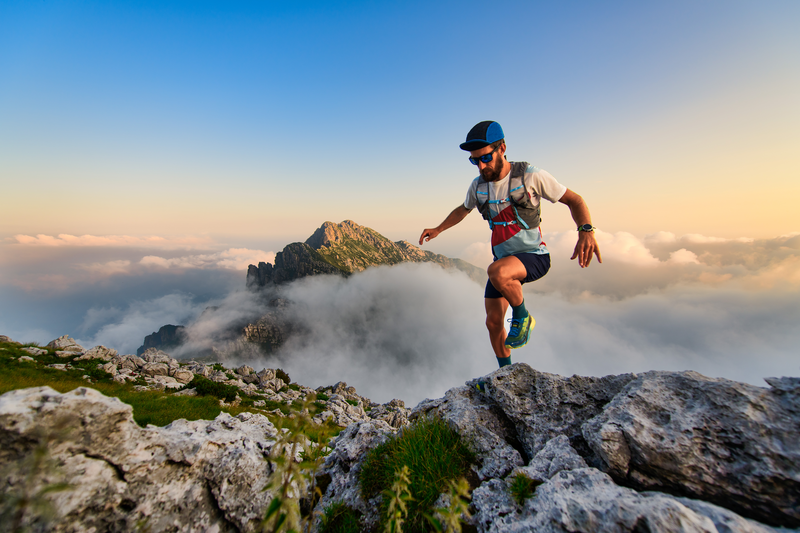
94% of researchers rate our articles as excellent or good
Learn more about the work of our research integrity team to safeguard the quality of each article we publish.
Find out more
REVIEW article
Front. Immunol. , 15 September 2022
Sec. Immunological Tolerance and Regulation
Volume 13 - 2022 | https://doi.org/10.3389/fimmu.2022.1021634
This article is part of the Research Topic The Role of Epigenetic Modification in MDSC Differentiation and Function View all 5 articles
Myeloid-derived suppressor cells (MDSCs) are a group of bone marrow derived heterogeneous cells, which is known for their immunosuppressive functions especially in tumors. Recently, MDSCs have receiving increasing attention in pathological conditions like infection, inflammation and autoimmune diseases. Inflammatory bowel diseases (IBD) are a series of immune-dysfunctional autoimmune diseases characterized by relapsing intestinal inflammation. The role of MDSCs in IBD remains controversial. Although most studies in vitro demonstrated its anti-inflammatory effects by inhibiting the proliferation and function of T cells, it was reported that MDSCs failed to relieve inflammation but even promoted inflammatory responses in experimental IBD. Here we summarize recent insights into the role of MDSCs in the development of IBD and the potential of MDSCs-targeted therapy.
Myeloid-derived suppressor cells (MDSCs) are a general term for the group of heterogeneous cells derived from bone marrow, which are generally considered to be immunosuppressive especially in a tumor-related context by inhibiting T cells and regulating immune responses (1). The term was first introduced by Gabrilovich in 2007 (2). Before 2007, these cells were described in various studies and named as natural suppressor cells or immature myeloid cells (IMCs) (3–7). Normally, IMCs act as the precursors of dendritic cells, macrophages and granulocytes to regulate immune responses. Few IMCs can be detected in the peripheral blood of healthy individuals. However, under pathological conditions such as tumors, infection or inflammation, the maturation of IMCs is interrupted. IMCs remain at different stages of differentiation, namely MDSCs (8–10). Commonly, MDSCs are divided into two groups according to their phenotypic features: granulocyte-like MDSCs (G-MDSC or PMN-MDSC) and monocyte-like MDSCs (M-MDSCs). For mice, MDSCs are considered to be CD11b+Gr-1+ and divided into G-MDSCs and M-MDSCs according to their binding specificity to different epitopes (Ly6G and Ly6C) of Gr-1, a myeloid differentiation antigen. Murine G-MDSCs are usually considered to be CD11b+Ly6G+Ly6Clow while M-MDSCs are CD11b+Ly6G-Ly6Chigh. Given the absence of Gr-1 genes in human, human G-MDSCs are considered as CD11b+CD14-CD15+ while M-MDSCs are HLA-DR-/lowCD11b+CD14+CD15- (7–9, 11). There also exists a subpopulation of human MDSCs named early-stage MDSCs (eMDSCs), immature MDSCs or promyelocytic MDSCs. eMDSCs comprise more immature progenitors and are considered to be HLA-DR-CD33+CD11b+Lin-1- in human (8). G-MDSCs and M-MDSCs are more differentiated than eMDSCs, but lack efficient markers to be differed from the mature fraction of monocytes and granulocytes.
Under pathological conditions, MDSCs proliferate greatly and are recruited to the lesion area to elicit their functional roles (10, 12–15). The expansion of MDSCs is mainly regulated by the JAK/STAT3 pathway (16–18). Cytokines inducing the expansion of MDSCs include prostaglandin E (PGE), cyclooxygenase-2 (COX2), prostaglandin-like stem cell factor (SCF), macrophage colony-stimulating factor (M-CSF), IL-6, granulomonocyte colony-stimulating factor (GM-CSF) and vascular endothelial cell growth factor (VEGF) (19–23). In addition to the expansion of MDSCs, the activation of MDSCs is also important for exerting their immune functions. Cytokines such as IFN-γ, and TGF-β are produced by activated T cells, impaired bacterial or tumor cells, and activate different signaling pathways in MDSCs (24–28). Blockade of these cytokines can remove MDSCs-mediated immunosuppression by inhibiting related signals such as STAT6, STAT1 and NF-κB (29–32).
Commonly, MDSCs are considered to be immunosuppressive and inhibit adaptive immune responses by inhibiting T cells or inducing Treg cells (21, 33–35). MDSCs can also inhibit innate immune responses by inhibiting the cytotoxicity of natural killer cells (NK cells), polarizing macrophages towards the anti-inflammatory phenotype via TGF-β, IL-10 and so on (36–38). However, the role of MDSCs is not always immunosuppressive. MDSCs were observed to activate NK cells by expressing RAE-1 (Retinoic acid early transcript 1) ligands for the NK cell group 2 member D receptor, which in turn dampened MDSCs (39). The contrast results may result from different phenotypes of MDSCs. Therefore, identifying specific molecules expressed by MDSCs to elicit immunosuppressive functions can be one of the future directions.
Inflammatory bowel diseases (IBD), consisted of Crohn’s disease (CD) and ulcerative colitis (UC), are an autoimmune disease characterized by chronic, transmural and relapsing gastrointestinal inflammation, and associated with a high risk of developing colorectal cancer (40–42). IBD can be divided into active and remission stages according to the disease activity. The etiology and detailed mechanism of the imbalance between anti-inflammatory and pro-inflammatory states remain to be elusive in IBD, making it still incurable today. Extensive infiltration of immune cells in intestines has been observed in IBD, reflecting their crucial roles in the disease development (43–45). Therefore, as an important immune cell, the potential role of MDSCs in IBD has gradually attracted attention from researchers, and controversial results were observed. In the review, we will demonstrate the existing studies of MDSCs in IBD reporting either its pro-inflammatory roles or anti-inflammatory roles, along with the application of MDSCs targeted therapies in IBD.
