- 1Department of Pediatrics, Duke University School of Medicine, Durham, NC, United States
- 2Department of Pediatrics, University of North Carolina at Chapel Hill, Chapel Hill, NC, United States
- 3Department of Nutrition, University of North Carolina at Chapel Hill, Chapel Hill, NC, United States
Disorders of systemic metabolism can influence immunity. Individuals with obesity are known to have increased inflammation, increased risk to select autoimmune diseases, impaired response to several infections, and impaired vaccine response. For example, over the last decade, it has become clear that individuals with obesity have increased risk of morbidity and mortality from influenza infection. Unsurprisingly, this finding is also observed in the current COVID-19 pandemic: individuals with obesity, particularly severe obesity, have increased risk of poor outcomes from SARS-CoV-2 infection, including increased rates of hospitalization, ICU admission, mechanical ventilation, and death. Several studies have now demonstrated a critical role for T cells in the context of obesity-associated immune dysfunction in response to viral infection, and one mechanism for this may be altered T cell metabolism. Indeed, recent studies have shown that activated T cells from obese mice have an altered metabolic profile characterized by increased glucose oxidation, both in vitro and in vivo following viral infection. For that reason, treatments that target abnormal immune cell metabolism in obesity may improve outcomes to viral infection. To that end, several recent studies have shown that use of the metabolic drug, metformin, can reverse abnormal T cell metabolism and restore T cell immunity, as well as survival, in response to viral infection. These findings will be discussed in detail here.
Introduction
Obesity is a significant public health concern due to both a high global prevalence and association with chronic disease. The prevalence of obesity has increased over the last few decades in the United States with the most recent CDC data showing that 42.4% of adults and 19.3% of children and adolescents have obesity (1). Moreover, obesity is the leading cause of preventable death and has become a global economic and health burden (2). Excess stores of adipose tissue in obesity lead to systemic low-grade inflammation which sets the stage for various pathological conditions, including type 2 diabetes mellitus, cardiovascular disease, nonalcoholic fatty liver disease, asthma, various types of cancer, neurodegenerative disease, and others (3). Although less well described, obesity is also associated with impaired immunity, characterized by increased risk of some autoimmune diseases (4–6), poor response to select infections (7, 8), and impaired vaccine response (8). As a specific example, obese individuals have a greater risk of morbidity and mortality from influenza (9–11). Unsurprisingly, this finding is also observed in the current COVID-19 pandemic; individuals with obesity, particularly severe obesity, have increased risk of poor outcomes from infection with SARS-CoV-2, including increased rates of hospitalization, ICU admission, mechanical ventilation, and death (12–17).
Several studies have now demonstrated a critical role for T cells in this setting, with primary and secondary T cell responses to influenza impaired in obese mice and humans (9, 18–20). One potential mechanism by which obesity may alter T cell response is through metabolic reprogramming. T cell function and metabolism are closely linked, and many studies over the last decade have clearly demonstrated that changes to T cell metabolism influence T cell response (21). More recent studies have described the effects of obesity on T cell metabolism and identified T cell metabolic reprogramming as a potential mechanism for impaired response to viral infection in obesity (22, 23). Understanding the mechanisms by which obesity leads to inflammation and impaired immune response to viral infection is necessary to identify targeted treatments to improve outcomes in this high-risk population.
Obesity and influenza outcomes
In 2009, a novel influenza A (H1N1) virus caused pandemic influenza. A systemic review with meta-analysis performed during the pandemic showed that obesity significantly increased the risk of morbidity and mortality from H1N1 influenza infection (24). A global observational study published in 2011 presented H1N1 influenza data from approximately 70,000 patients requiring hospitalization, 9,700 patients admitted to intensive care units, and 2,500 deaths from 19 countries. This study reported high proportions of obesity among patients admitted to the ICU and among fatal H1N1 cases (25). These results supported the observation that obesity may be a risk factor for severe influenza disease. Further, a case-cohort study comparing rates of hospitalization and deaths from 2009 H1N1 influenza found that morbid obesity was associated with increased risk of hospitalization and death in adult patients (10). Notably, the authors found that individuals with morbid obesity, even in the absence of other chronic medical conditions, were at increased risk for severe outcomes due to 2009 H1N1 infection (10). Another study examined 534 adult patients hospitalized in California with H1N1 influenza virus and found that 51% of the hospitalized patients had a BMI ≥ 30 (11), which was 2.2 times higher than the prevalence of obesity in adults from California (23.2%) and 1.5 times higher than the prevalence of obesity in US adults (33% at the time). Furthermore, the percentage of hospitalized patients with BMI ≥ 40 was 2.5 times higher than the prevalence of BMI ≥ 40 in the US population, and the percentage of hospitalized patients with BMI ≥ 45 was 10 times higher than the prevalence of BMI ≥ 45 in the US population (11). Importantly, individuals with severe obesity (BMI ≥ 40) had increased likelihood of mortality from influenza infection following hospitalization from H1N1 influenza (11). In a separate meta-analysis describing associations of obesity and poor outcomes during the 2009 H1N1 pandemic, the authors found that obesity doubled the risk of ICU admission and/or death (26). As in previous studies, they concluded that these associations were stronger in severely obese patients (26).
Following these observations made during the 2009 H1N1 influenza pandemic, it became clear that patients with obesity also had worse outcomes following infection with seasonal influenza. One meta-analysis designed to study the association between obesity and influenza infection showed that patients with obesity had a significantly higher risk for influenza infection, as well as an increased risk for hospitalization following infection (27). Patients with BMI ≥ 40 had an even higher risk of hospitalization (27). Moreover, patients with obesity were at increased risk for severe outcomes related to influenza infection including increased likelihood of admission to the ICU, need for mechanical ventilation, and death (27). Again, morbid obesity was found to be a significant predictor for severe outcomes and death from influenza (27).
