- 1Institute of Neuroanatomy, Medical Faculty, University of Bonn, Bonn, Germany
- 2Institute of Anatomy and Cell Biology, Friedrich-Alexander-Universität Erlangen-Nürnberg (FAU), Erlangen, Germany
- 3Institute of Neuropathology, University Hospitals Erlangen, Erlangen, Germany
- 4Department of Pathology, Amsterdam University Medical Center, Amsterdam, Netherlands
There has been a growing interest in the presence and role of B cell aggregates within the central nervous system of multiple sclerosis patients. However, very little is known about the expression profile of molecules associated with these aggregates and how they might be influencing aggregate development or persistence in the brain. The current study focuses on the effect of matrix metalloproteinase-3, which is associated with B cell aggregates in autopsied multiple sclerosis brain tissue, on B cells. Autopsied brain sections from multiple sclerosis cases and controls were screened for the presence of CD20+ B cell aggregates and expression of matrix metalloproteinase-3. Using flow cytometry, enzyme-linked immunosorbent assay and gene array as methods, in vitro studies were conducted using peripheral blood of healthy volunteers to demonstrate the effect of matrix metalloproteinase-3 on B cells. Autopsied brain sections from multiple sclerosis patients containing aggregates of B cells expressed a significantly higher amount of matrix metalloproteinase-3 compared to controls. In vitro experiments demonstrated that matrix metalloproteinase-3 dampened the overall activation status of B cells by downregulating CD69, CD80 and CD86. Furthermore, matrix metalloproteinase-3-treated B cells produced significantly lower amounts of interleukin-6. Gene array data confirmed that matrix metalloproteinase-3 altered the proliferation and survival profiles of B cells. Taken together, out data indicate a role for B cell modulatory properties of matrix metalloproteinase-3.
1 Introduction
Multiple sclerosis (MS) is a chronic neuroinflammatory demyelinating disease of the central nervous system (CNS) (1). The multifaceted roles of B cells in the pathogenesis of MS have gained increasing attention over the last years (2, 3), with one of the highlights being the clinical success story of peripheral blood B cell depletion with monoclonal anti-CD20 antibodies in relapsing-remitting (4, 5) and primary progressive MS patients (6, 7). Additional evidence for a key role of B cells in MS comes from neuropathological studies on autopsied brain sections (8, 9). On the one hand, studies that were performed more than a decade ago identified the presence of lymphoid-like B cell follicles in the inflamed meninges of up to 40% of MS patients with the secondary progressive disease form and observed a correlation with more severe clinical disease and cortical pathology (10–13). On the other hand, CD20+ B cell infiltrates were also found in primary progressive MS patients (14) and more recently, a prominent presence of B cells in lesions of patients with acute MS was demonstrated by post-mortem studies (15). While it has been suggested that these lymphoid-like B cell follicles may be drivers of disease progression (11–14, 16–19) the molecular signature associated with the presence of B cells within the CNS lesions and the triggers that convert B cells into pathogenic entities have not yet been clearly elucidated (20, 21).
To model the contribution of B cells in the human disease we immunized C57BL/6 mice with MP4 – a fusion protein consisting of the human isoform of myelin basic protein (MBP) and the three hydrophilic domains of proteolipid protein (PLP) (22). B cell aggregates resembling ectopic lymphoid-structures were predominantly observed in the cerebellum of MP4-immunized mice in the chronic stage of the disease (23, 24). To identify key molecules associated with or expressed within these follicles, gene expression profiles of B cell aggregates isolated from MP4-immunized mice were analyzed and led to the identification of matrix metalloproteinase (MMP)-3 (25), a member of the matrix metalloproteinase family. MMPs are pleiotropic proteases traditionally associated with the degradation and turnover of components of the extracellular matrix (ECM) (26). More recent evidence suggests that only 27% of MMP substrates are ECM or ECM-related proteins, while the majority of the known MMP substrates are non-ECM proteins including chemokines, cytokines, cell-surface receptors and proteins involved in immune signaling (27). An extension of these new findings is also a shift in the paradigm for MMP functions from being only destructive enzymes that are detrimental in inflammatory diseases to being regarded as cell signaling regulators and modulators of inflammatory and immune processes (28, 29).
In the context of neuroinflammation and MS, MMPs have been implicated both as positive and negative regulators of tissue injury (30–34). For instance, MMP-3 has been shown to degrade protein components that inhibit differentiation and maturation of oligodendrocytes (35) and to terminate inflammation within the CNS (36). Conversely, other reports have discussed the unfavorable role of MMP-3 as a driving factor for neuroinflammation and neurodegeneration (26, 37), including its association with increased blood-brain barrier (BBB) permeability (38–40).
To our knowledge, this study is the first to have addressed the relationship between MMP-3 and B cells. Based upon the preliminary data obtained from the MP4-induced experimental autoimmune encephalomyelitis (EAE) mouse model where gene expression of MMP-3 was spatially associated with the presence of B cell aggregates (25), here we analyzed the expression pattern of MMP-3 in MS brain tissue. Our data demonstrate a high level of MMP-3 expression especially in association with B cell aggregates. When incubating B cells of peripheral human blood with human recombinant MMP-3 (rMMP-3) we observed downregulated B cell activation and cytokine production, in particular of the pro-inflammatory cytokine interleukin-6 (IL-6). These data imply a favorable role of MMP-3 in polarizing B cells to a less pathogenic phenotype.
2 Materials and methods
2.1 Human brain tissue
Paraffin-embedded brain tissue sections of MS patients and control subjects were obtained from the Multiple Sclerosis and Parkinson’s Tissue Bank, Center for Brain Sciences, Imperial College London (approved by the Ethics Committee of the University of Würzburg, File 258/14) and the Department of Pathology, Amsterdam University Medical Center. Human brain tissue was obtained at autopsy using the rapid autopsy regimen of the Netherlands Brain Bank (co-ordinator Prof. I. Huitiga), Amsterdam, with approval of the Medical Ethical Committee of the Amsterdam UMC. All participants or next of kin had given informed consent for autopsy and use of tissues for research purposes. MS brain samples that were obtained from the Netherlands Brain Bank were selected from regions of interest (ROIs) after ex vivo MRI to detect lesions. Tissue blocks containing the ROI were cut in half and half fixed in 10% formalin and embedded in paraffin and the other half snap-frozen and stored in liquid nitrogen. General information and clinical details on the patient samples are listed in Table 1. 16 cases of MS and 15 controls were included in the study.
2.2 Klüver-Barrera staining
5 μm-thick paraffin sections were deparaffinized and subsequently placed in Luxol Fast Blue (Chroma) solution at room temperature overnight. The sections were washed with distilled water and incubated in a 0.1% lithium carbonate solution for 5 min. For differentiation, the tissue was exposed to 70% isopropanol for 30 sec and washed in distilled water. After incubation in 0.2% cresyl violet solution for 2 min, the slides were rinsed in distilled water and differentiated in 96% isopropanol for 2 min. Finally, the sections were dehydrated and mounted using entellan.
2.3 Immunohistochemistry
After deparaffinization of human brain sections, heat-induced antigen retrieval was performed in 10 mM sodium citrate buffer (pH 6.0). The following incubation steps were done in a humified chamber and slides were washed between every incubation. Sections were blocked with either 5% milk or 5% donkey serum in tris-buffered saline with 0.5% Tween-20 (TBS-T) (blocking buffer) at room temperature for 1 h. Primary antibodies were diluted in 0.5% blocking buffer and sections were incubated in primary antibody dilution at 4°C overnight. After washing the slides with TBS-T, the appropriate secondary antibodies diluted in 0.5% blocking buffer were applied to the sections at room temperature for 2 h in the dark. For double stainings, tissue sections were washed with TBS-T and incubated with the second primary antibody at room temperature for 3 h. The corresponding secondary antibodies were diluted as mentioned earlier and incubated at room temperature for 2 h. All sections were mounted using Fluoroshield mounting medium containing 4’,6’-diamidino-2-phenylindole (DAPI) (Abcam). Details on the primary and secondary antibodies are listed in Supplementary Table 1. Images were acquired using a Leica DM6 fluorescence microscope equipped with LAS X software at different magnifications as indicated in the figure legends.
2.4 Quantification of MMP-3 staining
Tile scans were done covering either white matter (WM) lesion or corresponding normal appearing white matter (NAWM) region of every tissue section from a total number of 16 MS patients. From the 15 controls tile scans covered the WM region. Subsequently, the LAS X software algorithm was used to choose the ROIs. The number of ROIs that were analyzed was relative to the area covered by the tile scan. This resulted in 3 – 25 individual ROIs/tile scan that were quantified. Care was taken that the number of ROIs was equal between WM lesion, NAWM and the corresponding control tissue WM region. All images were acquired and analyzed in a blinded fashion. Quantification was performed using Image J (NIH). To this end, a grid with a square size of 1.5 inches was generated and placed over the images. Every square containing MMP-3+ cells in each ROI was counted and mean values were calculated.
