- 1Université ParisCité, INSERM, PARCC, Paris, France
- 2Institut Curie, PSL Research University, Department of Translational Research, Paris, France
- 3INSERM U830, Equipe labellisée LNCC, Siredo Oncology Centre, Institut Curie, Paris, France
- 4Immunology, APHP, Hôpital Europeen Georges Pompidou and Hôpital Necker, Paris, France
- 5Division of Clinical Pharmacology, Department of Medicine IV, Klinikum der Universität München, Munich, Germany
- 6German Cancer Consortium (DKTK), Partner Site Munich, Munich, Germany
- 7Lung Oncology Unit, APHP, Hôpital Européen Georges Pompidou, Paris, France
- 8Equipe Labellisée Ligue contre le Cancer, Paris, France
CXCR6 is a receptor for the chemokine CXCL16, which exists as a membrane or soluble form. CXCR6 is a marker for resident memory T (TRM) cells that plays a role in immunosurveillance through their interaction with epithelial cells. The interaction of CXCR6 with CXCL16 expressed at the membrane of certain subpopulations of intratumor dendritic cells (DC) called DC3, ideally positions these CXCR6+ T cells to receive a proliferation signal from IL-15 also presented by DC3. Mice deficient in cxcr6 or blocking the interaction of CXCR6 with its ligand, experience a poorer control of tumor proliferation by CD8+ T cells, but also by NKT cells especially in the liver. Intranasal vaccination induces CXCL16 production in the lungs and is associated with infiltration by TRM expressing CXCR6, which are then required for the efficacy of anti-tumor vaccination. Therapeutically, the addition of CXCR6 to specific CAR-T cells enhances their intratumoral accumulation and prolongs survival in animal models of pancreatic, ovarian and lung cancer. Finally, CXCR6 is part of immunological signatures that predict response to immunotherapy based on anti-PD-(L)1 in various cancers. In contrast, a protumoral role of CXCR6+T cells has also been reported mainly in Non-alcoholic steatohepatitis (NASH) due to a non-antigen specific mechanism. The targeting and amplification of antigen-specific TRM expressing CXCR6 and its potential use as a biomarker of response to immunotherapy opens new perspectives in cancer treatment.
Introduction
CXCR6 was initially described as a co-receptor for HIV (1) expressed on human memory T cells (2, 3). It is also detected on natural killer (NK) cells (3), NKT cells (4), dendritic cells (DC) (5), alveolar macrophages (6) and innate lymphoid cells (ILC) (7). CXCR6 induction is slow (8 days) after activation (8, 9). IL-15 or antigen exposure followed by TGFβ induce CXCR6 (10) and this effect can be further increased by IL-21 (11).
CXCR6 does not appear to be required in the functionality of CD8+T cells (12–14).
Its unique ligand CXCL16 can exist both in a transmembrane and soluble form (2, 15, 16). Membrane CXCL16 acts as an adhesion molecule, whereas its proteolytically cleaved, soluble form acts as a chemoattractant (17). CXCL16 is expressed by epithelial cells, endothelial cells (18) and immune cells such as DC (19, 20).
Several previous studies and reviews highlighted the up-regulation of CXCL16 and/or CXCR6 by tumor cells and their role in tumor growth, migration and invasiveness (21–30). The authors found that CXCL16 is involved in the viability and invasion of tumor cells (22, 31), while the expression of CXCR6 by cancer cells triggers oncogenic pathways associated with cancer progression and metastasis (23, 31). Therefore, in this review, we decided to restrict our focus on the role of CXCR6 expressed by T cells in a tumor context.
CXCR6 a marker to define TRM
Resident memory T cells (TRM) are a population of T cells mainly present in tissues and defined usually by the expression of CD103, CD49a and CD69. The CD103 marker interacts with E-cadherin expressed by epithelial cells which explains the role of these cells in immunosurveillance of epithelial tissues (32–34).
CXCR6 is a core marker of TRM in various cancers (6, 35–38). In ovarian and lung cancer, at the protein level, CXCR6 was predominantly expressed on CD8+ TRM as compared with intratumoral effector CD8+ T cells or circulating T cells (14, 39).
CXCR6: Role in the differentiation, localization and survival of CD8+ T cells in tissues
Role of CXCR6 in the differentiation of T cells in tissues
During T cell differentiation program, precursor cells (TCF1+) are primed in the lymph node and then migrate into tissues, where they continue their differentiation. CXCR6 is poorly expressed by these TCF1+cells. It may explain why in studies using CXCR6+ and CXCR6− CD8+ T cells, the expression of CXCR6 does not appear to be critical for priming CD8+ T cells in lymphoid organs (13, 40). Interleukin-12 promotes loss of TCF-1 and conversion of progenitor into effector CD8+ T cells (41). CX3CR1 is induced following the TCF-1+ to TCF-1- conversion and characterizes the most highly functional and proliferative CD8+ T cell subsets (42, 43). These CX3CR1+ TCF1-Tbet+ CD8+ T cells are also called transitory CD8+ T cells in mice. They are found in lymph nodes and tissues. CXCR6 up-regulation immediately precedes or accompanies loss of TCF-1 expression in tumor-reactive PD-1+ CD8+T cells, whose specificity is infered by the expression of PD1, a marker enriched within anti-tumor T cells (Figure 1).
