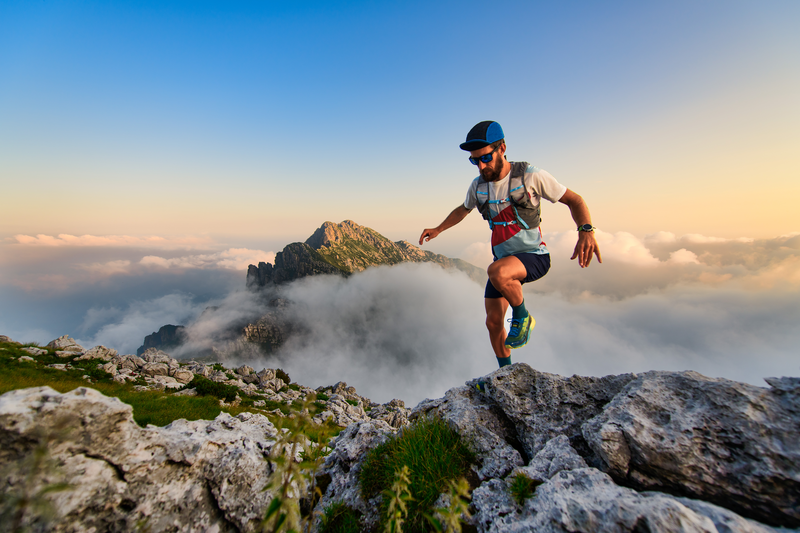
94% of researchers rate our articles as excellent or good
Learn more about the work of our research integrity team to safeguard the quality of each article we publish.
Find out more
REVIEW article
Front. Immunol. , 17 October 2022
Sec. Autoimmune and Autoinflammatory Disorders : Autoimmune Disorders
Volume 13 - 2022 | https://doi.org/10.3389/fimmu.2022.1020891
This article is part of the Research Topic Methods in Autoimmune and Autoinflammatory Disorders: 2022 View all 11 articles
The diagnosis and differential classification of systemic lupus erythematosus (SLE) is difficult, especially in patients with early-onset SLE who are susceptible to systemic multi-organ damage and serious complications and have difficulties in individualized treatment. At present, diagnosis is based mainly on clinical manifestations and the detection of serological antinuclear antibodies. The pathogenesis of SLE involves multiple factors, is clinically heterogeneous, and lacks specific biomarkers. Therefore, it is necessary to identify new biomarkers for the diagnosis and subtype classification of SLE. Non-coding RNAs (ncRNAs) are composed of microRNAs, long non-coding RNAs, small nucleolar RNAs, circular RNAs, and transfer RNAs. They play an important role in the occurrence and development of diseases and are used widely in the early diagnosis and prognosis of autoimmune diseases. In this review, we focus on the research progress in the diagnosis and prognostic assessment of SLE using humoral to tissue level ncRNAs.
Systemic lupus erythematosus (SLE) is a chronic autoimmune disease of unknown etiology that affects nearly all organs and is characterized by immune abnormalities. The prevalence of SLE is approximately 0.02% worldwide (1) and increased nearly three-fold at the end of the 20th century (2), with Israel, the Netherlands, the United States, and other countries showing a large increase (3, 4). Organ damage occurs in 33–50% of SLE patients within 5 years of diagnosis (5). Organ damage and patient mortality also worsen over time (6, 7), seriously affecting quality of life (8, 9) and increasing medical costs (10, 11). At present, the clinically recognized diagnosis is based on symptoms and anti-double-stranded DNA (dsDNA) and anti-Smith (SM) antibody levels after excluding other diagnoses. Due to the complex manifestations of the disease, which affects many organs, there is a high misdiagnosis rate and low diagnosis rate. With the continuous improvement of biotechnology, many studies have found that epigenetic modifications play a role in the pathogenesis of SLE by altering immune homeostasis via the regulation of related protein-coding genes (2, 12, 13). Therefore, it is critical to identify new biomarkers that can be used for early diagnosis, increase risk stratification in screening, and reduce organ damage. Non-coding RNAs (ncRNAs) are RNA molecules that do not encode proteins but participate in gene regulation, including mainly microRNAs (miRNAs), long non-coding RNAs (lncRNAs), small nucleolar RNAs (snoRNAs), circular RNAs (circRNAs), and transfer RNAs (tRNAs). ncRNAs play an important role in the regulation of gene transcription, post-transcription, and translation. The abnormal expression of ncRNAs in plasma, saliva, urine, or tissues is associated with autoimmune and inflammatory diseases. This review summarizes the application of ncRNAs as new markers in the diagnosis and prognostic assessment of SLE from the body fluid to tissue level, in order to develop new and non-invasive biomarkers for the diagnosis of SLE.
At present, some limitations still exist in the clinical diagnosis of SLE. For example, anti-dsDNA and anti-SM antibodies have high specificity for SLE, but anti-SM antibodies have very low sensitivity (14, 15). Anti-dsDNA and anti-SM antibodies are found in approximately 70% and 30% of SLE patients, respectively. Meanwhile, anti-Ro/SSA and anti-La/SSB antibodies are present in approximately 30% and 20% of SLE patients, respectively, but they are generally more strongly associated with Sjogren’s syndrome (14). Anti-U1 RNP antibodies are present in approximately 25% of SLE patients, but high levels of these antibodies are also found in patients with mixed connective tissue disease (14, 15). Anti-ribosomal P protein antibodies have high specificity for SLE, but low sensitivity (16).
Complete blood and classification counts, erythrocyte sedimentation rate, and C-reactive protein are commonly used in the evaluation of active SLE, but these indicators are not specific as infections can elevate their levels. Urinalysis, urine sediment examination, serum creatinine, random urine protein to creatinine ratio, and estimated glomerular filtration rate are commonly used to evaluate lupus nephritis (LN) and its severity.
Studies aiming to discover novel molecular markers based on epigenetics are being published in an endless stream. For example, DNA hypomethylation is detected in interferon (IFN)-regulated genes of lupus primitive T cells, including BST2, IFIT1, IFIT3, IFI44L, MX1, STAT1, TRIM22, and USP18 (17).Coit et al. also validated the type I IFN master regulator gene IRF7 (CD80, HERC5, IFI44, ISG15, ITGAX, and PARP12) as a specific biomarker in patients with LN (18). DNA methylated IFI44L promoter levels have been identified as a sensitive and specific biomarker for the diagnosis of SLE (19). Zhao et al. found that RFX1 inhibits Th17 cell differentiation and thus promotes SLE pathogenesis, possibly by increasing histone H3 acetylation and decreasing DNA methylation and H3K9 demethylation (20). Therefore, it is essential to find new biomarkers to replace traditional biomarkers for auxiliary diagnosis.
The etiology of SLE remains unknown. Studies suggest associations with genetic predisposition, hormonal, immune and environmental factors. The most common source of genetic susceptibility comes from major histocompatibility complex gene loci, such as class I and class II human leukocyte antigen molecules that play roles in immune surveillance, antigen processing and presentation, and complement activation during immune regulation (14). IRF5, PTPN22, SPP1, STAT4, TLR7, and TNFAIP3 are associated with the IFN-α pathway, and SLE susceptibility loci are involved in type I IFN or downstream signaling (21–24). TLR7 and TLR9 are involved in the IFN-α response (25), and immune complexes containing DNA/autoantibodies activate dendritic cells through the combined action of CD32 and TLR9 (26). Similarly, RNA-containing autoantigens activate dendritic cells via TLR7 (27). Both mechanisms include the stimulation of innate immunity (direct B cell production of autoantibodies) by TLR7 (which recognizes RNA) and TLR9 (which recognizes DNA), and stimulation of B cells by T cell activation in response to adaptive immunity and IFN-α (28).
The pathogenic effect of hormones on SLE may be related to their effect on the immune response. Estrogen stimulates the release of B cells, thymocytes, macrophages, CD8+ and CD4+ T cells, certain cytokines (e.g., interleukin [IL]-1), and human leukocyte antigen and endothelial cell adhesion molecule (VCAM and ICAM) expression (29, 30). Progesterone and prolactin also affect immune activity (31, 32). Progesterone reduces the proliferation of T cells and increases the levels of CD8 cells (31), and lupus episodes are associated with hyperprolactinemia (33). In addition, progesterone and high levels of estrogen can induce a Th2 response, which can lead to autoantibody production (34).