2.5 B cell isolation and cell culture
For in vitro B cell studies, blood was collected from n = 30 healthy controls (n = 13 males and n = 17 females) between the age of 22 and 35. Inclusion criteria were an age of at least 18, written informed consent and the absence of any diagnosed diseases, in particular the absence of autoimmune and neurological diseases. In addition, participants who had shown any signs of infection two weeks before the day of the blood collection were not included in the study. The study was performed pseudonymized and approved by the ethics committee of the University of Erlangen-Nürnberg, Germany (Files 185_18B and 74_18B). Approximately 30 mL of peripheral blood per person was collected using S-Monovette® tubes containing lithium heparin (Sarstedt). The blood sample was incubated with RosetteSep™ Human B cell enrichment cocktail (STEMCELL Technologies) and purified by Ficoll-Paque™ Plus (GE Healthcare) density centrifugation according to the manufacturer’s instructions. Isolated cells were counted and resuspended in complete B cell medium at a concentration of 150,000 cells/200 μL for all downstream applications. Complete B cell medium was prepared using Roswell Park Memorial Institute-1640 (RPMI-1640) medium (Sigma) containing 0.3 g/L L-glutamine, 10% fetal bovine serum (FBS) (ThermoFisher), 1% 100 IU/mL penicillin (Sigma) and 0.1 mg/mL streptomycin (Sigma) (P/S), 50 μM of β-mercaptoethanol (Sigma) and 10 mM HEPES. An aliquot of 450,000 freshly isolated B cells was separated for purity check by flow cytometry.
Purified B cells were cultured in the presence or absence of stimulation (15 ng/mL IL-2 (PeproTech) and 1 μg/mL synthetic toll-like receptor (TLR) 7/8 agonist R-848 (ENZO Life Sciences) in 96-well plates. B cells that were additionally stimulated with IL-2 and R-848 are referred to as pre-stimulated B cells and those without any stimulation as unstimulated B cells. While pre-stimulated B cells were incubated at 37°C and 5% CO2 for 24 h, unstimulated B cells were directly processed by adding active rMMP-3 (R&D Systems) or 4-aminophenylmercuric acetate (APMA) (Sigma). Every culture condition from every donor was plated, measured and analyzed in duplicates for all downstream applications.
2.6 Activation of rMMP-3
Several pro-MMPs, including pro-MMP-3, can be activated by mercurial compounds such as APMA (41). Accordingly, we used APMA to activate the pro-form of rMMP-3. rMMP-3 was activated in assay buffer (pH 7.5) at a concentration of 15 µg/mL using 1 mM APMA at 37°C for 24 h. Assay buffer was prepared using 50 mM Tris, 10 mM calcium chloride, 150 mM sodium chloride and 0.05% Brij-35. Activation of rMMP-3 was confirmed by Western blot (Figure S1).
2.7 Stimulation of B cells with active rMMP-3 or APMA
Active rMMP-3 at a titrated dose of 3 ng/well was added to pre-stimulated or un-stimulated B cells. Culturing of B cells in the presence of rMMP-3 was optimized to 20 h at 37°C and 5% CO2. APMA (1 µM/well) was used as a vehicle control for the different conditions.
2.8 Flow cytometry
2.8.1 Purity check of B cells
Following every B cell isolation, the purity of the enriched population was assessed by flow cytometry. Briefly, the isolated cell suspensions were incubated with BD Horizon™ Fixable Viability Stain 780 (FVS780) (BD Biosciences) at 4°C for 30 min in the dark. Cells were subsequently washed, centrifuged and incubated with anti-human CD19 and CD45 antibodies at 4°C in the dark for 30 min (dilutions are listed in Supplementary Table 1). After washing, cells were resuspended in an appropriate volume of FACSFlow™ (BD Biosciences) for sample measurement. Data were acquired on a CytoFLEX S flow cytometer (Beckman Coulter) equipped with CytExpert 2.2 software. After identification of CD45+ leukocytes, doublets and dead cells were excluded. Subsequently, gates were set on CD19+ B cells. Data analysis was performed with FlowJo software (version 10.8.0) (BD Biosciences).
2.8.2 B cell activation
To check the activation status of pre-stimulated or unstimulated B cells that had been treated with active human rMMP-3 or APMA for 20 h, the following cell surface markers were used: CD20, CD3, CD69, CD80 and CD86 (Supplementary Table 1). For live and dead cell discrimination, cells were stained with FVS780. Staining was performed as mentioned above. Details on the gating strategy used for the measurement of the B cell activation status are shown in Figure S2. Unstained samples, fluorescence minus one (FMO) (for CD3 staining) and isotype controls (for CD69, CD80 and CD86 staining) were also included.
2.8.3 Survival of B cells
To determine cell viability of pre-stimulated and unstimulated B cells after rMMP-3 or APMA treatment, gates were set on the leukocyte population and doublets removed. After identification of CD20+ B cells, CD3+ cells were excluded and viable B cells were defined as CD20+CD3- based on the FVS780 dye staining as mentioned above. The gating strategy is shown in Figure S3.
2.9 Antibody array and ELISA
To determine the secretion profile of cytokines by pre-stimulated B cells after treatment with rMMP-3 and APMA, a human cytokine antibody array kit with 23 targets (Abcam) was used. An equal volume of culture supernatant from rMMP-3- or APMA-treated B cells was used for the array and the assay was performed according to the manufacturer’s instructions. Based on our preliminary findings of the antibody array, quantitative analysis of IL-6 was done on B cell culture supernatant. IL-6 ELISA (ThermoFisher) was performed as per the manufacturer’s protocol.
2.10 RT2 profiler PCR array
Purified B cells were stimulated with R-848 and IL-2 prior to treatment and cultured as mentioned above with either active rMMP-3 or APMA. Total RNA was extracted from B cells using the RNeasy™ Mini kit (Qiagen) as per the manufacturer’s guidelines. In order to obtain a sufficient number of cells for the RNA extraction, B cells from 8 – 10 wells/condition were pooled together. After every extraction, the concentration and purity of the RNA yield was measured using a NanoPhotometer® (Implen). Reverse transcription of RNA to cDNA was done using the High Capacity cDNA Reverse Transcriptase kit (ThermoFisher) according to the protocol suggested by the manufacturer. The expression of 44 genes associated with B cell activation and B cell receptor signaling pathways were examined in duplicates, using a customised RT2 Profiler PCR array (Qiagen). Suitable controls including housekeeping genes and controls for genomic DNA contamination were included in the array. Relative quantification of mRNA levels was performed by real-time PCR using a Roche LightCycler® 480 instrument. The cycler conditions were set up following the manufacturer’s instructions. Analysis was performed using relative quantification, i.e., the gene expression profile of rMMP-3-treated B cells was determined relative to the vehicle control (APMA)-treated group. The values were normalized to the mean values of the housekeeping genes, glyceraldehyde-3-phosphate dehydrogenase (GAPDH) and transferrin receptor (TFRC).
2.11 Statistical analysis
Statistical analysis was perfomed using GraphPad Prism 8.0 (GraphPad Software Inc.). A Shapiro-Wilk normality test was used to test the Gaussian distribution of every data set. Differences between parametric groups were assessed using either a t test or a one-way ANOVA, while for non-parametric data sets, Wilcoxon test was used. The level of significance was set at 5%.
3 Results
3.1 MMP-3 expression is significantly upregulated in multiple sclerosis patients with B cell pathology
Immunohistochemical staining was conducted on tissue sections obtained from a cohort of 16 MS patients with a total number of 31 tissue blocks and 15 controls with one block per donor. First, MS patients were categorized into a “B cell” (n = 7), “T cell” (n = 6) or “No CD3/CD20 infiltration” (NI) (n = 3) group based on the presence or absence of CD20+ B cells and CD3+ T cells, respectively, confirmed by a CD20/CD3 double staining. As listed in Table 1, multiple blocks (1–5) were tested from patients that were categorized as “B cell positive” with at least one B cell infiltrate (counted as > 5 B cells) or B cell aggregate (counted as an aggregation of > 20 clustered B cells) in one of the blocks per patient. “T cell” patients had no B cells in the examined sections but showed pronounced CD3+ T cell infiltration. Representative images of a B cell aggregate in a “B cell” patient and a T cell infiltrate in a “T cell” patients are shown in Figure 1A. The expression pattern of MMP-3 was categorized into expression within the lesion or in the NAWM (Figure 1B), whereby lesions and NAWM were identified by Klüver-Barrera staining (Figure 1A). Although there was no difference in the expression of MMP-3 between a lesion and its corresponding NAWM, MMP-3 expression was significantly higher in the NAWM of patients in the “B cell” group compared to the “T cell” group (p < 0.001) and non-MS controls (p < 0.001), but not to the “NI” group (Figure 1C). Regarding the MMP-3 expression within the lesions, there was a significant difference between the “B cell” and the “T cell” group (p < 0.01) (Figure 1D). Figure 2A shows a representative image of the expression of MMP-3 associated with CD20+ B cell aggregates. GFAP+ astrocytes were identified as the primary source of MMP-3 in areas without CD20+ B cell aggregates, i.e., in lesion areas of both B cell (Figure 2B) and T cell patients (Figure 2C).