Human MDSCs denoted as CD14+HLA-DR-/low cells were first observed to accumulate in the peripheral blood of patients with active Crohn’s disease (CD) and ulcerative colitis (UC) in 2008 (46). This study enrolled 18 active UC, 21 active CD and 12 healthy controls. Compared with MDSCs from healthy controls, MDSCs from IBD patients exhibited more powerful immunosuppressive function by expressing arginine-1 (Arg-1) and inhibiting the IFN-γ release of anti-CD3/CD28-stimulated autologous peripheral blood mononuclear cells in vitro. The above findings were corroborated by another study in 2015, in which 33 CD patients including 16 active and 17 inactive ones, 36 UC patients including 21 active and 15 inactive ones, and 36 healthy controls were enrolled (47). In the study, CD14+HLA-DR-/low cells were identified as human M-MDSCs, while CD33+CD11b+HLA-DR- cells were identified as G-MDSCs. Consistent with the previous study, more M-MDSCs, namely CD14+HLA-DR-/low cells, were observed in the peripheral blood of IBD patients. Besides, its frequency was positively correlated with the disease activity. However, as for G-MDSCs, namely CD33+CD11b+HLA-DR- cells, researchers demonstrated that no significant difference was found between healthy controls and CD or UC patients. Although the study reported opposite results concerning human G-MDSCs and M-MDSCs in IBD, it should be noticed that the identification of G-MDSCs was not standard enough. Following the definition of M-MDSCs in this study, another study conducted in 2021 confirmed the significant increase of CD14+HLA-DR-/low MDSCs in UC by examining 36 UC patients and 34 healthy controls (48). On the other hand, another study conducted in 2017 focused on CD33+CD15+CD14–HLA-DR–/low MDSCs in IBD patients (49). The phenotype of MDSCs in the study is similar to G-MDSCs studied in the previous study (47). The study enrolled 25 UC patients including 12 active and 13 inactive ones, 22 CD patients including 11 active and 11 inactive ones, as well as 19 healthy controls. Significant differences in the frequency and number of CD33+CD15+CD14–HLA-DR–/low MDSCs were observed between healthy controls and active CD or UC patients. No significant difference was observed between healthy controls and inactive CD or UC. Moreover, CD33+CD15+CD14–HLA-DR–/low MDSCs purified from the peripheral blood of IBD patients promoted the proliferation of autologous sorted CD4+CD25– T cells in vitro. The findings raised an intriguing question about the function of MDSCs in IBD. Overall, the above studies demonstrated the accumulation of MDSCs in human IBD, and revealed that CD14+HLA-DR-/low MDSCs from the peripheral blood of IBD patients inhibited anti-CD3/CD28–stimulated autologous mononuclear cells while CD33+CD15+CD14–HLA-DR–/low MDSCs promoted the proliferation of autologous T cells. However, there remained a paucity in the field of human MDSCs in IBD. The identification of MDSCs including G-MDSCs and M-MDSCs varied in the above studies and the population sizes in the studies were relatively small. More studies are required to explore different functional roles of the subsets of MDSCs in IBD patients based on a more acute classification.
Despite of few studies of MDSCs in IBD patients, a number of studies employed murine colitis models to explore the functional roles of MDSCs. Dextran sulfate sodium (DSS)-induced, 4,6-trinitrobenzene sulfonic acid (TNBS)-induced, T cell transfer-induced or genetic defects-induced spontaneous murine colitis models were established to mimic the pathology of IBD (50). Murine MDSCs were identified as CD11b+Gr-1+ cells in most studies. Besides, G-MDSCs were identified to be CD11b+Ly6G+ and M-MDSCs were to be CD11b+Ly6C+. Haile et al. first identified the immunosuppressive role of MDSCs by employing DSS-induced and T cell transfer-induced murine colitis models in VILLIN-hemagglutinin (HA) transgenic mice of BALB/c background (46). The researchers showed that repetitive transfer of clone 4/T-cell receptor (CL4-TCR) transgenic CD8+ T cells into VILLIN-HA mice led to a significant increase in MDSCs frequency in spleens and intestines compared with a single transfer of T cells. Consistently, repetitive T cell transfer decreased the severity of inflammation. However, MDSCs frequency did not significantly increase in spleens or mesenteric lymph nodes in DSS-induced VILLIN-HA transgenic mice. The increase of GM-CSF and IFN-γ in intestines was only observed in T cell transfer-induced rather than DSS-induced VILLIN-HA transgenic mice, implying that GM-CSF and IFN-γ were involved in MDSC generation. Consistently, several studies confirmed the increase of MDSCs in the peripheral blood, bone marrow and spleens by establishing chronic colitis in C.B-17 SCID mice after adoptive transfer of CD4+CD45RBhigh T cells, or in BALB/c mice after DSS administration (47, 51). Besides, it was reported that the number of MDSCs significantly increased especially at the recovery phase of DSS-induced chronic colitis, thus contributing to the alleviation of inflammation (52, 53). As for the acute colitis induced by DSS or TNBS, the proportion of CD11b+Gr-1+ MDSCs and its subsets (CD11b+Ly6C+ M- and CD11b+Ly6G+ G-MDSCs) also increased in spleens and lamina propria and positively correlated with intestinal inflammatory scores (54).
To explore the interaction between MDSCs and T cells, Kurmaeva et al. isolated CD11b+Ly6G-Ly6Chigh MDSCs, namely M-MDSCs from recombination-activating gene 1 deficient (RAG-1-/-) mice with chronic colitis induced by T cell transfer, and added the group of M-MDSCs to activated CD4+ T cells in vitro (55). As expected, M-MDSCs suppressed T cell proliferation at a 1:1 ratio, while other subsets of CD11b+Gr-1+ MDSCs did not. Besides, the suppression was reversed after administrating an inducible nitric oxide synthase (iNOS)-specific inhibitor or immunoneutralization of IFN-γ, other than an arginase-1 inhibitor or excess of L-arginine. Further experiment revealed that the inhibition of T cells by M-MDSCs was mediated by cell contact, nitric oxide (NO), partially IFN-γ and prostaglandins. Furthermore, the co-culture of MDSCs and CD4+ T cells enhanced the generation of Th17 and foxp3+ T cells. However, the co-culture of MDSCs and Th1/Th17 CD4+ T effector cells isolated from inflamed colon lamina propria had no effect on the levels of IFN-γ, IL-2, IL-6, IL-17 and GM-CSF although the proliferation of T effector cells was inhibited. Taken together, the researchers identified the immunosuppressive function of MDSCs to restrain Th1 responses and trigger the polarization of activated T cells towards Th17 or an inducible Treg phenotype, which was not sufficient to prevent the development of colitis. All studies mentioned above mostly drew conclusions based on in vitro and gene-expression data, so that their conclusions are limited. Overall, the activation of MDSCs may be aimed at achieving homeostasis by suppressing the over activation of T cells but not capable of switching the immune environment in chronic inflammation into an anti-inflammatory state.