Although influenza vaccination provides the best method for protection against influenza infection, several studies have demonstrated that obesity impairs the response to vaccination (18, 20, 28). Indeed, obesity increases the risk for vaccine failure to influenza (18), hepatitis B (29) and tetanus (30). Adequate vaccine response depends on both B cell (antibody) and T cell (cellular) memory responses, and the mechanism for impaired vaccine response may be due to impaired T cell function, as T cells from obese adults vaccinated for influenza were less activated when stimulated with influenza virus compared to T cells from vaccinated healthy weight adults, and despite adequate serological response to vaccination (28). Moreover, individuals with obesity were found to be twice as likely to develop influenza infection than healthy weight controls, despite vaccination (28).
Obesity and SARS-CoV-2 outcomes
Similar to influenza, multiple studies have demonstrated that COVID-19 patients with obesity have increased rates of hospitalization, ICU admission, mechanical ventilation, and death compared to COVID-19 patients with normal weight (12–17). This increased risk of severe outcomes correlates with BMI, with increasing risk of severity as BMI rises from the overweight category to severe obesity (13). Severe COVID-19 infections are associated with pro-inflammatory injury and cytokine storm, leading to complications such as COVID-19 pneumonia, acute respiratory distress syndrome, and multi-organ failure (31). One hypothesis is that obesity-associated inflammation increases the susceptibility to severe COVID-19 infection (32). Inflammatory cytokines such as IL-6 and TNF are already increased in patients who are overweight or obese (33). Several recent studies have shown that individuals with moderate to severe COVID-19 also have high levels of inflammatory cytokines, such as IL-6, CRP, TNF, and IL-17 (31, 34–36). A recent multi-omics study revealed that pro-inflammatory proteins associated with immune cell activation strongly contributed to more severe disease (37). IL-6 and MCP-1 were further identified in a separate study to strongly associate with fatality (38). In addition to acute infection, persistent heightened inflammation and T cell dysregulation occurs in individuals following COVID-19 infection (39–42). Thus, the already pro-inflammatory state observed in obesity could contribute to the overwhelming cytokine storm seen in severe COVID-19, although further studies are needed to clarify the role of inflammatory cytokines in individuals with obesity and SARS-CoV-2 infection.
T cell dysfunction to viral infection in obesity
Data from a prospective study published in 2013 showed that individuals with obesity mounted a vigorous initial antibody response to influenza vaccination; however, they had a greater than four-fold decrease in vaccine titers at 12 months post vaccination compared to titers at 1 month (9). In comparison, less than 25% of healthy weight controls had a greater than four-fold decrease in vaccine titers at 12 months post vaccination compared to titers at 1 month. Moreover, vaccinated subjects with obesity had a lower percentage of cytotoxic CD8+ T cells, and these CD8+ cells produced lower amounts of IFN-γ and granzyme B, compared to normal weight subjects (9). This change in CD8+ T cell number and function is critical, as CD8+ T cells help slow the spread and reduce the severity of influenza infection by causing cytotoxicity of influenza infected cells. This study also showed that CD4+ T helper cells from subjects with obesity had defects in activation and function when challenged with pH1N1 ex vivo (9). Altogether, these findings suggest that abnormal T cell responses may explain, at least in part, the increased risk of morbidity and mortality from influenza infection in individuals with obesity (18). These human findings have been supported by work in a mouse model. Influenza-infected obese mice were found to accumulate fewer activated and functional memory T cells compared to lean mice, and did not maintain memory T cells over time, which resulted in increased mortality following a secondary influenza infection (20). Notably, this reduction in memory T cells includes resident memory T cells in the lung, which are critically important for protection against subsequent infections (22). These findings underscore the concern that current vaccine and vaccine approaches may be less effective in an increasingly obese population.
Altered T cell metabolism in obesity
A number of studies have demonstrated that T cell function and metabolism are closely linked, and that changes to T cell metabolism influence T cell activation, differentiation, and function (21). In recent studies performed in a mouse model of obesity, activated CD4+ T cells from diet induced obese mice had an altered metabolic profile characterized by increased glucose uptake, increased conversion of glucose to pyruvate, and increased mitochondrial oxidation (23). This represents a unique metabolic phenotype of glucose oxidation that is not utilized by T cells from lean animals and may mechanistically explain, at least in part, obesity-associated immune dysfunction (Figure 1). Similar increases in mitochondrial oxidation were seen in CD4+ T cells isolated from obese mice following influenza reinfection (22). Interestingly, weight loss following a primary influenza infection in obese mice did not restore T cell memory response to influenza reinfection or reverse the obesity-induced changes in T cell mitochondrial metabolism (22). This suggests that obesity induces a unique abnormal T cell metabolic profile that cannot be reversed by weight loss.