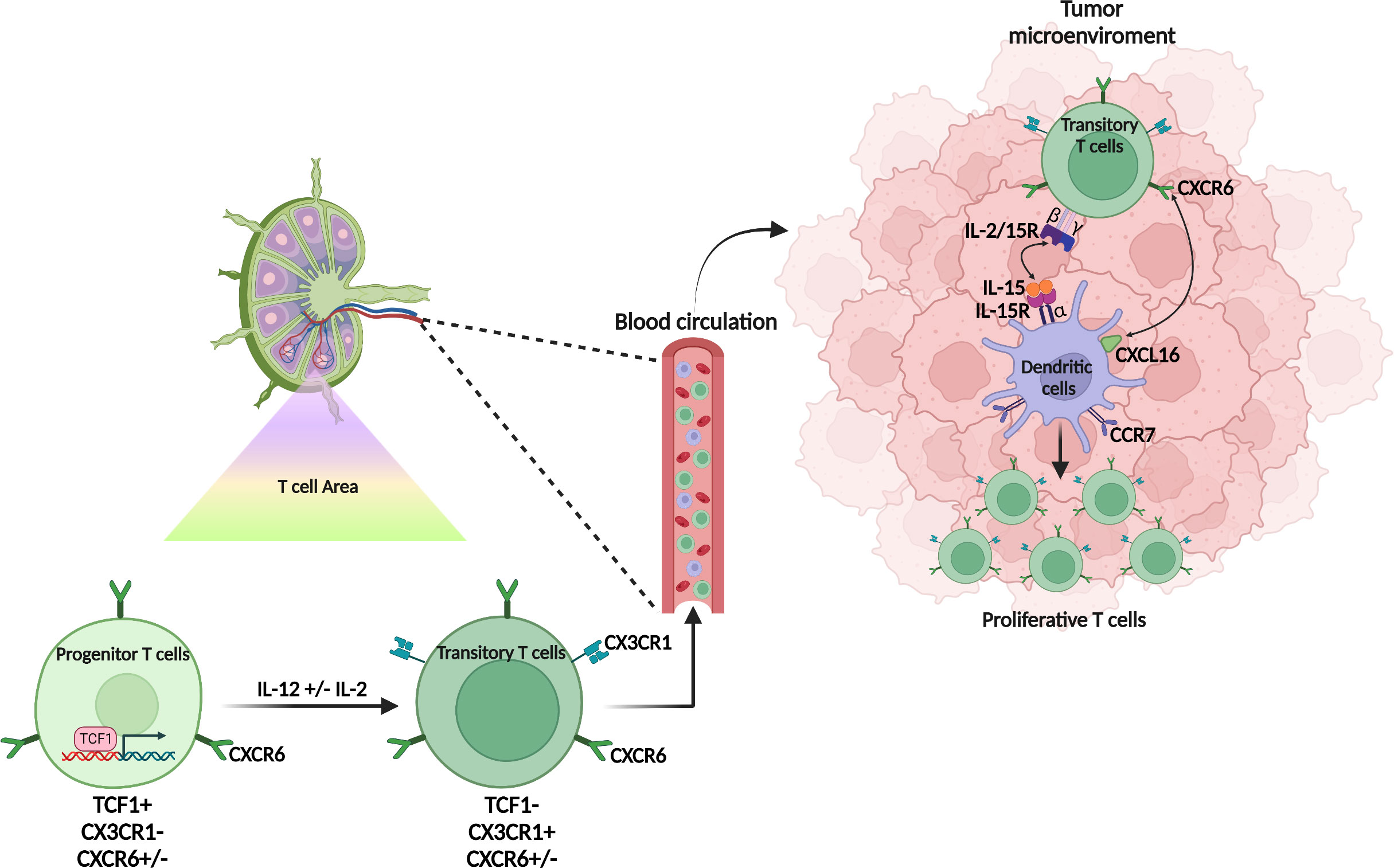
Figure 1 Origin and differentiation of CXCR6+ T cells. CXCR6+ T cells are scarcely present in the lymph node and CXCR6 weakly expressed by both the progenitor (TCF1+) or the transitory (TCF1-, CX3CR1+) T cell population. In the tumor microenvironment, the transitory T cells express CXCR6 which allows them to interact with the dendritic cell subpopulation (CCR7+ DC3) which expresses the membrane form of CXCL16. This contact promotes the interaction of IL-15 also expressed on the membrane of DC3 with the βγ chain of IL-15R expressed on CXCR6+ T cells. IL-15 induces the proliferation of CXCR6+ T cells and promotes their survival in the tumor microenvironment.
Cxcr6 deficiency in mice neither affects TCF1+CD8+ T cells in lymph node or tumor microenvironment (TME), nor the CX3CR1 population in lymph nodes. However, it inhibits the expansion of this transient CX3CR1 population in tissues and tumors and the survival of TCF1- populations (40). These elegant works on the role of CXCR6 in T cell differentiation focusing on anti-tumor T cells, do not specify its impact on the differentiation of exhausted T cells and TRM. Nevertheless, in the absence of CXCR6, the expression of Tim-3 - a marker of exhaustion - (34) on T cells, is decreased (40) as well as TRM in the TME (12).
CXCR6 dictates the interaction of T cells with dendritic cells and their subsequent survival
CXCR6 positions CD8+ cytotoxic T cells in a distinct perivascular niche of the tumor stroma that is populated by CCR7+ DC named DC3 expressing the CXCR6-ligand CXCL16 and trans-presenting the cytokine IL-15 (40). DC3s trans-present IL-15 to CXCR6+ TCF-1- effector CD8+ T cells to sustain their survival in the TME and avoid AICD (activation-induced-cell-death) (40). In vitro, IL-15 expanded CXCR6hi TCF-1- cells (40) (Figure 1).
DC3 express CCR7, IL12Rβ, Fascin1 (44, 45) and represent the DC population with the highest expression of CXCL16, CXCL15 and IL-15Rα (40). In humans, this DC3 population has been observed in the TME of breast cancer patients and promotes resident memory CD8+ T cell differentiation via a TGFβ signaling (see below) (46). CXCR6+ CD8+ T cells transferred into mice lacking IL-15 or DC do not survive (40).
Role of CXCR6 in the positioning and survival of CD8+ TRM in tissues
In mice, CD8+ T cells lacking expression of CXCR6 formed reduced numbers of skin TRM cells, but comparable numbers in the spleen with regards to wild type mice (47).
CXCR6 also positions tumor reactive CD8+ TRM with CXCL16+ DC clusters in the skin of melanoma-associated vitiligo, which favor their persistence (48).
In an ovarian cancer model, CXCR6-deficient mice have less TRM in the tumor (14).
It has also recently been shown that the CXCR6–CXCL16 axis plays a role in the seeding of airway TRM from lung interstitium (49, 50).
CXCR6 expressing CD4+ T cells, NKT and MAIT
In mouse and human, CXCR6 is more expressed in CD8+ T cells than in CD4+ T cells (40). In the Cancer Genome Atlas (TCGA) database, CXCR6 in tumor tissue correlated highly with CD8 expression and less with expression of CD4 and NK cells (40).
It has been reported that CXCR6+ CCR6+ CD4+ subset is enriched for conventional TH17 molecules (IL-17A, IL-23R, RORγt) and cytotoxic signatures (51, 52), while the CCR6- CXCR6+ CD4+ T cells expressed IFNγ and GM-CSF, which correspond to a T cell population derived from TH17 cells (52).
CXCR6 plays an essential role in NKT cell development, maturation, homeostatic distribution, glycolipid-induced effector responses, and infiltration into the liver (53, 54). The accumulation of CXCR6+ NKT cells in the liver is driven by up-regulated CXCL16 on hepatic sinusoidal endothelial cells, which is induced by gut microbiome-modified bile acids (55). Thus CXCR6-deficient mice show profoundly reduced numbers of NKT cells in the liver (53). In addition to regulating NKT cell homing, CXCR6 and CXCL16 have been shown to play a critical role in NKT cell activation in response to glycolipid antigens (15, 53).
MAIT cells are predominantly CXCR6+ but do not require CXCR6 for accumulation in lungs after an infection. However, CXCR6 does contribute to long-term retention of MAIT cells in the airway lumen (56).
Role of CXCR6+ T cells in cancer
Tumor proliferation control
In different preclinical models of melanoma, the absence of CXCR6 led to an acceleration of tumor growth (40, 57). In terms of mechanism, CXCR6 was not essential for extravasation of blood-borne CD8+ T cells into tumor tissue (40). Experiments involving the transfer of T lymphocytes expressing or not CXCR6, or blocking the CXCL16-CXCR6 interaction, have shown the requirement of CXCR6-CD8+T cells for the anti-tumor function of these T cells (12, 40, 57–59).
In different preclinical liver cancer models, the role of NKT and CD4+ T cells producing INFγ and TNFα has also been reported. Thus, in a model of hepatocarcinogenesis, cxcr6-deficient mice had significantly more senescent hepatocytes. NKT and CD4+ T cells promote the removal of senescent hepatocytes to prevent hepatocarcinogenesis, and this process required CXCR6 (60).