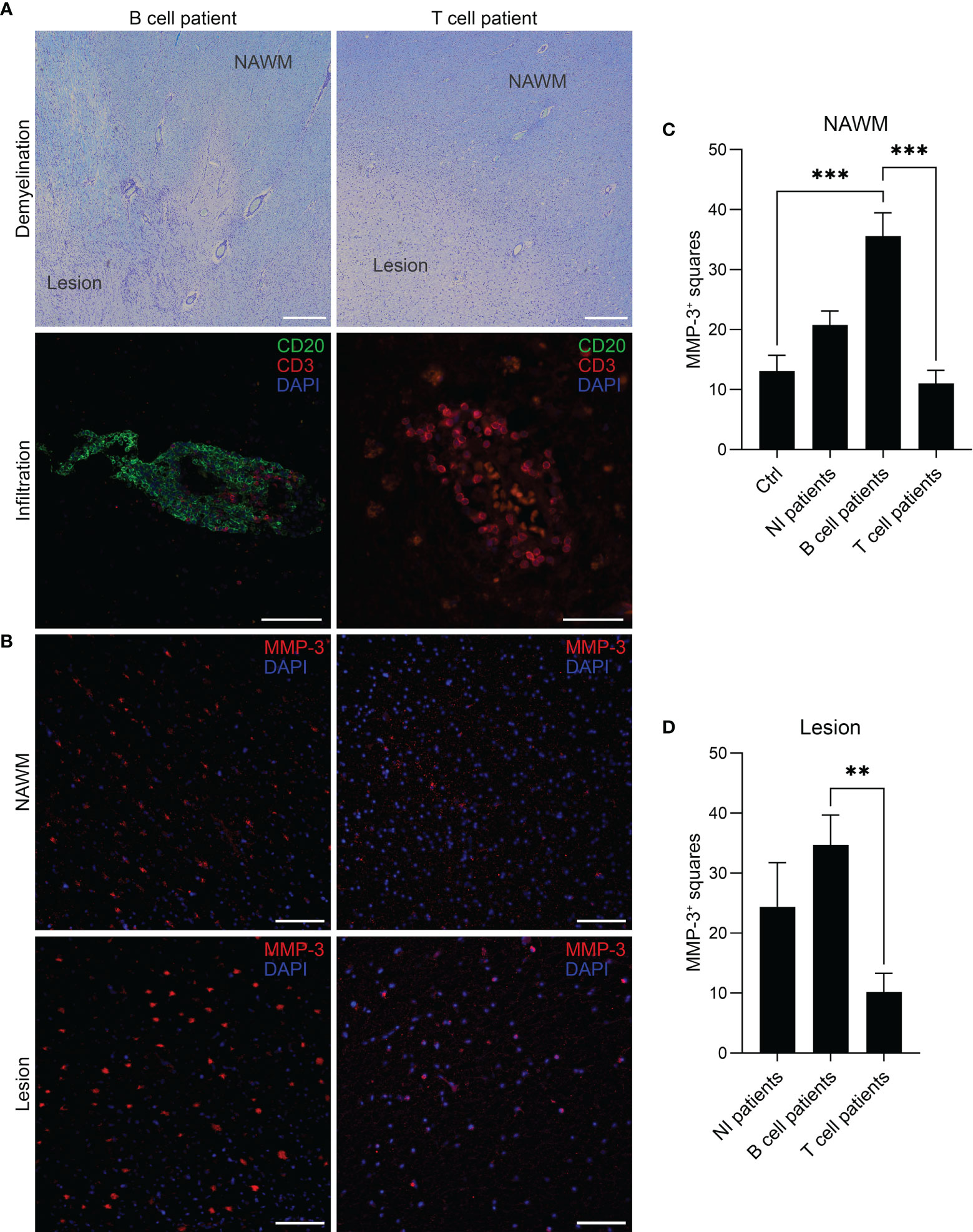
Figure 1 MMP-3 expression in MS brain tissue of different patient groups. (A) Representative images of a patient in the “B cell” and “T cell” group. Lesions and NAWM were determined by visualizing demyelinated and myelinated areas based on a Klüver-Barrera staining. Scale bars represent 500 µm. The B cell patient was characterized by the presence of both CD20+ B cells and CD3+ T cells, while the T cell patient showed infiltration of CD3+ T cells only. Scale bars represent 100 µm and 50 µm, respectively. (B) The images show MMP-3 expression in the same B cell and T cell patient (as shown in A) comparing lesion and NAWM. Scale bars represent 50 µm. (C, D) Quantification of MMP-3 expression in (C) NAWM and (D) lesion in different patient groups. The graph shows the number of squares containing MMP3+ cells by using an overlying grid. Bar graphs represent mean values + SEM. **p < 0.01, and ***p < 0.001; one-way ANOVA. NAWM, normal appearing white matter; NI, no CD3/CD20 infiltration.
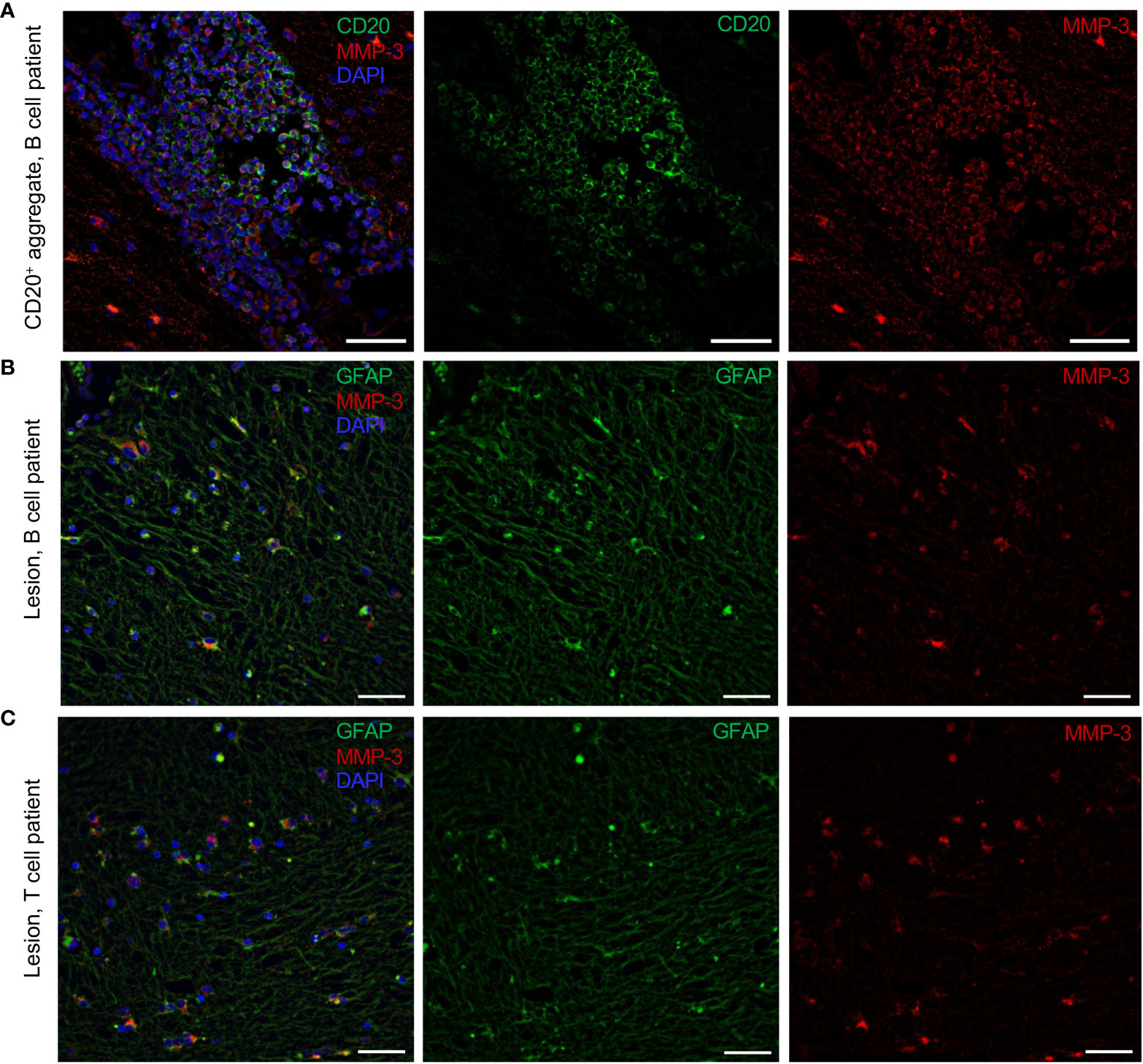
Figure 2 Cellular sources of MMP-3 expression in the MS brain. (A) Representative image of perivascular CD20+MMP-3+ B cells in MS brain sections. Parenchymal GFAP+MMP-3+ astrocytes in a (B) B cell patient and (C) T cell patient. Scale bars display 50 µm.
3.2 rMMP-3 downregulates the expression of activation and co-stimulatory markers on B cells
With the knowledge that MMP-3 is highly expressed in MS patients showing B cell pathology, the effect of MMP-3 on the activation status of B cells was determined using a panel of three pre-selected cell surface markers: CD69, which is an early surface activation marker (42), and CD80 and CD86 which are two co-stimulatory molecules that play an essential role in T cell activation (43).
Of note, while titrating the optimal APMA to MMP-3 ratio for the experiments, we noticed that an amount slightly higher than 1 µM APMA/150,000 cells/well was cytotoxic and reduced the viability of the B cells in culture. Furthermore, B cells treated with APMA alone showed alterations in their activation profile, in particular an increased expression of CD69 (Supplementary Table 2). Because APMA alone had an effect on the B cells, we studied the effect of rMMP on B cells relative to APMA for all the following experiments.
When unstimulated peripheral blood B cells from n = 4 – 6 healthy donors were treated with rMMP-3 for 20 h in culture, we did not observe any significant effect on the expression of the co-stimulatory molecules (Figure 3A). However, B cells from a set of n = 5 – 7 healthy donors that were first pre-stimulated with R-848 and IL-2 and then treated with rMMP-3 demonstrated a significantly lower expression of all three surface markers (CD69 p = 0.018; CD80 p = 0.034; CD86; p = 0.0004) (Figure 3B) compared to vehicle. Interestingly, MMP-3 significantly (p = 0.023) reduced cell viability of unstimulated B cells, while there was no significant difference between the rMMP-3-treated and control groups in pre-stimulated B cells (Figure 3A). Donors, whose rMMP-3-treated B cells showed a coefficient of variation (CV) higher than 20% between the technical replicates for any condition, were excluded from the analysis.