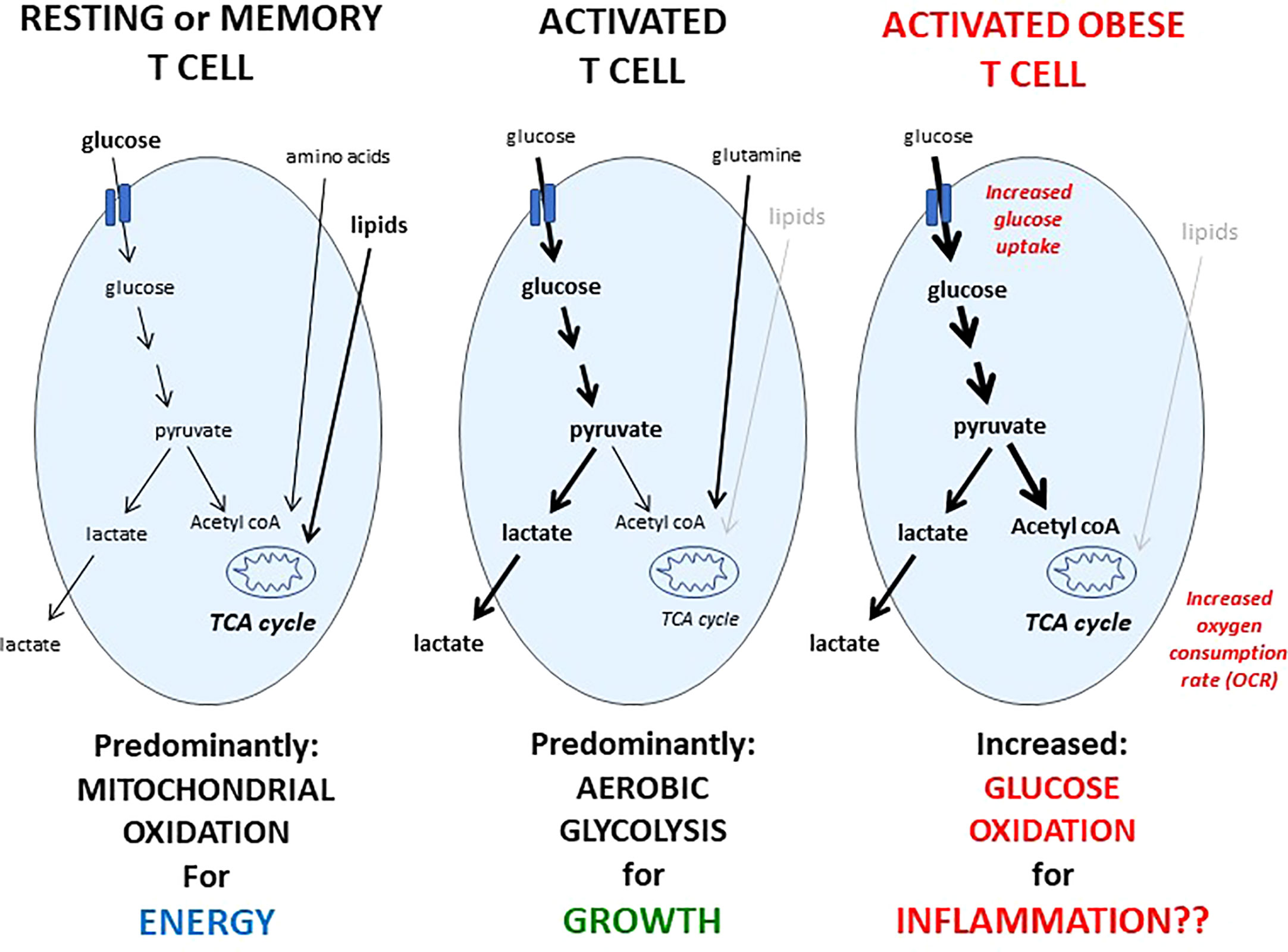
Figure 1 Metabolic phenotypes of resting, activated, or activated obese T cells. Resting T cells from lean humans or animals take up a mix of fuels: glucose, amino acids, and lipids, and predominantly metabolize these fuels in the mitochondria, which is an efficient way to generate ATP to fuel immune surveillance. Upon activation, the metabolic phenotype of a T cell changes, and glucose uptake and metabolism is significantly increased, although glutamine metabolism is also critical for T cell activation. In contrast to resting T cells, glucose taken up by activated T cells is converted into lactate in a metabolic program of aerobic glycolysis, which is a metabolic phenotype that generates critical biomass needed for growth, proliferation, and to produce critical factors needed for a successful immune response. Following activation, a portion of the T cell responders will become memory cells, and like resting T cells, memory T cells also rely on mitochondrial oxidation to fuel immune surveillance. Activated T cells from humans or mice with obesity demonstrate an increase in both glucose uptake and mitochondrial oxidation compared to activated T cells from lean animals, leading to a unique metabolic phenotype of glucose oxidation, which is not utilized by resting or memory T cells from lean animals, may explain mechanistically, at least in part, the T cell dysfunction seen in obesity, and may be targeted therapeutically.
Because weight loss was insufficient to reverse the obesity-associated abnormal T cell metabolism and function, other studies have attempted to use metabolic drugs to target T cell metabolism in obesity. One candidate was the commonly prescribed anti-diabetes drug, metformin. Metformin targets mitochondrial oxidation by disrupting electron transport in the mitochondria, presumably through inhibition of complex I of the electron transport chain (43). Inhibition of electron transport suppresses mitochondrial ATP production, and thereby activates the AMP-activated kinase (AMPK) (43), although metformin-induced activation of AMPK may not account for all actions of the drug (44, 45). Activated AMPK inhibits the activity of mammalian target of rapamycin (mTOR), a serine/threonine kinase which is a central regulator of growth, proliferation, and metabolism (46).
In recent studies, CD4+ T cells activated in the presence of metformin had decreased oxidative metabolism and changes in activation markers suggesting an improvement in memory potential, indicating a direct effect of metformin on T cell metabolism and function, and suggesting metformin might reverse T cell abnormalities in obese mice (23). Consistent with this, systemic treatment of obese mice with metformin was able to improve survival following an influenza challenge, compared to obese mice not treated with metformin or obese mice switched to low fat chow to induce weight loss (23). Moreover, CD4+ T cells isolated from influenza infected obese mice treated with metformin had normalization of T cell mitochondrial oxidation compared to untreated influenza infected obese mice (23), suggesting that metformin improved T cell immunity following infection, in part, through its ability to normalize T cell metabolism.