Although most studies proposed the immunosuppressive effect of MDSCs, several studies illustrated their pro-inflammatory roles under the inflammatory conditions of murine colitis. Varol et al. reported that adoptively transferred bone marrow-derived CD11bhighLy6Chigh MDSCs were transformed into pro-inflammatory CX3CR1+CD11b+ dendritic cells in mice with systemic depletion of intestinal dendritic cells (56). Moreover, these cells promoted intestinal inflammation after DSS administration. Rivollier et al. isolated CD115+CD11bhighLy6Chigh cells from the bone marrow of congenic (CD45.1+) mice and adoptively transferred them into recipient steady-state mice and chronic colitis mice (57). The results showed that these cells were selectively differentiated into anti-inflammatory F4/80highCX3CR1highCD11c+CD11b+CD103- macrophages in noninflamed colons and pro-inflammatory CD103-CX3CRintCD11b+ dendritic cells in inflamed colons respectively. Similarly, Ostanin et al. found that CD11b+Ly6CintGr-1+ cells isolated from colons of chronic colitis mice expressed enhanced levels of MHC-II and CD86, acting as antigen-presenting cells to induce T cell activation in vitro (58). The above studies claimed that MDSCs exerted a pro-inflammatory effect by differentiating into pro-inflammatory dendritic cell-like cells under the inflammatory environment. As mentioned earlier, Kontaki et al. declared in 2017 that CD33+CD15+CD14–HLA-DR–/low MDSCs isolated from IBD patients enhanced autologous T cell proliferation (49). They also established a TNBS-induced acute colitis model in Balb/c mice. CD11b+Gr-1+Ly6G+ MDSCs, namely G-MDSCs, increased significantly in the acute phase of TNBS-induce colitis while CD11b+Gr-1+Ly6C+ MDSCs, namely M-MDSCs, did not. MDSCs were isolated from bone marrows and spleens of colitis mice to detect the level of C/EBPβ, a master regulator of MDSCs’ suppressive function (59). Subsequently the absence of C/EBPβ suggested the loss of immunosuppressive function of MDSCs and implied that complex inflammatory environment in vivo altered the suppressive capacity of MDSCs. In addition, Tachibana et al. established a IL10-/-IL17A-/- spontaneous murine colitis model, eliciting a stronger inflammatory storm than the IL10-/- spontaneous murine colitis model (60). Noteworthy is that, the number of MDSCs including G-MDSCs and M-MDSCs also significantly increased in the bone marrows, spleens and peripheral blood of IL10-/-IL17A-/- mice. Both G-MDSCs and M-MDSCs expressed more iNOS and less Arg-1, releasing NO into the circulation to exacerbate colitis, probably relying on NO-induced disruption of the gut microbiota. Cell experiments revealed that G-MDSCs from IL10-/-IL17A-/- mice strongly inhibited the proliferation of CD4+ T cells but weakly inhibited the proliferation of CD8+ T cells. Furthermore, IFN-γ derived from CD8+ T cells enhanced the expression of iNOS in G-MDSCs to exacerbate inflammation in IL10-/-IL17A-/- mice.
Generally, these studies demonstrated the dual role of MDSCs in colitis and were briefly summarized in Table 1. Although most studies identified the immunosuppressive role of MDSCs in experimental IBD, the functional plasticity of heterogeneous populations was also observed. The disparity in the conclusions could be attributed to different disease stages, mice background, modeling time, drug brand and dosage used for modeling, and the difficulty in differing MDSCs from neutrophils phenotypically. Currently, most studies of MDSCs in IBD focused on its interaction with adaptive immune cells like T cells, Th1 cells, Th17 cells and Treg cells. A few studies demonstrated the relationship between MDSCs and innate immune cells like dendritic cells and macrophages. For adaptive immune cells, MDSCs inhibited the proliferation of CD4+ T cells at a 1:1 ratio by cell contact, NO, IFN-γ and prostaglandins and triggered the polarization of T cells towards Th17 or an inducible Treg phenotype (55). In turn, Treg cells inhibited the conversion of MDSCs into G-MDSCs depending on the expression of Tgfbr2 genes in MDSCs and secreted TGF-β to promote the expansion of MDSCs (61). MDSCs could also elicit its functional roles by secreting exosomes. Therefore, it was reported that the frequency of Th1 cells decreased after the administration of G-MDSCs-derived exosomes in experimental IBD (62). For innate immune cells, MDSCs inhibited the migration of CD11b+Ly6C+ macrophages towards colon lamina propria in order to restrict inflammation in IBD (52). However, MDSCs from inflamed colon lamina propria of IBD failed to inhibit the inflammatory effects of T cells (55). Furthermore, they could be differentiated into dendritic cell-like cells, acting as antigen-presenting cells to induce T cell activation (56–58). The relationship between MDSCs and NK cells has not been studied in IBD yet whereas other studies demonstrated the crosstalk between MDSCs and NK cells in tumors (36–39).
Considering the immunosuppressive function of MDSCs, murine colitis models were employed to detect the effects of different MDSCs-targeted methods on IBD. In general, there were three methods to target MDSCs, namely, the use of antibodies to deplete MDSCs, the use of molecules to regulate the expansion or differentiation of MDSCs, and the transfer of MDSCs or vesicles secreted by MDSCs into colitis models. The corresponding studies are summarized in Table 2. For studies using antibodies to deplete MDSCs, it should be noted that other immune cells expressing the antibody-specific markers were also removed. In 2007, Hoffmann et al. depleted MDSCs in colitis mice by intraperitoneal injection of anti-Gr-1 mAb and depleted MDSCs in colitis rats by rabbit polyclonal antiserum against polymorphonuclear neutrophils prior to colitis induction (77). The results showed that colitis was aggravated after the depletion of MDSCs, exhibiting a more rapid weight loss, increased mortality and increased bacterial translocation into cardiac blood, spleen, and mesenteric lymph nodes. Moreover, Nemoto et al. confirmed the detrimental role of MDSCs depletion by long-term administration of anti-Gr-1 mAb to colitic mice at 3 weeks after establishing colitis, which exacerbated inflammation with a systemic expansion of activated CD4+ T cells (51). The aggravation of colitis after MDSCs depletion was further confirmed in chemical-induced colitis by injecting anti-Gr-1 mAb intraperitoneally after colitis induction or performing splenectomy to deplete MDSCs prior to colitis induction (49, 53, 70–72).
A number of studies illustrated the administration of different molecules to treat colitis by regulating MDSCs. Several studies paid attention to natural products. Nagarkatti et al. found a significant expansion of MDSCs triggered by resveratrol and identified resveratrol as a potential therapeutic approach for IBD (63). In the study, resveratrol was orally administrated to IL10-/- spontaneous colitis mice every second day from the 18th weeks of chronic colitis. The number of MDSCs in spleens and colon lamina propria increased after resveratrol treatment. The majority of the MDSCs expressed Arg-1 and minimal levels of iNOS and were more potent at inhibiting T cells. As expected, the number of CD4+CD69+, CD4+CXCR3+ and CD4+CXCR10+ T cells decreased and the colon inflammation was dampened. Ma et al. illustrated that the injection of quercetin, a natural product ubiquitously existing in leaves, fruits or seeds of plants, enhanced the proliferation and the phosphorylation level of MDSCs to alleviate acute colitis after binding to the estrogen receptor (64). Butyrate, derived from anaerobic fermentation of indigestible starch and fibrin in the intestinal lumen, was orally administrated into a DSS-induced colitis model according to the study conducted by Xiao et al. (65). Butyrate assisted MDSCs in alleviating colitis and ultimately reduced the compensatory recruitment of MDSCs to the inflamed colon by inhibiting the upregulation of chemokine CC motif receptor 9 (CCR9).