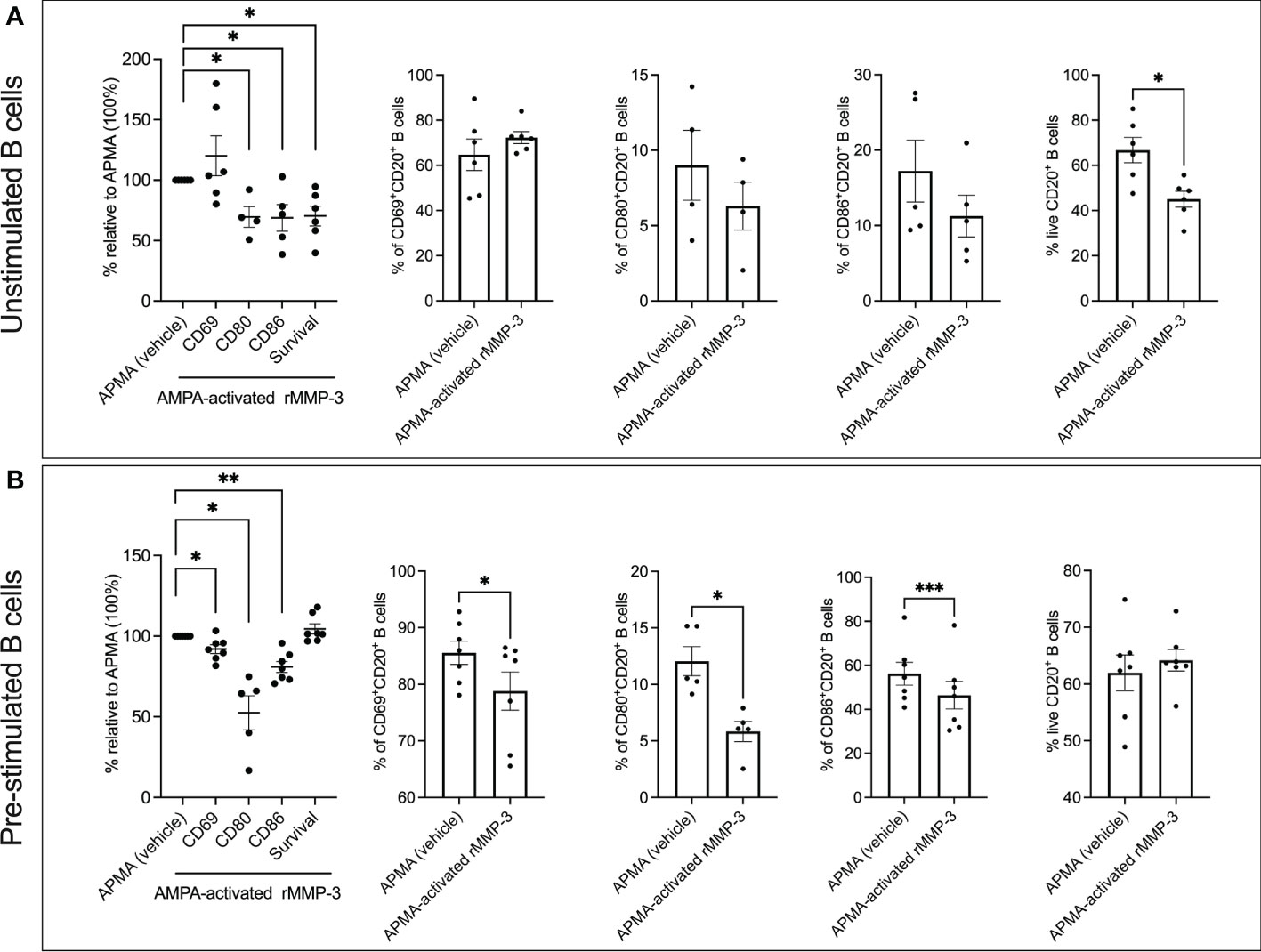
Figure 3 Analysis of activation markers and survival of B cells after MMP-3 stimulation. (A) Expression of CD69, CD80 and CD86 and viability of unstimulated (cells isolated from n = 4 – 6 healthy donors) B cells after treatment with APMA-activated rMMP-3 compared to APMA (vehicle) only. (B) Expression of CD69, CD80 and CD86 and viability of pre-stimulated (cells isolated from n = 5-7 healthy donors) B cells incubated with APMA-activated rMMP3 or APMA (vehicle) only. Relative expression of CD69, CD80 and CD86 and viability of either unstimulated (A) or pre-stimulated B cells (B) after treatment with rMMP-3 compared to vehicle is also shown. Graphs show mean values + SEM. *p < 0.05, **p < 0.01, ***p < 0.001; paired t test, Wilcoxon test. APMA, 4-aminophenylmercuric acetate.
3.3 B cells treated with rMMP-3 secrete significantly lower amounts of IL-6
To check if MMP-3 also had an effect on the cytokine profile of B cells, a dot blot antibody array was performed using the supernatants of B cells that had been pre-stimulated with R-848 and IL-2 (Figures 4A, B). A summary of the relative expression of the different cytokines in the rMMP-3-treated group compared to vehicle is included as Supplementary Table 3. The integrated density of the dot blot shows downregulation of the cytokines IL-6 and IL-8, for example, and an upregulation of tumor necrosis factor (TNF)-β from n = 1 donor. However, we specifically focused on the cytokine IL-6 (Figure 4C), which is a cytokine involved in immune responses and in chronic inflammation (44). The dot blot array was confirmed by ELISA and a significant difference between the vehicle- and rMMP-3-treated group in n = 9 healthy donors was observed (Figure 4D; p < 0.01).
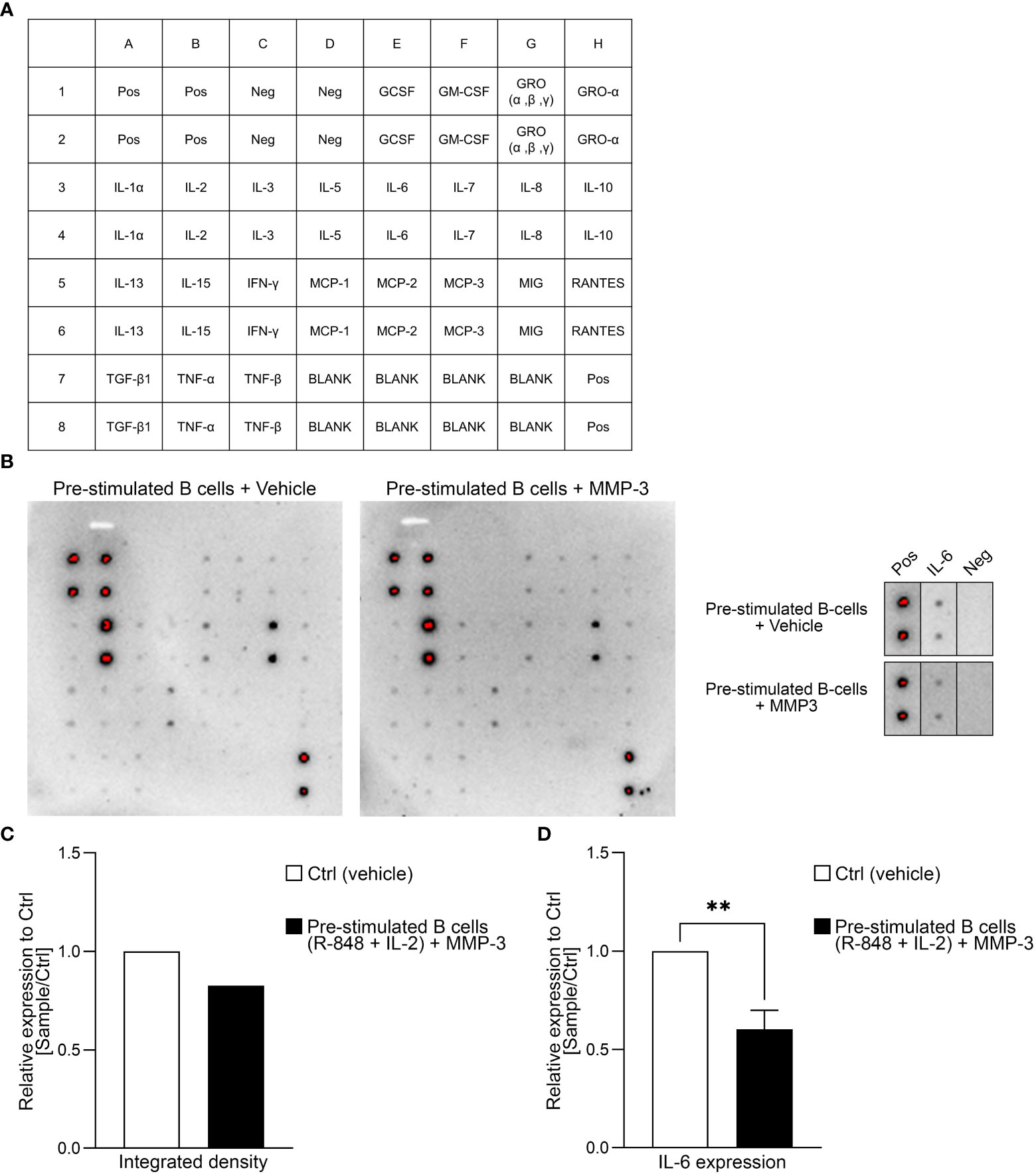
Figure 4 Cytokine profiling of MMP3-treated B cells. (A) Layout of the antibody array used for investigating cytokines. (B) The dot blot indicates the cytokine expression profile of pre-stimulated B cells after treatment with either MMP-3 or vehicle from one representative donor. Additionally, IL-6 production by B cells from the same donor is highlighted. (C) Relative amount of IL-6 production by pre-stimulated B cells treated with MMP-3 compared to vehicle was calculated by using integrated density from n = 1 donor, measured in duplicates. (D) Relative IL-6 production of pre-stimulated B cells (n = 9 healthy donors), measured by ELISA. Bars display mean values + SEM, **p < 0.01, paired t test. Pos, positive control; Neg, negative control.