Role in the control of metastases
Loss of CXCR6 expression on NKT-cells resulted in increased liver metastasis in a murine model (61). Cxcr6- deficient mice or CXCL16 neutralizing Ab resulted in an enhanced metastasis to the liver by B16 melanoma cells or Lewis Lung tumor cells (61). In another preclinical model of lung metastases from breast cancer, it has been shown that CXCR6- T effectors are the major subset preferentially egressing the tumor to form distant CXCR6+ TRM, whereas intratumoral CXCR6+ T cells are retained in the tumor (62). Breaking CXCR6-mediated retention in the tumor by anti-CXCL16 treatment resulted in more T cells egressing to the distant lung tissue and a decrease metastatic tumor burden (62).
Improvement of CAR-T cell therapy
Although CAR-T cell therapy in solid tumors has recently shown its feasibility and clinical signs of effectiveness (63, 64), the efficacy of adoptive cell therapy for solid tumors is hampered by the low infiltration of the tumor after transferred T cells. Recently, it could be shown that T cells expressing a chimeric antigen receptor (CAR) encoding mesothelin and co-transfected with CXCR6 enhanced the efficacy of adoptive cell therapy for pancreatic tumors (65). In mouse models, the addition of CXCR6 to CAR-T cells also enhanced their intratumoral accumulation and sustained their antitumoral activity. Survival was prolonged only when the CAR-T cells co-expressed CXCR6 (65). These results were also reproduced in an ovarian cancer mouse model (65).
Administration of CAR-T cells targeting murine ROR1 – a tumor antigen overexpressed in breast and lung cancer - after lymphodepletion with cyclophosphamide (Cy) transiently controlled lung tumor growth but infiltrated tumors poorly and lost function, as observed in human. Adding oxaliplatin (Ox) to the lymphodepletion regimen activated tumor macrophages to express T cell-recruiting chemokines, resulting in improved CAR-T cell infiltration (66).
At day 2 post-transfer, Ox/Cy enhanced accumulation of CAR-T cells in tumors excised from KPROR1 mice, and this accumulation was partially CXCR6-dependent, as CXCR6−/− CAR-T cells showed poorer tumor infiltration compared to their wild type counterparts (66).
Role in cancer vaccine efficacy
Previous works of our group showed that intranasal vaccination preferentially elicits TRM (67–69). As a continuation of this work, we showed that CXCR6 was preferentially expressed by CD8+ TRM after intranasal vaccination in mice with a vector targeting DC (70) and also on intratumoral CD8+ TRM derived from human lung cancer (12). We also demonstrated that vaccination of cxcr6-deficient mice induces a defect in the lung recruitment of antigen-specific CD8+ T cells, mostly in the TRM subsets, responsible for a partial loss of cancer vaccine efficacy (12). Interestingly, intranasal, but not intramuscular vaccination induced higher and more sustained concentrations of CXCL16, compared to other chemokines, in the bronchoalveolar lavage fluid and pulmonary parenchyma (12).
Role in immunogenicity of radiotherapy
Ionizing radiation appears to increase both in mouse and human the expression of CXCL16 in tumor cells (71) and specifically in breast, colon and prostate cancer (59), favoring the recruitment of effector cells at the tumor site
Cxcr6-deficient mice showed reduced infiltration of tumors by activated CD8+ T cells and impaired tumor regression following treatment by local irradiation of the tumor (59).
Pro-tumoral role of CXCR6+ T cells
In prostate cancer, inflammatory cytokines derived from the adjacent infiltrating CXCR6-positive T cells stimulate the production of CXCL16 by cancer cells and CXCL16 enhances the growth of CXCR6-expressing cancers and primary T cells (72).
In mice model of Non-alcoholic steatohepatitis (NASH), which causes severe and chronic liver inflammation leading to hepatocarcinoma (HCC), an indispensable role of T cells in liver immunopathology was demonstrated.
CXCR6+ CD8+ T cells showed killing of cells in an MHC-class-I-independent fashion after signaling through P2X7 purinergic receptors activated by ATP (11).
The exposure of CXCR6+ CD8+ T cells to ATP led to rapid upregulation of FasL and the death of hepatocytes. The blocking of FasL prevented auto-aggression by CD8 T cells in vitro and after adoptive transfer in vivo, and ameliorated liver damage in NASH mice (11).
Role of CXCR6+ T cells in clinical response and side effects of anti-PD-1 immunotherapy
In a preclinical model of melanoma and colorectal cancer, anti-PD-1 treatment significantly increased CXCR6 expression on infiltrating CD8+ T cells (57). Interestingly, the percentages of intratumoral CD8+ T cells and cytokines production, as well as the efficacy of therapy, were rapidly decreased in cxcr6−/− mice treated with PD-1 blockade therapy (57).
In contrast, when given prophylactically in a mice model of NASH, anti-PD1 treatment led to an increase in the incidence of NASH induced HCC and in the number and size of tumor nodules, which correlated with increased hepatic PD1+ CXCR6+ CD8+ T cells and TNF+ T cells (73). The increase in HCC triggered by anti-PD1 treatment was prevented by depletion of CD8+ T cells or TNF neutralization (73). These results may seem contradictory to the clinical data on the efficacy of anti-PD1 in liver cancer. In fact, a meta-analysis of 1,600 patients revealed that immunotherapy based on PD-1-PD-L1 blockade did not improve survival in patients with non-viral HCC (73).
CXCR6+ T cells have also been implicated in the side effects of immunotherapy. Thus, a striking accumulation of CXCR6+ CD8+ T cells with highly cytotoxic and proliferative states is observed in checkpoint inhibitor-induced colitis (74). Interestingly, administration of an anti-CXCL16 mAb reduced inflammation in a chemically induced experimental colitis model (75).
CXCR6, a prognostic and predictive biomarker for cancer immunotherapy
In most cancers (melanoma, head and neck cancer, lung adenocarcinoma, and breast cancer), patients with high CXCR6 expression had a greater survival probability (40). An exception concerns liver cancer, where CXCR6 and its cognate ligand CXCL16 have been associated with higher HCC invasiveness, poor prognosis and predictor of recurrence (18, 28).
The good prognosis related to CXCR6 expression is associated with CD8+ T cell infiltration often corresponding to a TRM phenotype.
Similarly, high expression of CXCR6 in colorectal cancer was associated with a good prognosis and positively correlated with the expression of CD8 in tumor (57).
Analysis of The Cancer Genome Atlas (TCGA) for ovarian cancer revealed CXCR6 expression to be associated with CD103 and increased patient survival (14).
Interestingly, CXCR6 constitutes 1 of 18 genes that are developed and validated as a clinical grade biomarker to predict the response to anti-PD-1 therapy in various cancers (76, 77).
How to elicit CXCR6+ T cells
To induce CXCR6+ T cells in the lungs and head and neck tissue, we have shown that the nasal route of immunization appears to be the most effective in different experiments (12). These T cells had a phenotype of CD8+ TRM. Similar results on the value of this mucosal route of immunization have been reported by different groups (78–80) (Figure 2).