In addition to natural products, the mediators of immune system were also examined and various inhibitors or agonists were administrated in colitis models. The deficiency of protein tyrosine phosphatase 1b enhanced the expansion of MDSCs by promoting the activity of STAT3 and thus alleviated the inflammation of DSS-induced colitis models (66). Knock-in of glycoprotein130 receptor prevented DSS-treated mice from developing acute colitis by activating STAT3 and promoting the expansion of G-MDSCs dramatically (67). Wang et al. transferred IL-37b gene into mesenchumal stromal cells (abbreviated as MSC-IL37b) and injected MSC-IL37b to DSS-induced colitis models (68). The results showed that the proportion of MDSCs in total splenic mononuclear cells as well as that of Tregs in splenic CD4+ cells significantly increased in MSC-IL-37b-treated colitis mice. IL-37b produced by MSC-IL-37b indirectly promoted the differentiation of MDSCs and Tregs in spleens. Lee et al. illustrated the interaction between Treg cells and MDSCs in IBD in 2016 (61). The study introduced Pdk1flox/floxCD4-Cre mice that can spontaneously develop colitis on the account of impaired function of Tregs. MDSCs from the Pdk1flox/floxCD4-Cre mice exhibited a weaker immunosuppressive function, including lower expression of iNOS, weaker suppression of CD4+ T cell proliferation and less efficient function to induce Treg cells in vitro. Adoptive transfer of wild-type Treg cells into Pdk1flox/floxCD4-Cre mice repaired the immunosuppressive function of MDSCs and attenuated colitis. Consistently, the total number of MDSCs increased, in which the proportion of G-MDSCs reduced while that of M-MDSCs increased, implying the regulatory function of Tregs in MDSCs expansion and differentiation. Besides, researchers demonstrated that Tregs-mediated inhibition of M-MDSCs conversion into G-MDSCs relied on the expression of Tgfbr2 genes in MDSCs. Moreover, in addition to regulating MDSCs differentiation, Tregs-derived TGF-β played a crucial role in enhancing the immunosuppressive function of MDSCs. Besides, M-MDSCs promoted the expansion of Tregs in vivo and in vitro. Therefore, a positive feedback loop between Tregs and MDSCs was established during murine colitis. Shi et al. revealed that INK128, an inhibitor of mammalian target of rapamycin (mTOR), inhibited the differentiation of MDSCs into mature macrophages and elicited the role of MDSCs to inhibit Th1 response and promote Tregs expansion (69). Besides, INK128 promoted the production of IFN-α which enhanced the immunosuppressive function of MDSCs by elevating the levels of Arg-1 and reactive oxygen species (ROS). Therefore, oral administration of INK128 attenuated DSS-induced colitis by targeting MDSCs. Zhou et al. demonstrated that GSK343, a selective inhibitor of enhancer of zeste homolog 2 (EZH2), increased MDSCs in the colonic lamina propria, bone marrows and peripheral blood and alleviated colitis (70). So did GSK126, another EZH2 inhibitor that was evaluated in clinical trials to treat cancer (ClinicalTrials.gov identifier: NCT02082977). In vitro experiments revealed that GSK343 promoted the generation of MDSCs from hematopoietic progenitor cells. Tachibana et al. illustrated that IL17A, a representative cytokine released by Th17 cells, negatively regulated the accumulation of G-MDSCs which was cooperatively promoted by IL17F and serum amyloid A1/2 (SAA1/2) in an IL-10-defcient background (60). Zheng et al. demonstrated that acetylcholine (ACh), a neurotransmitter that decreased in IBD as a result of the impaired enteric nervous system, regulated the differentiation of MDSCs by selectively increasing the frequency of M-MDSCs instead of G-MDSCs both in vivo and in vitro (71). ACh promoted the survival of M-MDSCs under 5-fluorouracil induced apoptosis and elevated the expression of C-C motif chemokine receptor 2 (CCR2) on M-MDSCs which implied the recruitment of MDSCs from circulation. These results partially account for the increase of M-MDSCs after ACh stimulation. Besides, ACh enhanced the IL-10 expression of M-MDSCs by activating the nAChR/ERK pathway, contributing to the inhibition of T cells. ACh administration in DSS-induced acute colitis models via enema significantly increased MDSCs in the colon lamina propria rather than that in the circulation or spleens, suggesting that only local immune environment in colons, instead of systemic immunity, was affected by ACh. Consistently, ACh treatment elicited MDSCs to decrease the generation of Th17 cells in colons and thus ameliorated DSS-induced colitis. The intraperitoneal injection of BQ123, an endothelin-A receptor antagonist, increased G-MDSCs rather than M-MDSCs in healthy mice (72). Meanwhile, BQ123 injection activated the suppressive function of G-MDSCs to alleviate DSS-induced acute colitis via IL13/STAT6/ARG1 pathway. Moreover, the intraperitoneal injection of SMM-189, a cannabinoid receptor 2 (CB2) inverse agonist, increased the number of MDSCs, reduced Th17 cells and alleviated acute colitis (73).
Interestingly, there were studies investigating the potential effect of drugs that were widely used in clinical practice. Lei et al. demonstrated that atorvastatin promoted the expansion of both human HLA-DR-/lowCD11b+CD33+ MDSCs and murine CD11b+Arg-1+ MDSCs in vitro (74). The co-culture of atorvastatin significantly promoted the differentiation of GM-CSF-stimulated murine bone marrow cells towards G-MDSCs rather than M-MDSCs, and decreased the frequency of CD11c+MHC-II+ dendritic cells. It was reported that the expansion of MDSCs by atorvastatin depended on the mevalonate pathway. Similarly, in vivo experiments revealed that the number of G-MDSCs rather than M-MDSCs significantly increased in livers, spleens and peripheral blood of healthy or colitic mice after the intraperitoneal injection of atorvastatin. Besides, in vitro T cell proliferation assays revealed the immunosuppressive functions of these MDSCs. However, the suppressive function was inhibited after adding an iNOS inhibitor, illustrating that atorvastatin-derived MDSCs depended on NO to inhibit the proliferation of T cells. In addition, the administration of sildenafil, an inhibitor of phosphodiesterase-5, alleviated DSS-induced colitis and reduced the number of iNOS+ G-MDSCs in the colons by blocking their recruitment (75).
Attempt to adoptively transfer MDSCs into colitis models was first made in 2008 by Haile et al. (46). MDSCs were isolated from murine colitis induced by repetitive T cell transfer, and were next co-transferred with HA-specific CD8+ T cells into naive VILLIN-HA mice. The inflammation was significantly ameliorated, indicating an anti-inflammatory effect of MDSCs on colitis induced by antigen-specific T cells. Intraperitoneal injection of MDSCs isolated from spleens of colitis mice at the convalescent phase dampened inflammatory responses in DSS-induced acute colitis models by inhibiting the migration of CD11b+ single positive population to colon lamina propria. It was reported that the CD11b+ single positive cells were composed of Ly6ChighLy6Glow macrophages (52). In 2012, Su et al. reported that bone marrow derived G-MDSCs isolated from healthy mice were intravenously injected to TNBS-induced mice and attenuated colitis by decreasing the level of IL-6 in serum and the activity of myeloperoxidase (MPO) in colons (76). Besides, the accumulation of injected G-MDSCs was more significant in inflamed colons than in the spleens and livers, revealing their tendency to migrate towards inflammatory sites in vivo. Consistently, Zhang et al. demonstrated that bone marrow derived MDSCs from healthy mice were injected intravenously after colitis induction by DSS and significantly attenuated colitis (66). Guan et al. also confirmed the therapeutic effects of transferred MDSCs either isolated from colitis mice or generated in vitro on TNBS-induced colitis, which downregulated the expression of pro-inflammatory cytokines in colons (54). In the study, the intravenous injection of MDSCs was prior to TNBS administration, and the total number of MDSCs decreased in spleens of recipient mice after colonic inflammation was controlled. The results strongly suggested the safety of MDSCs transfer because the number of MDSCs in spleens of recipient mice did not increased in the end. In addition, as mentioned earlier, atorvastatin-derived MDSCs were mostly G-MDSCs and significantly inhibited the proliferation of T cells (74). Therefore, G-MDSCs were isolated from DSS-induced or T cell transfer-induced colitis mice after atorvastatin treatment and were intravenously injected to the corresponding colitis models. Inflammation was largely attenuated due to the suppressive function of atorvastatin-derived G-MDSCs, along with a significant decrease of CD4+ T cells. In 2016, Lee et al. examined the therapeutic effects of TGF-β-mediated, in vitro differentiated MDSCs (MDSCsTGF-β) on DSS-induced colitis (61). According to the study, colitis was alleviated after the intravenous injection of bone marrow-derived M-MDSCs or M-MDSCsTGF-β. However, transfer of M-MDSCsTGF-β increased splenic Treg cells in colitis mice and were more efficient at repairing intestinal damages than the transfer of M-MDSCs. Consistently, M-MDSCsTGF-β showed more localization in intestines, accounting for the stronger therapeutic effects. Ma et al. intravenously injected MDSCs isolated from wild type spleens to ArgmyeKO mice (the deficiency of Arg-1 in myeloid cells) with DSS-induced acute colitis and eventually prolonged the survival of these mice (64). Colitis in ArgmyeKO mice were more severe than in wild type mice due to the deficiency of Arg-1 in myeloid cells. After the injection of MDSCs, levels of IL17A instead of IL17F increased in Th17 cells, suggesting that MDSCs released Arg-1 to regulate Th17 cells to inhibit colitis progression in ArgmyeKO mice. Besides, as mentioned earlier, Chen et al. demonstrated that the injection of BQ123, an endothelin-A receptor antagonist, activated G-MDSCs in mice (72). Therefore BQ123-induced G-MDSCs were intravenously transferred to DSS-induced colitis and significantly alleviated inflammation. However, BQ123-induced G-MDSCs failed to alleviate colitis in recombination-activating gene 2 (RAG2) deficient mice with impaired T- and B-cell development, suggesting that BQ123-induced G-MDSCs relied on inhibiting T cells to alleviate inflammation. In addition to MDSCs transfer, transfer of extracellular vesicles, namely exosomes, which were secreted by MDSCs was also examined. Wang et al. isolated exosomes secreted by G-MDSCs from spleens of tumor-bearing mice and intraperitoneally injected G-MDSCs derived exosomes into DSS-induced acute colitis models (62). Due to the activity of Arg-1 in G-MDSCs derived exosomes, the frequency of Treg cells increased and the frequency of Th1 cells decreased respectively in mesenteric lymph nodes of the colitis mice. Therefore, the inflammation was significantly attenuated.