3.4 rMMP-3 alters the activation, survival and proliferation profile of B cells
A customized gene expression array with 44 genes associated with B cell activation, maturation and differentiation pathways was used to study a more global influence of MMP-3 on B cells. As shown in Figure 5A, a differential gene expression pattern by rMMP-3-treated B cells was apparent on the individual level (n = 6 healthy donors). The heat map (Figure 5A and Supplementary Table 4) summarizes the log2fold change comparing rMMP-3-treated B cells to the vehicle group. A total of 13 of these genes (Figure 5B) were significantly downregulated (p < 0.05) by B cells cultured in the presence of rMMP-3 compared to vehicle-treated cells, indicating that MMP-3 can modulate the B cell response at the mRNA level.
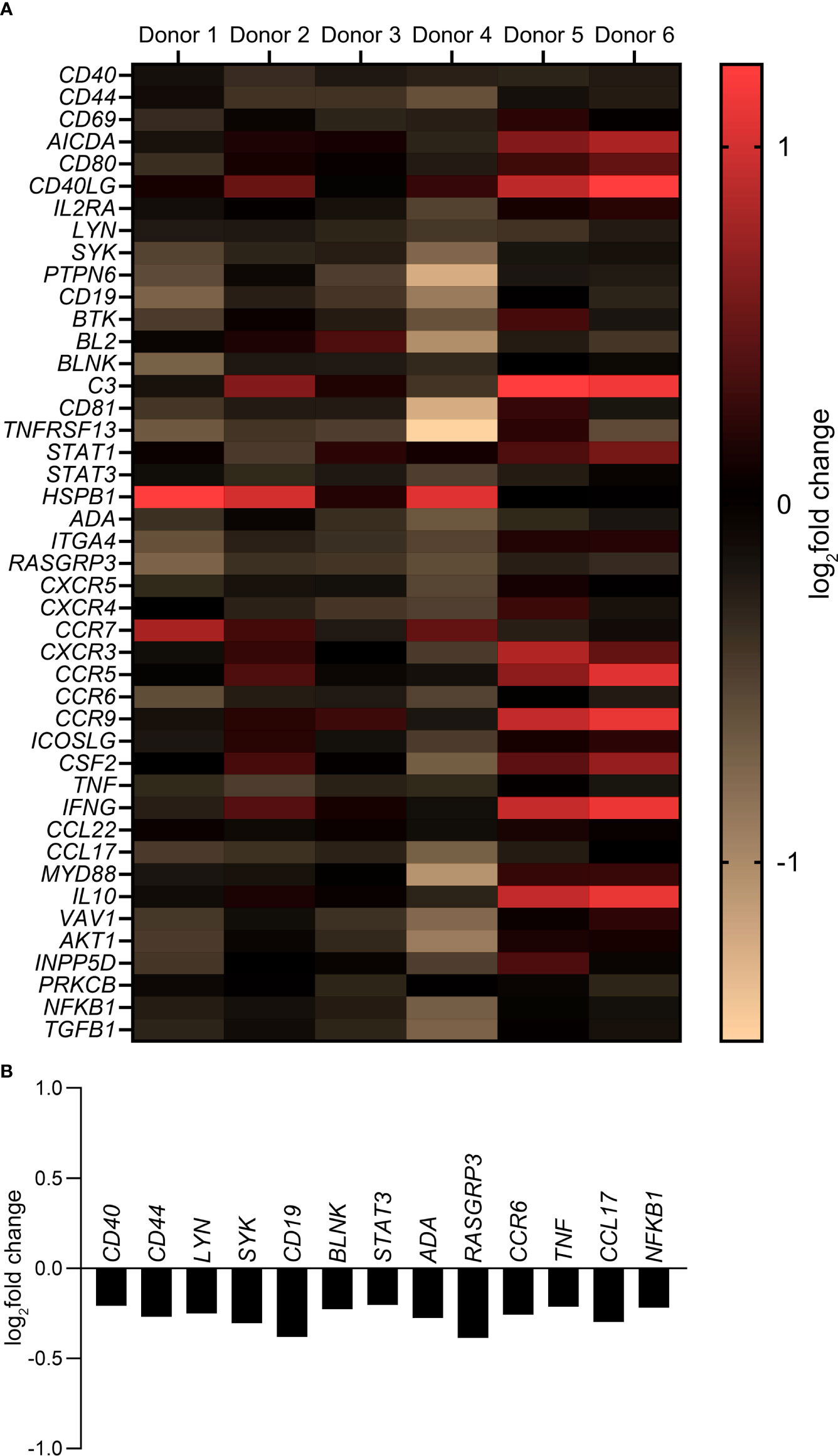
Figure 5 Gene expression profile of B cells after treatment with MMP-3. (A) Expression of B cell-related genes by pre-stimulated B cells treated with activated rMMP-3 relative to APMA in different donors (n = 6). B cells from 8 – 10 wells/condition/donor were pooled and measurements were done in duplicates. Values show the log2fold change. (B) The graph represents the log2fold change of significantly downregulated genes after MMP-3 treatment compared to the vehicle-treated group (p < 0.05) using paired t test.
4 Discussion
In the present study we demonstrate that MMP-3 has the potential to modulate B cell activity not just by downregulating cell surface antigens associated with B cell activation but also by reducing expression of genes involved in B cell activation, proliferation and differentiation pathways. Furthermore, MMP-3 had an effect on IL-6 production by B cells indicating a potential role in polarizing B cells towards a more anti-inflammatory phenotype.
The first evidence of a relation between MMP-3 and B cells was demonstrated by a higher expression of MMP-3 in MS patients with CNS B cell pathology compared to those without an obvious B cell pathology. Given that lymphoid-like B cell follicles are primarily present in the progressive phase of MS (10, 14, 45), we specifically studied such a cohort of patients. Indeed, our autopsy samples provide a snapshot rather than a complete cellular overview of the brain, however, our data focuses on an increased expression of MMP-3 spatially associated with the presence of B cells. Furthermore, a few CD20+ B cells also co-expressed MMP-3 within B cell aggregates. Future experiments using B cell subtype-specific markers would clarify the identity of these MMP3/CD20 double positive B cells and the functional relevance of this cell population within the aggregates.
In the following sets of experiments, we have demonstrated that rMMP-3 dampens the activation status of B cells and therefore also plays a critical role in the modulation of T cell activation (46). In MS, memory B cells are considered to be the main pathogenic B cell subtype (47–49). In this context, it has been shown that memory B cells can function as potent antigen presenting cells (APCs) (50) with proinflammatory tendencies including their capacity to express high levels of proinflammatory cytokines including GM-CSF, TNF-α and lymphotoxin (51). Class-switched memory B cells not only populate the cerebrospinal fluid (CSF) of MS patients (52) but are also found within the brain parenchyma (53) with studies demonstrating that the increased accumulation of class-switched memory B cells in the CSF also correlates with the intrathecal synthesis of IgG (54–56).
Accordingly, we investigated the effects of rMMP-3 on B cells stimulated with R-848 and IL-2 as activators that trigger efficient and selective proliferation and differentiation of memory B cells (57). Additionally, R-848 is known to be a potent B cell activator (58) that can induce the synthesis of increased amounts of cytokines and antibody production in B cells (59), while IL-2 promotes the proliferation of activated B cells (60–62). We focused on the effect of MMP-3 on the activation status of B cells based on the rationale that B cells found within the CNS/CSF compartment in progressive MS patients are found to be of an activated phenotype (8, 15, 49, 63). Our data indicate that MMP-3-mediated downregulation of activation markers (i.e., of CD69, CD80 and CD86) was significant in B cells pre-stimulated with R-848 and IL-2. To understand why there is a pronounced effect of MMP-3 on pre-stimulated (memory) B cells, a more detailed flow cytometric characterization needs to be performed in future studies to allow us to confirm the nature of the effect of MMP-3 on the different subtypes of B cells in greater detail.
CD69 has been identified as a B cell marker associated with MS (64) whereas CD80 and CD86 are costimulatory molecules that are involved in immune regulation and B cells expressing CD80/CD86 have been shown to drive pathogenesis in autoimmunity (65, 66). While CD86 initiates immune reactions, CD80 is known to be the more important costimulatory molecule during chronic inflammation (67). Both CD80 and CD86 are over-expressed on B cells in patients with MS (68, 69). On the one hand, CD80 expression by B cells was significantly increased in the CSF of stable relapsing-remitting MS patients (70) and on the other hand, this increase was linked to disease exacerbations (71). Furthermore, it was suggested that an increase in CD80 on a subpopulation of activated memory B cells may be central to the development of autoimmunity (72, 73). Our data therefore indicate a therapeutic potential of the interaction between MMP-3 and B cells where MMP-3 dampens the overall activation status of B cells via modulation of the co-stimulatory molecules CD80/CD86.