With the development of next-generation sequencing technology, an increasing number of studies have suggested that ncRNAs are associated with the occurrence and development of SLE (35). The heterogeneity of ncRNAs in the molecular pathogenesis of SLE is important for clinical practice because identifying these subtypes using different subtype-specific genetic markers can facilitate early diagnosis and personalized treatment for SLE patients.
The regulatory T lymphocyte recognition marker Foxp3 plays an important role in the pathogenesis of SLE. TACI, APRIL, and BR3 mRNA levels are increased in urine samples from SLE patients (36), and urinary Foxp3 mRNA levels can be used to assess LN severity and perform risk stratification (37). Similarly, the urinary and serum levels of CCL2, CCL5, and CXCL10 are increased in active LN patients (38). There is a strong association between SIRT1 expression in urinary granules and anti-dsDNA antibodies in patients with LN (39), which are noninvasive biomarkers of SLE and LN.
TLR-3 and TLR-8 expression are associated with disease severity and susceptibility by increasing the value of C3 and C4,. IFN-α and IFN-β mRNA expression levels in whole blood may be possible biomarkers for SLE (40). MMP-2 mRNA expression is significantly increased in peripheral blood, while MMP-9 and TIMP-1 levels are significantly decreased. The mRNA expression of both correlates with SLE Disease Activity Index (SLEDAI) scores (41). The MMP/TIMP ratio curve changes with the progression of SLE (42). There is also a synergistic increase in the risk of SLE (41, 43). IL-23A and IL-18 expression are increased in SLE peripheral blood, which is positive correlation with SLEDAI scores. IL-12B, TNFA and FOXP3 expression is decreased, which are negatively correlated with SLEDAI scores and associated with LN (44).These mRNAs can be used as potential prognostic and diagnostic biomarkers.
The expression of CD13/APN mRNA is 6.12 times higher in SLE patients than in healthy subjects (45). The mRNA levels of Sema3A and NRP-1 are decreased in serum and peripheral blood mononuclear cells (PBMCs) in SLE patients, with an area under the curve (AUC) of 0.876, sensitivity of 80.6%, and specificity of 77.5% (46). The elevated serum levels of IL-36α and IL-36γ may be involved in SLE arthritis (47, 48). The expression of high-mobility group box protein 1 and TLR4 is increased in the serum and cerebrospinal fluid of patients with neuropsychiatric SLE, and may be diagnostic markers (49).
Adhesion and migration mediated by adhesion molecules are associated with the pathogenesis of SLE, and the high expression of CD44v6 mRNA in PBMCs is correlated with disease duration (50). The mRNA expression of METTL14, ALKBH5, and YTHDF2 is increased in PBMCs of SLE patients. The expression levels of IL-10, STAT4(α), and TNFSF4, are correlated with cardiovascular injury. High levels of MECP2(α) and TNF-α mRNAs are correlated with renal injury. These mRNAs may be involved in the pathogenesis of SLE (51–53).
SLC7A5 mRNA is upregulated in B and T lymphocytes in the peripheral blood of SLE patients and is associated with renal injury (54). Human endogenous retroviral E clone 4-1 mRNA is upregulated in CD4+ T cells and positively correlated with SLE disease activity (55). MZB1 mRNA expression is increased in B cells of active SLE, and MZB1 may be a therapeutic target (56). In conclusion, the abnormal expression of mRNAs in whole blood, PBMCs, serum, and urine of SLE patients has certain application prospects for the early diagnosis and prognostic assessment of the disease. A summary of these potential mRNA biomarkers is shown in Table 1.
miRNAs are important regulators of gene expression that have attracted much attention in recent years. They play an important negative regulatory role in the signaling process of the innate immune response and inflammatory factors. The expression of miRNA-25, miR-1273h-5p, and miR-124-3p, miR-377-3p, and miR-29b is significantly increased in PBMCs of SLE patients (57–59). The levels of circulating miR-21, miR-155, and miR-423 are significantly increased in the peripheral blood of patients with LN, while the level of miR-150 is decreased, which is involved in the pathophysiology of LN (60, 61). miR-132 expression is significantly higher in LN patients compared with non-LN SLE patients, which is helpful for the early non-invasive diagnosis of LN (62). The expression of, hsa-miR-4511, hsa-miR-1260b, hsa-miR-589-3p, and other miRNAs is increased in the peripheral blood of patients with class IV LN (63). miR-99a-3p expression is decreased in the peripheral blood of SLE patients, and miR-99a-3p binds directly to EIF4EBP1 and induces autophagy (64). The expression of miR-146a, miR-155, miR-371b-5p, and miR-5100 is increased in the peripheral blood of SLE patients (65, 66). The expression level of miR-183-5p is positively correlated with SLEDAI scores and the amount of anti-dsDNA antibodies (67).
Serum hsa-miR-766-3p regulates the PI3K-AKT-mTOR pathway in SLE patients and participates in kidney injury (68). miR-181a and miR-223 can be used as serum biomarkers to diagnose SLE and predict LN in Egyptians (69). The high expression of miR-485-5p in the serum of SLE and LN patients is positively correlated with the glomerular filtration rate and serum creatinine, proteinuria, and inflammatory cytokine levels (70). miR-203 expression is decreased in serum samples from patients with active LN, and miR-203 attenuates the activation of IL-β, IL-6, and TNF-α in TRAF6-treated HRMC and HK-2 cells (71).
miR-103, miR-150, and miR-20a levels are significantly reduced in the plasma of SLE patients, which can be used to distinguish healthy people. miR-15b, miR-19b, miR-25, and miR-93 levels are correlated with SLE disease activity, while miR-15b and miR-22 levels are correlated with the glomerular filtration rate (72). The sensitivity and specificity of miR-145 and miR-183 expression levels and anti-dsDNA antibody concentrations in the plasma of patients with different types of LN are 90.5% and 84.2%, respectively (73). The plasma levels of miR-125a, miR-146a, and miR-155 in SLE patients are significantly increased with an AUC of 0.89. miR-146a plasma levels are significantly correlated with anti-dsDNA antibody and proteinuria levels (74).
Ets-1 and miR-326 mRNA expression is increased in CD19+ B cells of SLE patients, and miR-326 may be involved in the pathogenesis of SLE by targeting Ets-1 to promote B cell differentiation (75). miR-633 levels are significantly decreased in CD4+ T cells and negatively correlated with SLEDAI scores. Deletion of miR-633 may be involved in the pathogenesis of SLE by targeting AKT1 to activate the AKT/mTOR pathway (76).
The expression of exosomal miR-21 and miR-155 is upregulated in SLE patients (77), and exosomal miR-146a is associated with the active lupus phase, proteinuria, and histological features, possibly by inhibiting the negative regulation of inflammation by IRAK1 and TRAF6 (78). The levels of miR-31, miR-107, and miR-135b-5p are significantly increased in urine exosomes from patients with proliferative lupus glomerulonephritis with an AUC of 0.783 (79), and the expression of the miRNA let-7a and miR-21 is significantly downregulated, which could be used to guide the clinical staging of patients with LN (80). The increased expression of miR-21 and miR-150 and decreased expression of miR-29c in urine exosomes and cell particles of SLE patients promote renal fibrosis by increasing pro-fibrotic molecules through the SP1 and Smad3/TGF-β pathways (81). miR-3201 and miR-1273e are downregulated in the urine of patients with LN and are involved in the pathogenesis of intravascular glomerular inflammation (82).
In conclusion, the abnormal expression of miRNAs in the blood, exosomes, and immune cells of SLE patients and LN patients has potential significance in early diagnosis and prognostic assessment. The potential miRNA biomarkers used in the diagnosis and prognostic assessment of SLE are summarized in Table 2.
lncRNAs are non-coding RNAs that contain more than 200 nucleotides and extensively regulate biological processes (83). The expression of lncRNAs directly affects in the occurrence and development of SLE and may be related to organ damage, clinical symptoms, and changes in biomarkers of disease activity and progression. The downregulation of the lncRNA NONHSAT101022.2 in the peripheral blood of SLE patients may increase β2-AR signal transduction via cis-regulation of LMBRD2, thereby inducing natural killer cells to produce high levels of IFN-γ and worsening the progression of SLE (84). The expression of AC007278.2 continues to increase in the peripheral blood of SLE patients, which regulates autoimmunity and follicular T helper cell differentiation by affecting the expression of CCR7, AZU1, and TNIP3 (85).