Although most studied confirmed the beneficial effects of MDSCs transfer, Kontaki et al. made contrast conclusions. The study first intrasplenically injected bone marrow derived MDSCs generated in vitro along with DO11.10 T cells or along with ovalbuminpulsed splenic dendritic cells into healthy mice (49). The proliferation and activation of T cells or dendritic cells were inhibited as expected in vivo. However, the suppressive effects of MDSCs either generated in vitro or isolated from TNBS-induced mice failed in TNBS-induced acute colitis mice after intravenous injection. The inflammation in acute colitis was augmented, at least not alleviated by transferred MDSCs. The discrepancy from the study conducted by Guan et al. (54) may be attributed to the differences between murine models. Guan et al. set up a more chronic colitis model by administrating TNBS twice over 1-week interval. Whereas in this study, mice were sacrificed after 3 to 5 days after a single transrectal injection of TNBS, establishing an acute colitis model. Therefore, the pro-inflammatory responses of T cells may not be fully activated yet so that suppressive effects of MDSCs were not observed in the acute colitis model. The study implied that MDSCs transfer may play a better therapeutic role as a maintenance therapy by preventing reactivation of colitis in remission.
Although the number of MDSCs increases in IBD, the suppressive function is not sufficient enough to inhibit colonic inflammation in vivo. Moreover, MDSCs can switch to be pro-inflammatory by differentiating to dendritic cells under acute inflammatory conditions. The dual role of MDSCs has also been reported in other inflammatory and autoimmune diseases like multiple sclerosis or rheumatoid arthritis (78–81). Besides, the immune regulatory effects of MDSCs on T cells can be influenced by various mediators in vivo (Figure 1). Based on the thesis, MDSCs-targeted therapies have been investigated and reported to alleviate IBD in most studies, including administration of bioactive molecules regulating MDSCs, and adoptive transfer of MDSCs or MDSCs-derived exosomes. Moreover, transfer of MDSCs has been shown to be more suitable as a maintenance therapy during the remission stage of colitis according to previous studies.
Figure 1 MDSCs and their immune regulatory effects on T cells. During the recovery phase of IBD, MDSCs exert their suppressive effects on T cells by secreting cytokines such as Arg-1, NO and ROS as well as exosomes expressing these cytokines. Meanwhile, MDSCs induce the increase of Treg cells via IL-10. Treg cells secret TGF-β to enhance the suppressive function of MDSCs in turn and specifically increase the number of M-MDSCs. The activation of signals in MDSCs such as STAT3, STAT6 and C/EBPβ plays a key role in its functioning. Besides, studies have revealed that the administration of resveratrol, quercetin, acetylcholine (ACh) and so on promotes the generation of MDSCs while the activation of zeste homolog 2 (EZH2), mTOR, protein tyrosine phosphatase 1b (PTP1B) and so on inhibits MDSCs. This graph is drawn by Figdraw.
Challenges still exist in the field of MDSCs in IBD. First, given the similarity of MDSCs to monocytes and neutrophils both in phenotype and morphology, lack of specific markers remains one of the biggest controversies in the field of MDSCs. In fact, the definition of MDSCs is not consistent in current studies including M-MDSCs and G-MDSCs. Therefore, contrast conclusions have been made and it is difficult to conclude definitely that the disparity is due to different subsets of MDSCs or phases of the disease. Secondly, the function of M-MDSCs and G-MDSCs in IBD should be described respectively due to the heterogeneity observed in current studies and more studies in vivo are in need. Finally, although the safety and therapeutic effects of MDSCs transfer in experimental IBD have been demonstrated in several studies, it remains to be clear that how these transferred MDSCs influence inflammatory signals in local immune environment of colons and systemic immune system. Besides, the timing to administrate MDSCs transfer is crucial for the suppressive function of MDSCs considering the disease stage. A comprehensive understanding of the interaction between MDSCs and other immune mediators contributing to the intestinal inflammation should provide insights into the design of immunotherapeutic interventions.
FZ and WG drafted the manuscript and designed the figure and Table. JS, ZS, and DC revised the manuscript. ZS and DC conceived the topic. All authors contributed to the article and approved the submitted version.
This work was supported by the National Natural Science Foundation of China (Grant: 81871709, 82170533).
The authors declare that the research was conducted in the absence of any commercial or financial relationships that could be construed as a potential conflict of interest.
All claims expressed in this article are solely those of the authors and do not necessarily represent those of their affiliated organizations, or those of the publisher, the editors and the reviewers. Any product that may be evaluated in this article, or claim that may be made by its manufacturer, is not guaranteed or endorsed by the publisher.
1. Hegde S, Leader AM, Merad M. MDSC: Markers, development, states, and unaddressed complexity. Immunity (2021) 54(5):875–84. doi: 10.1016/j.immuni.2021.04.004
2. Nagaraj S, Gabrilovich DI. Myeloid-derived suppressor cells. Adv Exp Med Biol (2007) 601:213–23. doi: 10.1007/978-0-387-72005-0_22
3. Oseroff A, Okada S, Strober S. Natural suppressor (NS) cells found in the spleen of neonatal mice and adult mice given total lymphoid irradiation (TLI) express the null surface phenotype. J Immunol (1984) 132(1):101–10.