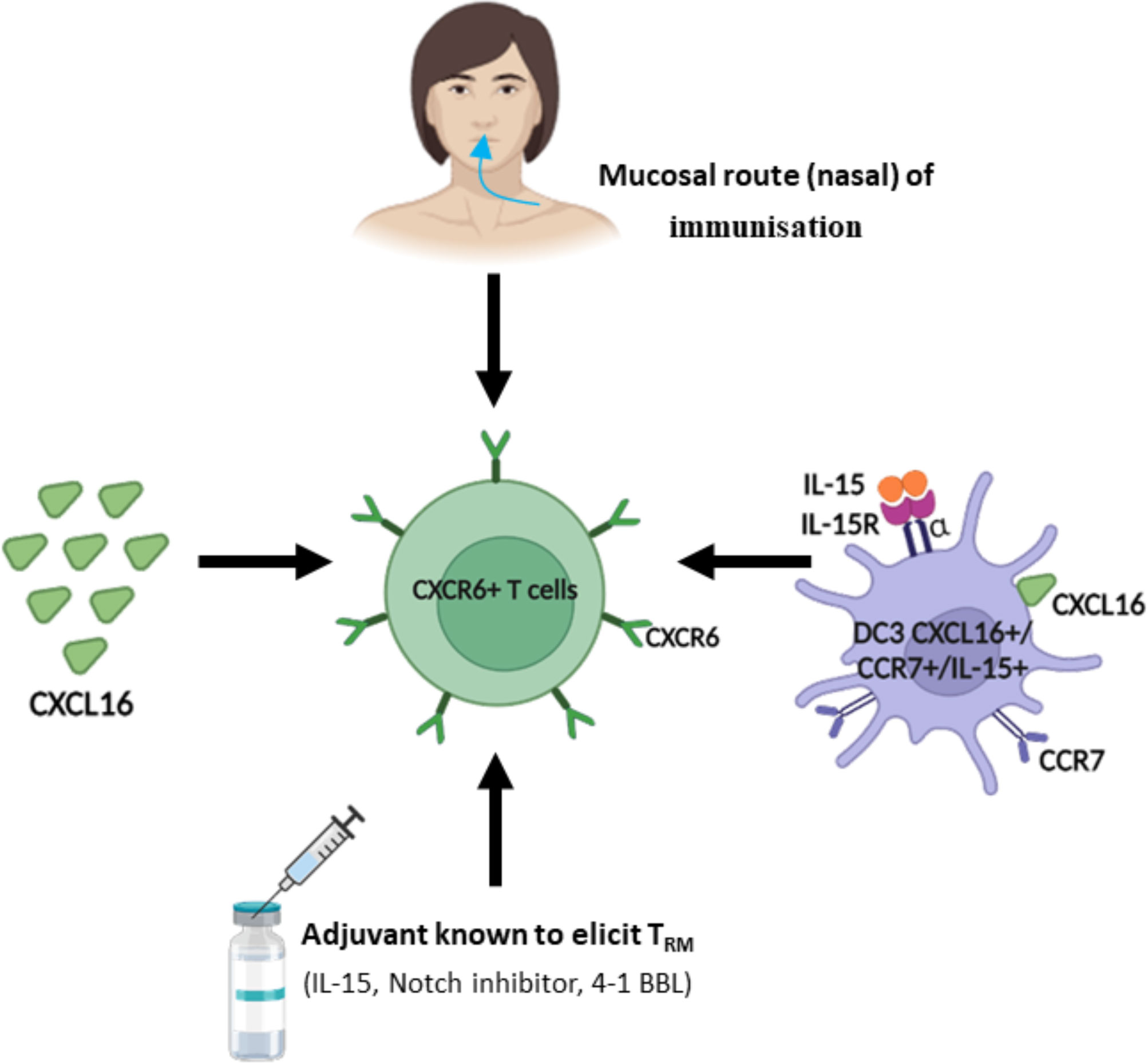
Figure 2 Strategy to regulate and induce CXCR6+ T cells.In light of the role of CXCR6+ T cells in tumor control, different strategies have been proposed to induce or increase their recruitment in the tumor microenvironment. As the majority of CXCR6+ T cells are included in the resident memory T cell population (TRM), many strategies are common with those aimed at inducing TRM.
This mucosal CD8+ T cell response can be maintained for several months. Interestingly, not all mucosal routes are equivalent in inducing these CXCR6+ T cells. For example, the oral route of immunization induces mainly the chemokine CCR9 but not CXCR6 (79). We and other groups have shown that this nasal immunization, but not the systemic routes (s.c or IM) induced CXCL16 in the lungs likely produced by epithelial cells and DC and promoting their interaction with CXCR6+ T cells (12, 78, 80). This induction of endogenous production of CXCL16 may explain conflicting results of CXCL16 administration as a vaccine adjuvant (12, 78, 79).
The subcutaneous route results in a small increase of CXCR6 in lymph node T cells (81). Parenteral routes are poorly effective in inducing these CXCR6+ T cells in the lungs (78).
In vitro, DC3 are able to induce CD8+ TRM expressing CXCR6 and promote their expansion (40). Some adjuvants (IL-15, 4-1BBL, Notch inhibitors, …) known to increase CD8+ TRM could be evaluated for their ability to induce CXCR6 (69) (Figure 2).
Discussion and conclusion
CXCR6 can be considered as a new class of chemokine receptor, whose main role could be to allow the positioning of T cells in close interaction with cells expressing CXCL16 at the membrane such as DC3, and so to promote T cell differentiation. The adhesion role of CXCL16 known for many years, has been strengthened recently and the CXCL16-CXCR6 interaction seems to participate in a synapse including IL-15 and IL-15Rα to promote tissue T cell differentiation, particularly toward a TRM phenotype (40, 48).
An ambivalent role of CXCR6+ T cells in tumor control has been reported. Thus, in many models, CXCR6+ CD8+ T cells of resident phenotype participate in the control of primary tumor proliferation and metastasis (12, 57, 62). On the contrary, in NASH models with significant chronic inflammation, CXCR6+ P2X7+ T cells are able to destroy hepatocytes in a non-MHC-restricted manner which distinguishes them from classical CD8+ TRM (11, 73).
This ambivalent role of CXCR6+ T cells in the regulation of tumor growth has also been established for other subpopulations of T cells such as TH17 (82–84) and for myeloid cell subpopulations (85–87). It may be explained by differences in fine phenotype and function for the same immune sub-population and may also depend on the tumor stage and the acute or chronic inflammatory context (88).
The mucosal nasal route of immunization preferentially induces these CXCR6+ T cells in the nasal and pulmonary mucosa as already reported for TRM which also express CXCR6 (12, 78, 79). The induction of CXCL16 by this immunization route may explain this specific recruitment of CXCR6+ T cells by this mucosal pathway.
In most models, these CXCR6+ T cells belong to the subpopulation of TRM. However, NKT cells also express CXCR6, which seems to play a major role in the development of these cells, especially in the liver. CXCR6+ NKT cells regulate hepatocarcinogenesis and metastasis formation and control hepatitis (53–55).
Finally, from a clinical perspective, CXCR6 expression in CAR+ T cells has improved their efficacy in various preclinical solid tumors and might indicate a track to translation of the approach (65, 66). Anti-PD-1 antibodies which increase TRM also modulates CXCR6 (57) and in fact, molecular signatures including CXCR6 appear to predict response to immunotherapy (76, 77), underpinning the high relevance of the pathway.
Author contributions
NM and ET designed the literature search and wrote the article with input from all authors. NM, ET, and NG designed the figures. All authors contributed to the article and approved the submitted version.