Another effect of MMP-3 on B cells was the significant decrease in the production of IL-6, which is a proinflammatory multifunctional cytokine that promotes T-helper cell differentiation (74, 75). The therapeutic benefit of neutralizing the effects of IL-6 has already been suggested for several autoimmune diseases including systemic lupus erythematosus (SLE) and rheumatoid arthritis (RA) (76). Furthermore, a pathogenic role of IL-6 producing B cells in MS has been described (77). However, future experiments should also study the effect of MMP-3 on other B cell-secreting cytokines using a multiplex platform.
Another result of our study was the effect of MMP-3 on B cells at the mRNA level. CD40, a co-stimulatory receptor protein that is required for the generation of germinal centers and isotype switching (78), was significantly downregulated in B cells treated with MMP-3 compared to vehicle. Other genes that were significantly affected by MMP-3 included LYN (79) and SYK (80) that are known to be involved in the survival of memory B cells, in addition to STAT3 (81)/BLNK (82) and RASGRP3 (83) which play a crucial role in B cell activation and maturation. The expression level of CD44, which is a cell surface glycoprotein involved in a number of signal transduction events (84), was also reduced in response to MMP-3 stimulation.
It is known that through their proteolytic activity, MMPs play a crucial role in regulating signaling pathways that control cell activation and survival. However, in this case, precisely which cellular pathways are induced in B cells in a MMP-3-dependent manner or the nature of this signaling cascade remains unknown. Our findings call for the need to re-examine or discover novel substrates for MMP-3 which may include cell-associated molecules like chemokines and cell receptors expressed by B cells (85) that may explain how MMP-3 modulates B cell activity. Additionally, investigating the effect of MMP-3 on the activation status of B cells from MS patients who have not undergone any treatment with immunomodulatory drugs would strengthen the relevance of our preliminary findings.
Data availability statement
The original contributions presented in the study are included in the article/Supplementary Material. Further inquiries can be directed to the corresponding author.
Ethics statement
The studies involving human participants were reviewed and approved by the Department of Pathology, Amsterdam University Medical Center, by the Ethics Committee of the University of Würzburg and the University of Erlangen-Nuremberg, Germany. All patients/participants provided their written informed consent to participate in this study.
Author contributions
RC and VS participated in the study design, performed all experiments, analyzed and interpreted the data and wrote the manuscript. SJ, MM and SA assisted in acquiring the human samples. SA revised the manuscript for intellectual content. SK was responsible for conceptualization, funding acquisition, study design and supervision and revised the manuscript. All authors contributed to the article and approved the submitted version.
Funding
This research was funded by a research grant from the DFG (KU2760/4-1) to Stefanie Kuerten.
Acknowledgments
We would like to thank Stefanie Schliwa, Benedikt Kleinsasser, Julia Lukaszczyk, Birgit Blanck and Stephanie Link for providing excellent technical assistance. We would also like to thank Benjamin Odermatt and Nina Ishorst for their help with analyzing the gene array data. Finally, we would also like to extend our appreciation to Christopher M. Overall and Lydia Sorokin for sharing their expertise on MMPs.
Conflict of interest
The authors declare that the research was conducted in the absence of any commercial or financial relationships that could be construed as a potential conflict of interest.
Publisher’s note
All claims expressed in this article are solely those of the authors and do not necessarily represent those of their affiliated organizations, or those of the publisher, the editors and the reviewers. Any product that may be evaluated in this article, or claim that may be made by its manufacturer, is not guaranteed or endorsed by the publisher.
Supplementary material
The Supplementary Material for this article can be found online at: https://www.frontiersin.org/articles/10.3389/fimmu.2022.1025377/full#supplementary-material
References
1. Filippi M, Bar-Or A, Piehl F, Preziosa P, Solari A, Vukusic S, et al. Multiple sclerosis. Nat Rev Dis Primers (2018) 4(43). doi: 10.1038/s41572-018-0041-4
2. Wang A, Rojas O, Lee D, Gommerman JL. Regulation of neuroinflammation by B cells and plasma cells. Immunol Rev (2021) 299(1):45–60. doi: 10.1111/imr.12929
3. Comi G, Bar-Or A, Lassmann H, Uccelli A, Hartung HP, Montalban X, et al. Role of B cells in multiple sclerosis and related disorders. Ann Neurol (2021) 89(1):13–23. doi: 10.1002/ana.25927
4. Hauser SL, Waubant E, Arnold DL, Vollmer T, Antel J, Fox RJ, et al. B-cell depletion with rituximab in relapsing-remitting multiple sclerosis. N Engl J Med (2008) 358(7):676–88. doi: 10.1056/NEJMoa0706383
5. Salzer J, Svenningsson R, Alping P, Novakova L, Björk A, Fink K, et al. Rituximab in multiple sclerosis: A retrospective observational study on safety and efficacy. Neurology (2016) 87(20):2074–81. doi: 10.1212/WNL.0000000000003331
6. Martin R, Sospedra M, Rosito M, Engelhardt B. Current multiple sclerosis treatments have improved our understanding of MS autoimmune pathogenesis. Eur J Immunol (2016) 46(9):2078–90. doi: 10.1002/eji.201646485
7. Montalban X, Hauser SL, Kappos L, Arnold DL, Bar-Or A, Comi G, et al. Ocrelizumab versus placebo in primary progressive multiple sclerosis. N Engl J Med (2017) 376(3):209–20. doi: 10.1056/NEJMoa1606468
8. Holloman JP, Axtell RC, Monson NL, Wu GF. The role of B cells in primary progressive multiple sclerosis. Front Neurol (2021) 12:680581. doi: 10.3389/fneur.2021.680581
9. Cencioni MT, Mattoscio M, Magliozzi R, Bar-Or A, Muraro PA. B cells in multiple sclerosis - from targeted depletion to immune reconstitution therapies. Nat Rev Neurol (2021) 17(7):399–414. doi: 10.1038/s41582-021-00498-5
10. Serafini B, Rosicarelli B, Magliozzi R, Stigliano E, Aloisi F. Detection of ectopic B-cell follicles with germinal centers in the meninges of patients with secondary progressive multiple sclerosis. Brain Pathol (2004) 14(2):164–74. doi: 10.1111/j.1750-3639.2004.tb00049.x
11. Howell OW, Reeves CA, Nicholas R, Carassiti D, Radotra B, Gentleman SM, et al. Meningeal inflammation is widespread and linked to cortical pathology in multiple sclerosis. Brain (2011) 134(9):2755–71. doi: 10.1093/brain/awr182
12. Magliozzi R, Howell O, Vora A, Serafini B, Nicholas R, Puopolo M, et al. Meningeal b-cell follicles in secondary progressive multiple sclerosis associate with early onset of disease and severe cortical pathology. Brain (2007) 130(4):1089–104. doi: 10.1093/brain/awm038
13. Magliozzi R, Howell OW, Reeves C, Roncaroli F, Nicholas R, Serafini B, et al. A gradient of neuronal loss and meningeal inflammation in multiple sclerosis. Ann Neurol (2010) 68(4):477–93. doi: 10.1002/ana.22230
14. Choi SR, Howell OW, Carassiti D, Magliozzi R, Gveric D, Muraro PA, et al. Meningeal inflammation plays a role in the pathology of primary progressive multiple sclerosis. Brain (2012) 135(10):2925–37. doi: 10.1093/brain/aws189
15. Machado-Santos J, Saji E, Tröscher AR, Paunovic M, Liblau R, Gabriely G, et al. The compartmentalized inflammatory response in the multiple sclerosis brain is composed of tissue-resident CD8+ T lymphocytes and B cells. Brain (2018) 141(7):2066–82. doi: 10.1093/brain/awy151
16. Reali C, Magliozzi R, Roncaroli F, Nicholas R, Howell OW, Reynolds R. B cell rich meningeal inflammation associates with increased spinal cord pathology in multiple sclerosis. Brain Pathol (2020) 30(4):779–93. doi: 10.1111/bpa.12841
17. Muñoz U, Sebal C, Escudero E, Esiri M, Tzartos J, Sloan C, et al. Main role of antibodies in demyelination and axonal damage in multiple sclerosis. Cell Mol Neurobiol (2021) 42(6):1809–27. doi: 10.1007/s10571-021-01059-6
18. Howell OW, Schulz-Trieglaff EK, Carassiti D, Gentleman SM, Nicholas R, Roncaroli F, et al. Extensive grey matter pathology in the cerebellum in multiple sclerosis is linked to inflammation in the subarachnoid space. Neuropathol Appl Neurobiol (2015) 41(6):798–813. doi: 10.1111/nan.12199