4. Strober S. Natural suppressor (NS) cells, neonatal tolerance, and total lymphoid irradiation: exploring obscure relationships. Annu Rev Immunol (1984) 2:219–37. doi: 10.1146/annurev.iy.02.040184.001251
5. Kusmartsev S, Gabrilovich DI. Role of immature myeloid cells in mechanisms of immune evasion in cancer. Cancer Immunol Immunother (2006) 55(3):237–45. doi: 10.1007/s00262-005-0048-z
6. Kusmartsev S, Nagaraj S, Gabrilovich DI. Tumor-associated CD8+ T cell tolerance induced by bone marrow-derived immature myeloid cells. J Immunol (2005) 175(7):4583–92. doi: 10.4049/jimmunol.175.7.4583
7. Talmadge JE, Gabrilovich DI. History of myeloid-derived suppressor cells. Nat Rev Cancer (2013) 13(10):739–52. doi: 10.1038/nrc3581
8. Bronte V, Brandau S, Chen SH, Colombo MP, Frey AB, Greten TF, et al. Recommendations for myeloid-derived suppressor cell nomenclature and characterization standards. Nat Commun (2016) 7:12150. doi: 10.1038/ncomms12150
9. Yaseen MM, Abuharfeil NM, Darmani H, Daoud A. Recent advances in myeloid-derived suppressor cell biology. Front Med (2021) 15(2):232–51. doi: 10.1007/s11684-020-0797-2
10. Veglia F, Perego M, Gabrilovich D. Myeloid-derived suppressor cells coming of age. Nat Immunol (2018) 19(2):108–19. doi: 10.1038/s41590-017-0022-x
11. Veglia F, Sanseviero E, Gabrilovich DI. Myeloid-derived suppressor cells in the era of increasing myeloid cell diversity. Nat Rev Immunol (2021) 21(8):485–98. doi: 10.1038/s41577-020-00490-y
12. Groth C, Hu X, Weber R, Fleming V, Altevogt P, Utikal J, et al. Immunosuppression mediated by myeloid-derived suppressor cells (MDSCs) during tumour progression. Br J Cancer (2019) 120(1):16–25. doi: 10.1038/s41416-018-0333-1
13. Wang S, Tan Q, Hou Y, Dou H. Emerging roles of myeloid-derived suppressor cells in diabetes. Front Pharmacol (2021) 12:798320. doi: 10.3389/fphar.2021.798320
14. Li Y, Zhang Q, Wu M, Zhang P, Huang L, Ai X, et al. Suppressing MDSC infiltration in tumor microenvironment serves as an option for treating ovarian cancer metastasis. Int J Biol Sci (2022) 18(9):3697–713. doi: 10.7150/ijbs.70013
15. Cheng JN, Yuan YX, Zhu B, Jia Q. Myeloid-derived suppressor cells: A multifaceted accomplice in tumor progression. Front Cell Dev Biol (2021) 9:740827. doi: 10.3389/fcell.2021.740827
16. Dysthe M, Parihar R. Myeloid-derived suppressor cells in the tumor microenvironment. Adv Exp Med Biol (2020) 1224:117–40. doi: 10.1007/978-3-030-35723-8_8
17. Yang Q, Qiu H, Xie H, Qi Y, Cha H, Qu J, et al. A schistosoma japonicum infection promotes the expansion of myeloid-derived suppressor cells by activating the JAK/STAT3 pathway. J Immunol (2017) 198(12):4716–27. doi: 10.4049/jimmunol.1601860
18. Feng L, Li G, An J, Liu C, Zhu X, Xu Y, et al. Exercise training protects against heart failure via expansion of myeloid-derived suppressor cells through regulating IL-10/STAT3/S100A9 pathway. Circ Heart Fail (2022) 15(3):e008550. doi: 10.1161/CIRCHEARTFAILURE.121.008550
19. Saleem SJ, Conrad DH. Hematopoietic cytokine-induced transcriptional regulation and notch signaling as modulators of MDSC expansion. Int Immunopharmacol (2011) 11(7):808–15. doi: 10.1016/j.intimp.2011.03.010
20. Ching MM, Reader J, Fulton AM. Eicosanoids in cancer: Prostaglandin E(2) receptor 4 in cancer therapeutics and immunotherapy. Front Pharmacol (2020) 11:819. doi: 10.3389/fphar.2020.00819
21. Yang T, Li J, Li R, Yang C, Zhang W, Qiu Y, et al. Correlation between MDSC and immune tolerance in transplantation: Cytokines, pathways and cell-cell interaction. Curr Gene Ther (2019) 19(2):81–92. doi: 10.2174/1566523219666190618093707
22. Chen J, Cai S, Li R, Xie J, Yang F, Liu T, et al. Blockade of cycloxygenase-2 ameliorates sepsis induced immune-suppression by regulating myeloid-derived suppressor cells. Int Immunopharmacol (2022) 104:108506. doi: 10.1016/j.intimp.2021.108506
23. Calì B, Agnellini AHR, Cioccarelli C, Sanchez-Rodriguez R, Predonzani A, Toffolo GI, et al. GM-CSF nitration is a new driver of myeloid suppressor cell activity in tumors. Front Immunol (2021) 12:718098. doi: 10.3389/fimmu.2021.718098
24. Ito A, Akama Y, Satoh-Takayama N, Saito K, Kato T, Kawamoto E, et al. Possible metastatic stage-dependent ILC2 activation induces differential functions of MDSCs through IL-13/IL-13Rα1 signaling during the progression of breast cancer lung metastasis. Cancers (Basel) (2022) 14(13):3267. doi: 10.3390/cancers14133267
25. Zhao N, Zhu W, Wang J, Liu W, Kang L, Yu R, et al. Group 2 innate lymphoid cells promote TNBC lung metastasis via the IL-13-MDSC axis in a murine tumor model. Int Immunopharmacol (2021) 99:107924. doi: 10.1016/j.intimp.2021.107924
26. Grover A, Sanseviero E, Timosenko E, Gabrilovich DI. Myeloid-derived suppressor cells: A propitious road to clinic. Cancer Discovery (2021) 11(11):2693–706. doi: 10.1158/2159-8290.CD-21-0764
27. Li K, Shi H, Zhang B, Ou X, Ma Q, Chen Y. Myeloid-derived suppressor cells as immunosuppressive regulators and therapeutic targets in cancer. Signal Transduct Target Ther (2021) 6(1):362. doi: 10.1038/s41392-021-00670-9
28. Cai B, Liu Y, Chong Y, Zhang H, Matsunaga A, Fang X, et al. IRAK1-regulated IFN-γ signaling induces MDSC to facilitate immune evasion in FGFR1-driven hematological malignancies. Mol Cancer (2021) 20(1):165. doi: 10.1186/s12943-021-01460-1
29. Porta C, Consonni FM, Morlacchi S, Sangaletti S, Bleve A, Totaro MG, et al. Tumor-derived prostaglandin E2 promotes p50 NF-κB-Dependent differentiation of monocytic MDSCs. Cancer Res (2020) 80(13):2874–88. doi: 10.1158/0008-5472.CAN-19-2843
30. Han X, Luan T, Sun Y, Yan W, Wang D, Zeng X. MicroRNA 449c mediates the generation of monocytic myeloid-derived suppressor cells by targeting STAT6. Mol Cells (2020) 43(9):793–803. doi: 10.14348/molcells.2020.2307
31. Ryan N, Anderson K, Volpedo G, Hamza O, Varikuti S, Satoskar AR, et al. STAT1 inhibits T-cell exhaustion and myeloid derived suppressor cell accumulation to promote antitumor immune responses in head and neck squamous cell carcinoma. Int J Cancer (2020) 146(6):1717–29. doi: 10.1002/ijc.32781
32. Xu W, Li S, Li M, Yang X, Xie S, Lin L, et al. Targeted elimination of myeloid-derived suppressor cells via regulation of the STAT pathway alleviates tumor immunosuppression in neuroblastoma. Immunol Lett (2021) 240:31–40. doi: 10.1016/j.imlet.2021.09.011