Funding
This research was funded by grants from Fondation ARC pour la recherche sur le cancer (Grant number SIGN’IT20181007747 and PGA12019110000946_1581), INCA (contract 2019-1-PLBIO-05-1)(PLBIO22-147), Foncer contre le Cancer, SIRIC CARPEM, Labex Immuno-Oncology, Ligue contre le cancer (MucoRNAVax), IDEX Université de Paris (Grant number AMI-CD27CD70BLOC), Inserm Transfert (Grant number: MAT-PI-20278-1). This study was supported by the Marie Sklodowska-Curie Program Training Network for Optimizing Adoptive T Cell Therapy of Cancer funded by the H2020 Program of the European Union (Grant 955575, to SK); by the Hector Foundation (to SK); by the International Doctoral Program i-Target: Immunotargeting of Cancer funded by the Elite Network of Bavaria (to SK); by Melanoma Research Alliance Grants 409510 (to SK); by the Else Kröner-Fresenius-Stiftung (2021_EKFK_01, to SK); by the German Cancer Aid (to SK); by the Ernst-Jung-Stiftung (to SK); by the LMU Munich’s Institutional Strategy LMUexcellent within the framework of the German Excellence Initiative (to SE and SK); by the m4-award of the Bavarian Ministry for economical affairs (to SK), by the Go-Bio-initiative (to SK), by the Bundesministerium für Bildung und Forschung (to SK); by the European Research Council Grant 756017, ARMOR-T (to SK); by the Wilhelm-Sander-Stiftung (to SK), by the German Research Foundation (DFG) (to SK); by the SFB-TRR 338/1 2021–452881907 (to SK); by the Fritz-Bender Foundation (to SK) and by the Deutsche José-Carreras Leukämie Stiftung (to SK).
Acknowledgments
The figures were created with BioRender.com.
Conflict of interest
The authors declare that the research was conducted in the absence of any commercial or financial relationships that could be construed as a potential conflict of interest.
Publisher’s note
All claims expressed in this article are solely those of the authors and do not necessarily represent those of their affiliated organizations, or those of the publisher, the editors and the reviewers. Any product that may be evaluated in this article, or claim that may be made by its manufacturer, is not guaranteed or endorsed by the publisher.
References
1. Alkhatib G, Liao F, Berger EA, Farber JM, Peden KW. A new SIV co-receptor, STRL33. Nature (1997) 388(6639):238. doi: 10.1038/40789
2. Matloubian M, David A, Engel S, Ryan JE, Cyster JG. A transmembrane CXC chemokine is a ligand for HIV-coreceptor bonzo. Nat Immunol (2000) 1(4):298–304. doi: 10.1038/79738
3. Unutmaz D, Xiang W, Sunshine MJ, Campbell J, Butcher E, Littman DR. The primate lentiviral receptor Bonzo/STRL33 is coordinately regulated with CCR5 and its expression pattern is conserved between human and mouse. J Immunol (2000) 165(6):3284–92. doi: 10.4049/jimmunol.165.6.3284
4. Motsinger A, Haas DW, Stanic AK, Van Kaer L, Joyce S, Unutmaz D. CD1d-restricted human natural killer T cells are highly susceptible to human immunodeficiency virus 1 infection. J Exp Med (2002) 195(7):869–79. doi: 10.1084/jem.20011712
5. Berahovich RD, Lai NL, Wei Z, Lanier LL, Schall TJ. Evidence for NK cell subsets based on chemokine receptor expression. J Immunol (2006) 177(11):7833–40. doi: 10.4049/jimmunol.177.11.7833
6. Morgan AJ, Guillen C, Symon FA, Birring SS, Campbell JJ, Wardlaw AJ. CXCR6 identifies a putative population of retained human lung T cells characterised by co-expression of activation markers. Immunobiology (2008) 213(7):599–608. doi: 10.1016/j.imbio.2008.01.005
7. Chea S, Possot C, Perchet T, Petit M, Cumano A, Golub R. CXCR6 expression is important for retention and circulation of ILC precursors. Mediators Inflammation (2015) 2015:368427. doi: 10.1155/2015/368427
8. Agostini C, Cabrelle A, Calabrese F, Bortoli M, Scquizzato E, Carraro S, et al. Role for CXCR6 and its ligand CXCL16 in the pathogenesis of T-cell alveolitis in sarcoidosis. Am J Respir Crit Care Med (2005) 172(10):1290–8. doi: 10.1164/rccm.200501-142OC
9. Heesch K, Raczkowski F, Schumacher V, Hunemorder S, Panzer U, Mittrucker HW. The function of the chemokine receptor CXCR6 in the T cell response of mice against listeria monocytogenes. PloS One (2014) 9(5):e97701. doi: 10.1371/journal.pone.0097701
10. Pallett LJ, Davies J, Colbeck EJ, Robertson F, Hansi N, Easom NJW, et al. IL-2(high) tissue-resident T cells in the human liver: Sentinels for hepatotropic infection. J Exp Med (2017) 214(6):1567–80. doi: 10.1084/jem.20162115
11. Dudek M, Pfister D, Donakonda S, Filpe P, Schneider A, Laschinger M, et al. Auto-aggressive CXCR6(+) CD8 T cells cause liver immune pathology in NASH. Nature (2021) 592(7854):444–49. doi: 10.1038/s41586-021-03233-8
12. Karaki S, Blanc C, Tran T, Galy-Fauroux I, Mougel A, Dransart E, et al. CXCR6 deficiency impairs cancer vaccine efficacy and CD8(+) resident memory T-cell recruitment in head and neck and lung tumors. J Immunother Cancer (2021) 9(3):e001948. doi: 10.1136/jitc-2020-001948
13. Tse SW, Radtke AJ, Espinosa DA, Cockburn IA, Zavala F. The chemokine receptor CXCR6 is required for the maintenance of liver memory CD8(+) T cells specific for infectious pathogens. J Infect Dis (2014) 210(9):1508–16. doi: 10.1093/infdis/jiu281
14. Muthuswamy R, McGray AR, Battaglia S, He W, Miliotto A, Eppolito C, et al. CXCR6 by increasing retention of memory CD8(+) T cells in the ovarian tumor microenvironment promotes immunosurveillance and control of ovarian cancer. J Immunother Cancer (2021) 9(10):1–11. doi: 10.1136/jitc-2021-003329
15. Shimaoka T, Kume N, Minami M, Hayashida K, Kataoka H, Kita T, et al. Molecular cloning of a novel scavenger receptor for oxidized low density lipoprotein, SR-PSOX, on macrophages. J Biol Chem (2000) 275(52):40663–6. doi: 10.1074/jbc.C000761200
16. Wilbanks A, Zondlo SC, Murphy K, Mak S, Soler D, Langdon P, et al. Expression cloning of the STRL33/BONZO/TYMSTRligand reveals elements of CC, CXC, and CX3C chemokines. J Immunol (2001) 166(8):5145–54. doi: 10.4049/jimmunol.166.8.5145