19. Lovato L, Willis SN, Rodig SJ, Caron T, Almendinger SE, Howell OW, et al. Related B cell clones populate the meninges and parenchyma of patients with multiple sclerosis. Brain (2011) 134(2):534–41. doi: 10.1093/brain/awq350
20. Jain RW. Yong VW. B cells in central nervous system disease: Diversity, locations and pathophysiology. Nat Rev Immunol (2021) 2:513–24. doi: 10.1038/s41577-021-00652-6
21. Chunder R, Schropp V, Kuerten S. B cells in multiple sclerosis and virus-induced neuroinflammation. Front Neurol (2020) 11:591894. doi: 10.3389/fneur.2020.591894
22. Kuerten S, Kostova-Bales DA, Frenzel LP, Tigno JT, Tary-Lehmann M, Angelov DN, et al. MP4- and MOG:35-55-induced EAE in C57BL/6 mice differentially targets brain, spinal cord and cerebellum. J Neuroimmunol. (2007) 189(1-2):31–40. doi: 10.1016/j.jneuroim.2007.06.016
23. Kuerten S, Javeri S, Tary-Lehmann M, Lehmann PV, Angelov DN. Fundamental differences in the dynamics of CNS lesion development and composition in MP4- and MOG peptide 35-55-induced experimental autoimmune encephalomyelitis. Clin Immunol (2008) 129(2):256–67. doi: 10.1016/j.clim.2008.07.016
24. Kuerten S, Schickel A, Kerkloh C, Recks MS, Addicks K, Ruddle NH, et al. Tertiary lymphoid organ development coincides with determinant spreading of the myelin-specific T cell response. Acta Neuropathol. (2012) 124(6):861–73. doi: 10.1007/s00401-012-1023-3
25. Schropp V, Rohde J, Rovituso DM, Jabari S, Bharti R, Kuerten S. Contribution of LTi and TH17 cells to B cell aggregate formation in the central nervous system in a mouse model of multiple sclerosis. J Neuroinflammation. (2019) 16(1):111. doi: 10.1186/s12974-019-1500-x
26. Behl T, Kaur G, Sehgal A, Bhradwaj S, Singh S, Buhas C, et al. Multifaceted role of matrix metalloproteinases in neurodegenerative diseases: Pathophysiological and therapeutic perspectives. Int J Mol Sci (2021) 22(3):1413. doi: 10.3390/ijms22031413
27. Chopra S, Overall CM, Dufour A. Matrix metalloproteinases in the CNS: interferons get nervous. Cell Mol Life Sci (2019) 76(16):3083–95. doi: 10.1007/s00018-019-03171-9
28. Rodríguez D, Morrison CJ, Overall CM. Matrix metalloproteinases: What do they not do? new substrates and biological roles identified by murine models and proteomics. Biochim Biophys Acta (2010) 1803(1):39–54. doi: 10.1016/j.bbamcr.2009.09.015
29. Morrison CJ, Butler GS, Rodríguez D, Overall CM. Matrix metalloproteinase proteomics: substrates, targets, and therapy. Curr Opin Cell Biol (2009) 21(5):645–53. doi: 10.1016/j.ceb.2009.06.006
30. Hannocks MJ, Zhang X, Gerwien H, Chashchina A, Burmeister M, Korpos E, et al. The gelatinases, MMP-2 and MMP-9, as fine tuners of neuroinflammatory processes. Matrix Biol (2019) 75-76:102–13. doi: 10.1016/j.matbio.2017.11.007
31. Boziki M, Grigoriadis N. An update on the role of matrix metalloproteinases in the pathogenesis of multiple sclerosis. Med Chem (2018) 14(2):155–69. doi: 10.2174/1573406413666170906122803
32. Mirshafiey A, Asghari B, Ghalamfarsa G, Jadidi-Niaragh F, Azizi G. The significance of matrix metalloproteinases in the immunopathogenesis and treatment of multiple sclerosis. Sultan Qaboos Univ Med J (2014) 14(1):e13–25. doi: 10.12816/0003332
33. Lee EJ, Han JE, Woo MS, Shin JA, Park EM, Kang JL, et al. Matrix metalloproteinase-8 plays a pivotal role in neuroinflammation by modulating TNF-α activation. J Immunol (2014) 193(5):2384–93. doi: 10.4049/jimmunol.1303240
34. Muri L, Leppert D, Grandgirard D, Leib SL. MMPs and ADAMs in neurological infectious diseases and multiple sclerosis. Cell Mol Life Sci (2019) 76(16):3097–116. doi: 10.1007/s00018-019-03174-6
35. de Jong JM, Wang P, Oomkens M, Baron W. Remodeling of the interstitial extracellular matrix in white matter multiple sclerosis lesions: Implications for remyelination (failure). J Neurosci Res (2020) 98(7):1370–97. doi: 10.1002/jnr.24582
36. Yong V, Power C, Forsyth P, Edwards DR. Metalloproteinases in biology and pathology of the nervous system. Nat Rev Neurosci (2001) 2:502–11. doi: 10.1038/35081571
37. Rempe RG, Hartz AMS, Bauer B. Matrix metalloproteinases in the brain and blood-brain barrier: Versatile breakers and makers. J Cereb Blood Flow Metab (2016) 36(9):1481–507. doi: 10.1177/0271678X16655551
38. Ljubisavljevic S, Stojanovic I, Basic J, Vojinovic S, Stojanov D, Djordjevic G, et al. The role of matrix metalloproteinase 3 and 9 in the pathogenesis of acute neuroinflammation. Implications Dis modifying Ther J Mol Neurosci (2015) 56(4):840–7. doi: 10.1007/s12031-015-0521-x
39. Kim EM, Hwang O. Role of matrix metalloproteinase-3 in neurodegeneration. J Neurochem (2011) 116(1):22–32. doi: 10.1111/j.1471-4159.2010.07082.x
40. Gurney KJ, Estrada EY, Rosenberg GA. Blood-brain barrier disruption by stromelysin-1 facilitates neutrophil infiltration in neuroinflammation. Neurobiol Dis (2006) 23(1):87–96. doi: 10.1016/j.nbd.2006.02.006
41. Galazka G, Windsor LJ, Birkedal-Hansen H, Engler JA. APMA (4-aminophenylmercuric acetate) activation of stromelysin-1 involves protein interactions in addition to those with cysteine-75 in the propeptide. Biochemistry (1996) 35(34):11221–7. doi: 10.1021/bi960618e
42. Ziegler SF, Ramsdell F, Alderson MR. The activation antigen CD69. Stem Cells (1994) 12(5):456–65. doi: 10.1002/stem.5530120502
43. Lenschow DJ, Walunas TL, Bluestone JA. CD28/B7 system of T cell costimulation. Annu Rev Immunol (1996) 14:233–58. doi: 10.1146/annurev.immunol.14.1.233
44. Tanaka T, Narazaki M, Kishimoto T. IL-6 in inflammation, immunity, and disease. Cold Spring Harb Perspect Biol (2014) 6(10):a016295. doi: 10.1101/cshperspect.a016295
45. Moccia M, Haider L, Eshaghi A, van der Pavert SHP, Morra VB, Patel A, et al. B cells in the CNS at postmortem are associated with worse outcome and cell types in multiple sclerosis. Neurol Neuroimmunol Neuroinflamm. (2022) 9(1):e1108. doi: 10.1212/NXI.0000000000001108
46. Suvas S, Singh V, Sahdev S, Vohra H, Agrewala JN. Distinct role of CD80 and CD86 in the regulation of the activation of B cell and B cell lymphoma. J Biol Chem (2002) 277(10):7766–75. doi: 10.1074/jbc.M105902200
47. Claes N, Fraussen J, Stinissen P, Hupperts R, Somers V. B cells are multifunctional players in multiple sclerosis pathogenesis: Insights from therapeutic interventions. Front Immunol (2015) 6:642. doi: 10.3389/fimmu.2015.00642
48. Baker D, Marta M, Pryce G, Giovannoni G, Schmierer K. Memory B cells are major targets for effective immunotherapy in relapsing multiple sclerosis. EBioMedicine (2017) 16:41–50. doi: 10.1016/j.ebiom.2017.01.042
49. Lehmann-Horn K, Kronsbein HC, Weber MS. Targeting B cells in the treatment of multiple sclerosis: Recent advances and remaining challenges. Ther Adv Neurol Disord (2013) 6(3):161–73. doi: 10.1177/1756285612474333
50. Harp CT, Ireland S, Davis LS, Remington G, Cassidy B, Craven PD, et al. Memory B cells from a subset of treatment-naïve relapsing-remitting multiple sclerosis patients elicit CD4(+) T-cell proliferation and IFN-γ production in response to myelin basic protein and myelin oligodendrocyte glycoprotein. Eur J Immunol (2010) 40(10):2942–56. doi: 10.1002/eji.201040516
51. Duddy M, Niino M, Adatia F, Hebert S, Freedman M, Atkins H, et al. Distinct effector cytokine profiles of memory and naive human B cell subsets and implication in multiple sclerosis. J Immunol (2007) 178(10):6092–9. doi: 10.4049/jimmunol.178.10.6092
52. Michel L, Touil H, Pikor NB, Gommerman JL, Prat A, Bar-Or A. B cells in the multiple sclerosis central nervous system: Trafficking and contribution to CNS-compartmentalized inflammation. Front Immunol (2015) 6:636. doi: 10.3389/fimmu.2015.00636
53. DiSano KD, Gilli F, Pachner AR. Memory B cells in multiple sclerosis: Emerging players in disease pathogenesis. Front Immunol (2021) 12:676686. doi: 10.3389/fimmu.2021.676686
54. Cepok S, Rosche B, Grummel V, Vogel F, Zhou D, Sayn J, et al. Short-lived plasma blasts are the main B cell effector subset during the course of multiple sclerosis. Brain (2005) 128(7):1667–76. doi: 10.1093/brain/awh486
55. Cepok S, von Geldern G, Grummel V, Hochgesand S, Celik H, Hartung HP, et al. Accumulation of class switched IgD-IgM- memory B cells in the cerebrospinal fluid during neuroinflammation. J Neuroimmunol. (2006) 180:33–9. doi: 10.1016/j.jneuroim.2006.06.031