SARS-CoV-2 and metformin
Metformin is a commonly prescribed drug for the treatment of type 2 diabetes mellitus and is also used off-label to treat obesity and obesity-associated disease. Metformin belongs to the biguanide class of antidiabetic drugs, has an excellent safety profile, is affordable, and is well tolerated by most patients. Studies have shown that metformin may also have other positive effects on health including anti-inflammatory and immune modulating effects. For example, metformin has been shown to lower inflammatory cytokines IL-6 and TNF while increasing the anti-inflammatory cytokine IL-10 (47). Because severe COVID-19 infection can lead to a pro-inflammatory cytokine storm, ultimately leading to acute respiratory distress syndrome and multi-organ failure (48), it is plausible to consider that the anti-inflammatory properties of metformin could be beneficial in this context.
In addition to its anti-inflammatory and immune modulating effects, metformin has also been shown to attenuate lung injury and reverse disease in a mouse model of lung fibrosis (49), suggesting that metformin might have an analogous benefit in improving acute respiratory disease in COVID-19. Metformin also improves glucose levels, reduces body weight, and reduces insulin resistance; all of which are important factors for improving immune responses and infectious outcomes (50). Additionally, metformin may confer protection against severe SARS-CoV-2 by extending the half-life of the ACE2 receptor. Although ACE2 is required for viral entry into cells, treatments to increase ACE2 have been shown to protect against severe cardiopulmonary complications of COVID-19 infections. In this setting, metformin works by activating AMP kinase (AMPK), which leads to phosphorylation of ACE2, enhancing its stability and increasing the expression of ACE2 protein (51).
Over the course of the COVID-19 pandemic, a number of studies have examined the effect of metformin on infection outcomes. Several retrospective studies have demonstrated reduction in mortality in patients with COVID-19 (47, 52–63). Some studies found a benefit in all patients taking metformin, but other studies only reported benefit in patients with obesity or type 2 diabetes (47, 52–57). Furthermore, several of these studies were systematic reviews and meta-analyses looking at large numbers of patients with COVID-19. One large meta-analysis looking at 5 studies and 6,937 patients showed by pooled analysis that metformin use is associated with reduction in the mortality rate from COVID-19 infections (60). The French nationwide CORONADO study observing 2,449 patients with diabetes hospitalized with COVID-19 infection showed a lower mortality rate on day 7 and 28 of illness among metformin users (56). Another large meta-analysis and systematic review looking at 28 studies with 2,910,462 individuals with COVID-19 infection showed a reduced risk of mortality and hospitalization in those taking metformin (61). A meta-analysis of 19 studies showed that metformin is associated with 34% lower COVID-19 mortality and 27% lower hospitalization rate (61). Another systematic review and meta-analysis including 9 studies and over 10,000 subjects across multiple countries found that metformin use was associated with lower mortality in hospitalized patients with COVID-19 (62). Finally, a meta-analysis including over 20,000 patients with diabetes found that metformin was associated with decreased mortality and severity in COVID-19 (54).
Two studies found a reduction in mortality among female patients only (64, 65). The authors postulated the reduction in mortality was due to metformin’s “sex-specific immunomodulatory actions”, in which the benefits of metformin are more significant in females than in males (64). Notably, metformin has been shown to reduce systemic TNF levels to greater degree in females than in males, which may explain, at least in part, the sex-specific benefit of treatment in female patients (64).
Although metformin is associated with an increased risk of developing lactic acidosis during illness, several studies looked at the question of whether or not to continue metformin treatment in patients hospitalized for COVID-19 (55, 66–68). One study found that the risk of lactic acidosis associated with metformin exposure was higher in patients with severe COVID-19 disease, patients with kidney impairment, and in patients taking more than 2 doses of metformin daily (66). Interestingly, several studies reported that in-hospital metformin therapy may be beneficial and reduce the risk of mortality in patients hospitalized with COVID-19 (55, 67, 68). It is therefore reasonable to consider continuing metformin therapy during hospitalization if kidney function is carefully monitored; however, further studies are needed before making definitive recommendations.
Other studies have shown improvement in COVID-19 outcomes in those taking metformin prior to hospital admission, including outcomes such as decreased rate of ICU admission (69) and decreased rate of hospitalization (70). Given the overwhelming number of studies showing an association with metformin and improved COVID-19 outcomes, the TOGETHER trial was conducted in Brazil in 2021. This placebo-controlled, randomized, clinical trial included 418 subjects, half randomized to taking metformin and half to placebo. There were no significant differences between the metformin and placebo groups on viral clearance through day 7 of illness and no difference in reducing emergency visits or reducing hospitalization for severe disease (71). This study suggests that acute administration of metformin may not be as beneficial as longer-term use of metformin.
Discussion
Individuals with obesity are at increased risk of severe outcomes, including increased mortality, from both influenza and COVID-19. Because studies have shown that weight loss alone may be insufficient to reverse the immune dysfunction seen in obesity, there is a need to identify alternative therapeutic approaches to restore immune response to viral infections in the obese population. Although many immune cells are required for an effective anti-viral response, T cells are critical, and activated T cell function and metabolism are altered in obesity. The unique metabolic phenotype of glucose oxidation, which is not utilized by resting or memory T cells from lean animals but is seen in activated T cells from obese animals, may explain mechanistically, at least in part, the T cell dysfunction seen in obesity, and may be targeted therapeutically. Mouse studies have investigated the use of metformin to normalize T cell oxidative metabolism and restore immune response to viral infection in obese mice. Moreover, over the course of the last two years, several reports have shown that long-term use of metformin is associated with improved COVID-19 outcomes in humans. Although acute use of metformin does not appear to improve COVID-19 outcomes, long-term use of metformin in individuals with diabetes or obesity should be considered as potentially beneficial to both controlling systemic metabolism as well as improving immune responses to potentially life-threatening infections.