33. Serafini P, Mgebroff S, Noonan K, Borrello I. Myeloid-derived suppressor cells promote cross-tolerance in b-cell lymphoma by expanding regulatory T cells. Cancer Res (2008) 68(13):5439–49. doi: 10.1158/0008-5472.CAN-07-6621
34. Ferrer G, Jung B, Chiu PY, Aslam R, Palacios F, Mazzarello AN, et al. Myeloid-derived suppressor cell subtypes differentially influence T-cell function, T-helper subset differentiation, and clinical course in CLL. Leukemia (2021) 35(11):3163–75. doi: 10.1038/s41375-021-01249-7
35. Tucker SL, Sarr D, Rada B. Granulocytic myeloid-derived suppressor cells in cystic fibrosis. Front Immunol (2021) 12:745326. doi: 10.3389/fimmu.2021.745326
36. Mabuchi S, Yokoi E, Komura N, Kimura T. Myeloid-derived suppressor cells and their role in gynecological malignancies. Tumour Biol (2018) 40(7):1010428318776485. doi: 10.1177/1010428318776485
37. Yang Y, Li C, Liu T, Dai X, Bazhin AV. Myeloid-derived suppressor cells in tumors: From mechanisms to antigen specificity and microenvironmental regulation. Front Immunol (2020) 11:1371. doi: 10.3389/fimmu.2020.01371
38. Tumino N, Di Pace AL, Besi F, Quatrini L, Vacca P, Moretta L. Interaction between MDSC and NK cells in solid and hematological malignancies: Impact on HSCT. Front Immunol (2021) 12:638841. doi: 10.3389/fimmu.2021.638841
39. Nausch N, Galani IE, Schlecker E, Cerwenka A. Mononuclear myeloid-derived “suppressor” cells express RAE-1 and activate natural killer cells. Blood (2008) 112(10):4080–9. doi: 10.1182/blood-2008-03-143776
40. Flynn S, Eisenstein S. Inflammatory bowel disease presentation and diagnosis. Surg Clin North Am (2019) 99(6):1051–62. doi: 10.1016/j.suc.2019.08.001
41. Frigerio S, Lartey DA, D'Haens GR, Grootjans J. The role of the immune system in IBD-associated colorectal cancer: From pro to anti-tumorigenic mechanisms. Int J Mol Sci (2021) 22(23):12739. doi: 10.3390/ijms222312739
42. Noor NM, Sousa P, Paul S, Roblin X. Early diagnosis, early stratification, and early intervention to deliver precision medicine in IBD. Inflammation Bowel Dis (2022) 28(8):1254–64. doi: 10.1093/ibd/izab228
43. Holleran G, Lopetuso L, Petito V, Graziani C, Ianiro G, McNamara D, et al. The innate and adaptive immune system as targets for biologic therapies in inflammatory bowel disease. Int J Mol Sci (2017) 18(10):2020. doi: 10.3390/ijms18102020
44. Guan Q. A comprehensive review and update on the pathogenesis of inflammatory bowel disease. J Immunol Res (2019) 2019:7247238. doi: 10.1155/2019/7247238
45. Ramos GP, Papadakis KA. Mechanisms of disease: Inflammatory bowel diseases. Mayo Clin Proc (2019) 94(1):155–65. doi: 10.1016/j.mayocp.2018.09.013
46. Haile LA, et al. Myeloid-derived suppressor cells in inflammatory bowel disease: a new immunoregulatory pathway. Gastroenterology (2008) 135(3):871–81, 881.e1-5. doi: 10.1053/j.gastro.2008.06.032
47. Xi Q, et al. High frequency of mononuclear myeloid-derived suppressor cells is associated with exacerbation of inflammatory bowel disease. Immunol Invest (2015) 44(3):279–87. doi: 10.3109/08820139.2014.999937
48. Xue G, et al. Tim-4 expressing monocytes as a novel indicator to assess disease activity and severity of ulcerative colitis. Life Sci (2021) 269:119077. doi: 10.1016/j.lfs.2021.119077
49. Kontaki E, et al. Aberrant function of myeloid-derived suppressor cells (MDSCs) in experimental colitis and in inflammatory bowel disease (IBD) immune responses. Autoimmunity (2017) 50(3):170–81. doi: 10.1080/08916934.2017.1283405
50. Katsandegwaza B, Horsnell W, Smith K. Inflammatory bowel disease: A review of pre-clinical murine models of human disease. Int J Mol Sci (2022) 23(16):9344. doi: 10.3390/ijms23169344
51. Nemoto Y, Kanai T, Tohda S, Totsuka T, Okamoto R, Tsuchiya K, et al. Negative feedback regulation of colitogenic CD4+ T cells by increased granulopoiesis. Inflammation Bowel Dis (2008) 14(11):1491–503. doi: 10.1002/ibd.20531
52. Zhang R, Ito S, Nishio N, Cheng Z, Suzuki H, Isobe KI. Dextran sulphate sodium increases splenic Gr1(+)CD11b(+) cells which accelerate recovery from colitis following intravenous transplantation. Clin Exp Immunol (2011) 164(3):417–27. doi: 10.1111/j.1365-2249.2011.04374.x
53. Zhang R, Ito S, Nishio N, Cheng Z, Suzuki H, Isobe K. Up-regulation of Gr1+CD11b+ population in spleen of dextran sulfate sodium administered mice works to repair colitis. Inflamm Allergy Drug Targets (2011) 10(1):39–46. doi: 10.2174/187152811794352114
54. Guan Q, Moreno S, Qing G, Weiss CR, Lu L, Bernstein CN, et al. The role and potential therapeutic application of myeloid-derived suppressor cells in TNBS-induced colitis. J Leukoc Biol (2013) 94(4):803–11. doi: 10.1189/jlb.0113050
55. Kurmaeva E, Bhattacharya D, Goodman W, Omenetti S, Merendino A, Berney S, et al. Immunosuppressive monocytes: possible homeostatic mechanism to restrain chronic intestinal inflammation. J Leukoc Biol (2014) 96(3):377–89. doi: 10.1189/jlb.3HI0613-340RR
56. Varol C, Vallon-Eberhard A, Elinav E, Aychek T, Shapira Y, Luche H, et al. Intestinal lamina propria dendritic cell subsets have different origin and functions. Immunity (2009) 31(3):502–12. doi: 10.1016/j.immuni.2009.06.025
57. Rivollier A, He J, Kole A, Valatas V, Kelsall BL. Inflammation switches the differentiation program of Ly6Chi monocytes from antiinflammatory macrophages to inflammatory dendritic cells in the colon. J Exp Med (2012) 209(1):139–55. doi: 10.1084/jem.20101387
58. Ostanin DV, Kurmaeva E, Furr K, Bao R, Hoffman J, Berney S, et al. Acquisition of antigen-presenting functions by neutrophils isolated from mice with chronic colitis. J Immunol (2012) 188(3):1491–502. doi: 10.4049/jimmunol.1102296
59. Wang W, Xia X, Mao L, Wang S. The CCAAT/Enhancer-binding protein family: Its roles in MDSC expansion and function. Front Immunol (2019) 10:1804. doi: 10.3389/fimmu.2019.01804
60. Tachibana M, Watanabe N, Koda Y, Oya Y, Kaminuma O, Katayama K, et al. Ablation of IL-17A leads to severe colitis in IL-10-deficient mice: implications of myeloid-derived suppressor cells and NO production. Int Immunol (2020) 32(3):187–201. doi: 10.1093/intimm/dxz076
61. Lee CR, Kwak Y, Yang T, Han JH, Park SH, Ye MB, et al. Myeloid-derived suppressor cells are controlled by regulatory T cells via TGF-β during murine colitis. Cell Rep (2016) 17(12):3219–32. doi: 10.1016/j.celrep.2016.11.062