The expression levels of lnc-DC and GAS5 are decreased in the plasma of SLE patients, while the expression of linc0597 is increased. lnc-DC can be used to differentiate LN patients from SLE patients (86). The plasma lncRNAs GAS5, lnc7074, linc0597, linc0640, and linc5150 may be involved in the pathogenesis of SLE through the MAPK pathway (87).
The expression of the lncRNA FAS-AS1 is increased in the serum of SLE patients, which is associated with nephritis and the presence of anti-dsDNA antibodies, while the expression of the lncRNA PVT1 is decreased, which is significantly associated with oral ulcers, photosensitivity, and neurological manifestations (88). The serum levels of ANRIL and NOS3-AS A are increased in patients with atherosclerotic SLE. The expression level of ANRIL is negatively correlated with the duration of SLE, and the expression level of NOS3-AS is negatively correlated with total nitric oxide and high-density lipoprotein levels (89).
lnc0640 is overexpressed in PBMCs of SLE patients, and LNC3643 expression is significantly decreased in SLE patients with arthritis, rash, and pleurisy, and is inversely correlated with SLEDAI scores (90). H19 expression is upregulated in the serum and bone marrow mesenchymal stem cells of SLE patients and positively correlated with SLE disease activity, and thus may regulate bone marrow mesenchymal stem cell-mediated T follicular helper/regulatory T lymphocyte cell balance by inhibiting IL-2 transcription (91). The expression of lnc-FOSB-1:1 in neutrophils is significantly reduced in SLE patients and is associated with the risk of renal involvement (92). The levels of GAS5 and miR-21 are significantly increased in CD4+ T cells of SLE patients, and GAS5 is expressed at a higher level in SLE patients with ulcers (93). The lncRNA Gm20513 positively regulates the expression of H2-Aa in renal tissues of LN mice (94).
In conclusion, the abnormal expression of lncRNAs in the serum, plasma, renal tissue, PBMCs, neutrophils, and bone marrow mesenchymal stem cells of SLE patients, LN patients, and atherosclerotic SLE patients has potential significance in early diagnosis, differentiation of disease types, and assessment of prognosis. The potential lncRNA biomarkers used in the diagnosis and prognostic assessment of SLE are summarized in Table 3.
circRNAs are a class of highly stable ncRNAs that often show evolutionary conservation and tissue-specific expression and are helpful in diagnosing and assessing the prognosis of SLE. hsa_circ_0000479 in PBMCs of SLE patients affects the pathogenesis of SLE by reducing the expression of Wnt-16 protein (95). The expression of circPTPN22, hsa_circ_0044235, and hsa_circ_0068367 is decreased in PBMCs of SLE patients, which is negatively correlated with disease activity (96, 97). The expression levels of hsa_circRNA_100236, hsa_circRNA_101413, and hsa_circRNA_102489 are correlated with the presence of anti-dsDNA antibodies, IgG, and thrombocytopenia, respectively (97). The sensitivity and specificity of hsa_circ_0082688-hsa_circ_0008675 in PBMCs for the diagnosis of new-onset SLE with renal involvement are 79.17% and 96.64% (98), respectively. The expression of hsa_circ_0000479, hsa_circ_0082688, and hsa_circ_0082689 is increased in PBMCs of SLE patients, while hsa_circ_0000175 is decreased significantly. hsa_circ_0000479 has significant value in distinguishing SLE from rheumatoid arthritis, ankylosing spondylitis, and health composition (AUC = 0.825). hsa_circ_0000479 combined with the presence of anti-dsDNA antibodies can effectively distinguish SLE with a sensitivity of 86% and specificity of 100% (99). hsa_circ_104871 expression is significantly downregulated in the blood of SLE patients and negatively correlated with the severity of platelet involvement (100).
The downregulation of circIBTK expression in the peripheral blood of SLE patients is associated with SLEDAI scores and anti-dsDNA antibody and complement C3 levels, which may reverse miR-29b-induced DNA demethylation and AKT signaling activation (101). The downregulation of hsa_circ_0012919 expression in the peripheral blood of SLE patients can reverse the DNA hypomethylation of CD70 and CD11a in CD4+ T cells and regulate the expression of KLF13 and RANTES through miR-125a (102). circRACGAP1 expression is associated with anti-dsDNA antibody and complement C3 levels, which may affect the progression of SLE by binding to miR-22-3p to regulate the AKT signaling pathway (103). The circGARS sponge in the peripheral blood of SLE patients adsorbs miR-19 and activates the nuclear factor-κB (NF-κB) pathway by down-regulating TNFAIP3 expression (104). The expression levels of hsa_circ_0082688 and hsa_circ_0082689 are upregulated in the peripheral blood of SLE patients. The sensitivity, specificity, and accuracy of both circRNAs combined with anti-dsDNA antibody detection in the diagnosis of SLE are 95.65%, 100%, and 98.73% (99), respectively. The upregulation of hsa_circ_0002715 in whole blood of SLE patients is closely related to disease activity and the severity of hematological involvement (105). The increased expression of hsa_circ_0004156 and hsa_circ_0082626 is strongly associated with disease activity, autoantibody production, and clinical symptoms (106).
The expression of circRNA_002453 is significantly increased in the plasma of patients with LN, with a sensitivity of 0.900 and specificity of 0.841 (107). The levels of hsa_circRNA_001308 and hsa_circRNA_407176 are decreased in the plasma and PBMCs of SLE patients. The areas under the receiver operating characteristic curves for the expression of these two circRNAs were 0.662 and 0.599, respectively, in plasma, and were 0.722 and 0.806, respectively, in PBMCs (108). High T cells expressing hsa_circ_0010957 eliminate the expression of the proinflammatory protein IL-6 through the miR-125b/STAT3 signaling in SLE patients (109).
In conclusion, the abnormal expression of circRNAs in the peripheral blood, plasma, PBMCs, and T cells of SLE and LN patients is of potential significance in early diagnosis, identification of disease types, and assessment of prognosis. The potential circRNA biomarkers used in the diagnosis and prognostic assessment of SLE are summarized in Table 4.
The main role of tRNAs is to deliver amino acids to ribosomes for protein synthesis under the direction of mRNAs. A recent study has shown that dysregulated tRNAs and tRNA-derived small RNAs (tsRNAs) are involved in the pathophysiology of human diseases (110). A total of 160 tsRNAs were identified by high-throughput sequencing in LN renal tissue, of which 79 were upregulated and 81 were downregulated. Bioinformatics analysis predicts that tsRNAs may play a role in the pathogenesis of LN through cell adhesion molecules as well as the B-cell receptor, PI3K-AKT, and MAPK signaling pathways (111). A total of 101 tRNAs and 355 tsRNAs were differentially expressed in PBMCs of SLE patients. Gene Ontology analysis and Kyoto Encyclopedia of Genes and Genomes pathway analysis revaled that the changes in the target genes of tRNAs were most enriched in SLE. The target genes altered by tsRNAs were most enriched in Th1 and Th2 cell differentiation, the T cell receptor signaling pathway, and primary immune deficiency. These pathways may play a role in the development of SLE (112). Meanwhile, 482 differentially expressed tRFs were identified in the CD4+ T cells of SLE patients, among which tRF-3009 expression was upregulated and positively correlated with serum IFN-α levels, active LN, and SLEDAI scores (113). The expression of tRF-His-GTG-1 was significantly upregulated in the serum of SLE patients. The sensitivity and specificity of tRF-His-GTG-1 combined with the presence of anti-dsDNA antibodies for the diagnosis of SLE were 83.72% and 94.19%, respectively (114).
In conclusion, the abnormal expression of tRNAs, tsRNAs, tRFs, and tiRNAs in peripheral blood and kidney tissue of SLE patients and LN patients has potential significance in the early diagnosis and prognostic assessment of the disease. The potential tRNA biomarkers used in the diagnosis and prognostic assessment of SLE are summarized in Table 5.
snoRNAs have been shown to direct the chemical modifications of rRNAs (116). In addition, snoRNA-derived fragments have been shown to regulate gene expression and play a role in disease progression (117, 118). Decreased H/ACA box nucleolar RNA12 (SNORA12) expression in the T cells of SLE patients is negatively correlated with the disease activity score. SNORA12 overexpression decreases the expression of histone cluster 1H4 family member K and changes the expression of CD69. IFN-γ secretion is then inhibited and affects the development of SLE (115). The potential snoRNA biomarker used in the diagnosis and prognostic assessment of SLE are summarized in Table 5.