Author contributions
EG and NM contributed substantially to the writing in this review article.
Conflict of interest
The authors declare that the research was conducted in the absence of any commercial or financial relationships that could be construed as a potential conflict of interest.
Publisher’s note
All claims expressed in this article are solely those of the authors and do not necessarily represent those of their affiliated organizations, or those of the publisher, the editors and the reviewers. Any product that may be evaluated in this article, or claim that may be made by its manufacturer, is not guaranteed or endorsed by the publisher.
References
1. Skinner AC, Ravanbakht SN, Skelton JA, Perrin EM, Armstrong SC. Prevalence of obesity and severe obesity in US children, 1999–2016. Pediatrics (2018) 141(3):e20173459. doi: 10.1542/9781610022781-prevalence
2. Andersen CJ, Murphy KE, Fernandez ML. Impact of obesity and metabolic syndrome on immunity. Adv Nutr (2016) 7(1):66–75. doi: 10.3945/an.115.010207
3. Andrade FB, Gualberto A, Rezende C, Percegoni N, Gameiro J, Hottz ED. The weight of obesity in immunity from influenza to COVID-19. Front Cell Infect Microbiol (2021) 11:638852. doi: 10.3389/fcimb.2021.638852
4. Harpsøe MC, Basit S, Andersson M, Nielsen NM, Frisch M, Wohlfahrt J, et al. Body mass index and risk of autoimmune diseases: a study within the Danish national birth cohort. Int J Epidemiol (2014) 43(3):843–55. doi: 10.1093/ije/dyu045
5. Duntas LH, Biondi B. The interconnections between obesity, thyroid function, and autoimmunity: The multifold role of leptin. Thyroid (2013) 23(6):646–53. doi: 10.1089/thy.2011.0499
6. Lukens JR, Dixit VD, Kanneganti TD. Inflammasome activation in obesity-related inflammatory diseases and autoimmunity. Discov Med (2011) 12(62):65–74.
7. Falagas ME, Kompoti M. Obesity and infection. Lancet Infect Dis (2006) 6(7):438–46. doi: 10.1016/S1473-3099(06)70523-0
8. Cohen S, Danzaki K, Maciver NJ. Nutritional effects on T-cell immunometabolism. Eur J Immunol (2017) 47(2):225–35. doi: 10.1002/eji.201646423
9. Paich HA, Sheridan PA, Handy J, Karlsson EA, Schultz-Cherry S, Hudgens MG, et al. Overweight and obese adult humans have a defective cellular immune response to pandemic H1N1 influenza a virus. Obesity (2013) 21(11):2377–86. doi: 10.1002/oby.20383
10. Morgan OW, Bramley A, Fowlkes A, Freedman DS, Taylor TH, Gargiullo P, et al. Morbid obesity as a risk factor for hospitalization and death due to 2009 pandemic influenza A(H1N1) disease. PloS One (2010) 5(3):e9694. doi: 10.1371/journal.pone.0009694
11. Louie JK, Acosta M, Samuel MC, Schechter R, Vugia DJ, Harriman K, et al. A novel risk factor for a novel virus: obesity and 2009 pandemic influenza a (H1N1). Clin Infect Dis (2011) 52(3):301–12. doi: 10.1093/cid/ciq152
12. Czernichow S, Beeker N, Rives-Lange C, Guerot E, Diehl JL, Katsahian S, et al. Obesity doubles mortality in patients hospitalized for severe acute respiratory syndrome coronavirus 2 in Paris hospitals, France: A cohort study on 5,795 patients. Obesity (2020) 28(12):2282–9. doi: 10.1002/oby.23014
13. Popkin BM, Du S, Green WD, Beck MA, Algaith T, Herbst CH, et al. Individuals with obesity and COVID-19: A global perspective on the epidemiology and biological relationships. Obes Rev (2020) 21(11):e13128. doi: 10.1111/obr.13128
14. Petrilli CM, Jones SA, Yang J, Rajagopalan H, O’Donnell L, Chernyak Y, et al. Factors associated with hospital admission and critical illness among 5279 people with coronavirus disease 2019 in new York city: Prospective cohort study. BMJ (2020) 369:m1966. doi: 10.1136/bmj.m1966
15. Lighter J, Phillips M, Hochman S, Sterling S, Johnson D, Francois F, et al. Obesity in patients younger than 60 years is a risk factor for COVID-19 hospital admission. Clin Infect Diseases (2020) 71(15):896–7. doi: 10.1093/cid/ciaa415
16. Kalligeros M, Shehadeh F, Mylona EK, Benitez G, Beckwith CG, Chan PA, et al. Association of obesity with disease severity among patients with coronavirus disease 2019. Obesity (2020) 28(7):1200–4. doi: 10.1002/oby.22859
17. Simonnet A, Chetboun M, Poissy J, Raverdy V, Noulette J, Duhamel A, et al. High prevalence of obesity in severe acute respiratory syndrome coronavirus-2 (SARS-CoV-2) requiring invasive mechanical ventilation. Obes (Silver Spring) (2020) 28(7):1195–9. doi: 10.1002/oby.22831
18. Sheridan PA, Paich HA, Handy J, Karlsson EA, Hudgens MG, Sammon AB, et al. Obesity is associated with impaired immune response to influenza vaccination in humans. Int J Obes (2012) 36(8):1072–7. doi: 10.1038/ijo.2011.208
