, et al. "Go", "No go," or "Where to go"; does microbiota dictate T cell exhaustion, programming, and HIV persistence? Curr Opin HIV AIDS (2021) 16:215–22. doi: 10.1097/coh.0000000000000692
144. Winston JA, Theriot CM. Diversification of host bile acids by members of the gut microbiota. Gut Microbes (2020) 11:158–71. doi: 10.1080/19490976.2019.1674124
145. Jia W, Xie G, Jia W. Bile acid-microbiota crosstalk in gastrointestinal inflammation and carcinogenesis. Nat Rev Gastroenterol Hepatol (2018) 15:111–28. doi: 10.1038/nrgastro.2017.119
Keywords: human immunodeficiency virus (HIV), acquired immure deficiency syndrome (AIDS), poor immune reconstitution (PIR), plasma markers, fecal markers, innate immunity, microbial translocation
Citation: Xiao Q, Yu F, Yan L, Zhao H and Zhang F (2022) Alterations in circulating markers in HIV/AIDS patients with poor immune reconstitution: Novel insights from microbial translocation and innate immunity. Front. Immunol. 13:1026070. doi: 10.3389/fimmu.2022.1026070
Received: 23 August 2022; Accepted: 29 September 2022;
Published: 17 October 2022.
Edited by:
Zining Zhang, The First Affiliated Hospital of China Medical University, ChinaReviewed by:
Yan-Mei Jiao, Fifth Medical Center of the PLA General Hospital, ChinaYi-Qun Kuang, Kunming Medical University, China
Copyright © 2022 Xiao, Yu, Yan, Zhao and Zhang. This is an open-access article distributed under the terms of the Creative Commons Attribution License (CC BY). The use, distribution or reproduction in other forums is permitted, provided the original author(s) and the copyright owner(s) are credited and that the original publication in this journal is cited, in accordance with accepted academic practice. No use, distribution or reproduction is permitted which does not comply with these terms.
*Correspondence: Fujie Zhang, dHJlYXRtZW50QGNoaW5hYWlkcy5jbg==