SLE is a heterogeneous autoimmune disease with a mortality rate that is still 2–5 times that of the general population (119, 120). According to the Centers for Disease Control and Prevention mortality data from 2000 to 2015, SLE is among the top 20 leading causes of death in women aged 5–64 years (121). It can affect almost all organs, and patients often undergo multiple remissions and relapses with varying severity, making it sometimes difficult to make a definitive diagnosis. Delayed diagnosis is one of the most critical issues in SLE. Although referral rheumatologists have generally improved in differentiating SLE from multiple immune diseases, the activity of SLE, the diagnosis of LN and disease progression, and even the prediction of target organ damage are not well established. Increased disease activity is sufficient to alter treatment regimens (122–124). However, screening is now routinely performed in many countries, and techniques are under development that will be less invasive and will complement or perhaps even replace the current comprehensive diagnostic approach to SLE. In these new techniques, additional progress will be required to realize early diagnosis and to monitor the disease activity of SLE and LN as well as to identify potential therapeutic targets. Increasing evidence indicates that ncRNAs play a crucial role in the development and progression of SLE. The advent of high-throughput sequencing technology has also further advanced our understanding of the epigenetics and transcriptomics of SLE and other immune diseases. In this review, we described the potential of various RNAs to serve as biomarkers for SLE, perhaps allowing liquid biopsies to replace tissue samples and cell line models. In addition, the simultaneous application of multiple RNA biomarkers has the potential to enhance the sensitivity and specificity of diagnosis and prognosis. The ability to of these biomarkers to detect RNAs in various body fluids is advantageous because it allows for non-invasive diagnosis. There are many studies on RNA biomarkers for SLE, but no unified opinion. Ongoing research aims to determine which noninvasive diagnostic biomarkers for SLE are feasible and cost-effective, to understand which biomarkers can better assess patient outcomes, and to identify more personalized therapeutic targets.
JL, FX, and JF contributed equally to this work. JL and JF: organized the literature and original draft writing; ZH and JS: contributed to literature retrieval and data collation; WL, CH and JS: contributed to manuscript revision; HC and JH: conception, writing review, and approval of the submitted version. All authors contributed to the article and approved the submitted version.
This work was supported by the Medical Science and Technology Research Project of Guangdong Province (Nos. A2022524; A2020304), Science and Technology Program of Guangzhou (Nos. 202102080532; 202002030032; 202002020023), Health Commission Program of Guangzhou (20212A010025; 20201A010085), Science and Technology Project of Panyu, Guangzhou (2021-Z04-053; 2020-Z04-026; 2019-Z04-02), and Scientific Research Project of Guangzhou Panyu Central Hospital (Nos. 2022Y002; 2021Y004; 2021Y002).
We gratefully thank all the editors and reviewers for their valuable contributions.
The authors declare that the research was conducted in the absence of any commercial or financial relationships that could be construed as a potential conflict of interest.
All claims expressed in this article are solely those of the authors and do not necessarily represent those of their affiliated organizations, or those of the publisher, the editors and the reviewers. Any product that may be evaluated in this article, or claim that may be made by its manufacturer, is not guaranteed or endorsed by the publisher.
1. Tsokos GC. Systemic lupus erythematosus. N Engl J Med (2011) 365(22):2110–21. doi: 10.1056/NEJMra1100359
2. Uramoto KM, Michet CJ Jr., Thumboo J, Sunku J, O'Fallon WM, Gabriel SE. Trends in the incidence and mortality of systemic lupus erythematosus, 1950-1992. Arthritis Rheumatol (1999) 42(1):46–50. doi: 10.1002/1529-0131(199901)42:1<46::AID-ANR6>3.0.CO;2-2
3. Rees F, Doherty M, Grainge M, Davenport G, Lanyon P, Zhang W. The incidence and prevalence of systemic lupus erythematosus in the UK, 1999-2012. Ann Rheum Dis (2016) 75(1):136–41. doi: 10.1136/annrheumdis-2014-206334
4. Rees F, Doherty M, Grainge MJ, Lanyon P, Zhang W. The worldwide incidence and prevalence of systemic lupus erythematosus: a systematic review of epidemiological studies. Rheumatol (Oxford) (2017) 56(11):1945–61. doi: 10.1093/rheumatology/kex260
5. Nusbaum JS, Mirza I, Shum J, Freilich RW, Cohen RE, Pillinger MH, et al. Sex differences in systemic lupus erythematosus: Epidemiology, clinical considerations, and disease pathogenesis. Mayo Clin Proc (2020) 95(2):384–94. doi: 10.1016/j.mayocp.2019.09.012
6. Taraborelli M, Cavazzana I, Martinazzi N, Lazzaroni MG, Fredi M, Andreoli L, et al. Organ damage accrual and distribution in systemic lupus erythematosus patients followed-up for more than 10 years. Lupus (2017) 26(11):1197–204. doi: 10.1177/0961203317693096
7. Durcan L, O'Dwyer T, Petri M. Management strategies and future directions for systemic lupus erythematosus in adults. Lancet (2019) 393(10188):2332–43. doi: 10.1016/S0140-6736(19)30237-5
8. D'Cruz DP. Systemic lupus erythematosus. Bmj (2006) 332(7546):890–4. doi: 10.1136/bmj.332.7546.890
9. Lau CS, Mak A. The socioeconomic burden of SLE. Nat Rev Rheumatol (2009) 5(7):400–4. doi: 10.1038/nrrheum.2009.106
10. Chiu YM, Chuang MT, Lang HC. Medical costs incurred by organ damage caused by active disease, comorbidities and side effect of treatments in systemic lupus erythematosus patients: a Taiwan nationwide population-based study. Rheumatol Int (2016) 36(11):1507–14. doi: 10.1007/s00296-016-3551-y
11. Barber MRW, Hanly JG, Su L, Urowitz MB, St Pierre Y, Romero-Diaz J, et al. Economic evaluation of damage accrual in an international systemic lupus erythematosus inception cohort using a multistate model approach. Arthritis Care Res (Hoboken) (2020) 72(12):1800–8. doi: 10.1002/acr.24092
12. Batista PJ, Chang HY. Long noncoding RNAs: cellular address codes in development and disease. Cell (2013) 152(6):1298–307. doi: 10.1016/j.cell.2013.02.012
13. Sigdel KR, Cheng A, Wang Y, Duan L, Zhang Y. The emerging functions of long noncoding RNA in immune cells: Autoimmune diseases. J Immunol Res (2015) 2015:848790. doi: 10.1155/2015/848790
14. Riemakasten G, Hiepe F. Autoantibodies. In: Wallace DJ, Hahn BH, editors. Dubois' lupus erythematosus and related syndromes, 8th ed. Philadelphia: Elsevier (2013). p. 282.