17. Koenen A, Babendreyer A, Schumacher J, Pasqualon T, Schwarz N, Seifert A, et al. The DRF motif of CXCR6 as chemokine receptor adaptation to adhesion. PloS One (2017) 12(3):e0173486. doi: 10.1371/journal.pone.0173486
18. Heydtmann M, Lalor PF, Eksteen JA, Hubscher SG, Briskin M, Adams DH. CXC chemokine ligand 16 promotes integrin-mediated adhesion of liver-infiltrating lymphocytes to cholangiocytes and hepatocytes within the inflamed human liver. J Immunol (2005) 174(2):1055–62. doi: 10.4049/jimmunol.174.2.1055
19. Tabata S, Kadowaki N, Kitawaki T, Shimaoka T, Yonehara S, Yoshie O, et al. Distribution and kinetics of SR-PSOX/CXCL16 and CXCR6 expression on human dendritic cell subsets and CD4+ T cells. J Leukoc Biol (2005) 77(5):777–86. doi: 10.1189/jlb.1204733
20. Shimaoka T, Nakayama T, Kume N, Takahashi S, Yamaguchi J, Minami M, et al. Cutting edge: SR-PSOX/CXC chemokine ligand 16 mediates bacterial phagocytosis by APCs through its chemokine domain. J Immunol (2003) 171(4):1647–51. doi: 10.4049/jimmunol.171.4.1647
21. Hattermann K, Held-Feindt J, Ludwig A, Mentlein R. The CXCL16-CXCR6 chemokine axis in glial tumors. J Neuroimmunol (2013) 260(1-2):47–54. doi: 10.1016/j.jneuroim.2013.04.006
22. Hu W, Liu Y, Zhou W, Si L, Ren L. CXCL16 and CXCR6 are coexpressed in human lung cancer in vivo and mediate the invasion of lung cancer cell lines in vitro. PloS One (2014) 9(6):e99056. doi: 10.1371/journal.pone.0099056
23. Wang J, Lu Y, Wang J, Koch AE, Zhang J, Taichman RS. CXCR6 induces prostate cancer progression by the AKT/mammalian target of rapamycin signaling pathway. Cancer Res (2008) 68(24):10367–76. doi: 10.1158/0008-5472.CAN-08-2780
24. Mir H, Kaur G, Kapur N, Bae S, Lillard JW Jr., Singh S. Higher CXCL16 exodomain is associated with aggressive ovarian cancer and promotes the disease by CXCR6 activation and MMP modulation. Sci Rep (2019) 9(1):2527. doi: 10.1038/s41598-019-38766-6
25. AbdelMageed M, Ali H, Olsson L, Lindmark G, Hammarstrom ML, Hammarstrom S, et al. The chemokine CXCL16 is a new biomarker for lymph node analysis of colon cancer outcome. Int J Mol Sci (2019) 20(22):1–16. doi: 10.3390/ijms20225793
26. Kim MJ, Sun HJ, Song YS, Yoo SK, Kim YA, Seo JS, et al. CXCL16 positively correlated with M2-macrophage infiltration, enhanced angiogenesis, and poor prognosis in thyroid cancer. Sci Rep (2019) 9(1):13288. doi: 10.1038/s41598-019-49613-z
27. Ikeda T, Nishita M, Hoshi K, Honda T, Kakeji Y, Minami Y. Mesenchymal stem cell-derived CXCL16 promotes progression of gastric cancer cells by STAT3-mediated expression of Ror1. Cancer Sci (2020) 111(4):1254–65. doi: 10.1111/cas.14339
28. Gao Q, Zhao YJ, Wang XY, Qiu SJ, Shi YH, Sun J, et al. CXCR6 upregulation contributes to a proinflammatory tumor microenvironment that drives metastasis and poor patient outcomes in hepatocellular carcinoma. Cancer Res (2012) 72(14):3546–56. doi: 10.1158/0008-5472.CAN-11-4032
29. Zhu Y, Zou C, Zhang Z, Qian CN, Yang X, Shi J, et al. MEK inhibitor diminishes nasopharyngeal carcinoma (NPC) cell growth and NPC-induced osteoclastogenesis via modulating CCL2 and CXCL16 expressions. Tumour Biol (2015) 36(11):8811–8. doi: 10.1007/s13277-015-3595-8
30. Guo H, Wang F, Diao Y, Zhang Z, Chen Q, Qian CN, et al. Knockdown of Notch1 inhibits nasopharyngeal carcinoma cell growth and metastasis via downregulation of CCL2, CXCL16, and uPA. Mol Carcinog (2019) 58(10):1886–96. doi: 10.1002/mc.23082
31. Hu W, Zhen X, Xiong B, Wang B, Zhang W, Zhou W. CXCR6 is expressed in human prostate cancer in vivo and is involved in the in vitro invasion of PC3 and LNCap cells. Cancer Sci (2008) 99(7):1362–9. doi: 10.1111/j.1349-7006.2008.00833.x
32. Paolini L, Saldmann A, Tartour E. CD8+T cell in cancer immunotherapy: role and value of its therapeutic targeting. Bull Acad Natl Med (2021) 205:354–63. doi: 10.1016/j.banm.2021.01.018
33. Mami-Chouaib F, Tartour E. Editorial: Tissue resident memory T cells. Front Immunol (2019) 10:1018. doi: 10.3389/fimmu.2019.01018
34. Mami-Chouaib F, Blanc C, Corgnac S, Hans S, Malenica I, Granier C, et al. Resident memory T cells, critical components in tumor immunology. J Immunother Cancer (2018) 6(1):87. doi: 10.1186/s40425-018-0399-6
35. Kumar BV, Ma W, Miron M, Granot T, Guyer RS, Carpenter DJ, et al. Human tissue-resident memory T cells are defined by core transcriptional and functional signatures in lymphoid and mucosal sites. Cell Rep (2017) 20(12):2921–34. doi: 10.1016/j.celrep.2017.08.078
36. Ganesan AP, Clarke J, Wood O, Garrido-Martin EM, Chee SJ, Mellows T, et al. Tissue-resident memory features are linked to the magnitude of cytotoxic T cell responses in human lung cancer. Nat Immunol (2017) 18(8):940–50. doi: 10.1038/ni.3775
37. Mackay LK, Minnich M, Kragten NA, Liao Y, Nota B, Seillet C, et al. Hobit and Blimp1 instruct a universal transcriptional program of tissue residency in lymphocytes. Science (2016) 352(6284):459–63. doi: 10.1126/science.aad2035
38. Guo X, Zhang Y, Zheng L, Zheng C, Song J, Zhang Q, et al. Global characterization of T cells in non-small-cell lung cancer by single-cell sequencing. Nat Med (2018) 24(7):978–85. doi: 10.1038/s41591-018-0045-3
39. Hombrink P, Helbig C, Backer RA, Piet B, Oja AE, Stark R, et al. Programs for the persistence, vigilance and control of human CD8+ lung-resident memory T cells. Nat Immunol (2016) 17(12):1467–78. doi: 10.1038/ni.3589
40. Di Pilato M, Kfuri-Rubens R, Pruessmann JN, Ozga AJ, Messemaker M, Cadilha BL, et al. CXCR6 positions cytotoxic T cells to receive critical survival signals in the tumor microenvironment. Cell (2021) 184(17):4512–30 e22. doi: 10.1016/j.cell.2021.07.015
41. Danilo M, Chennupati V, Silva JG, Siegert S, Held W. Suppression of Tcf1 by inflammatory cytokines facilitates effector CD8 T cell differentiation. Cell Rep (2018) 22(8):2107–17. doi: 10.1016/j.celrep.2018.01.072