62. Wang Y, Tian J, Tang X, Rui K, Tian X, Ma J, et al. Exosomes released by granulocytic myeloid-derived suppressor cells attenuate DSS-induced colitis in mice. Oncotarget (2016) 7(13):15356–68. doi: 10.18632/oncotarget.7324
63. Singh UP, Singh NP, Singh B, Hofseth LJ, Taub DD, Price RL, et al. Role of resveratrol-induced CD11b(+) gr-1(+) myeloid derived suppressor cells (MDSCs) in the reduction of CXCR3(+) T cells and amelioration of chronic colitis in IL-10(-/-) mice. Brain Behav Immun (2012) 26(1):72–82. doi: 10.1016/j.bbi.2011.07.236
64. Ma Z, Zhen Y, Hu C, Yi H. Myeloid-derived suppressor cell-derived arginase-1 oppositely modulates IL-17A and IL-17F through the ESR/STAT3 pathway during colitis in mice. Front Immunol (2020) 11:687. doi: 10.3389/fimmu.2020.00687
65. Xiao T, Zhang P, Feng T, Lu K, Wang X, Zhou S, et al. Butyrate functions in concert with myeloid-derived suppressor cells recruited by CCR9 to alleviate DSS-induced murine colitis. Int Immunopharmacol (2021) 99:108034. doi: 10.1016/j.intimp.2021.108034
66. Zhang J, Wang B, Zhang W, Wei Y, Bian Z, Zhang CY, et al. Protein tyrosine phosphatase 1B deficiency ameliorates murine experimental colitis via the expansion of myeloid-derived suppressor cells. PloS One (2013) 8(8):e70828. doi: 10.1371/journal.pone.0070828
67. Däbritz J, Judd LM, Chalinor HV, Menheniott TR, Giraud AS. Altered gp130 signalling ameliorates experimental colitis via myeloid cell-specific STAT3 activation and myeloid-derived suppressor cells. Sci Rep (2016) 6:20584. doi: 10.1038/srep20584
68. Wang WQ, Dong K, Zhou L, Jiao GH, Zhu CZ, Li WW, et al. IL-37b gene transfer enhances the therapeutic efficacy of mesenchumal stromal cells in DSS-induced colitis mice. Acta Pharmacol Sin (2015) 36(11):1377–87. doi: 10.1038/aps.2015.51
69. Shi G, Li D, Ren J, Li X, Wang T, Dou H, et al. mTOR inhibitor INK128 attenuates dextran sodium sulfate-induced colitis by promotion of MDSCs on treg cell expansion. J Cell Physiol (2019) 234(2):1618–29. doi: 10.1002/jcp.27032
70. Zhou J, Huang S, Wang Z, Huang J, Xu L, Tang X, et al. Targeting EZH2 histone methyltransferase activity alleviates experimental intestinal inflammation. Nat Commun (2019) 10(1):2427. doi: 10.1038/s41467-019-10176-2
71. Zheng W, Song H, Luo Z, Wu H, Chen L, Wang Y, et al. Acetylcholine ameliorates colitis by promoting IL-10 secretion of monocytic myeloid-derived suppressor cells through the nAChR/ERK pathway. Proc Natl Acad Sci U.S.A. (2021) 118(11):e2017762118. doi: 10.1073/pnas.2017762118
72. Chen Z, Zhang X, Lv S, Xing Z, Shi M, Li X, et al. Treatment with endothelin-a receptor antagonist BQ123 attenuates acute inflammation in mice through T-Cell-Dependent polymorphonuclear myeloid-derived suppressor cell activation. Front Immunol (2021) 12:641874. doi: 10.3389/fimmu.2021.641874
73. Kiran S, Rakib A, Moore BM, Singh UP. Cannabinoid receptor 2 (CB2) inverse agonist SMM-189 induces expression of endogenous CB2 and protein kinase a that differentially modulates the immune response and suppresses experimental colitis. Pharmaceutics (2022) 14(5):936. doi: 10.3390/pharmaceutics14050936
74. Lei A, Yang Q, Li X, Chen H, Shi M, Xiao Q, et al. Atorvastatin promotes the expansion of myeloid-derived suppressor cells and attenuates murine colitis. Immunology (2016) 149(4):432–46. doi: 10.1111/imm.12662
75. Lin S, Wang J, Wang L, Wen J, Guo Y, Qiao W, et al. Phosphodiesterase-5 inhibition suppresses colonic inflammation-induced tumorigenesis via blocking the recruitment of MDSC. Am J Cancer Res (2017) 7(1):41–52.
76. Su H, Cong X, Liu YL. Transplantation of granulocytic myeloid-derived suppressor cells (G-MDSCs) could reduce colitis in experimental murine models. J Dig Dis (2013) 14(5):251–8. doi: 10.1111/1751-2980.12029
77. Kühl AA, Kakirman H, Janotta M, Dreher S, Cremer P, Pawlowski NN, et al. Aggravation of different types of experimental colitis by depletion or adhesion blockade of neutrophils. Gastroenterology (2007) 133(6):1882–92. doi: 10.1053/j.gastro.2007.08.073
78. Schrijver IT, Théroude C, Roger T. Myeloid-derived suppressor cells in sepsis. Front Immunol (2019) 10:327. doi: 10.3389/fimmu.2019.00327
79. Wang Z, Zheng G, Li G, Wang M, Ma Z, Li H, et al. Methylprednisolone alleviates multiple sclerosis by expanding myeloid-derived suppressor cells via glucocorticoid receptor β and S100A8/9 up-regulation. J Cell Mol Med (2020) 24(23):13703–14. doi: 10.1111/jcmm.15928
80. Navashenaq JG, Shabgah AG, Hedayati-Moghadam M, Ariaee N, Mohammadi H, Hemmatzadeh M, et al. The role of myeloid-derived suppressor cells in rheumatoid arthritis: An update. Life Sci (2021) 269:119083. doi: 10.1016/j.lfs.2021.119083
Keywords: myeloid-derived suppressor cells, immune regulation, immunosuppressive function, intestinal inflammation, inflammatory bowel disease
Citation: Zhao F, Gong W, Song J, Shen Z and Cui D (2022) The paradoxical role of MDSCs in inflammatory bowel diseases: From bench to bedside. Front. Immunol. 13:1021634. doi: 10.3389/fimmu.2022.1021634
Received: 17 August 2022; Accepted: 02 September 2022;
Published: 15 September 2022.
Edited by:
Lele Zhu, University of Texas MD Anderson Cancer Center, United StatesReviewed by:
Liuling Xiao, Houston Methodist Research Institute, United StatesCopyright © 2022 Zhao, Gong, Song, Shen and Cui. This is an open-access article distributed under the terms of the Creative Commons Attribution License (CC BY). The use, distribution or reproduction in other forums is permitted, provided the original author(s) and the copyright owner(s) are credited and that the original publication in this journal is cited, in accordance with accepted academic practice. No use, distribution or reproduction is permitted which does not comply with these terms.
*Correspondence: Zhe Shen, c2hlbnpkckB6anUuZWR1LmNu; Dawei Cui, ZGF3ZWljdWlAemp1LmVkdS5jbg==
Disclaimer: All claims expressed in this article are solely those of the authors and do not necessarily represent those of their affiliated organizations, or those of the publisher, the editors and the reviewers. Any product that may be evaluated in this article or claim that may be made by its manufacturer is not guaranteed or endorsed by the publisher.
Research integrity at Frontiers
Learn more about the work of our research integrity team to safeguard the quality of each article we publish.