19. Smith AG, Sheridan PA, Harp JB, Beck MA. Diet-induced obese mice have increased mortality and altered immune responses when infected with influenza virus. J Nutr (2007) 137(5):1236–43. doi: 10.1093/jn/137.5.1236
20. Karlsson EA, Sheridan PA, Beck MA. Diet-induced obesity impairs the T cell memory response to influenza virus infection. J Immunol (2010) 184(6):3127–33. doi: 10.4049/jimmunol.0903220
21. MacIver NJ, Michalek RD, Rathmell JC. Metabolic regulation of T lymphocytes. Annu Rev Immunol (2013) 31:259–83. doi: 10.1146/annurev-immunol-032712-095956
22. Rebeles J, Green WD, Alwarawrah Y, Nichols AG, Eisner W, Danzaki K, et al. Obesity-induced changes in T-cell metabolism are associated with impaired memory T-cell response to influenza and are not reversed with weight loss. J Infect Dis (2019) 219(10):1652–61. doi: 10.1093/infdis/jiy700
23. Alwarawrah Y, Nichols AG, Green WD, Eisner W, Kiernan K, Warren J, et al. Targeting T-cell oxidative metabolism to improve influenza survival in a mouse model of obesity. Int J Obes (Lond) (2020) 44(12):2419–29. doi: 10.1038/s41366-020-00692-3
24. Sun Y, Wang Q, Yang G, Lin C, Zhang Y, Yang P. Weight and prognosis for influenza A(H1N1)pdm09 infection during the pandemic period between 2009 and 2011: A systematic review of observational studies with meta-analysis. Infect Diseases (2016) 48(11-12):813–22. doi: 10.1080/23744235.2016.1201721
25. Van Kerkhove MD, Vandemaele KAH, Shinde V, Jaramillo-Gutierrez G, Koukounari A, Donnelly CA, et al. (H1N1) infection: A global pooled analysis. PloS Med (2011) 8(7):e1001053. doi: 10.1371/journal.pmed.1001053
26. Fezeu L, Julia C, Henegar A, Bitu J, Hu FB, Grobbee DE, et al. Obesity is associated with higher risk of intensive care unit admission and death in influenza a (H1N1) patients: A systematic review and meta-analysis. Obes Rev (2011) 12(8):653–9. doi: 10.1111/j.1467-789X.2011.00864.x
27. Zhao X, Gang X, He G, Li Z, Lv Y, Han Q, et al. Obesity increases the severity and mortality of influenza and COVID-19: A systematic review and meta-analysis. Front Endocrinol (Lausanne) (2020) 11:595109. doi: 10.3389/fendo.2020.595109
28. Neidich SD, Green WD, Rebeles J, Karlsson EA, Schultz-Cherry S, Noah TL, et al. Increased risk of influenza among vaccinated adults who are obese. Int J Obes (2017) 41(9):1324–30. doi: 10.1038/ijo.2017.131
29. Bandaru P, Rajkumar H, Nappanveettil G. Altered or impaired immune response to hepatitis b vaccine in WNIN/GR-ob rat: An obese rat model with impaired glucose tolerance. ISRN Endocrinology (2011) 2011:1–7. doi: 10.5402/2011/980105
30. Eliakim A, Swindt C, Zaldivar F, Casali P, Cooper DM. Reduced tetanus antibody titers in overweight children. Autoimmunity (2006) 39(2):137–41. doi: 10.1080/08916930600597326
31. Huang C, Wang Y, Li X, Ren L, Zhao J, Hu Y, et al. Clinical features of patients infected with 2019 novel coronavirus in wuhan, China. Lancet (2020) 395(10223):497–506. doi: 10.1016/S0140-6736(20)30183-5
32. Sales-Peres SHC, de Azevedo-Silva LJ, Bonato RCS, Sales-Peres MC, Pinto A, Santiago Junior JF. Coronavirus (SARS-CoV-2) and the risk of obesity for critically illness and ICU admitted: Meta-analysis of the epidemiological evidence. Obes Res Clin Pract (2020) 14(5):389–97. doi: 10.1016/j.orcp.2020.07.007
33. Calder PC, Ahluwalia N, Brouns F, Buetler T, Clement K, Cunningham K, et al. Dietary factors and low-grade inflammation in relation to overweight and obesity. Br J Nutr (2011) 106(S3):S5–S78. doi: 10.1017/S0007114511005460
34. Wang J, Jiang M, Chen X, Montaner LJ. Cytokine storm and leukocyte changes in mild versus severe SARS-CoV-2 infection: Review of 3939 COVID-19 patients in China and emerging pathogenesis and therapy concepts. J Leukoc Biol (2020) 108(1):17–41. doi: 10.1002/JLB.3COVR0520-272R
35. Aziz M, Fatima R, Assaly R. Elevated interleukin-6 and severe COVID-19: A meta-analysis. J Med Virol (2020) 92(11):2283–5. doi: 10.1002/jmv.25948
36. Lippi G, Plebani M. Laboratory abnormalities in patients with COVID-2019 infection. Clin Chem Lab Med (2020) 58(7):1131–4. doi: 10.1515/cclm-2020-0198
37. Su Y, Chen D, Yuan D, Lausted C, Choi J, Dai CL, et al. Multi-omics resolves a sharp disease-state shift between mild and moderate COVID-19. Cell (2020) 183(6):1479–95 e20. doi: 10.1016/j.cell.2020.10.037
38. Pons MJ, Ymana B, Mayanga-Herrera A, Saenz Y, Alvarez-Erviti L, Tapia-Rojas S, et al. Cytokine profiles associated with worse prognosis in a hospitalized Peruvian COVID-19 cohort. Front Immunol (2021) 12:700921. doi: 10.3389/fimmu.2021.700921