42. Hudson WH, Gensheimer J, Hashimoto M, Wieland A, Valanparambil RM, Li P, et al. Proliferating transitory T cells with an effector-like transcriptional signature emerge from PD-1(+) stem-like CD8(+) T cells during chronic infection. Immunity (2019) 51(6):1043–58 e4. doi: 10.1016/j.immuni.2019.11.002
43. Zander R, Schauder D, Xin G, Nguyen C, Wu X, Zajac A, et al. CD4(+) T cell help is required for the formation of a cytolytic CD8(+) T cell subset that protects against chronic infection and cancer. Immunity (2019) 51(6):1028–42 e4. doi: 10.1016/j.immuni.2019.10.009
44. Gerhard GM, Bill R, Messemaker M, Klein AM, Pittet MJ. Tumor-infiltrating dendritic cell states are conserved across solid human cancers. J Exp Med (2021) 218(1):1–13. doi: 10.1084/jem.20200264
45. Zilionis R, Engblom C, Pfirschke C, Savova V, Zemmour D, Saatcioglu HD, et al. Single-cell transcriptomics of human and mouse lung cancers reveals conserved myeloid populations across individuals and species. Immunity (2019) 50(5):1317–34 e10. doi: 10.1016/j.immuni.2019.03.009
46. Bourdely P, Anselmi G, Vaivode K, Ramos RN, Missolo-Koussou Y, Hidalgo S, et al. Transcriptional and functional analysis of CD1c(+) human dendritic cells identifies a CD163(+) subset priming CD8(+)CD103(+) T cells. Immunity (2020) 53(2):335–52 e8. doi: 10.1016/j.immuni.2020.06.002
47. Zaid A, Hor JL, Christo SN, Groom JR, Heath WR, Mackay LK, et al. Chemokine receptor-dependent control of skin tissue-resident memory T cell formation. J Immunol (2017) 199(7):2451–59. doi: 10.4049/jimmunol.1700571
48. Vella JL, Molodtsov A, Angeles CV, Branchini BR, Turk MJ, Huang YH. Dendritic cells maintain anti-tumor immunity by positioning CD8 skin-resident memory T cells. Life Sci Alliance (2021) 4(10):1–17. doi: 10.26508/lsa.202101056
49. Wein AN, McMaster SR, Takamura S, Dunbar PR, Cartwright EK, Hayward SL, et al. CXCR6 regulates localization of tissue-resident memory CD8 T cells to the airways. J Exp Med (2019) 216(12):2748–62. doi: 10.1084/jem.20181308
50. Takamura S, Kato S, Motozono C, Shimaoka T, Ueha S, Matsuo K, et al. Interstitial-resident memory CD8(+) T cells sustain frontline epithelial memory in the lung. J Exp Med (2019) 216(12):2736–47. doi: 10.1084/jem.20190557
51. Mandai Y, Takahashi D, Hase K, Obata Y, Furusawa Y, Ebisawa M, et al. Distinct roles for CXCR6(+) and CXCR6(-) CD4(+) T cells in the pathogenesis of chronic colitis. PloS One (2013) 8(6):e65488. doi: 10.1371/journal.pone.0065488
52. Hou L, Yuki K. CCR6 and CXCR6 identify the Th17 cells with cytotoxicity in experimental autoimmune encephalomyelitis. Front Immunol (2022) 13:819224. doi: 10.3389/fimmu.2022.819224
53. Geissmann F, Cameron TO, Sidobre S, Manlongat N, Kronenberg M, Briskin MJ, et al. Intravascular immune surveillance by CXCR6+ NKT cells patrolling liver sinusoids. PloS Biol (2005) 3(4):e113. doi: 10.1371/journal.pbio.0030113
54. Germanov E, Veinotte L, Cullen R, Chamberlain E, Butcher EC, Johnston B. Critical role for the chemokine receptor CXCR6 in homeostasis and activation of CD1d-restricted NKT cells. J Immunol (2008) 181(1):81–91. doi: 10.4049/jimmunol.181.1.81
55. Ma C, Han M, Heinrich B, Fu Q, Zhang Q, Sandhu M, et al. Gut microbiome-mediated bile acid metabolism regulates liver cancer via NKT cells. Science (2018) 360(6391):1–23. doi: 10.1126/science.aan5931
56. Yu H, Yang A, Liu L, Mak JYW, Fairlie DP, Cowley S. CXCL16 stimulates antigen-induced MAIT cell accumulation but trafficking during lung infection is CXCR6-independent. Front Immunol (2020) 11:1773. doi: 10.3389/fimmu.2020.01773
57. Wang B, Wang Y, Sun X, Deng G, Huang W, Wu X, et al. CXCR6 is required for antitumor efficacy of intratumoral CD8(+) T cell. J Immunother Cancer (2021) 9(8):1–13. doi: 10.1136/jitc-2021-003100
58. Demaria S, Kawashima N, Yang AM, Devitt ML, Babb JS, Allison JP, et al. Immune-mediated inhibition of metastases after treatment with local radiation and CTLA-4 blockade in a mouse model of breast cancer. Clin Cancer Res (2005) 11(2 Pt 1):728–34. doi: 10.1158/1078-0432.728.11.2
59. Matsumura S, Wang B, Kawashima N, Braunstein S, Badura M, Cameron TO, et al. Radiation-induced CXCL16 release by breast cancer cells attracts effector T cells. J Immunol (2008) 181(5):3099–107. doi: 10.4049/jimmunol.181.5.3099
60. Mossanen JC, Kohlhepp M, Wehr A, Krenkel O, Liepelt A, Roeth AA, et al. CXCR6 inhibits hepatocarcinogenesis by promoting natural killer T- and CD4(+) T-Cell-Dependent control of senescence. Gastroenterology (2019) 156(6):1877–89 e4. doi: 10.1053/j.gastro.2019.01.247
61. Cullen R, Germanov E, Shimaoka T, Johnston B. Enhanced tumor metastasis in response to blockade of the chemokine receptor CXCR6 is overcome by NKT cell activation. J Immunol (2009) 183(9):5807–15. doi: 10.4049/jimmunol.0803520
62. Christian LS, Wang L, Lim B, Deng D, Wu H, Wang XF, et al. Resident memory T cells in tumor-distant tissues fortify against metastasis formation. Cell Rep (2021) 35(6):109118. doi: 10.1016/j.celrep.2021.109118
63. Feng Z, He X, Zhang X, Wu Y, Xing B, Knowles A, et al. Potent suppression of neuroendocrine tumors and gastrointestinal cancers by CDH17CAR T cells without toxicity to normal tissues. Nat Cancer (2022) 3(5):581–94. doi: 10.1038/s43018-022-00344-7
64. Leidner R, Sanjuan Silva N, Huang H, Sprott D, Zheng C, Shih YP, et al. Neoantigen T-cell receptor gene therapy in pancreatic cancer. N Engl J Med (2022) 386(22):2112–19. doi: 10.1056/NEJMoa2119662
65. Lesch S, Blumenberg V, Stoiber S, Gottschlich A, Ogonek J, Cadilha BL, et al. T Cells armed with c-X-C chemokine receptor type 6 enhance adoptive cell therapy for pancreatic tumours. Nat BioMed Eng (2021) 5(11):1246–60. doi: 10.1038/s41551-021-00737-6
66. Srivastava S, Furlan SN, Jaeger-Ruckstuhl CA, Sarvothama M, Berger C, Smythe KS, et al. Immunogenic chemotherapy enhances recruitment of CAR-T cells to lung tumors and improves antitumor efficacy when combined with checkpoint blockade. Cancer Cell (2021) 39(2):193–208 e10. doi: 10.1016/j.ccell.2020.11.005