15. Benito-Garcia E, Schur PH, Lahita R. Guidelines for immunologic laboratory testing in the rheumatic diseases: anti-Sm and anti-RNP antibody tests. Arthritis Rheumatol (2004) 51(6):1030–44. doi: 10.1002/art.20836
16. Mahler M, Agmon-Levin N, van Liempt M, Shoenfeld Y, Waka A, Hiepe F, et al. Multi-center evaluation of autoantibodies to the major ribosomal p C22 epitope. Rheumatol Int (2012) 32(3):691–8. doi: 10.1007/s00296-010-1685-x
17. Coit P, Jeffries M, Altorok N, Dozmorov MG, Koelsch KA, Wren JD, et al. Genome-wide DNA methylation study suggests epigenetic accessibility and transcriptional poising of interferon-regulated genes in naïve CD4+ T cells from lupus patients. J Autoimmun (2013) 43:78–84. doi: 10.1016/j.jaut.2013.04.003
18. Coit P, Renauer P, Jeffries MA, Merrill JT, McCune WJ, Maksimowicz-McKinnon K, et al. Renal involvement in lupus is characterized by unique DNA methylation changes in naïve CD4+ T cells. J Autoimmun (2015) 61:29–35. doi: 10.1016/j.jaut.2015.05.003
19. Zhao M, Zhou Y, Zhu B, Wan M, Jiang T, Tan Q, et al. IFI44L promoter methylation as a blood biomarker for systemic lupus erythematosus. Ann Rheum Dis (2016) 75(11):1998–2006. doi: 10.1136/annrheumdis-2015-208410
20. Zhao M, Tan Y, Peng Q, Huang C, Guo Y, Liang G, et al. IL-6/STAT3 pathway induced deficiency of RFX1 contributes to Th17-dependent autoimmune diseases via epigenetic regulation. Nat Commun (2018) 9(1):583. doi: 10.1038/s41467-018-02890-0
21. Bronson PG, Chaivorapol C, Ortmann W, Behrens TW, Graham RR. The genetics of type I interferon in systemic lupus erythematosus. Curr Opin Immunol (2012) 24(5):530–7. doi: 10.1016/j.coi.2012.07.008
22. Kariuki SN, Kirou KA, MacDermott EJ, Barillas-Arias L, Crow MK, Niewold TB. Cutting edge: autoimmune disease risk variant of STAT4 confers increased sensitivity to IFN-alpha in lupus patients in vivo. J Immunol (2009) 182(1):34–8. doi: 10.4049/jimmunol.182.1.34
23. Niewold TB, Kelly JA, Flesch MH, Espinoza LR, Harley JB, Crow MK. Association of the IRF5 risk haplotype with high serum interferon-alpha activity in systemic lupus erythematosus patients. Arthritis Rheumatol (2008) 58(8):2481–7. doi: 10.1002/art.23613
24. Kariuki SN, Crow MK, Niewold TB. The PTPN22 C1858T polymorphism is associated with skewing of cytokine profiles toward high interferon-alpha activity and low tumor necrosis factor alpha levels in patients with lupus. Arthritis Rheumatol (2008) 58(9):2818–23. doi: 10.1002/art.23728
25. Barrat FJ, Meeker T, Gregorio J, Chan JH, Uematsu S, Akira S, et al. Nucleic acids of mammalian origin can act as endogenous ligands for toll-like receptors and may promote systemic lupus erythematosus. J Exp Med (2005) 202(8):1131–9. doi: 10.1084/jem.20050914
26. Means TK, Latz E, Hayashi F, Murali MR, Golenbock DT, Luster AD. Human lupus autoantibody-DNA complexes activate DCs through cooperation of CD32 and TLR9. J Clin Invest (2005) 115(2):407–17. doi: 10.1172/JCI23025
27. Christensen SR, Shlomchik MJ. Regulation of lupus-related autoantibody production and clinical disease by toll-like receptors. Semin Immunol (2007) 19(1):11–23. doi: 10.1016/j.smim.2006.12.005
28. Gensous N, Boizard-Moracchini A, Lazaro E, Richez C, Blanco P. Update on the cellular pathogenesis of lupus. Curr Opin Rheumatol (2021) 33(2):190–6. doi: 10.1097/BOR.0000000000000775
29. Cutolo M, Sulli A, Seriolo B, Accardo S, Masi AT. Estrogens, the immune response and autoimmunity. Clin Exp Rheumatol (1995) 13(2):217–26.
30. Cohen-Solal JF, Jeganathan V, Hill L, Kawabata D, Rodriguez-Pinto D, Grimaldi C, et al. Hormonal regulation of b-cell function and systemic lupus erythematosus. Lupus (2008) 17(6):528–32. doi: 10.1177/0961203308089402
31. Clemens LE, Siiteri PK, Stites DP. Mechanism of immunosuppression of progesterone on maternal lymphocyte activation during pregnancy. J Immunol (1979) 122(5):1978–85.
32. Buskila D, Sukenik S, Shoenfeld Y. The possible role of prolactin in autoimmunity. Am J Reprod Immunol (1991) 26(3):118–23. doi: 10.1111/j.1600-0897.1991.tb00708.x
33. Blanco-Favela F, Quintal-Alvarez G, Leaños-Miranda A. Association between prolactin and disease activity in systemic lupus erythematosus. influence of statistical power. J Rheumatol (1999) 26(1):55–9.
34. Lahita RG. The role of sex hormones in systemic lupus erythematosus. Curr Opin Rheumatol (1999) 11(5):352–6. doi: 10.1097/00002281-199909000-00005
35. Stagakis E, Bertsias G, Verginis P, Nakou M, Hatziapostolou M, Kritikos H, et al. Identification of novel microRNA signatures linked to human lupus disease activity and pathogenesis: miR-21 regulates aberrant T cell responses through regulation of PDCD4 expression. Ann Rheum Dis (2011) 70(8):1496–506. doi: 10.1136/ard.2010.139857
36. Aguirre-Valencia D, Ríos-Serna LJ, Posso-Osorio I, Naranjo-Escobar J, López D, Bedoya-Joaqui V, et al. Expression of BAFF, APRIL, and cognate receptor genes in lupus nephritis and potential use as urinary biomarkers. J Transl Autoimmun (2020) 3:100027. doi: 10.1016/j.jtauto.2019.100027
37. Fayed A, Mohamed A, Ahmed RA, Abouzeid S, Soliman A, Fathy A. Evaluation of urinary FOXP3 mRNA as a biomarker of lupus nephritis in Egyptian patients with systemic lupus erythematosus. Lupus (2021) 30(10):1631–6. doi: 10.1177/09612033211030559
38. Aringer M, Costenbader K, Daikh D, Brinks R, Mosca M, Ramsey-Goldman R, et al. 2019 European league against Rheumatism/American college of rheumatology classification criteria for systemic lupus erythematosus. Ann Rheum Dis (2019) 78(9):1151–9. doi: 10.1136/annrheumdis-2018-214819
39. Olivares D, Perez-Hernandez J, Forner MJ, Perez-Soriano C, Tormos MC, Saez GT, et al. Urinary levels of sirtuin-1 associated with disease activity in lupus nephritis. Clin Sci (Lond) (2018) 132(5):569–79. doi: 10.1042/CS20171410
40. Paradowska-Gorycka A, Wajda A, Stypinska B, Walczuk E, Rzeszotarska E, Walczyk M, et al. Variety of endosomal TLRs and interferons (IFN-α, IFN-β, IFN-γ) expression profiles in patients with SLE, SSc and MCTD. Clin Exp Immunol (2021) 204(1):49–63. doi: 10.1111/cei.13566
41. Vira H, Pradhan V, Umare V, Chaudhary A, Rajadhyksha A, Nadkar M, et al. Role of polymorphisms in MMP-9 and TIMP-1 as biomarkers for susceptibility to systemic lupus erythematosus patients. biomark Med (2019) 13(1):33–43. doi: 10.2217/bmm-2018-0169
42. Vira HJ, Pradhan VD, Umare VD, Chaudhary AK, Rajadhyksha AG, Nadkar MY, et al. Expression of the matrix metalloproteinases MMP-2 and MMP-9 and their inhibitors TIMP-1 and TIMP-2 in systemic lupus erythematosus patients. Neth J Med (2020) 78(5):261–8.
43. Vira HJ, Pradhan VD, Umare VD, Chaudhary AK, Rajadhyksha AG, Nadkar MY, et al. Role of MMP-2 and its inhibitor TIMP-2 as biomarkers for susceptibility to systemic lupus erythematosus. biomark Med (2020) 14(12):1109–19. doi: 10.2217/bmm-2020-0180
44. Miteva LD, Manolova IM, Ivanova MG, Stoilov RM, Stanilova SA. High interleukin-18 and low FOXP3 mRNAs in peripheral blood of women with severe systemic lupus erythematosus: a cross-sectional study. Rheumatol Int (2020) 40(5):727–35. doi: 10.1007/s00296-020-04542-3
45. Behzadi M, Ahmadzadeh A, Valizadeh M, Haji Molla Hoseini M, Yeganeh F. CD13/aminopeptidase n mRNA expression and enzyme activity in systemic lupus erythematosus. Acta Reumatol Port (2017) 42(2):162–7.