39. Yang X, Yu Y, Xu J, Shu H, Xia J, Liu H, et al. Clinical course and outcomes of critically ill patients with SARS-CoV-2 pneumonia in wuhan, China: A single-centered, retrospective, observational study. Lancet Respir Med (2020) 8(5):475–81. doi: 10.1016/S2213-2600(20)30079-5
40. Blanco-Melo D, Nilsson-Payant BE, Liu WC, Uhl S, Hoagland D, Moller R, et al. Imbalanced host response to SARS-CoV-2 drives development of COVID-19. Cell (2020) 181(5):1036–45 e9. doi: 10.1016/j.cell.2020.04.026
41. Zhang JJ, Dong X, Cao YY, Yuan YD, Yang YB, Yan YQ, et al. Clinical characteristics of 140 patients infected with SARS-CoV-2 in wuhan, China. Allergy (2020) 75(7):1730–41. doi: 10.1111/all.14238
42. Hasichaolu, Zhang X, Li X, Li X, Li D. Circulating cytokines and lymphocyte subsets in patients who have recovered from COVID-19. BioMed Res Int (2020) 2020:7570981. doi: 10.1155/2020/7570981
43. Rena G, Hardie DG, Pearson ER. The mechanisms of action of metformin. Diabetologia (2017) 60(9):1577–85. doi: 10.1007/s00125-017-4342-z
44. Ala M, Ala M. Metformin for cardiovascular protection, inflammatory bowel disease, osteoporosis, periodontitis, polycystic ovarian syndrome, neurodegeneration, cancer, inflammation and senescence: What is next? ACS Pharmacol Transl Sci (2021) 4(6):1747–70. doi: 10.1021/acsptsci.1c00167
45. Kaneto H, Kimura T, Obata A, Shimoda M, Kaku K. Multifaceted mechanisms of action of metformin which have been unraveled one after another in the long history. Int J Mol Sci (2021) 22(5):2596. doi: 10.3390/ijms22052596
46. Gonzalez A, Hall MN, Lin SC, Hardie DG. AMPK and TOR: The yin and yang of cellular nutrient sensing and growth control. Cell Metab (2020) 31(3):472–92. doi: 10.1016/j.cmet.2020.01.015
47. Luo P, Qiu L, Liu Y, Liu X-L, Zheng J-L, Xue H-Y, et al. Metformin treatment was associated with decreased mortality in COVID-19 patients with diabetes in a retrospective analysis. Am J Trop Med Hygiene (2020) 103(1):69–72. doi: 10.4269/ajtmh.20-0375
48. Ye Q, Wang B, Mao J. The pathogenesis and treatment of the `Cytokine storm’ in COVID-19. J Infection (2020) 80(6):607–13. doi: 10.1016/j.jinf.2020.03.037
49. Rangarajan S, Bone NB, Zmijewska AA, Jiang S, Park DW, Bernard K, et al. Metformin reverses established lung fibrosis in a bleomycin model. Nat Med (2018) 24(8):1121–7. doi: 10.1038/s41591-018-0087-6
50. Scheen AJ. Metformin and COVID-19: From cellular mechanisms to reduced mortality. Diabetes Metab (2020) 46(6):423–6. doi: 10.1016/j.diabet.2020.07.006
51. Zhang J, Dong J, Martin M, He M, Gongol B, Marin TL, et al. AMP-activated protein kinase phosphorylation of angiotensin-converting enzyme 2 in endothelium mitigates pulmonary hypertension. Am J Respir Crit Care Med (2018) 198(4):509–20. doi: 10.1164/rccm.201712-2570OC
52. Li J, Wei Q, Li WX, McCowen KC, Xiong W, Liu J, et al. Metformin use in diabetes prior to hospitalization: Effects on mortality in covid-19. Endocr Pract (2020) 26(10):1166–72. doi: 10.4158/EP-2020-0466
53. Poly TN, Islam MM, Li YJ, Lin MC, Hsu MH, Wang YC. Metformin use is associated with decreased mortality in COVID-19 patients with diabetes: Evidence from retrospective studies and biological mechanism. J Clin Med (2021) 10(16):3507. doi: 10.3390/jcm10163507
54. Yang W, Sun X, Zhang J, Zhang K. The effect of metformin on mortality and severity in COVID-19 patients with diabetes mellitus. Diabetes Res Clin Pract (2021) 178:108977. doi: 10.1016/j.diabres.2021.108977
55. Ong AN, Tan CC, Cañete MT, Lim BA, Robles J. Association between metformin use and mortality among patients with type 2 diabetes mellitus hospitalized for COVID-19 infection. J ASEAN Fed Endocr Soc (2021) 36(2):133–41. doi: 10.15605/jafes.036.02.20
56. Lalau JD, Al-Salameh A, Hadjadj S, Goronflot T, Wiernsperger N, Pichelin M, et al. Metformin use is associated with a reduced risk of mortality in patients with diabetes hospitalised for COVID-19. Diabetes Metab (2021) 47(5):101216. doi: 10.1016/j.diabet.2020.101216
57. Zangiabadian M, Nejadghaderi SA, Zahmatkesh MM, Hajikhani B, Mirsaeidi M, Nasiri MJ. The efficacy and potential mechanisms of metformin in the treatment of COVID-19 in the diabetics: A systematic review. Front Endocrinol (Lausanne) (2021) 12:645194. doi: 10.3389/fendo.2021.645194
58. Lally MA, Tsoukas P, Halladay CW, O’Neill E, Gravenstein S, Rudolph JL. Metformin is associated with decreased 30-day mortality among nursing home residents infected with SARS-CoV2. J Am Med Dir Assoc (2021) 22(1):193–8. doi: 10.1016/j.jamda.2020.10.031