67. Sandoval F, Terme M, Nizard M, Badoual C, Bureau MF, Freyburger L, et al. Mucosal imprinting of vaccine-induced CD8+ T cells is crucial to inhibit the growth of mucosal tumors. Sci Transl Med (2013) 5(172):172ra20. doi: 10.1126/scitranslmed.3004888
68. Nizard M, Roussel H, Diniz MO, Karaki S, Tran T, Voron T, et al. Induction of resident memory T cells enhances the efficacy of cancer vaccine. Nat Commun (2017) 8:15221. doi: 10.1038/ncomms15221
69. Blanc C, Hans S, Tran T, Granier C, Saldman A, Anson M, et al. Targeting resident memory T cells for cancer immunotherapy. Front Immunol (2018) 9:1722. doi: 10.3389/fimmu.2018.01722
70. Tartour E, Johannes L. STxB as an antigen delivery tool for mucosal vaccination. Toxins (Basel) (2022) 14(3):1–20. doi: 10.3390/toxins14030202
71. Matsumura S, Demaria S. Up-regulation of the pro-inflammatory chemokine CXCL16 is a common response of tumor cells to ionizing radiation. Radiat Res (2010) 173(4):418–25. doi: 10.1667/RR1860.1
72. Darash-Yahana M, Gillespie JW, Hewitt SM, Chen YY, Maeda S, Stein I, et al. The chemokine CXCL16 and its receptor, CXCR6, as markers and promoters of inflammation-associated cancers. PloS One (2009) 4(8):e6695. doi: 10.1371/journal.pone.0006695
73. Pfister D, Nunez NG, Pinyol R, Govaere O, Pinter M, Szydlowska M, et al. NASH limits anti-tumour surveillance in immunotherapy-treated HCC. Nature (2021) 592(7854):450–56. doi: 10.1038/s41586-021-03362-0
74. Luoma AM, Suo S, Williams HL, Sharova T, Sullivan K, Manos M, et al. Molecular pathways of colon inflammation induced by cancer immunotherapy. Cell (2020) 182(3):655–71 e22. doi: 10.1016/j.cell.2020.06.001
75. Uza N, Nakase H, Yamamoto S, Yoshino T, Takeda Y, Ueno S, et al. SR-PSOX/CXCL16 plays a critical role in the progression of colonic inflammation. Gut (2011) 60(11):1494–505. doi: 10.1136/gut.2010.221879
76. Ayers M, Lunceford J, Nebozhyn M, Murphy E, Loboda A, Kaufman DR, et al. IFN-gamma-related mRNA profile predicts clinical response to PD-1 blockade. J Clin Invest (2017) 127(8):2930–40. doi: 10.1172/JCI91190
77. Cristescu R, Mogg R, Ayers M, Albright A, Murphy E, Yearley J, et al. Pan-tumor genomic biomarkers for PD-1 checkpoint blockade-based immunotherapy. Science (2018) 362(6411):1–27. doi: 10.1126/science.aar3593
78. Lee LN, Ronan EO, de Lara C, Franken KL, Ottenhoff TH, Tchilian EZ, et al. CXCR6 is a marker for protective antigen-specific cells in the lungs after intranasal immunization against mycobacterium tuberculosis. Infect Immun (2011) 79(8):3328–37. doi: 10.1128/IAI.01133-10
79. Jiang JQ, He XS, Feng N, Greenberg HB. Qualitative and quantitative characteristics of rotavirus-specific CD8 T cells vary depending on the route of infection. J Virol (2008) 82(14):6812–9. doi: 10.1128/JVI.00450-08
80. Wu Q, Kang S, Huang J, Wan S, Yang B, Wu C. Antigen-specific tissue-resident memory T cells in the respiratory system were generated following intranasal vaccination of mice with BCG. J Immunol Res (2021) 2021:6660379. doi: 10.1155/2021/6660379
81. Latta M, Mohan K, Issekutz TB. CXCR6 is expressed on T cells in both T helper type 1 (Th1) inflammation and allergen-induced Th2 lung inflammation but is only a weak mediator of chemotaxis. Immunology (2007) 121(4):555–64. doi: 10.1111/j.1365-2567.2007.02603.x
82. Benchetrit F, Ciree A, Vives V, Warnier G, Gey A, Sautes-Fridman C, et al. Interleukin-17 inhibits tumor cell growth by means of a T-cell-dependent mechanism. Blood (2002) 99(6):2114–21. doi: 10.1182/blood.V99.6.2114
83. Tartour E, Fossiez F, Joyeux I, Galinha A, Gey A, Claret E, et al. Interleukin 17, a T-cell-derived cytokine, promotes tumorigenicity of human cervical tumors in nude mice. Cancer Res (1999) 59(15):3698–704.
84. Martin F, Apetoh L, Ghiringhelli F. Controversies on the role of Th17 in cancer: a TGF-beta-dependent immunosuppressive activity? Trends Mol Med (2012) 18(12):742–9. doi: 10.1016/j.molmed.2012.09.007
85. Oudard S, Benhamouda N, Escudier B, Ravel P, Tran T, Levionnois E, et al. Decrease of pro-angiogenic monocytes predicts clinical response to anti-angiogenic treatment in patients with metastatic renal cell carcinoma. Cells (2021) 11(1):1–15. doi: 10.3390/cells11010017
86. Molina-Cerrillo J, Alonso-Gordoa T, Garrido P. Tumor-associated macrophages: “Good cop-bad cop”. Cancer (2019) 125(11):1941–42. doi: 10.1002/cncr.32016
87. Rossi JF, Lu ZY, Massart C, Levon K. Dynamic Immune/Inflammation precision medicine: The good and the bad inflammation in infection and cancer. Front Immunol (2021) 12:595722. doi: 10.3389/fimmu.2021.595722
Keywords: CXCR6, CXCL16, resident memory T cell, dendritic cell, mucosal vaccination, CAR T cell, immunotherapy
Citation: Mabrouk N, Tran T, Sam I, Pourmir I, Gruel N, Granier C, Pineau J, Gey A, Kobold S, Fabre E and Tartour E (2022) CXCR6 expressing T cells: Functions and role in the control of tumors. Front. Immunol. 13:1022136. doi: 10.3389/fimmu.2022.1022136
Received: 18 August 2022; Accepted: 27 September 2022;
Published: 12 October 2022.
Edited by:
Yang Sun, Nanjing University, ChinaReviewed by:
Yu Tian, ShengJing Hospital of China Medical University, ChinaCopyright © 2022 Mabrouk, Tran, Sam, Pourmir, Gruel, Granier, Pineau, Gey, Kobold, Fabre and Tartour. This is an open-access article distributed under the terms of the Creative Commons Attribution License (CC BY). The use, distribution or reproduction in other forums is permitted, provided the original author(s) and the copyright owner(s) are credited and that the original publication in this journal is cited, in accordance with accepted academic practice. No use, distribution or reproduction is permitted which does not comply with these terms.
*Correspondence: Eric Tartour, ZXJpYy50YXJ0b3VyQGFwaHAuZnI=