46. Gao H, Ma XX, Guo Q, Zou YD, Zhong YC, Xie LF, et al. Expression and clinical significance of semaphorin 3A in serum and mononuclear cells in patients with systemic lupus erythematosus. Zhonghua Yi Xue Za Zhi (2017) 97(5):370–4. doi: 10.3760/cma.j.issn.0376-2491.2017.05.010
47. Mohamed AE, Zaki HM, Hosny MM, Fouad MM, Moneim NHA. Association of interleukin-36α gene expression in Egyptian patients with systemic lupus erythematosus with organ involvement and disease activity. Egypt J Immunol (2021) 28(2):75–84. doi: 10.55133/eji.280208
48. Mai SZ, Li CJ, Xie XY, Xiong H, Xu M, Zeng FQ, et al. Increased serum IL-36α and IL-36γ levels in patients with systemic lupus erythematosus: Association with disease activity and arthritis. Int Immunopharmacol (2018) 58:103–8. doi: 10.1016/j.intimp.2018.03.011
49. Huang Q, Shen S, Qu H, Huang Y, Wu D, Jiang H, et al. Expression of HMGB1 and TLR4 in neuropsychiatric systemic lupus erythematosus patients with seizure disorders. Ann Transl Med (2020) 8(1):9. doi: 10.21037/atm.2019.12.44
50. Latini A, Novelli L, Ceccarelli F, Barbati C, Perricone C, De Benedittis G, et al. mRNA expression analysis confirms CD44 splicing impairment in systemic lupus erythematosus patients. Lupus (2021) 30(7):1086–93. doi: 10.1177/09612033211004725
51. Luo Q, Fu B, Zhang L, Guo Y, Huang Z, Li J. Decreased peripheral blood ALKBH5 correlates with markers of autoimmune response in systemic lupus erythematosus. Dis Markers (2020) 2020:8193895. doi: 10.1155/2020/8193895
52. Luo Q, Rao J, Zhang L, Fu B, Guo Y, Huang Z, et al. The study of METTL14, ALKBH5, and YTHDF2 in peripheral blood mononuclear cells from systemic lupus erythematosus. Mol Genet Genomic Med (2020) 8(9):e1298. doi: 10.1002/mgg3.1298
53. Uzrail AH, Assaf AM, Abdalla SS. Correlations of expression levels of a panel of genes (IRF5, STAT4, TNFSF4, MECP2, and TLR7) and cytokine levels (IL-2, IL-6, IL-10, IL-12, IFN-γ, and TNF-α) with systemic lupus erythematosus outcomes in Jordanian patients. BioMed Res Int (2019) 2019:1703842. doi: 10.1155/2019/1703842
54. Tian J, Li X, Jiang Y, Gao F, Ju B, Chen J, et al. SLC7A5 expression is up-regulated in peripheral blood T and b lymphocytes of systemic lupus erythematosus patients, associating with renal damage. Clin Immunol (2022) 237:108987. doi: 10.1016/j.clim.2022.108987
55. Wang X, Zhao C, Zhang C, Mei X, Song J, Sun Y, et al. Increased HERV-e clone 4-1 expression contributes to DNA hypomethylation and IL-17 release from CD4(+) T cells via miR-302d/MBD2 in systemic lupus erythematosus. Cell Commun Signal (2019) 17(1):94. doi: 10.1186/s12964-019-0416-5
56. Miyagawa-Hayashino A, Yoshifuji H, Kitagori K, Ito S, Oku T, Hirayama Y, et al. Increase of MZB1 in b cells in systemic lupus erythematosus: proteomic analysis of biopsied lymph nodes. Arthritis Res Ther (2018) 20(1):13. doi: 10.1186/s13075-018-1511-5
57. Guo G, Wang H, Shi X, Ye L, Wu K, Lin K, et al. NovelmiRNA-25 inhibits AMPD2 in peripheral blood mononuclear cells of patients with systemic lupus erythematosus and represents a promising novel biomarker. J Transl Med (2018) 16(1):370. doi: 10.1186/s12967-018-1739-5
58. Yan L, Jiang L, Wang B, Hu Q, Deng S, Huang J, et al. Novel microRNA biomarkers of systemic lupus erythematosus in plasma: miR-124-3p and miR-377-3p. Clin Biochem (2022) 107:55–61. doi: 10.1016/j.clinbiochem.2022.05.004
59. Wang X, Zhang C, Wu Z, Chen Y, Shi W. CircIBTK inhibits DNA demethylation and activation of AKT signaling pathway via miR-29b in peripheral blood mononuclear cells in systemic lupus erythematosus. Arthritis Res Ther (2018) 20(1):118. doi: 10.1186/s13075-018-1618-8
60. Nakhjavani M, Etemadi J, Pourlak T, Mirhosaini Z, Zununi Vahed S, Abediazar S. Plasma levels of miR-21, miR-150, miR-423 in patients with lupus nephritis. Iran J Kidney Dis (2019) 13(3):198–206.
61. Khoshmirsafa M, Kianmehr N, Falak R, Mowla SJ, Seif F, Mirzaei B, et al. Elevated expression of miR-21 and miR-155 in peripheral blood mononuclear cells as potential biomarkers for lupus nephritis. Int J Rheum Dis (2019) 22(3):458–67. doi: 10.1111/1756-185X.13410
62. Ahmed RF, Shaker OG, Abdelghany HM, Helmy Abdallah N, Elsayed SH, Kamel BA. Role of micro-RNA132 and its long non coding SOX2 in diagnosis of lupus nephritis. Lupus (2022) 31(1):89–96. doi: 10.1177/09612033211067166
63. Navarro-Quiroz E, Pacheco-Lugo L, Navarro-Quiroz R, Lorenzi H, España-Puccini P, Díaz-Olmos Y, et al. Profiling analysis of circulating microRNA in peripheral blood of patients with class IV lupus nephritis. PloS One (2017) 12(11):e0187973. doi: 10.1371/journal.pone.0187973
64. Yang M, Yang B, Deng D. Targeting of EIF4EBP1 by miR-99a-3p affects the functions of b lymphocytes via autophagy and aggravates SLE disease progression. J Cell Mol Med (2021) 25(21):10291–305. doi: 10.1111/jcmm.16991
65. Zeng L, Wu JL, Liu LM, Jiang JQ, Wu HJ, Zhao M, et al. Serum miRNA-371b-5p and miRNA-5100 act as biomarkers for systemic lupus erythematosus. Clin Immunol (2018) 196:103–9. doi: 10.1016/j.clim.2018.10.004
66. Shumnalieva R, Kachakova D, Shoumnalieva-Ivanova V, Miteva P, Kaneva R, Monov S. Whole peripheral blood miR-146a and miR-155 expression levels in systemic lupus erythematosus patients. Acta Reumatol Port (2018) 43(3):217–25.
67. Zhou S, Zhang J, Luan P, Ma Z, Dang J, Zhu H, et al. miR-183-5p is a potential molecular marker of systemic lupus erythematosus. J Immunol Res (2021) 2021:5547635. doi: 10.1155/2021/5547635
68. Jafari Ghods F, Topal Sarikaya A, Arda N, Hamuryudan V. MiRNA and mRNA profiling in systemic lupus reveals a novel set of cytokine - related miRNAs and their target genes in cases with and without renal involvement. Kidney Blood Press Res (2017) 42(6):1322–37. doi: 10.1159/000485987
69. Abdul-Maksoud RS, Rashad NM, Elsayed WSH, Ali MA, Kamal NM, Zidan HE. Circulating miR-181a and miR-223 expression with the potential value of biomarkers for the diagnosis of systemic lupus erythematosus and predicting lupus nephritis. J Gene Med (2021) 23(5):e3326. doi: 10.1002/jgm.3326
70. Wu Q, Qin Y, Shi M, Yan L. Diagnostic significance of circulating miR-485-5p in patients with lupus nephritis and its predictive value evaluation for the clinical outcomes. J Chin Med Assoc (2021) 84(5):491–7. doi: 10.1097/JCMA.0000000000000522
71. Zhang L, Zhang X. Downregulated miR-203 attenuates IL-β, IL-6, and TNF-α activation in TRAF6-treated human renal mesangial and tubular epithelial cells. Int J Clin Exp Pathol (2020) 13(2):324–31.