59. Saygili ES, Karakiliç E, Mert E, Şener A, Mirci A. Preadmission usage of metformin and mortality in COVID-19 patients including the post-discharge period. Ir J Med Sci (2022) 191(2):569–75. doi: 10.1007/s11845-021-02823-9
60. Hariyanto TI, Kurniawan A. Metformin use is associated with reduced mortality rate from coronavirus disease 2019 (COVID-19) infection. Obes Med (2020) 19:100290. doi: 10.1016/j.obmed.2020.100290
61. Li Y, Yang X, Yan P, Sun T, Zeng Z, Li S. Metformin in patients with COVID-19: A systematic review and meta-analysis. Front Med (Lausanne) (2021) 8:704666. doi: 10.3389/fmed.2021.704666
62. Lukito AA, Pranata R, Henrina J, Lim MA, Lawrensia S, Suastika K. The effect of metformin consumption on mortality in hospitalized COVID-19 patients: a systematic review and meta-analysis. Diabetes Metab Syndr (2020) 14(6):2177–83. doi: 10.1016/j.dsx.2020.11.006
63. Crouse AB, Grimes T, Li P, Might M, Ovalle F, Shalev A. Metformin use is associated with reduced mortality in a diverse population with COVID-19 and diabetes. Front Endocrinol (Lausanne) (2020) 11:600439. doi: 10.3389/fendo.2020.600439
64. Bramante CT, Ingraham NE, Murray TA, Marmor S, Hovertsen S, Gronski J, et al. Metformin and risk of mortality in patients hospitalised with COVID-19: A retrospective cohort analysis. Lancet Healthy Longev (2021) 2(1):e34–41. doi: 10.1016/S2666-7568(20)30033-7
65. Jiang N, Chen Z, Liu L, Yin X, Yang H, Tan X, et al. Association of metformin with mortality or ARDS in patients with COVID-19 and type 2 diabetes: A retrospective cohort study. Diabetes Res Clin Pract (2021) 173:108619. doi: 10.1016/j.diabres.2020.108619
66. Cheng X, Liu Y-M, Li H, Zhang X, Lei F, Qin J-J, et al. Metformin is associated with higher incidence of acidosis, but not mortality, in individuals with COVID-19 and pre-existing type 2 diabetes. Cell Metab (2020) 32(4):537–47.e3. doi: 10.1016/j.cmet.2020.08.013
67. Lazarus G, Suhardi IP, Wiyarta E, Rasyidah RA, Barliana JD. Is there a need to reconsider the use of metformin in COVID-19 patients with type 2 diabetes mellitus? Int J Diabetes Dev Ctries (2021) 41(3):377–82. doi: 10.1007/s13410-021-00924-w
68. Li J, Wei Q, McCowen KC, Xiong W, Liu J, Jiang W, et al. Inpatient use of metformin and acarbose is associated with reduced mortality of COVID-19 patients with type 2 diabetes mellitus. Endocrinol Diabetes Metab (2022) 5(1):e00301. doi: 10.1002/edm2.301
69. Cheng X, Xin S, Chen Y, Li L, Chen W, Li W, et al. Effects of metformin, insulin on COVID-19 patients with pre-existed type 2 diabetes: A multicentral retrospective study. Life Sci (2021) 275:119371. doi: 10.1016/j.lfs.2021.119371
70. Ojeda-Fernandez L, Foresta A, Macaluso G, Colacioppo P, Tettamanti M, Zambon A, et al. Metformin use is associated with a decrease in the risk of hospitalization and mortality in COVID-19 patients with diabetes: A population-based study in Lombardy. Diabetes Obes Metab (2022) 24(5):891–898. doi: 10.1111/DOM.14648/v2/response1
71. Reis G, Dos Santos Moreira Silva EA, Medeiros Silva DC, Thabane L, Cruz Milagres A, Ferreira TS, et al. Effect of early treatment with metformin on risk of emergency care and hospitalization among patients with COVID-19: The TOGETHER randomized platform clinical trial. Lancet Reg Health Am (2022) 6:100142. doi: 10.1016/j.lana.2021.100142
Keywords: obesity, influenza, coronavirus disease 2019 (COVID-19), T cells, metabolism, metformin
Citation: Greene E and MacIver NJ (2022) Targeting T cell (oxidative) metabolism to improve immunity to viral infection in the context of obesity. Front. Immunol. 13:1025495. doi: 10.3389/fimmu.2022.1025495
Received: 23 August 2022; Accepted: 23 September 2022;
Published: 06 October 2022.
Edited by:
Caroline Richard, University of Alberta, CanadaReviewed by:
Isabelle Wolowczuk, INSERM U1019 Centre d’Infection et Immunité de Lille (CIIL), FranceCopyright © 2022 Greene and MacIver. This is an open-access article distributed under the terms of the Creative Commons Attribution License (CC BY). The use, distribution or reproduction in other forums is permitted, provided the original author(s) and the copyright owner(s) are credited and that the original publication in this journal is cited, in accordance with accepted academic practice. No use, distribution or reproduction is permitted which does not comply with these terms.
*Correspondence: Nancie J. MacIver, nancie_maciver@med.unc.edu