56. Obermeier B, Mentele R, Malotka J, Kellermann J, Kümpfel T, Wekerle H, et al. Matching of oligoclonal immunoglobulin transcriptomes and proteomes of cerebrospinal fluid in multiple sclerosis. Nat Med (2008) 14:688–93. doi: 10.1038/nm1714
57. Pinna D, Corti D, Jarrossay D, Sallusto F, Lanzavecchia A. Clonal dissection of the human memory b-cell repertoire following infection and vaccination. Eur J Immunol (2009) 39(5):1260–70. doi: 10.1002/eji.200839129
58. Bishop GA, Hsing Y, Hostager BS, Jalukar SV, Ramirez LM, Tomai MA. Molecular mechanisms of b lymphocyte activation by the immune response modifier r-848. J Immunol (2000) 165(10):5552–7. doi: 10.4049/jimmunol.165.10.5552
59. Poovassery JS, Vanden Bush TJ, Bishop GA. Antigen receptor signals rescue B cells from TLR tolerance. J Immunol (2009) 183(5):2974–83. doi: 10.4049/jimmunol.0900495
60. Mingari MC, Gerosa F, Carra G, Accolla RS, Moretta A, Zubler RH, et al. Human interleukin-2 promotes proliferation of activated B cells via surface receptors similar to those of activated T cells. Nature (1984) 312(5995):641–3. doi: 10.1038/312641a0
61. Hipp N, Symington H, Pastoret C, Caron G, Monvoisin C, Tarte K, et al. IL-2 imprints human naive B cell fate towards plasma cell through ERK/ELK1-mediated BACH2 repression. Nat Commun (2017) 8(1):1443. doi: 10.1038/s41467-017-01475-7
62. Lagoo A, Tseng CK, Sell S. Molecular signals in B cell activation. II. IL-2-mediated signals are required in late G1 for transition to s phase after ionomycin and PMA treatment. Cell Immunol (1990) 127(2):497–505. doi: 10.1016/0008-8749(90)90149-l
63. Romme Christensen J, Börnsen L, Ratzer R, Piehl F, Khademi M, Olsson T, et al. Systemic inflammation in progressive multiple sclerosis involves follicular T-helper, Th17- and activated b-cells and correlates with progression. PloS One (2013) 8(3):e57820. doi: 10.1371/journal.pone.0057820
64. Jogansson D, Rauld C, Roux J, Regairaz C, Galli E, Callegari I, et al. Mass cytometry of CSF identifies an MS-associated b-cell population. Neurol Neuroimmunol Neuroinflamm. (2021) 8(2):e943. doi: 10.1212/NXI.0000000000000943
65. Menezes SM, Decanine D, Brassat D, Khouri R, Schnitman SV, Kruschewsky R, et al. CD80+ and CD86+ B cells as biomarkers and possible therapeutic targets in HTLV-1 associated myelopathy/tropical spastic paraparesis and multiple sclerosis. J Neuroinflammation. (2014) 11:18. doi: 10.1186/1742-2094-11-18
66. Ramgolam VS, Sha Y, Marcus KL, Choudhary N, Troiani L, Chopra M, et al. B cells as a therapeutic target for IFN-β in relapsing-remitting multiple sclerosis. J Immunol (2011) 186(7):4518–26. doi: 10.4049/jimmunol.1000271
67. Racke MK, Scott DE, Quigley L, Gray GS, Abe R, June CH, et al. Distinct roles for B7-1 (CD-80) and B7-2 (CD-86) in the initiation of experimental allergic encephalomyelitis. J Clin Invest. (1995) 96(5):2195–203. doi: 10.1172/JCI118274
68. Aung LL, Balashov KE. Decreased dicer expression is linked to increased expression of co-stimulatory molecule CD80 on B cells in multiple sclerosis. Mult Scler. (2015) 21(9):1131–8. doi: 10.1177/1352458514560923
69. Fraussen J, Claes N, Van Wijmeersch B, van Horssen J, Stinissen P, Hupperts R, et al. B cells of multiple sclerosis patients induce autoreactive proinflammatory T cell responses. Clin Immunol (2016) 173:124–32. doi: 10.1016/j.clim.2016.10.001
70. Svenningsson A, Dotevall L, Stemme S, Andersen O. Increased expression of B7-1 costimulatory molecule on cerebrospinal fluid cells of patients with multiple sclerosis and infectious central nervous system disease. J Neuroimmunol. (1997) 75(1-2):59–68. doi: 10.1016/s0165-5728(96)00234-2
71. Genç K, Dona DL, Reder AT. Increased CD80(+) B cells in active multiple sclerosis and reversal by interferon beta-1b therapy. J Clin Invest. (1997) 99(11):2664–71. doi: 10.1172/JCI119455
72. Sellebjerg F, Jensen J, Jensen CV, Wiik A. Expansion of CD5 - B cells in multiple sclerosis correlates with CD80 (B7-1) expression. Scand J Immunol (2002) 56(1):101–7. doi: 10.1046/j.1365-3083.2002.01108.x
73. O'Neill SK, Cao Y, Hamel KM, Doodes PD, Hutas G, Finnegan A. Expression of CD80/86 on B cells is essential for autoreactive T cell activation and the development of arthritis. J Immunol (2007) 179(8):5109–16. doi: 10.4049/jimmunol.179.8.5109
74. Arkatkar T, Du SW, Jacobs HM, Dam EM, Hou B, Buckner JH, et al. B cell-derived IL-6 initiates spontaneous germinal center formation during systemic autoimmunity. J Exp Med (2017) 214(11):3207–17. doi: 10.1084/jem.20170580
75. Barr TA, Shen P, Brown S, Lampropoulou V, Roch T, Lawrie S, et al. B cell depletion therapy ameliorates autoimmune disease through ablation of IL-6-producing B cells. J Exp Med (2012) 209(5):1001–10. doi: 10.1084/jem.20111675
76. Maeda K, Mehta H, Drevets DA, Coggeshall KM. IL-6 increases b-cell IgG production in a feed-forward proinflammatory mechanism to skew hematopoiesis and elevate myeloid production. Blood (2010) 115(23):4699–706. doi: 10.1182/blood-2009-07-230631
77. van Langelaar J, Rijvers L, Smolders J, van Luijn MM. B and T cells driving multiple sclerosis: Identity, mechanisms and potential triggers. Front Immunol (2020) 11:760. doi: 10.3389/fimmu.2020.00760
78. Lee BO, Moyron-Quiroz J, Rangel-Moreno J, Kusser KL, Hartson L, Sprague F, et al. CD40, but not CD154, expression on B cells is necessary for optimal primary B cell responses. J Immunol (2003) 171(11):5707–17. doi: 10.4049/jimmunol.171.11.5707
79. Meade J, Fernandez C, Turner M. The tyrosine kinase Lyn is required for B cell development beyond the T1 stage in the spleen: rescue by over-expression of bcl-2. Eur J Immunol (2002) 32(4):1029–34. doi: 10.1002/1521-4141(200204)32:4<1029
80. Ackermann JA, Nys J, Schweighoffer E, McCleary S, Smithers N, Tybulewicz VL. Syk tyrosine kinase is critical for B cell antibody responses and memory B cell survival. J Immunol (2015) 194(10):4650–6. doi: 10.4049/jimmunol.1500461
81. Oladipupo F, Yu CR, Olumuyide E, Jittaysothorn Y, Choi JK, Egwuagu CE. STAT3 deficiency in B cells exacerbates uveitis by promoting expansion of pathogenic lymphocytes and suppressing regulatory B cells (Bregs) and Tregs. Sci Rep (2020) 10. doi: 10.1038/s41598-020-73093-1
82. Jin G, Hamaguchi Y, Matsushita T, Hasegawa M, Huu DL, Ishiura N, et al. B-cell linker protein expression contributes to controlling allergic and autoimmune diseases by mediating IL-10 production in regulatory B cells. J Allergy Clin Immunol (2013) 131(6):1674–82. doi: 10.1016/j.jaci.2013.01.044
83. Aiba Y, Oh-hora M, Kiyonaka S, Kimura Y, Hijikata H, Mori Y, et al. Activation of RasGRP3 by phosphorylation of thr-133 is required for B cell receptor-mediated ras activation. Proc Natl Acad Sci U.S.A (2004) 101(47):16612–7. doi: 10.1073/pnas.0407468101
84. Kremmidiotis G, Zola H. Changes in CD44 expression during B cell differentiation in the human tonsil. Cell Immunol (1995) 161(2):147–57. doi: 10.1006/cimm.1995.1021
Keywords: B cells, central nervous system, immune modulation, matrix metalloproteinase-3, multiple sclerosis
Citation: Chunder R, Schropp V, Jabari S, Marzin M, Amor S and Kuerten S (2022) Identification of a novel role for matrix metalloproteinase-3 in the modulation of B cell responses in multiple sclerosis. Front. Immunol. 13:1025377. doi: 10.3389/fimmu.2022.1025377
Received: 22 August 2022; Accepted: 26 September 2022;
Published: 26 October 2022.
Edited by:
Francesca Gilli, Dartmouth College, United StatesReviewed by:
Horea Rus, University of Maryland, United StatesKrista DiSano, United States Department of Veterans Affairs, United States
Copyright © 2022 Chunder, Schropp, Jabari, Marzin, Amor and Kuerten. This is an open-access article distributed under the terms of the Creative Commons Attribution License (CC BY). The use, distribution or reproduction in other forums is permitted, provided the original author(s) and the copyright owner(s) are credited and that the original publication in this journal is cited, in accordance with accepted academic practice. No use, distribution or reproduction is permitted which does not comply with these terms.
*Correspondence: Stefanie Kuerten, c3RlZmFuaWUua3VlcnRlbkB1bmktYm9ubi5kZQ==
†These authors have contributed equally to this work and share first authorship