72. Zhang H, Huang X, Ye L, Guo G, Li X, Chen C, et al. B cell-related circulating MicroRNAs with the potential value of biomarkers in the differential diagnosis, and distinguishment between the disease activity and lupus nephritis for systemic lupus erythematosus. Front Immunol (2018) 9:1473. doi: 10.3389/fimmu.2018.01473
73. Lin LJ, Mai LJ, Chen G, Zhao EN, Xue M, Su XD. Expression and diagnostic value of plasma miR-145 and miR-183 in children with lupus nephritis. Zhongguo Dang Dai Er Ke Za Zhi (2020) 22(6):632–7. doi: 10.7499/j.issn.1008-8830.2001013
74. Zununi Vahed S, Nakhjavani M, Etemadi J, Jamshidi H, Jadidian N, Pourlak T, et al. Altered levels of immune-regulatory microRNAs in plasma samples of patients with lupus nephritis. Bioimpacts (2018) 8(3):177–83. doi: 10.15171/bi.2018.20
75. Jin L, Fang X, Dai C, Xiang N, Tao J, Sun X, et al. The potential role of ets-1 and miR-326 in CD19(+)B cells in the pathogenesis of patients with systemic lupus erythematosus. Clin Rheumatol (2019) 38(4):1031–8. doi: 10.1007/s10067-018-4371-0
76. Chen S, Wang Y, Qin H, Lin J, Xie L, Chen S, et al. Downregulation of miR-633 activated AKT/mTOR pathway by targeting AKT1 in lupus CD4(+) T cells. Lupus (2019) 28(4):510–9. doi: 10.1177/0961203319829853
77. Li W, Liu S, Chen Y, Weng R, Zhang K, He X, et al. Circulating exosomal microRNAs as biomarkers of systemic lupus erythematosus. Clinics (Sao Paulo) (2020) 75:e1528. doi: 10.6061/clinics/2020/e1528
78. Perez-Hernandez J, Martinez-Arroyo O, Ortega A, Galera M, Solis-Salguero MA, Chaves FJ, et al. Urinary exosomal miR-146a as a marker of albuminuria, activity changes and disease flares in lupus nephritis. J Nephrol (2021) 34(4):1157–67. doi: 10.1007/s40620-020-00832-y
79. Garcia-Vives E, Solé C, Moliné T, Vidal M, Agraz I, Ordi-Ros J, et al. The urinary exosomal miRNA expression profile is predictive of clinical response in lupus nephritis. Int J Mol Sci (2020) 21(4):1372. doi: 10.3390/ijms21041372
80. Tangtanatakul P, Klinchanhom S, Sodsai P, Sutichet T, Promjeen C, Avihingsanon Y, et al. Down-regulation of let-7a and miR-21 in urine exosomes from lupus nephritis patients during disease flare. Asian Pac J Allergy Immunol (2019) 37(4):189–97. doi: 10.12932/AP-130318-0280
81. Solé C, Moliné T, Vidal M, Ordi-Ros J, Cortés-Hernández J. An exosomal urinary miRNA signature for early diagnosis of renal fibrosis in lupus nephritis. Cells (2019) 8(8):773. doi: 10.3390/cells8080773
82. Cardenas-Gonzalez M, Srivastava A, Pavkovic M, Bijol V, Rennke HG, Stillman IE, et al. Identification, confirmation, and replication of novel urinary MicroRNA biomarkers in lupus nephritis and diabetic nephropathy. Clin Chem (2017) 63(9):1515–26. doi: 10.1373/clinchem.2017.274175
83. Zou Y, Xu H. Involvement of long noncoding RNAs in the pathogenesis of autoimmune diseases. J Transl Autoimmun (2020) 3:100044. doi: 10.1016/j.jtauto.2020.100044
84. Cheng Q, Chen M, Chen X, Chen X, Jiang H, Wu H, et al. Novel long non-coding RNA expression profile of peripheral blood mononuclear cells reveals potential biomarkers and regulatory mechanisms in systemic lupus erythematosus. Front Cell Dev Biol (2021) 9:639321. doi: 10.3389/fcell.2021.639321
85. You Y, Zhao X, Wu Y, Mao J, Ge L, Guo J, et al. Integrated transcriptome profiling revealed that elevated long non-coding RNA-AC007278.2 expression repressed CCR7 transcription in systemic lupus erythematosus. Front Immunol (2021) 12:615859. doi: 10.3389/fimmu.2021.615859
86. Wu GC, Li J, Leng RX, Li XP, Li XM, Wang DG, et al. Identification of long non-coding RNAs GAS5, linc0597 and lnc-DC in plasma as novel biomarkers for systemic lupus erythematosus. Oncotarget (2017) 8(14):23650–63. doi: 10.18632/oncotarget.15569
87. Wu GC, Hu Y, Guan SY, Ye DQ, Pan HF. Differential plasma expression profiles of long non-coding RNAs reveal potential biomarkers for systemic lupus erythematosus. Biomolecules (2019) 9(6):206. doi: 10.3390/biom9060206
88. Ali MA, Shaker OG, Khalefa AA, Abdelwahed MY, Ali E, Ezzat EM, et al. Serum long noncoding RNAs FAS-AS1 & PVT1 are novel biomarkers for systemic lupus erythematous. Br J BioMed Sci (2020) 77(4):208–12. doi: 10.1080/09674845.2020.1765459
89. Abd-Elmawla MA, Fawzy MW, Rizk SM, Shaheen AA. Role of long non-coding RNAs expression (ANRIL, NOS3-AS, and APOA1-AS) in development of atherosclerosis in Egyptian systemic lupus erythematosus patients. Clin Rheumatol (2018) 37(12):3319–28. doi: 10.1007/s10067-018-4269-x
90. Guan X, Liu D, Xing Y, Guan X. Study on the relationship between lncRNA gene polymorphism and systemic lupus erythematosus. Comput Math Methods Med (2022) 2022:4446016. doi: 10.1155/2022/4446016
91. Chen X, Luo X, Wei Y, Sun H, Dai L, Tangzhou Y, et al. LncRNA H19 induces immune dysregulation of BMMSCs, at least partly, by inhibiting IL-2 production. Mol Med (2021) 27(1):61. doi: 10.1186/s10020-021-00326-y
92. Cai B, Cai J, Yin Z, Jiang X, Yao C, Ma J, et al. Long non-coding RNA expression profiles in neutrophils revealed potential biomarker for prediction of renal involvement in SLE patients. Rheumatol (Oxford) (2021) 60(4):1734–46. doi: 10.1093/rheumatology/keaa575
93. Suo QF, Sheng J, Qiang FY, Tang ZS, Yang YY. Association of long non-coding RNA GAS5 and miR-21 levels in CD4(+) T cells with clinical features of systemic lupus erythematosus. Exp Ther Med (2018) 15(1):345–50. doi: 10.3892/etm.2017.5429
94. Wang J, Wu X, Tu Y, Dang J, Cai Z, Liao W, et al. An integrated analysis of lncRNA and mRNA expression profiles in the kidneys of mice with lupus nephritis. PeerJ (2021) 9:e10668. doi: 10.7717/peerj.10668
95. Guo G, Wang H, Ye L, Shi X, Yan K, Lin K, et al. Hsa_circ_0000479 as a novel diagnostic biomarker of systemic lupus erythematosus. Front Immunol (2019) 10:2281. doi: 10.3389/fimmu.2019.02281
96. Miao Q, Zhong Z, Jiang Z, Lin Y, Ni B, Yang W, et al. RNA-Seq of circular RNAs identified circPTPN22 as a potential new activity indicator in systemic lupus erythematosus. Lupus (2019) 28(4):520–8. doi: 10.1177/0961203319830493
97. Zheng F, Yu X, Tang D, Hong X, Zhang X, Liu D, et al. The identification of circular RNAs from peripheral blood mononuclear cells in systemic lupus erythematosus. BMC Med Genomics (2021) 14(1):70. doi: 10.1186/s12920-021-00919-w
98. Luo Q, Zhang L, Xiong L, Fu B, Guo Y, Huang Z, et al. Peripheral blood circular RNA hsa_circ_0082688-hsa_circ_0008675 can be used as a candidate biomarker of systemic lupus erythematosus with renal involvement. Clin Exp Rheumatol (2020) 38(5):822–33.
99. Luo Q, Zhang L, Fang L, Fu B, Guo Y, Huang Z, et al. Circular RNAs hsa_circ_0000479 in peripheral blood mononuclear cells as novel biomarkers for systemic lupus erythematosus. Autoimmunity (2020) 53(3):167–76. doi: 10.1080/08916934.2020.1728529
100. Huang Z, Zhang L, Zeng L, Luo Q. Expression and significance of circular RNA HSA_cirC_104871 in peripheral blood mononuclear cells of patients with systemic lupus erythematosus. J Anhui Med University (2019) 54(12):1947–51. doi: 10.19405/j.cnki.issn1000-1492.2019.12.022
101. Wang X, Zhang C, Wu Z, Chen Y, Shi W. CircIBTK inhibits DNA demethylation and activation of AKT signaling pathway via miR-29b in peripheral blood mononuclear cells in systemic lupus erythematosus. Arthritis Res Ther (2018) 20(1):118. doi: 10.1186/s13075-018-1618-8
102. Zhang C, Wang X, Chen Y, Wu Z, Zhang C, Shi W. The down-regulation of hsa_circ_0012919, the sponge for miR-125a-3p, contributes to DNA methylation of CD11a and CD70 in CD4(+) T cells of systemic lupus erythematous. Clin Sci (Lond) (2018) 132(21):2285–98. doi: 10.1042/CS20180403
103. Mei HY, Liu J, Shen XP, Wu R. A novel circRNA, circRACGAP1, hampers the progression of systemic lupus erythematosus via miR-22-3p-mediated AKT signalling. Autoimmunity (2022) 55(6):360–70. doi: 10.1080/08916934.2022.2073590
104. Zhao X, Dong R, Zhang L, Guo J, Shi Y, Ge L, et al. N6-methyladenosine-dependent modification of circGARS acts as a new player that promotes SLE progression through the NF-κB/A20 axis. Arthritis Res Ther (2022) 24(1):37. doi: 10.1186/s13075-022-02732-x
105. Luo Q, Huang Z, Zhang L, Li J. Expression and significance of circrNA Hsa_circ_0002715 in whole blood of patients with systemic lupus erythematosus. J Anhui Med University (2020) 55(7):1130–6. doi: 10.19405/j.cnki.issn1000-1492.2020.07.029
106. Luo Q, Huang Z, Zhang L, Li J. Expression and clinical significance of circrnas Hsa_circ_0004156 and HSA_circ_0082626 in whole blood of patients with systemic lupus erythematosus. Chin J Immunol (2020) 36(20):2500–5-10.
107. Ouyang Q, Huang Q, Jiang Z, Zhao J, Shi GP, Yang M. Using plasma circRNA_002453 as a novel biomarker in the diagnosis of lupus nephritis. Mol Immunol (2018) 101:531–8. doi: 10.1016/j.molimm.2018.07.029
108. Zhang MY, Wang JB, Zhu ZW, Li LJ, Liu RS, Yang XK, et al. Differentially expressed circular RNAs in systemic lupus erythematosus and their clinical significance. BioMed Pharmacother (2018) 107:1720–7. doi: 10.1016/j.biopha.2018.08.161
109. He S, Du H, Wang Y, Shi X, Zhou Y. Hsa_circ_0010957 level is increased and sponges microRNA-125b in CD4(+) T cells of patients with systemic lupus erythematosus. Mol Med Rep (2021) 23(6):469. doi: 10.3892/mmr.2021.12108
110. Liu B, Cao J, Wang X, Guo C, Liu Y, Wang T. Deciphering the tRNA-derived small RNAs: origin, development, and future. Cell Death Dis (2021) 13(1):24. doi: 10.1038/s41419-021-04472-3
111. Liang Y, Zhang J, Qiu W, Chen B, Zhou Y, Chen X, et al. Dysregulation of tRNA-derived small RNAs and their potential roles in lupus nephritis. Lupus (2021) 30(14):2248–55. doi: 10.1177/09612033211061482
112. Xu H, Chen W, Zheng F, Tang D, Dai W, Huang S, et al. The potential role of tRNAs and small RNAs derived from tRNAs in the occurrence and development of systemic lupus erythematosus. Biochem Biophys Res Commun (2020) 527(2):561–7. doi: 10.1016/j.bbrc.2020.04.114
113. Geng G, Wang H, Xin W, Liu Z, Chen J, Danting Z, et al. tRNA derived fragment (tRF)-3009 participates in modulation of IFN-α-induced CD4(+) T cell oxidative phosphorylation in lupus patients. J Transl Med (2021) 19(1):305. doi: 10.1186/s12967-021-02967-3
114. Yang P, Zhang X, Chen S, Tao Y, Ning M, Zhu Y, et al. A novel serum tsRNA for diagnosis and prediction of nephritis in SLE. Front Immunol (2021) 12:735105. doi: 10.3389/fimmu.2021.735105
115. Lai NS, Yu HC, Huang KY, Tung CH, Huang HB, Lu MC. Decreased T cell expression of H/ACA box small nucleolar RNA 12 promotes lupus pathogenesis in patients with systemic lupus erythematosus. Lupus (2018) 27(9):1499–508. doi: 10.1177/0961203318778362
116. Wajahat M, Bracken CP, Orang A. Emerging functions for snoRNAs and snoRNA-derived fragments. Int J Mol Sci (2021) 22(19):10193. doi: 10.3390/ijms221910193
117. Ender C, Krek A, Friedländer MR, Beitzinger M, Weinmann L, Chen W, et al. A human snoRNA with microRNA-like functions. Mol Cell (2008) 32(4):519–28. doi: 10.1016/j.molcel.2008.10.017
118. Zhong F, Zhou N, Wu K, Guo Y, Tan W, Zhang H, et al. A SnoRNA-derived piRNA interacts with human interleukin-4 pre-mRNA and induces its decay in nuclear exosomes. Nucleic Acids Res (2015) 43(21):10474–91. doi: 10.1093/nar/gkv954
119. Borchers AT, Keen CL, Shoenfeld Y, Gershwin ME. Surviving the butterfly and the wolf: mortality trends in systemic lupus erythematosus. Autoimmun Rev (2004) 3(6):423–53. doi: 10.1016/j.autrev.2004.04.002
120. Singh RR, Yen EY. SLE mortality remains disproportionately high, despite improvements over the last decade. Lupus (2018) 27(10):1577–81. doi: 10.1177/0961203318786436
121. Yen EY, Singh RR. Brief report: Lupus-an unrecognized leading cause of death in young females: A population-based study using nationwide death certificates, 2000-2015. Arthritis Rheumatol (2018) 70(8):1251–5. doi: 10.1002/art.40512
122. Ruperto N, Hanrahan LM, Alarcón GS, Belmont HM, Brey RL, Brunetta P, et al. International consensus for a definition of disease flare in lupus. Lupus (2011) 20(5):453–62. doi: 10.1177/0961203310388445
123. Gordon C, Sutcliffe N, Skan J, Stoll T, Isenberg DA. Definition and treatment of lupus flares measured by the BILAG index. Rheumatol (Oxford) (2003) 42(11):1372–9. doi: 10.1093/rheumatology/keg382
Keywords: systemic lupus erythematosus, biomarker, mRNA, microRNA, lncRNA, snoRNA, circRNA, diagnosis
Citation: Liang J, Xie F, Feng J, Huang C, Shen J, Han Z, Luo W, He J and Chen H (2022) Progress in the application of body fluid and tissue level mRNAs-non-coding RNAs for the early diagnosis and prognostic evaluation of systemic lupus erythematosus. Front. Immunol. 13:1020891. doi: 10.3389/fimmu.2022.1020891
Received: 16 August 2022; Accepted: 29 September 2022;
Published: 17 October 2022.
Edited by:
Huji Xu, Tsinghua University, ChinaReviewed by:
Hsien-bin Huang, National Chung Cheng University, TaiwanCopyright © 2022 Liang, Xie, Feng, Huang, Shen, Han, Luo, He and Chen. This is an open-access article distributed under the terms of the Creative Commons Attribution License (CC BY). The use, distribution or reproduction in other forums is permitted, provided the original author(s) and the copyright owner(s) are credited and that the original publication in this journal is cited, in accordance with accepted academic practice. No use, distribution or reproduction is permitted which does not comply with these terms.
*Correspondence: Hanwei Chen, ZG9jdGVyd2VpQHNpbmEuY29t; Jinhua He, MzMyNTE4NTc5QHFxLmNvbQ==
†These authors have contributed equally to this work and share first authorship
Disclaimer: All claims expressed in this article are solely those of the authors and do not necessarily represent those of their affiliated organizations, or those of the publisher, the editors and the reviewers. Any product that may be evaluated in this article or claim that may be made by its manufacturer is not guaranteed or endorsed by the publisher.
Research integrity at Frontiers
Learn more about the work of our research integrity team to safeguard the quality of each article we publish.